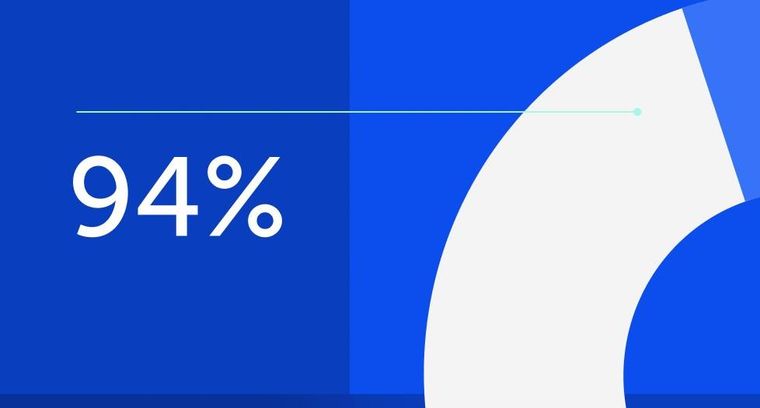
94% of researchers rate our articles as excellent or good
Learn more about the work of our research integrity team to safeguard the quality of each article we publish.
Find out more
ORIGINAL RESEARCH article
Front. Environ. Sci., 11 February 2025
Sec. Soil Processes
Volume 13 - 2025 | https://doi.org/10.3389/fenvs.2025.1506113
This article is part of the Research TopicNitrate from Field to Stream: Characterization and MitigationView all 13 articles
Artificial drainage via subterranean drainage pipes called tiles transport nitrate from fields to streams, impairing downstream surface waters. Increased crop rotational diversity may conserve nitrogen, support greater system resiliency, and reduce tile nitrate loss. This 9-year study compares tile nitrate loss under a 3-year rotation of corn-soybean-winter wheat/double crop soybean plus cereal rye after corn versus a conventionally managed 2-year corn-soybean rotation. Tile flow and tile nitrate concentration were measured from 2015 through 2023 to determine annual flow weighted mean concentrations and loads of tile nitrate. Cereal rye following corn greatly reduced annual tile nitrate; however, more than 6 Mg ha−1 of above ground biomass may have decreased soybean yield. Winter wheat was efficient at using split-applied fertilizer nitrogen (N) as annual tile nitrate concentrations were reduced similar to cereal rye. Double crop soybean after winter wheat may be the most productive phase of the rotation; however, an early killing freeze greatly reduced soybean yield and increased tile nitrate loss. Interestingly, the subsequent corn crop may have benefitted from this unexpected N source. Overall, tile nitrate loss was approximately 50% less in the diverse 3-year rotational system, suggesting that a more diverse rotational system is efficient at retaining N, conserving nitrogen in the field, and reducing N loss to surface water. This study demonstrated proof of concept that a more diverse crop rotation can reduce tile nitrate loss from fields to streams, while maintaining crop productivity.
Nitrate loss from agricultural fields via subterranean drainage pipes called tiles is a major source of riverine nitrate load in central Illinois (David et al., 1997; David et al., 2010; Gentry et al., 1998; Gentry et al., 2009; Tilman et al., 2011; Mitchell et al., 2000; Pittelkow et al., 2017). A recent report by the Census of Agriculture estimated that approximately 3.6 million hectares in Illinois (36% of the total farmland) contain tile drainage (USDA National Agricultural Statistics Service, 2017). The predominant cropping system in Illinois is a 2-year rotation of corn (Zea Mays L.) and soybean (Glycine max L.) with nearly a 50:50 mix of corn and soybean produced annually in central Illinois. Current Illinois fertilizer N recommendations for corn in central Illinois is approximately 200 kg ha−1 based on the Maximum Return to Nitrogen calculator [Home:: Nitrogen Rate Calculator (cornnratecalc.org)]; whereas soybean does not require fertilizer N (Vonk et al., 2024). Typical losses of riverine nitrate in central Illinois headwater streams average approximately 30 kg ha−1 yr−1 (Gentry et al., 2014) with nitrate concentrations in the spring frequently greater than the US EPA drinking water standard of 10 mg L−1 NO3−N (Royer et al., 2006). A contributing factor to large riverine nitrate loads in these extensively tile-drained watershed is the common practice of applying fertilizer N for next year’s corn production in the late fall following soybean harvest (Welch et al., 1971; Randall and Vetsch, 2005; Gentry et al., 2014). Moving fall N application to the spring has been shown to reduce tile nitrate (David et al., 2015; Pittelkow et al., 2017; Gentry et al., 2024). Various split applications of fertilizer N applied in the spring, at planting, and early side-dress can reduce tile nitrate loss (Clark et al., 2020; Gentry et al., 2024); however, legacy N from previous fertilizer N applications contributes to tile nitrate loss for several years, masking immediate gains in water quality from improved fertilizer N use efficiency (David et al., 2016a; Yu et al., 2024).
Another important source of tile and riverine N load is nitrate liberated from soil N mineralization, especially following soybean (Gentry et al., 2001; Gentry et al., 2024). Although the soybean crop depletes soil N (Ciampitti and Salvagiotti, 2018), there is more soil inorganic N available to corn following soybean than when corn follows corn, reducing the fertilizer N requirement of rotated corn (Sawyer et al., 2006; Gentry et al., 2013). This rotation effect formerly known as the soybean N credit is largely due to greater net soil N mineralization following soybean than corn (Green and Blackmer, 1995; Gentry et al., 2001). Although both crops have residue with a wide carbon (C): N ratio, corn has approximately 3 times more crop residue than soybean. Therefore, corn residue has a greater N immobilization potential which can result in reduced plant available N as well as reduced tile nitrate loss during the non-growing season. In contrast, the soybean phase may be the weak link in soil C replenishment during the corn-soybean rotation, resulting in the loss of long-term soil organic C following soybean (Coulter et al., 2009).
Longer and more diverse crop rotations have been shown to benefit corn (Kazula and Lauer, 2018), and soybean (Janovicek et al., 2021), reduce nutrient loss (Hanrahan et al., 2024), suppress disease (Peralta et al., 2018), improve soil health (Baldwin-Kordick et al., 2022) and increase system resiliency (Bowles et al., 2020). A more diverse rotation with winter wheat (Triticum Aestivum L.) may indirectly benefit corn and soybean production by breaking pest cycles (Rocha et al., 2021). However, adding winter wheat after soybean may directly benefit wheat by providing the opportunity to scavenge mineralized N in the fall, generally before the tile drainage season begins. Although winter wheat receives fertilizer N, it is often split applied in the spring when the crop is actively growing and may be inherently more efficient than fertilizer N application to corn. Another benefit of adding winter wheat is the possibility of producing double crop soybean in the same year. A 3-year rotation of corn, soybean and winter wheat plus double crop soybean is a common cropping system in southern Illinois and the winter wheat/double crop soybean phase of the rotation can be the most profitable (Schnitkey et al., 2022; Shrestha et al., 2021).
Overwintering grass cover crops such as cereal rye (Secale cereale L.) can act as a N catch crop, accumulating soil inorganic N and reducing tile nitrate loss (Kaspar et al., 2012). Gentry et al. (2024) used cereal rye after corn in a 2-year corn and soybean rotation to reduce tile nitrate by more than 40%. Historically, cereal rye cover crops have been used to reduced soil erosion (Basche et al., 2016), weed pressure (Nichols et al., 2020b), and weed seedbank (Nichols et al., 2020a). Producing sufficient cereal rye biomass to reduce tile nitrate also contributes extra C to the system, which may enhance N immobilization (Basche et al., 2016). Several studies indicate a yield penalty with cereal rye before corn (Crandall et al., 2005; Qin et al., 2021), but not before soybean (Ruffo et al., 2004; Behnke et al., 2020; Silva et al., 2024) likely due to its N fixing capability. Additionally, using cereal rye before soybean reduces the risk of enhancing nitrous oxide production from the interaction of cover crop decomposition and added fertilizer N (Preza-Fontes et al., 2021).
