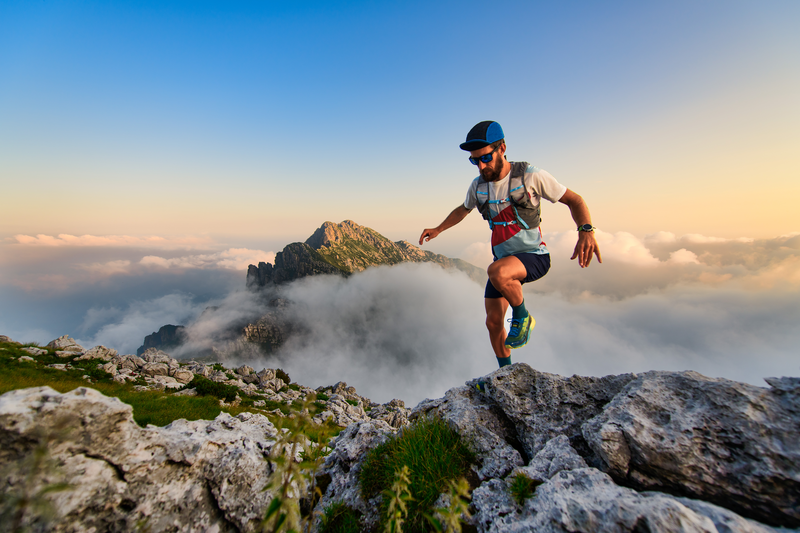
94% of researchers rate our articles as excellent or good
Learn more about the work of our research integrity team to safeguard the quality of each article we publish.
Find out more
ORIGINAL RESEARCH article
Front. Environ. Sci. , 10 January 2025
Sec. Ecosystem Restoration
Volume 12 - 2024 | https://doi.org/10.3389/fenvs.2024.1538243
This article is part of the Research Topic Ecosystem Services and Sustainable Restoration Interlinking Soil, Geological, and Vegetation Interactions for Sustainable Development View all articles
Introduction: This study evaluates the effectiveness of soil reconstruction and restoration in the Jiangcang coal mining area on the Qinghai-Tibet Plateau, where harsh environmental conditions pose significant challenges to ecological restoration.
Methods: Two phases of ecological restoration were implemented, with outcomes assessed based on vegetation coverage, species diversity, biomass, soil properties, and community similarity.
Results: The results demonstrate that soil reconstruction significantly improved soil fertility, vegetation coverage, and community stability without noticeable degradation over time. The use of sheep manure increased species diversity by introducing native seeds, addressing the shortage of suitable grass species in alpine areas. Comparatively, the second phase of restoration, which included soil reconstruction, has elevated the vegetation coverage to 80%, matching natural background levels, and has also demonstrated superior outcomes in terms of soil stability, nutrient content, and other aspects compared to traditional methods. While aboveground biomass showed rapid recovery, belowground biomass lagged, indicating a need for longer-term restoration. Restored slopes exhibited higher similarity to natural alpine meadows compared to platforms, suggesting the dominance of the artificially seeded species on the platform areas hinders the reproduction of other species, which is unfavorable for the evolution of vegetation diversity.
Discussion: This study emphasizes the effectiveness of soil reconstruction, organic amendment, and other restoration measures, providing important experience and reference for mine ecological restoration in similar high-altitude mining areas.
The Qinghai-Tibet Plateau is known for its harsh climatic conditions, high altitudes, and fragile ecosystems, which make ecological restoration efforts particularly challenging (Carabassa et al., 2020; Cheng et al., 2022). Mining activities in this region have caused severe ecological damage, particularly in high-altitude areas where permafrost and alpine meadow ecosystems are widespread (Zhang et al., 2023; Hu et al., 2018). The Muli coal mine, located at the northeastern edge of the Qinghai-Tibet Plateau, is the largest coal mine in Qinghai Province and a significant coking coal resource in northwest China (He et al., 2024). Decades of open-pit mining have led to substantial environmental degradation, including the destruction of water, soil, and vegetation resources, as well as the thawing of permafrost, which has further contributed to the degradation of alpine meadows and increased soil erosion (Reid and Naeth, 2005a; Misebo et al., 2022). The permafrost degradation not only reduces the stability of the soil but also negatively impacts the region’s hydrological balance, making effective ecological restoration a critical need (Rocha and Martins, 2021; Scotton, 2021).
In recent years, the Chinese government has increased its emphasis on ecological restoration and environmental protection, with specific policies targeting mining areas in the Qinghai-Tibet Plateau. As part of this effort, numerous projects have been launched to restore vegetation and improve soil quality in these degraded regions (Wong, 2003; Yang et al., 2019). The unique climatic conditions, such as short growing seasons, low temperatures, and limited precipitation, further complicate restoration efforts, necessitating the development of adaptive restoration techniques tailored to these extreme environments (Yang et al., 2019; Zhang et al., 2023). High-altitude mining areas face severe soil degradation, loss of vegetation, and destabilized ecosystems, which exacerbate ecological fragility in regions like the Qinghai-Tibet Plateau (Zheng et al., 2023; Lu et al., 2024). Recent studies have highlighted the importance of integrating soil amendments, particularly organic inputs, to facilitate vegetation establishment and improve soil quality. For example, soil organic amendments, such as sheep manure and compost, can significantly enhance soil structure, fertility, and microbial biomass, providing essential support for plant growth (Worlanyo and Jiangfeng, 2021; Liu et al., 2022). Research on alpine mining areas in regions with similar conditions, such as the Andes and parts of Central Asia, has demonstrated the importance of selecting site-specific strategies for soil and vegetation restoration (Lim et al., 2022). Techniques like hydroseeding, the use of erosion control mats, and mixed seeding of native grass and shrub species have shown positive results in improving vegetation coverage and biodiversity (Wang et al., 2018; Penalver-Alcala et al., 2021). However, much of the research in China has focused on the Juhugeng mining area (Zhong et al., 2021; Feng et al., 2023), while restoration studies specifically targeting the Jiangcang mining area remain limited.
Over the past decade, there has been significant progress in understanding the effects of mining activities on fragile ecosystems and developing restoration techniques. Studies have shown that soil reconstruction and the use of native plant species can enhance the recovery of disturbed ecosystems in high-altitude regions (Feng et al., 2024). Soil reconstruction involving the amendment of soil with organic materials, such as sheep manure, has been shown to significantly improve soil fertility and aid in the establishment of vegetation in degraded mining areas (Cross and Lambers, 2017). Research in other regions with similar climatic conditions has highlighted the effectiveness of organic amendments in enhancing soil microbial activity and improving soil structure, which are critical for successful restoration (Luna et al., 2018; Figueiredo et al., 2024). However, most research has focused on the Juhugeng mining area, while studies on the Jiangcang mining area remain limited (Naeth and Wikinson, 2014).
Additionally, the use of remote sensing and GIS technologies has advanced the monitoring of vegetation recovery in mining areas, allowing researchers to assess changes in vegetation coverage and landscape patterns over time (Courtney et al., 2011; Pardo et al., 2014). These technological advancements have enabled more accurate assessments of restoration effectiveness and have highlighted the importance of long-term monitoring to understand the dynamics of ecosystem recovery (He et al., 2024). Despite these advancements, there remains a lack of comprehensive studies that integrate both aboveground and belowground ecological indicators to evaluate restoration success in the Qinghai-Tibet Plateau’s mining areas (Heiskanen et al., 2022; Kim et al., 2020).
Although some progress has been made in relevant research, significant gaps still exist in current studies. Many studies have primarily concentrated on the short-term effects of ecological restoration, with limited focus on the long-term sustainability of restored ecosystems (Kim et al., 2020). In particular, the underground biomass recovery, which is crucial for long-term ecosystem stability, has not been adequately addressed (Jambhulkar and Kumar, 2019). The recovery of belowground biomass plays a key role in maintaining soil structure, enhancing nutrient cycling, and supporting long-term vegetation stability (Reid and Naeth, 2005b; Lupton et al., 2013). Moreover, few studies have explored the effects of different restoration techniques in the specific context of the Qinghai-Tibet Plateau, where permafrost and high-altitude conditions pose unique challenges (Zhou et al., 2015; Rothman et al., 2021). There is also a need for research that evaluates the impact of different types of organic amendments and their effectiveness under varying environmental conditions in high-altitude ecosystems (Yang et al., 2016).
