- 1Institute of Surface-Earth System Science, School of Earth System Science, Tianjin University, Tianjin, China
- 2Tianjin Key Laboratory of Earth’s Critical Zone Science and Sustainable Development in Bohai Rim, Tianjin University, Tianjin, China
- 3Tianjin Bohai Rim Coastal Earth Critical Zone National Observation and Research Station (Bohai National CZO), Tianjin University, Tianjin, China
- 4Haihe Laboratory of Sustainable Chemical Transformations, Tianjin, China
- 5State Key Laboratory of Remote Sensing Science, Aerospace Information Research Institute, Chinese Academy of Sciences, Beijing, China
- 6School of Life and Environmental Sciences, Schmid College of Science and Technology, Chapman University, Orange, CA, United States
Since the Anthropocene, ecosystems have been continuously deteriorating due to global climate change and human intervention. Exploring the changing characteristics of land use/land cover (LULC), landscape pattern and ecosystem service (ES) and their drivers is crucial for regional ecosystem management and sustainable development. Taking the Bohai Rim region of China as an example, we used the land use transfer matrix, landscape pattern index and InVEST model to analyze the changing characteristics of LULC, landscape pattern and six key ESs [crop production (CP), water yield (WY), carbon storage (CS), soil conservation (SC), habitat quality (HQ), landscape aesthetics (LA)] during 2000–2020. Detailed analysis of the natural and anthropogenic factors affecting the landscape pattern and ES changes has been considered in this study. The results show that the areas of forest, water and impervious land increased, while those of cropland, shrubs, grassland and barren land decreased during 2000–2020. The landscape was fragmented, complex and decentralized during 2000–2015, while the three trends eased during 2015–2020. From 2000 to 2020, CP, WY, and SC capacity show an increasing trend, while CS, HQ, and LA capacity tend to a decline. Natural factors (e.g., precipitation, temperature, altitude) and human factors (e.g., technological progress, policy changes, and LULC forms) are the main factors affecting landscape pattern and ESs. The present study can provide theoretical basis for ecological restoration, ecological product value realization, and land planning in the typical developed urban area.
1 Introduction
Land is dynamic, its monitoring provides information about the human impacts on natural ecosystems environment, socio-economics and policies. Land use/Land cover (LULC) change is related to many ecological problems, such as soil erosion, land desertification and biodiversity loss (Zhang et al., 2020). It changes the supply of ecosystem products and services by affecting ecosystem structure and processes (e.g., vegetation cover, carbon sources and sinks), and has become the most important driving force affecting landscape pattern and ecosystem service (ES) (Assesment, 2005). Human estimated that LULC change has affected almost a third (32%) of the global land area in just 6 decades (1960–2019) and the area affected by global LULC change is nearly four times greater than previously thought (Winkler et al., 2021). A finite land area implies that fulfilling sustainable development goals requires increasing land-use efficiency of both storing carbon and producing food (Searchinger et al., 2018). Quantifying the dynamics of LULC change is critical in tackling global societal challenges such as food security, climate change, and biodiversity loss.
Landscape pattern refers to the structural composition and spatial configuration of landscape elements (forests, cropland, cities, etc.) (Wang et al., 2022), which is used to describe how landscape elements are spatially distributed and interconnected (Fu et al., 2022). Landscape pattern is influenced by human activities and climate change, its changes at the regional scale can directly affect energy flow and material circulation of ecosystem (Teutschbein et al., 2018), thus influencing the effects of ESs (Shao et al., 2023). The knowledge of the landscape pattern changes is the basis for comprehensively understanding regional ecological and environment changes, identifying ecological risks (Jiang et al., 2023), and rational planning LULC policies reasonably (van der Plas et al., 2019). Therefore, a comprehensive quantification of global landscape fragmentation is urgently required to guide landscape protection and restoration policies (Ma et al., 2023).
ESs refer to the benefits that humans obtain from ecosystems, typically divided into provisioning, supporting, regulating and cultural services (Assesment, 2005). ESs are considered as a bridge between natural ecosystems and human societies. The strategic planning for ESs could reduce tradeoffs between environmental quality and development (Bai et al., 2018). However, ESs have been continuously degraded since the Anthropocene as a result of global climate change and human intervention (Liu et al., 2024a). The Millennium Ecosystem Assessment (MA) report points out more than 60% of the world’s ecosystems are currently or have already degraded (Assesment, 2005). The Intergovernmental Science-Policy Platform on Biodiversity and Ecosystem Services (IPBES) found that 14 of 18 ecosystem components have declined since 1970 (Liu et al., 2022a). Therefore, it is necessary to accurately assess the spatiotemporal distribution of ESs and identify their potential drivers. This is the basis for avoiding the continuous degradation of ESs and the prerequisite for optimizing land use policies and delineating ecological red lines (Jiang et al., 2019; Ouyang et al., 2016).
The study of landscape heterogeneity is the focus of landscape pattern analysis and the landscape pattern indices are usually used to quantitatively evaluate regional landscape patterns, describe the spatial morphology, structure and heterogeneity of regional landscapes (Li et al., 2017). Currently, the research on landscape pattern has expanded to the fields of landscape ecological risk, ecological security pattern, ecological restoration, and has become the core of landscape ecology research. After the discussion of concepts, connotations and classification methods (Costanza et al., 1997; Fisher et al., 2009), the research on ESs has mainly focused on trade-offs and synergies, ecosystem service bundles (ESB). There are two main types of evaluation methods for ESs, namely, the value and the material quality methods. The material quantity method can objectively reflect the formation mechanism of ESs. The evaluation results are objective, stable, highly reliable, and do not change dramatically with the scarcity of ES. In particular, assessments using satellite remote sensing data have the advantages of finer spatial resolution, more comprehensive coverage and better temporal monitoring capabilities compared to traditional administrative unit-scale assessments. They avoid the locality and partiality that may exist in ground surveys and can more effectively identify the spatial distribution and changing patterns of ESs. The InVEST model can evaluate a wide range of ESs and can be combined with multi-source data, which has been widely used globally. However, existing studies on landscape patterns and ESs are mostly single-type studies, lacking systematic studies combining the two. At the same time, most studies are concentrated at a single city scale, with fewer studies at the city cluster scale, and they mostly concentrated in metropolitan areas such as the Yangtze River Delta and the Pearl River Delta, lacking studies on a broader scale of urban agglomerations. In addition, most studies focus on terrestrial ESs, with fewer studies on coastal and offshore ESs (Liu et al., 2020).
The Bohai Rim region is a key area in the Beijing-Tianjin-Hebei coordinated economic development strategy, and is rich in coastal ecological resources, with numerous types of estuarine deltas and coastal wetland reserves. However, it is also an ecologically fragile area. Since the area is a natural semi-enclosed bay surrounded by land on three sides, it has inherent characteristics such as insufficient hydrodynamics and weak water exchange capacity. In recent years, with the dual impact of global climate change and rapid urbanization, a series of ecological and environmental problems have emerged, including increased land pollution emissions, serious industrial and agricultural pollution (Zhao et al., 2021), fragmentation and isolation of coastal key belt landscapes, decreased ecological connectivity (Liu et al., 2020), and a significant reduction in natural wetland area (Wang et al., 2021). At present, it is urgent to conduct in-depth research on the landscape pattern and ESs in the Bohai Rim region.
In this study, we quantitatively assessed six landscape pattern indices and six ESs in the Bohai Rim region based on the analysis of LULC changes from 2000 to 2020 using Fragstats software, InVEST model, statistical data and landscape indicator assessment. Here, we focused to (1) clarify the changing characteristics of LULC, landscape patterns and ESs in the Bohai Rim region, (2) analyze the possible drivers affecting their spatial and temporal distributions, (3) propose detailed ecological management recommendations for different key zones in the Bohai Rim region.