Using a systems approach, the objective of this study was to evaluate crop yield and tile nitrate loss in a more diverse 3-year crop rotation of corn-soybean-winter wheat/double crop soybean plus cereal rye following corn (experimental treatment) versus a conventionally managed 2-year crop rotation of corn and soybean with full width tillage and fall fertilizer N application (control treatment). We hypothesized that the diverse rotation with a longer duration of living roots and greater C input compared to the conventional system could reduce tile nitrate loss. In addition, we hypothesize that the diverse rotation may support greater corn and soybean grain yields. The control treatment was produced by summing tile flow and tile loads from two adjacent fields on the opposite phase of the 2-year rotation, which represented the integrated loss of nitrate from field tiles to streams across central Illinois. The experiment was replicated in time with a 9-year period of record (2015–2023) for the 3-year rotation versus an 8-year period of record (2016–2023) for the 2-year rotation. Additionally, this systems comparison study assessed N retention in the predominant cropping system of southern Illinois, investigating the outcome of moving this cropping system north into the intensively tile drained region of central Illinois.
This field study was conducted on a private farm in southern Piatt County, IL and is in Upper Kaskaskia River watershed. The predominant soil types on this farm are Drummer and Flanagan (fine-silty, mixed, superactive, mesic Typic Endoaquolls) containing approximately 4% organic matter and are characterized as poorly drained with 0%–2% slopes (Soil Survey Staff, Natural Resources Conservation Services and USDA, 2024). Based on data collected near Tuscola IL, the 30-year mean annual precipitation is 1041 mm and the mean annual temperature is 11.2°C. Precipitation was measured on site using a Davis Instruments Vantage Pro2 Plus weather station and expressed on a water year basis (October 1-September 30).
Prior to the initiation of this study, the entire farm was either planted to corn or soybean in a corn-soybean rotation with winter wheat planted only the year of tile installation. In the summer of 2014, a pattern tile system was installed, creating a field plot with a drainage area of 30.02 ha (Figure 1). Tiles (10.2 cm in diameter) were installed to an average depth of approximately 1.2 m and lateral tiles were spaced 30.1 m apart throughout the field. The tile outlet discharged to a dredged ditch that divided the farm. This field plot was under the 3-year rotation beginning with corn in 2015. That same year, on the other side of the ditch, three adjacent pattern tile systems were installed with drainage areas of 8.10, 3.16, and 9.70 ha. Tile size, depth, and spacing were the same as the other field and tile outlets discharged into the dredged ditch. By isolating the two smaller tile systems into one field, two distinct field plots were created with drainage areas of 11.26 and 9.70 ha (Figure 1). These field plots were under the conventionally managed 2-year rotation and maintained on the opposite phase of the rotation. Combining the tile data across both phases of the 2-year rotation created a larger drainage area of 20.96 ha for the control treatment, closer in size to the experimental treatment. During the study period, three cycles through the 3-year rotation and four cycles through the 2-year rotation were completed.
Field operations are listed chronologically in Supplementary Tables S1, S2 including dates for crop planting and harvest, cereal rye planting and termination, tillage, and fertilizer applications. The aim was to plant corn and soybean in late April through mid-May, plant winter wheat in early October, and harvest wheat and plant double crop soybean before July 4th. In the 2-year rotation corn was planted in April in 2017 and 2018; however, wet months of May in 2019 and 2020 delayed corn planting until early June. Similar to corn, soybean planting in the 2-year rotation was delayed until June in 2019; however, in 2020 soybean was planted in April before the wet period. In the diverse 3-year rotation, corn was planted in April in 2015 and 2018, and May in 2021. Soybean in the diverse rotation was planted in May in 2016 and 2022; however, planting was delayed until June in 2019. Wheat was planted in early to mid-October. An early wheat harvest on 27 June 2017, allowed for an early double crop soybean planting. Wheat harvest was July 6th and seventh in 2020 and 2023, delaying double crop soybean planting. The 3-year rotation requires greater planning, more equipment initially, and timeliness of field operations.
Cereal rye was planted in October following corn in the 3-year rotation (Supplementary Table S2). Cereal rye was drill-planted at 67 kg ha−1 and terminated in the spring prior to soybean planting in 2016 and 2022; however, in 2019, soybean was planted directly into live cereal rye Cereal rye termination dates were in April of 2016, June of 2019, and May of 2022. Above ground cereal rye biomass was randomly collected from a 0.25 m2 area from nine locations across the field. Samples were dried at 65°C for 48 h and weighed. Cover crop biomass was ground and analyzed for N concentration by combustion analysis (A&L Great Lakes Laboratories, Inc., Ft. Wayne, IN).
Conventionally managed corn in the 2-year rotation received fertilizer N at a rate of 202 kg ha−1 (Supplementary Table S1). Depending on precipitation following crop harvest, fertilizer N was applied in the fall as anhydrous ammonia with a nitrification inhibitor after soil temperatures were less than 10°C at the 10 cm depth. In 2016 and 2017, N application occurred at planting as a starter fertilizer (UAN at 32%) and the remainder at early side-dress (UAN at 32%). In 2018 and 2019, fertilizer N was applied at a 2/3rds rate in the fall with the remainder nearly equally split between the starter fertilizer at planting and at early side-dress. In 2020–2023, fertilizer N was applied at a 2/3rds rate in the fall with the remainder applied at planting. Soybean received no fertilizer N. Overall, both field plots under the 2-year rotation received 808 kg ha−1 over the 8-year period (101 kg ha−1 yr−1).
In the 3-year rotation, fertilizer N was applied to corn at a rate of 202 kg ha-1 and winter wheat at a rate of 134 kg ha−1, whereas soybean received no N (Supplementary Table S2). For corn, starter fertilizer N (22–45 kg ha−1) was applied at planting (UAN at 32%) with the remainder at early side-dress (UAN at 32%). Starter fertilizer N rate was increased over time in the 3-year rotation, decreasing the N rate at side-dress. For wheat, 18–26 kg ha−1 of N was applied with fertilizer phosphorus (P) in the fall while the remainder was split applied (50:50 or 40:60) in March and April. Overall, the field under the 3-year rotation received 1008 kg ha−1 over the 9-year period (112 kg ha−1 yr−1).
For all three study fields, P and potassium (K) fertilizers were co-applied in the fall each year at recommended rates based on the Illinois Agronomy Handbook (University of Illinois, 2021), except for an accidentally omission of fertilizer application in 2019 following corn harvest in the field plots under the 2-year rotation. Fertilizer P was applied as triple super phosphate to corn and soybean; whereas mono-ammonium phosphate was applied to wheat to provide N to the wheat seedling. Fertilizer K was applied as muriate of potash (Supplementary Tables S1, S2).
Conventional tillage in the 2-year rotation consisted of full width tillage either in the spring or fall; however, wet weather limited fall tillage operations in some years (Supplementary Table S1). Conventional tillage implements were either a field cultivator, chisel-plow or disk-chisel. In the 3-year rotation, strip-till occurred before corn either in the fall or early spring; whereas soybean, winter wheat, and double crop soybean were no-till planted (Supplementary Table S2).
As with tillage operations, planting and harvest dates were controlled by the weather, and planting delays occurred during the wet springs of 2018 and 2019, which led to late harvests (Supplementary Tables S1, S2). Crop grain yields were determined using a yield monitor and represent whole field averages. Yield monitor was calibrated using a weigh wagon and moisture meter in the field and measuring grain weight over a predetermined area. Yield maps and field yield averages were determined by PAC Interactive (Monticello, IL). Crop grain yields are presented at 15.5, 13.0, and 14.5% moisture for corn, soybean and winter wheat, respectively.
The herbicide programs for both cropping systems were designed to include multiple modes of action to control the onslaught of herbicide-tolerant weeds (Supplementary Table S3). There were eight different herbicide compounds applied to cover pre-emergence and post-emergence applications to corn and soybean in both the 2-year and 3-year rotations. In addition, the 3-year rotation received glyphosate as a preplant application to terminate the cereal rye cover crop and again as a post-emergence double crop soybean. Winter wheat received only one herbicide compound as a post-emergence application.
Drain tile flow was monitored with a 45° V-notch weir stoplog fitted at the bottom of an in-line water control structures (Agri Drain, Inc., Adair, IA). Water height within the structure was determined using a combination pressure transducer and datalogger (Solinst, Inc., Georgetown, Ontario, Canada). Tile flow rate was calculated using the v-notch weir equation described by Chun and Cooke (2008). Flow was logged on 15-minute intervals and daily average flow was calculated using the 96 logged data points per day. During extreme precipitation events, rising ditch water submerged tile outlets, impeding tile flow. When this occurred, tile flow was manually set to zero until water height in the control structures began to recede. At that point, we assumed a linear increase in tile flow until maximum flow resumed (Li et al., 2020).