In this study, we address these gaps by evaluating the long-term effectiveness of soil reconstruction and ecological restoration techniques in the Jiangcang mining area of the Muli coal mine. Our research focuses on comparing two phases of ecological restoration, assessing parameters such as vegetation coverage, species diversity, biomass, and soil stability. Our hypotheses are that soil reconstruction techniques using organic amendments can significantly enhance soil fertility and vegetation recovery in high-altitude mining areas, and that the use of native plant species will lead to greater ecosystem stability and resilience compared to traditional restoration methods. We utilized organic amendments, including sheep manure, and a mixed seeding approach using native species to facilitate the restoration process. By monitoring changes over time, we aim to provide insights into the effectiveness of these restoration techniques in enhancing ecosystem resilience under harsh environmental conditions. Our approach also integrates both aboveground and belowground ecological indicators to provide a comprehensive understanding of restoration success (Williams and Thomas, 2023). The Objectives of this study is (1) to evaluate the effectiveness of soil reconstruction techniques in improving soil fertility and vegetation recovery in the Jiangcang mining area, (2) to compare the restoration outcomes of different ecological restoration approaches, including aboveground and belowground ecological indicators, and (3) to assess the long-term sustainability of restored ecosystems under high-altitude, harsh environmental conditions. The significance of this study lies in its contribution to understanding the long-term dynamics of ecological restoration in high-altitude mining areas. Our findings highlight the importance of soil reconstruction and the use of native species for successful restoration outcomes. This research also provides valuable insights into the challenges and potential solutions for restoring ecosystems in regions characterized by permafrost and alpine climates, which are particularly vulnerable to environmental degradation (Gao et al., 2019). The results of this study can inform future restoration projects in similar environments, contributing to the broader goal of achieving sustainable ecological management in high-altitude, fragile ecosystems.
The study area is located in the Jiangcang mining area of the Muli coal mine, situated in Tianjun County, Haixi Mongolian and Tibetan Autonomous Prefecture, Qinghai Province, China. The region lies between approximately 97°30′to 100°00′E longitude and 37°30′to 39°00′N latitude, covering a total area of 17,800 ha. It is bounded approximately 110 km southeast of Gangcha County, 45 km west of the Juhugeng mining area, and 150 km southwest of Tianjun County (Figure 1). The study area includes multiple mining sites, such as Jiangcang No. 1, No. 2, No. 4, and No. 5 pits, as well as several waste rock dumps. The region is characterized by extensive permafrost, with permafrost thickness ranging from 62.5 to 174 m (Table 1) and the active layer typically less than 2 m. Permafrost begins to thaw in April and refreezes by September, while average temperatures in June through August reach above 9°C, providing a short growing season of approximately 90 days that supports revegetation efforts. The annual average precipitation exceeds 450 mm, with 80% of the rainfall occurring between May and September, which is sufficient to meet the water requirements for plant growth and establishment.
Table 1. Depth statistics of the permafrost bottom boundary from near-steady-state logging at boreholes in jiangcang mining area.
The Jiangcang mining area features a typical cold alpine ecosystem, representing the broader Qinghai-Tibet Plateau’s environmental characteristics. The topography is marked by high-altitude ranges, with an elevation between 3,500 m and 4,500 m, averaging at 3,900 m above sea level, making it particularly sensitive to external disturbances like mining, with two main vegetation types: alpine meadow and alpine marsh meadow. These vegetation types are closely linked to the region’s hydrogeological conditions, which control the distribution of plant communities. Alpine marsh meadow dominates areas with permafrost, while alpine meadow is more common in the foreland slopes and thawing regions. Alpine marsh meadow vegetation is typically low-growing and structurally simple, with dominant species including Kobresia tibetica and Carex orbicularis. Associated species include Carex moorcroftii, Carex atrata, Allium przewalskianum, Polygonum viviparum, and other perennial herbs that thrive in cold, moist conditions. Alpine meadow vegetation, in contrast, is dominated by Artemisia species, which are characterized by their low, dense growth habit and their ability to withstand harsh conditions, with species such as Artemisia nanschanica, Pedicularis kansuensis Maxim., and Elymus nutans being prevalent.
The mining activities have created six large excavation pits that have severely disturbed the original landscape and surface vegetation. Open-pit mining has generated significant amounts of waste rock and spoil, which are deposited in layers around the pits, forming extensive waste rock dumps with heights ranging from several meters to over 50 m. This has caused severe damage to the landscape and vegetation, particularly affecting alpine meadows in the vicinity of pits, dumps, roads, and industrial sites, leading to extensive degradation of meadow habitats. Additionally, mining activities have disrupted the permafrost, resulting in the formation of groundwater “windows” that allow groundwater to flow into the pits, leading to extensive ponding and altering the freeze-thaw dynamics. Given the area’s high-altitude conditions and short growing season, ecological restoration in this region is particularly challenging, requiring careful management to ensure successful revegetation and ecosystem recovery.
The Jiangcang mining area was discovered in the 1950s, with initial exploration activities conducted in 1956. The coal deposits are characterized by shallow burial with thin Quaternary unconsolidated sediments overlying or directly exposed at the surface, forming a partially concealed coalfield. Following the increased demand for energy and the prosperity of the coal market after 2002, several coal enterprises, including Qinghai Qinghua, Qinghai Aokai, Qinghai Jiangcang Energy, and Qinghai Coking Coal, began mining activities in the Jiangcang area. Mining commenced in 2003, with Pits No. 1, No. 2, No. 4, and No. 5 brought into production, while Pit No. 3 remained undeveloped. Open-pit mining was the primary extraction method used, and peak mining activity occurred between 2008 and 2019. Overburden and coal gangue were disposed of in stepped layers around the excavation pits, causing significant topographic changes and extensive land occupation.
Two major ecological restoration initiatives have been undertaken in the study area to date. The first large-scale restoration effort took place from 2014 to 2016, focusing on reshaping 19 waste rock dumps formed by open-pit mining and establishing vegetation. The restoration techniques included slope reshaping, organic fertilizer application, mixed seeding of grass species, and covering with non-woven fabric. These methods were relatively traditional and showed limited success in restoring meadow vegetation.
The second large-scale restoration was conducted from 2020 to 2021, building on the lessons learned from the earlier efforts. This phase aimed at improving restoration outcomes by reconstructing the soil profile, using the native soil as a reference. Restoration measures for Pits No. 4 and No. 5 included slope reshaping, screening of waste rock to separate usable soil, mixing of screened soil with sheep manure and slow-release fertilizers, mixed seeding of grass species, and covering with coir fiber blankets. This combination was designed to improve soil fertility and enhance vegetation establishment.
In the study area, specific steps in the second restoration included.
(1) Slope Reshaping: Mechanical reshaping of the terrain to a slope gradient of less than 25°, with larger stones placed at the bottom and finer particles at the top. Utilize the self-weight of machinery for compaction to prevent large-scale settlement of the landform after shaping.
(2) Soil Screening: The waste rock underwent on-site screening, where gravel was crushed by excavators. Subsequently, the soil was double-turned to guarantee uniformity of particles, and any rocks exceeding 5 cm in size were removed using a stone picker. This screened soil was then utilized as the foundation for the reconstructed soils.
(3) Soil Amendment with Sheep Manure and Slow-Release Fertilizer: The soil was evenly mixed with 495 m3 of sheep manure and 22.5 tons of slow-release fertilizer per hectare. Given that plant roots primarily occupy the top 20 cm of soil, and accounting for an anticipated 5–10 cm of natural subsidence, the final soil layer thickness was set at 25 cm.
