- 1Graduate Faculty of Environment, University of Tehran, Tehran, Iran
- 2Faculty of Arts and Sciences, Department of Biology, Giresun University, Giresun, Türkiye
Sediment pollution from potentially hazardous elements (PHE) generated by human activity has grown into a worldwide problem. In this regard, for the first time, surface sediment samples were obtained from six points along the Shahid Rajaee Reservoir for this investigation. Elements concentrations (Cu, Cr, Co, Mn, Fe, Pb, Ni, and Zn) were assessed using ICP-MS. Based on the individual indices (geoaccumulation index, contamination factor), all elements at all sample sites were found to be low in contamination, except Pb and Zn (at sites 1 and 2). The combined effect of PHEs in the sediment samples did not show a considerable concern, according to the synergistic indices. The health risk assessment revealed that recreational receptors were unlikely to face non-carcinogenic or carcinogenic hazards as a consequence of exposure to PHEs in sediments with unintentional ingesting and contact with the skin. The Tessier technique demonstrated that all PHEs were mostly connected with the oxidizable fraction. According to statistical study, while Cu, Co, Cr, Ni, Fe, and Mn were obtained from lithogenic sources, Pb and Zn came from natural as well as human-made sources.
Highlights
• Based on the health risk assessment recreational receptors were unlikely to face non-carcinogenic or carcinogenic hazards from PHE exposure in sediments.
• Cu, Co, Cr, Ni, Fe, and Mn were obtained from lithogenic sources, while Pb and Zn came from natural as well as human-made sources.
• CF and Igeo indicated that the sediments were only polluted with Pb and Zn.
1 Introduction
PHEs are thought to be of significant ecological value since they cannot be entirely eliminated, destroyed, or deteriorated during the self-purification process of water; instead, they pile up in reservoirs and enter into the food web from there (Li et al., 2023; Din et al., 2024; Tokatli et al., 2024; Yang et al., 2024). The concentration of PHEs in a reservoir increases mostly in the sediment’s lowermost layer. Anthropogenic activities are largely responsible for the entry of these elements into the environment; but, natural events like rock weathering and volcanic activity may also significantly contribute to the enrichment of water bodies with PHE (Kankılıç et al., 2013).
Reservoir sediment often accumulates at a rapid pace (≥ 2 cm/year). There is limited information about PHE contamination in reservoirs and its effects after protracted usage (Vukovic et al., 2014). PHEs stored in sediments may be released back into the water column as a consequence of shifts in sediment hydrodynamic circumstances and characteristics, resulting in secondary contamination. As a result, sediments act as both essential sinks and possible sources of PHEs in aquatic environments (Xiao et al., 2019; Varol et al., 2020; Kuang et al., 2023). As a consequence, measuring PHE levels in sediments and recognizing human and natural portions is critical in evaluating the restoration strategies and the ecological integrity of sediments, in addition to providing crucial data for analyzing environmental health issues (Kachoueiyan et al., 2024; Tiabou et al., 2024).
PHEs exist as exchangeable (F1), carbonate (F2), reducible (F3), oxidizable (F4), and residual (F5) fractions (Zhang et al., 2017). PHE fractions have varying negative environmental consequences. The majority of assessment techniques rely only on the overall element content. Nevertheless, total levels of elements simply represent the overall amount of contamination and provide no information about bioavailability or chemical activity. In reality, the toxicity of PHEs is determined by their chemical speciation rather than their total concentration. The assessment centered on the speciation of elements is more useful in understanding their environmental concerns (Gu, 2018; Lin et al., 2022; Sun et al., 2024).
Shahid Rajaee Reservoir is situated in northern Iran. The reservoir is utilized for flood management, as well as to provide water for hydroelectric power generation and drinking water to Sari City’s residents (Rahmani et al., 2022). Considering its significance, a comprehensive assessment of the PHEs in Shahid Rajaee reservoir sediment is needed to protect water supplies and human wellbeing. Nonetheless, as far as we know, no studies on the level of PHEs in Shahid Rajaee reservoir sediments have been conducted, nor have the ecological and health risk evaluation, source, and speciation of PHEs in Shahid Rajaee reservoir been thoroughly investigated. To provide a scientific basis to measure the overall condition of the study area, the primary aims of this research were to 1) assess the spatial distribution of elements (Cu, Co, Mn, Cr, Ni, Fe, Zn, and Pb) in sediments of the Shahid Rajaee Reservoir, and evaluate PHEs contamination using pollution indices, 2) determine the geochemical speciation of PHEs in the sediments using the Tessier sequential extraction procedure, 3) examine their potential health and environmental risk assessment and analyze elements origin using statistical techniques.
2 Materials and methods
2.1 Study area
Shahid Rajaee Dam is located in Tange-Soleiman, Mazandaran state, around 40 km southeast of Sari. It is built on the Tajan River in the region to offer vital irrigation water for lush agricultural fields in the dam’s downstream plains, as well as to provide hydroelectricity, flood control, and tourism attractions in Northern Iran’s gorgeous woodlands. The dam is a double-curvature concrete arch dam with a crest length of 430 m, a foundation width of 27 m, and a crest height of 7 m. The height above the foundation is 138 m, while the distance from the riverbank is 116 m. The spillway controls 800 cubic meters of water each second, and the lake’s size at normal water level is 158 cubic meters. Figure 1 depicts the location of the Shahid Rajaee Dam.
