- 1Department of Environmental Science, Policy, and Management, University of California, Berkeley, Berkeley, CA, United States
- 2Landscape Architecture and Environmental Planning, University of California, Berkeley, Berkeley, CA, United States
The uneven spatial distribution of water resources and demands across the US have motivated a wide range of inter-basin water transfers. By redistributing water resources, Inter-basin water transfer projects can lead to specific environmental changes such as altered river flows, changes in water quality, and loss of ecologically important habitats, impacts which depend on project scale and management. Early water transfer projects were undertaken prior to legislation Since the primary focus is not on the environmental impacts of these projects, they are often not documented historically. We provided a comprehensive inventory of inter-basin water transfer projects (built, incomplete, proposed) in the US, identified patterns of these projects’ characteristics, analyzed the growing role of environmental planning in these projects, and drew lessons to inform future proposals. We categorized historical US water transfer projects into three periods: 1900–1930, 1930–1970, and 1970–2020, analyzing projects over 40 km long and 50 MCM/year using diverse sources, to assess their development from an environmental planning perspective. Results of this study show that the early projects, mostly gravity-driven and smaller-in-scale, grow in scale and require more pumping stations (energy-intensive) to lift the water over high elevations. California and Colorado are the most active, using these transfers for the first time. Federal agencies have reduced funding for most transfers due to a growing recognition of the environmental impacts, which were not adequately addressed in planning of early projects. Environmental impacts are crucial for the planning and operation of inter-basin water transfer projects. We recommend that assessments of climate change vulnerability should also be considered essential for future project proposals.
1 Introduction
“In the West, it is said, water flows uphill toward money,” observed Marc Reisner (Reisner, 1986). Water resources are unevenly distributed across North America, especially within the western half of the continent. The western US is arid to semi-arid, with less than 500 mm of annual rainfall. In the mid-nineteenth century, Mormons pioneered the dream of utilizing river water for irrigation by constructing dams and diverting rivers (Worster, 1985). This endeavor was expanded in the 1930s when the federal government constructed numerous dams on every major western river, setting the groundwork for the modern cities, farms, wealth, and power of the West. The drier regions of the western US heavily depend on inter-basin water transfers to support growing industrial and agricultural needs. These transfers involve moving surface water from one river basin to another with higher demand. Alternative terms for inter-basin water transfer are varied and include inter-regional water transfer (Golubev and Biswas, 1979; Quinn, 1968), long-distance water transfer (Biswas, 1983), large-scale water transfer (Golubev and Biswas, 1984; Yan et al., 2012), inter-river transfer, inter-catchment water transfer (Davies et al., 1992), mega inter-basin water transfer (E. Zhang et al., 2018), massive water diversion project (Lasserre, 2005), and water transfer megaproject (Shumilova et al., 2018; Tockner et al., 2016). Authors using these alternative terms typically specify minimum volumes of annual delivery and the distance over which water is moved. These criteria help differentiate large water transfer projects from smaller, local canals, conduits, and ditches. As the volume of and the distance covered by these transfers increases, so does the likelihood of adverse environmental impacts (Shiklomanov, 1999).
Donald Worster described the American West as a modern hydraulic society, characterized by the intensive, large-scale manipulation of water and its products in an arid environment (Worster, 1985). Most inter-basin water transfer projects in the US were initiated before the mid-1970s. Many studies have focused on evaluating the effectiveness of current or proposed inter-basin water transfer projects in meeting water demands (Bharati et al., 2008; Duan et al., 2023; Zhou et al., 2017) or assessing their economic benefits (Karamouz et al., 2010; Liu et al., 2023; Reza et al., 2013; Water Resources Group, 2010). Some of the earliest studies discussing the environmental and ecological impacts did not emerge until the mid-1980s and 1990s (Davies et al., 1992; Meader, 1992; Micklin, 1984; Petitjean and Davies, 1988; Snaddon et al., 1998). The construction of dams and irrigation systems resulted in severe impacts on the ecological integrity of river systems and the broader landscape across North America (Reisner, 1986). The bulk of the projects were built when there was no legal requirement to analyze and document environmental impacts of these projects, but some have been documented in the years since.
In the late 20th century, modern development approaches began to incorporate environmental protection and social prosperity as core pillars of water management strategies (Katirtzidou et al., 2023). However, it was not until the 1990s that studies examining the environmental impacts of these existing and proposed projects became common, and environmental impacts used as a criterion to decide whether such transfers are justified (Laassilia et al., 2021).