Tile nutrient concentrations were determined from 500 mL water samples collected using automated water samplers (ISCO 3700, Teledyne, Lincoln, NE) or from grab samples during periods when air temperatures decreased below 0°C. Samplers were set to sequential time mode on an 8-h basis to capture nutrient concentration changes during tile flow events. If no flow events occurred, tile water samples were collected weekly. Tile water samples were collected from automated samplers within 24 h. Samples were vacuum filtered through a 0.45 um membrane and analyzed for nitrate. Nitrate-N concentrations were measured using ion chromatography (ICS-1600, Dionex, Sunnyvale, CA) with a minimum detection limit of 0.1 mg N L−1. We used linear interpolation to estimate nitrate concentration for each day between tile sampling dates. Annual tile nitrate loads were calculated by multiplying daily average flow by daily nitrate concentration, expressed on a water year basis. Annual flow-weighted mean concentration (FWMC) of nitrate-N was calculated by dividing annual tile load by annual tile flow. Overall FWMC of tile nitrate-N was calculated by summing annual tile loads and dividing by the sum of annual tile flows over the period of record for each cropping systems.
A balanced T-test analysis was conducted to evaluate differences in the average annual tile flow, tile nitrate load, FWMC of nitrate, and crop grain yield between the two conventionally managed field plots under either a corn-soybean or soybean-corn rotation (n = 4) over the 8-year period of record. Without significant differences between the two conventionally managed fields, an annual integrated tile flow, tile nitrate load, and FWMC of tile nitrate was calculated across corn and soybean phases to create the control treatment. The 3-year rotation represented the experimental treatment. T-test analysis was conducted to evaluate differences in tile flow, tile FWMC of nitrate, and nitrate load between the experimental treatment, (n = 9) and the control treatment (n = 8). T-test analysis was conducted on corn and soybean grain yields to evaluate differences between the experimental treatment (n = 3) and the control treatment (n = 8). All analyses were performed using R Statistical Software (v4.2.2; R Core Team, 2022).
Precipitation during the growing season on this farm was sufficient to produce crop yields that equaled or surpassed the county yield estimate each year of this study. In the two conventionally managed field plots, corn grain yields ranged from 13.6 Mg ha−1 in 2020 to 17.0 Mg ha−1 in 2018, averaging 14.7 over the 8 growing seasons (Table 1). Conventionally managed soybean grain yields varied minimally during this same period, ranging from 5.3 Mg ha−1 in 2017 to 5.8 Mg ha−1 in 2016, equaled again in 2018, with an average of 5.5 Mg ha−1.
Table 1. Crop grain yields in both phases of the conventionally managed 2-year rotation and in the 3-year rotation from 2015 through 2023.
There was no comparison between the two rotational cropping systems for corn yield in 2015 as the control treatment was initiated in 2016. Corn grain yields were 2% less in 2018 and 6% greater in 2021 in the 3-year versus the 2-year rotation. Soybean grain yields were 11% and 13% less, and 4% greater, in 2016, 2019, and 2022, respectively, in the 3-year versus the 2-year rotation. Average crop grain yields in the 3-year rotation were 15.9 Mg ha−1 for corn, 5.3 Mg ha−1 for soybean, 6.6 Mg ha−1 for winter wheat, and 2.5 Mg ha−1 for double crop soybean. There were no significant differences between average corn (p > 0.05) and soybean (p > 0.05) yields between the experimental treatment and the control. Double-crop soybean grain yield varied widely and ranged from a low of 1.3 Mg ha−1 due to an early frost versus 3.7 Mg ha−1 when allowed to reach maturity.
Depending on termination date, cereal rye biomass production varied more than four-fold during the study. The late termination on 2 June 2019, produced the greatest amount of above ground cereal rye biomass (5.94 Mg ha−1), with early termination on 16 April 2016, producing the least biomass (1.37 Mg ha−1), and termination on 10 May 2022, producing an intermediate amount (2.38 Mg ha−1) (Table 2). Cereal rye biomass N accumulation ranged from 24 to 46 kg ha−1 representing less than a two-fold increase as biomass N concentration decreased with increasing biomass production, widening the C:N ratio (Table 2). With approximately 43% C, cereal rye biomass added from 0.6 to 2.5 Mg ha−1 of C to the 3-year rotation.
Table 2. Cereal rye biomass and biomass nitrogen in above ground biomass before soybean in the 3-year rotation from 2015 through 2023.
Annual precipitation at this research site ranged from 818 to 1192 mm from 2015–2023, averaging 1012 mm, which was only 3% less than the 30-year average of 1041 mm (Table 3). Compared to the 30-year average, annual precipitation was 15% greater in 2016 and 21% less in 2017. Annual precipitation was characterized as average in 2020, above average in in 2015, 2016, and 2019, and below average in 2017, 2018, 2021, 2022, and 2023.
Table 3. Annual precipitation and annual tile flows in both phases of the conventionally managed 2-year rotation and in the 3-year rotation from 2015 through 2023.
At this site, tile flow most frequently occurred from January through June, averaging 125 days of flow per year; however, with consecutive wet years in 2015 and 2016, tile flow occurred every month in 2016. Generally, years with greater annual precipitation produced greater annual tile flow. An exception was in 2020 when most of the precipitation occurred during the first 6 months, greatly elevating annual tile flow for all three field plots. Combined tile flow from all three field plots accounted for approximately 15% of the annual precipitation during the study period. There were no significant differences for annual tile flow between the two conventionally managed field plots (p > 0.05) (Table 3). Although annual tile flow was less under the diverse 3-year rotation, there was no significant difference between the experimental treatment and the control treatment (p > 0.05) (Table 3).
The pattern of tile nitrate concentration in tiles under the two conventionally managed field plots demonstrated elevated tile nitrate concentrations under corn compared to soybean (Figures 2A, B). Tile nitrate concentrations increased during the spring under corn, whereas under soybean concentrations remained relatively unchanged in 2018, 2020, and 2023. Tile nitrate concentrations were greater than 10 mg L−1 in 35% of the samples and less than 5 mg L−1 in 15% of the samples collected in the 2-year rotation.
Figure 2. (A–C) Pattern of tile nitrate concentrations in both phases of the conventionally managed 2-year rotation and in the 3-year rotation from 2015 through 2023. Water years are separated by vertical lines and crop phases are represented by squares for corn, circles for soybean, and triangles for winter wheat/double-crop soybean.
In contrast, the pattern of tile nitrate concentrations in the 3-year rotation was dissimilar to the pattern observed in tiles under the 2-year rotation (Figure 2C). Tile nitrate was greater than 10 mg L−1 in only 1% of the samples and less than 5 mg L−1 in 70% of the samples in the 3-year rotation. Tile nitrate concentrations decreased under cereal rye each year and under winter wheat in 2023. Following the severe freeze and premature death of the double crop soybean in October of 2020, tile nitrate concentrations were elevated in 2021 (Figure 2C). When tile flow began in March of 2021, the initial tile nitrate concentrations were greater than all other years, gradually increasing to 9 mg L−1 prior to starter fertilizer N application at corn planting. Following side-dress fertilizer N application that year, tile nitrate reached 14 mg L−1 during high tile flow in June causing substantial nitrate loss.
The annual FWMC of tile nitrate in the two field plots in the 2-year rotation ranged from 6.9 to 12.7 mg L−1 under corn and from 3.7 to 8.7 mg L−1 under soybean (Table 4). The overall FWMC of tile nitrate in each field plot over the 8-year period of record was not significantly different (7.7 and 8.3 mg L−1) (p > 0.05). The annual integrated FWMC of tile nitrate across both phases of the 2-year rotation ranged from 5.9 mg L−1–9.9 mg L−1 (Figure 3). The overall integrated FWMC of tile nitrate across both crop phases of the 2-year rotation over the 8-year period of record was 8.0 mg L−1 and represented nitrate loss from the control treatment (Figure 3).
Table 4. Annual tile flow weighted mean nitrate concentrations and annual tile nitrate loads in both phases of the conventionally managed 2-year rotation and in the diverse 3-year rotation from 2015 through 2023.
