- Department of Biochemistry and Microbiology, Faculty of Sciences, Engineering and Agriculture, University of Venda, Thohoyandou, South Africa
Introduction: Vibrio cholera is increasingly emerging as a significant public health concern in developing countries. Choleragenic Vibrio cholerae O1 and O139 has reported to cause devastating disease and economic burdens in developing countries. In rural areas of the Vhembe district, most rivers and several communal boreholes are polluted as a result of sanitation issues around these water sources. The aim of this study was to determine the presence of choleragenic V. cholerae O1 and O139 in rivers and communal boreholes.
Methods: The analysis of physicochemical parameters and molecular techniques was used to establish the adaptation and detect the serogroups of V. cholerae in the water samples.
Results and Discussion: The results reported that electrical conductivities (EC) ranged between 18.78 and 154 μS/cm, with rivers such as Madandze and Mvudi recording >80 μS/cm, and those of the communal boreholes were ranged between 23.4 and 295 μS/cm, which were above the acceptable South African water quality guidelines of 0–70 μS/cm for rivers and communal boreholes. The results further revealed that most of the rivers detected positive for V. cholerae, except for the Mukhase river; the downstream points of Livuvhu and Nwedi rivers and the upstream point of the Nzhelele river; and several of the communal boreholes (Mak B1, B2, Kwe B3, 4, and 6) also tested positive for the presence of V. cholerae. The toxigenic Vibrios was also reported in Mutshundudi, Tshinane rivers, the upstream of Dzindi, Madanzhe, Nwedi, and the downstream of Sambandou rivers, as well as Mak B1, B2, and Kwe3 of communal boreholes. The serogroup O1 was detected on the Mutshundudi and Tshinane rivers, while serogroup O139 was detected upstream of the Dzindi, Madanzhe, Mutshundudi, and Tshinane rivers. There was also detection of the O1 serogroup in the communal boreholes (Mak B1 and Kwe B3), while O139 was only detected in one communal borehole (Mak B2). The development of robust policies, including an integrated water and sanitation safety surveillance web tool for monitoring water resources and public health protection, is required to make sure that drinking water in rural communities is safe for consumption.
1 Introduction
Access to safe, clean water and sanitation is a core human right, and it will also drive the achievement of sustainable development goals (SDGs), particularly Agendas 3 and 6 (Sikder et al., 2023; United Nations, 2024). According to the World Health Organisation (WHO), the common definition of safe drinking water and improved sanitation is access to piped water, protected wells, springs, rainwater, packaged water, boreholes, and sanitation facilities capable of effectively managing human excreta and urine (Bain et al., 2018).
The bacteria that cause cholera disease is known as V. cholerae, and it’s transmitted either by food or water that is contaminated by this microorganism (Harris et al., 2012). V. cholerae has continued to ravage millions across the globe, especially in underdeveloped and developing countries such as South Africa and other Sub-Saharan, Asian, and Caribbean countries. This is because they have lagged in terms of inadequate access to safe, clean water and inadequate sanitation facilities, while these have been reduced or eradicated in developed countries (USA, United Kingdom, Canada, among other countries) with the development of an adequate safe water and sanitation infrastructure (Ali et al., 2015). Ozochi et al. (2024) further opined that the resent cholera cases around the world suggested that the outbreaks are due to ctx-positive V. cholerae O1. Currently, most of the pandemics or cases are not known, even with the World Health Organisation (WHO), which maintains a repository of cases or mortality through their weekly database, and they record less than 10% of cases due to inadequate reporting, especially in poor countries (WHO, 2014). According to Masuet et al. (2011) and Griffith et al. (2006), inadequate reporting of V. cholerae across the globe is a result of economic challenges, lack of political and social goodwill, inadequate capacity of both laboratories and epidemiological surveillance systems, and poor patient records. The outbreaks also arise from floods, conflicts, or extreme weather conditions that have triggered faecal contamination of water sources (Almagro-Moreno and Taylor, 2013).
There are approximately 200 serogroups of Vibrio cholera that are known, of which O1 and O139 are known for pathogenic symptoms and serious diarrhoea in humans (Almagro-Moreno and Taylor, 2013; Sharma et al., 2021). According to WHO (2005), choleragenic V. cholerae O1 and O139 are the only causative agents of cholera, a waterborne and foodborne disease with epidemic and pandemic potential. The most significant symptoms are watery diarrhoea, discomfort of the abdomen, and anorexia, which cause mild to severe gastrointestinal illness, dehydration, and death (WHO, 2005). The two serogroups are known for producing cholera toxins (CT) and toxin-coregulated pilus (TCP). There are also two non-O1 and non-O139 serogroups that are also associated with mild gastroenteritis and bloody diarrhoea to some extent and do not cause cholera (Yadava, Jain, and Goel, 2013; Bhandari, 2023). The two toxins (CT and TCP) produced by choleragenic serogroups are major triggers of watery diarrhoea and colonisation factors in humans (Arteaga et al., 2020).
Kaper, Morris, and Levine (1995) further stated that the O1 serogroup is further divided into three serotypes, mainly Inaba, Ogawa, and Hikojima, which are further divided into two biotypes, which are classical and El Tor. According to Marin et al. (2013), the O1 serogroup is widespread across the globe, while O139 is only restricted to the Asian continent. Mondiale de la Santé and the World Health Organisation (WHO) (2017) also reported that El Tor survives longer in environments that are linked to mild cases, which are shredded in high numbers in the faeces.
Most of the rivers and communal boreholes in rural areas such as the Vhembe district of South Africa and other developing countries are the immediate sources of water for domestic, agricultural, and other activities due to inadequate access to clean water and sanitation, which are not or are partially treated by dysfunctional treatment facilities (Potgieter et al., 2020). The irony is that most of the surface and ground water sources are highly polluted, triggering contamination and cases of V. cholerae in these areas. Potgieter et al. (2020) further stated that to reverse pollution challenges, there is a need for effective, robust, and continuous surveillance of bacterial, microbiological, and physiochemical parameters to establish any possible risks that are associated with pollution of a given water source for the rural populace, leading to V. cholerae cases or epidemics. Given the extent of potential pathogenic cases of the O1 and O139 serogroups in rural areas of Vhembe district, there is a need for continuous surveillance, rapid detection, and identification because the devastation can be catastrophic, as reported by Keddy et al. (2013). This will not only revert the community’s ill-health issues but also assist in developing mitigation measures in terms of an integrated water sanitation and hygiene system (Keddy et al., 2013; Tasnia, 2023). Therefore, the main aim of this study was to establish the presence of choleragenic serogroups O1 and O139 in rivers and communal boreholes in the Vhembe district of Limpopo Province.