1.1 Environmental impacts of water transfers
Environmental impacts vary by the location of the water transfer scheme (Greer, 1983; Hagan and Roberts, 1972) and the scale of analysis (Choi et al., 2020; Cox, 1999; Snaddon et al., 1999). The location of the water transfer scheme includes exporting basins, routes of conveyance, and areas of delivery. The ‘donor’ river basin is where water is taken, the ‘recipient’ river basin where the water is delivered. The conveyance routes are usually a series of canals or pipelines that connect the donor and recipient river basins; in some cases, the diversion is driven by gravity, while others require pumping. The environmental impacts are different between donor basins and recipient basins (Zhuang, 2016). The donor basin has less water available and may experience salinization, aridification, and loss of habitats and livelihoods (Li et al., 2016), as well as significant impacts on the aquatic environment (Das et al., 2006; Herrmann, 1983). The recipient basin may experience increased water consumption or the spread of diseases (Bui et al., 2020). Recent studies have considered the efficiency of inter-basin transfers in alleviating inter-regional water stress, within the context of a changing climate and shifting water supply-demand dynamics (Duan et al., 2022; Maknoon et al., 2012; Zhang et al., 2016).
Although there was no requirement to analyze potential environmental impacts prior to construction of the massive water transfers from the Sacramento-San Joaquin Delta in California, those impacts have subsequently been documented. As described further below, the (federal) Central Valley Project diverts water from the Sacramento-San Joaquin Delta principally to irrigated agriculture in the San Joaquin Valley. The diversions were increased with implementation of the California State Water Project, which diverts water both to agriculture and urban areas in central and southern California. These diversions have reduced freshwater flows into the San Francisco Bay, resulting in a “freshwater-starved estuary” suffering from water quality degradation, seawater intrusion, and resultant loss of habitat and species (Bobker et al., 2016; Feng et al., 2017). These impacts were subject of litigation and hearings before the California Water Resources Control Board and continue to be controversial. As summarized by the Congressional Research Service, “Development of the Central Valley Project resulted in significant changes to the area’s natural hydrology. However, construction of most Central Valley Project facilities predated major federal natural resources and environmental protection laws. Much of the current debate related to the Central Valley Project revolves around how to deal with changes to the hydrologic system that were not significantly mitigated for when the project was constructed. Thus, multiple ongoing efforts to protect species and restore habitat have been authorized and are incorporated into project operations” (Stern and Sheikh, 2018).
One of the most visible sets of impacts is the direct and indirect mortality to fish at intakes to the pumps that lift water from the Delta into the canals, where the water then flows by gravity southward. The combined capacity of the state and federal pumps is 425 cubic meters per second, and during low river flows, the withdrawal of water at the pumps is so large that it creates a net upstream flow in the San Joaquin River, physically drawing fish (especially small fish, juveniles, and eggs) into the pumps. The flow reversal also confuses upstream and downstream migrating fish, and in effect, turns large areas of the Delta from suitable habitat into a lethal zone for fish (Bay Institute, 2012). The agencies running the pumping plant attempt to “salvage” fish by diverting fish away from the pumps before they are sucked in, and then trucking these fish to discharge points in the estuary downstream. However, of these “salvaged” fish, few survive the handling they suffer at the pumps and the injuries they sustain from predators in the canals leading to the pumps, and few avoid the predators that await them at the established discharge points in the estuary. And this toll does not include the uncounted numbers of fish devoured by the densely packed predators in the canals and forebay leading to the pumps. In some years, 40% of the population of the delta smelt (federally-listed as threatened and state listed as endangered species) and 15% of winter-run chinook salmon (federally and state listed as endangered) are killed at the pumps.
In addition, there are indirect effects, because the altered flow patterns divert out-migrating juveniles (such as salmon, steelhead, and sturgeon) from their natural migration pathways into less suitable habitats (Bay Institute, 2012). As these effects have been well documented and contentious, one attempt to reduce the problems was the California Water Fix and Eco-Restore project (described below). To mitigate for impacts of the Central Valley Project’s Trinity River diversion, the USBR initiated and has supported the Trinity River Restoration Program, which includes environmental flow releases that are significantly higher than the releases made strictly for project operations in the first years of the project operation, along with a range of physical modifications to habitat along the river corridor. Similarly, the USBR has supported the San Joaquin River Restoration Program and the CalFed Bay-Delta Ecosystem Restoration Program (Stern et al., 2020).
Another well-known California example of environmental impacts in the donor river basin from California is the drying of Mono and Owens Lakes in eastern California for diversion to Los Angeles via the Los Angeles Aqueduct. The original aqueduct simply took water from the Owens River, diverted south of Lone Pine. However, because of concerns that this supply would not be sufficient, the City of Los Angeles Department of Water and Power (LADWP) expanded its net northward, and by 1940 had secured rights to the waters of Rush and Lee Vining Creeks (tributaries to Mono Lake), which were diverted southward to the Owens River basin through a tunnel excavated under the Mono Craters. Total exports to Los Angeles increased in 1970, with completion of the Second Los Angeles Aqueduct.