2 Materials and methods
2.1 Study area
The Bohai Rim region is an important industrial and agricultural base in China, with rich agricultural, mineral, oil and gas and tourism resources. This region, centered on Beijing and Tianjin, is an important economic zone in eastern China with a concentrated population and increasingly concentrated industries. With a variety of ecosystems, including estuarine delta wetlands, cropland, breeding ponds, salt flats, forests, coastal mudflats and towns, the Bohai Rim region is an important part of the ecosystems in northern China and provides a wide range of ESs (Ning et al., 2011). With the rapid development of urbanization and industrialization in the Bohai Rim region, coastal development activities have become increasingly frequent, and LULC patterns have changed significantly. In addition, the Bohai Sea is surrounded by land on three sides, and there are problems such as innate hydrodynamic insufficiency and weak exchange capacity of water bodies. Global climate change and human activities have exposed the ecological environment in the Bohai Rim region to multiple risks such as coastal wetland shrinkage, nearshore soil salinization, biodiversity reduction, and water environment deterioration. The Bohai Rim region studied in this article includes 15 coastal cities, including Tianjin, Hebei Province (Qinhuangdao, Tangshan, Cangzhou), Shandong Province (Yantai, Weihai, Dongying, Qingdao, Binzhou, Weifang), and Liaoning Province (Dalian, Yingkou, Panjin, Jinzhou, and Huludao) (Figure 1).
2.2 Data sources and processing
The main data of this study includes two categories: spatial data and statistical data. All spatial data were uniformly converted to the WGS_1984_UTM_50N coordinate system, and specific data information is shown in Table 1. Based on current situation of study area, we reclassified the snow land in the original LULC data into barren land and the wetland into water, which is divided into 7 types: cropland, forest, shrub, grassland, water, barren land, and impervious land. The MOD16A2GF product has a temporal resolution of 8 days, and the MOD13A3 product is monthly NDVI raster data. The two are batch projected by MRT (MODIS Reprojection Tools) software, and the Maximum Value Composites method was used to form the PET and NDVI datasets of each year. DEM data was filled in ArcGIS software. The final assessment results of each ES are at a resolution of 30 m.
2.3 Research methods
2.3.1 LULC change analysis
The method of LULC transfer matrix is used to study LULC change, and the application of its results can clearly represent the specific structural characteristics of LULC and the changes of functional types of LULC. The expression is shown in Equation 1:
In the formula, S represents the area (km2), Sij represents the area transfer matrix, n represents the LULC type, i and j represent different LULC types during the study period. If i = j represents the area of LULC type that has not changed (Lai et al., 2016).
2.3.2 Landscape pattern index selection
This study selected patch density (PD), edge density (ED), landscape shape index (LSI), aggregation index (AI), contagion (CONTAG), and Shannon’s diversity index (SHDI) from two aspects: patch metrics and landscape metrics. They were used to reflect landscape fragmentation, complexity, and aggregation. Detailed information of each index is shown in Table 2. All landscape pattern indices were calculated in Fragstats 4.2 software.
2.3.3 Ecosystem services assessment
Based on the ES classification system proposed by The Millennium Ecosystem Assessment (MA), and according to the actual situation and research foundation of the Bohai Rim region, six important ESs were selected: crop production (CP), water yield (WY), carbon storage (CS), soil conservation (SC), habitat quality (HQ), and landscape aesthetics (LA) (Table 3).
CP and WY are Provisioning services. In the past 20 years, cropland in the Bohai Rim region has been continuously decreasing, the coastal land has been salinized, and extreme droughts and floods disasters have occurred frequently, which has a great impact on CP. Therefore, evaluating CP and WY is of great significance to ensuring the wellbeing of the people‘s lives. The coastal wetlands in the Bohai Rim region have been seriously degraded, resulting in a decrease in CS capacity and weakened flood control capacity. Deforestation and inappropriate construction activities have increased the risk of soil erosion and caused the loss of fertile topsoil. Therefore, CS and SC were selected as the research objects of regulating services. The coastal zone around the Bohai Rim region has been seriously eroded and faces multiple ecological risks such as eutrophication and biodiversity degradation. Therefore, HQ was selected as the research object of supporting services. The Bohai Rim region has a wide variety of landforms, covering mountains, plains, estuaries, coasts, islands, wetlands, etc., which is a microcosm of China’s landform diversity. Therefore, LA were selected to represent the cultural services of the region.
2.3.3.1 CP
The CP data obtained from the statistical yearbooks of provinces and cities in the Bohai Rim region includes grains, beans, and potatoes, all of which are produced on cropland. Previous studies have shown that there is a significant linear relationship between CP and NDVI, therefore CP is allocated to cropland grids according to the NDVI value (Equation 2).
In the formula: Gi is the crop production in the i-grid (kg); Gsum is the total crop production in the study area (kg); NDVIi is the normalized vegetation index of the i-grid; NDVIsum is the sum of NDVI values for cropland in the study area.
2.3.3.2 WY
WY affects human production and life in various ways. This study uses the Annual Water Yield module in the InVEST model to estimate WY services, the specific model parameters are presented in the Supplementary Table S1. This module is based on the principle of water balance and uses the difference between regional rainfall and evapotranspiration to determine WY (Equation 3) (Hu et al., 2019).
In the formula: Y(x) is the annual water yield of regional unit x (mm), P(x) and AET(x) are the annual rainfall and actual evapotranspiration of grid x (mm), respectively.
2.3.3.3 CS
The widely distributed wetland ecosystems in the Bohai Rim region are particularly important carbon sinks that can store a large amount of organic carbon. The Carbon Storage module of the InVEST has been widely used to evaluate CS. It divides the CS of each LULC type into four basic carbon pools: aboveground biomass carbon (Cabove), belowground biomass carbon (Cbelow), soil organic carbon (Csoil), dead organic matter carbon (Cdead) (Zhu et al., 2020), The calculation formula is as follows (Equations 4, 5):
In the formula: i represents a certain LULC type;
2.3.3.4 SC
The InVEST model Sediment Delivery Ratio module (SDR) was used to simulate and evaluate SC. This module is a spatial display model for raster calculation. Based on the modified general soil loss equation, it uses LULC data, DEM data, soil property data, precipitation data, vegetation cover factors, soil and water conservation measures factors to calculate the annual soil erosion and sediment transport rate of the grid, and then simulates the raster soil erosion and sediment transport process (Liu et al., 2023), The calculation formula is as follows (Equations 6–8):
In the formula:
2.3.3.5 HQ
The maintenance of biodiversity is closely related to HQ. Use the Habitat Quality module of the InVEST to evaluate the HQ index, with a range of values from 0 to 1. The larger the value, the higher the HQ. The formula is as follows (Equation 9):
In the formula,
2.3.3.6 LA
LA refers to the benefits and comfort that individuals gain from interacting with visually pleasing natural landscapes, and is an intangible cultural service (Langemeyer et al., 2018). The evaluation of LA is often based on two criteria: naturalness and landscape diversity. Naturalness refers to the degree of human interference, while landscape diversity reflects the diversity of landscape structure (Frank et al., 2013). Naturalness is quantified using the chlorophyll index from 1 (natural) to 7 (artificial) (Xia et al., 2023b). Landscape diversity was quantified using the Shannon’s diversity index (SHDI) in Fragstats 4.2 software. The final LA index was obtained by adding the normalized values of naturalness and landscape diversity (Equation 10).
In the formula:
3 Results
3.1 LULC distribution characteristics
The LULC types in the Bohai Rim region are mainly cropland land, impervious land and forest, among which cropland accounts for the largest proportion, exceeding 60% at all stages of the study period. Impervious land and forest account for more than 14% and 11%. Shrubs account for the smallest proportion, with an average area of only 1.1 km2 over 20 years (Table 4).
From the perspective of the spatial distribution (Figure 2), cropland is widely distributed, covering most of the Bohai Rim region; forest is mainly distributed in the north and northwest, shrubs and grasslands are mainly distributed in the northwest of the study area and the northern area of Shandong Peninsula; water is mainly distributed on the edge of the Bohai Bay; barren land is mainly distributed in the edge of Laizhou Bay and Bohai Bay, as well as the Yellow River Delta; impervious land are mainly distributed in the marginal areas of the coast and the city centers.