Figure 3. Annual tile flow weighted mean nitrate concentrations across both phases of the conventionally managed 2-year rotation compared to the 3-year rotation from 2015 through 2023. Horizontal lines represent overall tile flow weighted mean nitrate concentration over three cycles of the diverse rotation and four cycles of the conventional rotation.
In the 3-year rotation annual FWMC of tile nitrate under corn ranged from 4.5 to 10.4 mg L−1 (Table 4). Annual FWMC of nitrate under soybean ranged from 1.9 to 4.1 mg L−1 (Table 4) Annual FWMC of tile nitrate under winter wheat/double crop soybean ranged from 1.9 to 4.7 mg L−1 (Table 4). The overall FWMC of tile nitrate in the 3-year rotation over the 9-year period of record was 4.2 mg L−1, which was significantly less than the control treatment (p < 0.05) (Figure 3).
Annual tile nitrate loads ranged from 5.6 kg ha−1 in 2023 to 27.6 kg ha−1 in 2019 under corn in the 2-year rotation, while tile nitrate loads under soybean ranged from 3.1 kg ha−1–22.5 kg ha−1 in those same years (Table 4). Average annual tile nitrate loads in the 2-year rotation under conventional corn and conventional soybean were 15.1 kg ha−1 and 9.9 kg ha−1 during the 8-year period of record. Average annual tile nitrate loads from the two conventionally managed field plots were not significantly different at 11.7 and 13.4 kg ha−1. The overall average tile nitrate load in the 2-year rotation over the 8-year period of record was 12.5 kg ha−1.
In the 3-year rotation, the greatest tile nitrate load occurred during the wet spring of 2020 under the wheat/double crop soybean phase (Table 4). The second greatest annual load occurred in 2021 following the early freeze of the double crop soybean crop. Annual tile nitrate loads in the 3-year rotation ranged from 2.2 to 12.7 kg ha−1, averaging 4.9 kg ha−1 over the 9-year period of record (Table 4), which was significantly less than the control (p < 0.05) (Figure 3). Note: With the inherent challenges in measuring tile flow; tile nitrate concentration data may better differentiate treatment response.
Growing conditions were favorable to produce above average corn and soybean yields on this farm compared with Piatt County estimates. The average grain yields of conventionally managed corn and soybean were 14.7 Mg ha−1 and 5.5 Mg ha−1 at this site which were 7% and 15% greater than the county yield estimates over the 8-year period of record (Table 1). Piatt County’s corn and soybean yields consistently rank amongst the highest producing counties in Illinois with record corn and soybean yields of 15.2 Mg ha−1 and 5.1 Mg ha−1 in 2018 (USDA National Agricultural Statistics Service, 2022). This county produced the largest soybean yields in Illinois for 3 years in a row during the study period (2020, 2021, and 2022), producing the largest soybean yield for any county in the entire U.S. in 2022 (USDA National Agricultural Statistics Service, 2022). These data clearly indicate that our study site was located in one of the most productive areas in the U.S Corn Belt.
With the predominant cropping system under a corn and soybean rotation in central Illinois, there are few fields under winter wheat production and no county yield estimate. Winter wheat is generally not grown on the productive tile drained soils of central Illinois due to lower economic return compared to corn and soybean (G. Schnitkey, personal communication). However, on sloping lands in southern Illinois, winter wheat is more commonly grown and is often followed by double-crop soybean. With more time to grow wheat and soybean in the same year, this phase of the corn-soybean-wheat rotation can be the most profitable in southern Illinois. At the latitude of this study site, however, producers are aware of the risk of early frost, which can negatively impact double crop soybean yield and profitability. It is interesting to note that the USDA has updated frost-free dates twice in the past 12 years due to recent data trends, moving frost-free zone boundaries approximately 100 km north. With lengthening growing seasons, the 3-year rotation of corn-soybean-wheat/double crop soybean may become more common in the intensively tile drained watershed of central Illinois (USDA National Agricultural Statistics Service, 2022).
Numerous studies (Davis et al., 2012; Gaudin et al., 2015; Bowles et al., 2020) suggest longer more diverse crop rotations may enhance both corn and soybean yields, however, our results were mixed. In general, earlier crop planting dates at this site produced greater grain yields for both corn and soybean. In 2018, the weather supported an extended grain filling period and produced large corn grain yields for both cropping systems that were approximately 10% greater than the record-breaking county estimate. In 2021, corn grain yield was 0.8 Mg ha−1 greater in the 3-year rotation which may have been related to the unexpected N source from the frozen double crop soybean.
Soybean yields in the 3-year rotation were decreased in 2016 and 2019 due to issues related to the cereal rye cover crop. In 2016, soybean planting was delayed 17 days by wet soil conditions in the 3-year rotation following cover crop termination, decreasing yield potential and overall soybean grain yield. In 2019, a large cover crop biomass was produced due to wet conditions preventing timely termination. Without a roller crimper, the soybean crop emerged in standing cereal rye, reducing early plant vigor likely due to the combination of less light interception by the developing crop and greater N immobilization following cover crop senescence (Nevins et al., 2020). In 2022, however, soybean grain yield was greater in the 3-year rotation, which is approximately the amount of yield increase needed to offset the price of establishing and terminating cereal rye before soybean.
Winter wheat grain yields at this site were 32% greater than average winter wheat yields in the southern Illinois region (USDA National Agricultural Statistics Service, 2022). Double-crop soybean grain yield was 67% of the full season soybean yield when allowed to reach maturity while only 25% of the full season soybean yield after the killing freeze. Double-crop soybean planting date is critical, therefore, searching for high yielding wheat varieties that mature several days earlier is an important goal of current wheat breeding programs (J. Rutkowski, personal communications). To maximize yield and profitability of this cropping system, nested microplot studies are being initiated at this site to evaluate wheat varieties and seeding rates for double crop soybean.
The timing of conventional fertilizer N application to corn varies due to convenience and risk for a given farmer (David et al., 2015). In central Illinois, approximately half of the fields under corn receive fertilizer N in the fall (Gentry et al., 2014). Following university guidelines, the application of fall N with a nitrification inhibitor occurred only after soil temperatures were consistently less than 4°C (University of Illinois Agronomy Handbook). Numerous studies report increased tile nitrate loss under fall fertilizer N application (Welch et al., 1971; David et al., 2015; Pittelkow et al., 2017; Gentry et al., 2024). Split-application of fertilizer N has been proposed to reduce tile nitrate loss, improving synchronization of fertilizer application with crop growth (Dinnes et al., 2002). Testing this hypothesis in central Illinois, Gentry et al. (2024) evenly split-applied fertilizer N in the fall and spring and found approximately a 10% reduction in tile nitrate compared to 100% applied in the fall. With more producers split-applying fertilizer N, we chose to split-apply a 2/3rds fertilizer N rate in the fall with the remainder in the spring to represent conventional fertilizer application. Although we used the same total fertilizer N rate for corn in both rotational cropping systems, fall fertilizer N application occurred 6 out of the 8 years in the 2-year rotation, increasing tile nitrate loss. In the 3-year rotation, fertilizer N was split-applied in season for both corn and wheat for improved synchrony between application and uptake. Overall, the 3-year rotation received approximately 10% more fertilizer N than the 2-year rotation (112 vs. 101 kg ha−1 yr−1).
The advent of herbicides has made modern agriculture more efficient and more profitable by reducing reliance on mechanical weed control. Regardless of tillage differences between the cropping systems, herbicide use was similar and can be considered conventional for both rotational cropping systems as corn and soybean as well as the double crop soybean in the diverse rotation required an additional herbicide application of glyphosate. Wheat production required less herbicide application compared to corn and soybean production. Weeds were effectively eliminated from both cropping systems and played no role in experimental outcomes.
With effective herbicidal control of weeds in conventional cropping systems, tillage is a method of managing crop residue, especially for corn; however, soil conditioning is also a consideration as a warm seedbed speeds germination and enhances early crop growth (Licht and Al-kaisi, 2005). Soil disturbance from tillage aerates the soil, energizing soil microorganisms, and stimulating soil N mineralization, which may decrease soil organic C (Randall et al., 1997; Balesdent et al., 2000). Therefore, we hypothesize that tillage has the potential to increase nitrate leaching and tile nitrate loss compared to no-till and reduced tillage systems. In contrast, however, no-till improves soil aggregate stability and soil structure, which increases water infiltration rates and possibly tile nitrate loss.