The impacts of the original aqueduct included the drying out of Owens Lake, a terminal lake deprived of its inflow, within 11 years of the start of diversions. The dried out lakebed exposed salt flats, with easily eroded fine particles that were entrained in dust storms across the valley, with high concentrations of PM10 (particles finer than 10 microns) (Ashoori et al., 2015; Libecap, 2007). The expansion of the aqueduct northward into the Mono Lake basin had a similar effect on Mono Lake, also a saline, terminal lake. Mono lake is an important stop on the Pacific Coast Flyway, in part because of the abundant food source in the native brine shrimp that it supports. However, as tributary inflow to the lake was reduced (with no change in evapotranspiration rates) the level of the lake dropped about 12 m (from 1940 to 1980), and the volume of the lake decreased. As a result, the lake salinity increased, threatening to become too salty for brine shrimp and thus threatening the lake’s delicate ecosystem and the sustainability of an important stop-over on the Pacific Flyway. To protect the lake ecosystem, a group of environmental organizations (building on initial work of graduate students at the University of California) filed suit challenging the water exports based on their impact on the ecosystem. In “the California Supreme Court's famous decision in National Audubon Society v. Superior Court, 658 P.2d 709 (Cal. 1983), known more widely as the Mono Lake case,” the concept of public trust was applied to Mono Lake (Ryan, 2015). This was an innovative legal theory. “the decision spawned a quiet legal revolution in public trust ideals, which has redounded to other states and even nations as far distant as India” (Ryan, 2015). The immediate, practical result was that the City of Los Angeles was required to limit its diversions from the tributary streams until lake levels recovered and thereafter.
The Central Valley Project and California State Water Project, as well as the Los Angeles Aqueduct, illustrate the nature of some of the many impacts from large water diversions, impacts that in these cases were not explicitly considered when the projects were planned because they preceded passage of the National Environmental Policy Act (NEPA) and the California Environmental Quality Act (CEQA).
1.2 Evolution of water transfers in North America
While individual water transfer projects have been studied over the years, there has been no prior systematic study of the evolution of project planning and environmental impact analysis in the states. The generalizations made by Donald Worster hold true as we have documented the evolution of these projects over time, with environmental planning considerations becoming more important. In recent years, comprehensive datasets on inter-basin water transfers in North America have been compiled, providing valuable insights into the geographic distribution and characteristics of these transfers (Dobbs et al., 2023; Siddik et al., 2023). However, this study differs by focusing on the evolution of project planning from an environmental perspective, categorizing projects into different historical phases, and assessing their environmental and climate change implications in depth. Understanding this evolution is crucial for developing sustainable water management practices and mitigating adverse environmental impacts in future projects. Why aren't more projects like these being built? Although many inter-basin water transfer projects have been proposed, very few have been constructed since the 1970s. In this study, we review inter-basin water transfer projects in the US, examine the evolving role of environmental planning and assessments, and consider climate change vulnerability assessments for current and future transfers. We document 35 projects (currently operating, under construction, or proposed), review the available environmental information, identify gaps in environmental assessment, and the need for climate change vulnerability assessments for inter-basin water transfer projects.
2 Materials and methods
We first divided US water transfer projects into three distinct historical periods based on characteristics of the projects: 1900–1930, 1930–1970, and 1970–2020. This study only considered US water transfer projects at least 40 km in length and transporting at least 50 million cubic meters (MCM) per year. The secondary data for this study came from academic journal articles, reports of non-governmental organizations, information available in online newspapers. Primary sources included data obtained directly from relevant agencies (e.g., U.S. Bureau of Reclamation (Reclamation), U.S. Army Corps of Engineers (USACE)) and their official websites. For each project, we compiled data including donor and recipient basins, purpose, length, volume, costs, pumping requirements, project proponents, and for most projects, whether the system was gravity-driven or required pumping stations. We also documented construction period, distance over which water is transferred, average delivery, and construction cost. The construction period was the interval between the beginning year of construction to the beginning year of operation of the water transfer project, excluding extensions of new branches or water infrastructures after the first operation year. Reclamation is the principal agency responsible for many US water transfer projects. Reclamation maintains data on transfer projects in a consistent format, but some projects were built by state or municipal agencies, who provided data on their projects in various formats. The length of the water transfer scheme we used includes only the inter-basin transfer and excludes the distribution canals and ditches. Agencies commonly reported the annual deliveries in gallons per day or acre-feet per day, which we converted to annual values in SI units (International System of Units). Construction costs were reported in different years, so to facilitate comparison we converted all values to 2020 USD, adjusting for inflation following standard adjustments of the US Department of Labor Bureau of Labor Statistics. We present these data in an updated map of inter-basin water transfer projects in the US, tables and figures for built, incomplete, and proposed projects in chronological order. Incomplete projects refer to those currently under construction or in progress, which may still be awaiting final approvals, funding, or completion of certain phases of development. These data allowed us to document the evolution of these projects from an environmental planning perspective.
3 Results
We documented and mapped 35 projects, including 18 completed projects, five currently under construction, and 12 proposed projects that have been under consideration for years or are currently in various stages of development (Figure 1). Prior studies had mapped water transfers in 17 Western states as of 1968 (Quinn, 1968), with an update as of 1999 (Micklin, 1984; Snaddon et al., 1999).