From the perspective of the temporal distribution, the proportions of forest, water and impervious land have expanded from 11.16%, 3.45%, 14.08% in 2000 to 11.67%, 4.05%, 21.60% in 2020; while the area proportions of cropland, grassland and barren land decreased from 67.21%, 2.63%, 1.47%–60.71%, 1.77% and 0.21%. From 2000 to 2020, the area of forest and shrub showed a trend of first decreasing and then expanding. This study further calculated the LULC transfer matrix (Table 5) and drew the LULC transfer Sankey diagram (Figure 3). During the study period, 12,841.78 km2 of cropland was transferred out and 2,957.05 km2 was transferred in, mainly transforming into impervious land, forest and water; 761.37 km2 of impervious land was transferred out and 12,184.74 km2 was transferred in, mainly from cropland and water. 1,556.34 km2 of forest was transferred out and 2,322.11 km2 was transferred in, mainly from cropland and water; 2,111.99 km2 of water was transferred out and 3,023.37 km2 were transferred in, mainly from cropland and barren land. Overall, the LULC types in the Bohai Rim region changed drastically, mainly among cropland, impervious land, forest, and water.
3.2 Landscape pattern index characteristics
At the landscape metrics (Figure 4), PD and SHDI both increased from 2000 to 2015 and decreased from 2015 to 2020, indicating that the degree of regional landscape fragmentation first increased and then decreased. ED and LSI increased rapidly from 2000 to 2015 and decreased from 2015 to 2020, indicating that the landscape shape first became complex and then tended to be simplified. AI and CONTAG both decreased significantly from 2000 to 2015 and increased from 2015 to 2020, indicating that the connectivity between landscape patches weakened and the separation increased in the early stage, which was alleviated in the later stage.
At the patch metrics (Figure 5), PD, ED and LSI of cropland increased, while AI decreased, indicating that cropland landscape has an obvious trend of fragmentation, complexity and dispersion. PD of forest decreased from 2000 to 2010 and increased from 2010 to 2020, its ED and LSI continued to decrease, and AI continued to increase, indicating that the degree of fragmentation in forest first decreased and then increased, with a process of simplified shape and aggregation of patches. Shrubs have fewer patches, are easily affected by external conditions, and change erratically. PD, ED, LSI and AI of grassland continued to decrease. PD, ED and LSI of water increased from 2000 to 2015, then decreased slightly from 2015 to 2020, but were still higher than the level of 2000; its AI decreased during 2000–2015 and increased significantly from 2015 to 2020. PD and LSI of barren land increased, AI decreased, ED increased during 2000–2015 and decreased to a level lower than that in 2000 during 2015–2020. PD and LSI of the impervious land continued to decrease, while ED and AI continued to increase.
3.3 Distribution characteristics of ESs
3.3.1 Provisioning services
3.3.1.1 CP
In general, CP shows an increasing trend from 2000 to 2020 (Figure 6A). The total crop production in 2000, 2005, 2010, 2015 and 2020 was 2365.12wt, 2802.27wt, 3,265.03wt, 3,049.83wt, 3,139.67wt, and the yield per unit area was 1,526.20 kg/hm2, 1808.29 kg/hm2, 2106.91 kg/hm2, 1968.04 kg/hm2, 2026.01 kg/hm2 respectively. CP continued to increase from 2000 to 2010, increasing by 38% in 2010 compared with 2000, and decreased slightly from 2010 to 2020.
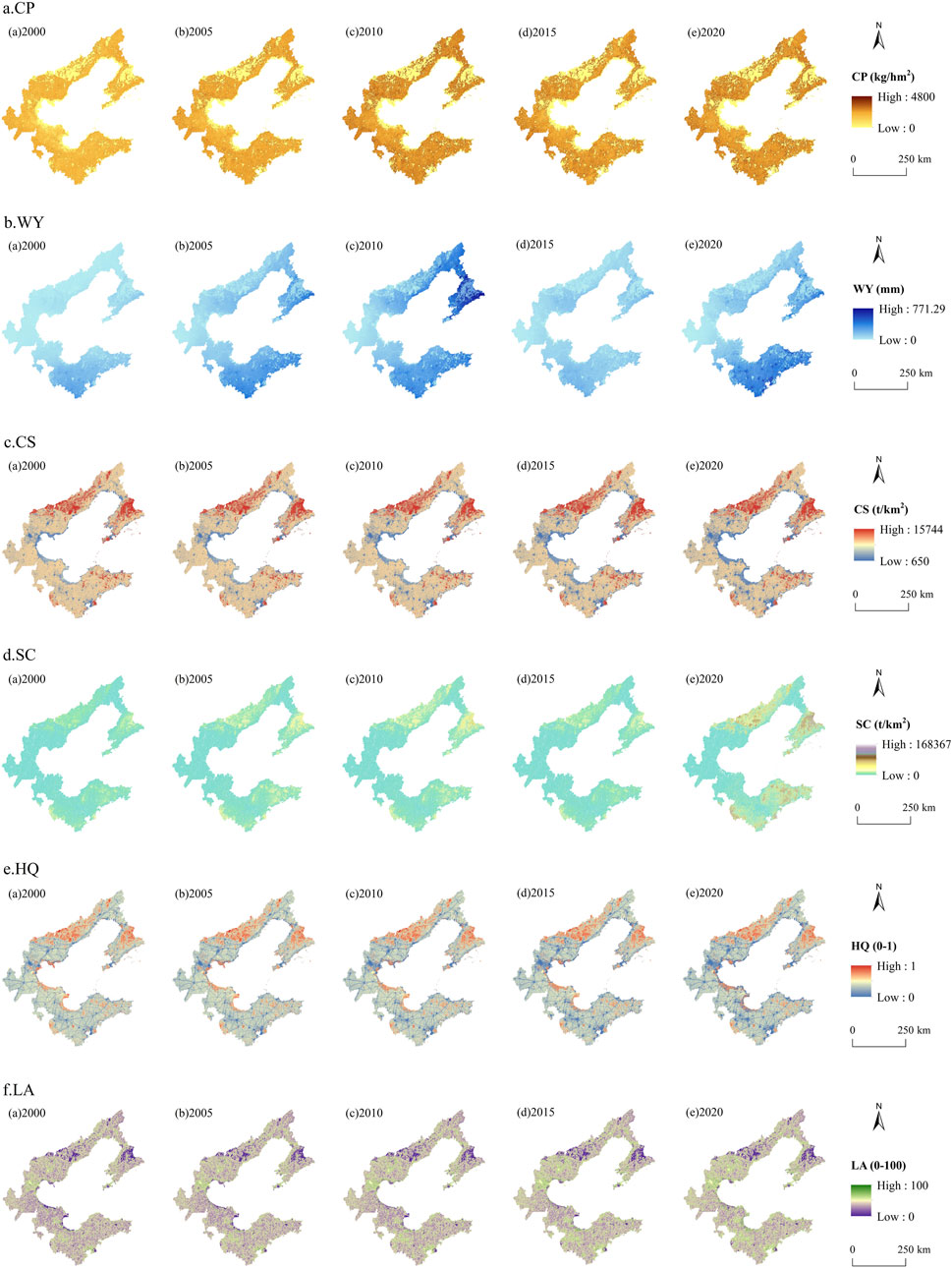
Figure 6. Spatial distribution of ecosystem services in the Bohai Rim region of China during 2000–2020.
3.3.1.2 WY
WY has generally shown an increasing trend (Figure 6B). The total water yield in 2000, 2005, 2010, 2015 and 2020 was 10.07 billion m3, 23.56 billion m3, 25.73 billion m3, 12.86 billion m3 and 26.35 billion m3. In 2015, WY decreased by 12.863 billion m3 compared with 2010. The spatial distribution of WY in the Bohai Rim region is high in the north and south and low in the center. The high-value areas are mainly distributed in the Shandong Peninsula, the coast of Liaodong Bay and the Liaodong Peninsula, while the low-value areas are mainly distributed in Tianjin, Cangzhou, the northern part of Qinhuangdao and Huludao, and the central and northern part of Dalian.