(4) Mixed Seeding of Grass Species:Obtaining wild grass seeds in plateau and high-altitude areas is challenging. After conducting an analysis of the market supply capacity of grass seeds and ensuring no exotic species were introduced, E. nutans, Poa pratensis var. Qinghaiensis, Festuca sinensis, and Kentucky bluegrass were ultimately chosen as the grass species for sowing. These species were mixed at a ratio of 1:1:1:1, with a total seeding rate of 180 kg per hectare.
(5) Coir Fiber Blanket Covering: The surface was covered with coir fiber blankets to retain moisture, prevent surface runoff, and reduce nutrient loss, ultimately promoting successful revegetation.
According to remote sensing interpretation results from 2002 to 2022, the area of land used for mining activities in the study area increased annually from 2003 to 2019, reaching a peak of 3,085.74 ha in 2019. This expansion came at the cost of reducing surrounding grasslands and bare land, with losses amounting to 11,183.26 ha and 3,667.88 ha, respectively, approximately five times the increase in mining land. Since the initiation of the second ecological restoration action in 2020, the area used for mining activities has gradually decreased to 1,911.78 ha, with a total restoration area of approximately 1,000 ha. Concurrently, the area of low-coverage grassland has recovered by 2,184.3 ha compared to 2015.
This study focuses on the Jiangcang mining area to evaluate the effectiveness of soil reconstruction and revegetation. Currently, the area has undergone complete revegetation and supplementary improvements in treated zones. Areas that could fully reflect the plant species and growth conditions under different restoration measures were selected as sampling plots. In total, 10 sampling points were set up, distributed across the waste rock platforms and slopes of Pits No. 4 and No. 5 (from the second restoration phase), the south waste dump platform of Pit No. 2 (from the first restoration phase), and the natural background area. The specific distribution of vegetation survey sampling points is shown in Figure 2.
The plot size for herbaceous vegetation was preliminarily set to 1 m × 1 m, considering the growth status of the vegetation and terrain. The sample size was selected to adequately represent the characteristics of the vegetation while ensuring ease of observation and sampling. The survey data collected from vegetation plots included weather, elevation, coordinates, plant species, dominant species, abundance, biomass, average height, average coverage, average crown width, and growth status for each sampling point. These data were used for a comprehensive assessment of the vegetation ecosystem in the study area. The main survey indicators are detailed in Table 2.
Species diversity reflects the complexity of species composition within a community. In this study, we employed the Shannon diversity index, Pielou’s evenness index, and Margalef’s richness index to quantify and assess this diversity. The specific calculation formulas for these indices are as follows:
Where Pi represents the percentage of the number of species i in the entire sample plot; S denotes the total number of species; and N stands for the total number of individuals of all species.
The Sorensen community similarity coefficient represents the degree of similarity between different community structures, indicating the extent of species overlap between sampling areas. It is utilized to assess the differences among communities and is calculated using the following formula:
Where C is the number of species shared between communities S1 and S2, and SC is the similarity coefficient between the communities.
Vegetation coverage in the mining area from 2020 to August 2024 was calculated using Sentinel data and the mixed pixel decomposition method. In remote sensing imagery, each pixel generally comprises multiple components, each contributing to the composite signal observed. To estimate vegetation coverage, a pixel decomposition model was applied. The specific calculation formula for this model is as follows:
Where: FVC is Fractional Vegetation Coverage; NDVI is NDVI value of the mixed pixel; NDVIsoil is NDVI value of a pure bare soil pixel; NDVIveg is NDVI value of a pure vegetation pixel.
Statistical analyses were performed using SPSS 26.0 and R 4.2.1. Descriptive statistics were calculated to summarize soil properties and vegetation attributes. Differences among treatments (restored pits No. 4, No. 5, No. 2, and control) were analyzed using one-way ANOVA, with post hoc tests (Tukey’s HSD) for pairwise comparisons. t-tests were used to compare specific groups (e.g., restored vs control). Pearson correlation analysis was conducted to examine relationships between soil physicochemical properties and vegetation parameters. Network correlation analysis was employed to visualize the interactions among soil and vegetation variables. Statistical significance was set at p < 0.05.
An analysis of plant community species surveys in the alpine grassland of the study area revealed that vegetation in mining pits and waste rock dumps was almost entirely destroyed following mining activities. After the restoration and revegetation efforts, approximately 20 plant species were identified in the sampling plots. Besides the four artificially planted species—E. nutans, P. pratensis var. Qinghaiensis, F. sinensis, and K. bluegrass—the natural accompanying vegetation included species such as P. kansuensis Maxim., Astragalus polycladus Bur. Et Franch., Oxytropis ochrocephala Bunge, Elsholtzia densa Benth., Androsace umbellata, Polygonum sibiricum, Microula sikkimensis (C. B. Clarke) Hemsl., Capsella bursa-pastoris, etc., showing relatively rich vegetation diversity. The untreated areas within the mining zone were largely devoid of vegetation. The primary dominant species in the rehabilitated areas were the four artificially planted species. Other natural species present in the area likely germinated from seeds carried by sheep manure used in the restoration, indicating that the use of sheep manure played a significant role in the natural regeneration and establishment of wild species that are otherwise difficult to obtain.
Based on Sentinel data from 2020 to August 2024, the vegetation coverage (Fractional Vegetation Cover, FVC) of Jiangcang mining area, specifically Pits No. 4, No. 5, and the south waste dump of Pit No. 2, along with surrounding reference areas, was analyzed, and the average changes in vegetation coverage in these four regions were recorded (Figure 3). The results show that the vegetation coverage in Jiangcang Pit No. 4 initially increased, then decreased, followed by another increase, with a peak in 2021 at 77.45%. In 2020, the vegetation coverage of Pit No. 4 was 53.21%. After the second round of restoration and revegetation efforts, the coverage remained above 70% from 2021 to 2024, indicating that the restoration efforts were effective, and the ecological conditions improved significantly compared to 2020. The trends in Pit No. 5 were similar to those in Pit No. 4. In 2020, the vegetation coverage of Pit No. 5 was 54.37%, which peaked at 72.75% in 2021. By 2024, the vegetation coverage had increased by 16.32% compared to 2020, suggesting successful restoration over the past 5 years. The south waste dump of Pit No. 2 was restored using traditional revegetation measures in 2015. From 2020 to 2024, the average vegetation coverage remained around 50%, with little change, indicating that the effectiveness of the first restoration was limited. The background area of Jiangcang mining site showed average vegetation coverage values ranging from 77% to 84% between 2020 and 2024, indicating generally good ecological conditions. Overall, the vegetation coverage across the mining area initially increased and then decreased, with a peak in 2021 at 83.26%. Afterward, the coverage slightly decreased and stabilized around 80%. All regions showed a peak in vegetation coverage in 2021. A comparison with precipitation data indicated that there was no significant increase in rainfall in 2021, suggesting that the extended vegetation growing period due to a shorter freeze period may have contributed to the higher vegetation coverage observed that year. According to remote sensing interpretation results from 2002 to 2022, the area of land used for mining activities in the study area increased annually from 2003 to 2019, reaching a peak of 3,085.74 ha in 2019. This expansion came at the cost of reducing surrounding grasslands and bare land, with losses amounting to 11,183.26 ha and 3,667.88 ha, respectively, approximately five times the increase in mining land. Since the initiation of the second ecological restoration action in 2020, the area used for mining activities has gradually decreased to 1,911.78 ha, with a total restoration area of approximately 1,000 ha. Concurrently, the area of low-coverage grassland has recovered by 2,184.3 ha compared to 2015.
Figure 3. Changes in average vegetation coverage from 2020 to 2024. (A) No.5. (B) No.4. (C) No.2. (D) Control treatment.
The second phase of restoration in Jiangcang Pits No. 4 and No. 5 was largely completed between 2020 and the first half of 2021. Vegetation in the restoration areas quickly recovered during 2021. To further investigate whether the vegetation could safely overwinter and the sustainability of restoration effects, this study analyzed vegetation coverage trends from 2021 to 2024, as shown in Figure 4.