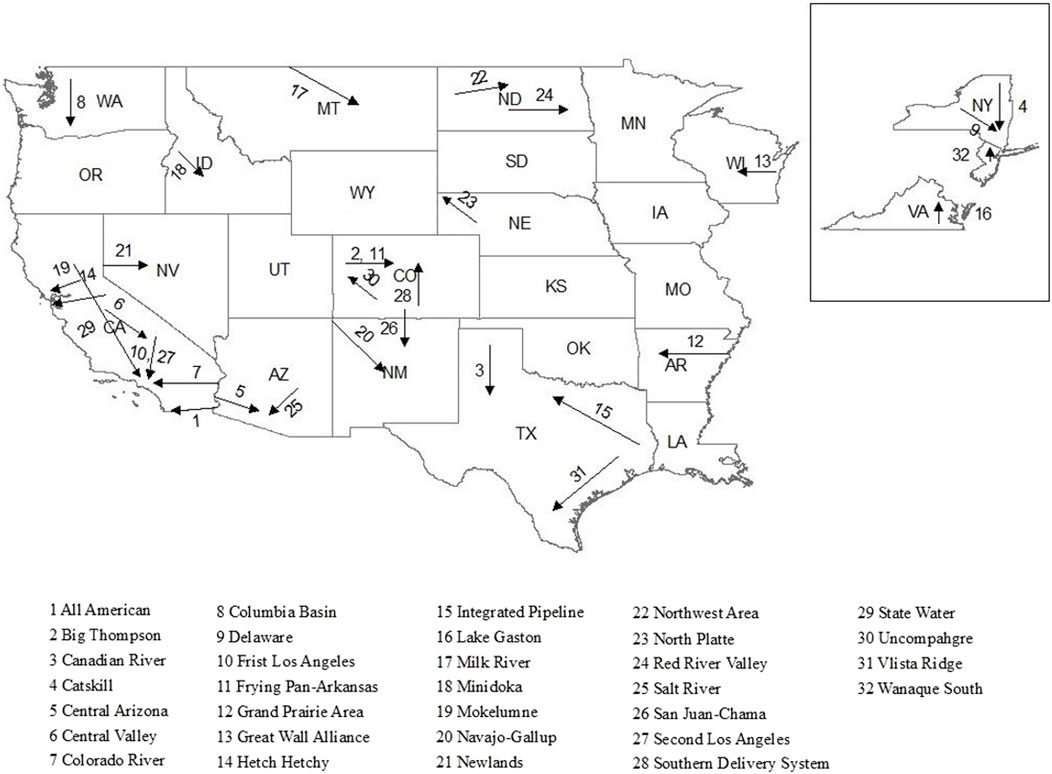
Figure 1. A map of 25 water transfer projects built and seven under construction (n = 32). Arrows indicate the direction of transfer and approximate location. See Tables 1, 2 for details and sources.
Table 1 shows the built water transfer projects and characteristics of 18 projects. Those built projects include the Newlands Project (Simonds, 1996), Milk River Project (Simonds, 1998), First Los Angeles Aqueduct (DWR, 1957), Hetch Hetchy Aqueduct (O’Shaughnessy, 1931), Mokelumne Aqueduct (DWR, 1957), Central Valley Project (Krug, 1949), Colorado River Aqueduct (MWDSC, 1950), All American Canal (IID, 2020), Big Thompson Project (Autobee, 1996), Delaware Aqueduct (Unrau, 1983), San Juan-Chama Project (Glaser, 2001), California State Water Project (DWR, 1957), Fryingpan-Arkansas Project (Rogers, 2006), Canadian River Project (Stene, 1995), Second Los Angeles Aqueduct (Socha, 1965), Central Arizona Project (Zuniga, 2000), Lake Gaston Water Supply Project (Cox, 2007), and Lake Texoma Water Supply Project (Burkhart, 2013).