An interesting phenomenon that has occurred in the 3-year rotation is the return and establishment of earthworms (Lumbricus teresteres L.). Tillage is clearly detrimental to earthworm survivability and no-till systems have been shown to have increased abundance (Kladivko et al., 1997). With precision farming techniques, moving the center of the tilled strip 25 cm each corn year in a 76 cm row width completely tills the entire field every 9 years under the 3-year rotation. Although conventional herbicide practices occurred in both rotational cropping systems, visual inspection indicated worm middens in the 3-year rotation and none in the two conventionally managed fields under corn and soybean, suggesting that full width tillage limited the prevalence of worms rather than herbicide toxicity. Anecdotally, this past spring we observed short-term ponding following an intense precipitation event only in the conventionally managed field plots, suggesting that infiltrations rates are different between the two cropping systems. This study is on-going and water infiltration rate studies are planned to evaluate the influence of earthworms on tile flow and nitrate loss over time.
The conservation technique of adding a cereal rye cover crop after corn in a corn-soybean rotation has been shown to greatly reduce tile nitrate concentrations and loads (Kaspar et al., 2012; Ruffatti et al., 2019; Waring et al., 2020; Gentry et al., 2024). Cereal rye following corn can capture unused fertilizer N and mineralized N during the non-crop growing season that may leach to tiles. Cereal rye biomass and biomass N accumulation was sufficient to decrease tile nitrate each time through the rotation with the greatest reduction in tile nitrate occurring when cereal rye was terminated in early June in 2019. Unlike end-of-pipe nitrate remediation techniques such as wetlands (Kovacic et al., 2000; Tilman et al., 2011), woodchip bioreactors (Christianson et al., 2011; David et al., 2016b), and saturated riparian buffers (Singh et al., 2024) that only treat the symptom of a declining soil C stock (i.e., tile nitrate loss from soil N mineralization), cereal rye adds carbon to the system, directly treating the cause of tile nitrate loss. Management practices that conserve N in the field have a strategic advantage over remediation techniques that return N to the atmosphere via denitrification.
In Illinois cover crops are being adopted on sloping lands and are more easily integrated into animal livestock operations (Plastina et al., 2023), whereas in the flat rich soils of central Illinois there are few livestock animals and less than 5% of the area in cover crops (USDA National Agricultural Statistics Service, 2022). The greatest impetus behind cover crop adoption is preventing soil erosion, while the greatest impediment is cost of cover crop establishment and termination (David et al., 2015; Roth et al., 2018). Cereal rye covers the soil quickly in the spring and is the most widely used cover crop in Illinois due to winter hardiness. Cereal rye is also known to suppress weed germination and growth (Kaspar et al., 2012), potentially offsetting some of the cover crop production cost by reducing herbicide use. Using a roller crimper at the appropriate cereal rye growth stage is an effective termination strategy, creating a thick mat of residue that reduces weed pressure, retains soil moisture, and reduces soil temperature (Mirsky et al., 2011). The additional C input from cereal rye can tie up soil nitrate via microbial N immobilization (McSwiney et al., 2010; Nevins et al., 2020). Simple stoichiometry indicates that to build soil organic matter, soil N will need to increase concomitantly with soil C, therefore, net N immobilization may be the key to soil C sequestration (Cao et al., 2021).
Yu et al. (2024) measured the natural abundance of heavy isotopes in tile water and identified sources as fertilizer N, soil organic matter decomposition, and legacy nitrate with a release lag time of several years. Legacy N may explain the muted tile nitrate response to a reduced fertilizer N rate (75%) treatment in a replicated tile drainage experiment in central Illinois that surprisingly decreased corn yield more than tile nitrate loss (Gentry et al., 2024). Similar to that study, tile nitrate concentrations in the conventionally managed plots were greater under corn than soybean. Tile nitrate concentrations tended to increase through the spring with numerous samples during the corn phase containing greater than the US EPA drinking water standard of 10 mg L−1 nitrate-N, especially during the wet springs of 2019 and 2020. Even though fertilizer N application in the fall was limited to 2/3rds of the full rate, fall N appeared to increase tile nitrate loss (2017 through 2023) compared to years without fall N application (2015 and 2016). Although no fall fertilizer N was applied in the first 2 years of the study, tile nitrate concentrations increased in the spring, suggesting the source was in part from soil N mineralization.
The downward trend in tile nitrate in the spring under cereal rye demonstrated the efficacy of an overwintering grass cover crops to act as a catch crop, absorbing mineralized N as well as legacy N during the non-crop growing season. Winter wheat appeared effective at utilizing fertilizer N, especially in the spring of 2023, when the pattern of tile nitrate concentrations was similar to years under cereal rye. Compared to conventional corn, fertilizer N was spoon-fed to winter wheat with approximately 20% of the fertilizer N applied with the phosphorus fertilizer in the fall and the remainder split applied in the spring. Wheat was actively growing when fertilizer N was applied in the spring, which reduced tile nitrate loss despite the input of fertilizer N and concurrent soil N mineralization following soybean production.
The early freeze in 2020 that reduced double crop soybean yield occurred during mid grain fill (R5 growth stage) when soybean leaves, stems, and nodules contain high N concentrations (Bender et al., 2015). We speculate that organic N from soybean roots and shoots leaked into the soil, nitrified, and leached to tiles during the subsequent spring. Even with the annual FWMC of tile nitrate greater than 10 mg L−1 in 2021, the overall FWMC was only 4.2 mg L−1 in the 3-year rotation, compared to the overall FWMC of tile nitrate of 8.0 mg L−1 in the 2-year rotation, indicating a 50% reduction in FWMC of tile nitrate with the diverse cropping system. This unique long-term drainage study provided proof of concept that a longer more diverse cropping system can reduce tile nitrate loss, while maintaining system productivity.
Average annual tile nitrate loads from the conventionally managed field plots on this farm were less than reported for other tile nitrate studies in central Illinois (David et al., 1997; Royer et al., 2006; Rufatti et al., 2019; Gentry et al., 2024). Annual tile flow in this study may be a conservative estimate due to the assumption that tile flow stopped during high ditch water flow. Similar to Gentry et al. (2024), 60% of the tile nitrate load occurred under corn and 40% under soybean. Annual tile nitrate loads in the 2-year rotation clearly demonstrated that years with below normal precipitation transport the least amount of tile nitrate and tile flow generally ranked in order with annual precipitation. In the 3-year rotation, crop phase had a greater influence on annual tile nitrate load as cereal rye and winter wheat greatly reduced tile nitrate concentrations and loads. Additionally, tile flow tended to cease earlier under winter wheat compared to under corn or soybean, helping to reduce tile nitrate load.
The two wettest years (2019 and 2020) produced the greatest tile nitrate loads under conventionally managed corn as the combination of fall fertilizer N application and wet spring conditions delaying corn planting, exacerbated tile nitrate loads those years (27.6 and 26.2 kg ha−1). The largest annual difference in tile nitrate loads between the two cropping systems occurred in 2019 when cereal rye accumulated 6 Mg ha−1 of above-ground biomass and annual tile nitrate load was only 3.4 kg ha−1, representing a 90% reduction in tile nitrate load. This comparison demonstrates the magnitude of tile nitrate reduction possible in a row crop production system without having to convert to a perennial crop (McIsaac et al., 2010; Heaton et al., 2008).
Using an overwintering grass cover crop to reduce tile nitrate loss is not a new idea as Armsby (1884) suggested using rye to keep growing roots in the soil as extensively as possible to curtail N loss from manured plots. With cereal rye after corn and winter wheat after soybean, the 3-year rotation had living roots 30 out of 36 months versus 10 out of 24 months in the conventionally managed 2-year rotation. Available C is a critical factor in N retention in soils and extra C added into the diverse 3-year cropping systems may tighten the N cycle (McSwiney et al., 2010), sequestering C and reducing tile nitrate loss. Overall, by maintaining crop yields and reducing tile nitrate loss, this study may be the first of its kind to demonstrate sustainable intensification in a high-yielding, dry land production system in the US Corn Belt (Cassman and Grassini, 2020; Heaton et al., 2013; Tilman et al., 2011).
