- 1School of Fine Arts, Nanjing Normal University, Nanjing, China
- 2College of Art and Design, Nanjing Forestry University, Nanjing, China
Introduction: Urban green spaces play a crucial role in mitigating climate change by sequestering atmospheric carbon dioxide. This study aimed to evaluate the carbon sequestration potential of common plant species in urban residential areas and provide recommendations for optimizing green space design and management.
Methods: The research was conducted in four residential areas of Nanjing, China, where key growth parameters of 20 plant species, including evergreen trees, deciduous trees, evergreen shrubs, and deciduous shrubs, were measured. The assimilation method was employed to calculate carbon sequestration per unit canopy area and for entire plants.
Results: The results showed that the carbon sequestration capacities of different plant species and types exhibited significant differences, with p-values less than 0.05. In terms of daily carbon sequestration per unit canopy projection area, the ranking was as follows: evergreen trees > evergreen shrubs > deciduous trees > deciduous shrubs. For total plant carbon sequestration, the ranking was: evergreen trees > deciduous trees > evergreen shrubs > deciduous shrubs. Evergreen trees performed excellently in both carbon sequestration metrics, with the average daily carbon sequestration per unit canopy projection area and for the entire plant being 18.0024 g/(m2·d) and 462.28 g/d, respectively. The study also observed seasonal variations, with carbon sequestration rates being higher in autumn and summer compared to spring and winter. During the summer, the average daily carbon sequestration per unit canopy projection area and for the entire plant were 11.975 g/(m2·d) and 161.744 g/d, respectively, while in autumn, these values were 13.886 g/(m2·d) and 98.458 g/d. Seasonal variations were also observed, with autumn and summer exhibiting higher carbon sequestration rates compared to spring and winter. Additionally, CO2 concentrations were monitored across the four residential areas, providing insights into the spatial and temporal dynamics of carbon sequestration.
Discussion: Based on the findings, optimization strategies were proposed, such as prioritizing the selection and integration of high-performing evergreen tree species in urban green space design and incorporating diverse plant types to enhance year-round carbon sequestration. This study contributes to the development of sustainable urban planning and landscape management practices, promoting the role of green spaces in mitigating climate change and enhancing urban resilience.
1 Introduction
The global climate change has emerged as the most pressing environmental challenge confronting human development, with anthropogenic activities resulting in greenhouse gas emissions recognized as the primary driver of global warming since the mid-20th century (Jiang et al., 2023; Tao et al., 2023; Zhang et al., 2023; Moody et al., 2021). Urban areas not only serve as focal points for fossil fuel consumption and greenhouse gas emissions but also experience significant impacts of climate change on human life. Amidst the persistent threat of climate change, reducing urban carbon dioxide emissions and enhancing carbon sequestration capabilities are identified as crucial strategies to combat climate change and alleviate its effects on cities (Yu et al., 2024a; Wang et al., 2013).
China, being one of the largest carbon dioxide emitters globally, announced in September 2020 its commitment to peak carbon dioxide emissions by 2030 and achieve carbon neutrality by 2060, aiming to advance the adoption of sustainable development practices (Zhang et al., 2024). Urban green spaces, being the sole ecological spaces in cities closely linked to nature, assume a vital role in upholding carbon equilibrium, fostering human health, and offering ecosystem services (Leppänen et al., 2024; Yuan et al., 2023; Dong et al., 2023). Notably, green spaces within residential areas form the central element of urban green space networks. By augmenting the quantity and enhancing the quality of carbon sinks, it becomes possible not only to sustain the carbon-oxygen balance in cities, enhance the environment, and regulate urban microclimates but also to effectively alleviate carbon emission pressures and optimize ecological advantages (Wang et al., 2014; Lindén et al., 2020). Therefore, gaining a comprehensive understanding of current carbon sink measurement techniques, analyzing future development trajectories, and visualizing carbon management are key catalysts in attaining the “dual carbon” objectives.
The calculation methods for estimating carbon sequestration capacity in urban residential areas generally involve field-based data collection, statistical methods, remote sensing technology, GIS, and ecosystem modeling. Field-based data collection and statistical methods entail measuring vegetation biomass in green spaces like trees, shrubs, and grass, and estimating their carbon absorption rates. Common measurement indicators used include tree diameter at breast height, height, canopy width, etc., combined with plant growth models for estimating carbon sequestration. Tadesse et al. employed a systematic sampling design, establishing 30 sample plots along seven transects to assess woody plants, collect soil samples, and measure the biomass of dead branches and fallen leaves. The biomass carbon stock was subsequently estimated using an allometric growth equation (Tadesse and Tamiru, 2024). Bulusu et al. conducted a comprehensive literature review, summarizing research on above-ground carbon (AGC) and soil organic carbon (SOC) storage in Miombo woodlands from 1960 to 2018, with a focus on carbon storage variations across different forest types (Bulusu et al., 2021). Liu et al. utilized forest survey data and literature review to compile a biomass dataset for mature forests in China, analyzing the spatial distribution of carbon density and employing interpolation techniques to estimate the carbon carrying capacity of Chinese forests (Liu et al., 2014). Liu et al. taking Shenyang as a case study, evaluated the carbon storage and sequestration potential of urban forests through field surveys and high-resolution imagery, calculating their monetary value and exploring their contribution to offsetting carbon emissions. However, the statistical methods employed in field surveys are susceptible to human error and limited sampling scope, which may lead to deviations from actual conditions (Liu and Li, 2012). Additionally, these methods are unsuitable for large-scale regional assessments, as they cannot fully capture the spatial distribution of carbon sink capacity across a given area. Large-scale field surveys also require considerable human and financial resources and are often inefficient, posing challenges in meeting the demands of extensive carbon sink evaluations.
Remote sensing technology and geographic information systems (GIS) are instrumental in mapping urban green space distribution and vegetation cover through satellite imagery and GIS tools. By analyzing remote sensing data alongside vegetation indices, such as the Normalized Difference Vegetation Index (NDVI), researchers can assess the health and growth of vegetation, providing an indirect estimate of the carbon sequestration capacity of green spaces (Huang et al., 2023; Yu et al., 2019). Pascual et al. integrated airborne laser scanning data with multi-source satellite imagery to estimate the above-ground carbon density and productivity of Hawaiian forests. This approach led to the creation of forest cover maps and the development of a carbon sequestration potential index, which identifies regions with the highest afforestation potential (Pascual et al., 2021). Torre-Tojal et al. (2022) leveraged forest resource survey data, allometric growth equations, and LiDAR data, employing a random forest model to estimate the biomass of radiata pine in the Basque Country. The model was optimized through hyperparameter tuning and cross-validation, resulting in an R2 value greater than 0.7. Niu et al. introduced the CEVSA-ES model, which combines remote sensing leaf area index data to evaluate ecosystem services across China’s four major ecosystems (Niu et al., 2021). This model innovatively incorporates the effects of soil erosion on the carbon cycle and refines the carbon-water cycle algorithm. Despite the advancements in remote sensing and GIS technologies, model inversion techniques are influenced by input parameters, spectral resolution, and sensor accuracy, which can lead to substantial errors. These technologies face challenges in distinguishing complex vegetation structures and differentiating between various carbon pools, such as soil and dead organic matter. Even with improved resolution, accurately assessing the carbon sequestration potential of individual vegetation units in complex urban environments remains difficult. Additionally, the high costs associated with equipment and the acquisition of image data, particularly from commercial satellite sources, pose significant barriers to the widespread application of these technologies.
The ecosystem modeling approach employs various ecosystem process models, such as InVEST, i-Tree, and the assimilation method, to simulate the carbon cycle processes within urban green spaces. This approach integrates carbon inputs, such as photosynthesis, with carbon outputs, including respiration and decomposition, to comprehensively estimate the dynamic changes in carbon sequestration across these green spaces (Yu et al., 2024b; Alberdi et al., 2020; Sun and Liu, 2020). The InVEST model evaluates the carbon sequestration capacity of green spaces by simulating ecosystem processes and incorporating spatial data alongside ecological models. It further analyzes the impact of land use changes on carbon sequestration, thereby classifying it as an ecosystem modeling technique, and integrates GIS and remote sensing data. He et al. combined the LUSD (Land Use and Spatial Development) urban model with the InVEST model to assess the effects of urban expansion on regional carbon storage, simulating and forecasting urban growth patterns (He et al., 2016). Kaur et al. applied the InVEST model to carbon sequestration quantification for the years 2000 and 2020, aggregating biophysical data. i-Tree, a widely used model, assesses the ecosystem services provided by urban trees and forests, estimating carbon storage, air purification, and stormwater interception capabilities based on field data (Kaur et al., 2022). Ismaili Alaoui et al. integrated the i-Tree model with drone-based modeling and field surveys to estimate carbon storage in urban parks, reporting a carbon storage of 15.3 tons per hectare, with an average carbon storage of 8.6 tons per planted area (Ismaili Alaoui et al., 2023). Liu et al. introduced the SVGD-AE method, which combines Stein Variational Gradient Descent (SVGD) with Autoencoder (AE) neural networks in a geostatistical inversion framework to estimate geological carbon sequestration, thereby highlighting the application of advanced modeling techniques in quantifying carbon sequestration (Liu et al., 2024). The ecosystem modeling approach integrates remote sensing data with field observation data, enabling analyses across regional to global scales and effectively addressing data gaps. The model’s grid resolution is flexible and adjustable, catering to the diverse needs of various research endeavors. Once the model is established, its operational costs are relatively low, making it especially suitable for long-term simulations and large-scale studies. The method employed in this study, known as the equivalence quantification method, is a type of ecosystem model that simulates carbon storage and sequestration capacity using field data and input variables such as climate and vegetation. It incorporates key processes such as photosynthesis, respiration, and soil carbon turnover, facilitating large-scale spatial analysis and trend forecasting, while maintaining both cost-effectiveness and applicability.