3.3.2 Regulating services
3.3.2.1 CS
From a temporal perspective, carbon storage shows a decreasing trend (Figure 6C). The total carbon storage in 2000, 2005, 2010, 2015 and 2020 was 134.87
3.3.2.2 SC
(2) SC: From a temporal perspective, soil conservation generally showed an increasing trend (Figure 6D). The SC level in 2000, 2005, 2010, 2015 and 2020 were 3,579.86 t/km2, 5,853.93 t/km2, 6,463.65 t/km2, 4,236.31 t/km2 and 7,071.22 t/km2. In 2015, SC significantly decreased, with a decrease of 33,848.90wt compared to 2010. From a spatial perspective, SC was generally low but locally high. Liaoning Province has the strongest SC capacity at 8,302.31 t/km2, which is 1.53 times the average value of the Bohai Rim region. Yingkou has the highest average SC capacity at 13,866.25 t/km2. Tianjin has the lowest SC capacity, which is 1,363.94 t/km2, only 25% of the average value.
3.3.3 Supporting services
3.3.3.1 HQ
From a temporal perspective, HQ index has been declining (Figure 6E). The average HQ index in the study area in 2000, 2005, 2010, 2015 and 2020 was 0.39, 0.38, 0.37, 0.36 and 0.35. In the past 20 years, the HQ index has decreased by 10.26%, indicating that the overall HQ of the ecosystem in the Bohai Rim region has been deteriorating. From a spatial perspective, the HQ in the Bohai Rim region has significant spatial heterogeneity, with high values mainly distributed in Qinhuangdao, Dalian, Yingkou, Huludao, Bohai Bay and the coast of Laizhou Bay. Low values are mainly distributed in Qingdao, Tianjin, Cangzhou and Weifang. These areas have large populations, high human activity intensity, large demand for infrastructure construction, and rapid economic development, which drives the gradual expansion of non-habitat areas.
3.3.4 Cultural services
3.3.4.1 LA
From a temporal perspective, LA index in the Bohai Rim region has been continuously increasing (Figure 6F). The average LA index of the study area in 2000, 2005, 2010, 2015, and 2020 were 40.07, 40.81, 41.70, 42.70, and 43.56. From 2000 to 2020, the LA index increased by 8.71%, indicating that the quality of LA has continued to deteriorate. From a spatial perspective, Yingkou, Qinhuangdao, and Huludao have the lowest LA values, indicating that residents can gain more benefits and comfort from the interaction with the natural landscape in these areas. The higher cultural services in these areas can help realize the value of cultural ecological products, such as developing ecotourism, promoting cultural tourism consumption and creating characteristic tourism brands. Tianjin, Tangshan and Qingdao have the highest LA values. This means that the natural landscapes in these areas provide residents with lower spiritual comfort, which is not conducive to the realization of ecological product value and regional sustainable development.
4 Discussion
4.1 InVEST model results accuracy verification
The verification of the WY is based on the data from the Water Resources Bulletin. The actual water resources in the study area in 2000, 2005, 2010, 2015, and 2020 were 10.423 billion m3, 23.135 billion m3, 23.267 billion m3, 14.07 billion m3 and 23.54 billion m3 respectively. The error between the model simulation results and the actual water resources is controlled at around 10%, so the results are reliable.
The carbon density data of CS were considered in accordance with the following principles: the four basic carbon pool data were considered from published data obtained from field investigations. The missing data were used from neighboring regions. This approach and criteria ensured the credibility of the results to the greatest extent.
The assessment results obtained from the SC module were verified with reference to relevant research (Feng et al., 2022; Wang et al., 2022). Since the Bohai Rim region spans multiple watershed divisions, the results are also within the overall range. Therefore, it has a reasonable value.
The evaluation results of the HQ by province are basically consistent with the findings of Tianjin (Li et al., 2022b) and Dongying (Ding et al., 2021), with a difference of about 10%. In addition, the HQ change rate of Dongying from 2000 to 2020 is found to be consistent with the trends in the Yellow River Delta (Liu et al., 2022b), with a change rate of about 15.8%, this provides a good confidence.
4.2 Analysis of factors influencing landscape pattern and ESs
4.2.1 Analysis of factors influencing landscape patterns
In general, before 2015, landscape fragmentation increased, and the patch shapes tended to be complex and dispersed. This situation improved after 2015. The reasons for the landscape fragmentation, complexity, and dispersion from 2000 to 2015 include two aspects. First, intense human activities such as cropland occupation, urban construction, and rail transit construction divided the originally coherent natural patches into discrete small patches. Second, returning cropland to forests and lakes, various reclamation activities have led to a significant transformation in LULC patterns, exacerbating the degree of regional landscape fragmentation, complexity and decentralization. In 2015, China issued the “Opinions on Accelerating the Construction of Ecological Civilization”, to increase the forest, grassland and wetland coverage rate by 2020. The “National Land Planning Outline (2016–2030)” and the “National Agricultural Sustainable Development Plan (2015–2030)” followed strategies at the same time, both requiring strict ecological protection system. The fragmented patches cropland areas have been integrated; the main landscape types are considered as a large area. Highly urbanized areas continued to expand, and small built-up areas are integrated with large landscapes, making the landscape homogenized. The degree of patch aggregation was high, and the fragmentation and complexity of the landscape were alleviated.
The continuous policy of returning cropland to forest and the occupation of cropland have increased the fragmentation and complexity of the cropland landscape and reduced its aggregation. The policy of returning cropland to forest requires converting eligible sloping cropland with a degree of 25 or above into forests and grasslands, which has formed a larger area of fragmented forest in the later period (Hao et al., 2017), making the degree of forest fragmentation increased during 2010–2020. It can be seen from the LULC transfer matrix that the area of grassland transferred out is larger than the area transferred in, and the fragmented patches are converted into cropland and forest, which are integrated with the larger landscape. Therefore, the degree of landscape fragmentation of grassland continues to decrease, and patches become more simplified and dispersed. From 2000 to 2015, various human activities such as land reclamation, aquaculture, and reservoir construction, as well as the policy of returning cropland to lakes, have made the regional water landscape fragmented, complex, and decentralized. In 2015, China further increased its support for wetland protection, and the central government allocated $223.2 million in wetland subsidies, covering wetland protection and restoration, returning cropland to wetlands, and ecological compensation. With the new relevant policies, the area and quantity of wetlands increased significantly from 2015 to 2020, expanding the connectivity between wetlands and making their shapes simpler. As a result, the fragmentation, complexity, and dispersion of water landscape patches were reduced. The area of barren land decreased while the impervious land expands year by year. Therefore, the landscape pattern of the two is opposite. In the process of barren land converting into impervious land, although the landscape pattern is fragmented, complex, and dispersed. The expansion of the impervious land increases the uniformity of its patches, reducing the degree of fragmentation and the complexity of the landscape, increasing the aggregation of patches.
4.2.2 Analysis of factors influencing ESs
The natural factors, precipitation, temperature and altitude are the main factors affecting ESs (Figure 7). In 2015, the average precipitation in the study area was only 562 mm, about 83% of that in 2010 (Figure 8), resulting in a decrease of 215.2wt in CP in 2015 compared with 2010, a decline of about 6.6%; WY decreased by 12.862 billion m3 compared with 2010, a decline of about 50%; the decline in precipitation directly changed the precipitation runoff erosion rate (Zhang et al., 2022), that contributed decline of 2,227.34 t/km2 in SC intensity in the Bohai Rim region, which is consistent with the Luan River Basin (Liu et al., 2023). The temperature mainly affects ESs through its influence on regional evapotranspiration. In 2015, the average temperature in the study area was 12.51°C, which was 0.81°C higher than in 2010 (Figure 8). This increased the potential evaporation of vegetation and significantly reduced the WY. The northern parts of Dalian and Huludao, the southern part of Yingkou in Liaoning Province are located at higher altitudes, with less urbanization and human activities. Vegetation coverage, soil thickness and biodiversity all increase with altitude, and the SC capacity is the strongest. Similarly, Yantai and the southwestern part of Weifang in Shandong Province are at higher altitudes, coupled with higher annual precipitation throughout the province, so the SC capacity is relatively strong. However, most areas of Hebei province and Tianjin are at a low altitude, with low vegetation coverage, few artificial protection measures, and high intensity of urban construction, resulting in low SC function.