2.2 Sampling and sample analysis
Surface sediments were gathered from six stations around the Rajayi reservoir by a stainless steel grab. To prevent contamination, samples were packed in sacks and stored at 4°C before being transported to the laboratory. The sediment loss on ignition (LOI) was measured using ASTM-D7348-08. The samples of sediment were sifted using 63 μm sifter after air-drying at room temperature. The Tessier sequential extraction approach was utilized to investigate the repartition of metals in five different fractions: exchangeable (F1), carbonate (F2), reducible (F3), oxidizable (F4), and residual (F5). Cu, Co, Cr, Pb, Mn, Zn, Fe, and Ni concentrations were determined by ICP-MS (ELAN DRC-e; PerkinElmer). In order to assess the analytical procedure’s validity and accuracy, duplicate tests and certified reference substances (MESS-3) from the National Research Council of Canada were examined. The sequential extraction procedure achieved a recovery rate of 95%–104% for all components.
2.3 Sediment assessment procedures
2.3.1 Pollution assessment indexes
Supplementary Table S1 demonstrates the indexes used, including formulae and their ranges. The level of contamination in the sediment samples by PHEs was determined using the contamination factor (CF), geoaccumulation index (Igeo), pollution load index (PLI) and modified degree of contamination (mDc). To assess the ecological risk of PHEs, the potential ecological risk (PER) and toxic risk index (TRI) were used (Ustaoğlu et al., 2022). TRI, PER, mDc, and PLI are synergistic indexes that demonstrate the overall contamination level of various PHEs and their merged dangers to the environment. Nevertheless, Igeo, and CF are individual indices that represent the contamination level of a specific PHE and its impact on the environment (Islam et al., 2023).
2.3.2 Health risk assessment (HRA)
The interaction between the human health, ecosystem, and pollutants in the environment can be evaluated by calculating the human health risk using USEPA criteria. The current research evaluates carcinogenic and non-carcinogenic hazards via the ingestion, and dermal routes. Exposure to an element might cause health risks to vary by location. The following Equations 1–7 were used to determine CRs and HQs (USEPA, 2023). Supplementary Tables S3, S4 provide information on the equations.
Non-carcinogenic risks:
Carcinogenic risks:
Where:
Where:
Furthermore, the total non-carcinogenic risks including, hazard index (HI), total HI (THI), and total carcinogenic risk (TCR) levels were calculated. The subsequent formulae were used to calculate the TCR, HI, and THI values (Tokatlı et al., 2023):
2.3.3 Sediment quality guidelines
Sediment quality guidelines (SQGs) offer a valuable tool for screening contamination of sediments by comparison of the pollution concentrations to the relevant quality standard. These recommendations assess the extent to which the sediment-associated chemical estate may harm different species in aquatic environments and are intended to aid in sediment quality interpretation. SQGs have been used for a variety of purposes, including interpreting historical data, designing monitoring programs, developing sediment quality remediation goals, conducting ecological risk assessments and remedial research, and evaluating the prospective dredged substances quality (Varol, 2011). In this investigation, the Cr, Zn, Cu, Ni, and Pb concentrations in the sediment samples were compared to the threshold and probable impact concentrations (TEC and PEC) established by MacDonald et al. (2000) (MacDonald et al., 2000). Element level less than TEC implies that benthic life would be unaffected, however, PHE content more than PEC suggests that detrimental environmental consequences may happen.
2.4 Data analysis
The SPSS software (IBM SPSS Statistics 27) was used for the analysis of data, including the generation of descriptive statistics. OriginPro 2022 were used for visualizations and statistical analyses. Pearson correlation was utilized to determine the correlations between elements. According to Fowler et al. (2013) (Fowler et al., 2013), correlation intensity was defined by the following values: 0.0 ≤ r < 0.19 (a very weak correlations), 0.19 ≤ r < 0.39 (weak correlations), 0.39 ≤ r < 0.69 (moderate correlations), and r ≥ 0.69 (high correlations). Hierarchical cluster analysis (HCA) was used to assess element similarity and their potential sources. Variances were considered significant if p < 0.05.
3 Results and discussion
3.1 Spatial variation of studied elements in sediments
Sediment samples from six stations in the Shahid Rajayee Reservoir were analyzed for eight elements (Cu, Cr, Co, Pb, Ni, Zn, Mn, and Fe). The findings showed that the mean concentrations of the examined elements were ranked as Fe > Mn > Zn > Ni > Pb > Cr > Co > Cu, with mean values of 8500, 242.40, 170.80, 32.37, 29.82, 26.49, 17.93, and 14.19 mg/kg. In sediment and soil investigations, Fe is often found at the greatest levels, followed by Mn (Table 1). Table 1 also compares the average concentration of studied elements with similar environments around the world. The mean Zn contents in the Shahid Rajayee Reservoir was the greatest when compared to comparable environments described in Table 1. Chromium levels were greater (26.49 mg/kg) than those found in dam reservoirs in Poland (7.74 mg/kg) (Sojka et al., 2022) and Aguamilpa, Mexico (9.26 mg/kg). The Aguamilpa Dam is part of the reservoir waterfall system, which consists of four reservoirs along the Santiago River’s middle and lower reaches. For decades, this structure has absorbed industrial and urban from Guadalajara’s urban region, as well as runoff from agricultural lands in the area surrounding the river (Rangel-Peraza et al., 2015). Furthermore, only the Manwan Reservoir in China has a bigger PHE load than the Shahid Rajayee Reservoir. The Manwan hydroelectric dam is positioned in the central reach of the Lancang River, and according to the findings, the origins of PTEs in this region were anthropogenic, that include tributary river industrial operations (smelting and heavy metal mining) and agricultural practices (pesticide usage and fertilization) (Wang et al., 2012). The research region had relatively higher Pb levels than the Wadi Al-Aqiq water reservoir dam in Saudi Arabia (Alghamdi et al., 2019), the Ziqlab reservoir in Jordan (Al-Taani et al., 2015), and Aguamilpa in Mexico (Rangel-Peraza et al., 2015). However, compared to the current research, Cr and Co concentrations were found to be greater in the sediments of the Hongfeng reservoir in China (Wang et al., 2015) and the Castilseras reservoir in Spain. The Castilseras reservoir is an artificial water body situated alongside the Valdeazogues River, which runs south from the Almadén mercury mining sector and gets major input from numerous nearby cinnabar mines (García-Ordiales et al., 2016).