The vegetation evolution trend in Jiangcang Pit No. 4 from 2021 to 2024 shows an overall increase in vegetation coverage. The area with no significant increase was 1.77 km2, accounting for 70.80% of the mining area. The area with slightly significant and significant increases combined was 0.35 km2, covering 13.92% of the mining area, mainly distributed in the eastern and central parts of Pit No. 4. The area with no significant decrease was 0.27 km2, representing 10.67% of the mining area, mainly in the northern and central parts of the mining area, with scattered distribution. The combined area of significant and slightly significant decreases was only 0.0032 km2, accounting for just 0.14% of the mining area. The area with no change was 0.11 km2, covering 4.48% of the mining area, mainly concentrated in the central part of Pit No. 4.
In Jiangcang Pit No. 5, the trend from 2021 to 2024 shows a decrease in vegetation coverage of 0.42 km2 and an increase of 2.24 km2, accounting for 17.66% and 76.57% of the mining area, respectively. The area with no significant increase was the largest, accounting for more than 60% of the mining area. The area with no significant decrease was 0.48 km2, representing 16.28% of the mining area, mainly in the central and northern parts of the mining area. Significant decreases were mainly distributed in the central-western parts of the mining area, accounting for only 0.31% of the total area. Significant increases were observed around the central mining area, covering 4.37% of the total area. Areas with no change in vegetation coverage were mainly located in the southwestern part of the mining area, with an area of 0.13 km2.
The vegetation evolution trends indicate that following restoration using soil reconstruction methods, although the growing season in 2021 was slightly shortened due to restoration construction, newly established vegetation was minimally affected by winter temperatures. Over the following 3 years, vegetation did not show significant degradation, with areas showing significant or slightly significant increases in vegetation coverage accounting for more than 70% of the total mining area. This suggests that the vegetation evolution trends after restoration did not show obvious degradation, and the ecological environment of the mining area significantly improved, trending toward favorable recovery.
Based on the 2022 biomass data from sampling points in the study area, the aboveground and belowground biomass of the natural alpine meadow background area remained higher than that of the restored waste rock platforms and slopes. The belowground biomass of the natural grassland was approximately four times that of the aboveground biomass. Figure 5 shows that after the second restoration, the aboveground biomass in Pits No. 4 and No. 5 was significantly higher than that in Pit No. 2, but still lower than that of the natural vegetation, which is highly correlated with the vegetation coverage of the sampling points. Figure 6 illustrates that belowground biomass showed little difference between the two restoration phases, both being significantly lower than the natural grassland background value, indicating that belowground biomass recovery requires long-term accumulation. Notably, the restoration in Pit No. 2 was conducted in 2015, and after 7 years of belowground biomass accumulation, there was little difference compared to Pits No. 4 and No. 5, restored in 2021, further demonstrating the effectiveness of the second soil reconstruction measures.
Statistical analysis of plant community crown width, height, abundance, and coverage in different areas of the north waste rock dump in Pit No. 5 is presented in Table 3. The natural alpine meadow background area is characterized by moderate crown width, low grass clusters, moderate abundance, and high coverage. The revegetated waste rock platform had higher crown width and height, with significant variability in crown width and diverse plant species distribution. The vegetation on the sunny slope of the waste rock dump exhibited lower crown width and abundance, with a wide range of coverage and significant differences in community structure. This indicates that the waste rock platform, with better water and nutrient conditions, supports rapid growth of the restored grass species (mainly Poaceae), resulting in height and crown width that even exceed those in the background area. In contrast, the sunny slope area showed poorer restoration outcomes due to limited water and nutrient availability compared to the platform area.
In-depth analysis of plant community diversity indices for the north waste rock dump in Pit No. 5 revealed that the natural grassland background area had the highest diversity, evenness, and richness indices, indicating a stable community structure with rich and evenly distributed plant species. The sunny slope of the waste rock dump also exhibited relatively high diversity, evenness, and richness indices, suggesting a diverse and evenly distributed plant community. In contrast, the platform area performed the worst in terms of species diversity. Table 4 data indicate that the restored area still requires a long period of natural succession to reach the diversity levels of the background area. The slope area showed better diversity than the platform area, likely due to the dominance of Poaceae pioneer species on the platform, which inhibited the propagation of other species. In contrast, the weaker dominance of species on the sunny slope provided more opportunities for other species to grow, resulting in better diversity.
As shown in Table 5, the similarity between the sunny slope of the waste rock dump and the natural grassland background area was relatively high after artificial restoration, indicating that revegetated slopes are more likely to return to a natural grassland community structure. However, the similarity between the platform and the natural grassland was lower, suggesting that the associated original grassland species are less prevalent on the platform, and it will take longer to restore to a natural state.
The soil properties of the restored mining areas (No. 5, No. 4, No. 2 pits) and the control treatment were analyzed and compared, including bulk density (BD), soil water content, soil organic carbon (SOC), pH, electrical conductivity (EC), total nitrogen (TN), total phosphorus (TP), total potassium (TK), and particle size distribution (clay, silt, and sand). Figure 7A shows that soil bulk density (BD) is higher in the restored areas (No. 5 and No. 4) compared to Pit No. 2 and the control treatment. The control treatment has the lowest BD, indicating less compaction compared to the reclaimed mining areas. This suggests that soil compaction may still be an issue in the reclaimed areas, potentially hindering root growth and overall soil quality improvement. In terms of soil water content, as depicted in Figure 7B, the control treatment exhibited significantly higher water content compared to all restored areas. The water content was lowest in Pit No. 2, suggesting that this area may have limited water retention capacity, likely due to inadequate soil structure or insufficient organic amendments during restoration. Figure 7C shows that soil organic carbon (SOC) levels are substantially higher in the control treatment compared to the restored areas, particularly in Pit No. 2, which has the lowest SOC among all the areas. This indicates that the organic matter content in the restored soils is not yet comparable to the control, which might affect soil fertility and microbial activity. Figure 7D indicates similar pH levels across all treatments, suggesting that soil acidity is relatively consistent regardless of the restoration efforts. For the nutrient content, total nitrogen (TN) depicted in Figure 7E is significantly higher in the control compared to the restored sites, with Pit No. 2 showing the lowest nitrogen content. This highlights the challenge of replenishing soil nitrogen in reclaimed mining areas. Total phosphorus (TP) levels in Figure 7F are slightly higher in Pits No. 5 and No. 4 compared to Pit No. 2, though all remain lower than the control. Figure 7G shows that total potassium (TK) is relatively stable across all areas, with no substantial differences observed. Figure 7H shows that the electrical conductivity in the restored areas is higher than the control treatment, which is speculated to be due to the effect of the slag in the waste rock dump.
Figure 7. Comparison of soil properties among restored mining pits (No. 5, No. 4, No. 2) and control treatment. (A) Soil Bulk Density; (B) Soil Water Content; (C) Soil Organic Carbon; (D) pH; (E) Total Nitrogen; (F) Total Phosphorus; (G) Total Potassium; (H) Electrical Conductivity; (I) Clay Content; (J) Slit Content; (K) Sand Content.
The particle size distribution analysis (Figures 7I–K) reveals variations in clay, silt, and sand contents. The clay content (Figure 7I) is highest in Pit No. 5, which suggests better aggregation and potentially improved soil stability, while Pit No. 2 has the lowest clay content. Silt content (Figure 7J) is also lowest in Pit No. 2, indicating poor soil texture compared to other areas. Conversely, sand content (Figure 7K) is highest in Pit No. 2, which could be contributing to the lower water retention and organic matter levels observed.
Overall, the comparison of soil properties across the restored areas and the control treatment shows that while there have been improvements in soil quality in Pits No. 4 and No. 5, the soil in Pit No. 2 still lags in several key properties, such as soil water content, organic carbon, and nutrient levels. The findings suggest that further amendments or different restoration strategies may be needed in Pit No. 2 to enhance its soil properties to levels comparable to the control or other restored areas.