This study aims to assess the carbon sequestration capacity of different vegetation types in residential green spaces in Nanjing, along with the factors influencing this capacity, to provide scientific guidance for optimizing the ecological functions of urban green spaces. The research hypothesis posits that vegetation types—specifically evergreen trees, deciduous trees, evergreen shrubs, and deciduous shrubs—along with key growth parameters such as diameter at breast height, crown spread, and plant height, significantly affect carbon sequestration capacity. Additionally, seasonal variations are expected to play a critical role in modulating carbon sequestration.
2 Materials and methods
2.1 Experimental area
Nanjing is located in the central region of the Yangtze River Delta in eastern China, serving as a key gateway city in the Yangtze River Delta. Its geographical coordinates range from 31°14′ to 32°37′ north latitude and 118°22′ to 119°14′ east longitude. The total area is 6,587.04 km2, with an urban built-up area of 868.28 km2 as of 2020. Nanjing has a subtropical humid climate with distinct seasons and abundant rainfall. The forest coverage rate is 31.3%, and the urban greening coverage rate is 45.16%, ranking it among the top three in China. It is one of China’s four major garden cities and is known as the “Green Capital.”
To investigate the differences in carbon sequestration capacity across various plant types, this study selects four representative residential areas in Nanjing, highlighted with red pentagrams in Figure 1. These areas—Tanqiao Apartment, Luotuolula Town, Yinxiang Xinyu, and Yihe South Park—are all situated within the subtropical monsoon climate zone, characterized by warm, humid weather and distinct seasons. The regions experience abundant annual rainfall and ample sunlight, particularly in the spring and summer, providing favorable conditions for plant growth. The soil in these areas is diverse, fertile, and well-drained, promoting healthy root development. Due to the varying functions and designs of the residential communities, the plant species in each area differ notably. Specifically, Yihe South Park is predominantly planted with evergreen trees, Luotuolula Town features mainly deciduous trees, Tanqiao Apartment is primarily planted with evergreen shrubs, and Yinxiang Xinyu mostly contains deciduous shrubs.
The Yihe South Park (
2.2 Experimental method
This study is based on a comprehensive survey of plant types in the residential green spaces of four communities, systematically documenting the distribution of plant species within each sample plot. To investigate the carbon sequestration capacity of these plants, we selected the 20 most commonly planted species for detailed analysis (Rasoolzadeh et al., 2024). Growth parameters, including diameter at breast height, crown spread, and plant height, were measured using a tape measure, and these key growth characteristics were recorded for each species. Using these parameters, along with a plant taxonomy reference, the plants were classified into categories of evergreen trees, deciduous trees, evergreen shrubs, and deciduous shrubs, as summarized in the Table 1.
Based on the principles of plant photosynthesis, the daily carbon fixation of plants was calculated using the daily variation in the net photosynthetic rate, measured with a Li-6400XT portable photosynthesis system produced by Li-COR Biosciences. The seasonal divisions were based on the Spring Equinox, Summer Solstice, Autumn Equinox, and Winter Solstice. For each season, three clear, windless, and cloudless days were selected: March 24–30, 2023 (Spring), June 23–30, 2023 (Summer), September 22–30, 2023 (Autumn), and December 20–30, 2023 (Winter). During these days, measurements were taken under natural sunlight from 8:00 a.m. to 6:00 p.m., at 2-h intervals. The measurement sequence for the plants was kept consistent throughout the day. Three healthy, pest-free plants were selected, with five mature, sun-exposed leaves of similar size chosen from each plant. At each time point, five instantaneous net photosynthetic rate readings were recorded per leaf, and the average value was calculated. A total of 1,350 measurements were recorded for each tree in every season. The leaf area (LAI) was measured using optical instruments and image analysis. Canopy images were captured with a fisheye lens and digital camera, and processed with Hemiview canopy analysis software to calculate parameters such as solar radiation transmittance, canopy gap size, and gap fraction, ultimately determining the effective LAI (Bai and Ding, 2024; Jin et al., 2023; Nayak et al., 2022).
The Formula 1 for calculating the net assimilation rate of the current day is:
In the formula, LAI represents the leaf area index of the plant; W is the total dry weight of the plant’s leaves, kg; C is the plant’s crown area,
Select five representative healthy plants for measurement and compute the average value. Capture photographs of the plants under overcast conditions to avoid direct sunlight. The Formula 2 for calculating the net assimilation rate P on the measurement day is (Liu et al., 2013; Smith and Ramsay, 2018; Author Anonymous, 2002):
In the equation, P represents the net assimilation total of the day,
This study assumes that the carbon dioxide released by plants during nocturnal dark respiration constitutes 20% of the daytime assimilation rate, treating this proportion as a fixed value without adjustments based on empirical data. Additionally, it is assumed that plants exclusively undergo dark respiration at night, with no photosynthetic activity occurring during this period. Consequently, the calculation of daytime net photosynthesis excludes respiratory processes (Kohonen et al., 2022). Measurements were taken at the seasonal nodes of the vernal equinox, summer solstice, autumnal equinox, and winter solstice, under the assumption that environmental conditions within each season remain relatively stable, and that nocturnal respiration rates are not subject to significant fluctuations. Furthermore, the plants selected for this study were healthy, pest-free individuals, with the assumption that their respiratory metabolism was not notably influenced by disease or external stress factors. The proportion of dark respiration is treated as an empirical constant, with no further differentiation made for plant type, age, or seasonal variations in respiration intensity. The total daily assimilation rate is converted into a fixed carbon dioxide mass for each measurement day, as described in the following Formula 3 (Dou et al., 2023):
In the equation,
Using a fixed percentage (20%) to estimate carbon dioxide release during nighttime respiration offers a simplified approach to modeling but is not without limitations. Respiration rates are highly variable and influenced by factors such as plant species, age, environmental conditions, and seasonal dynamics. As a result, the fixed-percentage method often fails to accurately capture the complexity of actual respiration processes. Furthermore, plant respiration patterns exhibit significant fluctuations over the course of their growth cycles. To improve the accuracy of such estimations, direct measurements—such as those obtained using the Li-6400XT photosynthesis system—can provide more precise and reliable data, thereby minimizing errors associated with the fixed-percentage assumption. In addition, regression models derived from empirical data can establish relationships between respiration rates and environmental variables, further refining carbon sequestration estimates. While the fixed-percentage approach offers simplicity, the integration of dynamic, data-driven methods yields more accurate and scientifically robust results.
The carbon sequestration per unit of canopy projection area is a critical parameter for quantifying urban carbon sequestration. As a standardized metric, it enables the evaluation of carbon uptake efficiency across different tree species, reduces uncertainties arising from the structural complexity of vegetation, and supports both dynamic monitoring and regional-scale assessments of carbon sequestration capacity. This metric provides a scientific basis for advancing urban carbon neutrality strategies. Moreover, it facilitates the comparison and optimization of green space distribution, enhances the efficiency of urban carbon sinks, and can be updated to account for plant growth, thereby meeting the requirements for long-term monitoring and predictive modeling. Overall, this approach is of considerable value for the management and optimization of urban ecosystems. The Formula 4 for calculating the daily carbon fixation per unit canopy projection area for individual plants is as follows:
The total carbon sequestration by the entire plant is:
In the Formula 5, C represents the crown width area, in square meters, m2.