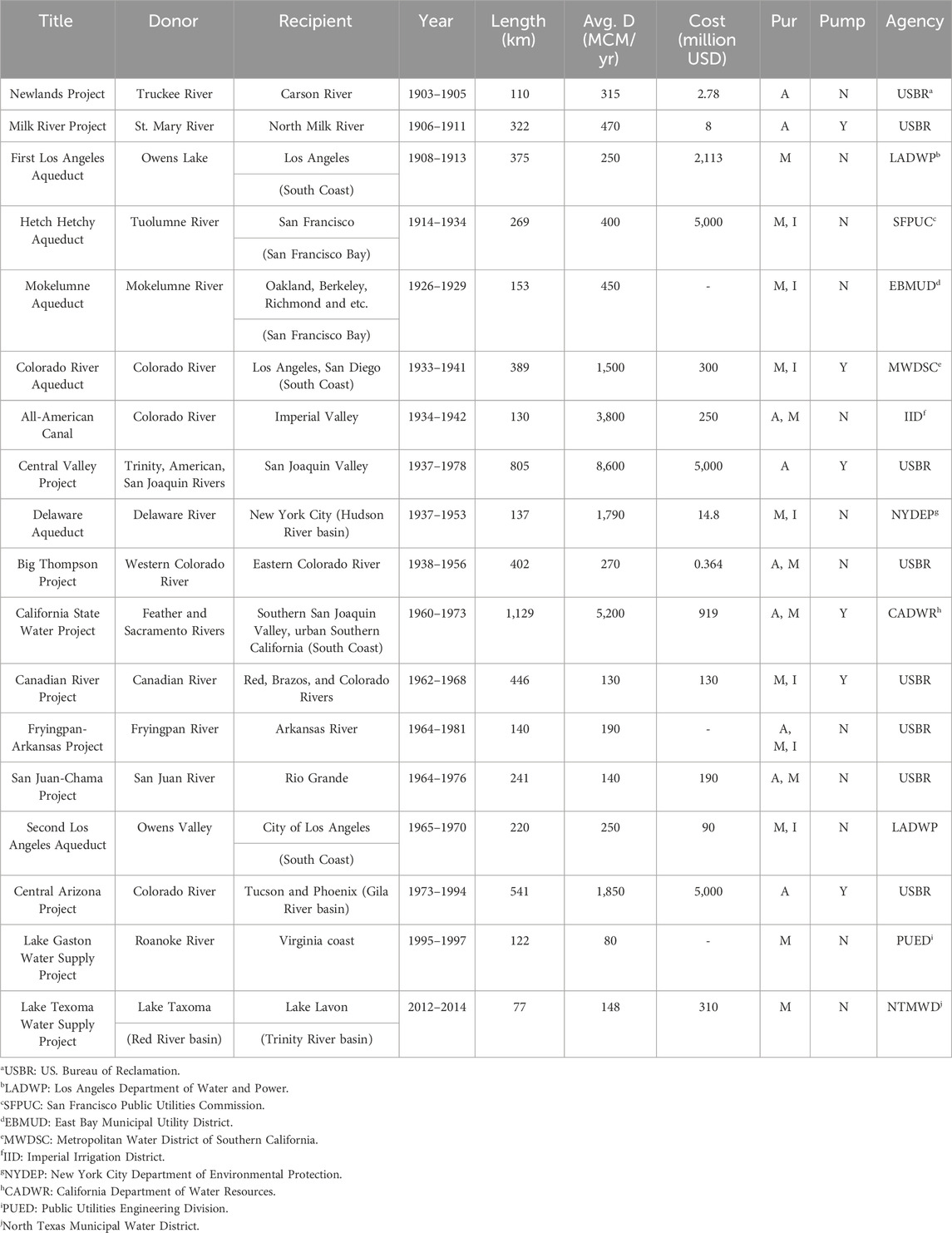
Table 1. Built water transfer projects and their main qualitative characteristics (n = 18). The length of the project is in km, the average delivery (Avg. D) is in million cubic meter per year (MCM/yr), and the cost is in million US dollars. The purpose (Pur) of the project is either agricultural (A), municipal (M), or industrial (I). The years refer to the period over which the project was under construction.
Table 2 shows incomplete water transfer projects and the main qualitative characteristics of five projects. Those incomplete projects include the Grand Prairie Area Demonstration Project (USACE, 1999), Northwest Area Water Supply Project (NDWC, 2019), Navajo-Gallup Water Supply Project (USBR, 2020), Integrated Pipeline Project (TRWD, 2013), and Red River Valley Water Supply Project (Manitoba Government, 2020).
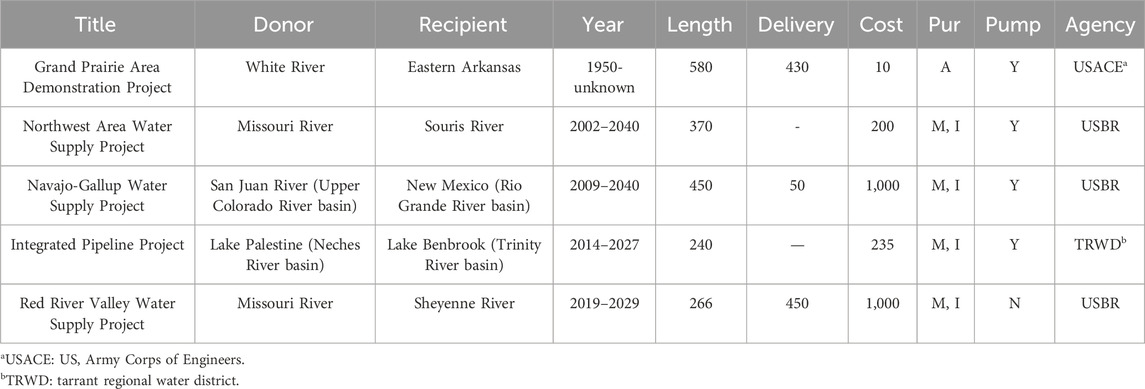
Table 2. Incomplete water transfer projects and their main qualitative characteristics (n = 5). The length of the project is in km, the average delivery (Delivery) is in million cubic meter per year, and the cost is in million US dollars. The purpose (Pur) of the project is either agricultural (A), municipal (M), or industrial (I). The years refer to the period over which the project was under construction.