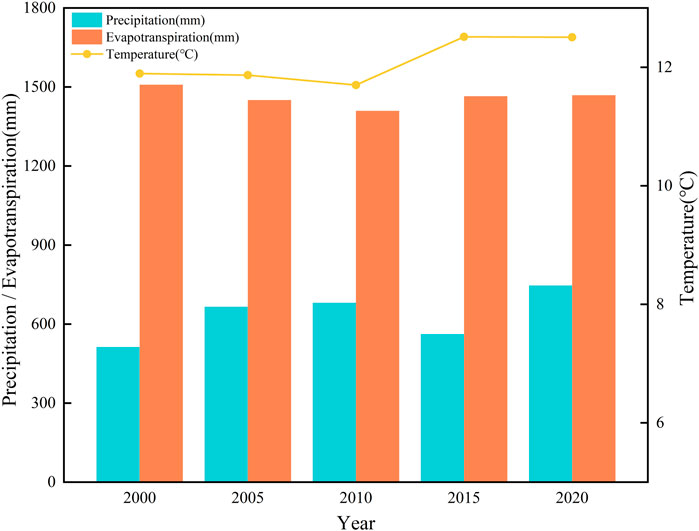
Figure 8. Changes in average temperature, precipitation and evapotranspiration in the Bohai Rim region during 2000–2020.
The human factors such as, development of technology, policy changes and LULC patterns are the main factors affecting ESs. From 2000 to 2010, crop varieties and planting technologies developed rapidly, and CP increased rapidly. In 2020, the Bohai Rim region faced multiple threats such as severe floods and COVID-19 epidemic, and the total CP declined. However, the government increased its support for crop production, implemented various policies to support agriculture and benefit farmers, actively responded to epidemics and disasters. So the impact of agricultural disasters on CP was limited. The LULC form is an important factor affecting regional ESs. Shandong Province is in the eastern coastal area and the middle and lower reaches of the Yellow River. It has superior geographical conditions and rich natural resources, with a small proportion of mountains, a vast area of cropland, and the CP level is high. Hebei and Liaoning provinces have widespread forest distribution and a large proportion of aquaculture. The effective cropland planting area is relatively small, and the degree of nearshore soil salinization is serious, resulting in low regional CP. Different LULC have different supply capacities for ESs due to differences in vegetation coverage, vegetation canopy height, canopy interception, soil moisture content, evapotranspiration capacity, and litter water holding capacity. The vegetation coverage of cropland and impervious land is low, the soil has a weak ability to absorb water, making it easy to form runoff (Guo et al., 2023), so the WY is relatively high. At the same time, these areas are either typical areas of urban expansion or key areas for agricultural planting. Urban construction and human activities have caused serious damage to HQ and LA. Due to the lack of artificial protection measures, SC and CS are also relatively low. Forests, shrubs and grasslands, due to their strong interception effect on precipitation and high evapotranspiration coefficients, are not conducive to water retention and have low WY level. However, due to its high vegetation coverage and less interference from human activities, the corresponding soil texture is less erodible, resulting in higher SC, HQ, and LA level.
4.3 Typical area analysis
The Bohai Rim region is vast, and due to differences in natural resource endowments and socio-economic factors among administrative, various ESs are highly heterogeneous and dynamic. For example, when comparing Qinhuangdao and Tianjin, the dominant ESs in Tianjin are provisioning services, while those in Qinhuangdao are regulating, supporting and cultural services (Table 6). The average CP in Qinhuangdao in the past 20 years is 80.28wt, accounting for 2.74% of the total area. While Tianjin has a greater advantage in CP, with an average of 166.95wt, accounting for 5.71%, about twice that of Qinhuangdao. However, the regulating service of Qinhuangdao is much higher than that of Tianjin, especially SC. The area of Tianjin is about 11,966.45 km2 and the SC is 1632.15wt, while the area of Qinhuangdao is about 7750 km2 and the SC is 7407.67wt, about 4.5 times those of Tianjin. In the past 20 years, the supporting and cultural service of Qinhuangdao have been much higher than the average level of the Bohai Rim region, while Tianjin is lower than it (Figure 9).
In the past 20 years, CP in Tianjin has continued to improve (Table 7). Compared with Qinhuangdao, the growth trend in Tianjin is stable, indicating that it has a stronger ability to resist natural risks and human interference, and is a key area for CP. WY in Tianjin has continued to decline since it increased to a maximum of 0.63 billion m3 in 2005, while the overall precipitation has not changed much from 2005 to 2020. It indicates that the WY in Tianjin is highly related to human activities. Although the amount of WY increased due to the dramatic expansion of impervious land, this high value has been offset by the reduction in cropland with similarly high WY capacity. At the same time, with the implementation of policy documents such as returning cropland to the lake, “Tianjin Land Use Master Plan (2006–2020),” “Tianjin Wetland Protection Ordinance,” “Tianjin Wetland Nature Reserve Plan (2017–2025),” Tianjin has established an eco-friendly land use pattern that coordinates rivers, beaches, bays, lakes and seas. The internal structure and spatial layout of ecological construction and wetland protection land have been optimized, and many artificial wetlands have been integrated to form a wetland ecological network with higher connectivity and better aggregation, making WY in Tianjin more balanced and reducing the risk of floods caused by urban construction.
Qinhuangdao has distinct regional characteristic and is a famous coastal touristic area, leisure and holiday resort in China. Tourism and port logistics are its traditional advantageous industries. Qinhuangdao has outstanding capabilities in regulating, supporting and cultural services, with CS, SC, HQ, and LA all at a leading level in the Bohai Rim region (Table 8). This is mainly due to the warm temperate semi-humid continental monsoon climate in this region, which is greatly affected by the ocean. The climate is mild, with little rain and dryness in spring, warm and no scorching heat in summer, cool and sunny in autumn, and long and no severe cold in winter. In addition, the northern part of Qinhuangdao is dominated by mountainous and low hills, with forests, shrubs and grasslands as its main LULC types, which have good CS, SC and HQ capabilities. However, in the past 20 years due to the development of large-scale construction projects such as Qinhuangdao Economic and Technological Development Zone, Qinhuangdao Seaport Economic Development Zone, and Qinhuangdao Port, the ESs have degraded to varying degrees, among which CS has declined by 243wt, HQ by 4.92% and LA index has increased by 4.18%. In the future, Qinhuangdao should continue to leverage its regional advantages. The southeastern coastal areas should promote the concentrated and contiguous protection of agricultural land, strictly control the occupation of cropland by new construction, and revitalize existing construction land. The northern areas should effectively protect water sources and ecologically fragile areas, conduct comprehensive management of soil erosion and land degradation to ensure the continuous supply of ESs.