The most challenging aspects of managing the diverse rotation was readiness and timeliness of field operations due to the limited number of days to conduct field work in the spring. The first-time cereal rye was grown following corn the decision not to plant soybean in the 3-year rotation on May 5th (conventional soybean planting date) may have been a costly misstep as it led to reduced yield. The combination of the 17-day planting delay and the use of a shorter season maturity soybean variety may explain the grain yield reduction. Three years later, when wet weather delayed cover crop termination in 2019, soybean was “planted green” and the large amount of cereal rye biomass interfered with both the physical planting operation and early soybean growth (Reed et al., 2019).
Consideration for balancing cover crop biomass production with timely row crop management must be weighed against nutrient loss reduction goals. Using a replicated tile drainage study, Gentry et al. (2024) found that cereal rye biomass accumulation of approximately 1 Mg ha−1 was sufficient to significantly reduce tile FWMC of nitrate, whereas this study indicated that 6 Mg ha−1 may be more than required. Therefore, we recommend producers aim for at least 1 Mg ha−1 of above ground biomass (stem length 15–20 cm) to 6 Mg ha−1 (beginning flowering) to maximize tile nitrate reduction and weed control without decreasing soybean yield.
This study demonstrated winter wheat was efficient at using split-applied fertilizer N and helped reduce the loss of mineralized N following soybean. Winter wheat occupies the space of an overwintering grass cover crop and demonstrated the potential to reduce tile nitrate during its phase of the 3-year rotation. Planting double crop soybean after winter wheat can be highly productive at this latitude, however, early frost greatly reduced soybean yield and increased tile nitrate loss. Interestingly, the subsequent corn crop appeared to be benefitted from this unexpected N source.
Future studies at this research site will focus on refining management practices within the diverse cropping system and documenting economic viability and competitiveness with the conventional 2-year rotation. This study suggests the common rotation of corn-soybean-wheat/double crop soybean in southern Illinois is efficient at retaining N and reducing N loss. Overall, this study demonstrated proof of concept that a more diverse crop rotation can greatly reduce tile nitrate loss from fields to streams, while maintaining crop productivity.
The raw data supporting the conclusions of this article will be made available by the authors, without undue reservation.
LG: Conceptualization, Funding acquisition, Investigation, Writing–original draft. CM: Data curation, Investigation, Writing–review and editing. JG: Investigation, Methodology, Writing–review and editing. CG: Formal Analysis, Investigation, Writing–review and editing. EM: Conceptualization, Investigation, Methodology, Writing–review and editing. DS: Conceptualization, Investigation, Methodology, Writing–review and editing. AV: Investigation, Writing–review and editing. ZY: Funding acquisition, Project administration, Writing–review and editing.
The author(s) declare that financial support was received for the research, authorship, and/or publication of this article. We thank the Illinois Nutrient Research and Education Council (Grant number: 2015-5-360350-374) for their generous continuous support of this long-term research project.
The authors declare that the research was conducted in the absence of any commercial or financial relationships that could be construed as a potential conflict of interest.
The author(s) declare that no Generative AI was used in the creation of this manuscript.
All claims expressed in this article are solely those of the authors and do not necessarily represent those of their affiliated organizations, or those of the publisher, the editors and the reviewers. Any product that may be evaluated in this article, or claim that may be made by its manufacturer, is not guaranteed or endorsed by the publisher.
The Supplementary Material for this article can be found online at: https://www.frontiersin.org/articles/10.3389/fenvs.2025.1506113/full#supplementary-material
Armsby, H. P. (1884). Loss of nitrogen from arable soils. Science 3, 17–19. doi:10.1126/science.ns-3.48.17
Baldwin-Kordick, R., De, M., Lopez, M. D., Liebman, M., Lauter, N., Marino, J., et al. (2022). Comprehensive impacts of diversified cropping on soil health and sustainability. Agroecol. Sustain. Food Syst. 46 (3), 331–363. doi:10.1080/21683565.2021.2019167
Balesdent, J., Chenu, C., and Balabane, M. (2000). Relationship of soil organic matter dynamics to physical protection and tillage. Soil Tillage Res. 53, 215–230. doi:10.1016/S0167-1987(99)00107-5
Basche, A. D., Archontoulis, S. V., Kaspar, T. C., Jaynes, D. B., Parkin, T. B., and Miguez, F. E. (2016). Simulating long-term impacts of cover crops and climate change on crop production and environmental outcomes in the Midwestern United States. Agric. Ecosyst. Environ. 218, 95–106. doi:10.1016/j.agee.2015.11.011
Behnke, G. D., Kim, N., and Villamil, M. B. (2020). Agronomic assessment of cover cropping and tillage practices across environments. Agron. J. 112 (5), 3913–3928. doi:10.1002/agj2.20337
Bender, R. R., Haegele, J. W., and Below, F. (2015). Nutrient uptake, partitioning, and remobilization in modern soybean varieties. Agron. J. 107, 563–573. doi:10.2134/agronj14.0435
Bowles, T. M., Mooshammer, M., Socolar, Y., Calderón, F., Cavigelli, M. A., Culman, S. W., et al. (2020). Long-term evidence shows that crop-rotation diversification increases agricultural resilience to adverse growing conditions in North America. One Earth 2 (3), 284–293. doi:10.1016/j.oneear.2020.02.007
Cao, Y., He, Z., Zhu, T., and Zhao, F. (2021). Organic-C quality as a key driver of microbial nitrogen immobilization in soil: a meta-analysis. Geoderma 383, 114784. doi:10.1016/j.geoderma.2020.114784
Cassman, K. G., and Grassini, P. (2020). A global perspective on sustainable intensification research. Nat. Sustain. 3, 262–268. doi:10.1038/s41893-020-0507-8
Chun, J. A., and Cooke, R. A. (2008). Technical note: Calibrating Agridrain water level control structures using generalized weir and orifice equations. Appli. Enginee. Agricu. 24 (5), 595–602. doi:10.13031/2013.25274
Christianson, L. E., Bhandari, A., and Helmers, M. J. (2011). Pilot-scale evaluation of denitrification drainage bioreactors: reactor geometry and performance. J. Environ. Eng. 137, 213–220. doi:10.1061/(ASCE)EE.1943-7870.0000316
Ciampitti, I. A., and Salvagiotti, F. (2018). New insights into soybean biological nitrogen fixation. Agron. J. 110, 1185–1196. doi:10.2134/agronj2017.06.0348
Clark, J. D., Fernandez, F. G., Camberato, J. J., Carter, P. R., Fergusun, R. B., Franzen, D. W., et al. (2020). Weather and soil in the US Midwest influence the effectiveness of single- and split-nitrogen applications in corn production. Agron. J. 112 (6), 5288–5299. doi:10.1002/agj2.20446
Coulter, J. A., Nafziger, E. D., and Wander, M. M. (2009). Soil organic matter response to cropping system and nitrogen fertilization. Agron. J. 101, 592–599. doi:10.2134/agronj2008.0152x
Crandall, S. M., Ruffo, M. L., and Bollero, G. A. (2005). Cropping system and nitrogen dynamics under a cereal winter cover crop preceding corn. Plant Soil 268 (1), 209–219. doi:10.1007/s11104-004-0272-x
David, M. B., Drinkwater, L. E., and McIsaac, G. F. (2010). Sources of nitrate yields in the Mississippi River basin. J. Environ. Qual. 39, 1657–1667. doi:10.2134/jeq2010.0115
David, M. B., Flint, C. G., Gentry, L. E., Dolan, M. K., Czapar, G. F., Cooke, R. A., et al. (2015). Navigating the socio-bio-geo-chemistry and engineering of nitrogen management in two Illinois tile-drained watersheds. J. Environ. Qual. 44, 368–381. doi:10.2134/jeq2014.01.0036
David, M. B., Gentry, L. E., Cooke, R. A., and Herbstritt, S. M. (2016a). Temperature and substrate control woodchip bioreactor performance in reducing tile nitrate loads in east-central Illinois. J. Environ. Qual. 45, 822–829. doi:10.2134/jeq2015.06.0296