2.3 Statistical analysis
In this study, Principal Component Analysis (PCA) was first employed for dimensionality reduction and feature selection. By transforming the original variables into a set of uncorrelated principal components, PCA identifies the directions of maximum variance in the data. This approach effectively reduces dimensionality while retaining the most critical information that explains the majority of the variance. The process simplifies the data structure, eliminates redundancy among variables, and generates a robust feature set for subsequent analyses (Marteau et al., 2023).
Following PCA, Spearman’s rank correlation analysis was conducted to explore the relationships between tree species, seasonal variations, and plant carbon sequestration capacity. The Spearman correlation coefficient, ranging from −1 to +1, was calculated to measure the strength and direction of these relationships. A coefficient of 0 indicates no correlation, +1 represents a perfect positive correlation, and −1 signifies a perfect negative correlation. This analysis provides insights into how tree species and seasonal dynamics influence carbon sequestration, offering valuable information for ecological management and urban planning.
Finally, one-way Analysis of Variance (ANOVA) was applied to evaluate the statistical significance of differences in daily carbon sequestration per unit canopy projection area and total plant carbon sequestration. ANOVA determines whether the mean values of a dependent variable differ significantly across multiple groups. To further investigate pairwise group differences, post hoc analysis was conducted using the Games-Howell test, which is particularly suitable for datasets with unequal sample sizes or heterogeneous variances. The Games-Howell test also controls for multiple comparisons, enhancing the reliability of the results. A p-value less than 0.05 was considered statistically significant, indicating meaningful differences between groups. This method demonstrates strong robustness under complex data conditions, ensuring the accuracy, reliability, and scientific rigor of the findings.
3 Results
3.1 Plant carbon sequestration capacity
As summarized in the Table 2, the growth parameters of trees exhibit considerable variation. The diameter at breast height (DBH) ranges from 13.7 to 66.3 cm, canopy spread spans 2.8–6.7 m, individual tree height varies between 3.3 and 18.2 m, and the height of the lowest branches ranges from 0.88 to 5.6 m. In contrast, shrubs demonstrate significantly smaller growth dimensions. For shrubs, DBH ranges from 0.3 to 2.4 cm, canopy spread varies between 0.7 and 1.8 m, individual height spans 0.7–3.6 m, and the height of the lowest branches ranges from 0.1 to 0.5 m.
Figure 2 illustrates the carbon sequestration performance of the tested plant species, including two key metrics: carbon sequestration per unit canopy projection area and total carbon sequestration at the whole-plant level. Carbon sequestration per unit canopy projection area represents the amount of CO₂ fixed through photosynthesis within the area covered by the plant’s canopy projection. In contrast, total carbon sequestration measures the overall CO₂ absorption and fixation by the entire plant. These parameters are critical for evaluating the carbon sequestration capacity of plants and their role as carbon sinks within ecosystems.
The carbon sequestration per unit canopy projection area among the tested plants ranges from 3.2285 to 29.095 g/(m2·d). Magnolia grandiflora demonstrates the highest rate at 29.095 g/(m2·d), while L. indica cv. exhibits the lowest rate at 3.2285 g/(m2·d). Among the tested species, M. grandiflora, C. camphora, and O. fragrans rank among the top three in carbon sequestration efficiency, while Z. serrata, C. praecox, and L. indica cv. show the weakest performance. Total daily carbon sequestration values range from 11.868 to 1060.512 g/d. Cinnamomum camphora achieves the highest total sequestration rate at 1060.512 g/d, followed by M. grandiflora (830.516 g/d), S. babylonica (219.429 g/d), and P. serrulata (120.934 g/d). By contrast, L. indica cv. exhibits the lowest total carbon sequestration at just 8.92 g/d. Shrubs such as Rhododendron simsii, C. praecox, and C. speciosa display significantly lower carbon sequestration capacities compared to larger tree species such as C. camphora. Overall, trees consistently outperform shrubs in terms of carbon sequestration, with shrubs exhibiting values well below the average.
Among evergreen trees, carbon sequestration per unit canopy projection area ranges from 11.033 to 29.095 g/(m2·d). Magnolia grandiflora exhibits the highest rate, followed by C. camphora and O. fragrans, while I. crenata shows the lowest. In deciduous trees, species such as S. babylonica, Prunus × yedoensis, and C. sinensis demonstrate relatively high sequestration rates, with Z. serrata having the lowest rate at just 5.7 g/(m2·d). Among evergreen shrubs, P. serrulata has the highest carbon sequestration per unit canopy projection area at 16.155 g/(m2·d). Other evergreen shrub species exhibit similar values, all exceeding 12 g/(m2·d). In deciduous shrubs, sequestration rates range from 3.2285 to 10.02 g/(m2·d), with Ligustrum quihoui achieving the highest rate at 10.02 g/(m2·d) and L. indica cv. the lowest at 3.23 g/(m2·d).
For total plant carbon sequestration, evergreen trees demonstrate a wide range of values, from 111.758 to 1060.512 g/d. Cinnamomum camphora exhibits the highest total carbon sequestration capacity, followed by M. grandiflora and O. fragrans, while I. crenata shows the weakest performance. Among deciduous trees, S. babylonica, G. biloba, and C. sinensis demonstrate stronger carbon sequestration capacities, with S. babylonica reaching 219.492 g/d—nearly four times that of Z. serrata. In evergreen shrubs, C. japonica and P. serrulata exhibit the highest total carbon sequestration rates, at 43.6 g/d and 120.934 g/d, respectively, significantly outperforming other evergreen shrub species. Among deciduous shrubs, L. quihoui exhibits the highest total carbon sequestration rate at 18.212 g/d, while L. indica cv. shows the lowest rate at 8.917 g/d.
3.2 Seasonal and plant type variations in carbon sequestration
Figure 3 presents the results of a Principal Component Analysis (PCA) performed on the experimental data, effectively underscoring the primary research focus. The data exhibit a predominantly bimodal distribution along the Y-axis, with the left-hand cluster (red points) corresponding to carbon sequestration per unit canopy projection area and the right-hand cluster (orange points) representing total plant carbon sequestration. This study aims to elucidate the effects of seasonal variation and plant species on both carbon sequestration metrics, specifically unit canopy projection area carbon sequestration and total plant carbon sequestration.
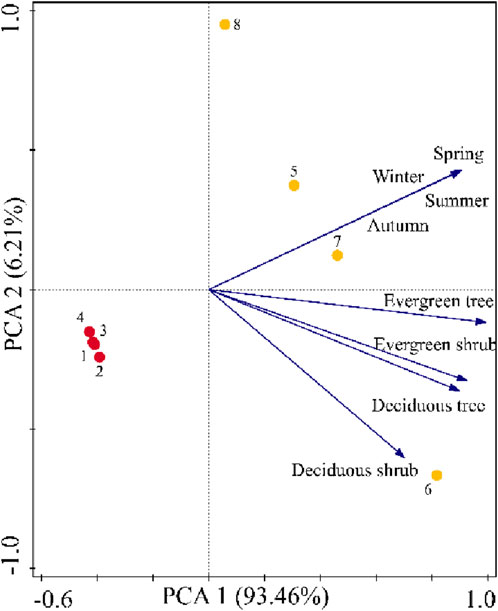
Figure 3. Principal component analysis (PCA) of seasonal and species effects on daily carbon sequestration.
Table 3 presents the Spearman correlation coefficients for carbon sequestration per unit canopy projection area across different seasons and plant types. A seasonal correlation analysis reveals a significant positive correlation between spring and all other seasons (summer, autumn, and winter), with coefficients of 0.727, 0.646, and 0.450 (p < 0.01), respectively. This suggests a strong consistency in carbon sequestration dynamics between spring and the other seasons, likely driven by the favorable temperatures and sunlight conditions in spring, which enhance photosynthetic activity and increase carbon fixation capacity. Notably, the correlation between summer and autumn is particularly strong, reaching 0.889, indicating a high degree of similarity in carbon sequestration patterns between these two seasons. In contrast, winter exhibits weaker correlations with other seasons, particularly with spring, where the coefficient is only 0.450. This can be attributed to the reduced temperatures and daylight hours in winter, which decrease photosynthetic rates and, consequently, carbon sequestration.
When examining correlations across plant types, we find that evergreen trees exhibit relatively stable carbon sequestration across seasons, with a correlation coefficient of 0.643 (p < 0.01) with spring, indicating minimal seasonal variation in their carbon fixation. In contrast, deciduous trees display weaker seasonal correlations, particularly in autumn and winter, with coefficients of 0.124 and 0.072 (p < 0.05), respectively. This suggests that deciduous trees are more sensitive to seasonal fluctuations, especially in autumn and winter, when leaf loss and reduced photosynthetic activity lead to a significant decrease in carbon sequestration. Evergreen shrubs generally exhibit low correlations with seasonal variations, with coefficients of −0.103 and −0.053 for spring and summer (p > 0.05), indicating that their carbon sequestration fluctuates less across seasons. This could be due to their evergreen nature and relatively low photosynthetic efficiency. In contrast, deciduous shrubs display a more complex seasonal pattern: while correlations with other seasons are generally low, the intra-group correlation is negative (−0.692, p < 0.01), suggesting that their carbon sequestration is highly sensitive to seasonal changes, with pronounced fluctuations in carbon fixation across different seasons.
