- 1School of Tourism and Culture, Guangdong Eco-engineering Polytechnic, Guangzhou, China
- 2School of Resource and Environmental Sciences, Wuhan University, Wuhan, China
- 3Collaborative Innovation Center for Emissions Trading System Co-constructed by the Province and Ministry, Wuhan, China
- 4Guangdong Provincial Academy of Environmental Science, Guangzhou, China
- 5Guangzhou Hongjing Ecological Technology Co., Ltd., Guangzhou, China
The Pearl River Estuary, a vital ecological and economic zone in Southern China, has been heavily impacted by industrial discharges, leading to significant heavy metal contamination. To address the ecological implications of different chemical forms of heavy metals, this study systematically evaluated the total concentrations and chemical speciation of Cu, Zn, Cd, and Pb in surface sediments (0–2 cm) collected from 17 sites. Chemical speciation was determined using a modified BCR sequential extraction procedure, and pollution and ecological risks were assessed via the geo-accumulation index (
1 Introduction
The estuarine region is a critical transitional zone between terrestrial and marine ecosystems, where intricate interactions occur among physical, chemical, biological, and geological processes. Due to their inherent ecological sensitivity and fragility, estuarine environments are highly vulnerable to anthropogenic disturbances (Elliott and Quintino, 2007; Min et al., 2021). The Pearl River Estuary, one of China’s three largest estuaries, serves as a critical breeding and conservation ground for juvenile fish and shrimp and provides habitat for numerous rare aquatic species (Chan and Wang, 2019). Recent rapid industrial, agricultural, and marine fishery development along the estuary’s coastline has led to excessive pollutant discharges, resulting in severe environmental degradation-most notably, the pervasive contamination by heavy metals (Zhao et al., 2020; Niu et al., 2021a). Similar studies in other coastal and lagoon systems have shown comparable pollution patterns, highlighting the importance of understanding regional environmental conditions and pollution trends (Ustaoglu et al., 2024).
Heavy metals are characterized by their intrinsic biological toxicity, environmental persistence, and bioaccumulation potential. Once introduced into aquatic systems, such as rivers and estuaries, these metals accumulate in sediments, posing severe threats to benthic organisms and aquatic life, and may ultimately impact human health through biomagnification along the food chain. Consequently, heavy metals are considered a priority pollutant in aquatic ecosystems (Ndimele, 2012; Fu et al., 2014; Li et al., 2014). Research on the bioaccumulation of metals across different species offers further insight into the potential health risks and ecological implications (Yuksel et al., 2024). In response to these environmental challenges, the Guangdong Province Heavy Metal Pollution Prevention Plan and several ecological restoration projects have been implemented to reduce heavy metal emissions and improve water quality in the Pearl River Estuary (Zhen et al., 2016; Zhao et al., 2020). The Pearl River Estuary, acting as a “source-sink” transition zone, is heavily influenced by hydrodynamic and tidal forces, which intensify the dispersal and accumulation of heavy metals, making it a significant pollution hotspot for surrounding cities such as Guangzhou, Dongguan, and Shenzhen in the Greater Bay Area (Niu et al., 2021a). Therefore, a systematic evaluation of heavy metal contamination in the estuarine sediments is urgently needed (Wang et al., 2011; Huang et al., 2018; Wang et al., 2023).
The bioavailability and mobility of heavy metals in sediments are influenced not only by their total concentrations but also by their chemical forms and binding states. Sequential chemical extraction methods have been widely adopted to investigate these chemical forms in solid media such as sediments and soils (Choleva et al., 2020). Tessier first introduced the sequential extraction approach in 1979, and the European Community Bureau of Reference (BCR) later developed a widely used three-step sequential extraction protocol in 1987. The BCR protocol has since undergone numerous modifications to enhance its precision and applicability (Usero et al., 1998; Zemberyová et al., 2006). The fundamental principle of these multi-step extraction methods is to simulate varying environmental conditions using chemical reagents of increasing strength, gradually isolating different chemical forms of heavy metals. Tessier’s method categorizes heavy metals into five chemical forms: exchangeable, carbonate-bound, iron-manganese oxide-bound, organic matter-bound, and residual. Subsequently, Rauret improved the BCR method in 1999 by grouping metals into four forms: acid-extractable, reducible, oxidizable, and residual. The acid-extractable form encompasses the exchangeable and carbonate-bound metals from Tessier’s classification (Rauret et al., 1999; Anju and Banerjee, 2010). The exchangeable form is primarily adsorbed onto clay or humic substances. It is the most susceptible to release and migration, while the carbonate-bound form is quickly released under acidic conditions (Morera et al., 2001). The acid-extractable form is generally the most responsive to environmental changes, presenting high ecological risk and toxicity. Conversely, the residual form is bound within mineral and silicate lattices, rendering it relatively stable and posing minimal ecological risk (Sundaray et al., 2011). The distribution of heavy metals in various chemical forms indicates their mobility and transformation potential in sediments and soils, reflecting their bioavailability and ecological risks (Kim et al., 2015).
Numerous studies have assessed the ecological risks of heavy metals in surface sediments by analyzing their total concentrations, chemical forms, and distribution patterns using various technical methods (Yu et al., 2011). Among these, the Index of Geo-Accumulation (
Although extensive research has been conducted on heavy metal accumulation in the Pearl River Estuary, most studies have focused on total concentrations and pollution risks (Ip et al., 2004; Yang et al., 2012; Zhao et al., 2020), with limited attention paid to the chemical forms and their associated ecological risks. Comparative studies across various regions can provide a broader context, highlight unique pollution characteristics, or align them with global patterns (Yuksel et al., 2021; Topaldemir et al., 2023). Therefore, this study aims to bridge this gap by employing the
2 Materials and methods
2.1 Study area overview
The Pearl River, formed by the convergence of the Xi River, Bei River, Dong River, and numerous tributaries within the Pearl River Delta, is the most extensive river system in southern China. The third longest in the country, spanning 2,320 km with a drainage area of 450,000 square kilometers, of which 440,000 square kilometers are within Chinese territory (Chen et al., 2008). The Pearl River’s annual runoff exceeds 330 billion cubic meters, second only to the Yangtze River, and it produces seven times the annual runoff of the Yellow River (Xu et al., 2010). This study focuses on the surface sediments of the Pearl River Estuary, extending from Duntouji in Guangzhou (113.51°E, 23.05°N) in the north to near the Jitimen Bridge in Zhuhai (113.26°E, 22.09°N), including the Lingding Yang region, which is characterized as a tide-dominated estuarine bay. Sampling was conducted in January 2022, with 17 sampling sites established across the study area, as depicted in Figure 1.