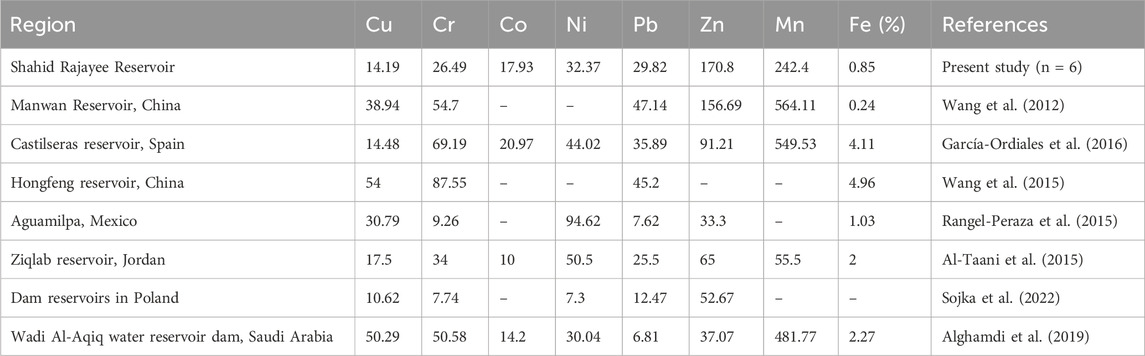
Table 1. Comparison of the studied elements level in sediments of the Shahid Rajayee Reservoir with other regions (mg/kg).
3.2 Contamination and ecological risk assessment
3.2.1 Sediment contamination assessment
The results of Igeo is presented in Figure 2. Igeo values for Cu ranged from −3.29 to −2.1, Co from – 1.21 to – 0.92, Cr from – 3.11 to −2.20, Ni from – 1.89 to – 1.59, Pb from – 0.29 to 1.61, Zn from – 0.43 to – 1.14, and Mn from – 4.18 to – 1.14. The computed Igeo values indicated that Cu, Cr, Co, Ni, and Mn were considered “practically unpolluted” at all locations along the sediment in the research area. However, Pb at stations S1 and S2 had Igeo values of 1.61 and 1.19, respectively, and Zn in S1 and S5 had Igeo values of 1.14 and 1.08, which were classified as “moderately polluted”. Additionally, the Igeo values for Pb at station S4 and Zn in stations S2 and S4 were between 0 and 1, indicating that they were in the “unpolluted to moderately polluted” category (Figure 2).
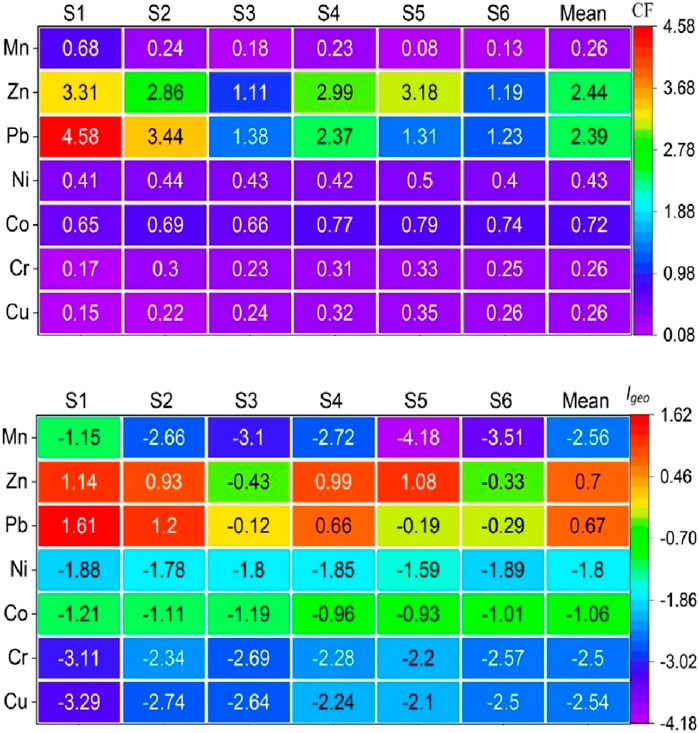
Figure 2. Contamination factor (CF) and geoaccumulation index (Igeo) of Cu, Cr, Co, Pb, Ni, Mn, and Zn.
The CF for Cr, Cu, Co, Mn, and Ni were <1 in sediments at all locations, indicating “low contamination” by mentioned elements. The CF for Pb at S1 and S2, as well as Zn at S1 and S5, indicated “considerable contamination,” whereas the CF values for these two elements at other stations suggested “moderate contamination.” In this research, Pb exhibited the highest CF values of the elements examined.
The calculated PLI for each sampling station is presented in Figure 3. The PLI across the study area varies from 0.452 in site 6 to 0.686 in site 1, suggesting that the study area is in the class of no pollution (PLI <1). Moreover, Figure 3 displays the modified degree of contamination (mCd) values in the sediments. The mDc levels varied from 0.602 in site 6 to 1.421 in site 1, suggesting zero to very low contamination since the calculated mDc was <1.5 in all stations.
3.2.2 Potential ecological risk (PER) and toxic risk index (TRI)
Figure 3 presents the toxic risk index (TRI) and the potential ecological risk index (PER). The PER varied from 15.02 in site 6 to 33.27 in site 1. The study area shows a low ecological risk index in all the studied sites (PER <150). The toxic risk index (TRI) values also range from 2.99 in sites 6 to 4.53 in site 1 of the study area (Figure 3). The entire study area is in the class of no toxic risk index (TRI<5). These results indicate that the study area witnessed a low toxicity effect of PHEs in the study area.