Table 4 presents the Spearman correlation coefficients for carbon fixation in whole plants across different seasons and plant types. Notably, the spring season exhibits significant positive correlations with the other seasons (summer, autumn, and winter), with coefficients of 0.98, 0.976, and 0.543 (p < 0.01), respectively. This suggests a high degree of consistency in carbon fixation between spring and the other seasons, likely attributed to the optimal growth conditions in spring, including sufficient sunlight and favorable temperatures, which enhance photosynthesis and carbon fixation capacity. A strong correlation is observed between summer and autumn, with a coefficient of 0.984, indicating near-perfect synchrony in carbon fixation between these two seasons. In contrast, the correlation in winter is weaker, particularly with spring and summer, with coefficients of 0.543 and 0.588, respectively. This is likely due to the limiting effects of low temperatures and short daylight hours on photosynthesis and carbon fixation during winter.
Among the different plant types, evergreen trees exhibit relatively strong seasonal correlations, particularly in spring, summer, and autumn, with coefficients of 0.448, 0.464, and 0.402 (p < 0.05), respectively. This suggests that evergreen trees maintain relatively stable carbon fixation across seasons, characterized by consistent photosynthetic activity. In contrast, deciduous trees display weaker seasonal correlations, especially in autumn and winter, with coefficients of 0.074 and −0.052, indicating that their carbon fixation capacity is highly influenced by seasonal changes, particularly during autumn and winter when leaf loss reduces photosynthesis and carbon fixation. Evergreen shrubs also exhibit low seasonal correlations, with coefficients of −0.335 and −0.265 in spring and summer (p > 0.05), which may be attributed to their lower photosynthetic efficiency and less pronounced changes in carbon fixation during seasonal transitions. The seasonal correlation of deciduous shrubs is more complex, with an overall weak correlation. However, in spring and winter, the correlation coefficients are 0.178 and 0.271, respectively, suggesting that deciduous shrubs maintain some photosynthetic activity during these seasons. Furthermore, the correlation between deciduous shrubs and other plant types (such as evergreen trees, deciduous trees, and evergreen shrubs) is relatively low, but the within-group correlation is stronger (e.g., with evergreen shrubs at −0.759, p < 0.01), indicating that the carbon fixation of deciduous shrubs is strongly influenced by seasonal changes, with more significant fluctuations in carbon fixation across different seasons.
3.3 Carbon sequestration potential of different plant types
To further elucidate the carbon sequestration potential of diverse plant species, we categorized the plant samples into four distinct groups: Evergreen Trees, Deciduous Trees, Evergreen Shrubs, and Deciduous Shrubs. The comparative analysis of daily carbon sequestration rates for these plant types is presented in the table below, along with the results of the corresponding statistical significance tests. Specifically, the table displays the mean values, mean differences, standard errors, and p-values for both daily carbon sequestration per unit canopy projection area (denoted as A) and total daily carbon sequestration (denoted as B).
Table 5 presents a comprehensive comparison of the average daily carbon sequestration rates per unit canopy projection area across various plant categories. Evergreen trees exhibit the highest sequestration rate, at 18.0024 g/(m2·d), significantly outperforming all other plant types. In contrast, deciduous shrubs display the lowest rate, at 6.1474 g/(m2·d). Notably, both evergreen trees and shrubs demonstrate higher carbon sequestration rates compared to their deciduous counterparts. The superior performance of evergreen trees can be attributed to specific biological traits, including leaf morphology, leaf area index, photosynthetic rate, and respiration rate, which directly enhance their carbon fixation capacity. To further elucidate these differences, we conducted additional analyses using SPSS software and the Games-Howell post hoc test. These results indicate that the carbon sequestration rate of evergreen trees is 8.0228 g/(m2·d) higher than that of deciduous trees, 4.2284 g/(m2·d) higher than evergreen shrubs, and 11.8550 g/(m2·d) higher than deciduous shrubs, with all differences achieving statistical significance. Furthermore, we observed significant differences in carbon sequestration between deciduous trees and evergreen shrubs [3.794 g/(m2·d)], deciduous trees and deciduous shrubs [3.833 g/(m2·d)], and evergreen shrubs and deciduous shrubs [7.622 g/(m2·d)]. These findings underscore the substantial variability in carbon sequestration potential among different plant types.
In addition to the average daily carbon sequestration rates presented in Table 5, the table also provides the total carbon sequestration values for the entire plant (denoted as B) for each plant type. Evergreen trees exhibit the highest total carbon sequestration, averaging 462.28 g/d, while deciduous shrubs sequester the least, at only 13.618 g/d. Notably, within each plant category, evergreen trees sequester significantly more carbon than deciduous trees, and evergreen shrubs outperform deciduous shrubs. The ranking of carbon sequestration by plant type is as follows: evergreen trees > deciduous trees > evergreen shrubs > deciduous shrubs. Overall, trees generally sequester more carbon than shrubs, with this difference being statistically significant. This is primarily due to the larger size of trees, their broader leaf area, and more developed root systems, all of which enable them to accumulate more biomass (such as wood, bark, branches, and leaves) and absorb more water and nutrients through their stronger root systems, thereby enhancing their carbon sequestration capacity. In contrast, shrubs, due to their smaller size and simpler biological structures, exhibit weaker carbon sequestration capabilities. Further analysis using SPSS and the Games-Howell method confirmed significant differences in the daily carbon sequestration rates between plant types. Specifically, evergreen trees sequester 344.80 g/d more carbon than deciduous trees, 408.692 g/d more than evergreen shrubs, and 448.66 g/d more than deciduous shrubs, with all differences being statistically significant. Additionally, the differences between deciduous trees and evergreen shrubs (63.896 g/d), deciduous trees and deciduous shrubs (103.867 g/d), and evergreen shrubs and deciduous shrubs (39.97 g/d) were also found to be statistically significant.
In conclusion, evergreen trees demonstrate a marked superiority in carbon sequestration capacity per unit canopy projection area and total daily carbon sequestration compared to other plant types, with significant differences observed. This is primarily attributed to the larger canopy size and greater biomass of evergreen trees, which provide a greater surface area and extended time for photosynthesis, thereby facilitating the maximization of carbon sequestration through their long-lasting green foliage. In contrast, deciduous shrubs exhibit a weaker carbon sequestration capacity due to their smaller size and limited canopy and biomass, resulting in lower per unit canopy projection area and total daily carbon sequestration values compared to other plant types. Statistical analysis conducted using SPSS software and the Games-Howell method revealed a significant difference in carbon sequestration per unit canopy projection area between evergreen trees and deciduous trees and shrubs, with p-values below 0.001 and 0.05, respectively. These findings suggest that the ecological characteristics of plant species, including leaf morphology and photosynthetic rate, have a significant impact on carbon sequestration efficiency. This statistical analysis highlights the substantial differences in carbon sequestration capacities among plant types, providing valuable scientific insights for plant selection in ecological planning and management. The results of this study underscore the importance of considering the ecological characteristics of plant species in efforts to mitigate climate change and promote sustainable ecosystem management.
3.4 Seasonal carbon sequestration
The seasonal influence on daily carbon sequestration rates per unit canopy projection area varies significantly across plant species and environmental factors. To elucidate the dynamics of plant carbon sequestration capacity across different seasons, this study conducted a comprehensive analysis of the daily carbon sequestration rates for 20 plant species spanning the four seasons, as summarized in Table 6. The results indicate that autumn is characterized by the highest average daily carbon sequestration rate, at 13.886 g/(m2·d), whereas summer exhibits a lower average value, which may be attributed to the more favorable light conditions in autumn that enhance photosynthesis. Notably, despite the reduced daylight duration in autumn compared to summer, the absence of stomatal closure in autumn facilitates sustained photosynthesis. In contrast, spring and winter exhibit the lowest carbon sequestration rates, averaging 6.363 g/(m2·d) and 1.621 g/(m2·d), respectively. This disparity is likely due to the detrimental effects of low temperatures in winter, which compromise the efficiency of photosynthesis and, consequently, lower carbon sequestration. The results of this study underscore the importance of considering seasonal variations in plant carbon sequestration capacity when developing strategies to mitigate climate change. Further research is needed to elucidate the underlying mechanisms driving these seasonal patterns and to explore potential applications for optimizing carbon sequestration through plant management practices.