2 Methods and materials
2.1 Study area description
This District Municipality of Vhembe (Figure 1) is found in the province of Limpopo, further north of South Africa, and occupies a landmass of approximately twenty thousand square kilometres, according to Vhembe (2013). This area is blessed with fertile soil, and agricultural activities are taking place across the region. According to Kachienga et al. (2024), this area has both seasonal and permanent rivers. There are a few existing communal boreholes that are still found in some villages that lack access to municipal water supply. Permanent rivers (n = 11) and communal boreholes (n = 12) that were sampled for this study are indicated by the red dots in Figure 1.
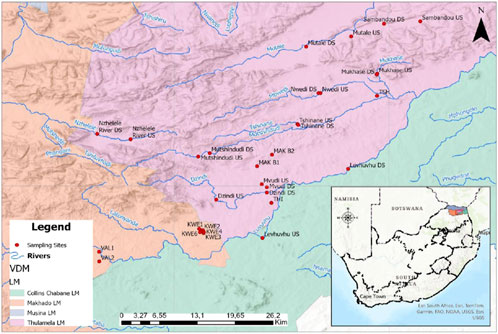
Figure 1. Map showing names of the rivers and communal boreholes (red dots) that were sampled in Vhembe District (developed by the department of environmental science, University of Venda).
2.2 Collection of water samples
Water samples were collected between the months of April and July 2024 in 2-L sterile plastic bottles using aseptic techniques from rivers at specific sites as described by Kachienga et al. (2024) and communal boreholes. The collection was done once off, and the samples were in duplicates from both river (down and downstream) and communal boreholes. The samples were transported to the laboratory at the University of Venda in a cooler box containing ice and analysed upon arrival (Rice et al., 2012).
2.3 Physicochemical analysis of river and communal borehole samples
Various parameters, such as total dissolved solids (TDS), temperature, electrical conductivity (EC), and pH, were determined on site using a pH probe (Hach Intellical™ PHC101). The dissolved oxygen (DO) was analysed using a DO probe (Hach LDO® Model 2). In addition, a Lovibond TB 211 turbidimeter (Lovibond. IR, Germany) was used to analyse turbidity on site.
2.4 Culturing/enrichment of isolated Vibrio cholerae colonies
The filtration of the samples was done according to the protocol of the National Center for Infectious Diseases, 1994; Momtaz et al. (2013), where a 47 mm cellulose acetate filter with a 0.45 μm pore size was used to filter the samples. The enrichment of filtrate that was trapped in the filter paper after sample filtration was done in 100 mL of sterile alkaline peptone water (NutriSelect® Plus, Sigma Aldrich, SA); thereafter, the incubation was done at 37°C for one full day.
The enriched samples (10 μL) were transferred into the sterile thiosulphate citrate bile salt (TCBS) (Mass Group Ltd. Reinfeld, Germany) agar plates and then incubated for 24 h at 37°C. The analysis was done in triplicate for each sample. The presumptive positive V. cholerae and their colonies forming units were determined according to Kachienga et al. (2024). The preservation of the presumptive Vibrio colonies was done according to Huq et al. (2012), and we further tested using biochemical tests for the confirmation of presumptive positive V. cholerae. The presumptive Vibrio colonies obtained were further used to extract the genomic DNA, which was used for molecular analysis for confirmation of toxigenic and serogroups of choleragenic V. cholerae.
2.5 API 20E technique (biochemical test) for analysing of the isolates
The presumptive colonies were tested with the API 20E system, and the interpretation of the isolates was based on the API 20E analytical profile index (Version 5, Biomerieux Industries, France). This technique was used to identify the presumptive positive V. cholerae obtained from other closely related Vibrionaceae species that might be present.
2.6 DNA extraction and PCR conditions
DNA extraction from samples was carried out using the ZymoBIOMICSTM DNA Miniprep Kit (Zymo Research, CA, USA) per the protocol of the manufacturer from the purified preserved presumptive Vibrio colonies. The NanoDropTM 2000 spectrophotometer (Thermo Scientific, Johannesburg, South Africa) was used to quantify the extracted DNA. The PCR reactions were performed in a total volume of 25 μL, as previously reported (Kachienga et al., 2024). The PCR technique was necessary to confirm the presence of V. cholerae, toxigenic V. cholerae, and serogroups of choleragenic V. cholerae in the samples using specific primers reported in Table 1. Amplification reactions were done in the following order: heat denaturation at 94°C for 2 min, followed by 35 cycles of heat denaturation at 94°C for 1 min, annealing at 58°C for 1 min, and DNA extension at 72°C for 1 min (Momtaz et al., 2013) using a qPCR Eppendorf Master Cycler 5330 (Eppendorf-Nethel-Hinz GmbH, Hamburg, Germany). The specific primers used included OmpW, ctxA, and O1rfb/O139rfb for detecting V. cholerae, toxigenic V. cholerae, and serogroups of choleragenic V. cholerae (Nandi et al., 2000; Huang et al., 2009; Mehrabadi et al., 2012) (Table 1).
2.7 Statistical analysis
The correlation of physiochemical parameters based on different sampling sites (upstream and downstream rivers, communal boreholes) through principal component analysis (PCoA) was done using ClustVis (Metsalu and Vilo, 2015). The clustring together of these parameters visa vis their sampling sites based on the geographical areas and the surrounding activities.
3 Results and discussion
3.1 Activities around the rivers of study
The activities observed around these water sampling points were agricultural (subsistence and commercial farming), brickmaking, laundry, car washing, and open defecation by community members, amongst others. Various activities taking place around the water sources and occasional flooding trigger the presence of pathogenic microbes through runoff into the water sources. Yeung and Thorsen (2016) further reported that most of the urinal infections are also spread during recreational activities in and around rivers and communal boreholes.