3.3 Health risk assessment
Table 2 displays the carcinogenic risk (CR and TCR) and non-carcinogenic (HQ, HI, and THI) levels for PHEs. All PHEs’ HQdermal and HQingestion values were below the permitted limit of one, showing that studied PHEs had no negative health effects on recreational users (children and adults). Likewise, THI (HQingestion + HQdermal) and the HIingestion and HIdermal values were beneath one, suggesting that the combined impacts of the seven PHEs in Rajayi reservoir sediments would cause no health danger to receptors. Furthermore, the HIdermal and HQdermal levels for each of the receptors were below the HIingestion and HQingestion levels. This shows that the ingesting route had a stronger negative effect on receptor health than the dermal contact path (Canpolat et al., 2022).
Carcinogenic risk (CR) values for Cr, Ni, and Pb ingestion pathways were 7.6 × 10−6 and 3.16 × 10−5, and 1.45 × 10−7 respectively, whereas their dermal pathways showed CR values of 1.67 × 10−6, 2.26 × 10−6, and 4.16 × 10−10. The CR and TCR values in the research region were within the permitted range of 1 × 10−4 and 1 × 10−6, indicating that exposure to Pb, Ni, and Cr in the sediments would not produce carcinogenic health consequences for recreationists.
Since the pollutants exposure technique exhibits spatiotemporal variation, the input variables for the exposure model might be changed across both space and time. As a result, further research is needed into the geographical and temporal modeling of various contaminants, sources, and pathways. Although the model may overestimate or underestimate, the findings may nevertheless give some useful insights and forecasts for local governments to deal with the present severe environmental concerns.
3.4 Application of sediment quality guidelines
It is critical to assess if the concentrations of PHE in sediments constitute a hazard to aquatic species. In this investigation, the concentrations of the PHE in sediment samples were contrasted to consensus-based PEC and TEC levels (Supplementary Table S2). Cr and Cu levels were below the TEC in all samples. Ni, Zn, and Pb were found between the TEC and PEC in 100%, 66.67%, and 33.4%, respectively. The findings indicate that none of the abovementioned ingredients have the potential to harm sediment-dwelling organisms.
3.5 Speciation of studied elements
The speciation features of elements in sediment are crucial for researching their origins and bioavailability. PHE migration is intimately associated with speciation. PHE speciation influences their ecological toxicity and natural cycle (Lin et al., 2022). Figure 4 depicts the distribution of the exchangeable (F1), carbonate (F2), reducible (F3), oxidizable (F4), and residual (F5) of the PHE fractions in the Shahid Rajaee Reservoir sediments. Ni, Cu, Co, Pb, Mn, Cr, and Zn the average concentrations in F1 and F2 bound fractions (labile fractions) were 17.6%, 17.5%, 16.5%, 14.5%, 12.6%, 12%, and 7.1% of the total quantity, respectively. The exchangeable and carbonate-bound fractions are partly the result of human intrusions. Pollutants from these fractions are readily discharged into the water, posing a significant danger to aquatic organisms (Saleem et al., 2018; Kachoueiyan et al., 2023).
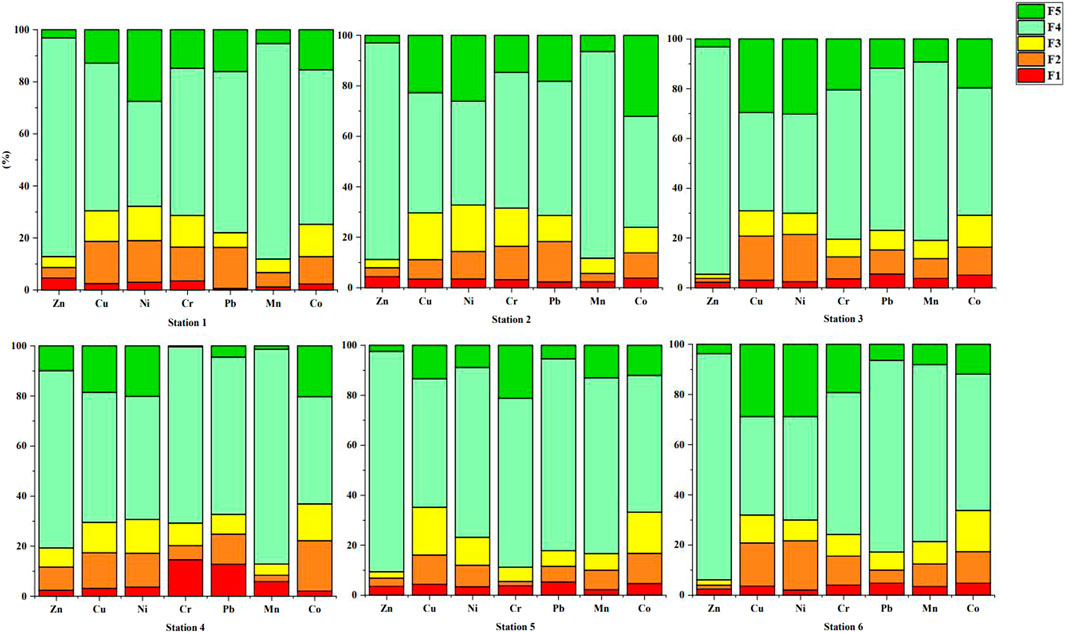
Figure 4. Percentage of PHEs fractions in the sediments of the Shahid Rajaee Reservoir, Iran (F1: Exchangable, F2: Carbonate, F3: Reducible, F4: Oxidizable and F5: Residual fraction).