2.2 Sample collection and processing
Due to the mild and consistent climate conditions in the Pearl River Estuary region throughout the year, coupled with the continuous and stable industrial activities along its banks, the levels of heavy metal pollution in this area are generally consistent year-round (Ye et al., 2020). Sediment samples were collected using a grab sampler to obtain undisturbed surface sediments (0–2 cm) and stored in polyethylene bags at 0°C–4°C. The samples were air-dried, and visible gravel, plants, and animal residues were removed. Subsequently, the sediments were oven-dried at 105°C to a constant weight, ground using an agate mortar, and sieved through a 200-mesh screen. The processed samples were stored in a desiccator for further analysis. According to the GB 15618–2008 Environmental Quality Standards for Soils, Cu, Zn, Cd, and Pb were selected as target heavy metals of concern (Ministry of Environmental Protection of China, 2008). The total concentrations of these metals in the sediment samples were determined after digestion using a mixture of HCl, HNO₃, HF, and HClO₄, following the four-acid digestion method (GB/T17140) (Standardization Administration of China, 1997). Cd and Pb concentrations were measured using a graphite furnace atomic absorption spectrophotometer, while Cu and Zn were quantified using a flame atomic absorption spectrophotometer. These different analytical techniques are chosen based on the concentration sensitivity and detection requirements specific to each metal. The graphite furnace atomic absorption spectrophotometer (GFAAS) offers a significantly higher sensitivity compared to FAAS, making it ideal for detecting trace levels of metals like Cd and Pb, which are often present in lower concentrations. In contrast, flame atomic absorption spectrophotometry (FAAS) is suitable for metals such as Cu and Zn, which are generally found in higher concentrations in environmental samples. FAAS is also efficient for rapid analysis when ultra-trace sensitivity is not required. Utilizing these methods in tandem ensures accurate measurement across different concentration ranges, enhancing the precision and reliability of the heavy metal quantification (Garnier et al., 2006; Medeiros et al., 2020).
The chemical forms of the heavy metals were extracted using the modified BCR sequential extraction procedure (Rauret et al., 1999; Sungur et al., 2014). The specific extraction protocol consisted of (1) Acid-extractable fraction (F1), including exchangeable and carbonate-bound metals, extracted using 0.11 mol·L⁻1 acetic acid; (2) Reducible fraction (F2), representing metals bound to iron-manganese oxides, extracted using 0.5 mol·L⁻1 hydroxylamine hydrochloride; (3) Oxidizable fraction (F3), representing metals bound to organic matter and sulfides, extracted by digesting the F2 residue with 30% (8.8 mol·L⁻1) hydrogen peroxide followed by 1 mol·L⁻1 ammonium acetate; and (4) Residual fraction (F4), representing metals bound to silicates or within the mineral lattice, extracted by digesting the F3 residue using a mixture of three acids.
Three parallel samples and an environmental standard reference material (ESS-4) were used for quality control to ensure analytical precision and accuracy. The relative error of heavy metal concentrations in parallel samples was maintained below 10%, and the recovery rates of standard reference materials ranged from 95% to 120%. The detection limits for Cu, Zn, Cd, and Pb were 0.5 mg·kg⁻1, 7.0 mg·kg⁻1, 0.07 mg·kg⁻1, and 2.0 mg·kg⁻1, respectively.
While the BCR sequential extraction procedure is widely used for assessing heavy metal speciation, potential limitations exist. Interferences from other sediment components, such as organic matter or carbonate content, may affect the extraction accuracy, particularly for acid-extractable and reducible fractions. Additionally, the four-acid digestion method used for total concentration measurement could lead to variations in recovery rates for certain metals due to complex matrix effects (Ryan et al., 2008).
2.3 Data analysis
2.3.1 Pollution characteristics and risk assessment based on total heavy metal concentrations
2.3.1.1 Index of geo-accumulation ( )
The Index of Geo-Accumulation (
2.3.1.2 Potential ecological risk assessment method (RI)
The potential ecological risk assessment method, proposed by Hakanson (1980), evaluates the ecological risks posed by heavy metals in sediments (Maanan et al., 2015; Zhang et al., 2016). The values are calculated using Equation 2 as follows:
where
The comprehensive ecological risk of multiple heavy metals is calculated using Equation 3 as follows:
RI represents the comprehensive potential ecological risk index for multiple heavy metals, and
2.3.2 Risk assessment based on different chemical forms of heavy metals
The Risk Assessment Code (RAC) method provides a deeper understanding of the relationship between bioavailability, mineral mobility, and the environmental risks of heavy metals (Yang et al., 2014). RAC is calculated as the proportion of the acid-extractable fraction relative to the total content of an element (Lv et al., 2013), as shown in Equation 4 below:
where
3 Results
3.1 Descriptive statistics of total Cu, Zn, Cd, and Pb in sediments
3.1.1 Concentrations of Cu, Zn, Cd, and Pb in surface sediments of the Pearl River estuary
The concentrations of Cu, Zn, Cd, and Pb in surface sediments of the Pearl River Estuary are illustrated in Figure 2. The measured concentrations varied as follows: Cu ranged from 30.14 to 150.74 mg·kg⁻1, Zn from 78.63 to 367.16 mg·kg⁻1, Cd from 0.18 to 2.63 mg·kg⁻1, and Pb from 40.57 to 115.11 mg·kg⁻1. All sampling sites exhibited heavy metal concentrations exceeding the background values for soils in Guangdong Province. Among these, Cd showed the most significant elevation, with a mean concentration of 1.05 mg·kg⁻1, surpassing the background value by 39 times. The mean concentrations of Cu (58.71 mg·kg⁻1), Zn (88.23 mg·kg⁻1), and Pb (67.17 mg·kg⁻1) exceeded the background values by 3.85, 2.54, and 1.10 times, respectively.
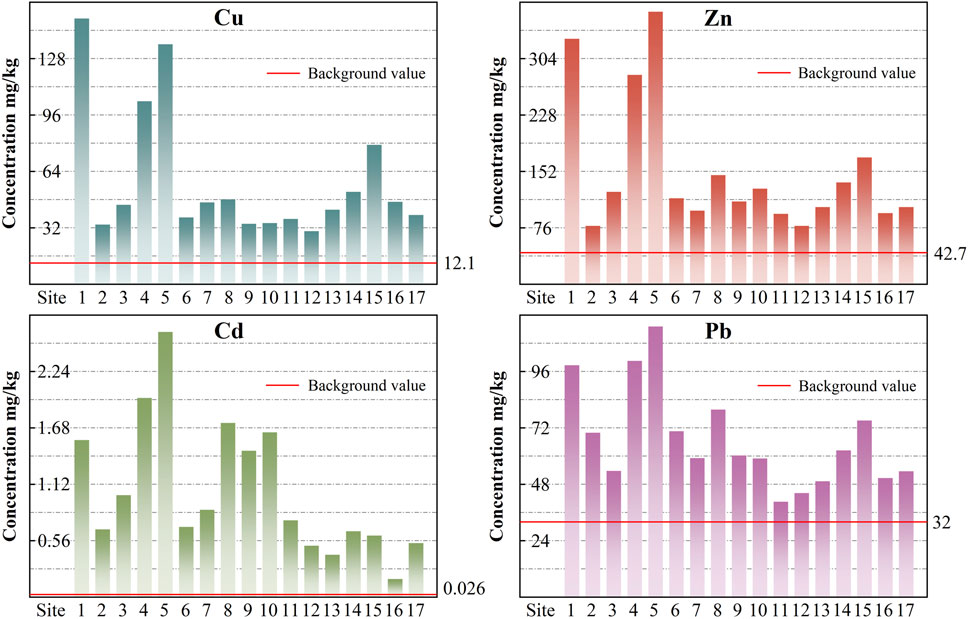
Figure 2. Descriptive statistics and comparative parameters of Cu, Zn, Cd, and Pb in surface sediments of the Pearl River Estuary (unit: mg·kg⁻1).