The network correlation analysis provides a visual representation of the relationships between soil physicochemical properties and vegetation attributes across the three restored mining pits (No. 5, No. 4, and No. 2) and the control treatment (Figure 8). In this analysis, the strength and direction of correlations are indicated by the color and thickness of the connecting lines, while the spatial arrangement reflects the relative proximity of interrelated variables. In Pit No. 5, soil organic matter (SOM), total nitrogen (TN), and soil water content (SWC) serve as central nodes in the network, displaying strong positive correlations with belowground biomass (BGB) and vegetation fractional cover (VFC), as indicated by thick green lines. Notably, bulk density (BD) shows a negative relationship with SOM and TN, emphasizing the detrimental impact of soil compaction on soil quality and vegetation attributes. Similarly, sand content exhibits a strong negative correlation with SWC, suggesting its adverse effect on water retention. Pit No. 4 exhibits a similar network structure, with SOM and TN again emerging as key variables positively linked to VFC and BGB. However, clay content displays a stronger correlation with SWC, implying improved soil stability and water-holding capacity in this restored area. Unlike Pit No. 5, the influence of BD is more subdued, indicating lesser soil compaction and enhanced restoration outcomes. In Pit No. 2, the relationships between soil properties and vegetation attributes are less pronounced. While SOM and SWC are still positively correlated with VFC and BGB, the overall network connectivity is weaker, as reflected in the thinner lines. Sand content remains negatively correlated with SWC, and the weaker centrality of SOM and TN highlights the limited soil fertility and restoration progress in this area. The control treatment (Figure 8D) reveals the most interconnected network, where SOM, TN, and SWC act as critical hubs positively influencing vegetation attributes such as aboveground biomass (AGB), BGB, and VFC. The strong positive correlation between clay content and SWC highlights the importance of soil texture in maintaining ecosystem resilience. In contrast, BD exhibits consistent negative correlations with SOM and TN, further corroborating its role as a limiting factor for soil and vegetation quality. The network correlation analysis underscores the pivotal role of SOM, TN, and SWC in supporting vegetation recovery and ecological stability across the restored areas. The control treatment serves as a benchmark, demonstrating the optimal balance between soil properties and vegetation attributes. Among the restored pits, Pit No. 5 exhibits the most promising restoration outcomes, followed by Pit No. 4, while Pit No. 2 requires additional interventions to enhance its soil and vegetation recovery.
Figure 8. Network correlation analysis of soil physicochemical properties and vegetation attributes in restored mining areas and control treatment. (A) No.5. (B) No.4. (C) No.2. (D) Control treatment.
To further understand the interactions between soil physicochemical properties and vegetation recovery in the restored mining areas, a correlation matrix was constructed (Figure 9). The analysis highlights key relationships among soil properties—such as pH, total nitrogen (TN), soil organic matter (SOM), and soil texture components (sand, silt, clay)—and vegetation attributes, including aboveground biomass (AGB), belowground biomass (BGB), and vegetation fractional cover (VFC).
Figure 9. Correlation matrix of soil physicochemical properties and vegetation attributes in the restored mining areas.
Figure 9 illustrates a strong positive correlation between SOM and TN (r = 0.93) and between SOM and soil water content (SWC) (r = 0.91), indicating that soils with higher organic matter content have better nitrogen levels and water retention capacity. Similarly, SWC positively correlates with both BGB (r = 0.89) and VFC (r = 0.76), suggesting that enhanced soil moisture availability plays a critical role in promoting root biomass accumulation and vegetation coverage. The analysis also identifies a notable negative correlation between bulk density (BD) and SOM (r = −0.50), as well as BD and SWC (r = −0.41), underscoring the adverse effects of soil compaction on organic matter accumulation and water retention. Furthermore, the proportions of sand, silt, and clay show distinct relationships with soil nutrient dynamics and vegetation growth. For example, clay content positively correlates with SWC (r = 0.64), while sand content exhibits a negative correlation with TN (r = −0.77), indicating that finer soil textures contribute to improved nutrient retention and water availability. Vegetation attributes also reveal significant correlations with soil properties. AGB and BGB are strongly associated with TN (r = 0.83 and r = 0.86, respectively) and SOM (r = 0.86 and r = 0.89, respectively). These findings emphasize the importance of nutrient-rich soils for vegetation recovery. Additionally, VFC correlates positively with SOM (r = 0.77) and SWC (r = 0.76), highlighting the influence of soil organic content and moisture on vegetation coverage and ecosystem restoration.
The overall trend of vegetation coverage in the Jiangcang mining area showed a significant recovery after the second phase of restoration. The vegetation coverage of Pits No. 4 and No. 5 has increased from 50% to approximately 80%,indicating that restoration measures, such as soil reconstruction and mixed seeding of native species, were effective (Wang et al., 2018; van der Bauwhede et al., 2024). However, the comparison with the background area suggests that although vegetation recovery was considerable, it did not fully reach natural levels, especially in the initial years, which has also been reported in other high-altitude restoration projects (Sole et al., 2023; Lu et al., 2024). The increased vegetation coverage can be attributed to the use of native species adapted to the harsh environment, which has been shown to promote ecosystem resilience (Chazdon, 2008; Chaturvedi and Singh, 2017).
The differences in vegetation coverage trends between Pits No. 4, No. 5, and the south waste dump of Pit No. 2 highlight the importance of the restoration methods used. Traditional restoration measures like those in Pit No. 2 often yield limited long-term improvements, while advanced soil reconstruction techniques, including organic amendments, are more effective (Macdonald et al., 2015; He et al., 2024). These findings are consistent with results from similar restoration efforts in North America, where modernized techniques have proven to provide better revegetation outcomes (Petrova et al., 2022). The use of soil amendments, particularly sheep manure, has demonstrated a positive impact on soil moisture retention and nutrient cycling in the restored areas (Asensio et al., 2014). Sheep manure not only provides essential organic matter but also enhances soil structure, which improves water infiltration and retention, particularly in high-altitude environments where precipitation is limited (Aronson et al., 1993; Lim et al., 2022). Additionally, the gradual release of nutrients such as nitrogen, phosphorus, and potassium from manure supports microbial activity and promotes plant growth, thereby strengthening the soil-plant interactions (Lu et al., 2024). These processes collectively accelerate vegetation establishment and improve overall ecosystem stability, as observed in the increased vegetation coverage and biomass in our study. Such findings align with previous research highlighting the role of organic amendments in improving soil water dynamics and nutrient availability, which are critical for restoration success in degraded alpine ecosystems (Moreno-de las Heras, 2009; Liu et al., 2022).
The temporal trend of vegetation coverage peaking in 2021 suggests that climate factors, such as a shorter freeze period, contributed to an extended growing season and thereby enhanced recovery (Li et al., 2013; Pedrol et al., 2014). The interplay between restoration practices and climatic conditions is critical, as evidenced by similar studies in alpine regions in Europe (Madejon et al., 2021), which indicate that adaptive management strategies must consider both the restoration methods and the natural climatic variability to achieve optimal outcomes.
The plant community characteristics, including biomass, crown width, and abundance, showed positive responses to soil reconstruction in Pits No. 4 and No. 5, aligning with studies showing that organic amendments significantly enhance plant productivity in restored ecosystems (Asensio et al., 2013; Yang et al., 2019). Aboveground biomass was notably higher in these pits compared to earlier restoration efforts, though still lower than that of the natural background area (Bardgett et al., 2005). This is in line with global restoration practices where aboveground biomass is often a primary indicator of successful recovery (Bullock et al., 2011; Rocha et al., 2021). Belowground biomass is essential for enhancing soil stability, water retention, and nutrient cycling, forming the foundation for sustainable vegetation recovery. Previous studies have shown that organic amendments, such as sheep manure, can improve soil microbial diversity and activity, which are critical for underground biomass development and overall soil health in high-altitude mining areas (Soria et al., 2021; Zhong et al., 2021).