To investigate the impact of seasonal variations on carbon sequestration, we conducted a one-way analysis of variance (ANOVA) using SPSS software, followed by post hoc comparisons with the Games-Howell method. The results revealed significant differences in daily carbon sequestration rates per unit canopy projection area between autumn and winter [p = 0.324, with a difference of 12.265 g/(m2·d)], as well as between summer and winter [p < 0.001, with a difference of 10.355 g/(m2·d)]. Moreover, significant differences were observed between spring and summer (p < 0.001) and between spring and winter (p < 0.001). In contrast, the differences between spring and autumn [p > 0.05, with a difference of 7.523 g/(m2·d)] and autumn and summer [p > 0.05, with a difference of 1.91 g/(m2·d)] were not statistically significant.
Table 7 reveals significant seasonal fluctuations in total plant carbon sequestration. Summer exhibits the highest rate, averaging 161.745 g/d, driven primarily by the peak growth period, characterized by enhanced leaf physiological activity and optimal photosynthetic efficiency. In contrast, winter sees a sharp decline to 35.49 g/d, the lowest of the year, due to the suppression of photosynthesis by low temperatures. Summer’s favorable conditions, with abundant foliage and optimal light intensity, facilitate high photosynthetic rates, while winter’s reduced chlorophyll content and leaf drop limit photosynthetic efficiency. Spring and autumn carbon sequestration rates (81.295 g/d and 98.454 g/d, respectively) fall between the summer and winter values, reflecting moderate sunlight and temperatures conducive to photosynthesis. Spring sees a gradual intensification of photosynthetic activity as plants recover from dormancy, and daylight hours increase, while autumn’s remaining light intensity and temperatures still support effective photosynthesis, despite shorter daylight hours.
To evaluate the impact of seasonal variation on plant carbon sequestration, a one-way analysis of variance (ANOVA) was conducted using SPSS software, followed by post hoc comparisons with the Games-Howell method. The results reveal a significant seasonal difference in total plant carbon sequestration between summer and winter (p = 0.001, difference = 126.251 g/d), indicating a highly significant seasonal variation. Although the difference between summer and spring is smaller (p = 0.044, difference = 80.455 g/d), it is still statistically significant. Notably, significant seasonal differences were also observed between spring and winter (p < 0.05, difference = 45.80 g/d), autumn and summer (p < 0.05, difference = 63.29 g/d), and autumn and winter (p < 0.05, difference = 62.97 g/d). In contrast, the difference between spring and autumn (p = 0.877, difference = 45.80 g/d) is not statistically significant, suggesting minimal seasonal variation. The seasonal comparison between autumn and winter is particularly pronounced, with a highly significant difference (p = 0.011) and a large difference in carbon sequestration (62.97 g/d).
This study did not directly investigate the impact of seasonal variations on carbon sequestration capacity. However, it indirectly explored the influence of seasonal changes by comparing the carbon sequestration abilities of different plant types (evergreen vs. deciduous). Evergreen trees, which continue to undergo photosynthesis year-round, exhibit higher carbon sequestration capacity, whereas deciduous plants show lower sequestration rates due to reduced photosynthetic activity during winter leaf drop. By analyzing the average and standard deviation of carbon sequestration per unit canopy projection area, the study provided further insight into the potential impact of seasonal variations on plant carbon sequestration efficiency, shedding light on the year-round carbon sink capacity of plants.
3.5 Seasonal CO2 concentration variations
Figure 4 illustrates the variations in CO2 concentration across four zones during different seasons. The data reveal that CO2 concentrations are generally higher in winter, with average values exceeding 700 μmol mol−1. In contrast, summer concentrations average about 460 μmol mol−1, reflecting a significant seasonal difference of 250 μmol mol−1. Additionally, CO2 concentrations vary among the four zones. The Yihe South Park zone consistently exhibits the lowest CO2 levels across all seasons, with a summer concentration of 451.24 μmol mol−1 and a winter peak of 713.37 μmol mol−1. Conversely, the Yinxiang Xinyu zone maintains higher CO2 concentrations year-round, reaching up to 761.32 μmol mol−1 in winter. Arranged from lowest to highest CO2 concentration, the zones are: Yihe South Park, Luotuolula Town, Tanqiao Apartment, and Yinxiang Xinyu. These variations are primarily attributed to the different types of vegetation in each zone: Yihe South Park predominantly features Evergreen trees, Luotuolula Town is characterized by Deciduous trees, Tanqiao Apartment is primarily composed of Evergreen shrubs, and Yinxiang Xinyu contains mostly Deciduous shrubs. The differences in carbon sequestration capabilities among these plant types result in the observed CO2 concentration variations, which are more pronounced in winter.
Figure 5 illustrates the seasonal variation in carbon dioxide concentration across four regions. The data reveal a clear pattern, with CO2 levels lowest in summer (464.195 μmol mol−1) and highest in winter (732.49 μmol mol−1). Spring and autumn exhibit intermediate concentrations (621.058 μmol mol−1 and 571.01 μmol mol−1, respectively). This seasonal variability is likely driven by the contrasting patterns of plant growth and photosynthesis. In summer, enhanced plant growth and photosynthesis lead to increased CO2 absorption for carbon fixation, thereby reducing atmospheric CO2 levels. In contrast, winter sees reduced plant activity and weaker photosynthesis, resulting in decreased carbon fixation and potentially increased CO2 release from respiration and other biological processes. The CO2 levels in spring and autumn are probably influenced by the varying intensities of plant growth and photosynthesis, falling between the extremes observed in summer and winter.
4 Discussion
This study uses a standardized quantification method to assess the climate change mitigation and carbon sequestration potential of urban residential green spaces. The findings show that evergreen trees and woody plants exhibit significantly higher carbon sequestration capacity compared to deciduous plants and shrubs. These results are consistent with existing literature, which highlights the strong correlation between plant types and their carbon sequestration capabilities. Evergreen plants, which maintain photosynthesis throughout the year, can absorb carbon dioxide continuously, whereas deciduous plants experience a significant reduction in carbon sequestration capacity during winter due to the decline in photosynthetic activity associated with leaf drop. A study on urban tree carbon storage and sequestration in the United States found that tree density and species type have a substantial impact on carbon storage, with variations in tree carbon storage density and annual sequestration rates across cities providing valuable insights into the role of plant types in carbon sequestration (Nowak et al., 2013).
This study provides a more comprehensive and quantitative evaluation of the carbon sequestration potential of urban residential green spaces through a standardized methodology. Unlike previous research, which often focuses on individual plant species or specific environmental factors, this study fills a gap by incorporating a multidimensional analysis. Research on the relationship between urban park ecosystem services and vegetation types highlights the trade-offs between vegetation diversity and ecosystem services, further underscoring the importance of different plant types in carbon sequestration (Mexia et al., 2018). Additionally, studies on county-level ecological space management and carbon sink capacity optimization have proposed strategies to enhance carbon sink capacity through improved ecological space management, offering practical insights for optimizing carbon sequestration in urban green spaces (Li et al., 2024).
However, most previous studies have focused on single factors, neglecting the integrated impact of plant species, green space layout, and management strategies. In contrast, this study examines the carbon sequestration potential of different plant species and their seasonal variations, while also investigating the role of green space design and management practices. This multidimensional approach bridges gaps in the current literature and offers new perspectives and practical pathways for enhancing the carbon sequestration efficiency of urban green spaces.
4.1 The limitations of the method
The fixed percentage method, which typically employs a 20% estimate of nighttime respiration carbon emissions, is a widely used approach for quantifying plant carbon losses. However, this method has several limitations that can lead to biased estimates of carbon sequestration. Firstly, it fails to account for the temperature effect on nighttime respiration rates, which can be up to 40%–50% higher in high-temperature environments, resulting in underestimation of carbon sequestration. For instance, if a plant’s photosynthetic carbon uptake is 100 g/m2/day, the fixed percentage method would estimate nighttime respiration at 20 g, but the actual rate could be as high as 30 g, leading to a 12.5% error in the carbon sequestration estimate. Secondly, water stress can significantly reduce respiration rates, particularly in arid regions, where the fixed percentage method may overestimate nighttime respiration, introducing errors as high as 40%. Under drought conditions, a plant with 100 g of photosynthetic carbon uptake might have an actual nighttime respiration of only 12 g, but the fixed percentage method would still estimate it at 20 g, causing a 40% error. Furthermore, the fixed percentage method does not account for species-specific differences in respiration rates, which can lead to significant errors in carbon sequestration estimates. For example, coniferous trees and herbaceous plants may exhibit significantly different respiration rates, even within the same environment, resulting in errors of 10%–20%. Finally, the method fails to consider changes in respiration rates across different growth stages, which can lead to underestimation of carbon sequestration during active growth periods and overestimation during dormancy, with errors potentially reaching 25%. To enhance the accuracy of carbon sequestration estimates, future research should incorporate more precise, real-time respiration measurement methods, such as automated gas exchange systems, to reduce the errors and uncertainties introduced by the fixed percentage assumption (Zhao et al., 2022).