3.1.2 Geo-accumulation index ( ) and pollution degree assessment of heavy metals
The geo-accumulation index (
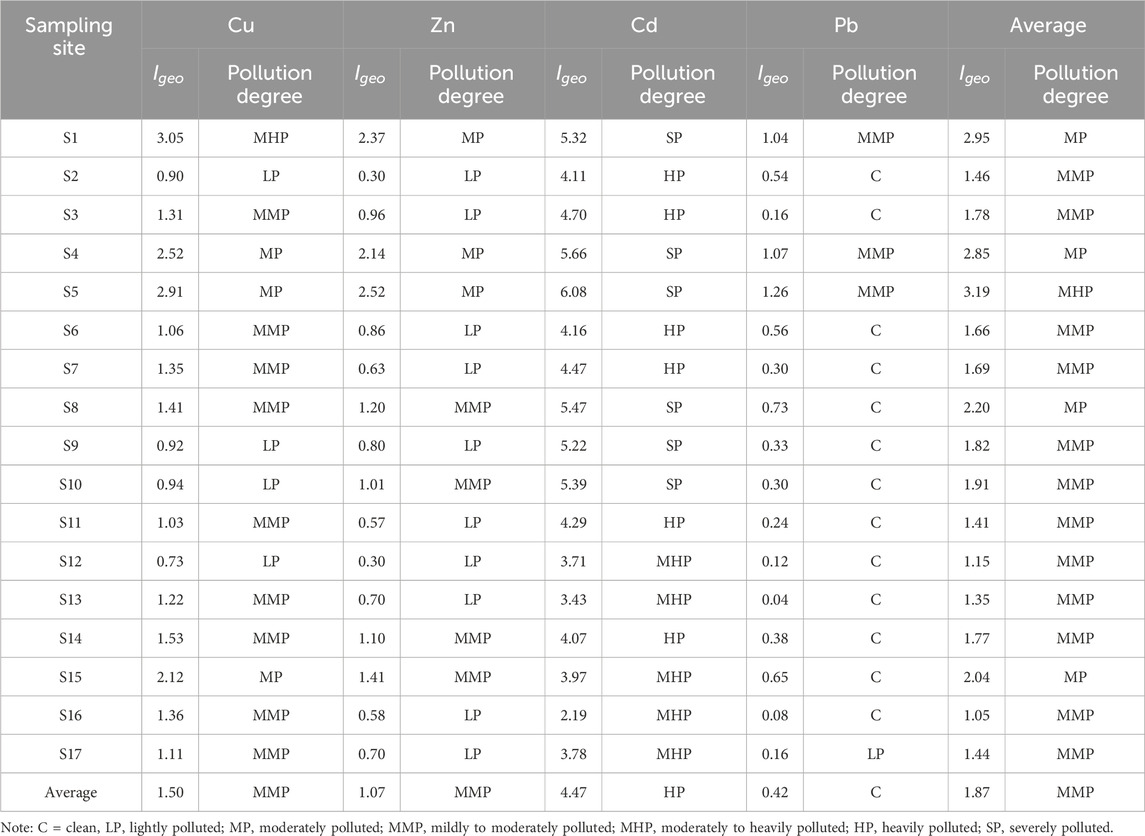
Table 4. Geo-accumulation index (
Overall, the pollution ranking for the four metals from highest to lowest is: Cd (Class 5) > Cu (Class 2) > Zn (Class 2) > Pb (Class 1). The average
3.1.3 Potential ecological risk assessment of heavy metals in sediments
The potential ecological risk indices (ERI) for Cu, Zn, Cd, and Pb in the surface sediments of the Pearl River Estuary are presented in Table 5. The ERI values for Cu ranged from 12.45 to 62.29, indicating low to moderate risk, whereas Zn and Pb showed low risk at all sampling points, with ERI values ranging from 1.84 to 8.60 and 6.34 to 17.99, respectively. In contrast, Cd exhibited extremely high ERI values, ranging from 205.93 to 3,036.77, suggesting severe ecological risk across all sites.
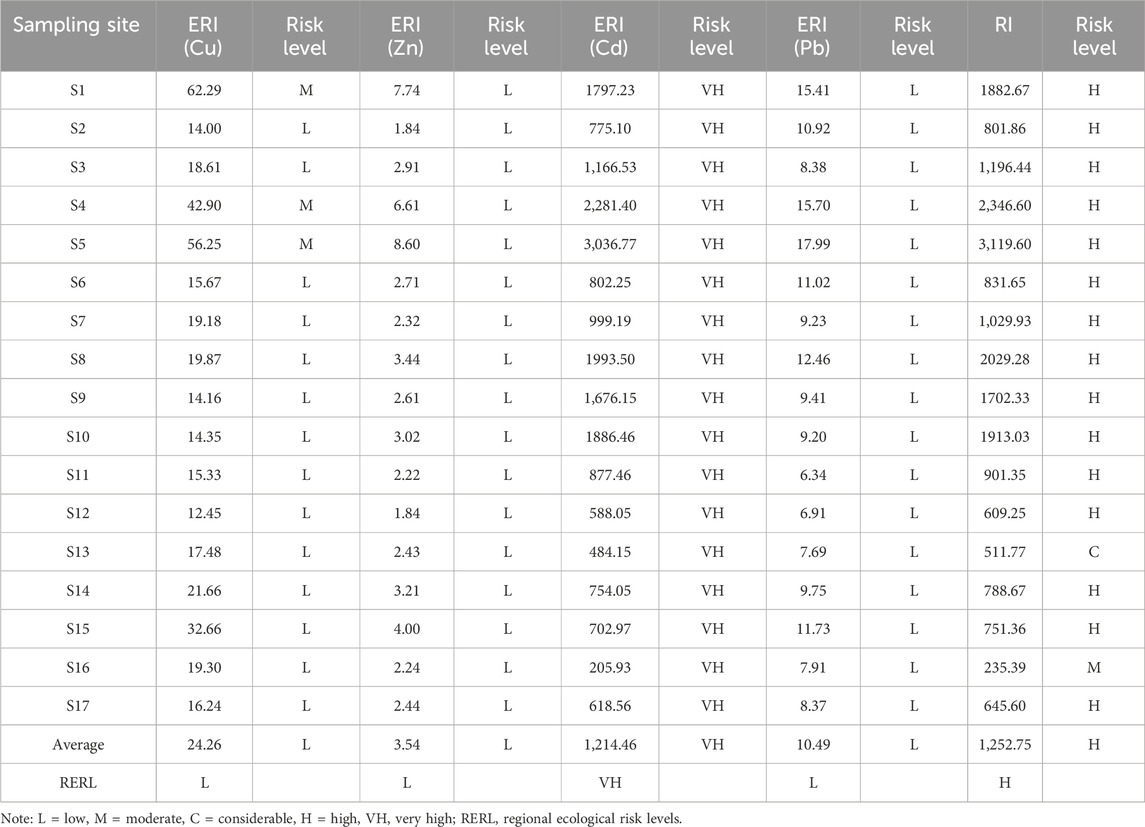
Table 5. Potential ecological risk assessment of Cu, Zn, Cd, and Pb in surface sediments of the Pearl River Estuary.