3.2 Physicochemical parameters of the rivers
Polluted water is associated with a heavy burden on the population’s overall health, and this has negatively impacted the life expectancy in developing countries such as South Africa (WHO, 2002). Cases of V. cholera have become a burden in poorer communities, especially in sub-Saharan countries where there is inadequate safe, clean water and sanitation infrastructure (Khouadja et al., 2014). According to Huq et al. (2012), assessment of vital parameters (temperature, turbidity, TDS, pH, DO, and EC) is necessary to establish the survival and adaptation of pathogenic and serogroups O1 and 139 and non-O1/O139. The results of the above parameters are captured in Table 2.
The pH counts of the rivers ranged from 7.03 to 8.21 for rivers and 6.88 to 8.21 for communal boreholes. They were both within the acceptable South African water quality guidelines of 6.5–8.5 for aquatic environments (rivers) and 6.0–9.0 for domestic usage (communal bore holes) (Department of Water Affairs and Forestry, 1996a). A pH between 7.5 and 9.0 normally influences the survival or adaptability of microorganisms; moreover, a pH > 8.5 is optimal for their survival in water (Grandjean et al., 2005). V. cholerae, especially serogroup O1, is extremely sensitive to acidic environments, and normachlorohydric people are not easily susceptible to cholera. This type of vibrio is also sensitive to desiccation and heat (ICMSF, 1996).
The average electrical conductivities (EC) for rivers and communal boreholes ranged between 18.78 and 154 μS/cm and 23.4 and 295 μS/cm (Table 2), which were above the acceptable South African water quality guidelines of 0–70 μS/cm for rivers and domestic use (Department of Water Affairs and Forestry, 1996a). The higher conductivity value recorded is directly proportional to an increase in ions and organic compounds that can conduct electric current (Holmes, 1996; Ferreira, 2011). Atekwana et al. (2004) further reported that the high levels of conductivity and TDS in water and the environment are due to the biodegradation of organic compounds by natural microorganisms. The presence of chitinase that is produced by V. cholera also assists them in adapting and surviving in extreme conditions such as saline and acidic environments, as reported by Huq et al. (2012).
The overall range for the TDS for the rivers in this study ranged between 10 and 90 mg/L, while for communal boreholes, it ranged between 70 and 320 mg/L. These data revealed that both rivers and communal boreholes were still within the acceptable South African water quality guidelines of 0–450 mg/L (Department of Water Affairs and Forestry, 1996a; Department of Water Affairs and Forestry, 1996b).
The average temperature obtained ranged between 18.05°C and 30.60°C for both rivers and communal boreholes. According to Franco et al. (1997), temperature is vital in triggering the formation of environmental biofilms and sediments that are supporting the survival of V. cholera, which will increase their counts and human illness. Huq et al. (2012) also state that a wider range of temperatures is critical for the heterogeneous nature of V. cholera. Temperature and other parameters, such as salinity, are the main drivers of the adaptability and survival of V. cholerae in any given environment, including contaminated water (Takahashi et al., 2023).
Most of the rivers ranged between 1.01 and 14.85 NTU, while the turbidity of communal boreholes ranged between 0.71 and 13.23 NTU. Despite all, most of the rivers were found to be within the acceptable set standards limit of <25 NTU for river water containing sediments and untreated aquatic water (Department of Water Affairs and Forestry, 1996a). However, only some communal boreholes (Val B1, Kwe B1, B3, and B4) were reported to be within the acceptable South African water quality guidelines of 0–1 NTU for domestic use (Department of Water Affairs and Forestry, 1996b). Turbidity is influenced by the formation of biofilms, sediments, and eutrophication by the presence of nitrates and phosphates because of the agricultural, bathing, laundry, and brick-making activities inside and along the water sources (Fong et al., 2010). Ntema et al. (2014) also stated that the presence of debris and biotic surfaces in the rivers also facilitates V. cholera attachment and mobility.
Based on the availability of DO, the study revealed that most of the rivers were above the acceptable South African water quality guidelines of 6.5–8.0 ppm, except for Madanzhe River, whose DO counts were below the acceptable water quality guidelines. This is driven by the high-water runoff, higher flow rates of waters within these rivers, and pollution of rivers.
The principal component analysis (PCoA) correlation of different physicochemical parameters also revealed that most of the rivers and boreholes are clustered based on the geographical areas and the surrounding activities. The rivers such as Mvudi, Livuvhu, Tshinane, and Nwedi have clustered together based on PCoA in terms of high levels of variance in physicochemical parameters because farming and laundry activities around these water catchments, while Madandze, Mutshundudi, Nzhelele, and Mukhase rivers were also clustering together due to anthropogenic activities such as brickmaking and farming around them (Figure 2). These activities play a role in the physicochemical parameters obtained from the samples from these river sources.
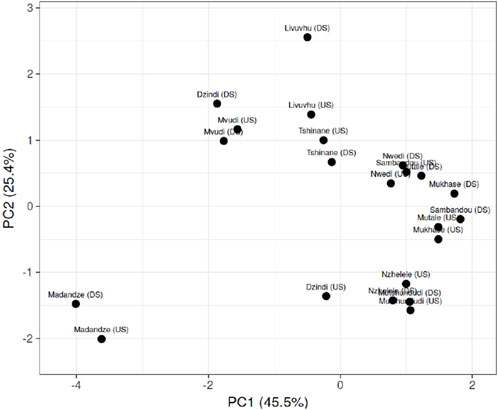
Figure 2. PCoA showing the correlation of physiochemical parameters on the difference between upstream and downstream rivers sampled.
Figure 3 reported that the Mak B1, B2, and Val B1 boreholes were clustered due to high levels of variance in physicochemical parameters. This was also due to the similarity of geographical location and poor sanitation around these boreholes that influenced their physicochemical parameters. The Kwe B3, Kwe B4, Thi B1, and Tsh B1 boreholes highly clustered together, while the Kwe B2, B5, and B6 boreholes least clustered together based on the variance of parameters. Most of these boreholes that were highly clustered were also influenced by agricultural activities and salty water that was associated with them. The boreholes that were least clustered, such as Kwe B2, B5, and B6, were difficult to identify because they were from similar geographical settings yet they least clustered.