Furthermore, neglecting the impact of tree age on carbon storage potential may lead to inaccurate estimates, as tree age is closely related to carbon storage capacity. Younger trees, with limited carbon storage potential, can be overestimated, while older trees, with higher carbon storage potential, can be underestimated. Shrubs, with their shorter life cycle, have limited carbon sequestration capacity. Failing to consider tree age can result in inaccurate assessments of carbon storage potential, affecting forest management decisions. To address this, future research should incorporate tree age as a factor, refine age categories, and utilize models relating tree age to biomass to ensure more accurate carbon storage estimates. It is also essential to distinguish and evaluate the carbon storage characteristics of trees and shrubs separately for their carbon sequestration potential. This distinction is crucial for developing effective strategies for mitigating urban warming.
Additionally, this study’s reliance on a limited sample size (1,350 samples over 3 days during each season) may not provide a comprehensive seasonal overview. To address this, future research should increase the sample size to explore more factors influencing plant carbon sequestration and ultimately develop more effective strategies for mitigating urban warming.
4.2 Optimizing urban carbon sequestration
4.2.1 Optimizing carbon estimation
To more accurately estimate the carbon sequestration potential of urban green spaces, a species-weighted average method should be adopted when calculating the carbon sequestration capacity of individual plants. This approach integrates the carbon sequestration abilities of various plant types and species, providing a more nuanced understanding of how plant diversity and environmental factors influence carbon sequestration outcomes. In addition, factors such as plant density, spatial configuration, and total area should be carefully considered during the assessment process. High-density planting can significantly increase above-ground biomass, thereby enhancing overall carbon sequestration. To further improve predictive accuracy, dynamic models should be employed, incorporating variables such as plant growth cycles, seasonal fluctuations, and climate conditions. This will enable more precise estimation of carbon sequestration potential. Root carbon storage should also be included in the evaluation framework, as roots play a critical role in carbon storage within green spaces. Implementing these optimization strategies will facilitate a more comprehensive and precise estimation of the carbon sequestration capacity of urban green spaces (Chen, 2015; Zaid et al., 2018).
4.2.2 Evergreen trees strategy
When planning urban green space systems, evergreen trees should be prioritized due to their high carbon sequestration capacity per unit of crown area and overall carbon storage potential. These plants offer a consistent rate of carbon fixation throughout the year, including during winter, providing long-term and stable ecological services to the ecosystem. In contrast, deciduous plants enter dormancy in winter and temporarily cease photosynthesis, reducing their carbon sequestration capacity. However, their efficient carbon fixation capacity during the growing season should not be overlooked. Deciduous trees, with their large size and broad leaf area, can absorb and store significant amounts of carbon dioxide during their growing period. While evergreen trees have slower growth rates, which may affect short-term carbon sink benefits in the early stages of green space development, deciduous species can provide a temporary boost to carbon sequestration capacity during the growing season. Therefore, it is essential to carefully weigh the advantages and disadvantages of evergreen versus deciduous species in the planning process (Jevon et al., 2024; Zhao et al., 2023).
To maximize the carbon sequestration potential of plants while promoting sustainable urban ecological development, a carefully designed plant configuration strategy is necessary. This strategy requires consideration of the ecological adaptability and growth requirements of different plant species to ensure the stability of the plant community and its efficient carbon sink function. A scientifically based approach is essential to determine the optimal planting ratio of evergreen shrubs, deciduous trees, and deciduous shrubs. This should be achieved by adjusting the planting ratio according to the carbon fixation capacity and ecological characteristics of each species. It is generally recommended to increase the planting area of evergreen shrubs and deciduous trees, while strategically incorporating deciduous shrubs. This approach not only enhances the landscape’s spatial layers and seasonal diversity but also ensures a balanced carbon sequestration capacity while maintaining ecological services. By adopting this strategy, it is possible to promote a sustainable urban ecological environment that maximizes carbon sequestration potential while maintaining ecological stability.
4.2.3 Winter greening management
Seasonal climate variations, particularly changes in solar radiation, temperature, precipitation, and daylight duration, have a direct and significant impact on plant photosynthetic efficiency. In spring and summer, characterized by extended daylight and high solar radiation intensity, photosynthetic activity is markedly enhanced, leading to increased carbon fixation. Conversely, the shorter daylight hours and lower temperatures of autumn and winter substantially limit photosynthesis, resulting in a pronounced decline in carbon fixation capacity. Plants also exhibit adaptive physiological responses to these seasonal changes. For instance, deciduous species enter dormancy in autumn, shedding leaves and nearly halting photosynthetic activity, while evergreen species, though retaining their foliage, experience reduced photosynthetic efficiency during colder months. The harsh environmental conditions of winter, including low temperatures and dryness, pose substantial challenges to plant survival, necessitating effective winter greening management strategies. Scientifically guided maintenance practices, such as optimized watering and fertilization, can help sustain plant vitality and partially mitigate the seasonal reduction in carbon sequestration capacity. This is particularly important for enhancing urban carbon sequestration. Strategic watering practices, tailored to plant species, growth stages, and soil moisture levels, are critical to ensuring adequate hydration without the risks associated with overwatering or underwatering. Similarly, careful fertilization—encompassing appropriate nutrient selection, application methods, and regulation of quantity and frequency—minimizes the risk of damage from over-fertilization while supporting plant health during winter.
Winter pruning also plays a vital role in green infrastructure management. By removing dead leaves, damaged branches, and weak plants, pruning not only promotes photosynthetic efficiency and growth but also improves plant structure and aesthetic value. Collectively, these measures contribute to maintaining plant health and enhancing urban ecosystem carbon sequestration, even under the limiting conditions of winter (Shirley et al., 2022; Filipiak et al., 2023).
4.2.4 Scientific greening strategy
Scientific greening principles and technologies enable urban landscaping to harmonize with natural laws, maximizing carbon sequestration potential. A continuous monitoring and evaluation system ensures that greening projects progress steadily, allowing for timely identification and correction of deviations, and guaranteeing the sustainability and stability of carbon sequestration effects. Promoting scientific greening principles and enhancing monitoring and evaluation frameworks are crucial for building a healthy, stable ecosystem, improving urban ecological carrying capacity, and enhancing environmental resilience. To achieve these goals, public education and demonstration efforts are essential, increasing awareness and participation in greening initiatives and fostering a societal atmosphere that supports and values greening efforts (Dai et al., 2024). Effective greening projects can disseminate scientific greening principles and technologies through various activities, such as lectures, exhibitions, and workshops, while tailoring plans to the region’s climate, soil, and water conditions to ensure scientific soundness and regional appropriateness. Advanced greening technologies, including water-saving irrigation, soil improvement, and pest control, should be actively introduced and promoted. Encouraging the development of plant varieties adapted to local climate and soil conditions can further enhance carbon sequestration capacity and environmental adaptability.
To ensure long-term effectiveness, a comprehensive monitoring system is critical. Monitoring stations should be established within greening areas, equipped with advanced tools, and detailed monitoring plans should be developed to collect key data, including species composition, leaf area index, soil moisture, and light intensity. The monitoring results should inform adjusting greening strategies and optimizing resource allocation, allowing for optimized carbon storage efficiency. For instance, using data on leaf area index and carbon sequestration potential can inform the optimal planting ratio of evergreen and deciduous trees. In cases where carbon sequestration is insufficient, solutions can include increasing vegetation density, adjusting irrigation and fertilization practices, or selecting plant varieties with higher carbon storage capacity. Regular assessments of greening project implementation will ensure that strategies are adjusted in a timely manner, enabling better long-term ecological and carbon sequestration benefits (Gao et al., 2023).
4.2.5 Advancing carbon sequestration estimation
To improve the accuracy of plant carbon sequestration estimates, advanced modeling techniques and diverse data sources, including machine learning, big data analysis, and remote sensing technologies, must be integrated. By combining remote sensing imagery, climate models, plant growth models, and soil carbon storage data, machine learning algorithms can accurately identify and classify various plant species, green space types, and environmental conditions, resulting in more precise carbon sequestration predictions. Furthermore, incorporating long-term monitoring data and historical climate records enables artificial intelligence to recognize patterns and analyze trends, providing insights into the impact of climate change on urban carbon sequestration potential and informing mitigation strategies. This integrated, data-driven approach not only enhances the accuracy of estimates but also enables real-time updates and adjustments, thereby increasing the reliability of future carbon sequestration projections. The resulting scientifically robust decision-making tools empower urban planners to design green space systems that align with sustainable development goals, effectively combat climate change, and support the achievement of carbon neutrality targets. By leveraging the power of data analytics and machine learning, urban planners can create greener, more resilient cities that mitigate the effects of climate change and promote a sustainable future.