F3 is the percentage that accumulates in the environment by coprecipitation or adsorption with (Fe)/(Mn) oxides. When water redox potential reduces or the water becomes hypoxic, Fe/Mn oxides are reduced, releasing elements into the environment (Ahn et al., 2020), causing water pollution. The F3 of PHEs in sediments in the Shahid Rajaee Reservoir ranged from 3.6% to 13.8%, with the principal PHEs in the F3 fraction occurring in the following order of quantity: Co (13.82) > Cu (13.81%) > Ni (12.2%) > Cr (9.64%) > Pb (7.54%) > Mn (6.45%) > Zn (3.6%). F4, or the fraction associated with organic matter and sulfides, is made up of element ions that bind to sulfide ions or organic matter active groups (ligands) to generate water-insoluble compounds (Shen et al., 2022). When there is a significant concentration of organic matter in the sediment, F4 may be mineralized and degraded by microorganisms, releasing a part of the PHEs into the overlying water or being absorbed by aquatic life. Based on the results, the level of organic matter (LOI = 7%) was insignificant, hence, the risk of releasing PHEs in the water column from sediment is almost low. All of the elements investigated at all stations were mainly linked to F4 fractions. The average F4 percentage of Zn, Mn, Pb, Cr, Co, Cu, and Ni in sediment was 85%, 77%, 66%, 60.8%, 51%, 47.7%, and 46.6% of their total quantity, respectively.
F5 is the proportion of elements in the crystal structure of primary and secondary ores that are exceptionally stable (Uddin et al., 2022). F5 has minimal effect on the mobility and bioavailability of elements in sediments, hence it is typically regarded as environmentally acceptable. F5 represented 3.77%–23.62% of all PHEs in sediment samples, with the following abundances: Ni (23.63%) > Cu (21%), Co (18.57%), Cr (17.5%), Pb (11.94%), Zn (4.23%), and Mn (3.77%).
3.6 Source apportionment of studied elements
Multivariate analysis, i.e., hierarchical cluster analysis (HCA) and correlation analysis (CA), are excellent procedures for tracing element origins (Aydın et al., 2023; Esmaeilzadeh and Mehdinia, 2024). CA (Figure 5A) reveals two main clusters: cluster one (Cu, Co, Cr, Ni, and Fe) and cluster two (Pb, Mn, and Zn). The elements found in the two clusters formed match the factors revealed by the Pearson correlation. The Pearson correlation (Figure 5B) for the sediment samples showed that Cu had a high correlation with Co (r = 0.92), Cr (r = 0.87), and Ni (r = 0.61), and Fe is also highly linked with Ni (r = 0.63), suggesting that these elements originate from a same source. The mean Igeo values of Co, Cu, Cr, and Ni were less than 0 and between 0 and 1, indicating that all four PHEs are mostly derived from crustal components or natural erosion.
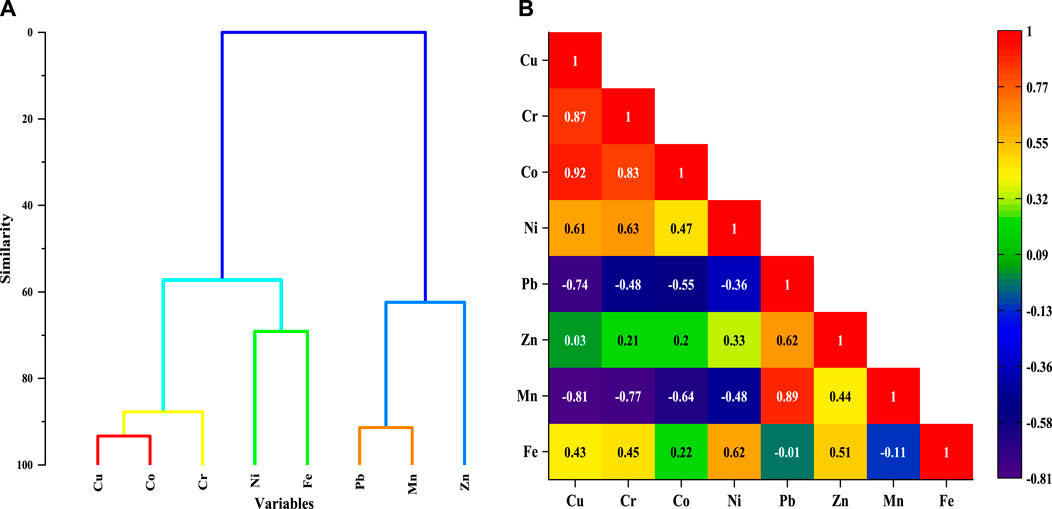
Figure 5. Hierarchical cluster analysis and Pearson correlation of studied elements in sediments of the Shahid Rajaee Reservoir.
Moreover, Mn displays a significant correlation with Pb (r = 0.89). Previous research suggested that Mn is a lithogenic metal, originating mostly from natural sources via erosion and weathering of soil and rock parent materials (Li et al., 2021; Proshad et al., 2022). In this investigation, Mn showed low CF and Igeo values. Thus, it is presumed that Mn was mostly influenced by the parent materials. Pb had substantial positive relationships with Mn, indicating that Pb also has natural origins. However, according to CF and Igeo readings, Pb at stations 1 and 2 was “moderately polluted.” As a result, it is possible to infer that Pb concentrations have both natural and anthropogenic origins. Prim Road is near the research area. Vehicle fuel emissions may cause Pb concentration in sediments (Shi et al., 2022). Fuel emissions from automobiles on Prim Road are likely to contaminate sediments with Pb. The connection between Zn and Pb was somewhat positive (r = 0.62). Additionally, Zn concentrations may be linked to human activities, as it was found to have a positive connection with Pb. Zn is an element that plants require for growth, and when applied as fertilizer, it can gets taken up surface sediments (Jin et al., 2019). It needs to be noted that there are agricultural fields around the research location. In order to minimize total pollution from automobiles, vehicle emissions regulations should be enhanced. Moreover, by employing sustainable agriculture techniques, the inputs of PHE can be mitigated. This finding is consistent with the results of the contamination factor analysis. Overall, all PHEs had natural origins except for Pb and Zn, which were derived both from natural and human activities. These findings validated pollution indices results.