Table 3 shows the once proposed water transfer projects and the main qualitative characteristics of 12 projects. Those once proposed water transfer projects include North American Water and Power Alliance (Micklin, 1984), Texas Water System (TWDB, 1968), California Undersea Aqueduct (Mccammon and Lee, 1966), Great Kansas Aqueduct (Shumilova et al., 2018), Alaska Subsea Pipeline (NDWC, 2019), Lake Powell Pipeline (WCWCD, 2019), Flaming Gorge Pipeline (Shumilova et al., 2018), Yampa River Pumpback Project (Shumilova et al., 2018), California Water Fix and Eco Restore Project (MWDSC, 2017), Missouri River Pipeline (Chawaga, 2019), Eastern Nevada to Las Vegas Pipeline (Donnelly, 2020), Seattle-California Pipeline (Byron and Wiley, 2015).
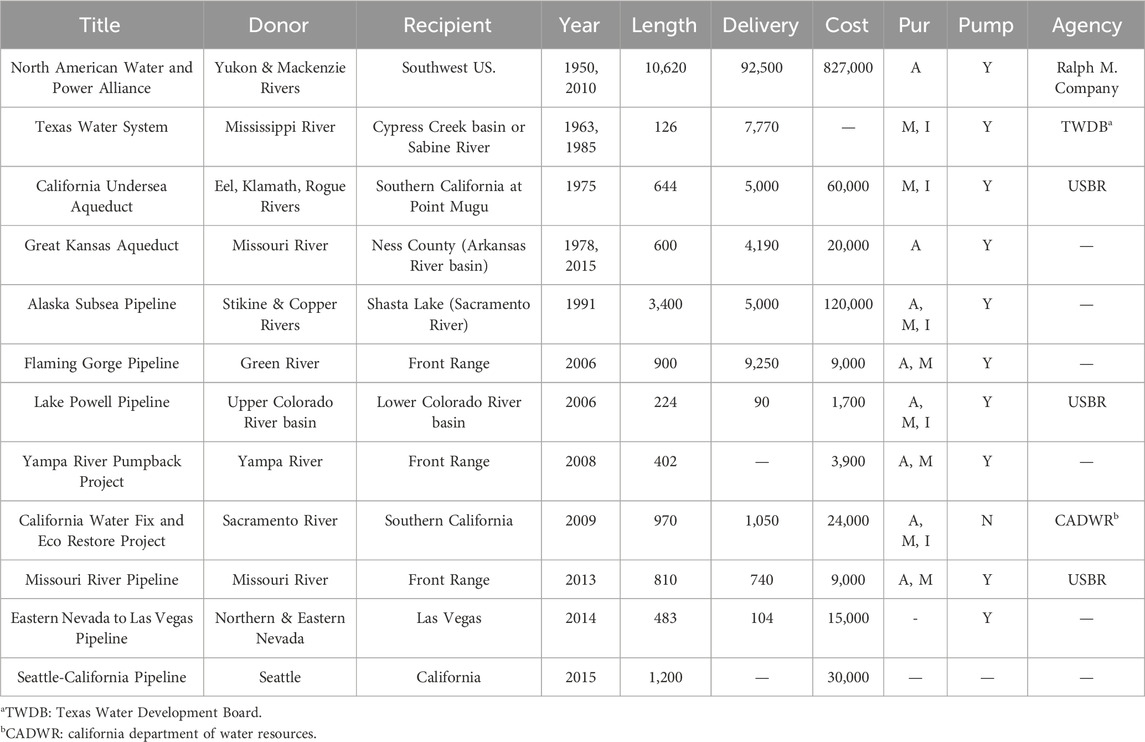
Table 3. Once proposed water transfer projects and their main qualitative characteristics (n = 12). The length of the project is in km, the average delivery (Delivery) is in million cubic meter per year, and the cost is in million US dollars. The purpose (Pur) of the project is either agricultural (A), municipal (M), or industrial (I). The years refer to the period over which the project was under construction.
3.1 Geographic distribution
The inter-basin water transfer projects are unevenly distributed, particularly between the Eastern and Western states (Table 1). Drier sections of the Western US rely heavily on inter-basin water transfers to meet growing demand. With an uneven water distribution, California is home to the greatest number of inter-basin water transfer projects, while Colorado is the largest water supplier to neighboring states. Most projects are intrastate rather than interstate (Figure 1). When Southern California cannot meet the growing water demand through local supplies, the water agencies start importing water from Northern California and the Colorado River Basin. The Colorado River Basin is a well-tapped export source for California (Colorado River Aqueduct and All-American Canal) and six other states. Proposed projects in California include collecting water from the Eel and Klamath Rivers (California Undersea Aqueduct), Sacramento River (California Water Fix and Eco Restore Project), and Seattle (Seattle-California Pipeline) to Southern California. Other proposed projects include sending water from the Green River, Yampa River, and Missouri River to the Front Range in Colorado; and the most comprehensive of plans, the North American Water and Power Alliance Project from Yukon and Mackenzie Rivers to Southwest US (Table 3). With the extensiveness of transfer project implementation, California and Colorado have been the most active in the construction, early adaptation, and use of these transfers. These transfers are not limited to the Western states. New York City has transferred municipal water from the Delaware River Basin for 8 decades (Delaware Aqueduct) (Table 1). Others are beginning to implement them for the first time, such as Virginia Beach, which brings water from the Roanoke River via the Lake Gaston Water Supply Project.