5 Conclusion
This study highlights the pivotal role of urban green spaces in mitigating climate change, with a specific emphasis on their carbon sequestration potential in residential areas. By analyzing four representative residential areas in Nanjing, the research reveals how plant types and seasonal variations influence carbon sequestration. Evergreen trees demonstrated the highest sequestration potential, particularly in autumn, while total sequestration across all vegetation peaked during the summer. Key growth parameters, including tree diameter, canopy spread, and height, were strongly correlated with carbon sequestration capacity.
To enhance carbon sequestration efforts, future research should expand to include diverse urban green spaces, such as parks and riparian zones, and leverage advanced tools like remote sensing and ecosystem models to improve data accuracy. Additionally, the effects of human management practices, such as pruning, on carbon sequestration should be investigated to provide actionable insights for urban planning.
The study offers several policy recommendations: prioritize the planting of evergreen tree species, optimize vegetation composition to align with seasonal dynamics, and integrate carbon sequestration targets into urban planning frameworks. These strategies will not only support carbon neutrality goals but also enhance urban ecosystem services and resilience.
Data availability statement
The original contributions presented in the study are included in the article/supplementary material, further inquiries can be directed to the corresponding author.
Author contributions
HL: Conceptualization, Data curation, Formal Analysis, Funding acquisition, Investigation, Methodology, Resources, Software, Validation, Visualization, Writing–original draft. JZ: Project administration, Supervision, Writing–review and editing. ZW: Project administration, Supervision, Writing–review and editing.
Funding
The author(s) declare that financial support was received for the research, authorship, and/or publication of this article. This research was supported by Art Project of National Social Science Foundation. National Social Science Office Project Number: 2023BG01252 “Research on Rural Landscape Ecological Design of Yangtze River Delta under the Background of Yangtze River Protection”.
Acknowledgments
All authors thank the reviewers and all members of our team for their insightful comments.
Conflict of interest
The authors declare that the research was conducted in the absence of any commercial or financial relationships that could be construed as a potential conflict of interest.
Generative AI statement
The author(s) declare that no Generative AI was used in the creation of this manuscript.
Publisher’s note
All claims expressed in this article are solely those of the authors and do not necessarily represent those of their affiliated organizations, or those of the publisher, the editors and the reviewers. Any product that may be evaluated in this article, or claim that may be made by its manufacturer, is not guaranteed or endorsed by the publisher.
References
Alberdi, I., Moreno-Fernández, D., Cañellas, I., Adame, P., and Hernández, L. (2020). Deadwood stocks in south-western European forests: ecological patterns and large scale assessments. Sci. Total Environ. 747, 141237. doi:10.1016/j.scitotenv.2020.141237
Author Anonymous (2002). Comparison-of-Film-and-Digital-Hemispherical-photogr_2002_Agricultural-and-F.Pdf. Agric. For. Meteorology 112, 51–56. doi:10.1016/S0168-1923(02)00042-4
Bai, Y., and Ding, G. (2024). Estimation of changes in carbon sequestration and its economic value with various stand density and rotation age of pinus massoniana plantations in China. Sci. Rep. 14, 16852. doi:10.1038/s41598-024-67307-z
Bulusu, M., Martius, C., and Clendenning, J. (2021). Carbon stocks in Miombo woodlands: evidence from over 50 years. Forests 12, 862. doi:10.3390/f12070862
Chen, W. Y. (2015). The role of urban green infrastructure in offsetting carbon emissions in 35 major Chinese cities: a nationwide estimate. Cities 44, 112–120. doi:10.1016/j.cities.2015.01.005
Dai, E., Zhao, Z., Jia, L., and Jiang, X. (2024). Contribution of ecosystem services improvement on achieving sustainable development goals under ecological engineering projects on the qinghai-tibet plateau. Ecol. Eng. 199, 107146. doi:10.1016/j.ecoleng.2023.107146
Dong, H., Chen, Y., and Huang, X. (2023). A new framework for analysis of the spatial patterns of 15-minute neighbourhood green space to enhance carbon sequestration performance: a case study in nanjing, China. Ecol. Indic. 156, 111196. doi:10.1016/j.ecolind.2023.111196
Dou, Y., Tian, F., Wigneron, J.-P., Tagesson, T., Du, J., Brandt, M., et al. (2023). Reliability of using vegetation optical depth for estimating decadal and interannual carbon dynamics. Remote Sens. Environ. 285, 113390. doi:10.1016/j.rse.2022.113390
Filipiak, M., Gabriel, D., and Kuka, K. (2023). Simulation-based assessment of the soil organic carbon sequestration in grasslands in relation to management and climate change scenarios. Heliyon 9, e17287. doi:10.1016/j.heliyon.2023.e17287
Gao, T., Song, X., Ren, Y., Liu, H., Qu, H., and Dong, X. (2023). Thinning intensity affects carbon sequestration and release in seasonal freeze–thaw areas. J. For. Res. 34, 993–1006. doi:10.1007/s11676-022-01564-4
He, C., Zhang, D., Huang, Q., and Zhao, Y. (2016). Assessing the potential impacts of urban expansion on regional carbon storage by linking the LUSD-urban and InVEST models. Environ. Model. and Softw. 75, 44–58. doi:10.1016/j.envsoft.2015.09.015
Huang, X., Cheng, F., Wang, J., Yi, B., and Bao, Y. (2023). Comparative study on remote sensing methods for forest height mapping in complex mountainous environments. Remote Sens. 15, 2275. doi:10.3390/rs15092275
Ismaili Alaoui, H., Chemchaoui, A., El Asri, B., Ghazi, S., Brhadda, N., and Ziri, R. (2023). Modeling predictive changes of carbon storage using invest model in the beht watershed (Morocco). Model. Earth Syst. Environ. 9, 4313–4322. doi:10.1007/s40808-023-01697-3
Jevon, F., Crown, C. A., Clark, J. A. G., Doroski, D. A., Darling, L., Sonti, N. F., et al. (2024). Native trees are responsible for the high carbon density in urban natural area forests across eight United States cities. J. Appl. Ecol. 62, 132–143. doi:10.1111/1365-2664.14823
Jiang, Y., Liu, Y., Sun, Y., and Li, X. (2023). Distribution of CO2 concentration and its spatial influencing indices in urban park green space. Forests 14, 1396. doi:10.3390/f14071396
Jin, S., Zhang, E., Guo, H., Hu, C., Zhang, Y., and Yan, D. (2023). Comprehensive evaluation of carbon sequestration potential of landscape tree species and its influencing factors analysis: implications for urban green space management. Carbon Balance Manage 18, 17. doi:10.1186/s13021-023-00238-w
Kaur, S., Babbar, D., Sarif, O., Ghatak, A., and Jaafari, A. (2022). “Assessment of carbon sequestration using InVEST model in Delhi, India,” in Conservation, management and monitoring of forest resources in India. Editors M. Sahana, G. Areendran, and K. Raj (Cham: Springer International Publishing), 33–56.
