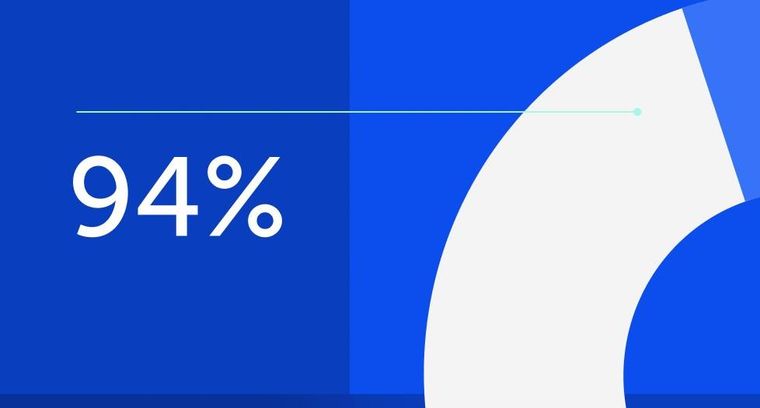
94% of researchers rate our articles as excellent or good
Learn more about the work of our research integrity team to safeguard the quality of each article we publish.
Find out more
SYSTEMATIC REVIEW article
Front. Environ. Sci., 07 April 2025
Sec. Soil Processes
Volume 13 - 2025 | https://doi.org/10.3389/fenvs.2025.1495991
This article is part of the Research TopicSoil Carbon Sequestration and Microbial Energy MetabolismView all 5 articles
Increasing soil organic carbon (SOC) in cropland soils improves soil health and water quality, crop productivity, and resilience to climate-driven changes by influencing key soil processes. However, research on cropland SOC sequestration based on bibliometric analyses of highly cited documents is lacking. This bibliometric study investigated the current status and development characteristics, research impact, intellectual base, and research hotspots of highly cited cropland SOC sequestration research using the Web of Science Core Collection databases from 2012 to 2022. The analysis and visualization tools such as Biblioshiny, VOSviewer, CiteSpace, Power BI, and Flourish Studio, provided a comprehensive approach for research evaluation, identifying trends, and knowledge mapping of cropland SOC sequestration research. The findings indicate that the United States and China dominate global research, with the Chinese Academy of Sciences as the leading institution. Key journals include Soil Biology and Biochemistry and Geoderma. e. The trend topic graph indicated that “soil organic carbon” and “soil organic matter” are the most persistent themes since 2015. In contrast, recent research focuses on “climate change mitigation,” “soil health” and “soil aggregation”. Moreover, burst analysis of citation and keywords revealed significant insights into the potential role of microbes in transforming and stabilizing soil organic matter. The findings of the present study emphasized that adopting management practices aimed at enhancing carbon inputs increases C sequestration in croplands, thus improving soil health and help in advancing Sustainable Development Goals (SDGs).
Global atmospheric CO2 concentrations have increased by 50% since pre-industrial times compared with current atmospheric CO2 concentrations (Pro Oxygen, 2024). It is projected that the CO2 concentration will reach 936 ppm (Representative Concentration Pathways-RCP 8.5) by 2,100 (Canadell et al., 2023). The ongoing increase in atmospheric CO2 concentration is driving climate change and has implications for global warming and the natural carbon cycle (Canadell et al., 2023). Climate change poses a severe threat to natural ecosystems and societies worldwide, disrupting ecosystem services (Priya et al., 2023), biodiversity (Wernberg et al., 2024), human health (Bowen et al., 2024), and agricultural productivity (Waqas et al., 2019), therefore threatening global food security. Thus, mitigating climate change has emerged as a significant ecological and societal challenge for protecting natural ecosystems.
Soils represent the largest terrestrial carbon sink, comprising distinct pools of soil organic carbon (SOC) and soil inorganic carbon (SIC). Enhancing soil organic carbon (SOC) sequestration in terrestrial ecosystems is a key strategy for mitigating climate change by removing atmospheric CO2 (Don et al., 2024). Global SOC stocks are estimated at 1,500–1,600 Pg to 1-m depth and 2,376–2,456 Pg to 2 m depth (Batjes and Sombroek, 1997; Monger and Martinez-Rios, 2000; Lal, 2004b; Batjes, 2014). However, when considering deeper profiles, the total global SOC stock may reach nearly 3,000 Pg (Köchy et al., 2015), exceeding the combined atmospheric and vegetation carbon pools. Even small changes in SOC can substantially impact the carbon cycle and atmospheric CO2 levels (Minasny et al., 2017; Friedlingstein et al., 2022), which has implications for natural ecosystems and biodiversity.
Climatic changes (e.g., warming) and anthropogenic land use changes for agriculture can stimulate the loss of C from soils, accelerating the rise in atmospheric CO2 (Crowther et al., 2016; Melillo et al., 2017; Sanderman et al., 2017). The management of soils for SOM accumulation is therefore considered a viable option to mitigate climate change and maintain soil fertility (Paustian et al., 2016; Minasny et al., 2017). The United Nations Framework Convention on Climate Change (UNFCCC) defines “carbon sinks” as processes, activities, or mechanisms that remove carbon dioxide from the atmosphere (UNFCCC, 2024). Hence, various C sequestration projects were initiated across countries to enhance C accrual in soils including 4 per mille (France), Conservation Reserve Program Initiative (USDA, 2021), 4 per 1,000 initiative (Minasny et al., 2017), United Kingdom Research and Innovation (UKRI)’s Greenhouse gas removal (GGR) initiative (UKRI, 2021), and RECSOIL-Recarbonization of global soils (ITPS, 2021) etc.
Cropland soils offer a significant potential for enhanced carbon sequestration to mitigate climate change. Adopting sustainable practices, such as conservation tillage, crop rotation, and organic amendments, can promote SOC accumulation, allowing croplands to act as carbon sinks and offset anthropogenic emissions. Several global and regional studies demonstrate this potential, with estimates of 0.90–1.85 Pg C ha−1 in global soils (Janzen et al., 2022); 4.53–4.98 Pg C ha−1 sequestration in Chinese croplands (Zuo et al., 2023); 2.22 Pg C ha−1 in Australian croplands (Viscarra Rossel et al., 2024), 0.34 Pg C ha−1 in Russian croplands (Romanenkov et al., 2024). While natural factors such as climate, biophysics, and landscapes are dominant drivers of SOC variability (Batjes, 2016; Hengl et al., 2017; Moinet et al., 2023), human intervention through land use and management can dramatically alter terrestrial carbon over shorter timescales (Hansis et al., 2015; Bastos et al., 2021). For instance, balanced mineral fertilization application exhibits a potential for SOC sequestration of 0.01–0.03 Pg C per year over no fertilization in cropland in China. In contrast, balanced mineral fertilization integrated with organic amendments has the potential to sequester 0.04–0.06 Pg C per year (Waqas et al., 2020). However, there is a lack of comprehensive global understanding of cropland carbon sequestration research foci, key players, and knowledge gaps. Globally, cropland SOC decreased by 0.2 per 1,000 GtC per year from 1975 to 2010 relative to potential vegetation, although absolute stocks increased from 705 to 712 GtC (Lal, 2016). Regional trends vary, with SOC losses in cropland expansion areas, such as Brazil, and gains in some abandoned croplands (Sanderman et al., 2017). Tropical croplands tend to have reduced relative SOC, whereas irrigated drylands show increased SOC stocks (Ghimire and Khanal, 2020). Understanding global research trends and literature exploration of cropland carbon sequestration is crucial for developing effective mitigation strategies.
Since the 1980s, the United States has led research endeavors on cropland carbon sequestration, followed by other countries. Bibliometric analysis can quantitatively and qualitatively assess temporal trends across scientific fields and be used to identify, organize, and parse the key components of a scientific domain (Vaz et al., 2017; Mourao and Martinho, 2020), and decipher the development trends of a research field (Albort-Morant et al., 2017).
Despite extensive research on cropland carbon sequestration, nevertheless, bibliometric analyses of highly-cited documents on C sequestration in global croplands have not yet been reported. This bibliometric study provides a detailed assessment of the global research landscape by determining major actors, research areas, and gaps. The specific objectives of this study include: 1. Analyze the fundamental attributes of the literature, such as article quantity, citations, research classifications, and prominent journals. 2. Evaluate the research’s impact by investigating key countries, institutions, and authors, and recognize the intellectual foundation of the research. 3. Reveal research topics, emerging trends, and future research possibilities, while also examining the association between the Sustainable Development Goals (SDGs) and cropland organic carbon (SOC) sequestration in the most cited literature. We hypothesized a significant increase in this research in recent years, focusing on sustainable management practices to enhance soil carbon storage in croplands. By elucidating the current state and future directions, this study can inform researchers, policymakers, and stakeholders, guiding future efforts to optimize climate change mitigation strategies through C sequestration in cropland soils.
Bibliometric analysis is an effective tool for quantifying scientific advancement across multiple disciplines and has become a robust approach for investigating the literature (Wong et al., 2020), and a useful technique that can evaluate global research patterns from various standpoints such as active and productive countries, institutions, authors, and journals, and aims to provide a glean foresight into navigating prospective research (Liu et al., 2011). Thomson Reuters developed the Web of Science (WoS) database, covering about 264 key disciplines, including Science (178), Social science (58), and Arts, and Humanities (28). It is the most effective search engine and the most relevant database for retrieving scientific and bibliometric data (Analytics, 2017).
The query returned 42,808 records, of which we initially selected 403 highly cited articles. After a rigorous review, we found 261 irrelevant documents and excluded them from further analysis. Finally, 142 records were included in the further analysis. Given this, the Digital Object Identifiers (DOIs) were extracted from the Web of Science data and a query consisting of 142 DOIs was formulated and searched in Web of Science and downloaded data used for statistical analyses. A new query consisting of similar DOIs was formulated and executed using the Scopus database. The records of 142 search results were downloaded from Web of Science and Scopus for analysis. The following search query was entered in the Web of Science search box on 20 January 2023 (AB= (“Soil organic carbon” OR “Soil organic matter” OR “cropland Carbon sequestration*” OR “cropland C storage*”) OR TI= (“Soil organic carbon” OR “Soil organic matter” OR “cropland Carbon sequestration*” OR “cropland C storage*”) OR AK= (“Soil organic carbon” OR “Soil organic matter” OR “cropland Carbon sequestration*” OR “cropland C storage*”).
This study employed bibliometric techniques to appraise research productivity and its impact on C sequestration in cropland. The study mainly focused on highly cited documents (including research articles and review articles) published in the Web of Science and Scopus databases. We carefully identified the relevant keywords, excluded irrelevant keywords, and finalized them to formalize the search query. This study used a variety of software tools, including Microsoft (MS) Access and MS Excel (365) for data processing, while Biblioshiny, VOSviewer, CiteSpace, Microsoft Power BI, and Flourish Studio were used for data analysis and visualization to obtain the co-occurrence network, intellectual base, and research hotspots, to provide a foundation for interpreting the research conducted in this field and helping scholars to carry out a prospective research agenda. Bibliometrix is an open-source platform for performing a comprehensive mapping analysis of the scientific literature. It provides a set of tools for quantitative research in bibliometrics and scientometrics. It can facilitate data collection, data analysis (descriptive analysis of a bibliographic data frame, network creation for bibliographic coupling, co-citation, collaboration, and co-occurrence analyses), and data visualization (conceptual structure and Network mapping). Biblioshiny is an interactive web-based graphical user interface (GUI) for Bibliometrix that enables users to explore bibliometric data and carry out relevant bibliometric and visual analyses of citation networks, keyword trends (keywords cloud map, co-occurrence network, and tree map), scientific productivity (at country and continent scales), timeline visualization, and thematic mapping and evolution of research topics (Aria and Cuccurullo, 2017). A chord diagram was employed to assess the relationships among the top countries. Co-occurrence analysis was used to assess the association between the keywords used in publications co-authored to determine research topic evolution and research trends (Andrade et al., 2019). Moreover, in this study, keyword cluster analysis was carried out to determine the primary cluster for cropland carbon sequestration research trends by reviewing selected research articles in the period (2012–2022). The VOSviewer is a computer-based tool developed by van Eck and Waltman (van Eck and Waltman, 2010) to create a clear mapping of different author keywords and system-supplied keywords (Keywords Plus) by parsing the bibliometric data connections and demonstrating them in the form of separate clusters for visualizing bibliometric networks including journals, individual articles, and researchers based on citations, co-citation, and co-authorship patterns. CiteSpace is a Java-based application for the analysis and data visualization of trends and patterns in research literature. It is developed to visualize prospective knowledge domains (Chen, 2004). In the current study, CiteSpace (ver.6.2. R6) software was employed to analyze and visualize the top keywords and documents, and research hotspots using citation burst analysis based on Kleinberg’s algorithm (Kleinberg, 2002). The parameter settings in CiteSpace can be found in the Supplementary Materials. Microsoft Power Business Intelligence (BI) is an integrated, scalable desktop-based data visualization package tool designed by Microsoft that focuses mainly on self-service and enterprise business intelligence. For the current study, we visualize a thematic diagram and four-field Sankey plot using Power BI. Flourish Studio is a powerful online data visualization tool. This platform can be employed to construct a wide range of both static and dynamic visualizations such as bar charts, scatter plots, network graphs, etc. (https://flourish.studio/). In the current study, it was used to analyze the number of publications and citations. Additionally, frequency analysis of author keywords is essential to assist the authors in understanding the research hotspots in cropland carbon sequestration. Moreover, author keyword co-occurrence analysis also helps to construct and visualize connections between distinct research networks. Furthermore, coalescing keyword co-occurrence analysis and frequency of author keywords is a valuable approach for developing an effective comprehension of the evolution of the research topics and proposing prospective research avenues.