The diversity indices, such as Shannon diversity and Margalef richness, were lower in the restored platform areas compared to the background, reflecting the challenges of restoring full biodiversity within a short period (Cardenas-Aguiar et al., 2022; He et al., 2024). The dominance of Poaceae species in these areas has been found to suppress the establishment of other species, as observed in previous studies of restored mining sites in Australia and South Africa (Cardenas-Aguiar et al., 2022). Conversely, the sunny slope areas showed better diversity due to reduced dominance, which allowed a wider range of species to establish, supporting findings from similar studies in North America (Courtney et al., 2011; Worlanyo and Jiangfeng, 2021).
Belowground biomass in the restored areas remained significantly lower than in the natural background, suggesting that root recovery takes longer and requires a sustained accumulation of organic matter (Feng et al., 2023; Williams and Thomas, 2023). This trend has also been observed in long-term studies of alpine restoration in Europe and North America, where belowground recovery often lags behind aboveground regeneration (Bardgett et al., 2005; Kim et al., 2020). The importance of belowground processes for the long-term success of ecosystem restoration cannot be overstated, as they are crucial for nutrient cycling and soil stabilization (Pardo et al., 2014).
The similarity between the restored sunny slope areas and the natural alpine meadow suggests that these areas are more conducive to returning to a natural community structure (Reid and Naeth, 2005b; Wong, 2003). Similar outcomes have been reported in high-altitude restoration in the Rocky Mountains, where restored communities on slopes showed greater similarity to natural vegetation compared to flat, disturbed areas (Zheng et al., 2023). This indicates that the microenvironmental conditions of slopes, along with less intense competition from dominant species, can facilitate the natural re-establishment of diverse plant communities (Petrova et al., 2022; Zhang et al., 2023).
The lower similarity between the platform areas and natural grassland highlights the challenge of restoring these areas, particularly due to the dominance of pioneer species such as grasses (Pardo et al., 2014; Zhou et al., 2015). Similar results have been found in mining restoration projects in Chile, where dominant grasses inhibited the establishment of other species, reducing overall community similarity to natural areas (Aronson et al., 1993; Carabassa et al., 2020). Therefore, efforts should focus on reducing dominance through mixed seeding strategies and diversified organic amendments, as supported by research from the Mediterranean region (Cardenas-Aguiar et al., 2022). The effectiveness of restoration measures in high-altitude mining areas, such as those on the Qinghai-Tibet Plateau, aligns with findings from similar environments worldwide. For instance, studies in the Andes and the Rocky Mountains highlight that organic soil amendments, including livestock manure and biochar, significantly enhance soil fertility and facilitate vegetation recovery under harsh alpine climates (Scotton, 2021; Zhang et al., 2023). In such regions, the short growing season and limited soil nutrient availability are critical constraints for restoration success. Compared to the humid, temperate climates where restoration can rely on natural seed banks and rapid organic matter accumulation (Jones et al., 2018), high-altitude regions often require tailored interventions like soil reconstruction, organic amendments, and native species seeding to achieve long-term recovery (Zhong et al., 2021). Furthermore, restoration strategies must account for varying precipitation patterns, as areas with arid conditions may demand additional irrigation measures to support seed germination and root establishment (Zhang et al., 2023). This comparison highlights the importance of integrating climatic conditions into the design of restoration measures to optimize their effectiveness across diverse high-altitude ecosystems.
Overall, the vegetation coverage and community characteristics in the Jiangcang mining area indicate substantial recovery potential using soil reconstruction and ecological restoration. However, gaps in belowground biomass and lower community similarity in some restored areas suggest a need for adaptive management. This includes monitoring species composition, adjusting soil amendment practices, and supplementary seeding with a focus on underrepresented species, aligning with global best practices for restoring resilience and stability in degraded ecosystems (Chazdon, 2008; Feng et al., 2023; Lu et al., 2024).
The restoration efforts in the Jiangcang mining area on the Qinghai-Tibet Plateau have demonstrated important lessons and outcomes for ecological restoration in high-altitude and fragile ecosystems. The use of sheep manure as an organic amendment not only enhanced soil fertility but also contributed to increased biodiversity by introducing wild grass seeds, thereby addressing the shortage of suitable native grass species in alpine regions. This approach was crucial in improving species diversity and promoting natural regeneration, which are often challenging in such harsh environments.
A comparative analysis of the two phases of mining area restoration revealed that traditional revegetation techniques without soil reconstruction yielded limited success, whereas the second phase, which included soil reconstruction and amendments, significantly improved the stability and fertility of the substrate. This resulted in higher vegetation coverage and increased ecosystem stability, indicating the effectiveness of enhanced restoration measures. The observed improvements in vegetation coverage and ecological conditions, without signs of degradation, further suggest that the restored mining area is on a favorable trajectory towards ecological recovery.
Although aboveground biomass showed notable recovery, approaching the levels observed in natural background areas, belowground biomass still lagged significantly behind. This indicates that while aboveground recovery can be relatively rapid, belowground biomass, which plays a crucial role in nutrient cycling and long-term ecosystem stability, requires a longer time to accumulate. The dominance of pioneer species in the restored platform areas, particularly Poaceae, was found to inhibit the development of a diverse plant community. In contrast, the slopes of the waste rock dump showed higher community similarity to natural alpine meadows, suggesting that reduced dominance of pioneer species can create opportunities for more diverse species to establish, leading to a more stable and natural community structure over time.
In summary, this study demonstrates that adaptive restoration techniques, particularly soil reconstruction and organic amendments, are essential for promoting both aboveground and belowground ecosystem recovery in high-altitude mining areas. The results underscore the need for restoration strategies that enhance soil health, manage pioneer species dominance, and support biodiversity. Moving forward, continuous long-term monitoring and adaptive management are critical to sustaining the observed ecological improvements and guiding future restoration efforts in similarly fragile ecosystems worldwide.
The original contributions presented in the study are included in the article/Supplementary Material, further inquiries can be directed to the corresponding author.
SF: Formal Analysis, Methodology, Visualization, Writing–original draft, Writing–review and editing. ZL: Funding acquisition, Investigation, Writing–original draft, Writing–review and editing. CZ: Software, Writing–original draft, Writing–review and editing. RQ: Conceptualization, Writing–original draft, Writing–review and editing. LY: Data curation, Resources, Writing–original draft, Writing–review and editing.
The author(s) declare that no financial support was received for the research, authorship, and/or publication of this article.
Authors SF, ZL, CZ, and RQ were employed by China Geo-Engineering Corporation.
The remaining author declares that the research was conducted in the absence of any commercial or financial relationships that could be construed as a potential conflict of interest.
The author(s) declare that no Generative AI was used in the creation of this manuscript.
All claims expressed in this article are solely those of the authors and do not necessarily represent those of their affiliated organizations, or those of the publisher, the editors and the reviewers. Any product that may be evaluated in this article, or claim that may be made by its manufacturer, is not guaranteed or endorsed by the publisher.