3.2 Purpose and travel distance
The early transfers (1900–1930) involved the first agricultural irrigation (Reclamation) projects, such as Newlands (Nevada) and Milk River (Montana), and fast-growing cities tapping water from distant rivers draining pristine basins, such as the Owens River diverted through the Los Angeles Aqueduct, the Tuolumne River carried by the Hetch Hetchy Aqueduct to San Francisco, and the Delaware River headwaters transported by the Delaware Aqueduct to New York City. The urban water supply projects were generally smaller in scale and less technically complex. However, by volume, the irrigation projects involved larger volumes but shorter distances (Figures 2A,B). The primary purpose for mid-20th Century (1930–1970) projects was irrigated agriculture, while industrial and municipal applications were growing. The Central Valley Project transformed the semi-arid desert environment of the San Joaquin Valley into productive farmland. The All-American Canal drew water from the Colorado River to boost agricultural production in the Imperial Valley. These projects became larger, longer, and more technically complex. The later transfers (1970–2020) provided water for municipal and industrial uses, unlike most of Reclamation’s efforts to solely enhance water supplies for irrigation. These projects were smaller in scale except for the Central Arizona Project. The incomplete Northwest Area Water Supply Project and Navajo-Gallup Water Supply Project aim to supply surface water for industrial and municipal uses, reducing pressure on groundwater. Proposed future projects would fetch water from long distances, like the North American Water and Power Alliance (10,620 km), the Alaska Subsea Pipeline (3,400 km), and the Seattle-California Pipeline (1,200 km) (Table 3). As local agencies exhausted local water supplies first as they were generally less expensive, drawing from more distant water sources may be difficult to avoid.
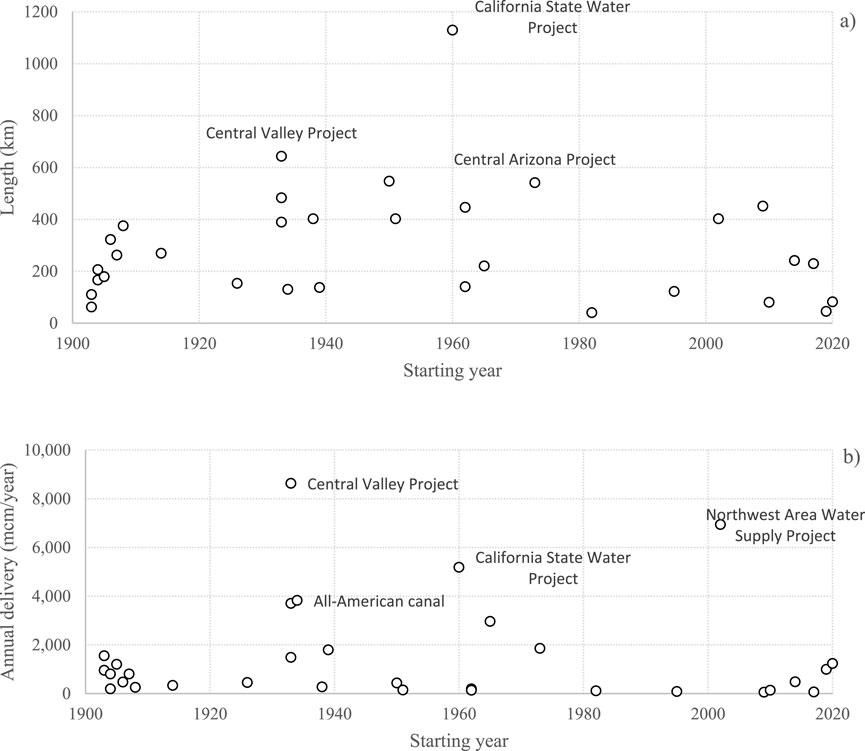
Figure 2. (A) A plot of the beginning construction year for existing projects against the length (m) (n = 32); (B) A plot of the beginning year of construction for existing projects against the annual delivery (mcm/year) (not all projects had data available on annual delivery) (n = 32).
3.3 Energy requirement
The early transfers (1900–1930) moved water by gravity alone, except for the Milk River Project, which required pumping units to irrigate lands above the gravity system (Table 1). More projects from the mid-20th Century (1930–1970) involved pumping stations as inter-basin water transfer projects became bigger and longer (Yi et al., 2022). Among the three transfers built between 1970–2020, the Central Arizona Project was the only project that involved pumping. Pumping water could be unavoidable when an alternative transfer route cannot overcome geographical conditions. The Great Kansas Aqueduct and the Grand Prairie Area Demonstration Project, due to geographical restrictions, proposed pumping systems, while the California Water Fix and Eco Restore Project and the California Undersea Aqueduct proposed running by gravity with minimal pumping (Table 3). However, unlike other under-construction projects that involved pumping, the Red River Valley Water Supply Project will run by gravity alone (Table 2). Despite efforts to utilize non-pumping transport, pumping is needed for many systems, but its energy consumption involves negative environmental impacts.