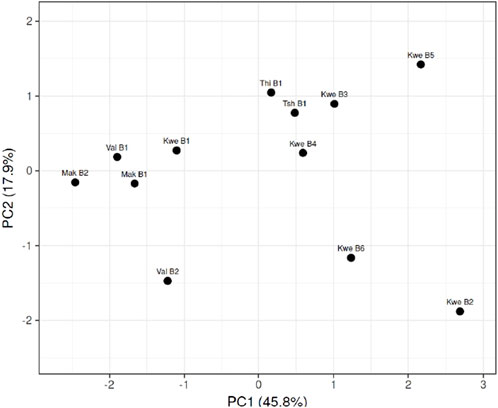
Figure 3. PCoA showing the correlation of physiochemical parameters on different communal boreholes sampled.
3.3 The biochemical test results using analytical profile index (API)
The API test is one of the biochemical tests that is used to identify various families of microorganisms, such as Enterobacteriaceae and V. cholerae. Most of these rivers reported presumptive Vibrio flavialis, Erwina spp., Raoutella planticolla or terrigena, Aeromonas spp., Klebsiella spp., and Serrati spp., except for Madanzhe, Sambandou, and Nzhelele, which also had presumptive V. cholerae after being identified on the downstream site by the API test (Biomerieux Industries, South Africa) (Table 3). There were no microorganisms identified in the Makhase River. In communal boreholes, most of them reported presumptive V. flavialis and/or Aeromonas spp., Erwina spp., and Raoutella planticolla or terrigena, and communal boreholes such as Mak B1, B2 and Val B1, B2 reported none of the microorganisms (Table 3). These Erwina spp. bacteria are known to be well adapted to growth on vegetables because they are capable of fermenting many of the sugars and alcohols that exist in certain vegetables, unlike other common bacteria (Warriner et al., 2003; Gustaw et al., 2021). They might find their way into rivers and boreholes because of runoff from the surrounding farms or backyards from floods or rainfall. Raoutella planticolla or terrigena, Aeromonas spp., Klebsiella spp., and Serrati spp. are some of the microorganisms that are Gram-positive and sugar fermenters that lead to yellow colonies (Abbott, 2011; Yeung and Thorsen, 2016). They also cause urinary tract infections.
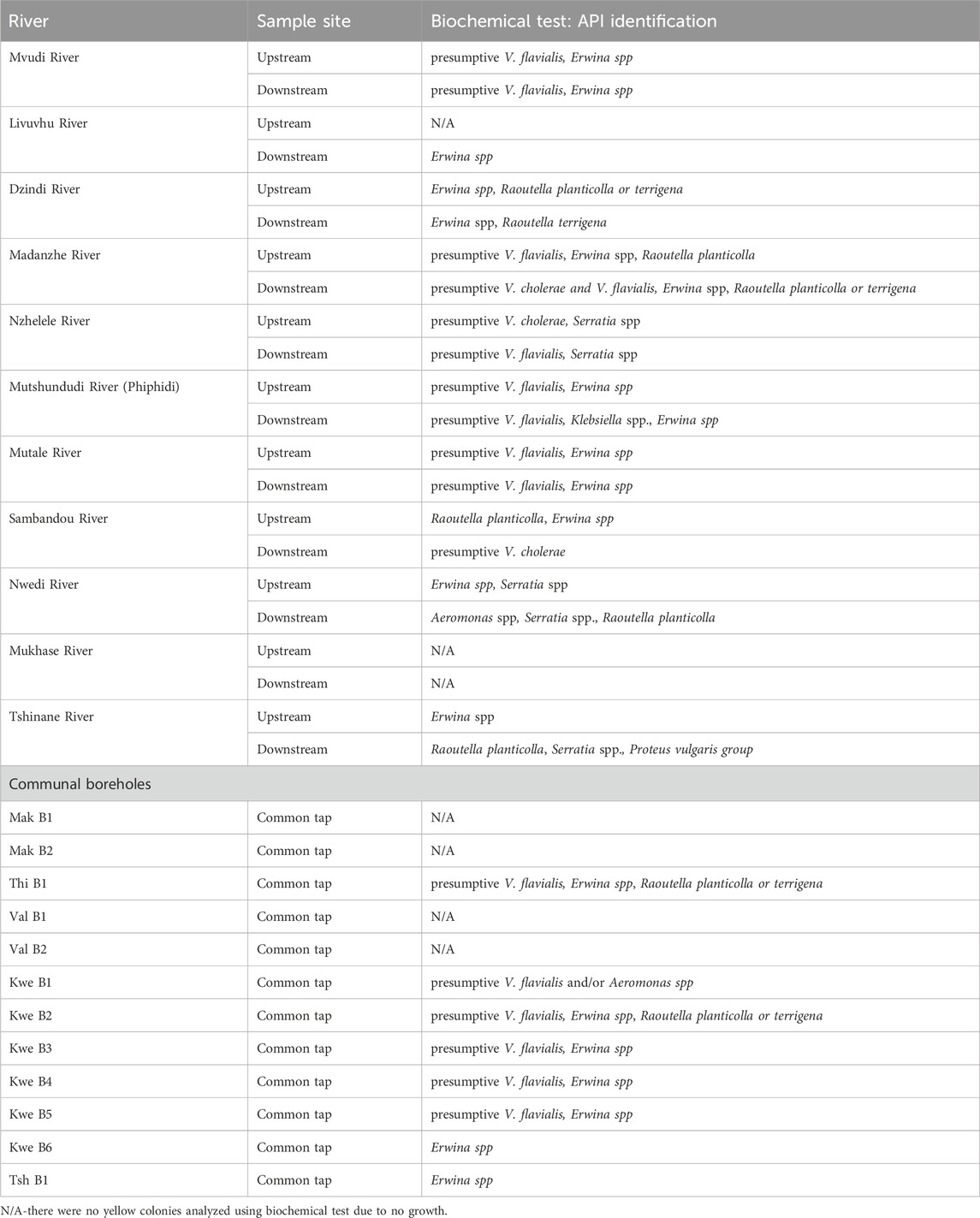
Table 3. The biochemical test: API results for the identification of Vibrio cholerae in rivers and communal borehole samples.