4.4 Policy planning suggestions
The natural environmental conditions and human activities are important factors influencing the regional landscape patterns and ESs. No single development policy can improve all landscape patterns and ESs. How to resolve the contradiction between protection and development is the core issue of ecological civilization construction (Li et al., 2022a). Firstly, people should actively respond to the influence of natural factors. The Bohai Rim region has a temperate monsoon climate with uneven spatial distribution of rainfall, which is vulnerable to drought risks. Therefore, the provisioning service levels such as crop production and water yield in most areas fluctuate greatly. It is necessary to vigorously develop regional agricultural irrigation technologies and optimize agricultural irrigation strategies. The drip irrigation method will improve CP levels and save water resources (Wu, 2013). Water resources can be planned and managed to avoid waste through rainwater collection, wastewater recycling and freshwater protection. According to the characteristics of the ecosystem, sloping cropland and other cropland with low CP should be converted into forests and grasslands. In areas with heavy precipitation and strong soil erosion, forests should be considered priority, the areas with low precipitation and weak soil erosion, grasslands should be considered as priority basis. Selecting drought-resistant and water-saving species can also help alleviate water shortages. In addition, continuing to scientifically promote the implementation of water diversion projects is also one of the basic policies that local governments must adhere to, ensuring accurate and precise water diversion, refining water allocation plans, and strengthening precise scheduling from water sources to ecosystems.
Second, the irregular urban expansion should be restricted, and the urban landscape structure should be optimized. Correlativity study showed that rapid urbanization expansion and high-intensity land development are the main reasons for the ESs decline in the Yangtze River Delta urban agglomeration (Lu et al., 2024). Some scholars have compared the response of ESs in Shenzhen and Hong Kong to rural-urban transitions. And a persistent downward trend in the ESs was observed for both cities, although the decline in Shenzhen was much stronger than in Hong Kong. This was because as an island city, Hong Kong’s urban expansion was much slower compared to Shenzhen’s extremely rapid urbanization due to limitations in land availability (Xu et al., 2020). Therefore the results of ESs assessments should be incorporated into urban landscape planning. Adopting a green, low-carbon, and resource-saving urban development model. For example, when planning urban construction, attention should be paid to reducing the occupation of ecological land such as forests and grasslands, rationally constructing urban green spaces, integrating transportation networks, water networks, and ecological networks (Fu et al., 2022), configuring green infrastructure such as sponge cities to increase urban infiltration and reduce runoff (Yang et al., 2024), so as to improve WY levels, reduce land use intensity and ecological risks in urbanized areas. For urban parks and green spaces, it is necessary to arrange them in a hierarchical manner according to the spatial structure of population concentration, so as to form a complete park system, which can provide cultural services accurately while ensuring the fairness of green spaces, and ultimately promote the improvement of human wellbeing through ESs (Tu et al., 2019).
Third, people should strengthen the protection and restoration of key land types. Protect forests, shrubs, grasslands and wetlands with high regulating and supporting services, and maintain a sustainable supply of ESs by optimizing nature reserve management strategies and implementing key ecosystem protection projects (Ma et al., 2021). At the same time, strengthening the landscape connectivity of key land types, aggregating small plots into larger patches can improve the supply level of ESs. To protect cropland resources, improve cropland quality, county-level people’s governments should strengthen daily supervision of the conversion of cropland into construction land within the county area, and vigorously develop intensive cropland (Xing et al., 2020). When developing ecosystem optimization strategies, the resilience of different ecosystems should be considered (Zhao et al., 2019). For example, forest ecosystems tend to have stronger natural recovery capabilities and ecological resilience, while cropland, barren land, and wetland ecosystems are relatively fragile and less resilient (Liu et al., 2018; Seidl et al., 2014). Conducting refined design in this way will be more conducive to achieving sustainable management of regional ecosystems.
4.5 Limitations and prospects
This study uses InVEST model to evaluate ESs, the quality of evaluation depends on the accuracy of the LULC data classification. However, the accuracy of the Wuhan University CLCD dataset used in this paper is only 79.31%, and no more detailed secondary classification was performed when using it due to the difficulty in obtaining other parameters. In the future research, more detailed LULC classification and higher resolution, more accurate LULC datasets should be used.
In addition, this study only evaluated six key ESs that dominate the Bohai Rim region. Due to the large scope of study area, it cannot fully represent the overall ecological status of the region. In the future, scholars can add ESs that reflect regional characteristics, such as aquaculture and water purification. Moreover, the use of LA to characterize cultural services is usually limited to specific locations (Dou et al., 2022), and it is difficult to accurately reflect their overall situation. In the future, when conducting cultural service assessments, it is necessary to comprehensively select assessment indicators based on research needs and data availability. Finally, although the assessment results have been validated by other studies, the lack of measured data may still lead to certain uncertainties in the results. Future studies can support the assessment results by obtaining observational data from field surveys.
5 Conclusion
This study uses spatial and statistical data to quantify LULC, landscape pattern and ESs in the Bohai Rim region from 2000 to 2020 and analyses their change characteristics and influencing factors. The results show that from 2000 to 2020, the area of forest, water and impervious land increased, while the area of cropland, shrubs, grassland, and barren land decreased. From 2000 to 2015, the landscape in the Bohai Rim region were small and fragmented, complex and dispersed. Subsequently, during 2015–2020, landscape fragmentation weakened, the morphological structure was simplified, and the patches tended to aggregate. From 2000 to 2020, CP, WY and SC capacity show an increasing trend, while CS, HQ and LA capacity show a decline trend. This study suggests that natural factors such as precipitation, temperature and altitude, as well as human factors such as technological progress, policy changes and LULC types are the main factors affecting the changes in landscape pattern and ESs. In the future, ecological managers and policymakers should focus on optimizing regional irrigation strategies and the methods of returning farmland to forest and grassland to reduce the constraints of natural factors on the supply of ESs; adopt methods such as configuring sponge cities, developing urban green spaces to limit the irregular expansion of cities and optimize urban landscape structure; continue to strengthen the protection and restoration of key land types, and protect high-quality forests, grasslands and wetlands to maintain a sustainable supply of ESs. The present study helps us to fully understand the changing characteristics and influencing factors of LULC, landscape pattern and ESs in the Bohai Rim region, and provide a basis for regional ecological restoration, ecological product value realization and territorial spatial planning.
Data availability statement
The original contributions presented in the study are included in the article/Supplementary Material, further inquiries can be directed to the corresponding author.
Author contributions
JL: Conceptualization, Formal Analysis, Investigation, Methodology, Writing–original draft, Writing–review and editing. WC: Project administration, Supervision, Writing–review and editing. HD: Project administration, Supervision, Writing–review and editing. ZL: Data curation, Software, Writing–review and editing. MX: Writing–review and editing. RS: Writing–review and editing. CL: Funding acquisition, Project administration, Supervision, Writing–review and editing.
Funding
The author(s) declare that financial support was received for the research, authorship, and/or publication of this article. This research was funded by the National Key Research and Development Program of China (Grant No. 2022YFF130100202), the National Natural Science Foundation of China (Grant Nos 42293262 and 42271077) and the Science and Technology Innovation Leading Talent Cultivation Project (Climbing Plan) of Tianjin University (Grant No. 2023XPD-0021).
Acknowledgments
We appreciate reviewers and their valuable comments. Also, we thank Editors for the editing and comments. We also thank the Haihe Laboratory of Sustainable Chemical Transformations for financial support.
Conflict of interest
The authors declare that the research was conducted in the absence of any commercial or financial relationships that could be construed as a potential conflict of interest.
Publisher’s note
All claims expressed in this article are solely those of the authors and do not necessarily represent those of their affiliated organizations, or those of the publisher, the editors and the reviewers. Any product that may be evaluated in this article, or claim that may be made by its manufacturer, is not guaranteed or endorsed by the publisher.
Supplementary material
The Supplementary Material for this article can be found online at: https://www.frontiersin.org/articles/10.3389/fenvs.2024.1500045/full#supplementary-material
References
Assesment, M. E. (2005). Ecosystems and human well-being: synthesis. Phys. Teach. 34, 534. Available at: unep.org/resources/report/ecosystem-and-human-well-being-synthesis.