David, M. B., Gentry, L. E., Kovacic, D. A., and Smith, K. M. (1997). Nitrogen balance in and export from an agricultural watershed. J. Environ. Qual. 26, 1038–1048. doi:10.2134/jeq1997.00472425002600040015x
David, M. B., Mitchell, C. A., Gentry, L. E., and Salemme, R. K. (2016b). Chloride sources and losses in two tile-drained agricultural watersheds. J. Environ. Qual. 45, 341–348. doi:10.2134/jeq2015.06.0302
Davis, A. S., Hill, J. D., Chase, C. A., Johanns, A. M., and Liebman, M. (2012). Increasing cropping system diversity balances productivity, profitability and environmental health. PLoS One 7, e47149. doi:10.1371/journal.pone.0047149
Dinnes, D. L., Karlen, D. L., Jaynes, D. B., Kaspar, T. C., Hatfield, J. L., Colvin, T. S., et al. (2002). Nitrogen management strategies to reduce NO3 leaching in tile-drained midwestern soils. Agron. J. 94, 153–171. doi:10.2134/agronj2002.0153
Gaudin, A. C. M., Tolhurst, T. N., Ker, A. P., Janovicek, K., Tortora, C., Martin, R. C., et al. (2015). Increasing crop diversity mitigates weather variations and improves yield stability. PLoS One 10, e0113261. doi:10.1371/journal.pone.0113261
Gentry, L. E., Below, F. E., David, M. B., and Bergerou, J. A. (2001). Source of the soybean N credit in maize production. Plant Soil 236, 175–184. doi:10.1023/A:1012707617126
Gentry, L. E., David, M. B., Below, F. E., Royer, T. V., and McIsaac, G. F. (2009). Nitrogen mass balance of a tile-drained agricultural watershed in east-central Illinois. J. Environ. Qual. 38, 1841–1847. doi:10.2134/jeq2008.0406
Gentry, L. E., David, M. B., and McIsaac, G. F. (2014). Variation in riverine nitrate flux and fall nitrogen fertilizer application in East-Central Illinois. J. Environ. Qual. 43, 1467–1474. doi:10.2134/jeq2013.12.0499
Gentry, L. E., David, M. B., Smith, K. M., and Kovacic, D. A. (1998). Nitrogen cycling and tile drainage nitrate loss in a corn/soybean watershed. Agric. Ecosyst. Environ. 68, 85–97. doi:10.1016/S0167-8809(97)00139-4
Gentry, L. E., Green, J. M., Mitchell, C. A., Andino, L. F., Rolf, M. K., Schaefer, D., et al. (2024). Split fertilizer nitrogen application with a cereal rye cover crop reduces tile nitrate loads in a corn-soybean rotation. J. Environ. Qual. 53 (1), 90–100. doi:10.1002/jeq2.20530
Gentry, L. F., Ruffo, M. L., and Below, F. E. (2013). Identifying factors controlling the continuous corn yield penalty. Agron. J. 105, 295–303. doi:10.2134/agronj2012.0246
Green, C. J., and Blackmer, A. M. (1995). Residue decomposition effects on nitrogen availability to corn following corn or soybean. Soil Sci. Soc. Am. J. 59, 1065–1070. doi:10.2136/sssaj1995.03615995005900040016x
Hanrahan, B. R., King, K. W., and Stinner, J. H. (2024). Nitrogen balances and losses in conservation cropping systems across a tile-drained landscape in Ohio, United States. J. Soil Wat. Conserv. 79 (3), 145–154. doi:10.2489/jswc.2024.00055
Heaton, E. A., Dohleman, F. G., and Long, S. P. (2008). Meeting US biofuel goals with less land: the potential of Miscanthus. Glob. Chang. Biol. 14, 2000–2014. doi:10.1111/j.1365-2486.2008.01662.x
Heaton, E. A., Schulte, L. A., Berti, M., Langeveld, H., Zegada-Lizarazu, W., Parrish, D., et al. (2013). Managing a second-generation crop portfolio through sustainable intensification: examples from the USA and the EU. Biofuels, Bioprod. Biorefining 7 (6), 702–714. doi:10.1002/bbb.1429
Janovicek, K., Hooker, D., Weersink, A., Vyn, R., and Deen, B. (2021). Corn and soybean yields and returns are greater in rotations with wheat. Agron. J. 113, 1691–1711. doi:10.1002/agj2.20605
Kaspar, T. C., Jaynes, D. B., Parkin, T. B., Moorman, T. B., and Singer, J. W. (2012). Effectiveness of oat and rye cover crops in reducing nitrate losses in drainage water. Agric. Water Manage. 110, 25–33. doi:10.1016/j.agwat.2012.03.010
Kazula, M. J., and Lauer, J. G. (2018). The influence of crop rotation on corn total biomass production. J. Soil Water Conserv. 73 (5), 541–548. doi:10.2489/jswc.73.5.541
Kladivko, E. J., Akhouri, N. M., and Weesies, G. (1997). Earthworm populations and species distributions under no-till and conventional tillage in Indiana and Illinois. Soil Biol. Biochem. 29, 613–615. doi:10.1016/s0038-0717(96)00187-3
Kovacic, D. A., David, M. B., Gentry, L. E., Starks, K. M., and Cooke, R. A. (2000). Effectiveness of constructed wetlands in reducing nitrogen and phosphorus export from agricultural tile drainage. J. Environ. Qual. 29, 1262–1274. doi:10.2134/jeq2000.00472425002900040033x
Li, Z., Chu, M. L., Gentry, L., Li, Y., Mitchell, C., and Arai, Y. (2020). Passive detection of phosphorus in agricultural tile waters using reactive hybrid anion exchange resins. Water 12, 2808. doi:10.3390/w12102808
Licht, M. A., and Al-Kaisi, M. (2005). Strip-tillage effect on seedbed soil temperature and other soil physical properties. Soil Tillage Res. 80, 233–249. doi:10.1016/j.still.2004.03.017
McIsaac, G. F., David, M. B., and Mitchell, C. A. (2010). Miscanthus and switchgrass production in central Illinois: impacts on hydrology and inorganic nitrogen leaching. J. Environ. Qual. 39, 1790–1799. doi:10.2134/jeq2009.0497
McSwiney, C. P., Snapp, S. S., and Gentry, L. E. (2010). Use of N immobilization to tighten the N cycle in conventional agroecosystems. Ecol. Appl. 20, 648–662. doi:10.1890/09-0077.1
Mirsky, S. B., Curran, W. S., Mortensen, D. A., Ryan, M. R., and Shumway, D. L. (2011). Timing of cover crop management effects on weed suppression in no-till planted soybean using a roller crimper. Weed Sci. 59, 380–389. doi:10.1614/WS-D-10-00101.1
Mitchell, J. K., McIsaac, G. F., Walker, S. E., and Hirschi, M. C. (2000). Nitrate in river and subsurface drainage flows from an east central Illinois watershed. Trans. ASAE 43, 337–342. doi:10.13031/2013.2709
Nevins, C. J., Lacey, C., and Armstrong, S. (2020). The synchrony of cover crop decomposition, enzyme activity, and nitrogen availability in a corn agroecosystem in the Midwest United States. Soil Tillage Res. 197, 104518. doi:10.1016/j.still.2019.104518
Nichols, V., English, L., Carlson, S., Gailans, S., and Liebman, M. (2020a). Effects of long-term cover cropping on weed seedbanks. Front. Agron. 2, 591091. doi:10.3389/fagro.2020.591091
Nichols, V., Martinez-Feria, R., Weisberger, D., Carlson, S., Basso, B., and Basche, A. (2020b). Cover crops and weed suppression in the US Midwest: a meta-analysis and modeling study. Agric. Environ. Lett. 5 (1), e20022. doi:10.1002/ael2.20022
Peralta, A. L., Sun, Y., McDaniel, M. D., and Lennon, J. T. (2018). Crop rotational diversity increases disease suppressive capacity of soil microbiomes. Ecosphere 9, e02235. doi:10.1002/ecs2.2235
Pittelkow, C. M., Clover, M. W., Hoeft, R. G., Nafziger, E. D., Warren, J. J., Gonzini, L. C., et al. (2017). Tile drainage nitrate losses and corn yield response to fall and spring nitrogen management. J. Environ. Qual. 46, 1057–1064. doi:10.2134/jeq2017.03.0109
Plastina, A., Acharya, J., Marcos, F. M., Parvej, M. R., Licht, M. A., and Robertson, A. E. (2023). Does grazing winter cereal rye in Iowa, USA, make it profitable? Renew. Agric. Food Syst. 38, e45. doi:10.1017/S1742170523000388
Preza-Fontes, G., Pittelkow, C. M., Greer, K. D., Bhattarai, R., and Christianson, L. E. (2021). Split nitrogen application with cover cropping reduces subsurface nitrate losses while maintaining corn yields. J. Environ. Qual. 50 (6), 1408–1418. doi:10.1002/jeq2.20283
Qin, Z., Guan, K., Zhou, W., Peng, B., Villamil, M. B., Jin, Z., et al. (2021). Assessing the impacts of cover crops on maize and soybean yield in the U.S. Midwestern agroecosystems. Field Crops Res. 273, 108264. doi:10.1016/j.fcr.2021.108264
Randall, G. W., Huggins, D. R., Russelle, M. P., Fuchs, D. J., Nelson, W. W., and Anderson, J. L. (1997). Nitrate losses through subsurface tile drainage in conservation reserve program, alfalfa, and row crop systems. J. Environ. Qual. 26, 1240–1247. doi:10.2134/jeq1997.00472425002600050007x
Randall, G. W., and Vetsch, J. A. (2005). Nitrate losses in subsurface drainage from a corn–soybean rotation as affected by fall and spring application of nitrogen and nitrapyrin. J. Environ. Qual. 34, 590–597. doi:10.2134/jeq2005.0590
R Core Team (2022). R: a language and environment for statistical computing. Vienna: R Foundation for Statistical Computing. Available at: https://www.R-project.org/.