3.4 Molecular detection of serogroups of the toxigenic V. Cholera O1 and O139 from various rivers and communal boreholes using qPCR analysis
Most of the rivers detected positive for V. cholerae using the OmpW primer, except for the Mukhase river, the downstream Livuvhu and Nwedi rivers, and the upstream Nzhelele river point (Table 4). Only Mak B1, B2, Kwe B3, 4 and 6 boreholes tested positive for V. cholerae, using the OmpW primer (Table 4), which specifically targets the outer membrane of V. cholerae, and according to Fu et al. (2018), this gene acts as a passage of iron and other organic molecules that are pumped across and regulate survival in extreme environments. The detection of V. cholerae was also directly connected to the higher physicochemical parameters such as EC, TDS, and turbidity that were reported in both river and communal boreholes. The higher values obtained trigger the survival and distribution of V. cholerae in these water sources.
From the rivers that tested positive for V. cholerae, the pathogenicity of these Vibrio isolates was assessed using the ctxA primer and Mutshundudi, Tshinane rivers, the upstream of Dzindi, Madanzhe, and Nwedi samples, and the downstream of Sambandou rivers reported positive for toxigenic V. cholerae (Table 4). Only Mak B1, B2, and Kwe 3 communal boreholes were found to have toxigenic V. cholerae (Table 4). Bina et al. (2003) reported that toxigenic V. cholerae produces a toxin-regulated pilus that facilitates colonisation of the intestine walls and is the main regulator of the ctxA gene, and this gene was targeted by the ctxA primer. According to Liang et al. (2007), colonisation results in the activation of the G protein, which stimulates the adenylate cyclase. This process leads to the loss of electrolytes from the cell membrane, resulting in vomiting and watery, loose stools into the large intestines (World Health Organization, 2017). It is imperative to understand that any mutation or mismatch in the ctxA gene sequence leads to either a positive ctxA or a non-toxin-producing strain (Hoshino et al., 1998; Takahashi et al., 2023). The presence of toxigenic V. cholerae in these water sources was driven by the conditions and activities around them, as shown in S1-3, where some of the water sources were closer to sewer lines, toilets, and backyard farming. For the contamination or pollution of communal boreholes, Barcelona et al. (1988) stipulated different routes such as infiltration, direct migration, inter-aquifer exchange, and recharge from surface water that are the major drivers. In the clinical context, pathogenic V. cholerae differs from the non-pathogenic ones based on toxin-co-regulated pilus (TCP) and cholera toxin (CTX), which allow colonisation of the gut encoded by the phage CTXφ, which uses the TCP to attach to the bacterium (Waldor and Mekalanos, 1996; Ja’afar et al., 2021). Toxigenic V. cholerae also utilises the commensal association, leading to the formation of biofilm with copepods on their chitinous surfaces, which further leads to the formation of algal (de Magny et al., 2011; Rita and Colwell, 2009).
Once the pathogenicity had been analysed using PCR, the serogroups of the detected toxigenic were determined using the O1 rfb and O139 rfb primers. Serogroup O1 was detected on the Mutshundudi and Tshinane rivers, while serogroup O139 was detected on the upstream of the Dzindi, Madanzhe, Mutshundudi, and Tshinane rivers (Table 4). There was detection of both the O1 and O139 serogroups in the communal boreholes Mak B1 and Kwe B3, while serogroup O139 was only detected in the communal borehole Mak B2 (Table 4). In a study done by Phetla (2021), there was a higher percentage of serogroup O1 from sewage sludge and the riverbed from the Limpopo region, and therefore, there was a potential for O1 circulation in water sources leading to sporadic cholera outbreaks. The study of Phetla (2021) also reported that the O139 V. cholerae serotype was not found, and it was similar to a study done by Ansaruzzaman et al. (2007), who reported that this serotype was not endemic to the African continent but more endemic to India, Bangladesh, and other parts of the Asian continent. However, in this study, serogroup O139 was identified in both rivers and communal boreholes. This might be due to cross-border migration from cholera-prone countries/areas into South Africa through seeking medical treatment, tourism, traders, relatives, and refugees from neighbouring cholera-endemic countries or communities with ongoing epidemics like Haiti, Malawi, or Mozambique and Asian countries (Chin et al., 2011; Zarocostas, 2017). The serogroups detected from rivers and communal boreholes in the study were also due to the discharge of raw sewage, especially on the upstream side of the Madanzhe River, and the discharge of partially or untreated wastewater effluents into the rivers. The communal boreholes were also closer to the septic pit, leaking sewer line, and latrine, as depicted in S1-3.
Keddy et al. (2013) and Alaoui et al. (2021) stated that non-O1 or non-O139 strains of V. cholerae have been identified in birds, which might act as carriers/hosts into the environment. Therefore, continuous surveillance of rivers and communal boreholes, an integrated approach, and robust policies are needed to monitor these cases in a timely and reliable manner to prevent further discharge of raw sewage into rivers and closer to communal boreholes.
S1-3 depict images of rivers (upstream and downstream) and communal boreholes where serogroups (O1 and O139) were discovered. The majority of the rivers were contaminated, and the communal boreholes were closer to the pit latrine while the sewer line also discharged dirty effluent directly into the river catchment.
3.5 Limitation of this study
The study involved analysing water samples from rivers and communal boreholes, which could also be reservoirs for other organisms such as fish, snails, and others that are involved in commensal association with V. cholerae, including feeding, but were not considered in this study (You et al., 2019; Cho et al., 2021). Further studies using appropriate techniques targeting ecosystems that were not covered by this study should be conducted to provide more informed and reliable information on the presence of choleragenic V. cholerae in both surface water sources and communal boreholes. This study was conducted for a period of 4 months only, and a longer study might be needed to establish the prevalence and distribution of V. cholerae O1 or O139 and the potential health risk to vulnerable communities in Vhembe district, which is predominantly in rural areas.
4 Conclusion
The findings of this investigation indicate that pathogenic serotypes (O1 and O139) of V. cholerae were found in rivers and several communal boreholes. The presence of all V. cholerae serotypes in these contaminated water sources should be closely monitored at all times because the communities that rely on them are at risk. As a result, continual surveillance of rivers and communal boreholes, an integrated approach, and strong policies are required to monitor these cases in a timely and consistent manner. This will also help to improve cleanliness around these sources of water. Further research is also needed to identify the ecology, virulence characteristics, and antibiotic sensitivity of the various serogroups (O1 and O139) found in this location.
Data availability statement
The original contributions presented in the study are included in the article/Supplementary Material, further inquiries can be directed to the corresponding author.