Bai, Y., Wong, C. P., Jiang, B., Hughes, A. C., Wang, M., and Wang, Q. (2018). Developing China's Ecological Redline Policy using ecosystem services assessments for land use planning. Nat. Commun. 9, 3034. doi:10.1038/s41467-018-05306-1
Costanza, R., Arge, , Groot, R. D., Farberk, S., Belt, M. V. D., Hannon, B., et al. (1997). The value of the world's ecosystem services and natural capital. Nature 387, 253–260. doi:10.1038/387253a0
Deng, Y., Jiang, W., Wang, W., Lu, J., and Chen, K. (2018). Urban expansion led to the degradation of habitat quality in the Beijing-Tianjin- Hebei Area. Acta Ecol. Sin. 38, 4516–4525. doi:10.5846/stxb201712062200
Ding, Q. L., Chen, Y., Bu, L. T., and Ye, Y. M. (2021). Multi-Scenario analysis of habitat quality in the Yellow River Delta by coupling FLUS with InVEST model. Int. J. Environ. Res. Public Health. 18, 2389. doi:10.3390/ijerph18052389
Dou, Y. A., Zhen, L., Bakker, M., Yu, X. B., Carsjens, G. J., Liu, J. G., et al. (2022). Investigating the potential impact of ecological restoration strategies on people-landscape interactions through cultural ecosystem services: a case study of Xilin Gol, China. J. Environ. Manage. 316, 115185. doi:10.1016/j.jenvman.2022.115185
Feng, X. R., Zhang, T., Feng, P., and Li, J. Z. (2022). Evaluation and tradeoff-synergy analysis of ecosystem services in Luanhe River Basin. Ecohydrology 15, 12. doi:10.1002/eco.2473
Fisher, B., Turner, R. K., and Morling, P. (2009). Defining and classifying ecosystem services for decision making. Ecol. Econ. 68, 643–653. doi:10.1016/j.ecolecon.2008.09.014
Frank, S., Fürst, C., Koschke, L., Witt, A., and Makeschin, F. (2013). Assessment of landscape aesthetics-Validation of a landscape metrics-based assessment by visual estimation of the scenic beauty. Ecol. Indic. 32, 222–231. doi:10.1016/j.ecolind.2013.03.026
Fu, F., Deng, S. M., Wu, D., Liu, W. W., and Bai, Z. H. (2022). Research on the spatiotemporal evolution of land use landscape pattern in a county area based on CA-Markov model. Sust. Cities Soc. 80, 103760. doi:10.1016/j.scs.2022.103760
Guo, Q., Yu, C. X., Xu, Z. H., Yang, Y., and Wang, X. (2023). Impacts of climate and land-use changes on water yields: similarities and differences among typical watersheds distributed throughout China. J. Hydrol.-Reg. Stud. 45, 101294. doi:10.1016/j.ejrh.2022.101294
Hao, R. F., Yu, D. Y., Liu, Y. P., Liu, Y., Qiao, J. M., Wang, X., et al. (2017). Impacts of changes in climate and landscape pattern on ecosystem services. Sci. Total Environ. 579, 718–728. doi:10.1016/j.scitotenv.2016.11.036
Hu, T., Wu, J. S., and Li, W. F. (2019). Assessing relationships of ecosystem services on multi-scale: a case study of soil erosion control and water yield in the Pearl River Delta. Ecol. Indic. 99, 193–202. doi:10.1016/j.ecolind.2018.11.066
Jiang, B., Bai, Y., Wong, C. P., Xu, X. B., and Alatalo, J. M. (2019). China's ecological civilization program-Implementing ecological redline policy. Land Use Pol. 81, 111–114. doi:10.1016/j.landusepol.2018.10.031
Jiang, B. H., Chen, W., Dai, X. A., Xu, M., Liu, L. F., Sakai, T., et al. (2023). Change of the spatial and temporal pattern of ecological vulnerability: a case study on Cheng-Yu urban agglomeration, Southwest China. Ecol. Indic. 149, 110161. doi:10.1016/j.ecolind.2023.110161
Lai, L., Huang, X. J., Yang, H., Chuai, X. W., Zhang, M., Zhong, T. Y., et al. (2016). Carbon emissions from land-use change and management in China between 1990 and 2010. Sci. Adv. 2, e1601063. doi:10.1126/sciadv.1601063
Langemeyer, J., Calcagni, F., and Baró, F. (2018). Mapping the intangible: using geolocated social media data to examine landscape aesthetics. Land Use Pol. 77, 542–552. doi:10.1016/j.landusepol.2018.05.049
Li, H. L., Peng, J., Liu, Y. X., and Hu, Y. N. (2017). Urbanization impact on landscape patterns in Beijing City, China: a spatial heterogeneity perspective. Ecol. Indic. 82, 50–60. doi:10.1016/j.ecolind.2017.06.032
Li, J., Xia, S., Yu, X., Li, S., Xu, C., Zhao, N., et al. (2020). Evaluation of carbon storage on terrestrial ecosystem in Hebei province based on InVEST model. J. Ecol. Rural. Environ. 36, 854–861. doi:10.19741/j.issn.1673-4831.2019.0918
Li, Q., Li, W. J., Wang, S., and Wang, J. F. (2022a). Assessing heterogeneity of trade-offs/synergies and values among ecosystem services in Beijing-Tianjin-Hebei urban agglomeration. Ecol. Indic. 140, 109026. doi:10.1016/j.ecolind.2022.109026
Li, X., Liu, Z. S., Li, S. J., and Li, Y. X. (2022b). Multi-Scenario simulation analysis of land use impacts on habitat quality in Tianjin based on the PLUS model coupled with the InVEST model. Sustainability 14, 6923. doi:10.3390/su14116923
Liu, C., Li, S., Liu, X., Wang, B., Lang, Y., Ding, H., et al. (2024a). Biogeochemical cycles in the Anthropocene and its significance. Earth Sci. Front. 31, 455–466. doi:10.13745/j.esf.sf.2024.1.26
Liu, C., Men, B., Shen, Y., and Pang, J. (2023). Soil conservation and water purification services and their trade-offs and synergies in Luanhe River Basin. Acta Ecol. Sin. 43, 5740–5752. doi:10.5846/stxb202207101963
Liu, H. X., Gao, C. Y., and Wang, G. P. (2018). Understand the resilience and regime shift of the wetland ecosystem after human disturbances. Sci. Total Environ. 643, 1031–1040. doi:10.1016/j.scitotenv.2018.06.276
Liu, Q., Qiao, J. J., Li, M. J., and Huang, M. J. (2024b). Spatiotemporal heterogeneity of ecosystem service interactions and their drivers at different spatial scales in the Yellow River Basin. Sci. Total Environ. 908, 168486. doi:10.1016/j.scitotenv.2023.168486
Liu, W., Zhan, J. Y., Zhao, F., Wang, C., Zhang, F., Teng, Y. M., et al. (2022a). Spatio-temporal variations of ecosystem services and their drivers in the Pearl River Delta, China. J. Clean. Prod. 337, 130466. doi:10.1016/j.jclepro.2022.130466
Liu, Y. B., Han, M., Wang, M., Fan, C., and Zhao, H. (2022b). Habitat quality assessment in the Yellow River Delta based on remote sensing and scenario analysis for land use/land cover. Sustainability 14, 15904. doi:10.3390/su142315904
Liu, Y. B., Hou, X. Y., Li, X. W., Song, B. Y., and Wang, C. (2020). Assessing and predicting changes in ecosystem service values based on land use/cover change in the Bohai Rim coastal zone. Ecol. Indic. 111, 106004. doi:10.1016/j.ecolind.2019.106004
Lu, Y. G., Wang, J. C., and Jiang, X. K. (2024). Spatial and temporal changes of ecosystem service value and its influencing mechanism in the Yangtze River Delta urban agglomeration. Sci. Rep. 14, 19476. doi:10.1038/s41598-024-70248-2
Ma, J., Li, J. W., Wu, W. B., and Liu, J. J. (2023). Global forest fragmentation change from 2000 to 2020. Nat. Commun. 14, 3752. doi:10.1038/s41467-023-39221-x
Ma, S., Qiao, Y. P., Wang, L. J., and Zhang, J. C. (2021). Terrain gradient variations in ecosystem services of different vegetation types in mountainous regions: vegetation resource conservation and sustainable development. For. Ecol. Manage. 482, 118856. doi:10.1016/j.foreco.2020.118856