First, this study assessed the growth pattern of highly cited research articles on carbon sequestration in croplands (i.e., research output) and identified the citation rate, usage, and age of the documents. The research assessment in this investigation comprised (i) publication output in terms of the number of published papers, total citations (TC), and usage (U1 and U2) between 2012 and 2022, (ii) highly cited documents on a global scale (iii) burst analysis of the top 11 documents based on citations, (iv) research output of the top 10 countries, (v) research productivity of the top 10 most productive institutions (v) the analysis of the top 10 authors, and the top 10 most productive journals, vi) the analysis of author keywords (including keywords cloud, co-occurrence network, and treemap of top ten author keywords), and citation burst analysis of top eight author keywords vii) timeline visualization of trend topics, and thematic map of Author keywords from 2012 to 2022. The impact factors (IF) were retrieved from the Journal Citation Reports (JCR) published in 2022. Total citations (TC) and the average number of citations per paper (TC/TP) were employed to assess different sources such as countries, institutions, authors, and journals. The Hirsch index (h-index) indicates that the author and/or journal has published h papers, and each paper has been cited at least h times. Various statistical tests were employed, including Pearson correlation analysis (used to measure the degree of relationship between two variables), an independent T-test or Student’s t-test (used to compare two sample means from unrelated groups), and an analysis of variance (ANOVA) (used to determine differences between research results from three or more unrelated samples or groups) to respond to the following questions:
If there is any significant correlation between the total citations (TC) of Scopus and the Web of Science and the age of the publications?
Is there any significant difference in securing TC between funded and non-funded publications indexed in the Web of Science?
Whether there is any significant difference in securing TC between open and non-open-access journals listed in the Web of Science?
Is there any considerable difference in TC between collaborative and non-collaborative research?
Is there any significant effect caused by the publication age on TC?
The finally refined 142 records relevant to carbon sequestration in croplands from 2012 to 2022 included five document types: article, article (early access), article (proceeding paper), review, and review (book chapter). Among documents, the “articles” were found to be the dominant type (total records = 109), followed by a review of 30 documents. Each of the remaining types contributed to only one record. Furthermore, there were 48 sources in total, encompassing journals. The average document Age was 5.75 years; the average number of citations per document was 270.5, and the total references cited in these documents were 10,717.
The trends and patterns of articles related to cropland carbon sequestration (between 2012–2022) provided by the Web of Science (WOS) database are shown in Figure 1, including the total citations (TC), article impact (i.e., TC/TP), and two types of usage (U1 and U2). Overall, 142 most-cited articles attracted a total of 38,407 (Web of Science) citations from 41 countries. The most publications (TP = 20) related to cropland carbon sequestration occurred in 2019, followed by 2021 and 2020. On average, over 3 years (from 2018 to 2022), 18.67 (60%) of highly cited documents were published in different journals, in contrast to those articles (average = 11.16) published during the initial 5 years (2012–2017). Given this, there were two turning points pertinent to the articles published: one was observed in 2019 when twenty articles were published for the first time, and the other one was in 2021 when 19 articles related to cropland carbon sequestration were published in 1 year. During the same period (2019–2021), the average annual publication volume exceeded 18 articles. This increasing number of research articles demonstrates that research on cropland carbon sequestration is in its growing state with a greater developmental scope in the future. Moreover, the citation-wise analysis ranked 2015 at the top position with 7,422 citations, followed by 2014 (6,596 citations), and 2013 (4,384 citations). During the initial 6 years (2012–2017), the total count was 29,485 citations (77% of total citations of 38,407), and 8,922 (23% of 38,407). Figure 1 also shows another effective index, article usage reports (U1: usage count in the last 180 days, and U2 stands for usage count since 2013), which inform the authors about how often their articles have been accessed (in terms of page views and downloads), and by which countries. The year 2021 had the highest U1 (2,117), followed by 2019 (U1 = 1884), and 2015 (U1 = 1,435). In contrast, 2015 had the highest U2 (13,156), followed by 2019 and 2014.
Figure 1. Line graph shows the total published articles (TP), Red-colored bars show the total citations (TC); Purple bars show usage 1 (U1): usage from the last 180 days; pink-coloured bars show usage 2 (U2) between period (2012–2022).
Table 1 shows the top ten highly cited global documents published in the respective journals, including their citation count (TC) and impact TC per year (TC/Year) from 2012 to 2022. The manuscript entitled “Total carbon and nitrogen in the soils of the world” authored by Batjes (Batjes, 2014) and published in the European Journal of Soil Science had the highest number of TC = 4,358, as well as the highest impact (544.75), followed by the article written by Lehmann and Kleber, (2015), published in Nature, which received 2,506 citations in 2022 with an impact of 358. The next two articles are “The Microbial Efficiency-Matrix Stabilization (MEMS) framework integrates plant litter decomposition with soil organic matter stabilization: do labile plant inputs form stable soil organic matter?” and “The knowns, known unknowns and unknowns of sequestration of soil organic carbon” written by Cotrufo et al. (2013) and Stockmann et al. (2013), published in Global Change Biology and Agriculture, Ecosystem and Environment received 1963 and 1,401 citations, respectively. The last listed document “The importance of anabolism in microbial control over soil carbon storage” authored by “Liang et al. (2017)” was published in Nature Microbiology, cited 902 times until 2022, with an impact of 180.40.
Citation burst analysis reflects data that tract special attention during a specific period (Kleinberg, 2002). Therefore, it can highlight robust developments from multiple perspectives. Table 2 shows the list of the top 11 documents that fulfilled the criteria of the citation burst analysis. The manuscript “SOM genesis: microbial biomass as a significant source” authored by Miltner et al. (2012) and published in Biogeochemistry Journal (from 2015 to 2017) showed the highest strength (4.11), followed by “The Microbial Efficiency-Matrix Stabilization (MEMS) framework integrates plant litter decomposition with soil organic matter stabilization: do labile plant inputs form stable soil organic matter?” authored by Cotrufo et al. (2013) published in “Global Change Biology.” The third article “The contentious nature of soil organic matter “Lehmann and Kleber (2015)” with the highest citation burst (3.81) from 2015 to 2018 was published in “Nature” journal. The last article “Climate-smart soils” Paustian et al. (2016) with the highest citation bursts, with a strength of 2.50, which was published in Nature.
Figure 2A shows the ranking of the top 10 countries actively involved in cropland carbon sequestration, based on their relative contribution in producing research publications. China is on the top with a frequency of 22 documents, followed by the United State (20) and Germany (9), while countries like Australia, Belgium, Canada, and the Netherlands produced six documents each. At the continental scale, Europe represents maximum research productivity (39%), followed by Asia (28%) and North America-20% (Figure 2B). Meanwhile, Figure 2C shows that documents from the United States of America attracted the highest total citations (TC = 13,219), followed by China (TC = 4,888), the Netherlands (3,774), and Germany (TC = 3,446). The minimum TC count was observed in Sweden (778) and Canada (658). Although contributing only six publications, the Netherlands still showed the highest average article citations (AAC = 944), followed by Sweden (389), the United States (378), and the United Kingdom (357). Switzerland (216), and China (169) follow other countries in terms of AAC (Figure 2D).
Figure 2. (a) Scientific Production of top-ten countries based on the frequency of the published highly cited articles; (b) the Continental scientific productivity in terms of highly cited publications (c) Total citations by the top-ten countries; (d) Average article citations (AAC) by the top-ten countries.
Inter-country collaboration is vital amid the growing phenomenon of globalization and the development of scientific communication (Pohl, 2020). Collaboration is driven by the reciprocity of scientific understanding, resources, skills, and proficiency among investigators (Vieira et al., 2022). A chord diagram shown in Figure 3A depicts the countries’ collaboration in publishing research related to cropland C sequestration. The circle is divided into different coloured chords representing countries, with the arc length of each country scaled to the respective contribution in frequency. The United States appears as the top collaborative country, as it collaborated with a maximum of 23 countries, followed by France and the United Kingdom, which collaborated with 16 and 14 countries, respectively. Additionally, Germany (11), China (10), and Australia (9) are among the top ten highly collaborative countries that collectively collaborated with 30 countries.
Figure 3. (a) shows the collaboration among different countries of the world. Different coloured chords represent different countries. The figure shows collaboration among 27 countries contributing highly cited documents. (b) Relative share of top-ten countries in terms of single-country publications (SCP) and multi-country publications (MCP).
The principal approach for interpreting the global distribution and development of a research domain is to assess national/regional collaboration, publication indices, and so on. The top 10 countries based on corresponding publications are shown in Figure 3B, where multiple-country publication (MCP) denotes the number of papers co-authored by researchers from different nations and single-country publication (SCP) represents the number of articles co-authored by those authors belonging to the same nation or country. These findings imply that the authors prioritize collaboration with researchers from other countries. Among the top 10 countries, the United States of America (United States of America) is leading all countries by publishing 19 SCP and 16 MCP, followed by China, which accounts for 11 SCP and 20 MCP; Germany contributed 12 documents (3 SCP; 9 MCP), Australia contributed 9 documents (SCP = 5; MCP = 4), United Kingdom contributed 6 documents (SCP = 3; MCP = 3), whereas Switzerland and Brazil contributed 4 documents (SCP = 0, MCP = 4), and 3 (SCP = 1; MCP = 2), respectively.
Approximately 362 global institutes have produced highly cited research on cropland C sequestration. Table 3 shows the top ten most productive institutes out of 362 institutes belonging to different countries, among which “The Chinese Academy of Sciences-CAS (China), topped the list in terms of TP, contributing 20 documents on cropland carbon sequestration with 4,213 citations with an impact (TC/TP) of 211. The first document related to cropland C sequestration was published in 2013 by researchers from CAS. This was followed by two French institutes: the French Research Universities (UDICE) and the French National Research Institute for Agriculture, Food, and Environment (INRAE), with 14 publications each, and TC of 2,724 and 2,673, respectively. Similarly, two American universities, namely, Colorado State University and the University of California, contributed 13 documents each. The University of Paris Saclay and the European Commission Joint Research Centre were placed at the bottom of the table, which contributed 10 documents each on cropland C sequestration research with TC of 2,603 and 1,411, respectively. Three out of the top ten institutions belong to the United States, which collectively produces 26% of research related to cropland C sequestration, with relatively higher research impact (TC/TP) values (Mean = 337) over other countries (Mean TC/TP = 206). Overall, the top 10 institutions produced 89% of highly cited research articles on cropland C sequestration.
Figure 4 shows the collaboration networks among global institutions that work on cropland carbon sequestration. There are a total of 6 clusters with 44 participating institutes. Cluster 1 (red color) was led by Colorado State University (total link strength, TLS = 43), and included Agriculture and Agri-Food Canada (TLS = 18), Swiss Federal Institute of Technology, University of Aberdeen, Northern Arizona University, University of California (UC) Davis, UC Santa Barbara, University of Massachusetts, University of New Hampshire, University of Wisconsin-Madison, University of Kassel, University of Paris Saclay, and Wageningen University and Joint Research Center. Cluster 2 (green) was led by Georg August University of Gottingen and included the Institute of Applied Ecology-CAS, University of Chinese Academy of Sciences, RUDN University, Institute of Geographical Science and Natural Resources, Institute of Physico-chemical and Biochemistry, Institute of Soil Science, Institute of Subtropical Agriculture-CAS, and University of Chinese Academy of Sciences. Cluster 3 (green) was led by Cornell University, Ohio State University, University of Sydney, Nanjing Agricultural University, University of Western Australia, Rothamsted Research, and Wageningen University and Research. Cluster 4 is dominated by Stanford University, including Oregon State University, University of California (UC) Berkeley, Stockholm University, the Physical and Life Science Directorate, Indiana University, and Yale University. Cluster 5 (color) was led by the Technical University of Munich, followed by the University of Bonn, the UFZ Helmholtz Center of Environment, and the Catholic University of Louvain. Cluster 6 (purple) is dominated by the Institute of Soil and Water Conservation-CAS, the Ministry of Water Resources, and Northwest Agriculture and Forestry University.
The authors and their collaborations are the key components of a scientific domain. By analyzing parameters such as total publications/documents (TP), total citations (TC), g-index, h-index, research impact (RI) i.e., TC/TP, and co-occurring author networks, we can conclude which authors show maximum productivity, and collaborate and cooperate closely in this field and show teamwork in their academic research. Moreover, based on co-citation analysis, we can identify the main researchers within a scientific community notably influencing a certain research field. Table 4 presents the top ten most productive authors of 786 authors in total, engaged in cropland carbon sequestration research. The other categories included in Table 4 are TC, h-index, g-index, TC/TP, and the publication year (PY). The h-index, as suggested by Hirsch, (2005), is a widely accepted research metric, which determines the productivity and impact of an author’s research output. The g-index was proposed by Egghe, (2006) as an improvement of the previously suggested h-index, which was mainly focused on highly cited papers. The results indicate that the researchers “Kuzyakov Y” (from the University of Gottingen, Germany) and “Lal R” (from Ohio State University, United States) published an equal number of publications (10) and secured the top positions, respectively. Interestingly, the researcher “Lal R” secured one citation more than “Kuzyakov Y.” Likewise, “Cotrufo MF” (from the United States), and “Van Wesemael B” (from Belgium) contributed an equal number of publications (TP = 7), but the former obtained more citations (TC = 2,877). The researcher “Lehmann J″ published only five documents related to cropland C sequestration but maintained the top position in securing citations among the top ten productive authors. Angers DA, Kogel-Knabner I, Lehmann J, and Minasny B contributed five documents related to cropland C sequestration research.