The average ERI values in descending order were as follows: Cd (1,214.46) > Cu (24.26) > Pb (10.49) > Zn (3.54). The comprehensive potential ecological risk index (RI) for the four heavy metals ranged from 235.39 at Site S16 to 3,119.60 at Site S5, with an average RI value of 1,252.75. Among the 17 sampling sites, Site S5 was categorized as a moderate ecological risk, Site S15 as a considerable ecological risk, and the remaining 15 as a high ecological risk.
3.2 Descriptive statistics of different chemical forms of Cu, Zn, Cd, and Pb in sediments
3.2.1 Percentage content of different chemical forms of Cu, Zn, Cd, and Pb in surface sediments
Figure 3 presents the percentage content of different Cu, Zn, Cd, and Pb chemical forms in the surface sediments. The distribution of chemical forms for each metal is as follows:
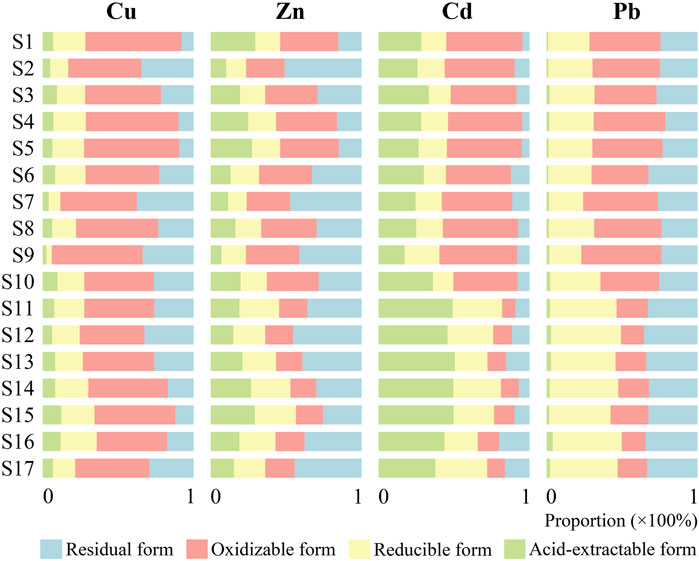
Figure 3. Percentage content of different chemical forms of Cu, Zn, Cd, and Pb in surface sediments.
Cu: The acid-extractable form accounted for 2.52%–12.38%, the reducible form 3.56%–23.98%, the oxidizable form 43.00%–63.66%, and the residual form 7.93%–37.50%. On average, the proportions of the chemical forms were ranked as follows: oxidizable (52.13%) > residual (22.75%) > reducible (17.57%) > acid-extractable (7.55%).
Zn: The acid-extractable form ranged from 7.21% to 29.73%, the reducible form from 12.15% to 27.22%, the oxidizable form from 17.13% to 40.48%, and the residual form from 14.89% to 50.93%. The average proportions were residual (33.07%) > oxidizable (28.08%) > reducible (19.58%) > acid-extractable (19.28%).
Cd: The acid-extractable form ranged from 17.37% to 50.63%, the reducible form from 13.63% to 34.33%, the oxidizable form from 8.87% to 51.49%, and the residual form from 4.52% to 19.91%. On average, the chemical form distribution was acid-extractable (35.45%) > reducible (32.82%) > oxidizable (21.86%) > residual (9.87%).
Pb: The acid-extractable form accounted for 1.07%–4.27%, the reducible form 21.23%–46.33%, the oxidizable form 15.38%–53.26%, and the residual form 21.04%–35.16%. The average distribution was: reducible (34.76%) > oxidizable (34.66%) > residual (28.45%) > acid-extractable (2.12%).
3.2.2 Risk assessment code (RAC) for different chemical forms of heavy metals
The Risk Assessment Code (RAC) values and corresponding risk levels for Cu, Zn, Cd, and Pb are shown in Figure 4. The results are summarized as follows:
Cu: RAC values ranged from 2.52% to 12.38%. Only two sampling sites (S15 and S16) were classified as medium risk (11.76%), while the remaining 15 sites showed low risk (88.24%).
Zn: RAC values ranged from 7.21% to 29.73%. Only Site S9 was classified as low risk, whereas the remaining 16 sampling sites were categorized as medium risk (94.12%).
Cd: RAC values ranged from 17.37% to 50.36%, indicating medium to very high risk. Seven sampling sites (S1, S4, S5, S2, S8, S7, and S9) were classified as medium risk (41.18%), nine sites (S15, S14, S11, S12, S16, S17, S10, S3, and S6) as high risk (52.94%), and Site S1 as very high risk.
Pb: RAC values ranged from 1.07% to 4.27%, indicating low risk across all sites.
The average RAC values across all sites rank as follows: Cd (35.45%) > Zn (19.28%) > Cu (7.55%) > Pb (2.12%). Based on these values, Cd is classified as high risk, Zn as medium risk, and Cu and Pb as low risk.
3.3 Comprehensive assessment of total and speciated heavy metal pollution risk
A comprehensive assessment of the total and speciated pollution risks for Cu, Zn, Cd, and Pb is presented in Table 6, using the
This assessment highlights that Cd poses the most significant risk across all indicators, necessitating prioritized mitigation efforts, whereas Cu and Pb present minimal risks. Despite Zn’s lower total concentration, its higher bioavailable fraction contributes to a medium risk, warranting attention to potential ecological impacts.
These ecological risks are closely linked to the chemical forms of the metals. For Cd, the dominance of its acid-extractable fraction indicates high bioavailability, which increases its mobility and potential for uptake by aquatic organisms, posing severe ecological threats. In contrast, Zn, although lower in total concentration, shows a substantial bioavailable fraction, highlighting its potential for mobility and ecological impact under variable environmental conditions. On the other hand, Cu and Pb have lower proportions of bioavailable forms and higher residual fractions, suggesting they are less mobile and present a reduced ecological risk (Xu et al., 2016; Liu et al., 2021).
4 Discussions
4.1 Total heavy metal pollution and ecological risk in the Pearl River Estuary
Cu, Zn, Cd, and Pb concentrations in the Pearl River Estuary are substantially higher than other major river systems in China, such as the Yangtze River and Bortala River (Xinjiang), as shown in Table 7. While the sediment Cd concentrations in the Yangtze and Liao Rivers slightly surpass those in the Pearl River Estuary, Cu, Zn, and Pb concentrations are significantly lower. This finding underscores that the Pearl River Estuary remains one of the most heavily contaminated estuaries in China concerning heavy metal pollution in sediments, likely due to the highly industrialized nature of the lower Pearl River region. Furthermore, all heavy metal concentrations in the sediments exceed the background values for Guangdong Province soils (Table 7), indicating substantial anthropogenic contributions to heavy metal contamination, particularly for Cd, which is elevated by 39 times compared to the background level. Numerous studies have consistently identified Cd as the most severe contaminant in this estuary over the years (Xie et al., 2012; Liu et al., 2017; Jia et al., 2021). Despite the implementation of stringent control measures in recent years, the persistence and accumulation of these metals imply that heavy metal pollution remains a critical environmental challenge in the region, especially for Cd, which necessitates sustained remediation efforts.