The Supplementary Material for this article can be found online at: https://www.frontiersin.org/articles/10.3389/fenvs.2024.1538243/full#supplementary-material
Aronson, J., Floret, C., Le Floc’h, E., Ovalle, C., and Pontanier, R. (1993). Restoration and rehabilitation of degraded ecosystems in arid and semi-arid regions: a view from the South. Restor. Ecol. 1 (1), 8–17. doi:10.1111/j.1526-100X.1993.tb00004.x
Asensio, V., Vega, F. A., Andrade, M. L., and Covelo, E. F. (2013). Tree vegetation and waste amendments to improve the physical condition of copper mine soils. CHEMOSPHERE 90, 603–610. doi:10.1016/j.chemosphere.2012.08.050
Asensio, V., Vega, F. A., and Covelo, E. F. (2014). Effect of soil reclamation process on soil C fractions. CHEMOSPHERE 95, 511–518. doi:10.1016/j.chemosphere.2013.09.108
Barberis, D., Lombardi, G., Enri, S. R., Pittarello, M., Viglietti, D., Freppaz, M., et al. (2023). Nitrogen fertilizer enhances vegetation establishment of a high-altitude machine-graded ski slope. Restor. Ecol. 31. doi:10.1111/rec.13777
Bardgett, R. D., Bowman, W. D., Kaufmann, R., and Schmidt, S. K. (2005). A temporal approach to linking aboveground and belowground ecology. TRENDS Ecol. and Evol. 20 (11), 634–641. doi:10.1016/j.tree.2005.08.005
Bullock, J. M., Aronson, J., Newton, A. C., Pywell, R. F., and Rey-Benayas, J. M. (2011). Restoration of ecosystem services and biodiversity: conflicts and opportunities. TRENDS Ecol. and Evol. 26 (10), 541–549. doi:10.1016/j.tree.2011.06.011
Carabassa, V., Domene, X., Diaz, E., and Alcaniz, J. M. (2020). Mid-term effects on ecosystem services of quarry restoration with Technosols under Mediterranean conditions: 10-year impacts on soil organic carbon and vegetation development. Restor. Ecol. 28, 960–970. doi:10.1111/rec.13072
Cardenas-Aguiar, E., Mendez, A., Paz-Ferreiro, J., and Gasco, G. (2022). The effects of rabbit manure-derived biochar on soil health and quality attributes of two mine tailings. SUSTAINABILITY 14, 1866. doi:10.3390/su14031866
Chaturvedi, R. K., and Singh, J. S. (2017). Diversity of ecosystem types in India: a review. Proc. Indian Natl. Sci. Acad. 83, 789–844. doi:10.16943/ptinsa/2017/41287
Chazdon, R. L. (2008). Beyond deforestation: restoring forests and ecosystem services on degraded lands. SCIENCE 320, 1458–1460. doi:10.1126/science.1155365
Cheng, H., Zhou, X., Dong, R., Wang, X., Liu, G., and Li, Q. (2022). Priming of soil organic carbon mineralization and its temperature sensitivity in response to vegetation restoration in a karst area of Southwest China. Sci. TOTAL Environ. 851, 158400. doi:10.1016/j.scitotenv.2022.158400
Courtney, R., Keith, A. M., and Harrington, T. (2011). Nematode assemblages in bauxite residue with different restoration histories. Restor. Ecol. 19, 758–764. doi:10.1111/j.1526-100X.2010.00734.x
Cross, A. T., and Lambers, H. (2017). Young calcareous soil chronosequences as a model for ecological restoration on alkaline mine tailings. Sci. TOTAL Environ. 607, 168–175. doi:10.1016/j.scitotenv.2017.07.005
Feng, T., Qi, Y., Zhang, Y., Fan, D., Wei, T., Wang, P., et al. (2024). Long-term effects of vegetation restoration and forest management on carbon pools and nutrient storages in northeastern Loess Plateau, China. J. Environ. Manag. 354, 120296. doi:10.1016/j.jenvman.2024.120296
Feng, T., Wei, T., Keesstra, S. D., Zhang, J., Bi, H., Wang, R., et al. (2023). Long-term effects of vegetation restoration on hydrological regulation functions and the implications to afforestation on the Loess Plateau. Agric. For. METEOROLOGY 330, 109313. doi:10.1016/j.agrformet.2023.109313
Figueiredo, M. A., Messias, M. C. T. B., Leite, M. G. P., and Kozovits, A. R. (2024). Topsoil volume optimization in the restoration of post-mined areas. Restor. Ecol. 32. doi:10.1111/rec.14222
He, Y., Tian, Z., Gu, Z., Wu, B., and Liang, Y. (2024). Controlling soil erosion of tailings from rare earth mines with paspalum wettsteinii and soil amendments. LAND Degrad. and Dev. doi:10.1002/ldr.5313
Heiskanen, J., Ruhanen, H., and Hagner, M. (2022). Effects of compost, biochar and ash mixed in till soil cover of mine tailings on plant growth and bioaccumulation of elements: a growing test in a greenhouse. HELIYON 8, e08838. doi:10.1016/j.heliyon.2022.e08838
Hu, Y.-L., Mgelwa, A. S., Singh, A. N., and Zeng, D.-H. (2018). Differential responses of the soil nutrient status, biomass production, and nutrient uptake for three plant species to organic amendments of placer gold mine-tailing soils. LAND Degrad. and Dev. 29, 2836–2845. doi:10.1002/ldr.3002
Jambhulkar, H. P., and Kumar, M. S. (2019). Eco-restoration approach for mine spoil overburden dump through biotechnological route. Environ. Monit. Assess. 191, 772. doi:10.1007/s10661-019-7873-6
Kim, M.-S., Min, H.-G., and Kim, J.-G. (2020). Integrating amendment and liquid fertilizer for aided-phytostabilization and its impacts on soil microbiological properties in arsenic-contaminated soil. Appl. SCIENCES-BASEL 10, 3985. doi:10.3390/app10113985
Li, S., Di, X., Wu, D., and Zhang, J. (2013). Effects of sewage sludge and nitrogen fertilizer on herbage growth and soil fertility improvement in restoration of the abandoned opencast mining areas in Shanxi, China. Environ. EARTH Sci. 70, 3323–3333. doi:10.1007/s12665-013-2397-9
Lim, B.-S., Kim, A.-R., Seol, J., Oh, W.-S., An, J.-H., Lim, C.-H., et al. (2022). Effects of soil amelioration and vegetation introduction on the restoration of abandoned coal mine spoils in South Korea. FORESTS 13, 483. doi:10.3390/f13030483
Liu, J., Zhang, S., Li, E., Zhu, Y., Cai, H., Xia, S., et al. (2022). Effects of cubic ecological restoration of mining wasteland and the preferred restoration scheme. Sci. TOTAL Environ. 851, 158155. doi:10.1016/j.scitotenv.2022.158155
Lu, Z., Yu, C., Liu, H., Zhang, J., Zhang, Y., Wang, J., et al. (2024). Application of new polymer soil amendment in ecological restoration of high-steep Rocky slope in seasonally frozen soil areas. POLYMERS 16, 1821. doi:10.3390/polym16131821
Luna, L., Vignozzi, N., Miralles, I., and Sole-Benet, A. (2018). Organic amendments and mulches modify soil porosity and infiltration in semiarid mine soils. LAND Degrad. and Dev. 29, 1019–1030. doi:10.1002/ldr.2830
Lupton, M. K., Rojas, C., Drohan, P., and Bruns, M. A. (2013). Vegetation and soil development in compost-amended iron oxide precipitates at a 50-year-old acid mine drainage barrens. Restor. Ecol. 21, 320–328. doi:10.1111/j.1526-100X.2012.00902.x
Macdonald, S. E., Landhaeusser, S. M., Skousen, J., Franklin, J., Frouz, J., Hall, S., et al. (2015). Forest restoration following surface mining disturbance: challenges and solutions. NEW For. 46, 703–732. doi:10.1007/s11056-015-9506-4
Madejon, P., Caro-Moreno, D., Navarro-Fernandez, C. M., Rossini-Oliva, S., and Maranon, T. (2021). Rehabilitation of waste rock piles: impact of acid drainage on potential toxicity by trace elements in plants and soil. J. Environ. Manag. 280, 111848. doi:10.1016/j.jenvman.2020.111848
Misebo, A. M., Pietrzykowski, M., and Wos, B. (2022). Soil carbon sequestration in novel ecosystems at post-mine sites-A new insight into the determination of key factors in the restoration of terrestrial ecosystems. FORESTS 13, 63. doi:10.3390/f13010063
Moreno-de las Heras, M. (2009). Development of soil physical structure and biological functionality in mining spoils affected by soil erosion in a Mediterranean-Continental environment. GEODERMA 149, 249–256. doi:10.1016/j.geoderma.2008.12.003