Author contributions
LK: Conceptualization, Data curation, Formal Analysis, Investigation, Methodology, Project administration, Resources, Software, Supervision, Validation, Visualization, Writing–original draft, Writing–review and editing. MR: Formal Analysis, Investigation, Methodology, Software, Writing–review and editing. AT: Investigation, Methodology, Supervision, Writing–review and editing. NP: Conceptualization, Data curation, Formal Analysis, Funding acquisition, Investigation, Methodology, Project administration, Resources, Software, Supervision, Validation, Visualization, Writing–review and editing.
Funding
The author(s) declare that no financial support was received for the research, authorship, and/or publication of this article.
Acknowledgments
The authors would like to thank Damien Jacobs and Miss Mafunise for dedicating their time during sampling period and always available to assist throughout the study. We also like to thank Farai from the department of Environmental Science for developing the map of the study sites.
Conflict of interest
The authors declare that the research was conducted in the absence of any commercial or financial relationships that could be construed as a potential conflict of interest.
Publisher’s note
All claims expressed in this article are solely those of the authors and do not necessarily represent those of their affiliated organizations, or those of the publisher, the editors and the reviewers. Any product that may be evaluated in this article, or claim that may be made by its manufacturer, is not guaranteed or endorsed by the publisher.
Supplementary material
The Supplementary Material for this article can be found online at: https://www.frontiersin.org/articles/10.3389/fenvs.2024.1498893/full#supplementary-material
References
Abbott, S. L. (2011). Klebsiella, enterobacter, citrobacter, serratia, plesiomonas, and other Enterobacteriaceae. Man. Clin. Microbiol., 639–657. doi:10.1128/9781555816728.ch37
Alaoui, H. L., Oufdou, K., and Mezrioui, N. E. (2021). Prevalence, antimicrobial resistance and pathogenicity of non-O1 Vibrio cholerae in suburban and rural groundwater supplies of marrakesh area (Morocco). IntechOpen: Infections and Sepsis Development.
Ali, M., Nelson, A. R., Lopez, A. L., and Sack, D. A. (2015). Updated global burden of cholera in endemic countries. PLoS neglected Trop. Dis. 9 (6), e0003832. doi:10.1371/journal.pntd.0003832
Almagro-Moreno, S., and Taylor, R. K. (2013). Cholera: environmental reservoirs and impact on disease transmission. Microbiol. Spectr. 1 (2), 10–1128. doi:10.1128/microbiolspec.oh-0003-2012
Ansaruzzaman, M., Bhuiyan, N. A., Safa, A., Sultana, M., Mcuamule, A., Mondlane, C., et al. (2007). Genetic diversity of El Tor strains of Vibrio cholerae O1 with hybrid traits isolated from Bangladesh and Mozambique. Int. J. Med. Microbiol. 297 (6), 443–449. doi:10.1016/j.ijmm.2007.01.009
Arteaga, M., Velasco, J., Rodriguez, S., Vidal, M., Arellano, C., Silva, F., et al. (2020). Genomic characterization of the non-O1/non-O139 Vibrio cholerae strain that caused a gastroenteritis outbreak in Santiago, Chile, 2018. Microb. genomics 6 (3), e000340. doi:10.1099/mgen.0.000340
Atekwana, E. A., Atekwana, E. A., Rowe, R. S., Werkema, D. D., and Legall, F. D. (2004). The relationship of total dissolved solids measurements to bulk electrical conductivity in an aquifer contaminated with hydrocarbon. J. Appl. Geophys. 56 (4), 281–294. doi:10.1016/s0926-9851(04)00057-6
Bain, R., Johnston, R., Mitis, F., Chatterley, C., and Slaymaker, T. (2018). Establishing sustainable development goal baselines for household drinking water, sanitation and hygiene services. Water 10, 1711. doi:10.3390/w10121711
Barcelona, M., Keely, J. F., Pettyjohn, W. A., and Wehrmann, A. (1988) “Handbook of groundwater protection,” in Science information centre. Washington DC, USA: Hemisphere Publishing Corporation.
Bhandari, M. (2023). Genomic and virulence studies of Vibrio cholerae O1 and non-O1, non-O139 in queensland Australia. Queensland, Australia: Queensland University of Technology.
Bina, J., Zhu, J., Dziejman, M., Faruque, S., Calderwood, S. J., and Mekalanos, J. (2003). ToxR regulon of Vibrio cholerae and its expression in Vibrios shed by cholera patients. Proc. Natl. Acad. Sci. U. S. A. 100 (5), 2801–2806. doi:10.1073/pnas.2628026100
Chin, C. S., Sorenson, J., Harris, J. B., Robins, W. P., Charles, R. C., Jean-Charles, R. R., et al. (2011). The origin of the Haitian cholera outbreak strain. N. Engl. J. Med. 364 (1), 33–42. doi:10.1056/nejmoa1012928
Cho, J. Y., Liu, R., Macbeth, J. C., and Hsiao, A. (2021). The interface of Vibrio cholerae and the gut microbiome. Gut Microbes 13 (1), 1937015. doi:10.1080/19490976.2021.1937015
de Magny, G. C., Mozumder, P. K., Grim, C. J., Hasan, N. A., Naser, M. N., Alam, M., et al. (2011). Role of zooplankton diversity in Vibrio cholerae population dynamics and in the incidence of cholera in the Bangladesh Sundarbans. Appl. Environ. Microbiol. 77 (17), 6125–6132. doi:10.1128/aem.01472-10
Department of Water Affairs and Forestry (DWAF) (1996a). “South African water quality guidelines,”, 7. Pretoria, South Africa: Aquatic Ecosystems.
Department of Water Affairs and Forestry (DWAF) (1996b). “South African water quality guidelines,”, 1. Pretoria, South Africa: Domestic Use.
Ferreira, S. L. (2011). Microbial and physico-chemical quality of groundwater in the North-West Province, South Africa. North-West University (South Africa)).