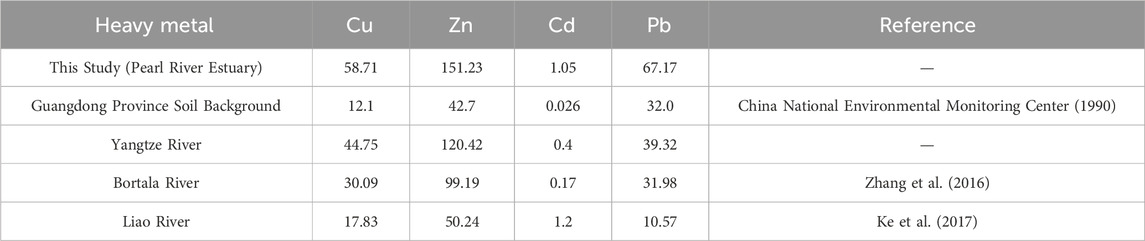
Table 7. Comparison of metal ion concentrations (mg·kg⁻1) in surface sediments of the Pearl River Estuary and other rivers in China.
Regarding potential ecological risk, the single-element ecological risk index (ERI) for Cu, Zn, and Pb at all sampling points indicates low risk (Table 5). In contrast, Cd poses a high ecological risk across the 17 sites. The contribution of each metal’s ERI to the comprehensive potential ecological risk index (RI) is ranked as follows: Cd (96.94%) > Cu (1.94%) > Pb (0.84%) > Zn (0.28%), clearly indicating Cd’s dominant role in the overall ecological risk. This result aligns with a study by Zhang et al. (2016), which assessed the potential ecological risk of heavy metals in the Bortala River. Their findings similarly showed that Cd accounted for 97% of the total ecological risk, followed by Pb, Cu, and Zn. The congruence between these studies further confirms that Cd exhibits the highest single-element ecological risk and makes the most significant contribution to the comprehensive ecological risk in the Pearl River Estuary.
4.2 Correlation analysis of heavy metals in sediments of the Pearl River estuary
Heavy metals in sediments often exhibit complex interrelationships, influenced by factors such as the original composition of heavy metals in the parent rock, soil formation processes, and anthropogenic activities. High and significant correlations between heavy metals can imply a common source of contamination (Zhang et al., 2016; Ke et al., 2017; Fu et al., 2022). This study conducted Pearson correlation analysis to examine the interrelationships between Cu, Zn, Cd, and Pb in the sediments from 17 sampling sites. As shown in Table 8, all four metals exhibited significant correlations (P < 0.01), suggesting that they share a common source, potentially linked to the intense industrial development in the lower reaches of the Pearl River. Many heavy metals are discharged into the river through industrial effluents, leading to severe sediment contamination in the estuary. These findings are consistent with research by Zhang et al. (2016), who studied the distribution of heavy metals in sediments from the Lingding Yang area of the Pearl River Estuary over the past century. Their results indicated that the strong correlations between heavy metals suggest a common source or that the environmental conditions and biogeochemical processes have remained highly consistent and stable. Other studies have attributed the primary sources of heavy metals in the Pearl River Estuary to industrial activities, shipping, and agricultural production, with Cd contamination particularly associated with the lead-zinc mining and smelting industries in the upper reaches of the Beijiang and Dongjiang Rivers in Guangdong Province (Gu et al., 2014; Du et al., 2019; Lu et al., 2020; Niu et al., 2021b). Pollutants from these industries are transported downstream, exacerbating heavy metal contamination in the estuarine sediments.
4.3 Chemical speciation and environmental risks of heavy metals
The RAC results indicate that Cd poses the highest pollution and ecological risk among the four metals. The acid-extractable fraction of Cd, representing its most bioavailable form, constitutes more than one-third of its total concentration, the highest proportion among the studied metals. Furthermore, the combined content of the acid-extractable, reducible, and oxidizable forms of Cd exceeds 90% of its total concentration, with the residual fraction contributing less than 10%. This distribution pattern suggests that anthropogenic activities heavily influence Cd in the surface sediments of the Pearl River Estuary, which are present predominantly in bioavailable forms. Consequently, Cd is highly susceptible to environmental changes, such as variations in water chemistry, and can be quickly released back into the water, where it may be assimilated by aquatic organisms, adversely impacting their growth and survival.
In contrast, Pb exhibits the lowest RAC value, with the acid-extractable fraction accounting for only 2.12% of its total concentration and a relatively high residual fraction (28.45%), indicating low ecological risk. Cu also has a low proportion of acid-extractable content but shows the highest percentage of oxidizable forms, exceeding 50% of its total concentration. This suggests Cu may migrate from sediments to the water column under oxidative conditions. On the other hand, Zn has a relatively high proportion of acid-extractable content and a substantial residual fraction (33.07%), the highest among the four metals. The other chemical forms of Zn (acid-extractable, reducible, and oxidizable) are present in relatively similar proportions, ranging from 19.28% to 28.08%, indicating that Zn can quickly shift between its bioavailable forms under both oxidative and reductive conditions.
Although research on heavy metals in river sediments across China began relatively late, most major river basins have been comprehensively studied. The distribution of heavy metal chemical forms shows significant variation among different river systems, likely due to the diverse anthropogenic activities and environmental conditions that influence heavy metal partitioning in sediments (Jiang et al., 2020; Deng et al., 2023).
The estuarine environmental conditions, such as pH, salinity, and organic matter content, are considered to interpret the observed speciation patterns further. For instance, the high bioavailability of cadmium (Cd) in the Pearl River Estuary may be attributed to the estuary’s slightly acidic conditions and moderate salinity levels, which enhance the solubility and mobility of Cd. Similarly, the presence of oxidizable forms of Cu is likely influenced by the organic-rich sediments found in this region, which provide binding sites for metal-organic complexes. These environmental factors contribute to the differential bioavailability and mobility observed for each metal.
5 Conclusion
The concentrations of Cu, Zn, Cd, and Pb in surface sediments across all sampling sites in the Pearl River Estuary significantly exceed the background values for soils in Guangdong Province, with Cd (1.05 mg·kg⁻1) showing the most pronounced contamination, surpassing the background value by a factor of 39. The geo-accumulation index (
This study reveals that Cd poses the highest ecological risk among the four metals, with its predominantly bioavailable forms making it highly susceptible to environmental changes and, thus, a significant threat to the estuarine ecosystem. These findings highlight the need for continuous, targeted management strategies to mitigate Cd contamination and reduce its ecological impact in the Pearl River Estuary. By focusing on heavy metal contamination and ecological risks within the estuary’s specific context, we used background values relevant to the region. While international standards, such as those from the European Union or EPA, provide useful comparative benchmarks, they may not fully capture the unique environmental and regulatory conditions of this estuarine ecosystem in southern China. Future research should incorporate these benchmarks, examine the long-term impacts of Cd bioavailability on aquatic health, and assess the efficacy of remediation techniques over time to support adaptive management in this unique ecological context.
Data availability statement
The original contributions presented in the study are included in the article/Supplementary Material, further inquiries can be directed to the corresponding authors.