Reed, H. K., Karsten, H. D., Curran, W. S., Tooker, J. F., and Duiker, S. W. (2019). Planting green effects on corn and soybean production. Agron. J. 111, 2314–2325. doi:10.2134/agronj2018.11.0711
Rocha, L. F., Pimentel, M. F., Bailey, J., Wyciskalla, T., Davidson, D., Fakhoury, A. M., et al. (2021). Impact of wheat on soybean cyst nematode population density in double-cropping soybean production. Front. Plant Sci. 12, 640714. doi:10.3389/fpls.2021.640714
Roth, R. T., Ruffatti, M. D., O'Rourke, P. D., and Armstrong, S. D. (2018). A cost analysis approach to valuing cover crop environmental and nitrogen cycling benefits: a Central Illinois on farm case study. Agric. Syst. 159, 69–77. doi:10.1016/j.agsy.2017.10.007
Royer, T. V., David, M. B., and Gentry, L. E. (2006). Timing of riverine export of nitrate and phosphorus from agricultural watersheds in Illinois: implications for reducing nutrient loading to the Mississippi River. Environ. Sci. Technol. 40, 4126–4131. doi:10.1021/es052573n
Ruffatti, M. D., Roth, R. T., Lacey, C. G., and Armstrong, S. D. (2019). Impacts of nitrogen application timing and cover crop inclusion on subsurface drainage water quality. Agric. Water Manage. 211, 81–88. doi:10.1016/j.agwat.2018.09.016
Ruffo, M. L., Bullock, D. G., and Bollero, G. A. (2004). Soybean yield as affected by biomass and nitrogen uptake of cereal rye in winter cover crop rotations. Agron. J. 96, 800–805. doi:10.2134/agronj2004.0800
Sawyer, J., Nafziger, E., Randall, G., Bundy, L., Rehm, G., and Joern, B. (2006). Concepts and rationale for regional nitrogen rate guidelines for corn. Publ. PM 2015. Ames: Iowa State Univ.
Schnitkey, G., Zulauf, C., Swanson, K., Paulson, N., Baltz, J., and Coppess, J. (2022). Projected wheat double-crop soybean profitability in 2023. Champaign: Department of Agricultural and Consumer Economics, University of Illinois at Urbana-Champaign, 126. farmdoc daily (12).
Shrestha, R. K., Richer, E., Clevenger, W. B., Davis, M., and Lindsey, L. E. (2021). Effect of mono-relay-and double-crop systems on yield and profitability. Agron. J. 113, 1747–1757. doi:10.1002/agj2.20598
Silva, T. S., Mourtzinis, S., McMechan, A. J., Carmona, G. I., Potter, B. D., Tilmon, K. J., et al. (2024). Cereal rye cover crop termination at or before soybean planting has minimal effect on soybean yield across the midwestern US. Field Crops Res. 312, 109393. doi:10.1016/j.fcr.2024.109393
Singh, G., Davis, M., Nelson, K. A., and Kaur, G. (2024). Drainage water management, woodchip bioreactor, and saturated riparian buffer as stacked conservation practices for improving crop yields and water quality. Environ. Technol. Innovation 36, 103779. doi:10.1016/j.eti.2024.103779
Soil Survey Staff, Natural Resources Conservation Services, and USDA (2024). Web soil survey Available at: https://websoilsurvey.sc.egov.usda.gov.
Tilman, D., Balzer, C., Hill, J., and Befort, B. L. (2011). Global food demand and the sustainable intensification of agriculture. Proc. Natl. Acad. Sci. U. S. A. 108 (50), 20,260–20,264. doi:10.1073/pnas.1116437108
University of Illinois (2021). Illinois Agronomy Handbook. Dep. Crop Sci. Univ. Ill. A. T. Urbana–Champaign. Available at: http://extension.cropsci.illinois.edu/handbook/(Accessed September 13, 2024).
USDA National Agricultural Statistics Service (2017). NASS - quick stats. USDA national agricultural Statistics Service. Available at: https://data.nal.usda.gov/dataset/nass-quick-stats.
USDA National Agricultural Statistics Service (2022). NASS – Census of agriculture. USDA national agricultural Statistics Service. Available at: https://www.nass.usda.gov/Publications/AgCensus/2022/#full_report.
Vonk, J., Nafziger, E. D., and Preza-Fontes, G. (2024). Soybean response to nitrogen fertilizer in different soils. Crop Forage Turfgrass Mgmt 10, e20304. doi:10.1002/cft2.20304
Waring, E. M., Lagzdins, A., Pederson, C., and Helmers, M. J. (2020). Influence of no-till and a winter rye cover crop on nitrate losses from tile-drained row-crop agriculture in Iowa. J. Environ. Qual. 49, 292–303. doi:10.1002/jeq2.20056
Welch, L. F., Mulvaney, D. L., Oldham, M. G., Boone, L. V., and Pendleton, J. W. (1971). Corn yields with fall, spring, and side-dress nitrogen. Agron. J. 63 (1), 119–123. doi:10.2134/agronj1971.00021962006300010037x
Keywords: tile drainage, nitrate, diverse crop rotation, cover crop, corn, soybean, winter wheat, doublecrop soybean
Citation: Gentry LE, Mitchell CA, Green JM, Guacho C, Miller E, Schaefer D, Vasquez AK and Yu Z (2025) A diverse rotation of corn-soybean-winter wheat/double crop soybean with cereal rye after corn reduces tile nitrate loss. Front. Environ. Sci. 13:1506113. doi: 10.3389/fenvs.2025.1506113
Received: 04 October 2024; Accepted: 06 January 2025;
Published: 11 February 2025.
Edited by:
Keith Schilling, The University of Iowa, United StatesReviewed by:
Yansheng Li, Chinese Academy of Sciences (CAS), ChinaCopyright © 2025 Gentry, Mitchell, Green, Guacho, Miller, Schaefer, Vasquez and Yu. This is an open-access article distributed under the terms of the Creative Commons Attribution License (CC BY). The use, distribution or reproduction in other forums is permitted, provided the original author(s) and the copyright owner(s) are credited and that the original publication in this journal is cited, in accordance with accepted academic practice. No use, distribution or reproduction is permitted which does not comply with these terms.
*Correspondence: Lowell E. Gentry, bGdlbnRyeUBpbGxpbm9pcy5lZHU=
†ORCID: Carlos E. Guacho, orcid.org/0009-0005-2324-2728
Disclaimer: All claims expressed in this article are solely those of the authors and do not necessarily represent those of their affiliated organizations, or those of the publisher, the editors and the reviewers. Any product that may be evaluated in this article or claim that may be made by its manufacturer is not guaranteed or endorsed by the publisher.
Research integrity at Frontiers
Learn more about the work of our research integrity team to safeguard the quality of each article we publish.