Fong, J. C., Syed, K. A., Klose, K. E., and Yildiz, F. H. (2010). Role of Vibrio Polysaccharide (vps) genes in VPS production, biofilm formation and Vibrio cholerae pathogenesis. Microbiology 156, 2757–2769. doi:10.1099/mic.0.040196-0
Franco, A. A., Fix, A. D., Prada, A., Paredes, E., Palomino, J. C., Wright, A. C., et al. (1997). Cholera in Lima, Peru, correlates with prior isolation of Vibrio cholerae from the environment. Am. J. Epidemiol. 146 (12), 1067–1075. doi:10.1093/oxfordjournals.aje.a009235
Fu, X., Zhang, J., Li, T., Zhang, M., Li, J., and Kan, B. (2018). The outer membrane protein OmpW enhanced V. cholerae growth in hypersaline conditions by transporting carnitine. Front. Microbiol. 8, 2703. doi:10.3389/fmicb.2017.02703
Grandjean, D., Fass, S., Tozza, D., Cavard, J., Lahoussine, V., Saby, S., et al. (2005). Coliform culturability in over-versus undersaturated drinking waters. Water Res. 39, 1878–1886. doi:10.1016/j.watres.2005.03.012
Griffith, D. C., Kelly-Hope, L. A., and Miller, M. A. (2006). Review of reported cholera outbreaks worldwide, 1995–2005. Am. J. Trop. Med. Hyg. 75, 973–977. PMID: 17123999. doi:10.4269/ajtmh.2006.75.973
Gustaw, K., Niedźwiedź, I., Rachwał, K., and Polak-Berecka, M. (2021). New insight into bacterial interaction with the matrix of plant-based fermented foods. Foods 10 (7), 1603. doi:10.3390/foods10071603
Harris, J. F., Ryan, E. T., Calderwood, S. B., and Calderwood, S. B. (2012). Cholera. Lancet 379, 2466–2476. doi:10.1016/S0140-6736(12)60436-X
Hoshino, K., Yamasaki, S., Mukhopadhyay, A. K., Chakraborty, S., Basu, A., Bhattacharya, S. K., et al. (1998). Development and evaluation of a multiplex PCR assay for rapid detection of toxigenic Vibrio cholerae O1 and O139. FEMS Immunol. Med. Microbiol. 20 (3), 201–207. doi:10.1016/s0928-8244(98)00014-5
Huang, J., Zhu, Y., Wen, H., Zhang, J., Niu, J., and Li, Q. (2009). Quadruplex real-time PCR assay for detection and identification of Vibrio cholerae O1 and O139 strains and determination of their toxigenic potential. Appl. Environ. Microbio. 75 (22), 6981–6985. doi:10.1128/AEM.00517-09
Huq, A., Haley, B. J., Taviani, E., Chen, A., Hasan, N. A., and Colwell, R. R. (2012). Detection, isolation, and identification of Vibrio cholerae from the environment. Curr. Protoc. Microbiol. 26 (1), Unit6A.5–65. doi:10.1002/9780471729259.mc06a05s26
ICMSF (International Commission for Microbiological Specifications for Foods) (1996). “Vibrio cholerae,” in Microorganisms in foods: 5. Characteristics of microbial pathogens (London, United Kingdom: Blackie Academic and Professional), 414–425.
Ja'afar, A. Z., Nillian, E., Bilung, L. M., Bebey, G., Zakaria, D., and Benjamin, P. G. (2021). Detection of cholera toxin (ctxA and ctxAB) genes in Vibrio cholerae isolated from clinical and environmental samples in Limbang Sarawak by multiplex polymerase chain reaction (PCR). Malays. J. Microbiol. 17 (1).
Kachienga, L., Prosperit, M., Traore, A. N., and Potgieter, N. (2024). Assessment of the presence of Vibrio cholera and detection of toxigenic Vibrio cholerae in river sources within the Vhembe District Municipality Limpopo province of South Africa. J. Water Health 2024062. doi:10.2166/wh.2024.062
Kaper, J. B., Morris, J. G., and Levine, M. M. (1995). Cholera. Clin. Microbiol. Rev. 8, 48–86. doi:10.1128/cmr.8.1.48-86.1995
Keddy, K. H., Sooka, A., Parsons, M. B., Njanpop-Lafourcade, B. M., Fitchet, K., and Smith, A. M. (2013). Diagnosis of Vibrio cholerae O1 infection in Africa. J. Infect. Dis. 208 (Suppl. l_1), S23–S31. doi:10.1093/infdis/jit196
Khouadja, S., Suffredini, E., Baccouche, B., Croci, L., and Bakhrouf, A. (2014). Occurrence of virulence genes among Vibrio cholerae and Vibrio parahaemolyticus strains from treated wastewaters. Environ. Monit. Assess. 186, 6935–6945. doi:10.1007/s10661-014-3900-9
Liang, W., Pascual-Montano, A., Silva, A. J., and Benitez, J. A. (2007). The cyclic AMP receptor protein modulates quorum sensing, motility and multiple genes that affect intestinal colonization in Vibrio cholerae. Microbiology 153 (9), 2964–2975. doi:10.1099/mic.0.2007/006668-0
Marin, M. A., Thompson, C. C., Freitas, F. S., Fonseca, E. L., Aboderin, A. O., Zailani, S. B., et al. (2013). Cholera outbreaks in Nigeria are associated with multidrug resistant atypical El Tor and non-O1/non-O139 Vibrio cholerae. PLoS Neglected Trop. Dis. 7 (2), 2049. doi:10.1371/journal.pntd.0002049
Masuet, A., umatell, C., Ramon Torrell, J. M., and Zuckerman, J. N. (2011). Review of oral cholera vaccines: efficacy in young children. Infect. drug Resist. 4, 155–160. doi:10.2147/idr.s10339
Mehrabadi, J. F., Morsali, P., Nejad, H. R., and Fooladi, A. A. I. (2012). Detection of toxigenic Vibrio cholerae with new multiplex PCR. J. Infect. Pub. Health. 5 (3), 263–267. doi:10.1016/j.jiph.2012.02.004
Metsalu, T., and Vilo, J. (2015). ClustVis: a web tool for visualizing clustering of multivariate data using Principal Component Analysis and heatmap. Nucleic acids Res. 43 (W1), W566–W570. doi:10.1093/nar/gkv468
Momtaz, H., Dehkordi, F. S., Rahimi, E., and Asgarifar, A. (2013). Detection of Escherichia coli, Salmonella species, and Vibrio cholerae in tap water and bottled drinking water in Isfahan, Iran. BMC Public Health 13, 556–557. doi:10.1186/1471-2458-13-556
Nandi, B., Nandy, R. K., Mukhophyay, S., Nair, G. B., Shimada, T., and Ghose, A. C. (2000). Rapid method for species-specific identification of Vibrio cholerae using primers targeted to the gene of outer membrane protein OmpW. J. Clin. Microbio 38, 4145–4151. doi:10.1128/jcm.38.11.4145-4151.2000
World Health Organization (WHO) (2017). 2017 Cholera vaccines: WHO position paper–August 2017–Vaccins anticholériques. Wkly. Epidemiol. Rec. Relevé épidémiologique Hebd. 92 (34), 477–498.