Authorship research also aims to conduct a descriptive bibliometric evaluation of the authorship patterns, expressing the attributes of the authors and authorship of the document and the strength of collaboration pertinent to a specific team of authors. Figure 5A illustrates the authorship patterns in cropland carbon sequestration research ranging from single-authored (AU-1) to sixty-two-authored (AU-62) patterns. The findings indicate a close collaborative research pattern, which suggests that the 3-authored pattern (AU-3) appears to be the most dominant, with the maximum number of publications (i.e., 19; 13.38%), followed by the AU-6 and AU-4 patterns, which contributed 17 (11.97%) and 16 (11.27%) documents, respectively. Both AU-2 and AU-5 published 15 documents. Collectively these six aforementioned authorship patterns contributed about 82 documents (58%) out of a total of 142 documents. Meanwhile, based on TC, the analysis concluded the two-author pattern (AU-2) first spot with 15 highly cited publications and 6,181 citations, succeeded by a four-author pattern (AU-4). Moreover, the data ranked AU-3 (TC = 4,832) and AU-5 (TC = 4,082) as the next two dominant patterns (each contributing to 15 documents). The single author pattern (AU-1) produced eight publications with 4,003 citations and remained in fifth place in the graph. The twenty-eighth-author pattern (AU-28) remained last, producing only one article and 68 citations.
Figure 5. (a) Authorship pattern indicating publications and citations by different combination of authors; AU1–AU62: Authorship pattern showing different authors included in each document; TP, total publications; TC, total citations (b) Collaboration network among different authors from different institutions and countries; Different colored dotted ellipses showing different clusters.
The network map of international cooperation among major authors working on cropland C sequestration research, which the authors ascribed based on the strength of their relationships, the size of the circle, whereas the label of an item related to the weight of the item (i.e., number of publications), and the line connecting the circles represents links. The distance between two authors in the visualization approximately depicts the relatedness of the authors in terms of co-citation links. In Figure 5B, six clusters are shown: the first cluster (blue) was dominated by Lehmann J (26), followed by Kogel-Knabner and Amelung W, and included Amundson, Berhe, Crowe, Doetterl, Liang, Mueller, and Schimel. Cluster 2 (red color) was dominated by Van Wesemael B (total link strength-TLS = 30) and included Montanarella L, Cotrufo MF, Don A, Haddix MI, Panagos P, Six J, Stevens A, and Van Wiesmeir. The third cluster (yellow color) contains eight authors, which were led by Minasny B (TLS = 36), Angers DA, McBratney, Arrouays D, Darrien D, Field DJ, and Paustian K. The fourth cluster (purple) was headed by Chenu C and included Smith P, Sousanna JF, Wollenberg E, Lal R, and Ciais P. Cluster 5 (sky blue) was led by Kuzyakov Y and included Blagodastkaya E, Ge TD, and Wu JS. Cluster 6 (brown) consisted of Bradford MA, Sanderman J, and Sokol NW.
Journals are the most important sources of scientific productivity. The main focus and research importance of a particular field can be identified by analyzing the distribution of relevant journals. The 142 selected articles on cropland carbon sequestration were published in 48 different journals. Table 5 shows the top ten most productive journals publishing research related to cropland carbon sequestration. Approximately 87 documents (61%) were published in the top ten journals. The journal “Soil Biology & Biochemistry” (IF 2022 = 9.7) appeared as the most productive journal that published 21 research articles (14.78%) related to cropland C sequestration, followed by “Geoderma” (IF 2022 = 6.1) (15 articles; 10.56%), and “Global Change Biology” (IF 2022 = 11.6) (14 articles; 9.86%), and “Soil & Tillage Research” which contributed 11 articles. Meanwhile, the citation analysis ranked the journal “Global Change Biology” at the top as it secured the highest number of citations (5,207), followed by “Soil Biology & Biochemistry” (4,629), and “Geoderma” (3,244). The journal “PLOS ONE” remains at the bottom of the list. The table also presents the h-index, g-index, and publication starting year (PY) of the top ten journals related to cropland carbon sequestration research. The highest RI values were shown by Nature (625), followed by Agriculture, Ecosystem, and Environment (509), however, Soil & Tillage Research showed the lowest value of TC/TP = 124.55 (Table 5). The journal with the next highest RI value (371.93) is Global Change Biology, followed by New Phytologist (291.5), Nature Geoscience (288.33), and Proceedings of the National Academy of Sciences of the United States of America-PNAS (254.25).
In scientific journals, keywords are crucial for providing insights into comprehending the research focus and domain trends, as well as identifying research gaps (da Cruz et al., 2021; Liu et al., 2024). The frequency analysis of keywords is crucial for exploring important and evolving topics and key issues related to a given field (Wang et al., 2018). Moreover, selecting high-frequency keywords is usually considered an important research theme for bibliometric analysis and research (Chen et al., 2023). Figure 6a–c presents the word cloud, co-occurrence network, and tree map of the 63 author-supplied keywords. The size of the words depicts the frequency of the usage of keywords by the authors. The word cloud (as shown in Figure 6a) presents the ranking of author-supplied keywords based on their frequency. Out of the total author keywords, “soil organic carbon” (with a frequency of 90; 20%) remained on the top. The “soil organic matter” (frequency = 64; 15%) is in the second spot followed by “soil carbon” (frequency = 54; 12%). “Agriculture” with a frequency of 23% and 5% placed at the last spot in the treemap. Fig. S6a-c presents a word cloud, co-occurrence network, and a treemap of system-supplied keywords (i.e., keyword plus) from the Web of Science. The graph highlights nitrogen (frequency: 28, 15%), sequestration (frequency: 24, 13%), and matter (frequency: 22, 12%) were found to be the most frequent keywords. Additionally, organic matter, climate change, dynamics, and stabilization were the most frequently used system-supplied keywords (keywords plus).
Figure 6. (a) Author keywords cloud (b) Co-occurrence network of author keywords (c) Treemap of the top ten author keywords with frequency and percentage of occurrences.
Burst detection is a valuable analytical method for identifying keywords that have attracted attention from related scientific communities over a certain period (Zhou et al., 2018). Hence, bursting keywords can be utilized as indicators to investigate research frontiers and predict research trends (Li and Chen, 2017; Hou et al., 2018). The time frame 2012–2022 is plotted horizontally on a blue line, and the period of a burst keyword is plotted on a red line, which indicates the beginning and end of the time interval of each burst (Zhou et al., 2018; Mehmood et al., 2023). Table 6 shows the top seven keywords out of all the author keywords and keywords plus based on the citation burst analysis, along with the strength and occurrences. Based on the strength, the studies related to the active theme “soil organic matter’ show a maximum burst strength = 3.51, followed by “turnover” (burst strength = 3.18) during the time frame (2012–2022).
The systematic mapping of the research themes facilitates the identification of scientific interests and temporal evolution, offering valuable insights into understanding future research directions. The timeline visualization/trend topic plot for cropland carbon sequestration, representing the periodic frequency of terms, from 2012 to 2022 (Figure 7A). The graph describes clusters of documents on global cropland C sequestration from left to right and the year of publication at the bottom. The graph arranged the topics vertically, showing the old topics at the bottom and the most recent topics at the top. The bubble size depicts the frequency of each term, where larger and smaller bubbles indicate higher and lower frequencies, respectively. Topics at the top of the diagram include “soil fertility”, “carbon storage”, “soil organic carbon”, and “climate change. Topics of “soil quality”, “nitrogen”, “decomposition”, and “soil carbon” were placed at the bottom. The keyword “soil organic carbon” emerged as the most frequently used keyword and remained a constantly important topic, followed by the keyword ‘‘greenhouse gas emissions” during the years 2016 and 2021. This trend analysis underpins emerging and perpetually important research areas and provides guidelines for researchers and policymakers.
Figure 7. (a) Temporal visualization of top trending topics (Author Keywords) from 2012 to 2022. The horizontal axis (x-axis) shows the timeline (in years) from 2012 to 2022 while the vertical axis (y-axis) shows the terms as trend topics. (b) Thematic map of Author keywords from 2012 to 2022. (c) Co-citation network of carbon dynamics and climate research (2012–2022), showing key clusters such as carbon sequestration (#0) and soil organic matter (#1). Node size represents citation frequency, link thickness indicates co-citation strength, and colors reflect publication years from 2012 (blue) to 2022 (red). .
Figure 7B represents four quadrants: the first quadrant depicts niche themes with lesser value or importance, however, they have a higher degree of development. The second quadrant represents popular topics with greater importance and evolution, while the third and fourth quadrants represent declining/emerging and basic topics, respectively. As shown in Figure 7B, the first and third quadrants contain no clusters, while the second and fourth quadrants contain two clusters each. The principle cluster is far from centrality-lies in the fourth quadrant (containing basic themes) compared with its counterpart which is near to centrality axis. These two clusters are led by “soil organic carbon” and “soil organic matter”, other themes are “soil carbon”, and “climate change”. The principle cluster near centrality is dominated by “mineralization”, “priming effect”, and “enzyme activity”.
Supplementary Figure S1 illustrates the thematic evolution of cropland carbon sequestration research as a two-field Sankey diagram. It divides the evolution into two distinct periods, i.e., 2012–2018, and 2019–2022. It is evident from the graph that the theme “soil organic matter” has a total occurrence of 103, and evolved as “soil organic carbon” with a total occurrence of 42 during 2019–2022. The theme ‘soil organic matter’ further evolved into themes such as ‘nitrogen’ (occurrence = 31), “microbial turnover” (occurrence = 9), and “decomposition” (occurrence = 21). While, the theme “soil organic carbon” evolved into “soil organic carbon” (occurrence = 46), “nitrogen” (occurrence = 13), and “agricultural management” (occurrence = 3) (Supplementary Table S1). As shown in Supplementary Figure S2, the keywords on cropland C sequestration were separated into eight clusters, and each cluster only presents the top three ranked keywords in terms of frequency. The seven clusters were mainly concentrated on motor themes and emerging or declining themes. “Stabilization,” “decomposition,” and “carbon” form the main cluster, followed by the cluster containing “management” microbial biomass” and matter fractions. The themes, ‘‘particle-size fractions” “black carbon” and “agricultural management” lie in the first quadrant near the cross-section of density and centrality. The themes of ‘‘bulk density” “spatial prediction” and “patterns” lie in the second quadrant. In the third quadrant, the themes, i.e., “long-term application,” “cattle manure,” and “physical properties” are relatively closer to the axis of relevance degree (centrality) than the themes “moisture” “sensitivity,” and “texture” which lie in the same quadrant. The themes ‘‘nitrogen” “sequestration” and “matter” lie in the fourth quadrant comprising basic themes.
Factorial analysis, which is a data reduction technique that includes various analyses such as corresponding analysis (CA), multiple corresponding analysis (MCA), and multidimensional scaling (MDS), is used to identify and understand the subfields of a research area. We used MCA coupled with the clustering of keywords, which were distinguished by four different colored areas. We set the minimum number of author keywords to ten. Supplementary Figure S5 visualizes the conceptual and substantial structure of the words that often appear in journals and papers on cropland C sequestration by mapping to connect the words. Author Keywords were placed according to Dimension-1 (44.7%), and Dimension-2 was 15.4%. The map shown in Supplementary Figure S5a, b is divided into three parts: blue, red, and green. The blue area focuses on conservation agricultural practices, such as organic amendments, including organic manure/animal manure, green manure, cover crops, and conservation tillage practices to improve soil health and crop yields, thereby increasing carbon sequestration and achieving climate change mitigation goals. The red area or cluster reflects the importance of SOM and its interaction with the microbial community and the role of microbes in biological and chemical processes in the soil, particularly related to microbial activity, SOM turnover and decomposition, and nutrient cycling. This further highlights the importance of soil organic matter and its interactions with microbial communities. The small green cluster presents themes relevant to the soil fractions and organic matter associated with the soil mineral matrix. The words closely confined to each other imply their close association with each other and their co-occurrence in the papers, and vice-versa (Figure 6).
A four-field Sankey plot is a visualization approach employed to demonstrate flows or relationships between four different categories or groups of data. Figure 8 shows four categories: i) author keywords, ii) journals, iii) countries, and iv) publication year. The thickness of the data flow shows the number of documents joining the top ten keywords to journals, countries, and publication years. The keywords “Soil organic carbon (occurrence = 108)”, and “soil organic matter” (frequency = 97) mainly occurred in “Soil Biology and Biochemistry,” “Global Change Biology,” and “Geoderma” journals. The next highly occurred keywords “Soil Carbon” and “Carbon sequestration” appeared 12 and 14 times in Geoderma and Soil Biology & Biochemistry journals, respectively, implicating these journals are the key platforms for publishing cropland C sequestration research. The middle-right column highlights the geographic distribution of research contributions, with countries such as the United States, China, Germany, and the United kingdom playing dominant roles in cropland C sequestration. Notably, the United States and China are among the leading contributors, accounting for author keywords frequency of 144 out of 609 in 2019, followed by China (100), and Germany (72), in 2021, and 2019, respectively. Moreover, the United States and China are the leading countries publishing in these journals with high impact such as “Nature Communications” and “PNAS.” In summary, this Sankey diagram comprehensively outlines the interrelationships between research themes, publication platforms, country-wise distribution, and temporal trends in cropland C sequestration research. The analysis also underscores a notable expansion in scholarly attention to this field especially during the years 2019–2021.
Figure 8. Four-Field Sankey diagram showing the relationship between Author keywords, Journals, Countries, and Publication year.
Figure 7C shows the progression as a timeline visualization from blue (2012) to red (2022), indicating key research trends, clusters of research, and influential themes at a time scale in the research domain of carbon sequestration in croplands. The network comprises 235 nodes and 494 edges, indicating correlations between articles and their citations. The clusters represent foundational and emerging topics in carbon sequestration. The largest cluster, containing 173 nodes, emphasizes a highly-cited and dominant research theme, i.e., carbon sequestration. A network density of 0.018 reflects the multidisciplinary nature of the field. Metrics such as the Weighted Mean Silhouette Score (0.9531) and Modularity Q (0.7778) demonstrate well-defined clusters and good network structure (Chen, 2004). Key clusters include #0 carbon sequestration, #1 soil organic matter, #2 land use, #3 priming effect, #4 climate change, #5 nitrogen fertilizers, and #6 microbial biomass carbon, revealing thematic focuses ranging from carbon storage strategies and soil health to land-use impacts and microbial contributions to carbon cycling. In summary, this visualization presents a comprehensive, evolving landscape of carbon sequestration in croplands research, with a major focus on carbon sequestration, soil organic matter, land use impacts, and microbial contributions to carbon cycling. The network provides insight into research interconnections, emerging topics, and foundational studies that are shaping contemporary research directions.