Author contributions
ZhL: Conceptualization, Data curation, Methodology, Writing–original draft, Writing–review and editing. QW: Data curation, Investigation, Software, Visualization, Writing–review and editing. CH: Data curation, Investigation, Software, Visualization, Writing–review and editing. PZ: Conceptualization, Data curation, Methodology, Supervision, Writing–original draft, Writing–review and editing. XW: Data curation, Investigation, Software, Visualization, Writing–review and editing. ZiL: Investigation, Validation, Writing–review and editing. LY: Investigation, Validation, Writing–review and editing. CQH: Conceptualization, Data curation, Methodology, Supervision, Writing–original draft, Writing–review and editing.
Funding
The author(s) declare that financial support was received for the research, authorship, and/or publication of this article. This work was supported by the Key Research and Development Program Project in Guangdong Province (2020B1111350001), the Science and Technology Innovation Project in Guangdong Forestry (2024KJCX005), and the UNEP/GEF “Implementation of the Strategic Action Plan for the South China Sea and Gulf of Thailand” (Mangroves, seagrass beds, wetlands) (S1-32GFL-000631, SB-009056).
Conflict of interest
Author LY was employed by Guangzhou Hongjing Ecological Technology Co., Ltd.
The remaining authors declare that the research was conducted in the absence of any commercial or financial relationships that could be construed as a potential conflict of interest.
Generative AI statement
The author(s) declare that no Generative AI was used in the creation of this manuscript.
Publisher’s note
All claims expressed in this article are solely those of the authors and do not necessarily represent those of their affiliated organizations, or those of the publisher, the editors and the reviewers. Any product that may be evaluated in this article, or claim that may be made by its manufacturer, is not guaranteed or endorsed by the publisher.
Supplementary material
The Supplementary Material for this article can be found online at: https://www.frontiersin.org/articles/10.3389/fenvs.2024.1509277/full#supplementary-material
References
Anju, M., and Banerjee, D. K. (2010). Comparison of two sequential extraction procedures for heavy metal partitioning in mine tailings. Chemosphere 78 (11), 1393–1402. doi:10.1016/j.chemosphere.2009.12.064
Chan, C. Y., and Wang, W. X. (2019). Biomarker responses in oysters Crassostrea hongkongensis in relation to metal contamination patterns in the Pearl River Estuary, southern China. Environ. Pollut. 251, 264–276. doi:10.1016/j.envpol.2019.04.140
Chen, C. T. A., Wang, S. L., Lu, X. X., Zhang, S. R., Lui, H. K., Tseng, H. C., et al. (2008). Hydrogeochemistry and greenhouse gases of the Pearl River, its estuary and beyond. Quat. Int. 186, 79–90. doi:10.1016/j.quaint.2007.08.024
China National Environmental Monitoring Center (1990). Background values of soil elements in China. Beijing, China: China Environmental Science Press.
Choleva, T. G., Tsogas, G. Z., Vlessidis, A. G., and Giokas, D. L. (2020). Development of a sequential extraction and speciation procedure for assessing the mobility and fractionation of metal nanoparticles in soils. Environ. Pollut. 363, 114407. doi:10.1016/j.envpol.2020.114407
Cui, J., Zang, S. Y., Zhai, D. L., and Wu, B. (2014). Potential ecological risk of heavy metals and metalloid in the sediments of Wuyuer River basin, Heilongjiang Province, China. Ecotoxicology 23 (4), 589–600. doi:10.1007/s10646-014-1182-1
Deng, X. D., Zhang, C., Zhai, F. J., Li, S. M., and Shan, B. Q. (2023). Heavy metal speciation and ecological risk assessment of soil in potential new submerged areas of Baiyangdian Lake. Acta Sci. Circumstantiae 43 (5), 398–408. [In Chinese]. doi:10.13671/j.hjkxxb.2022.0435
Du, J., Wang, Y. H., Huang, Q. H., Dai, Q., and Yang, Y. D. (2019). Temporal and spatial variation characteristics and influencing factors of heavy metals in suspended sediments of the Pearl River Estuary. Environ. Sci. 40 (2), 625–632. doi:10.13227/j.hjkx.201807028
Elliott, M., and Quintino, V. (2007). The estuarine quality paradox, environmental homeostasis and the difficulty of detecting anthropogenic stress in naturally stressed areas. Mar. Pollut. Bull. 54 (6), 640–645. doi:10.1016/j.marpolbul.2007.02.003
Forstner, U., Ahlf, W., and Calmano, W. (1993). Sediment quality objectives and criteria development in Germany. Water Sci. Technol. 28 (8-9), 307–316. doi:10.2166/wst.1993.0629
Fu, J., Zhao, C. P., Luo, Y. P., Liu, C. S., Kyzas, G. Z., Luo, Y., et al. (2014). Heavy metals in surface sediments of the Jialu River, China: their relations to environmental factors. J. Hazard. Mater. 270, 102–109. doi:10.1016/j.jhazmat.2014.01.044
Fu, T., Liang, H. H., Niu, L. X., Dang, H. M., Tao, W., and Yang, Q. S. (2022). Distribution characteristics and influencing factors of heavy metals at the sediment-water interface in the Pearl River Estuary during summer. Acta Oceanol. Sin. 44 (10), 182–192. [In Chinese]. doi:10.12284/hyxb2022166
Garnier, C., Lesven, L., Billon, G., Magnier, A., Mikkelsen, O., and Pizeta, I. (2006). Voltammetric procedure for trace metal analysis in polluted natural waters using homemade bare gold-disk microelectrodes. Anal. Bioanal. Chem. 386 (2), 313–323. doi:10.1007/s00216-006-0625-9
Gu, Y. G., Li, Q. S., Fang, J. H., He, B. Y., Fu, H. B., and Tong, Z. J. (2014). Identification of heavy metal sources in the reclaimed farmland soils of the pearl river estuary in China using a multivariate geostatistical approach. Ecotoxicol. Environ. Saf. 105, 7–12. doi:10.1016/j.ecoenv.2014.04.003
Hakanson, L. (1980). An ecological risk index for aquatic pollution control: a sedimentological approach. Water Res. 14 (8), 975–1001. doi:10.1016/0043-1354(80)90143-8
Huang, F. E., Xu, Y., Tan, Z. H., Wu, Z. B., Xu, H., Shen, L. L., et al. (2018). Assessment of pollution and identification of sources of heavy metals in sediments from west coast of Shenzhen, China. Environ. Sci. Pollut. Res. 25 (4), 3647–3656. doi:10.1007/s11356-017-0362-y