4 Conclusion
For the first time, this research examined the concentration, environmental concerns, health risk assessment, and sources of PHEs in Shahid Rajaee Reservoir sediment. Sediment pollution indicators, such as the contamination factor (CF) and geoaccumulation index (Igeo), revealed that sediments were exclusively contaminated with Pb and Zn. However, the cumulative indices, such as the pollution load index (PLI) and modified degree of contamination (mDc) values in the sediments, indicated zero to extremely low pollution. Based on the PER and TRI values, there are no ecological concerns associated with PHEs observed in the research area’s sediment. According to the sediment quality guidelines, none of the components examined had the potential to harm sediment-dwelling organisms. The HQ and THI readings were both less than one, suggesting that PHEs in the research area’s sediments would have no deleterious health impacts on recreational receptors. In a similar way, the TCR and CR values were at or below the danger range, indicating that carcinogenic health consequences were not anticipated for recreational users. CA and HCA findings revealed that all PHEs in the Shahid Rajaee Reservoir sediments were mostly derived from natural sources, while human activities contributed little to Pb and Zn. This research concluded that, although human activities have minimal effect on sedimentary PHEs in the study area, elements should be monitored and analyzed on a regular basis to guarantee ecosystem sustainability.
Data availability statement
The original contributions presented in the study are included in the article/Supplementary Material, further inquiries can be directed to the corresponding author.
Author contributions
FK: Conceptualization, Data curation, Formal Analysis, Investigation, Methodology, Project administration, Resources, Software, Visualization, Writing–review and editing. PA: Data curation, Visualization, Writing–original draft. AR: Resources, Writing–original draft, Writing–review and editing. FU: Data curation, Formal Analysis, Writing–original draft, Writing–review and editing. AK: Methodology, Resources, Supervision, Writing–review and editing.
Funding
The author(s) declare that no financial support was received for the research, authorship, and/or publication of this article.
Acknowledgments
We would express our gratitude to Graduate Faculty of Environment, University of Tehran, for providing us with the electro-flocculation laboratory to accomplish the experimental analyses of this work.
Conflict of interest
The authors declare that the research was conducted in the absence of any commercial or financial relationships that could be construed as a potential conflict of interest.
Publisher’s note
All claims expressed in this article are solely those of the authors and do not necessarily represent those of their affiliated organizations, or those of the publisher, the editors and the reviewers. Any product that may be evaluated in this article, or claim that may be made by its manufacturer, is not guaranteed or endorsed by the publisher.
Supplementary material
The Supplementary Material for this article can be found online at: https://www.frontiersin.org/articles/10.3389/fenvs.2024.1493547/full#supplementary-material
References
Ahn, Y., Yun, H.-S., Pandi, K., Park, S., Ji, M., and Choi, J. (2020). Heavy metal speciation with prediction model for heavy metal mobility and risk assessment in mine-affected soils. Environ. Sci. Pollut. Res. 27, 3213–3223. doi:10.1007/s11356-019-06922-0
Alghamdi, B. A., El Mannoubi, I., and Zabin, S. A. (2019). Heavy metals’ contamination in sediments of Wadi Al-Aqiq water reservoir dam at Al-Baha region, KSA: their identification and assessment. Hum. Ecol. risk Assess. Int. J. 25, 793–818. doi:10.1080/10807039.2018.1451746
Al-Taani, A. A., Batayneh, A. T., El-Radaideh, N., Ghrefat, H., Zumlot, T., Al-Rawabdeh, A. M., et al. (2015). Spatial distribution and pollution assessment of trace metals in surface sediments of Ziqlab Reservoir, Jordan. Environ. Monit. Assess. 187, 32–14. doi:10.1007/s10661-015-4289-9
Aydın, H., Tepe, Y., and Ustaoğlu, F. (2023). A holistic approach to the eco-geochemical risk assessment of trace elements in the estuarine sediments of the Southeastern Black Sea. Mar. Pollut. Bull. 189, 114732. doi:10.1016/j.marpolbul.2023.114732
Canpolat, Ö., Varol, M., Okan, Ö. Ö., and Eriş, K. K. (2022). Sediment contamination by trace elements and the associated ecological and health risk assessment: a case study from a large reservoir (Turkey). Environ. Res. 204, 112145. doi:10.1016/j.envres.2021.112145
Din, I. U., Muhammad, S., Faisal, S., Rehman, I. ur, and Ali, W. (2024). Heavy metal(loid)s contamination and ecotoxicological hazards in coal, dust, and soil adjacent to coal mining operations, Northwest Pakistan. J. Geochem. Explor. 256, 107332. doi:10.1016/j.gexplo.2023.107332
Esmaeilzadeh, M., and Mehdinia, A. (2024). Origin and comprehensive risk assessment of heavy metals in surface sediments along the Caspian Sea. Mar. Pollut. Bull. 205, 116587. doi:10.1016/j.marpolbul.2024.116587
Fowler, J., Cohen, L., and Jarvis, P. (2013). Practical statistics for field biology. John Wiley and Sons.