This study analyzed the correlation between the total citations (TC) of documents indexed in Scopus and Web of Science and revealed a significant correlation (r = 0.834; p < 0.01) between them. The study further explored the correlation of total citations (WOS), and the age or usage (U1 and U2) of the publications. The analysis revealed a significant strong correlation (p = 0.51) between the total citations secured by the publications indexed in the Web of Science and the age of the publications (Supplementary Figure S3). The study further applied a T-test to investigate the discrepancy in attracting citations between funded and non-funded publications (listed in WOS). The results show no clear difference in securing citations between funded and non-funded publications indexed in Web of Science (F = 0.146, significance = 0.703). It is intriguing to note that non-funded publications secured more citations than funded publications (Supplementary Table S2).
Similarly, another independent T-test was applied between open- and non-open-access journals indexed in the WOS showing a significant difference in means in terms of securing citations between open- and non-open-access journals (F = 6.195, Significance = 0.014; Supplementary Table S2). The table shows the ANOVA for the means of the TC within collaborative and non-collaborative research. Interestingly, the findings showed no significant difference in TC between collaborative and non-collaborative research (F = 2.593, Sig. = 0.078). The LSD multiple comparisons, as shown in Supplementary Table S2, demonstrate that non-collaborative research (single-author research) significantly differs from research published due to international collaboration. A linear regression model was applied considering publication age and TC as the independent and dependent variables to check the effect of publication age on the citations. The regression value (R2 ≥ 0.29; Supplementary Table S3) shows a considerable effect of publication age on TC, showing that TC increases with an increase in age (Supplementary Table S3; Supplementary Figure S4).
Carbon sequestration in croplands presents a dual landscape of challenges and opportunities. Challenges arise mainly from technical, economic, and environmental constraints. Precise measurement of soil organic carbon (SOC) is complex due to its variability regarding soil texture, soil depths, soil types, and management practices, which complicates validation for carbon credits (Baert et al., 2024). Soil degradation, erosion, and the adverse effects of intensive farming practices, such as monocropping, tillage operations, and overuse of chemical fertilizers, further diminish the carbon storage potential of croplands (Jat et al., 2022; Lessmann et al., 2022; Ellis and Swan, 2024). Moreover, climate variability, including extreme weather events, reduces SOC stability and undermines the C sequestration strategies (Wang et al., 2022; Tan and Kuebbing, 2023). Economically, the initial costs of adopting sustainable practices, limited access to carbon markets, and insufficient policy support hinder widespread implementation, particularly for smallholder farmers (Bhatnagar et al., 2024). Knowledge gaps exacerbate these barriers, as most farmers are devoid of the expertise and proper resources to implement carbon-accrual practices effectively (Hughes, 2024).
Despite these challenges, significant opportunities exist to enhance carbon sequestration on croplands. Regenerative agricultural practices such as agroforestry, cover cropping, and conservation tillage can substantially increase SOC content while providing co-benefits like improved water retention, soil fertility, soil structure, and biodiversity (Ellis and Swan, 2024; Nazir et al., 2024). Advances in technologies, including digital soil mapping, remote sensing (drones, satellites, etc.), and the use of soil sensors and employing data analytics and machine learning (including random forest plots), offer tools to optimize sequestration strategies and improve monitoring (Mandal et al., 2022; Razzaghi, 2025; #16; Figure 7C). Policy innovations, such as carbon markets and payment-for-ecosystem-services schemes, can incentivize farmers to adopt sustainable practices (Ingram et al., 2025; Rausser et al., 2025). Ameliorating degraded croplands and integrating bioenergy with carbon capture and storage (BECCS) systems also offer the potential for achieving net-negative emissions (Wu and Pfenninger, 2023). Optimizing strategies to local conditions and aligning policy frameworks with sequestration goals can amplify the efficacy of these opportunities, positioning croplands as vital contributors to global carbon mitigation endeavors (Oldfield et al., 2024).
It is well agreed that Sustainable Development Goals (SDGs) and innovative management practices can be directly linked to achieving improved soil health and productivity. With climate change becoming increasingly pronounced, it is more challenging for agriculture to reduce its net emissions and attain net-zero emissions. Acknowledging the possible challenges and potential implications of the changing climate, the United Nations General Assembly (UNGA) approved an “Agenda-2030 for Sustainable Development,” in September 2015. This comprehensive framework outlines a set of objectives and targets to achieve sustainable development with a focus on economic stability and environment protection, while also addressing various disparities still existed between developed and developing nations. About 193 UN Member countries have agreed to this agenda (UN). Food security mainly relies on healthy and productive soils and the ecosystem services they offer, especially in rural or agriculture-reliant areas. Maintaining soil health enhances crop productivity, supports human livelihoods, and underpins progress toward achieving the SDGs (Pereira et al., 2018). However, global food systems are associated with various unsustainable agricultural management practices, contributing to higher greenhouse gas emissions, land degradation, and loss of biodiversity. Therefore, storing atmospheric CO2 as organic carbon in cropland soils is considered an effective land-based strategy for climate change mitigation-SDG13 (Keesstra et al., 2016), addressing food insecurity (Lal, 2016), and reversing land degradation, which is a major threat to food security (Amelung et al., 2020). Additionally, this soil management approach offers co-benefits including nutrient and water conservation, which facilitates climate change adaptation and minimizes the risk of land degradation-SDG-12 (Lal et al., 2021).
Figure 9, Table 7 shows that the relevant SDGs aligned with C sequestration in croplands can be categorized into three main topics: i) Topic 1-People-centric SDGs (SDGs 1–3), ii) Topic 2-Planet-centric SDGs iii) Topic 3-Prosperity-centric SDGs. Topic 1-People-centric SDGs are among the most important goals essential for sustaining human life globally, as these goals are aimed at addressing poverty-related issues. ii) Topic 2-Planet-centric SDGs include Clean Water and Sanitation (SDG 6), SDG 12 (Responsible Consumption and Production), SDG 13 (Climate Action), SDG 14 (Life Below Water), and SDG 15 (Life on Land). Topic 3-Prosperity-centric SDG focusing on Sustainable Cities and Communities (SDG 11).
Figure 9. Conceptual representation of the inter-linkages between key sustainable development goals (SDGs) in terms of their advancement through increasing cropland carbon (SOC) sequestration. The direction of the arrow corresponds to the influence (→) of one SDG over the other.
Table 7. Advancing the sustainable development goals (SDGs) through management of soil quality and health.
Research on the sequestration of soil organic carbon (SOC) has substantially expanded over recent periods, based on the significance of SOC as a significant natural reserve that helps the provision of food, facilitates to regenerate fertility, and is necessary for various ecosystem services, such as nutrient transformations, water conservation, and habitat for biodiversity (Kopittke et al., 2022; Smith et al., 2015). Practices (to boost SOC stock) such as fertilizers application, complex crop rotations, cover cropping, and reduced tillage practices have been extensively debated over the last 20 years and it is agreed that those beneficial practices must be continued (Spiegel, 2012; Rumpel et al., 2018). SOC stocks are a function of soil organic matter (SOM) stabilization and decomposition processes in soil. Over the past decade, a paradigm shift has occurred, suggesting that the biotic and abiotic environment is more important for the persistence of SOM than the qualitative attributes of SOM, including its complexity and composition (Schmidt et al., 2011; Liang et al., 2017; Lehmann et al., 2020b).
Overall, the number of publications (TP) shows the research productivity across authors, countries, institutions, and journals, whereas TC of an article reflects the influence of individual papers. Impact factor (IF) and h-index further indicate journal prestige, though citations per paper or research impact (TC/TP) for a journal is often considered as a more suitable measure for assessing the relative importance of a journal within a specific field (Ji et al., 2014). Soil C sequestration research has garnered attention within the global scientific community owing to its strong links with existential global challenges, such as climate change, food security, and soil health (#0 research hotspot; Figure 7C). The scope of global C research has widened greatly over the recent decade, with a striking increase in the TP and U2, especially from 2015 to 2021 (Figure 1). Global soil cropland C sequestration research has been led by a few countries, with 10 countries publishing 63% (TP = 90) of the cropland C sequestration research (Figure 2A). Most of these are developed countries (economically stable), and have developed state-of-the-art research infrastructures and facilities such as universities and research institutions. This facilitates researchers’ access to high-quality resources and services needed to boost innovation, develop cutting-edge research techniques, foster collaboration with other researchers, work on mutual research projects, and share ideas to bolster future competitiveness. The top contributors for producing maximum documents are China (22 documents), the United States (20 documents), and Germany (9 documents), while Australia, Canada, Belgium, and the Netherlands produced six documents each (Figure 2A). Among the top 10 productive authors, Kuzyakov (University of Göttingen, Germany) and Lal (Ohio State University, United States) emerged as the most prolific authors, each with 10 publications and h-index scores of 10 and 9, respectively. Overall, five authors from the United States, and Germany conducted notable research on cropland C sequestration. The United States showed the maximum collaboration among all countries. Major reliance on research on cropland C sequestration in a few countries will underscore a research deficit for other countries, highlighting the necessity for greater international collaboration. Enhanced collaboration between developing and research-active nations is vital to advance innovative solutions for addressing climate-related challenges.
The network analysis of author collaborations reveals six clusters led by Lehmann J (26), Van Wesemael B (total link strength = 30), Minasny B (total link strength = 36), Chenu C, Kuzyakov Y, and Bradford MA (in the sequence of 1-6, respectively). Regarding the corresponding author countries, the United States and China led the top 10 countries in terms of correspondence (single corresponding publication-SCP and multiple correspondence publication-MCP). The top four author keywords such as soil organic carbon, soil organic matter, soil carbon, and nitrogen-account for 58% of the top 10 author keywords, while the top four Keywords Plus (Web of Science) contributed approximately 50% of keyword frequency.
In the current study, cropland C sequestration research featured 702 keywords, mainly encompassing three major topics or themes: i) management practices, ii) the role of microbes, and iii) the impacts of climate (Supplementary Figure S5). Agriculture is influenced by and a facilitator of climate change, as intensive agricultural practices, such as the use of synthetic fertilizers, pesticides, and animal wastes, contributing about 30% to total GHGs (i.e., CO2, N2O, and CH4) emission (#15; Figure 7C), thus increasing global warming and strongly influencing the sustainable production of agroecosystems for longer period. Under these circumstances, long-term C storage in soil is thought to be the viable option to offset the increase in GHG emissions. However, carbon sequestration in cropland soils is an intricate process mainly controlled by climate, cropping systems, soil physical and chemical variables, and management practices (Wiesmeier et al., 2019; Abrar et al., 2023). Soil organic matter (SOM) content (#1; Figure 7C) is an important determinant of soil health because it positively affects soil attributes and processes (Doran and Zeiss, 2000; Hijbeek et al., 2017), in addition to influencing the main factors contributing to the CO2 concentration in the atmosphere. Prudent management of this resource may help achieve climate-change mitigation goals as well as ensure food security at a global scale (Lal, 2004a).