Ip, C. C. M., Li, X. D., Zhang, G., Farmer, J. G., Wai, O. W. H., and Li, Y. S. (2004). Over one hundred years of trace metal fluxes in the sediments of the Pearl River Estuary, South China. Environ. Pollut. 132 (1), 157–172. doi:10.1016/j.envpol.2004.03.028
Jia, J. B., Zhang, J. C., Zhang, H. N., Wang, Y. T., Xie, J. L., Hu, H. H., et al. (2021). Heavy metal content and ecological risk assessment in the water of the Pearl River Estuary. J. Dongguan Univ. Technol. 28 (1), 54–60. doi:10.16002/j.cnki.10090312.2021.01.010
Jiang, T., Lin, W. W., Cao, Y. J., Li, K., Xuan, Y. X., Li, R., et al. (2020). Heavy metal pollution, ecological risk assessment, and source analysis of sediments in Qingliangshan Reservoir in the Meijiang River Basin. Environ. Sci. 41 (12), 5410–5418. [In Chinese]. doi:10.13227/j.hjkx.202003018
Karbassi, A. R., Monavari, S. M., Bidhendi, G. R. N., Nouri, J., and Nematpour, K. (2008). Metal pollution assessment of sediment and water in the Shur River. Environ. Monit. Assess. 147 (1-3), 107–116. doi:10.1007/s10661-007-0102-8
Ke, X., Gui, S. F., Huang, H., Zhang, H. J., Wang, C. Y., and Guo, W. (2017). Ecological risk assessment and source identification for heavy metals in surface sediment from the Liaohe River protected area, China. Chemosphere 175, 473–481. doi:10.1016/j.chemosphere.2017.02.029
Kim, R. Y., Yoon, J. K., Kin, T. S., Yang, J. E., Owens, G., and Kim, K. R. (2015). Bioavailability of heavy metals in soils: definitions and practical implementation-a critical review. Environ. Geochem. Health 37 (6), 1041–1061. doi:10.1007/s10653-015-9695-y
Li, M., Zang, S. Y., Xiao, H. F., and Wu, C. S. (2014). Speciation and distribution characteristics of heavy metals and pollution assessments in the sediments of Nashina Lake, Heilongjiang, China. Ecotoxicology 23 (4), 681–688. doi:10.1007/s10646-014-1180-3
Liu, B. X., Luo, J., Jiang, S., Wang, Y., Li, Y. C., Zhang, X. S., et al. (2021). Geochemical fractionation, bioavailability, and potential risk of heavy metals in sediments of the largest influent river into Chaohu Lake, China. Environ. Pollut. 290, 118018. doi:10.1016/j.envpol.2021.118018
Liu, J. D., Guo, L., and Ke, Z. X. (2017). Heavy metal pollution and ecological risk assessment of surface sediments in the Pearl River Estuary. J. Hydroecology 38 (1), 46–53. [In Chinese]. doi:10.15928/j.1674-3075.2017.01.008
Lu, Q. Q., Bai, J. H., Zhang, G. L., and Wu, J. J. (2020). Effects of coastal reclamation history on heavy metals in different types of wetland soils in the Pearl River Delta: levels, sources and ecological risks. J. Clean. Prod. 272, 122668. doi:10.1016/j.jclepro.2020.122668
Lv, S. C., Zhang, H., Shan, B. Q., and Li, L. Q. (2013). Spatial distribution and ecological risk assessment of heavy metals in the estuaries surface sediments from the Haihe River Basin. Environ. Sci. 34 (11), 4204–4210. [In Chinese]. doi:10.13227/j.hjkx.2013.11.023
Maanan, M., Saddik, M., Maanan, M., Chaibi, M., Assobhei, O., and Zourarah, B. (2015). Environmental and ecological risk assessment of heavy metals in sediments of Nador lagoon, Morocco. Ecol. Indic. 48, 616–626. doi:10.1016/j.ecolind.2014.09.034
Medeiros, DCCD, Piechontcoski, F., Watanabe, ERLD, Chabes, E. S., and Inglez, S. D. (2020). Fast and effective simultaneous determination of metals in soil samples by ultrasound-assisted extraction and flame atomic absorption spectrometry: assessment of trace elements contamination in agricultural and native forest soils from Parana-Brazil. Environ. Monit. Assess. 192 (2), 111. doi:10.1007/s10661-020-8065-0
Min, L., Hou, L. J., Yang, Y., Zhou, L. M., and Meadows, M. E. (2021). The case for a critical zone science approach to research on estuarine and coastal wetlands in the anthropocene. Estuaries Coasts 44 (4), 911–920. doi:10.1007/s12237-020-00851-9
Ministry of Environmental Protection of China (2008). Soil environmental quality standard (GB 15618-2008). Beijing, China: China Environmental Science Press.
Morera, M. T., Echeverria, J. C., Mazkiarán, C., and Garrido, J. J. (2001). Isotherms and sequential extraction procedures for evaluating sorption and distribution of heavy metals in soils. Environ. Pollut. 113 (2), 135–144. doi:10.1016/S0269-7491(00)00169-X
Ndimele, P. E. (2012). The effects of water hyacinth (Eichhornia crassipes [mart.] solms) infestation on the physico-chemistry, nutrient and heavy metal content of badagry creek and ologe lagoon, lagos, Nigeria. J. Environ. Sci. Technol. 5, 128–136. doi:10.3923/jest.2012.128.136
Niu, L. X., Cai, H. Y., Jia, L. W., Luo, X. X., Tao, W., Dong, Y. H., et al. (2021a). Metal pollution in the Pearl River Estuary and implications for estuary management: the influence of hydrological connectivity associated with estuarine mixing. Ecotoxicol. Environ. Saf. 225, 112747. doi:10.1016/j.ecoenv.2021.112747
Niu, L. X., Li, J. Y., Luo, X. X., Fu, T., Chen, O. U., and Yang, Q. S. (2021b). Identification of heavy metal pollution in estuarine sediments under long-term reclamation: ecological toxicity, sources and implications for estuary management. Environ. Pollut. 290, 118126. doi:10.1016/j.envpol.2021.118126
Rauret, G., López-Sánchez, J. F., Sahuquillo, A., Rubio, R., Davidson, C., Ure, A., et al. (1999). Improvement of the BCR three step sequential extraction procedure prior to the certification of new sediment and soil reference materials. J. Environ. Monit. 1 (1), 57–61. doi:10.1039/a807854h
Ryan, P. C., Hillier, S., and Wall, A. J. (2008). Stepwise effects of the BCR sequential chemical extraction procedure on dissolution and metal release from common ferromagnesian clay minerals: a combined solution chemistry and X-ray powder diffraction study. Sci. Total Environ. 407 (1), 603–614. doi:10.1016/j.scitotenv.2008.09.019
Standardization Administration of China (1997). Soil quality-Determination of lead and cadmium using KI-MIBK extraction flame atomic absorption spectrophotometry (GB/T 17140-1997). [In Chinese].