Kohonen, K.-M., Dewar, R., Tramontana, G., Mauranen, A., Kolari, P., Kooijmans, L. M. J., et al. (2022). Intercomparison of methods to estimate gross primary production based on CO2 and COS flux measurements. Biogeosciences 19, 4067–4088. doi:10.5194/bg-19-4067-2022
Leppänen, P.-K., Kinnunen, A., Hautamäki, R., Järvi, L., Havu, M., Junnila, S., et al. (2024). Impact of changing urban typologies on residential vegetation and its climate-effects – a case study from Helsinki, Finland. Urban For. and Urban Green. 96, 128343. doi:10.1016/j.ufug.2024.128343
Li, J., Zhang, Y., Xia, L., Wang, J., Ye, H., Liu, S., et al. (2024). Research on zoning and carbon sink enhancement strategies for ecological spaces in counties with different landform types. Sustainability 16, 5700. doi:10.3390/su16135700
Lindén, L., Riikonen, A., Setälä, H., and Yli-Pelkonen, V. (2020). Quantifying carbon stocks in urban parks under cold climate conditions. Urban For. and Urban Green. 49, 126633. doi:10.1016/j.ufug.2020.126633
Liu, C., Kang, S., Li, F., Li, S., and Du, T. (2013). Canopy leaf area index for apple tree using hemispherical photography in arid region. Sci. Hortic. 164, 610–615. doi:10.1016/j.scienta.2013.10.009
Liu, C., and Li, X. (2012). Carbon storage and sequestration by urban forests in Shenyang, China. Urban For. and Urban Green. 11, 121–128. doi:10.1016/j.ufug.2011.03.002
Liu, M., Grana, D., and Mukerji, T. (2024). Geostatistical inversion for subsurface characterization using Stein variational gradient descent with autoencoder neural network: an application to geologic carbon sequestration. JGR. Solid Earth 129. doi:10.1029/2024jb029073
Liu, Y., Yu, G., Wang, Q., Zhang, Y., and Xu, Z. (2014). Carbon carry capacity and carbon sequestration potential in China based on an integrated analysis of mature forest biomass. Sci. China Life Sci. 57, 1218–1229. doi:10.1007/s11427-014-4776-1
Marteau, A., Fourmaux, M., and Mevy, J.-P. (2023). The role of gorse (ulex parviflorus pourr. Scrubs) in a mediterranean shrubland undergoing climate change: approach by hyperspectral measurements. Plants 12, 879. doi:10.3390/plants12040879
Mexia, T., Vieira, J., Príncipe, A., Anjos, A., Silva, P., Lopes, N., et al. (2018). Ecosystem services: urban parks under a magnifying glass. Environ. Res. 160, 469–478. doi:10.1016/j.envres.2017.10.023
Moody, R., Geron, N., Healy, M., Rogan, J., and Martin, D. (2021). Modeling the spatial distribution of the current and future ecosystem services of urban tree planting in chicopee and fall river, Massachusetts. Urban For. and Urban Green. 66, 127403. doi:10.1016/j.ufug.2021.127403
Nayak, N., Mehrotra, R., and Mehrotra, S. (2022). Carbon biosequestration strategies: a review. Carbon Capture Sci. and Technol. 4, 100065. doi:10.1016/j.ccst.2022.100065
Niu, Z., He, H., Peng, S., Ren, X., Zhang, L., Gu, F., et al. (2021). A process-based model integrating remote sensing data for evaluating ecosystem services. J. Adv. Model Earth Syst. 13, e2020MS002451. doi:10.1029/2020MS002451
Nowak, D. J., Greenfield, E. J., Hoehn, R. E., and Lapoint, E. (2013). Carbon storage and sequestration by trees in urban and community areas of the United States. Environ. Pollut. 178, 229–236. doi:10.1016/j.envpol.2013.03.019
Pascual, A., Giardina, C. P., Selmants, P. C., Laramee, L. J., and Asner, G. P. (2021). A new remote sensing-based carbon sequestration potential index (CSPI): a tool to support land carbon management. For. Ecol. Manag. 494, 119343. doi:10.1016/j.foreco.2021.119343
Rasoolzadeh, R., Mobarghaee Dinan, N., Esmaeilzadeh, H., Rashidi, Y., Marcu, M. V., and Sadeghi, S. M. M. (2024). Carbon sequestration and storage of urban trees in a polluted semiarid city. Forests 15, 1488. doi:10.3390/f15091488
Shirley, I. A., Mekonnen, Z. A., Grant, R. F., Dafflon, B., Hubbard, S. S., and Riley, W. J. (2022). Rapidly changing high-latitude seasonality: implications for the 21st century carbon cycle in Alaska. Environ. Res. Lett. 17, 014032. doi:10.1088/1748-9326/ac4362
Smith, A. M., and Ramsay, P. M. (2018). A comparison of ground-based methods for estimating canopy closure for use in phenology research. Agric. For. Meteorology 252, 18–26. doi:10.1016/j.agrformet.2018.01.002
Sun, W., and Liu, X. (2020). Review on carbon storage estimation of forest ecosystem and applications in China. For. Ecosyst. 7, 4. doi:10.1186/s40663-019-0210-2
Tadesse, S., and Tamiru, A. (2024). Carbon stock of shatto forest along altitudinal gradients in masha district, southwest Ethiopia: a contribution to climate change mitigation. For. Sci. Technol. 20, 171–178. doi:10.1080/21580103.2024.2339823
Tao, P., Lin, Y., Wang, X., Li, J., Ma, C., Wang, Z., et al. (2023). Optimization of green spaces in plain urban areas to enhance carbon sequestration. Land 12, 1218. doi:10.3390/land12061218
Torre-Tojal, L., Bastarrika, A., Boyano, A., Lopez-Guede, J. M., and Graña, M. (2022). Above-ground biomass estimation from LiDAR data using random forest algorithms. J. Comput. Sci. 58, 101517. doi:10.1016/j.jocs.2021.101517
Wang, L., Sun, Z., Li, Z., Zhao, W. J., and Li, Y. (2013). An optimal model for carbon dioxide emission control in the low-carbon urban agglomeration based on sustainable development of economy, society and environment (2): a case study. Adv. Mater. Res. 610–613, 970–974. doi:10.4028/www.scientific.net/AMR.610-613.970
Wang, Y., Bakker, F., De Groot, R., and Wörtche, H. (2014). Effect of ecosystem services provided by urban green infrastructure on indoor environment: a literature review. Build. Environ. 77, 88–100. doi:10.1016/j.buildenv.2014.03.021
Yu, K., Smith, W. K., Trugman, A. T., Condit, R., Hubbell, S. P., Sardans, J., et al. (2019). Pervasive decreases in living vegetation carbon turnover time across forest climate zones. Proc. Natl. Acad. Sci. U.S.A. 116, 24662–24667. doi:10.1073/pnas.1821387116
Yu, X., Kuruppuarachchi, D., and Kumarasinghe, S. (2024a). Financial development, FDI, and CO2 emissions: does carbon pricing matter? Appl. Econ. 56, 2959–2974. doi:10.1080/00036846.2023.2203460
Yu, Z., Liu, S., Li, H., Liang, J., Liu, W., Piao, S., et al. (2024b). Maximizing carbon sequestration potential in Chinese forests through optimal management. Nat. Commun. 15, 3154. doi:10.1038/s41467-024-47143-5
Yuan, Y., Tang, S., Zhang, J., and Guo, W. (2023). Quantifying the relationship between urban blue-green landscape spatial pattern and carbon sequestration: a case study of nanjing’s central city. Ecol. Indic. 154, 110483. doi:10.1016/j.ecolind.2023.110483
Zaid, S. M., Perisamy, E., Hussein, H., Myeda, N. E., and Zainon, N. (2018). Vertical greenery system in urban tropical climate and its carbon sequestration potential: a review. Ecol. Indic. 91, 57–70. doi:10.1016/j.ecolind.2018.03.086
Zhang, Y., Miao, D., Hou, X., and Jia, M. (2024). Just transition for China’s coal regions towards carbon neutrality targets. SI 12, 7494. doi:10.17645/si.7494
Zhang, Z., Wan, X., Sheng, K., Sun, H., Jia, L., and Peng, J. (2023). Impact of carbon sequestration by terrestrial vegetation on economic growth: evidence from Chinese county satellite data. Sustainability 15, 1369. doi:10.3390/su15021369
Zhao, D., Cai, J., Xu, Y., Liu, Y., and Yao, M. (2023). Carbon sinks in urban public green spaces under carbon neutrality: a bibliometric analysis and systematic literature review. Urban For. and Urban Green. 86, 128037. doi:10.1016/j.ufug.2023.128037
Keywords: greenhouse gases, green spaces in urban residential areas, assimilation method, carbon sequestration per unit projected canopy area, carbon sequestration of the whole plant
Citation: Liu H, Zhang J and Wang Z (2025) Assessing and optimizing the potential for climate change mitigation and carbon sequestration in urban residential green spaces: energizing sustainable cities. Front. Environ. Sci. 13:1519297. doi: 10.3389/fenvs.2025.1519297
Received: 29 October 2024; Accepted: 13 January 2025;
Published: 27 January 2025.
Edited by:
Prashant Sharma, Dr. Yashwant Singh Parmar University of Horticulture and Forestry, IndiaReviewed by:
Akash Ravindra Chichaghare, Central Arid Zone Research Institute (ICAR), IndiaKamlesh Verma, Central Soil Salinity Research Institute (ICAR), India
Alok Kumar Singh, Banaras Hindu University, India
Copyright © 2025 Liu, Zhang and Wang. This is an open-access article distributed under the terms of the Creative Commons Attribution License (CC BY). The use, distribution or reproduction in other forums is permitted, provided the original author(s) and the copyright owner(s) are credited and that the original publication in this journal is cited, in accordance with accepted academic practice. No use, distribution or reproduction is permitted which does not comply with these terms.
*Correspondence: Hancheng Liu, bGl1aGFuY2hlbmdAbGR5LmVkdS5ycw==