National Center for Infectious Diseases (US) (1994). 1994 laboratory methods for the diagnosis of Vibrio cholerae. New York, USA: CDC/NCID.
Ntema, V. M., Potgieter, N., Van Blerk, G., and Barnard, T. G. (2014). Investigating the occurrence and survival of Vibrio cholerae in selected surface water sources in the KwaZulu-natal province of South Africa: Report to the water research commission. Water Res. Comm.
Ozochi, C. A., Okonkwo, C. C., Adukwu, E. C., Ujor, V. C., Enebe, M. C., and Chigor, V. N. (2024). Detection and quantitative microbial risk assessment of pathogenic Vibrio cholerae in a river used for drinking, domestic, fresh produce irrigation and recreational purposes. Discov. Water 4 (1), 7. doi:10.1007/s43832-024-00059-z
Phetla, V. (2021). Assessment of Vibrio cholerae and its biotypes associated with wastewater, sludge, river water and riverbed sediment: a case study in Limpopo province. J. Environ. Microbiol. 1 (1).
Potgieter, N., Karambwe, S., Mudau, L. S., Barnard, T., and Traore, A. (2020). Human enteric pathogens in eight rivers used as rural household drinking water sources in the northern region of South Africa. Int. J. Environ. Res. public health 17 (6), 2079. doi:10.3390/ijerph17062079
Rita, R., and Colwell, R. R. (2009). Cholera and climate: a demonstrated relationship. Trans. Am. Clin. Climatol. Assoc. 120, 119–128.
Sharma, A., Dutta, B. S., Rabha, D., Rasul, E. S., and Hazarika, N. K. (2021). Molecular characterization of Vibrio cholerae O1 strains circulating in Assam: a northeastern state of India. Iran. J. Microbiol. 13 (5), 583–591. doi:10.18502/ijm.v13i5.7420
Sikder, M., Deshpande, A., Hegde, S. T., Malembaka, E. B., Gallandat, K., Reiner, R. C., et al. (2023). Water, sanitation, and cholera in sub-saharan Africa. Environ. Sci. and Technol. 57 (28), 10185–10192. doi:10.1021/acs.est.3c01317
Takahashi, E., Kitahara, K., Miyoshi, S. I., Chowdhury, G., Mukhopadhyay, A. K., Dutta, S., et al. (2023). Environmental water in Kolkata is suitable for the survival of Vibrio cholerae O1. Environ. Res. 222, 115374. doi:10.1016/j.envres.2023.115374
Tasnia, R. (2023). Production of polyclonal antisera of vibrio cholerae O1 and O139 to detect pathogenic vibrio cholerae. Dhaka, Bangladesh: Brac University.
United Nations (2024). OHCHR, UN-habitat, WHO. The right to water, fact sheet No. 35 2010. Available at: https://www.ohchr.org/en/publications/fact-sheets/fact-sheet-no-35-right-water (Accessed July 10, 2024).
Vhembe, D. (2013). “Vhembe district municipality profile,” in Cooperative governance and traditional Affairs. Pretoria.
Waldor, M. K., and Mekalanos, J. J. (1996). Lysogenic conversion by a filamentous phage encoding cholera toxin. Science 272 (5270), 1910–1914. doi:10.1126/science.272.5270.1910
Warriner, K., Ibrahim, F., Dickinson, M., Wright, C., and Waites, W. M. (2003). Internalization of human pathogens within growing salad vegetables. Biotechnol. Genet. Eng. Rev. 20 (1), 117–136. doi:10.1080/02648725.2003.10648040
WHO (World Health Organization) (2005). Risk assessment of choleragenic Vibrio cholerae 01 and 0139 in warm water shrimp for international trade: interpretative summary and technical report. Geneva, Switzerland: World Health Organization.
Yadava, J. P., Jain, M., and Goel, A. K. (2013). Detection and confirmation of toxigenic Vibrio cholerae O1 in environmental and clinical samples by a direct cell multiplex PCR. Water sa. 39 (5), 611–614. doi:10.4314/wsa.v39i5.4
Yeung, M., and Thorsen, T. (2016). Development of a more sensitive and specific chromogenic agar medium for the detection of vibrio parahaemolyticus and other vibrio species. J. Vis. Exp. 117, 54493. doi:10.3791/54493
You, J. S., Yong, J. H., Kim, G. H., Moon, S., Nam, K. T., Ryu, J. H., et al. (2019). Commensal-derived metabolites govern Vibrio cholerae pathogenesis in host intestine. Microbiome 7, 1–18. doi:10.1186/s40168-019-0746-y
Keywords: serotype group, Vibrio cholerae O1, surface water, communal borehole, water quality, waterborne transmission
Citation: Kachienga L, Rikhotso MC, Traore AN and Potgieter N (2024) Surveillance of Vibrio cholerae serogroups (O1 and O139) from surface and ground water sources in the Vhembe district, Limpopo province, South Africa. Front. Environ. Sci. 12:1498893. doi: 10.3389/fenvs.2024.1498893
Received: 19 September 2024; Accepted: 26 November 2024;
Published: 11 December 2024.
Edited by:
Maria Elisa Magri, Federal University of Santa Catarina, BrazilReviewed by:
Mahmuda Yasmin, University of Dhaka, BangladeshHongxia Ming, National Marine Environmental Monitoring Center, China
Copyright © 2024 Kachienga, Rikhotso, Traore and Potgieter. This is an open-access article distributed under the terms of the Creative Commons Attribution License (CC BY). The use, distribution or reproduction in other forums is permitted, provided the original author(s) and the copyright owner(s) are credited and that the original publication in this journal is cited, in accordance with accepted academic practice. No use, distribution or reproduction is permitted which does not comply with these terms.
*Correspondence: Leonard Kachienga, bGVva2FjaGllbmdhQGdtYWlsLmNvbQ==