Management practices, such as fertilization, is one of the most important factors dictating SOM dynamics in croplands. However, the effect of different fertilization practices on SOM dynamics is not uniform and hence complicated based on different soil types, climatic conditions, land use and management, soil biota, soil properties, and other factors, etc. (Wiesmeier et al., 2019). Sole application of mineral fertilization, particularly based on nitrogen fertilizers (#5; Figure 7C), reduces the C/N ratio of soil (Abrar et al., 2021), enhances microbial activity (Ashraf et al., 2020), and promotes the positive priming of the fresh OM (Zhu et al., 2018). Carbon stocks can be maintained, preserved, or even increased over a longer period by adopting prudent management practices (Johnston and Sibly, 2018). Conversely, according to some recent research studies, the integrated application of manure/organic amendments along with mineral fertilizers is acknowledged as an effective strategy to not only provide direct C input but also improve the overall soil properties, thereby increasing C sequestration in croplands (Maltas et al., 2018; Abrar et al., 2020). Straw or residue return is another approach for adding organic matter to the soil. Conservation tillage positively influences SOC content by increasing the overall formation of macroaggregates, in contrast to conventional tillage methods, thus improving carbon sequestration and considerably minimizing soil carbon emissions. For instance, no-tillage or minimum tillage may limit the mineralization of carbon, thus, increasing the SOC content (Kan et al., 2021; Zhang et al., 2023; Besen et al., 2024), due to minimal interactions between crop residues and microbes, resulting in minimal decomposition of crop residues into litter. Moreover, slower nitrogen turnover and denitrification may offer better protective effects on soil carbon, nitrogen, and water (Lashermes et al., 2022). The pivotal role of soil microbes as catalysts for SOM formation has long been acknowledged (Kögel-Knabner, 2017). Notably, soil microbes not only accelerate the transformation and turnover of plant litter and SOM as well as contribute roughly 46% to SOM buildup through their microbial necromass (Liang et al., 2019), which has potential implications for deciphering the dynamics of SOM sequestration, especially the critical role of microbial necromass for SOM formation and stabilization (Hu et al., 2023). In the present study, the findings of the manuscript authored by Miltner et al. (Miltner et al., 2012) show the potential role of microbial biomass (research hotspot#10) as a significant source for SOM formation (Table 2; Figure 7C). The chemical composition and structure of SOM are considered significant for its stabilization and decomposition (Lehmann and Kleber, 2015). Although the major proportion of soil C is thought to be derived from plant sources (Kögel-Knabner, 2017), a considerable proportion may pass through microbial biomass before being transformed into SOM. Microbial biomass plays a critical role in biogeochemical cycling (Zheng et al., 2023). The role of soil microbes cannot be negated as they are the main regulators of SOM dynamics and nutrient availability. Microbes play a predominant role in determining the quantity of carbon stored (i.e., four times greater than any other process) in the soil (Tao et al., 2023), with implications for mitigating climate change and improving soil health for food production. Soil attributes, such as bulk density, texture, and porosity, significantly affect microbial activity. A well-structured soil may help foster niche diversity and eventually benefit from the high microbial carbon use efficiency-CUE (Kallenbach et al., 2019; Lehmann et al., 2020b). Accordingly, SOC accrual owing to high CUE could lead to enhanced soil fertility in the form of C sequestration through efficient soil microbial activity which accelerates SOM recycling and accrues recalcitrant microbial residues following microbial death (Mason et al., 2023; Tao et al., 2023). Soil microbes (about 90% dominated by bacteria and fungi) primarily regulate nutrient availability and organic matter dynamics. Recent research has underscored the vital roles that soil microbial communities play in contributing to SOC loss through microbial degradation (Wieder et al., 2013), as well as SOC formation and stability, as revealed through the correlation between microbial biomass, necromass and SOC content (Bradford et al., 2016; Malik et al., 2018; Wang et al., 2021). SOM degradation is the principal process defining the rate of SOC loss, as soil decomposers (mainly microorganisms) mineralize OM and release C back into the atmosphere as CO2. The addition of fresh plant-derived C may cause either positive or negative priming of the SOC (#3 research hotspot; Figure 7C). The input of substrates, such as amino sugars, that may be easily assimilated by microbes can enhance SOC mineralization in the case of positive priming (Hamer and Marschner, 2005). In addition, the enzymatic activities of different soil extracellular enzymes may accelerate the degradation of SOM by transforming complex plant and microbial residues into simple molecules (Sinsabaugh, 2010; Margida et al., 2020). The process through which aboveground and belowground litter is degraded and transformed for SOM formation is of prime importance. Litter quality (containing labile plant constituents) compared to input rates is relatively predominant, as plant residues of high quality can be assimilated more efficiently by microbes. These microbial degradation products would therefore become the principal precursors of stable SOM by promoting physical (aggregation) and chemical stabilization mechanisms through strong chemical bonding to the mineral matrix (Cotrufo et al., 2013). Furthermore, based on stabilization mechanisms, SOM may have four fractions: a fraction devoid of protection or unprotected, physically protected (aggregated), chemically protected, and biochemically protected (Six et al., 2002). The unprotected fraction is unaggregated, fraction-labile in nature, and is an important source of food and energy for soil microbes (Zhang et al., 2022; Wang and Kuzyakov, 2024). Physical protection of SOM against degradation is acquired by the formation of aggregates. The chemical stabilization of SOM is a function of its adsorption onto the soil mineral matrix, and SOM is biochemically protected by the formation of recalcitrant organic compounds (Six et al., 2002). These SOC stabilization mechanisms are considered robust indicators of gauging the causal influences of fertilization management practices (He et al., 2015).
Climate variables, such as temperature and precipitation, play vital roles in C sequestration at regional and global scales by controlling both the input of carbon into the soil and SOC degradation. Precipitation controls net primary productivity (NPP) in many water-limited terrestrial ecosystems, thereby influencing carbon input into the soil. While, humid conditions may induce soil acidification, which limits the decomposition of SOM (Meier and Leuschner, 2010). Soil organic C stocks are generally considered to decrease with increasing temperatures across different climatic regions globally (Koven et al., 2017; Doetterl et al., 2018). Higher soil temperatures enhance the rate of OM degradation, resulting in a decline in bulk soil C stocks (Hicks Pries et al., 2017; García-Palacios et al., 2021). In addition, the two generalized pools of SOM, particulate OM and mineral-associated OM, show different sensitivities to temperature; the sensitivity of POM is approximately 28%–53% greater than that of MAOM (Georgiou et al., 2024). Moreover, how carbon is allocated across these two underlying carbon pools influences the relative sensitivity of bulk SOC stocks to temperature.
Soil degradation threatens the sustenance of billions of people globally, especially 80% of those living in rural areas and 65% employed in the agriculture sector. Agriculture is key to addressing sustainable food production or zero hunger (SDG2) for a growing world population, which is projected to reach 8.5 billion by 2030 (UNFPA, 2023). Advancing SDG 1 (No poverty), and SDG 2, through maintaining and increasing SOM storage following the adoption of best management practices (BMP) such as cover cropping, crop rotations, and integrated nutrient and manure management, can improve soil health and productivity, hence contributing to the production of more food and goods, and increasing incomes for the world’s poor communities (Lal, 2016; Creegan and Flynn, 2020). Moreover, funding and incentivizing farmers through carbon crediting and payment for soil carbon storage may encourage them to adopt soil health practices aimed at increasing crop yields, thus helping to minimize rural poverty (SDG 1), reducing GHG emissions (SDG 13), enhancing resilience to climate change, and contributing to achieving better health outcomes-SDG 3 (Lal, 2016; Oldfield et al., 2022). In this context, several C crediting projects such as the United States and Australia-based soil carbon projects), have been launched to pay farmers for their C sequestration. For instance, In the United States, farmers can earn up to $30 per acre per year by subscribing the soil carbon projects (IndigoAg, 2024). Human health largely depends on soil health, not only via improved crop productivity and crop type but also through its nutritional value (Welch and Graham, 2004). Additionally, soils with an adequate supply of available micronutrients are linked to less malnutrition (Barrett and Bevis, 2015), which is directly dependent upon the increased SOM content (Wood et al., 2018). Together with these widely known attributes, the nutritional value of crops may also depend on robust soil biodiversity (Wall et al., 2015; Lehmann et al., 2020a), which can improve the bioavailability of micronutrients to crops (Jacoby et al., 2017) and overcome the negative impacts of plant disease in soil (Schlatter et al., 2017), and affect the taste, food storage, and food preparation (Rillig et al., 2018). Soil organic carbon (SOC), a major constituent of soil organic matter (SOM), is associated with increased plant productivity (Abrar et al., 2023). It also enhances the retention of moisture and nutrient availability in the soil, improves plant uptake of these nutrients, and ameliorates soil structure and aeration. Additionally, it enhances the resistance of soil to erosion, supports an active and biodiverse soil life (SDG15), and helps advance the overall goal of soil functioning. Nonetheless, this depends on promoting sustainable use of terrestrial ecosystems through BMPs such as conservation tillage, and application of organic amendments (Sanaullah et al., 2019; Yadav et al., 2023) is crucial in reversing land degradation and sequestering atmospheric carbon.
Soil serves as a source or sink of contaminants (Zimnicki et al., 2020), such as agrochemicals (pesticides, fungicides, and herbicides), heavy metals, microplastics, pathogens, and polycyclic aromatic hydrocarbons (Evans et al., 2019). Furthermore, nutrient pollution, especially nitrate from agricultural fertilizers use, is a pressing global issue, causing eutrophication of freshwater resources and negatively affecting the quality of water (Bijay-Singh and Craswell, 2021). Therefore, a trade-off exists between the management of soil health to support crop productivity and water quality, which should be given higher importance and treated with a multi-pronged approach, especially through adopting nature-based solutions. For instance, improving soil structure stability, specifically aggregate stability and strength, via enhancing carbon sequestration (Blanco-Canqui et al., 2006; Mustafa et al., 2020), can help reduce soil erosion and nutrient losses, thereby improving ground- and surface water quality (improving SDG6-related water quality), and increasing water-use efficiency. Healthy soils are also expected to ensure good water quality, as they can effectively buffer and retain heavy metals and organic toxins by irreversible adsorption to organic matter in the soil and biologically modify these entities (Lamichhane et al., 2016). Additionally, soil microbes can convert organic pollutants from more toxic to relatively less toxic forms (Hanson et al., 1999; Lehmann et al., 2020b). Hence, both SOM content and microbial activity are key indicators of soil health and ameliorate water quality. Soil is also a medium for responsible consumption and production, and the adoption of BMP, such as proper utilization of available space, and efficient use of mineral fertilizers and manure (SDG12), can help improve C sequestration in the soil, leading to healthy soil and enhanced agricultural productivity, which is safe for consumption (Mishra et al., 2022). Climate change is a pressing global issue, and croplands are considered to be important food systems, which may act as major C sinks for offsetting climate change-driven effects due to their tremendous capacity to store large amounts of carbon (SDG13). Concurrently, croplands can also act as a C source if not properly managed in terms of management practices. Climate change can directly influence soil ecosystem services, particularly crop productivity and water quality, and can also undermine other SDGs, specifically those related to soil health. For instance, climate change mitigation schemes, such as storing carbon as SOM in soil, can contribute to agriculture by improving crop health and production, thus providing resilience to drought and flooding (Lal, 2004a). Furthermore, increased SOM content can be achieved by applying organic fertilizers or soil amendments in greater quantities, along with reducing tillage operations (Karlen et al., 2019), to improve aggregation and limit C decomposition by microbes, which can also enhance plant growth. Climatic variables, such as temperature and precipitation, may positively or negatively influence C stocks in soil (Mishra et al., 2021; Abrar et al., 2023). Nonetheless, there are trade-offs between managing soil health for climate change and food production. For example, the application of nitrogen-based fertilizers, aimed at increasing crop production, may lead to a greater efflux of N2O (nitrous oxide), which is a strong greenhouse gas (Paustian et al., 2016). These examples underscore the existing challenges in optimizing the multifunctional usage of soils and why it is critical to achieve goals related to managing soil health.
Cities are the main contributors to economic growth, accounting for approximately 80% of the world’s Gross Domestic Product (GDP) and demonstrating greater productivity compared with rural areas (Sharif, 2023). The significance of healthy soils in providing ecosystem services is necessary for developing sustainable cities is gaining attention within the scientific community. Supporting urban agriculture and gardening, through ameliorating soil health in global cities is crucial for their adaptability to the challenges of future climate change, and hence an important initiative to achieving the global pursuit of United Nations SDGs (Lal et al., 2021). Urban soils face issues such as soil contamination (including metals, organics, and microbials), nutrient deficit, decline in SOC, and biodiversity loss. SDG 11 (Sustainable cities and communities-including targets 11.6 and 11.7) exclusively deals with making cities and metropolitans sustainable in terms of inclusivity, safety, and resilience. Carbon sequestration could help advance SDG 11, regarding the management of urban waste degradation, sustaining the poor’s livelihoods, effectively managing urban green spaces, and minimizing sediment pollution (Albaladejo et al., 2021; Yin et al., 2022). Moreover, Target 11.8 is directly associated with addressing soil issues, as soil C sequestration helps mitigate and adapting climate change, thus providing numerous ecosystem services for the benefit of humankind (Lal et al., 2021).
Over the past decade, there has been a significant increase in highly-cited publications on cropland carbon sequestration, which underscores the growing global interest in this critical issue. The findings suggest that cropland carbon sequestration holds promise for achieving Sustainable Development Goals (SDGs) by enhancing soil health. Recognizing soil health as a fundamental principle, rather than merely a measurable property, can unify researchers, farmers, and other stakeholders, fostering a collaborative approach to sustainable soil management. Prospective business models are also essential to align the commercial interests of farmers, land managers, and agricultural suppliers with the co-benefits of soil health. Given the extensive environmental and societal services provided by soil, legal recognition of soil health as a public or shared resource is warranted. Governments and inter-governmental organizations should develop a unified framework for quantifying soil health, which should be properly endorsed and implemented by stakeholders at regional, national, and global levels. This framework should guide policymakers in formulating strategies that enhance soil health and contribute to advancing the broader SDGs.
The datasets presented in this article are not readily available because We are not allowed to share the data. Requests to access the datasets should be directed to cmFuZGhhd2FzYWJAaG90bWFpbC5jb20=.
MA: Conceptualization, Data curation, Visualization, Writing – original draft. MW: Data curation, Formal Analysis, Writing – review and editing. KM: Data curation, Formal Analysis, Writing – review and editing. RF: Formal Analysis, Methodology, Writing – review and editing. MM: Formal Analysis, Investigation, Writing – review and editing. MK: Investigation, Methodology, Writing – review and editing. NS: Data curation, Resources, Writing – review and editing. MX: Formal Analysis, Resources, Writing – review and editing. JD: Conceptualization, Methodology, Resources, Writing – review and editing.
The author(s) declare that financial support was received for the research and/or publication of this article. This study was supported by the National Natural Science Foundation of China (42177299) and the Key Technologies Research & Development (R&D) Program of Guangdong Province (2023B0202080002).
The authors declare that the research was conducted in the absence of any commercial or financial relationships that could be construed as a potential conflict of interest.
All claims expressed in this article are solely those of the authors and do not necessarily represent those of their affiliated organizations, or those of the publisher, the editors and the reviewers. Any product that may be evaluated in this article, or claim that may be made by its manufacturer, is not guaranteed or endorsed by the publisher.