García-Ordiales, E., Esbrí, J. M., Covelli, S., López-Berdonces, M. A., Higueras, P. L., and Loredo, J. (2016). Heavy metal contamination in sediments of an artificial reservoir impacted by long-term mining activity in the Almadén mercury district (Spain). Environ. Sci. Pollut. Res. 23, 6024–6038. doi:10.1007/s11356-015-4770-6
Gu, Y.-G. (2018). Heavy metal fractionation and ecological risk implications in the intertidal surface sediments of Zhelin Bay, South China. Mar. Pollut. Bull. 129, 905–912. doi:10.1016/j.marpolbul.2017.10.047
Islam, A. R. M. T., Varol, M., Habib, M. A., and Khan, R. (2023). Risk assessment and source apportionment for metals in sediments of Kaptai Lake in Bangladesh using individual and synergistic indices and a receptor model. Mar. Pollut. Bull. 190, 114845. doi:10.1016/j.marpolbul.2023.114845
Jin, G., Fang, W., Shafi, M., Wu, D., Li, Y., Zhong, B., et al. (2019). Source apportionment of heavy metals in farmland soil with application of APCS-MLR model: a pilot study for restoration of farmland in Shaoxing City Zhejiang, China. Ecotoxicol. Environ. Saf. 184, 109495. doi:10.1016/j.ecoenv.2019.109495
Kachoueiyan, F., Karbassi, A., and Nasrabadi, T. (2023). Effect of electro-photocatalytic decomposition (mainly Eh–pH) on association of metals with sedimentary phases and their mobility in the wetland ecosystem. Environ. Earth Sci. 82, 437. doi:10.1007/s12665-023-11127-3
Kachoueiyan, F., Karbassi, A., Nasrabadi, T., Rashidiyan, M., and De-la-Torre, G. E. (2024). Speciation characteristics, ecological risk assessment, and source apportionment of heavy metals in the surface sediments of the Gomishan wetland. Mar. Pollut. Bull. 198, 115835. doi:10.1016/j.marpolbul.2023.115835
Kankılıç, G. B., Tüzün, İ., and Kadıoğlu, Y. K. (2013). Assessment of heavy metal levels in sediment samples of Kapulukaya Dam Lake (Kirikkale) and lower catchment area. Environ. Monit. Assess. 185, 6739–6750. doi:10.1007/s10661-013-3061-2
Kuang, Z., Wang, H., Han, B., Rao, Y., Gong, H., Zhang, W., et al. (2023). Coastal sediment heavy metal(loid) pollution under multifaceted anthropogenic stress: insights based on geochemical baselines and source-related risks. Chemosphere 339, 139653. doi:10.1016/j.chemosphere.2023.139653
Li, H.-S., Gu, Y.-G., Liang, R.-Z., Wang, Y.-S., Jordan, R. W., Wang, L.-G., et al. (2023). Heavy metals in riverine/estuarine sediments from an aquaculture wetland in metropolitan areas, China: characterization, bioavailability and probabilistic ecological risk. Environ. Pollut. 324, 121370. doi:10.1016/j.envpol.2023.121370
Li, Y., Chen, H., Song, L., Wu, J., Sun, W., and Teng, Y. (2021). Effects on microbiomes and resistomes and the source-specific ecological risks of heavy metals in the sediments of an urban river. J. Hazard. Mater. 409, 124472. doi:10.1016/j.jhazmat.2020.124472
Lin, S., Liu, X., Zhang, Z., and Zhang, Q. (2022). Speciation characteristics and ecological risk assessment of heavy metals in sediments from Caohai Lake, Guizhou Province. Stoch. Environ. Res. Risk Assess. 36, 3929–3944. doi:10.1007/s00477-022-02236-9
MacDonald, D. D., Ingersoll, C. G., and Berger, T. A. (2000). Development and evaluation of consensus-based sediment quality guidelines for freshwater ecosystems. Arch. Environ. Contam. Toxicol. 39, 20–31. doi:10.1007/s002440010075
Proshad, R., Kormoker, T., Abdullah Al, M., Islam, M. S., Khadka, S., and Idris, A. M. (2022). Receptor model-based source apportionment and ecological risk of metals in sediments of an urban river in Bangladesh. J. Hazard. Mater. 423, 127030. doi:10.1016/j.jhazmat.2021.127030
Rahmani, H., Shokri, M., Janikhalili, K., Abdoli, A., Cozzoli, F., and Basset, A. (2022). Relationships among biotic, abiotic parameters and ecological status in Shahid Rajaee reservoir (Iran). Biol. Bratisl. 77, 3159–3172. doi:10.1007/s11756-022-01203-7
Rangel-Peraza, J. G., de Anda, J., González-Farías, F. A., Rode, M., Sanhouse-García, A., and Bustos-Terrones, Y. A. (2015). Assessment of heavy metals in sediment of Aguamilpa Dam, Mexico. Environ. Monit. Assess. 187, 134–214. doi:10.1007/s10661-015-4359-z
Saleem, M., Iqbal, J., Akhter, G., and Shah, M. H. (2018). Fractionation, bioavailability, contamination and environmental risk of heavy metals in the sediments from a freshwater reservoir, Pakistan. J. Geochem. Explor. 184, 199–208. doi:10.1016/j.gexplo.2017.11.002
Shen, Q., Xiang, J., and Zhang, M. (2022). Distribution and chemical speciation of heavy metals in various size fractions of aggregates from zonal soils. Int. J. Environ. Anal. Chem. 102, 4272–4287. doi:10.1080/03067319.2020.1781840
Shi, W., Li, T., Feng, Y., Su, H., and Yang, Q. (2022). Source apportionment and risk assessment for available occurrence forms of heavy metals in Dongdahe Wetland sediments, southwest of China. Sci. Total Environ. 815, 152837. doi:10.1016/j.scitotenv.2021.152837