Naeth, M. A., and Wilkinson, S. R. (2014). Establishment of restoration trajectories for upland tundra communities on diamond mine wastes in the Canadian arctic. Restor. Ecol. 22, 534–543. doi:10.1111/rec.12106
Pardo, T., Martinez-Fernandez, D., Clemente, R., Walker, D. J., and Bernal, M. P. (2014). The use of olive-mill waste compost to promote the plant vegetation cover in a trace-element-contaminated soil. Environ. Sci. Pollut. Res. 21, 1029–1038. doi:10.1007/s11356-013-1988-z
Pedrol, N., Souza-Alonso, P., Puig, C. G., Gonzalez, L., Covelo, E. F., Asensio, V., et al. (2014). Improving soil fertility to support grass-legume revegetation on lignite mine spoils. Commun. SOIL Sci. PLANT ANALYSIS 45, 1565–1582. doi:10.1080/00103624.2013.875203
Penalver-Alcala, A., Alvarez-Rogel, J., Peixoto, S., Silva, I., Silva, A. R. R., and Gonzalez-Alcaraz, M. N. (2021). The relationships between functional and physicochemical soil parameters in metal(loid) mine tailings from Mediterranean semiarid areas support the value of spontaneous vegetation colonization for phytomanagement. Ecol. Eng. 168, 106293. doi:10.1016/j.ecoleng.2021.106293
Petrova, T. A., Rudzisha, E., Alekseenko, A. V., Bech, J., and Pashkevich, M. A. (2022). Rehabilitation of disturbed lands with industrial wastewater sludge. MINERALS 12, 376. doi:10.3390/min12030376
Reid, N., and Naeth, M. (2005a). Establishment of a vegetation cover on tundra kimberlite mine tailings: 1. A greenhouse study. Restor. Ecol. 13, 594–601. doi:10.1111/j.1526-100X.2005.00076.x
Reid, N., and Naeth, M. (2005b). Establishment of a vegetation cover on tundra kimberlite mine tailings: 2. A field study. Restor. Ecol. 13, 602–608. doi:10.1111/j.1526-100X.2005.00077.x
Rocha Martins, W. B., Schwartz, G., Ribeiro, S. S., Ferreira, G. C., Barbosa, R. de S., de Paula, M. T., et al. (2021). Ecosystem restoration after bauxite mining: favorable indicators for Technosols construction and soil management using liming and subsoiling. NEW For. 52, 971–994. doi:10.1007/s11056-021-09834-5
Rothman, S. E., Cole, C. A., Bruns, M. A., and Hall, M. (2021). The influence of soil amendments on a native wildflower seed mix in surface mine restoration. Restor. Ecol. 29. doi:10.1111/rec.13440
Scotton, M. (2021). Grassland restoration at a graded ski slope: effects of propagation material and fertilisation on plant cover and vegetation. AGRICULTURE-BASEL 11, 381. doi:10.3390/agriculture11050381
Sole, P., Ferrer, D., Raya, I., Pous, M., Gonzalez, R., Maranon-Jimenez, S., et al. (2023). Physical and chemical properties of limestone quarry technosols used in the restoration of mediterranean habitats. LAND 12, 1730. doi:10.3390/land12091730
Soria, R., Rodriguez-Berbel, N., Ortega, R., Lucas-Borja, M. E., and Miralles, I. (2021). Soil amendments from recycled waste differently affect CO₂ soil emissions in restored mining soils under semiarid conditions. J. Environ. Manag. 294, 112894. doi:10.1016/j.jenvman.2021.112894
van der Bauwhede, R., Troonbeeckx, J., Serbest, I., Moens, C., Desie, E., Katzensteiner, K., et al. (2024). Restoration rocks: the long-term impact of rock dust application on soil, tree foliar nutrition, tree radial growth, and understory biodiversity in Norway spruce forest stands. For. Ecol. Manag. 568, 122109. doi:10.1016/j.foreco.2024.122109
Wang, J., Luo, X., Zhang, Y., Huang, Y., Rajendran, M., and Xue, S. (2018). Plant species diversity for vegetation restoration in manganese tailing wasteland. Environ. Sci. Pollut. Res. 25, 24101–24110. doi:10.1007/s11356-018-2275-9
Williams, J. M., and Thomas, S. C. (2023). Effects of high-carbon wood ash biochar on volunteer vegetation establishment and community composition on metal mine tailings. Restor. Ecol. 31. doi:10.1111/rec.13861
Wong, M. (2003). Ecological restoration of mine degraded soils, with emphasis on metal contaminated soils. CHEMOSPHERE 50, 775–780. doi:10.1016/S0045-6535(02)00232-1
Worlanyo, A. S., and Jiangfeng, L. (2021). Evaluating the environmental and economic impact of mining for post-mined land restoration and land-use: a review. J. Environ. Manag. 279, 111623. doi:10.1016/j.jenvman.2020.111623
Yang, S., Liao, B., Xiao, R., and Li, J. (2019). Effects of amendments on soil microbial diversity, enzyme activity and nutrient accumulation after assisted phytostabilization of an extremely acidic metalliferous mine soil. Appl. SCIENCES-BASEL 9, 1552. doi:10.3390/app9081552
Yang, S., Liao, B., Yang, Z., Chai, L., and Li, J. (2016). Revegetation of extremely acid mine soils based on aided phytostabilization: a case study from southern China. Sci. TOTAL Environ. 562, 427–434. doi:10.1016/j.scitotenv.2016.03.208
Zhang, Y., Feng, T., Wang, L., Wang, X., Wei, T., and Wang, P. (2023). Effects of long-term vegetation restoration on soil physicochemical properties mainly achieved by the coupling contributions of biological synusiae to the Loess Plateau. Ecol. Indic. 152, 110353. doi:10.1016/j.ecolind.2023.110353
Zheng, T., Zeng, H., Zhang, X., Luo, J., Chen, X., Zhao, X., et al. (2023). Differential responses of soil community to reclamation with legumes versus grasses after an application of blended amendments in mining-disturbed soils. J. Clean. Prod. 418, 138113. doi:10.1016/j.jclepro.2023.138113
Zhong, H., Lambers, H., Wong, W. S., Dixon, K. W., Stevens, J. C., and Cross, A. T. (2021). Initiating pedogenesis of magnetite tailings using Lupinus angustifolius (narrow-leaf lupin) as an ecological engineer to promote native plant establishment. Sci. TOTAL Environ. 788, 147622. doi:10.1016/j.scitotenv.2021.147622
Keywords: Qinghai-Tibet plateau, soil reconstruction, ecological restoration, open-pit coal mining area, high-altitude vegetation recovery
Citation: Feng S, Li Z, Zhang C, Qi R and Yang L (2025) Ecological restoration in high-altitude mining areas: evaluation soil reconstruction and vegetation recovery in the Jiangcang coal mining area on the Qinghai-Tibet Plateau. Front. Environ. Sci. 12:1538243. doi: 10.3389/fenvs.2024.1538243
Received: 02 December 2024; Accepted: 20 December 2024;
Published: 10 January 2025.
Edited by:
Tianjiao Feng, Beijing Forestry University, ChinaReviewed by:
Chenxing Wang, Chinese Academy of Sciences (CAS), ChinaCopyright © 2025 Feng, Li, Zhang, Qi and Yang. This is an open-access article distributed under the terms of the Creative Commons Attribution License (CC BY). The use, distribution or reproduction in other forums is permitted, provided the original author(s) and the copyright owner(s) are credited and that the original publication in this journal is cited, in accordance with accepted academic practice. No use, distribution or reproduction is permitted which does not comply with these terms.
*Correspondence: Liya Yang, eWFuZ2xpeWFAbWFpbC5jZ3MuZ292LmNu
Disclaimer: All claims expressed in this article are solely those of the authors and do not necessarily represent those of their affiliated organizations, or those of the publisher, the editors and the reviewers. Any product that may be evaluated in this article or claim that may be made by its manufacturer is not guaranteed or endorsed by the publisher.
Research integrity at Frontiers
Learn more about the work of our research integrity team to safeguard the quality of each article we publish.