Sundaray, S. K., Nayak, B. B., Lin, S., and Bhatta, D. (2011). Geochemical speciation and risk assessment of heavy metals in the river estuarine sediments-A case study: mahanadi basin, India. J. Hazard. Mater. 186 (2-3), 1837–1846. doi:10.1016/j.jhazmat.2010.12.081
Sungur, A., Soylak, M., Yilmaz, S., and Ozcan, H. (2014). Determination of heavy metals in sediments of the Ergene River by BCR sequential extraction method. Environ. Earth Sci. 72 (9), 3293–3305. doi:10.1007/s12665-014-3233-6
Suresh, G., Ramasamy, V., Meenakshisundaram, V., Venkatachalapathy, R., and Ponnusamy, V. (2011). Influence of mineralogical and heavy metal composition on natural radionuclide concentrations in the river sediments. Appl. Radiat. Isotopes 69 (10), 1466–1474. doi:10.1016/j.apradiso.2011.05.020
Topaldemir, H., Tas, B., Yuksel, B., and Ustaoglu, F. (2023). Potentially hazardous elements in sediments and Ceratophyllum demersum: an ecotoxicological risk assessment in Milic Wetland, Samsun, Turkiye. Environ. Sci. Pollut. Res. 30 (10), 26397–26416. doi:10.1007/s11356-022-23937-2
Usero, J., Gamero, M., Morillo, J., and Gracia, I. (1998). Comparative study of three sequential extraction procedures for metals in marine sediments. Environ. Int. 24 (4), 487–496. doi:10.1016/S0160-4120(98)00028-2
Ustaoglu, F., Yüksel, B., Tepe, Y., Aydin, H., and Topaldemir, H. (2024). Metal pollution assessment in the surface sediments of a river system in Türkiye: integrating toxicological risk assessment and source identification. Mar. Pollut. Bull. 203, 116514. doi:10.1016/j.marpolbul.2024.116514
Wang, E. R., Zhang, K. F., Chang, S., Zhang, M. L., and Fu, Q. (2023). Spatiotemporal distribution and pollution risk assessment of heavy metals in sediments of main water supply reservoirs in central Zhuhai city. Environ. Sci. 44 (1), 189–197. doi:10.13227/j.hjkx.202204006
Wang, N. N., Wang, A. H., Kong, L. H., and He, M. C. (2018). Calculation and application of Sb toxicity coefficient for potential ecological risk assessment. Sci. Total Environ. 610, 167–174. doi:10.1016/j.scitotenv.2017.07.268
Wang, S. L., Lin, C. Y., and Cao, X. Z. (2011). Heavy metals content and distribution in the surface sediments of the Guangzhou section of the Pearl River, Southern China. Environ. Earth Sci. 64 (6), 1593–1605. doi:10.1007/s12665-011-1172-z
Xie, W. P., Wang, S. B., Zhu, X. P., Chen, K. C., Pan, D. B., Hong, X. Y., et al. (2012). Heavy metal content and pollution assessment of sediments in the lower reaches of the Pearl River. Environ. Sci. 33 (6), 1808–1815. [In Chines]. doi:10.13227/j.hjkx.2012.06.020
Xu, D. Q., Wang, Y. H., Zhang, R. J., Guo, J., Zhang, W., and Yu, K. F. (2016). Distribution, speciation, environmental risk, and source identification of heavy metals in surface sediments from the karst aquatic environment of the Lijiang River, Southwest China. Environ. Sci. Pollut. Res. 23 (9), 9122–9133. doi:10.1007/s11356-016-6147-x
Xu, K. H., Milliman, J. D., and Xu, H. (2010). Temporal trend of precipitation and runoff in major Chinese Rivers since 1951. Glob. Planet. Change 73 (3-4), 219–232. doi:10.1016/j.gloplacha.2010.07.002
Yang, J., Chen, L., Liu, L. Z., Shi, W. L., and Meng, X. Z. (2014). Comprehensive risk assessment of heavy metals in lake sediment from public parks in Shanghai. Ecotoxiccology Environ. Saf. 102, 129–135. doi:10.1016/j.ecoenv.2014.01.010
Yang, Y. Q., Chen, F. R., Zhang, L., Liu, J. S., Wu, S. J., and Kang, M. L. (2012). Comprehensive assessment of heavy metal contamination in sediment of the Pearl River Estuary and adjacent shelf. Mar. Pollut. Bull. 64 (9), 1947–1955. doi:10.1016/j.marpolbul.2012.04.024
Ye, Z. P., Chen, J. Y., Gao, L., Liang, Z. B., Li, S. H., Li, R., et al. (2020). 210Pb dating to investigate the historical variations and identification of different sources of heavy metal pollution in sediments of the Pearl River Estuary, Southern China. Mar. Pollut. Bull. 150, 110670. doi:10.1016/j.marpolbul.2019.110670
Yu, G. B., Liu, Y., Yu, S., Wu, S. C., Leung, A. O. W., Luo, X. S., et al. (2011). Inconsistency and comprehensiveness of risk assessments for heavy metals in urban surface sediments. Chemosphere 85 (6), 1080–1087. doi:10.1016/j.chemosphere.2011.07.039
Yuksel, B., Ustaoglu, F., Aydin, H., Tokatli, C., Topaldemir, H., Islam, M. S., et al. (2024). Appraisal of metallic accumulation in the surface sediment of a fish breeding dam in Turkiye: a stochastical approach to ecotoxicological risk assessment. Mar. Pollut. Bull. 203, 116488. doi:10.1016/j.marpolbul.2024.116488
Yuksel, B., Ustaoglu, F., Tokatlli, C., and Islam, M. S. (2021). Ecotoxicological risk assessment for sediments of cavuslu stream in Giresun, Turkey: association between garbage disposal facility and metallic accumulation. Environ. Sci. Pollut. Res. 29 (12), 17223–17240. doi:10.1007/s11356-021-17023-2
Zemberyová, M., Barteková, J., and Hagarová, I. (2006). The utilization of modified BCR three-step sequential extraction procedure for the fractionation of Cd, Cr, Cu, Ni, Pb and Zn in soil reference materials of different origins. Talanta 70 (5), 973–978. doi:10.1016/j.talanta.2006.05.057
Zhang, Z. Y., Li, J. Y., Mamat, Z., and Ye, Q. F. (2016). Sources identification and pollution evaluation of heavy metals in the surface sediments of Bortala River, Northwest China. Ecotoxiccology Environ. Saf. 126, 94–101. doi:10.1016/j.ecoenv.2015.12.025
Zhao, Y. P., Wu, R., Cui, J. L., Gan, S. C., Pan, J. C., and Guo, P. R. (2020). Improvement of water quality in the Pearl River Estuary, China: a long-term (2008-2017) case study of temporal-spatial variation, source identification and ecological risk of heavy metals in surface water of Guangzhou. Environ. Sci. Pollut. Res. 27 (17), 21084–21097. doi:10.1007/s11356-020-08378-z
Keywords: heavy metal contamination, chemical speciation, ecological risk assessment, surface sediments, Pearl River Estuary
Citation: Liu Z, Wu Q, He C, Zhang P, Wang X, Luo Z, Yang L and Huang C (2024) Ecological risk and chemical speciation of heavy metals in surface sediments of the Pearl River Estuary for comprehensive assessment. Front. Environ. Sci. 12:1509277. doi: 10.3389/fenvs.2024.1509277
Received: 10 October 2024; Accepted: 14 November 2024;
Published: 06 December 2024.
Edited by:
Yalçın Tepe, Giresun University, TürkiyeReviewed by:
Bayram Yuksel, Giresun University, TürkiyeMuhammad El-Alfy, National Institute of Oceanography and Fisheries (NIOF), Egypt
Copyright © 2024 Liu, Wu, He, Zhang, Wang, Luo, Yang and Huang. This is an open-access article distributed under the terms of the Creative Commons Attribution License (CC BY). The use, distribution or reproduction in other forums is permitted, provided the original author(s) and the copyright owner(s) are credited and that the original publication in this journal is cited, in accordance with accepted academic practice. No use, distribution or reproduction is permitted which does not comply with these terms.
*Correspondence: Peng Zhang, UGVuZ19aaGFuZ1BAMTI2LmNvbQ==; Chaoqing Huang, aHVhbmdjaGFvcWluZ0B3aHUuZWR1LmNu