The Supplementary Material for this article can be found online at: https://www.frontiersin.org/articles/10.3389/fenvs.2025.1495991/full#supplementary-material
Abrar, M. M., Shah, S. A. A., Sun, N., Mehmood, K., Aziz, T., Waqas, M. A., et al. (2023). Long-term manure application enhances organic carbon and nitrogen stocks in Mollisol subsoil. L. Degrad. Dev. 34, 815–832. doi:10.1002/ldr.4498
Abrar, M. M., Xu, H., Aziz, T., Sun, N., Mustafa, A., Aslam, M. W., et al. (2021). Carbon, nitrogen, and phosphorus stoichiometry mediate sensitivity of carbon stabilization mechanisms along with surface layers of a Mollisol after long-term fertilization in Northeast China. J. Soils Sediments 21, 705–723. doi:10.1007/s11368-020-02825-7
Abrar, M. M., Xu, M., Shah, S. A. A., Aslam, M. W., Aziz, T., Mustafa, A., et al. (2020). Variations in the profile distribution and protection mechanisms of organic carbon under long-term fertilization in a Chinese Mollisol. Sci. Total Environ. 723, 138181. doi:10.1016/j.scitotenv.2020.138181
Albaladejo, J., Díaz-Pereira, E., and de Vente, J. (2021). Eco-holistic soil conservation to support land degradation neutrality and the sustainable development goals. Catena 196, 104823. doi:10.1016/j.catena.2020.104823
Albort-Morant, G., Henseler, J., Leal-Millán, A., and Cepeda-Carrión, G. (2017). Mapping the field: a bibliometric analysis of green innovation. Sustainability 9, 1011. doi:10.3390/su9061011
Amelung, W., Bossio, D., de Vries, W., Kögel-Knabner, I., Lehmann, J., Amundson, R., et al. (2020). Towards a global-scale soil climate mitigation strategy. Nat. Commun. 11, 5427. doi:10.1038/s41467-020-18887-7
Andrade, D. F., Romanelli, J. P., and Pereira-Filho, E. R. (2019). Past and emerging topics related to electronic waste management: top countries, trends, and perspectives. Environ. Sci. Pollut. Res. 26, 17135–17151. doi:10.1007/s11356-019-05089-y
Aria, M., and Cuccurullo, C. (2017). bibliometrix: an R-tool for comprehensive science mapping analysis. J. Informetr. 11, 959–975. doi:10.1016/j.joi.2017.08.007
Ashraf, M. N., Hu, C., Wu, L., Duan, Y., Zhang, W., Aziz, T., et al. (2020). Soil and microbial biomass stoichiometry regulate soil organic carbon and nitrogen mineralization in rice-wheat rotation subjected to long-term fertilization. J. Soils Sediments 20, 3103–3113. doi:10.1007/s11368-020-02642-y
Baert, P., Vanmaercke, M., and Meersmans, J. (2024). Assessing the 3D distribution of soil organic carbon by integrating predictions of water and tillage erosion into a digital soil mapping-approach: a case study for silt loam cropland (Belgium). Geoderma 447, 116928. doi:10.1016/j.geoderma.2024.116928
Barrett, C. B., and Bevis, L. E. M. (2015). The self-reinforcing feedback between low soil fertility and chronic poverty. Nat. Geosci. 8, 907–912. doi:10.1038/ngeo2591
Bastos, A., Hartung, K., Nützel, T. B., Nabel, J. E. M. S., Houghton, R. A., and Pongratz, J. (2021). Comparison of uncertainties in land-use change fluxes from bookkeeping model parameterisation. Earth Syst. Dyn. 12, 745–762. doi:10.5194/esd-12-745-2021
Batjes, N. H. (2014). Total carbon and nitrogen in the soils of the world. Eur. J. Soil Sci. 65, 10–21. doi:10.1111/ejss.12114_2
Batjes, N. H. (2016). Harmonized soil property values for broad-scale modelling (WISE30sec) with estimates of global soil carbon stocks. Geoderma 269, 61–68. doi:10.1016/j.geoderma.2016.01.034
Batjes, N. H., and Sombroek, W. G. (1997). Possibilities for carbon sequestration in tropical and subtropical soils. Glob. Chang. Biol. 3, 161–173. doi:10.1046/j.1365-2486.1997.00062.x
Besen, M. R., Ribeiro, R. H., Bratti, F., Locatelli, J. L., Schmitt, D. E., and Piva, J. T. (2024). Cover cropping associated with no-tillage system promotes soil carbon sequestration and increases crop yield in Southern Brazil. Soil Tillage Res. 242, 106162. doi:10.1016/j.still.2024.106162
Bhatnagar, S., Chaudhary, R., Sharma, S., Janjhua, S., Thakur, P., Sharma, P., et al. (2024). Exploring the dynamics of climate-smart agricultural practices for sustainable resilience in a changing climate. Environ. Sustain. Indic. 100535.
Bijay-Singh, H., and Craswell, E. (2021). Fertilizers and nitrate pollution of surface and ground water: an increasingly pervasive global problem. SN Appl. Sci. doi:10.1007/s42452-021-04521-8
Blanco-Canqui, H., Lal, R., Post, W. M., Izaurralde, R. C., and Owens, L. B. (2006). Rapid changes in soil carbon and structural properties due to stover removal from no-till corn plots. Handb. Environ. Chem. Vol. 5 Water Pollut. 171, 468–482. doi:10.1097/01.ss.0000209364.85816.1b
Bowen, K. J., Ebi, K. L., Woodward, A., McIver, L., Tukuitonga, C., and Nayna Schwerdtle, P. (2024). Human health and climate change in the Pacific: a review of current knowledge. Clim. Dev. 16, 119–133. doi:10.1080/17565529.2023.2185479
Bradford, M. A., Wieder, W. R., Bonan, G. B., Fierer, N., Raymond, P. A., and Crowther, T. W. (2016). Managing uncertainty in soil carbon feedbacks to climate change. Nat. Clim. Chang. 6, 751–758. doi:10.1038/nclimate3071
Canadell, J. G., Monteiro, P. M. S., Costa, M. H., Cotrim da Cunha, L., Cox, P. M., Eliseev, A. V., et al. (2023). “Intergovernmental Panel on Climate Change (IPCC). Global carbon and other biogeochemical cycles and feedbacks,” in Climate change 2021: the physical science basis. Contribution of working group I to the sixth assessment report of the intergovernmental panel on climate change (Cambridge University Press), 673–816.
Chabbi, A., Lehmann, J., Ciais, P., Loescher, H. W., Cotrufo, M. F., Don, A., et al. (2017). Aligning agriculture and climate policy. Nat. Clim. Chang. 7, 307–309. doi:10.1038/nclimate3286
Chen, C. (2004). Searching for intellectual turning points: progressive knowledge domain visualization. Proc. Natl. Acad. Sci. U. S. A. 101, 5303–5310. doi:10.1073/pnas.0307513100
Chen, G., Bai, J., Bi, C., Wang, Y., and Cui, B. (2023). Global greenhouse gas emissions from aquaculture: a bibliometric analysis. Agric. Ecosyst. Environ. 348, 108405. doi:10.1016/j.agee.2023.108405
Cotrufo, M. F., Soong, J. L., Horton, A. J., Campbell, E. E., Haddix, M. L., Wall, D. H., et al. (2015). Formation of soil organic matter via biochemical and physical pathways of litter mass loss. Nat. Geosci. 8, 776–779. doi:10.1038/ngeo2520
Cotrufo, M. F., Wallenstein, M. D., Boot, C. M., Denef, K., and Paul, E. (2013). The Microbial Efficiency-Matrix Stabilization (MEMS) framework integrates plant litter decomposition with soil organic matter stabilization: do labile plant inputs form stable soil organic matter? Glob. Chang. Biol. 19, 988–995. doi:10.1111/gcb.12113
Creegan, E. F., and Flynn, R. (2020). in SDG 2 zero hunger BT - actioning the global goals for local impact: towards sustainability science, policy, education and practice. Editors I. B. Franco, T. Chatterji, E. Derbyshire, and J. Tracey (Singapore: Springer Singapore), 23–37. doi:10.1007/978-981-32-9927-6_3
Crowther, T. W., Todd-Brown, K. E. O., Rowe, C. W., Wieder, W. R., Carey, J. C., Machmuller, M. B., et al. (2016). Quantifying global soil carbon losses in response to warming. Nature 540, 104–108. doi:10.1038/nature20150
da Cruz, T. T., Balestieri, J. A. P., de Toledo Silva, J. M., Vilanova, M. R. N., Oliveira, O. J., and Ávila, I. (2021). Life cycle assessment of carbon capture and storage/utilization: from current state to future research directions and opportunities. Int. J. Greenh. Gas. Control 108, 103309. doi:10.1016/j.ijggc.2021.103309
Doetterl, S., Berhe, A. A., Arnold, C., Bodé, S., Fiener, P., Finke, P., et al. (2018). Links among warming, carbon and microbial dynamics mediated by soil mineral weathering. Nat. Geosci. 11, 589–593. doi:10.1038/s41561-018-0168-7
Don, A., Seidel, F., Leifeld, J., Kätterer, T., Martin, M., Pellerin, S., et al. (2024). Carbon sequestration in soils and climate change mitigation—definitions and pitfalls. Glob. Chang. Biol. 30, e16983. doi:10.1111/gcb.16983
Doran, J. W., and Zeiss, M. R. (2000). Soil health and sustainability: managing the biotic component of soil quality. Appl. soil Ecol. 15, 3–11. doi:10.1016/s0929-1393(00)00067-6
Dungait, J. A. J., Hopkins, D. W., Gregory, A. S., and Whitmore, A. P. (2012). Soil organic matter turnover is governed by accessibility not recalcitrance. Glob. Chang. Biol. 18, 1781–1796. doi:10.1111/j.1365-2486.2012.02665.x
Egghe, L. (2006). Theory and practise of the g-index. Scientometrics 69, 131–152. doi:10.1007/s11192-006-0144-7
Ellis, E., and Swan, A. (2024). Potential for U.S. Agriculture to Be greenhouse gas negative: chapter 2-the challenges and opportunities for soil carbon sequestration. Available online at: https://cast-science.org/wp-content/uploads/2024/11/USFRA_CAST-FullReport.pdf.
Evans, A. E., Mateo-Sagasta, J., Qadir, M., Boelee, E., and Ippolito, A. (2019). Agricultural water pollution: key knowledge gaps and research needs. Curr. Opin. Environ. Sustain. 36, 20–27. doi:10.1016/j.cosust.2018.10.003
Friedlingstein, P., Jones, M. W., O’Sullivan, M., Andrew, R. M., Bakker, D. C. E., Hauck, J., et al. (2022). Global carbon budget 2021. Earth Syst. Sci. Data 14, 1917–2005. doi:10.5194/essd-14-1917-2022
García-Palacios, P., Crowther, T. W., Dacal, M., Hartley, I. P., Reinsch, S., Rinnan, R., et al. (2021). Evidence for large microbial-mediated losses of soil carbon under anthropogenic warming. Nat. Rev. Earth Environ. 2, 507–517. doi:10.1038/s43017-021-00178-4
Georgiou, K., Koven, C. D., Wieder, W. R., Hartman, M. D., Riley, W. J., Pett-Ridge, J., et al. (2024). Emergent temperature sensitivity of soil organic carbon driven by mineral associations. Nat. Geosci. 17, 205–212. doi:10.1038/s41561-024-01384-7
Ghimire, R., and Khanal, B. R. (2020). Soil organic matter dynamics in semiarid agroecosystems transitioning to dryland. PeerJ 8, e10199. doi:10.7717/peerj.10199
Hamer, U., and Marschner, B. (2005). Priming effects in different soil types induced by fructose, alanine, oxalic acid and catechol additions. Soil Biol. biochem. 37, 445–454. doi:10.1016/j.soilbio.2004.07.037
Hansis, E., Davis, S. J., and Pongratz, J. (2015). Relevance of methodological choices for accounting of land use change carbon fluxes. Glob. Biogeochem. Cycles 29, 1230–1246. doi:10.1002/2014gb004997
Hanson, J. R., Macalady, J. L., Harris, D., and Scow, K. M. (1999). Linking toluene degradation with specific microbial populations in soil. Appl. Environ. Microbiol. 65, 5403–5408. doi:10.1128/aem.65.12.5403-5408.1999
He, Y. T., Zhang, W. J., Xu, M. G., Tong, X. G., Sun, F. X., Wang, J. Z., et al. (2015). Long-term combined chemical and manure fertilizations increase soil organic carbon and total nitrogen in aggregate fractions at three typical cropland soils in China. Sci. Total Environ. 532, 635–644. doi:10.1016/j.scitotenv.2015.06.011
Hengl, T., Mendes de Jesus, J., Heuvelink, G. B. M., Ruiperez Gonzalez, M., Kilibarda, M., Blagotić, A., et al. (2017). SoilGrids250m: global gridded soil information based on machine learning. PLoS One 12, e0169748. doi:10.1371/journal.pone.0169748
Hicks Pries, C. E., Castanha, C., Porras, R. C., and Torn, M. S. (2017). The whole-soil carbon flux in response to warming. Sci. 355, 1420–1423. doi:10.1126/science.aal1319
Hijbeek, R., van Ittersum, M. K., ten Berge, H. F. M., Gort, G., Spiegel, H., and Whitmore, A. P. (2017). Do organic inputs matter – a meta-analysis of additional yield effects for arable crops in Europe. Plant Soil 411, 293–303. doi:10.1007/s11104-016-3031-x
Hirsch, J. E. (2005). An index to quantify an individual’s scientific research output. Proc. Natl. Acad. Sci. U. S. A. 102, 16569–16572. doi:10.1073/pnas.0507655102
Hou, J., Yang, X., and Chen, C. (2018). Emerging trends and new developments in information science: a document co-citation analysis (2009–2016). Scientometrics 115, 869–892. doi:10.1007/s11192-018-2695-9
Hu, J., Du, M., Chen, J., Tie, L., Zhou, S., Buckeridge, K. M., et al. (2023). Microbial necromass under global change and implications for soil organic matter. Glob. Chang. Biol. 29, 3503–3515. doi:10.1111/gcb.16676
Hughes, H. M., McClelland, S. C., Schipanski, M. E, and Hillier, J. (2023). Modelling the soil C impacts of cover crops in temperate regions. Agric. Syst. 209, 103663.
IndigoAg (2024). Enrich your soil, improve your profit potential with Carbon by Indigo. Available online at: https://www.indigoag.com/carbon/for-farmers (Accessed July 18, 2024).