Sojka, M., Ptak, M., Jaskuła, J., and Krasniqi, V. (2022). Ecological and health risk assessments of heavy metals contained in sediments of Polish Dam Reservoirs. Int. J. Environ. Res. Public Health 20, 324. doi:10.3390/ijerph20010324
Sun, Q., Sun, B., Wang, D., Pu, Y., Zhan, M., Xu, X., et al. (2024). A review on the chemical speciation and influencing factors of heavy metals in Municipal Solid Waste landfill humus. Waste Dispos. sustain. Energy 6, 209–218. doi:10.1007/s42768-023-00186-8
Tiabou, A. F., Takem-Agbor, A. A., Yiika, L. P., Eseya Mengu, E., Kachoueiyan, F., and Agyingi, C. M. (2024). Distribution, source apportionment and ecological risk assessment of heavy metals in Limbe River sediments, Atlantic Coast, Cameroon Volcanic Line. Discov. Water 4, 62. doi:10.1007/s43832-024-00120-x
Tokatli, C., Mutlu, E., Ustaoğlu, F., Islam, A. R. T., and Muhammad, S. (2024). Spatiotemporal variations, health risk assessment, and sources of potentially toxic elements in potamic water of the Anday Stream Basin (Türkiye), Black Sea Region. Environ. Monit. Assess. 196, 420. doi:10.1007/s10661-024-12580-8
Tokatlı, C., Varol, M., Ustaoğlu, F., and Muhammad, S. (2023). Pollution characteristics, sources and health risks assessment of potentially hazardous elements in sediments of ten ponds in the Saros Bay region (Türkiye). Chemosphere 340, 139977. doi:10.1016/j.chemosphere.2023.139977
Uddin, M. M., Huang, C., Chen, X., and Huang, L. (2022). Speciation and mobility of heavy metals in the Yundang Lagoon catchment surface sediments after 30 years of continuous remediation and management at Xiamen in China. Chem. Ecol. 38, 48–71. doi:10.1080/02757540.2021.2005033
USEPA (2023). Regional Screening Levels (RSLS)–equations. Available at: https://www.epa.gov/risk/regional-screening-levels-rsis-equations (Accessed June 8, 2023).
Ustaoğlu, F., Kükrer, S., Taş, B., and Topaldemir, H. (2022). Evaluation of metal accumulation in Terme River sediments using ecological indices and a bioindicator species. Environ. Sci. Pollut. Res. 29, 47399–47415. doi:10.1007/s11356-022-19224-9
Varol, M. (2011). Assessment of heavy metal contamination in sediments of the Tigris River (Turkey) using pollution indices and multivariate statistical techniques. J. Hazard. Mater. 195, 355–364. doi:10.1016/j.jhazmat.2011.08.051
Varol, M., Canpolat, Ö., Eriş, K. K., and Çağlar, M. (2020). Trace metals in core sediments from a deep lake in eastern Turkey: vertical concentration profiles, eco-environmental risks and possible sources. Ecotoxicol. Environ. Saf. 189, 110060. doi:10.1016/j.ecoenv.2019.110060
Vukovic, D., Vukovic, Z., and Stankovic, S. (2014). The impact of the Danube Iron Gate Dam on heavy metal storage and sediment flux within the reservoir. Catena 113, 18–23. doi:10.1016/j.catena.2013.07.012
Wang, C., Liu, S., Zhao, Q., Deng, L., and Dong, S. (2012). Spatial variation and contamination assessment of heavy metals in sediments in the Manwan Reservoir, Lancang River. Ecotoxicol. Environ. Saf. 82, 32–39. doi:10.1016/j.ecoenv.2012.05.006
Wang, G., Yinglan, A., Jiang, H., Fu, Q., and Zheng, B. (2015). Modeling the source contribution of heavy metals in surficial sediment and analysis of their historical changes in the vertical sediments of a drinking water reservoir. J. Hydrol. 520, 37–51.
Xiao, H., Shahab, A., Li, J., Xi, B., Sun, X., He, H., et al. (2019). Distribution, ecological risk assessment and source identification of heavy metals in surface sediments of Huixian karst wetland, China. Ecotoxicol. Environ. Saf. 185, 109700. doi:10.1016/j.ecoenv.2019.109700
Yang, H., Feng, Q., Zhu, J., Liu, G., Dai, Y., Zhou, Q., et al. (2024). Towards sustainable futures: a review of sediment remediation and resource valorization techniques. J. Clean. Prod. 435, 140529. doi:10.1016/j.jclepro.2023.140529
Keywords: sediment pollution indices, sediment quality guidelines, risk assessment, ecological risk, potentially hazardous elements
Citation: Kachoueiyan F, Alipour Atmianlu P, Rajabi A, Ustaoğlu F and Karbassi A (2024) Contamination source, health and ecological risk assessment of hazardous elements in the sediment of the Shahid Rajaee reservoir. Front. Environ. Sci. 12:1493547. doi: 10.3389/fenvs.2024.1493547
Received: 09 September 2024; Accepted: 04 October 2024;
Published: 21 October 2024.
Edited by:
Antonije Onjia, University of Belgrade, SerbiaReviewed by:
Juan Manuel Trujillo-González, University of the Llanos, ColombiaAhmed Saqr, Mansoura University, Egypt
Copyright © 2024 Kachoueiyan, Alipour Atmianlu, Rajabi, Ustaoğlu and Karbassi. This is an open-access article distributed under the terms of the Creative Commons Attribution License (CC BY). The use, distribution or reproduction in other forums is permitted, provided the original author(s) and the copyright owner(s) are credited and that the original publication in this journal is cited, in accordance with accepted academic practice. No use, distribution or reproduction is permitted which does not comply with these terms.
*Correspondence: Fatemeh Kachoueiyan, ZmF0ZW1laGthY2hvdWVpeWFuQGdtYWlsLmNvbQ==