Ning, S. A., Hai, Y. Y., and Nan, J. (2011). Response of ecosystem service value to land use change in coastal zone of Bohai Sea in last 30 years. Adv. Mat. Res., 347–353. doi:10.4028/www.scientifc.net/AMR.347-353.3963
Ouyang, Z., Zheng, H., Xiao, Y., Polasky, S., Liu, J., Xu, W., et al. (2016). Improvements in ecosystem services from investments in natural capital. Science 352, 1455–1459. doi:10.1126/science.aaf2295
Searchinger, T. D., Wirsenius, S., Beringer, T., and Dumas, P. (2018). Assessing the efficiency of changes in land use for mitigating climate change. Nature 564, 249–253. doi:10.1038/s41586-018-0757-z
Seidl, R., Rammer, W., and Spies, T. A. (2014). Disturbance legacies increase the resilience of forest ecosystem structure, composition, and functioning. Ecol. Appl. 24, 2063–2077. doi:10.1890/14-0255.1
Shao, Y. J., Liu, Y. S., Li, Y. H., and Yuan, X. F. (2023). Regional ecosystem services relationships and their potential driving factors in the Yellow River Basin, China. J. Geogr. Sci. 33, 863–884. doi:10.1007/s11442-023-2110-1
Teutschbein, C., Grabs, T., Laudon, H., Karlsen, R. H., and Bishop, K. (2018). Simulating streamflow in ungauged basins under a changing climate: the importance of landscape characteristics. J. Hydrol. 561, 160–178. doi:10.1016/j.jhydrol.2018.03.060
Tu, X., Huang, G., and Wu, J. (2019). Review of the relationship between urban greenspace accessibility and human well-being. Acta Ecol. Sin. 39, 421–431. doi:10.5846/stxb201802030294
van der Plas, F., Allan, E., Fischer, M., Alt, F., Arndt, H., Binkenstein, J., et al. (2019). Towards the development of general rules describing landscape heterogeneity-multifunctionality relationships. J. Appl. Ecol. 56, 168–179. doi:10.1111/1365-2664.13260
Wang, H. R., Zhang, M. D., Wang, C. Y., Wang, K. Y., Wang, C., Li, Y., et al. (2022). Spatial and temporal changes of landscape patterns and their effects on ecosystem services in the huaihe River Basin, China. Land 11, 513. doi:10.3390/land11040513
Wang, X. X., Xiao, X. M., Xu, X., Zou, Z. H., Chen, B. Q., Qin, Y. W., et al. (2021). Rebound in China's coastal wetlands following conservation and restoration. Nat. Sustain. 4, 1076–1083. doi:10.1038/s41893-021-00793-5
Winkler, K., Fuchs, R., Rounsevell, M., and Herold, M. (2021). Global land use changes are four times greater than previously estimated. Nat. Commun. 12, 2501. doi:10.1038/s41467-021-22702-2
Wischmeier, W. H., Johnson, C. B., and Cross, B. V. (1971). A soil erodibility nomograph for farmland and construction sites. J. Soil Water Conserv. 26, 189–193. Available at: researchgate.net/publication/303183461_A_soil_erodibility_nomograph_for_farmland_and_construction_sites/citations.
Wu, J. G. (2013). Landscape sustainability science: ecosystem services and human well-being in changing landscapes. Landsc. Ecol. 28, 999–1023. doi:10.1007/s10980-013-9894-9
Xia, C., Guo, H., Zhao, J., Xue, F., Wang, C., Zhou, J., et al. (2023a). Dynamic responses of ecosystem services to urbanization at multi-spatial scales in the Beijing-Tianjin-Hebei region. Acta Ecol. Sin. 43, 2756–2769. doi:10.5846/stxb202204181049
Xia, H., Yuan, S. F., and Prishchepov, A. V. (2023b). Spatial-temporal heterogeneity of ecosystem service interactions and their social-ecological drivers: implications for spatial planning and management. Resour. Conserv. Recycl. 189, 106767. doi:10.1016/j.resconrec.2022.106767
Xing, L., Hu, M. S., and Wang, Y. (2020). Integrating ecosystem services value and uncertainty into regional ecological risk assessment: a case study of Hubei Province, Central China. Sci. Total Environ. 740, 140126. doi:10.1016/j.scitotenv.2020.140126
Xu, C., Jiang, W. Y., Huang, Q. Y., and Wang, Y. T. (2020). Ecosystem services response to rural-urban transitions in coastal and island cities: a comparison between Shenzhen and Hong Kong, China. J. Clean. Prod. 260, 121033. doi:10.1016/j.jclepro.2020.121033
Yang, K., Han, Q., and Vries, B. d. (2024). Urbanization effects on the food-water-energy nexus within ecosystem services: a case study of the Beijing-Tianjin-Hebei urban agglomeration in China. Ecol. Indic. 160, 111845. doi:10.1016/j.ecolind.2024.111845
Zhang, T. J., Zhang, S. P., Cao, Q., Wang, H. Y., and Li, Y. L. (2022). The spatiotemporal dynamics of ecosystem services bundles and the social-economic-ecological drivers in the Yellow River Delta region. Ecol. Indic. 135, 108573. doi:10.1016/j.ecolind.2022.108573
Zhang, W., Chang, W. J., Zhu, Z. C., and Hui, Z. (2020). Landscape ecological risk assessment of Chinese coastal cities based on land use change. Appl. Geogr. 117, 102174. doi:10.1016/j.apgeog.2020.102174
Zhang, W., and Fu, J. (2003). Rainfall erosivity estimation under different rainfall amount. Resour. Sci. 25, 35–41. resci.cn/CN/Y2003/V25/I1/35
Zhao, W. Z., Han, Z. L., Yan, X. L., and Zhong, J. Q. (2019). Land use management based on multi-scenario allocation and trade-offs of ecosystem services in Wafangdian County, Liaoning Province, China. PeerJ 7, e7673. doi:10.7717/peerj.7673
Zhao, X., Zhang, Q., He, G. Z., Zhang, L., and Lu, Y. L. (2021). Delineating pollution threat intensity from onshore industries to coastal wetlands in the Bohai Rim, the Yangtze River Delta, and the Pearl River Delta, China. J. Clean. Prod. 320, 128880. doi:10.1016/j.jclepro.2021.128880
Zhu, L. Y., Song, R. X., Sun, S., Li, Y., and Hu, K. (2022). Land use/land cover change and its impact on ecosystem carbon storage in coastal areas of China from 1980 to 2050. Ecol. Indic. 142, 109178. doi:10.1016/j.ecolind.2022.109178
Keywords: land use/land cover, landscape pattern, ecosystem service, Bohai Rim region, InVEST model
Citation: Liu J, Chen W, Ding H, Liu Z, Xu M, Singh RP and Liu C (2024) Changing characteristics of land cover, landscape pattern and ecosystem services in the Bohai Rim region of China. Front. Environ. Sci. 12:1500045. doi: 10.3389/fenvs.2024.1500045
Received: 22 September 2024; Accepted: 01 November 2024;
Published: 19 November 2024.
Edited by:
Salvador García-Ayllón Veintimilla, Polytechnic University of Cartagena, SpainReviewed by:
Cai Yongli, Shanghai Jiao Tong University, ChinaChen Wanxu, China University of Geosciences Wuhan, China
Copyright © 2024 Liu, Chen, Ding, Liu, Xu, Singh and Liu. This is an open-access article distributed under the terms of the Creative Commons Attribution License (CC BY). The use, distribution or reproduction in other forums is permitted, provided the original author(s) and the copyright owner(s) are credited and that the original publication in this journal is cited, in accordance with accepted academic practice. No use, distribution or reproduction is permitted which does not comply with these terms.
*Correspondence: Wei Chen, chenwei19@tju.edu.cn