Ingram, J., Maye, D., and Reed, M. (2025). Contestations in the emerging soil-based carbon economy: towards a research agenda. Sustain. Sci., 1–15. doi:10.1007/s11625-024-01609-z
Jacoby, R., Peukert, M., Succurro, A., Koprivova, A., and Kopriva, S. (2017). The role of soil microorganisms in plant mineral nutrition—current knowledge and future directions. Front. Plant Sci. 8, 1617. doi:10.3389/fpls.2017.01617
Janzen, H. H., van Groenigen, K. J., Powlson, D. S., Schwinghamer, T., and van Groenigen, J. W. (2022). Photosynthetic limits on carbon sequestration in croplands. Geoderma 416, 115810. doi:10.1016/j.geoderma.2022.115810
Jat, M. L., Chakraborty, D., Ladha, J. K., Parihar, C. M., Datta, A., Mandal, B., et al. (2022). Carbon sequestration potential, challenges, and strategies towards climate action in smallholder agricultural systems of South Asia. Crop Environ. 1, 86–101. doi:10.1016/j.crope.2022.03.005
Ji, Q., Pang, X., and Zhao, X. (2014). A bibliometric analysis of research on Antarctica during 1993–2012. Scientometrics 101, 1925–1939. doi:10.1007/s11192-014-1332-5
Johnston, A. S. A., and Sibly, R. M. (2018). The influence of soil communities on the temperature sensitivity of soil respiration. Nat. Ecol. Evol. 2, 1597–1602. doi:10.1038/s41559-018-0648-6
Kallenbach, C. M., Wallenstein, M. D., Schipanksi, M. E., and Stuart Grandy, A. (2019). Managing agroecosystems for soil microbial carbon use efficiency: ecological unknowns, potential outcomes, and a path forward. Front. Microbiol. 10, 1146. doi:10.3389/fmicb.2019.01146
Kan, Z. R., Liu, Q. Y., Virk, A. L., He, C., Qi, J. Y., Dang, Y. P., et al. (2021). Effects of experiment duration on carbon mineralization and accumulation under no-till. Soil Tillage Res. 209, 104939. doi:10.1016/j.still.2021.104939
Karlen, D. L., Veum, K. S., Sudduth, K. A., Obrycki, J. F., and Nunes, M. R. (2019). Soil health assessment: past accomplishments, current activities, and future opportunities. Soil Tillage Res. 195, 104365. doi:10.1016/j.still.2019.104365
Keesstra, S. D., Bouma, J., Wallinga, J., Tittonell, P., Smith, P., Cerdà, A., et al. (2016). The significance of soils and soil science towards realization of the United Nations Sustainable Development Goals. aura.abdn.ac.uk 2, 111–128. doi:10.5194/soil-2-111-2016
Köchy, M., Hiederer, R., and Freibauer, A. (2015). Global distribution of soil organic carbon – Part 1: masses and frequency distributions of SOC stocks for the tropics, permafrost regions, wetlands, and the world. SOIL. doi:10.5194/soil-1-351-2015
Kögel-Knabner, I. (2017). The macromolecular organic composition of plant and microbial residues as inputs to soil organic matter: fourteen years on. Soil Biol. biochem. 105, A3–A8. doi:10.1016/j.soilbio.2016.08.011
Kopittke, P. M., Berhe, A. A., Carrillo, Y., Cavagnaro, T. R., Chen, D., Chen, Q. L., et al. (2022). Ensuring planetary survival: the centrality of organic carbon in balancing the multifunctional nature of soils. Crit. Rev. Environ. Sci. Technol. 52, (23) 4308–4324.
Koven, C. D., Hugelius, G., Lawrence, D. M., and Wieder, W. R. (2017). Higher climatological temperature sensitivity of soil carbon in cold than warm climates. Nat. Clim. Chang. 7, 817–822. doi:10.1038/nclimate3421
Kuzyakov, Y., and Blagodatskaya, E. (2015). Microbial hotspots and hot moments in soil: concept and review. Soil Biol. biochem. 83, 184–199. doi:10.1016/j.soilbio.2015.01.025
Lal, R. (2004a). Soil carbon sequestration impacts on global climate change and food security. Sci. 304, 1623–1627. doi:10.1126/science.1097396
Lal, R. (2004b). Soil carbon sequestration to mitigate climate change. Geoderma 123, 1–22. doi:10.1016/j.geoderma.2004.01.032
Lal, R. (2016). Soil health and carbon management. Food Energy Secur 5, 212–222. doi:10.1002/fes3.96
Lal, R., Bouma, J., Brevik, E., Dawson, L., Field, D. J., Glaser, B., et al. (2021). Soils and sustainable development goals of the united nations: an international union of soil sciences perspective. Geoderma Reg. 25, e00398. doi:10.1016/j.geodrs.2021.e00398
Lamichhane, S., Bal Krishna, K. C., and Sarukkalige, R. (2016). Polycyclic aromatic hydrocarbons (PAHs) removal by sorption: a review. Chemosphere 148, 336–353. doi:10.1016/j.chemosphere.2016.01.036
Lashermes, G., Recous, S., Alavoine, G., Janz, B., Butterbach-Bahl, K., Ernfors, M., et al. (2022). N2O emissions from decomposing crop residues are strongly linked to their initial soluble fraction and early C mineralization. Sci. Total Environ. 806, 150883. doi:10.1016/j.scitotenv.2021.150883
Lehmann, J., Bossio, D. A., Kögel-Knabner, I., and Rillig, M. C. (2020a). The concept and future prospects of soil health. Nat. Rev. Earth Environ. 1, 544–553. doi:10.1038/s43017-020-0080-8
Lehmann, J., Hansel, C. M., Kaiser, C., Kleber, M., Maher, K., Manzoni, S., et al. (2020b). Persistence of soil organic carbon caused by functional complexity. Nat. Geosci. 13, 529–534. doi:10.1038/s41561-020-0612-3
Lehmann, J., and Kleber, M. (2015). The contentious nature of soil organic matter. Nature 528, 60–68. doi:10.1038/nature16069
Lessmann, M., Ros, G. H., Young, M. D., and de Vries, W. (2022). Global variation in soil carbon sequestration potential through improved cropland management. Glob. Chang. Biol. 28, 1162–1177. doi:10.1111/gcb.15954
Li, J., and Chen, C. M. (2017). CiteSpace: text mining and visualization in scientific literature. 2nd Edn. Beijing, China: Capital University of Economics and Business Press.
Liang, C., Amelung, W., Lehmann, J., and Kästner, M. (2019). Quantitative assessment of microbial necromass contribution to soil organic matter. Glob. Chang. Biol. 25, 3578–3590. doi:10.1111/gcb.14781
Liang, C., Schimel, J. P., and Jastrow, J. D. (2017). The importance of anabolism in microbial control over soil carbon storage. Nat. Microbiol. 2, 17105. doi:10.1038/nmicrobiol.2017.105
Liu, Q., Li, J., Ye, S., Guo, Y., and Wang, S. (2024). Characteristics and hotspots of forest litter decomposition research: a bibliometric analysis. L. Degrad. Dev. 35, 2684–2699. doi:10.1002/ldr.5096
Liu, X., Zhang, L., and Hong, S. (2011). Global biodiversity research during 1900–2009: a bibliometric analysis. Biodivers. Conserv. 20, 807–826. doi:10.1007/s10531-010-9981-z
Malik, A. A., Puissant, J., Buckeridge, K. M., Goodall, T., Jehmlich, N., Chowdhury, S., et al. (2018). Land use driven change in soil pH affects microbial carbon cycling processes. Nat. Commun. 9, 3591. doi:10.1038/s41467-018-05980-1
Maltas, A., Kebli, H., Oberholzer, H. R., Weisskopf, P., and Sinaj, S. (2018). The effects of organic and mineral fertilizers on carbon sequestration, soil properties, and crop yields from a long-term field experiment under a Swiss conventional farming system. L. Degrad. Dev. 29, 926–938. doi:10.1002/ldr.2913
Mandal, A., Majumder, A., Dhaliwal, S. S., Toor, A. S., Mani, P. K., Naresh, R. K., et al. (2022). Impact of agricultural management practices on soil carbon sequestration and its monitoring through simulation models and remote sensing techniques: a review. Crit. Rev. Environ. Sci. Technol. 52, 1–49. doi:10.1080/10643389.2020.1811590
Margida, M. G., Lashermes, G., and Moorhead, D. L. (2020). Estimating relative cellulolytic and ligninolytic enzyme activities as functions of lignin and cellulose content in decomposing plant litter. Soil Biol. biochem. 141, 107689. doi:10.1016/j.soilbio.2019.107689
Mason, A. R. G., Salomon, M. J., Lowe, A. J., and Cavagnaro, T. R. (2023). Microbial solutions to soil carbon sequestration. J. Clean. Prod. 417, 137993. doi:10.1016/j.jclepro.2023.137993
Mehmood, K., Qiu, X., and Abrar, M. M. (2023). Unearthing research trends in emissions and sustainable development: potential implications for future directions. Gondwana Res. 119, 227–245. doi:10.1016/j.gr.2023.02.009
Meier, I. C., and Leuschner, C. (2010). Variation of soil and biomass carbon pools in beech forests across a precipitation gradient. Glob. Chang. Biol. 16, 1035–1045. doi:10.1111/j.1365-2486.2009.02074.x
Melillo, J. M., Frey, S. D., DeAngelis, K. M., Werner, W. J., Bernard, M. J., Bowles, F. P., et al. (2017). Long-term pattern and magnitude of soil carbon feedback to the climate system in a warming world. Sci. 358, 101–105. doi:10.1126/science.aan2874
Miltner, A., Bombach, P., Schmidt-Brücken, B., and Kästner, M. (2012). SOM genesis: microbial biomass as a significant source. Biogeochemistry 111, 41–55. doi:10.1007/s10533-011-9658-z
Minasny, B., Malone, B. P., McBratney, A. B., Angers, D. A., Arrouays, D., Chambers, A., et al. (2017). Soil carbon 4 per mille. Geoderma 292, 59–86. doi:10.1016/j.geoderma.2017.01.002
Minasny, B., McBratney, A. B., Arrouays, D., Chabbi, A., Field, D. J., Kopittke, P. M., et al. (2023). Soil carbon sequestration: much more than a climate solution. Environ. Sci. Technol. 57, 19094–19098. doi:10.1021/acs.est.3c07312
Mishra, A. K., Grover, D., Das, R., Mishra, J., and Biswal, B. (2022). in Carbon sequestration potential in agricultural systems BT - agro-biodiversity and agri-ecosystem management. Editors P. Kumar, R. S. Tomar, J. A. Bhat, M. Dobriyal, and M. Rani (Singapore: Springer Nature Singapore), 87–111. doi:10.1007/978-981-19-0928-3_6
Mishra, U., Hugelius, G., Shelef, E., Yang, Y., Strauss, J., Lupachev, A., et al. (2021). Spatial heterogeneity and environmental predictors of permafrost region soil organic carbon stocks. Sci. Adv. 7, eaaz5236. doi:10.1126/sciadv.aaz5236
Moinet, G. Y. K., Hijbeek, R., van Vuuren, D. P., and Giller, K. E. (2023). Carbon for soils, not soils for carbon. Glob. Chang. Biol. 29, 2384–2398. doi:10.1111/gcb.16570
Monger, H. C., and Martinez-Rios, J. J. (2000). Inorganic carbon sequestration in grazing lands. potential U. S. grazing lands sequester carbon mitigate Greenh. Eff, 87–118.
Mourao, P. R., and Martinho, V. D. (2020). Forest entrepreneurship: a bibliometric analysis and a discussion about the co-authorship networks of an emerging scientific field. J. Clean. Prod. 256, 120413. doi:10.1016/j.jclepro.2020.120413
Mustafa, A., Minggang, X., Ali Shah, S. A., Abrar, M. M., Nan, S., Baoren, W., et al. (2020). Soil aggregation and soil aggregate stability regulate organic carbon and nitrogen storage in a red soil of southern China. J. Environ. Manage. 270, 110894. doi:10.1016/j.jenvman.2020.110894
Nazir, M. J., Li, G., Nazir, M. M., Zulfiqar, F., Siddique, K. H. M., Iqbal, B., et al. (2024). Harnessing soil carbon sequestration to address climate change challenges in agriculture. Soil Tillage Res. 237, 105959. doi:10.1016/j.still.2023.105959
Oldfield, E. E., Eagle, A. J., Rubin, R. L., Rudek, J., Sanderman, J., and Gordon, D. R. (2022). Crediting agricultural soil carbon sequestration. Sci. 375, 1222–1225. doi:10.1126/science.abl7991
Oldfield, E. E., Lavallee, J. M., Blesh, J., Bradford, M. A., Cameron-Harp, M., Cotrufo, M. F., et al. (2024). Greenhouse gas mitigation on croplands: clarifying the debate on knowns, unknowns and risks to move forward with effective management interventions. Carbon Manag. 15, 2365896. doi:10.1080/17583004.2024.2365896
Paustian, K., Lehmann, J., Ogle, S., Reay, D., Robertson, G. P., and Smith, P. (2016). Climate-smart soils. Nature 532, 49–57. doi:10.1038/nature17174
Pereira, P., Bogunovic, I., Muñoz-Rojas, M., and Brevik, E. C. (2018). Soil ecosystem services, sustainability, valuation and management. Curr. Opin. Environ. Sci. Heal. 5, 7–13. doi:10.1016/j.coesh.2017.12.003
Pohl, H. (2020). Collaboration with countries with rapidly growing research: supporting proactive development of international research collaboration. Scientometrics 122, 287–307. doi:10.1007/s11192-019-03287-6
Priya, A. K., Rajamanickam, S., Sivarethinamohan, S., Gaddam, M. K. R., Velusamy, P., et al. (2023). Impact of climate change and anthropogenic activities on aquatic ecosystem – a review. Environ. Res. doi:10.1016/j.envres.2023.117233
Pro Oxygen (2024). No title. Available online at: http://co2.earth/.
Rausser, G. C., Foster, W., and Choi, E. (2025). The political economy of industrial policies in natural resources.
Razzaghi, S. (2025). “Empowering agriculture in the face of climate change with smart solutions,” in Agriculture and water management under climate change (Springer), 153–172.
Rillig, M. C., Lehmann, A., Lehmann, J., Camenzind, T., and Rauh, C. (2018). Soil biodiversity effects from field to fork. Trends Plant Sci. 23, 17–24. doi:10.1016/j.tplants.2017.10.003
Romanenkov, V. A., Meshalkina, J. L., Gorbacheva, A. Y., Krenke, A. N., Petrov, I. K., Golozubov, O. M., et al. (2024). Maps of soil organic carbon sequestration potential in the Russian croplands. Eurasian Soil Sci. 57, 737–750. doi:10.1134/S106422932360375X
Rumpel, C., Amiraslani, F., Koutika, L. S., Smith, P., Whitehead, D., and Wollenberg, E. (2018). Put more carbon in soils to meet paris climate pledges. Nature 564, 32–34. doi:10.1038/d41586-018-07587-4
Sanaullah, M., Afzal, T., Shahzad, T., and Wakeel, A. (2019). in Carbon sequestration for sustainable agriculture BT - innovations in sustainable agriculture. Editors M. Farooq,, and M. Pisante (Cham: Springer International Publishing), 469–500. doi:10.1007/978-3-030-23169-9_15
Sanderman, J., Hengl, T., and Fiske, G. J. (2017). Soil carbon debt of 12,000 years of human land use. Proc. Natl. Acad. Sci. U. S. A. 114, 9575–9580. doi:10.1073/pnas.1706103114
Scharlemann, J. P. W., Tanner, E. V. J., Hiederer, R., and Kapos, V. (2014). Global soil carbon: understanding and managing the largest terrestrial carbon pool. Carbon Manag. 5, 81–91. doi:10.4155/cmt.13.77
Schlatter, D., Kinkel, L., Thomashow, L., Weller, D., and Paulitz, T. (2017). Disease suppressive soils: new insights from the soil microbiome. Phytopathology 107, 1284–1297. doi:10.1094/PHYTO-03-17-0111-RVW
Schmidt, M. W. I., Torn, M. S., Abiven, S., Dittmar, T., Guggenberger, G., Janssens, I. A., et al. (2011). Persistence of soil organic matter as an ecosystem property. Nature 478, 49–56. doi:10.1038/nature10386
Sharif, M. M. (2023). Promoting sustainable human settlements: its relevance to the 2030 agenda. U. N. Chron. 55, 23–25. doi:10.18356/eecd309c-en
Sinsabaugh, R. L. (2010). Phenol oxidase, peroxidase and organic matter dynamics of soil. Soil Biol. biochem. 42, 391–404. doi:10.1016/j.soilbio.2009.10.014
Sinsabaugh, R. L., Manzoni, S., Moorhead, D. L., and Richter, A. (2013). Carbon use efficiency of microbial communities: stoichiometry, methodology and modelling. Ecol. Lett. 16, 930–939. doi:10.1111/ele.12113
Six, J., Conant, R. T., Paul, E. A., and Paustian, K. (2002). Stabilisation mechanisms for carbon, 155–176.
Smith, P., Cotrufo, M. F., Rumpel, C., Paustian, K., Kuikman, P. J., Elliott, J. A., et al. (2015). Biogeochemical cycles and biodiversity as key drivers of ecosystem services provided by soils. Soil. 1, (2) 665–685. doi:10.1016/j.still.2017.12.002
Soussana, J.-F., Lutfalla, S., Ehrhardt, F., Rosenstock, T., Lamanna, C., Havlík, P., et al. (2019). Matching policy and science: rationale for the ‘4 per 1000 - soils for food security and climate’ initiative. Soil Tillage Res. 188, 3–15. doi:10.1016/j.still.2017.12.002
Spiegel, H. (2012). Impacts of arable management on soil organic carbon and nutritionally relevant elements in the soil-plant system.
Stockmann, U., Adams, M. A., Crawford, J. W., Field, D. J., Henakaarchchi, N., Jenkins, M., et al. (2013). The knowns, known unknowns and unknowns of sequestration of soil organic carbon. Agric. Ecosyst. Environ. 164, 80–99. doi:10.1016/j.agee.2012.10.001
Tan, S. S. X., and Kuebbing, S. E. (2023). A synthesis of the effect of regenerative agriculture on soil carbon sequestration in Southeast Asian croplands. Agric. Ecosyst. Environ. 349, 108450. doi:10.1016/j.agee.2023.108450
Tao, F., Huang, Y., Hungate, B. A., Manzoni, S., Frey, S. D., Schmidt, M. W. I., et al. (2023). Microbial carbon use efficiency promotes global soil carbon storage. Nature 618, 981–985. doi:10.1038/s41586-023-06042-3
UKRI (2021). UK invests over £30m in large-scale greenhouse gas removal. Available online at: https://www.ukri.org/news/uk-invests-over-30m-in-large-scale-greenhouse-gas-removal/(Accessed July 17, 2024).
UNEP (2019). Putting carbon back where it belongs: the potential of carbon sequestration in the soil - foresight brief No. 013 may 2019. Available online at: https://wedocs.unep.org/20.500.11822/28453.
UNFCCC (2024). Glossary. Available online at: https://unfccc.int/resource/cd_roms/na1/ghg_inventories/english/8_glossary/Glossary.htm.
UNFPA (2023). World population prospects 2022: summary of results. Available online at: https://www.un.org/develop%02ment/desa/pd/sites/www.un.org.development.
USDA (2021). USDA launches first phase of soil carbon monitoring efforts through conservation reserve program initiative. Available online at: https://www.fsa.usda.gov/news-room/news-releases/2021/usda-launches-first-phase-of-soil-carbon-monitoring-efforts-through-conservation-reserve-program-initiative (Accessed July 17, 2024).
van Eck, N. J., and Waltman, L. (2010). Software survey: VOSviewer, a computer program for bibliometric mapping. Scientometrics 84, 523–538. doi:10.1007/s11192-009-0146-3
Vaz, C. R., Rauen, T. R. S., and Lezana, Á. G. R. (2017). Sustainability and innovation in the automotive sector: a structured content analysis. Sustainability 9, 880. doi:10.3390/su9060880
Vieira, E. S., Cerdeira, J., and Teixeira, A. A. C. (2022). Which distance dimensions matter in international research collaboration? A cross-country analysis by scientific domain. J. Informetr. 16, 101259. doi:10.1016/j.joi.2022.101259
Viscarra Rossel, R. A., Webster, R., Zhang, M., Shen, Z., Dixon, K., Wang, Y., et al. (2024). How much organic carbon could the soil store? The carbon sequestration potential of Australian soil. Glob. Chang. Biol. 30, e17053. doi:10.1111/gcb.17053
Wall, D. H., Nielsen, U. N., and Six, J. (2015). Soil biodiversity and human health. Nature 528, 69–76. doi:10.1038/nature15744
Wang, C., and Kuzyakov, Y. (2024). Soil organic matter priming: the pH effects. Glob. Chang. Biol. 30, e17349. doi:10.1111/gcb.17349
Wang, C., Qu, L., Yang, L., Liu, D., Morrissey, E., Miao, R., et al. (2021). Large-scale importance of microbial carbon use efficiency and necromass to soil organic carbon. Glob. Chang. Biol. 27, 2039–2048. doi:10.1111/gcb.15550
Wang, Y., Tao, F., Chen, Y., and Yin, L. (2022). Interactive impacts of climate change and agricultural management on soil organic carbon sequestration potential of cropland in China over the coming decades. Sci. Total Environ. 817, 153018. doi:10.1016/j.scitotenv.2022.153018
Wang, Z., Zhao, Y., and Wang, B. (2018). A bibliometric analysis of climate change adaptation based on massive research literature data. J. Clean. Prod. 199, 1072–1082. doi:10.1016/j.jclepro.2018.06.183
Waqas, M. A., Kaya, C., Riaz, A., Farooq, M., Nawaz, I., Wilkes, A., et al. (2019). Potential mechanisms of abiotic stress tolerance in crop plants induced by thiourea. Front. Plant Sci. 10, 1336. doi:10.3389/fpls.2019.01336
Waqas, M. A., Li, Y., Smith, P., Wang, X., Ashraf, M. N., Noor, M. A., et al. (2020). The influence of nutrient management on soil organic carbon storage, crop production, and yield stability varies under different climates. J. Clean. Prod. 268, 121922. doi:10.1016/j.jclepro.2020.121922
Welch, R. M., and Graham, R. D. (2004). Breeding for micronutrients in staple food crops from a human nutrition perspective. J. Exp. Bot. 55, 353–364. doi:10.1093/jxb/erh064
Wernberg, T., Thomsen, M. S., Baum, J. K., Bishop, M. J., Bruno, J. F., Coleman, M. A., et al. (2024). Impacts of climate change on marine foundation species. Ann. Rev. Mar. Sci. 16, 247–282. doi:10.1146/annurev-marine-042023-093037
Wieder, W. R., Bonan, G. B., and Allison, S. D. (2013). Global soil carbon projections are improved by modelling microbial processes. Nat. Clim. Chang. 3, 909–912. doi:10.1038/nclimate1951
Wiesmeier, M., Urbanski, L., Hobley, E., Lang, B., von Lützow, M., Marin-Spiotta, E., et al. (2019). Soil organic carbon storage as a key function of soils - a review of drivers and indicators at various scales. Geoderma 333, 149–162. doi:10.1016/j.geoderma.2018.07.026
Wong, S. L., Nyakuma, B. B., Wong, K. Y., Lee, C. T., Lee, T. H., and Lee, C. H. (2020). Microplastics and nanoplastics in global food webs: a bibliometric analysis (2009–2019). Mar. Pollut. Bull. 158, 111432. doi:10.1016/j.marpolbul.2020.111432
Wood, S. A., Tirfessa, D., and Baudron, F. (2018). Soil organic matter underlies crop nutritional quality and productivity in smallholder agriculture. Agric. Ecosyst. Environ. 266, 100–108. doi:10.1016/j.agee.2018.07.025
Wu, F., and Pfenninger, S. (2023). Challenges and opportunities for bioenergy in Europe: national deployment, policy support, and possible future roles. Bioresour. Technol. Rep. 22, 101430. doi:10.1016/j.biteb.2023.101430
Yadav, S., Sonkar, V., and Malyan, S. K. (2023). in Soil carbon sequestration strategies: application of biochar an option to combat global warming BT - bio-inspired land remediation. Editor V. C. Pandey (Cham: Springer International Publishing), 353–374. doi:10.1007/978-3-031-04931-6_14
Yin, C., Zhao, W., and Pereira, P. (2022). Soil conservation service underpins sustainable development goals. Glob. Ecol. Conserv. 33, e01974. doi:10.1016/j.gecco.2021.e01974
Zhang, H., Fang, Y., Zhang, B., Luo, Y., Yi, X., Wu, J., et al. (2022). Land-use-driven change in soil labile carbon affects microbial community composition and function. Geoderma 426, 116056. doi:10.1016/j.geoderma.2022.116056
Zhang, X., Shen, S., Xue, S., Hu, Y., and Wang, X. (2023). Long-term tillage and cropping systems affect soil organic carbon components and mineralization in aggregates in semiarid regions. Soil Tillage Res. 231, 105742. doi:10.1016/j.still.2023.105742
Zheng, T., Miltner, A., Liang, C., Nowak, K. M., and Kästner, M. (2023). Turnover of bacterial biomass to soil organic matter via fungal biomass and its metabolic implications. Soil Biol. biochem. 180, 108995. doi:10.1016/j.soilbio.2023.108995
Zhou, W., Kou, A., Chen, J., and Ding, B. (2018). A retrospective analysis with bibliometric of energy security in 2000–2017. Energy Rep. 4, 724–732. doi:10.1016/j.egyr.2018.10.012
Zhu, Z., Ge, T., Luo, Y., Liu, S., Xu, X., Tong, C., et al. (2018). Microbial stoichiometric flexibility regulates rice straw mineralization and its priming effect in paddy soil. Soil Biol. biochem. 121, 67–76. doi:10.1016/j.soilbio.2018.03.003
Zimnicki, T., Boring, T., Evenson, G., Kalcic, M., Karlen, D. L., Wilson, R. S., et al. (2020). On quantifying water quality benefits of healthy soils. Bioscience 70, 343–352. doi:10.1093/biosci/biaa011
Keywords: bibliometric analysis, croplands, carbon sequestration, sustainable development, soil health, research hotspots, vosviewer
Citation: Abrar MM, Waqas MA, Mehmood K, Fan R, Memon MS, Khan MA, Siddique N, Xu M and Du J (2025) Organic carbon sequestration in global croplands: evidenced through a bibliometric approach. Front. Environ. Sci. 13:1495991. doi: 10.3389/fenvs.2025.1495991
Received: 13 September 2024; Accepted: 11 March 2025;
Published: 07 April 2025.
Edited by:
Xuejuan Bai, Hebei Normal University, ChinaReviewed by:
Vikas Sharma, Sant Baba Bhag Singh University, IndiaCopyright © 2025 Abrar, Waqas, Mehmood, Fan, Memon, Khan, Siddique, Xu and Du. This is an open-access article distributed under the terms of the Creative Commons Attribution License (CC BY). The use, distribution or reproduction in other forums is permitted, provided the original author(s) and the copyright owner(s) are credited and that the original publication in this journal is cited, in accordance with accepted academic practice. No use, distribution or reproduction is permitted which does not comply with these terms.
*Correspondence: Jianjun Du, amlhbmp1bmR1QDEyNi5jb20=; Muhammad Mohsin Abrar, cmFuZGhhd2FzYWJAaG90bWFpbC5jb20=
Disclaimer: All claims expressed in this article are solely those of the authors and do not necessarily represent those of their affiliated organizations, or those of the publisher, the editors and the reviewers. Any product that may be evaluated in this article or claim that may be made by its manufacturer is not guaranteed or endorsed by the publisher.
Research integrity at Frontiers
Learn more about the work of our research integrity team to safeguard the quality of each article we publish.