3.4 Agency, cost, and construction period
From 1900 until now, surveys by the United States Geological Survey (USGS) and the Reclamation have been the primary planning tools for identifying routes, the distance of pipelines, and the construction cost (Figure 3).
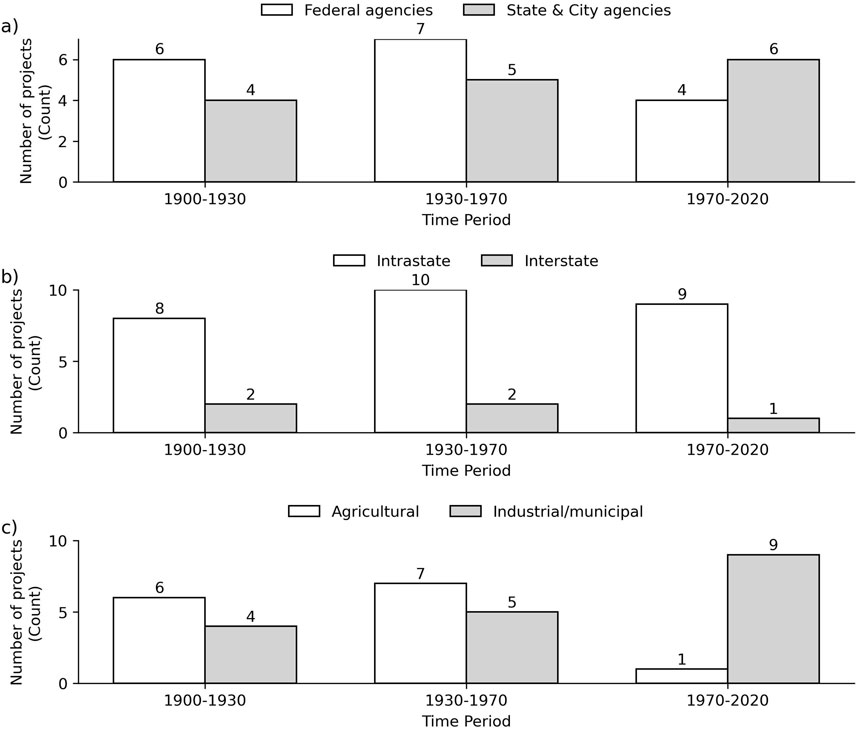
Figure 3. (A) A clustered column chart that plots three eras against the number of projects that are either funded by federal and, state or city (includes built and proposed but unbuilt projects) (n = 32); (B) A clustered column chart that plots three eras against the number of projects that are either interstate or intrastate projects (n = 32); (C) A clustered column chart that plots three eras against the primary water supply purpose (e.g., agricultural, industrial/municipal) (n = 32).
Under Reclamation Act of 1902, the US government funded irrigation projects (Newlands Project, Milk River Project) for the arid 20 Western states. The Depression slowed down government funding for new transfer projects, while Reclamation Act exceptionally funded the Central Valley Project in 1935. In the 1930s, transfers gradually involved government financing, facilitating larger transfers over greater distances. The Central Arizona Project, completed in 1994, was one of the last projects receiving Reclamation funding. As the federal cost share of large water projects decreased, higher local costs led local governments to reconsider the economic feasibility and environmental impacts of proposed projects. With less federal support, securing reliable funding for large projects became difficult, delaying construction of some projects for long periods. The Grand Prairie Area Demonstration Project encountered significant delays and was finally not completed due to a lack of local financial sponsors.
The construction period depends upon the route of the transfer scheme, geography, topography, distance, annual water delivery, agency, and funding. While a small-scale project (e.g., Newlands Project) took less than 3 years to build, grand-scale projects could continue for 20 years (e.g., Hetch Hetchy Aqueduct), while others could take at least 30 years to finish (e.g., Northwest Area Water Supply Project).
4 Discussion
As environmental concerns surrounding inter-basin water transfers have grown, technologies such as groundwater pumping, recycled water, and desalination have emerged as potential alternatives to meet water demand (Pittock et al., 2007; Quinn, 1968; Yi et al., 2024). However, it is unlikely that such alternatives can fully replace water transfer projects because they have costs and environmental impacts of their own, which must be weighed against the anticipated impacts of a water transfer scheme (Lasserre, 2005). Long-term strategies, such as water conservation and wastewater recycling, take considerable time to yield tangible results (Rinaudo and Barraqué, 2015). We see the negatives of water transfer projects preventing many currently proposed projects from being built today. Despite their drawbacks, inter-basin existing water transfers will likely continue to remain a significant component of US water management for decades to come. This broader context sets the stage for examining the historical evolution of inter-basin water transfer projects across different eras and their associated challenges.
4.1 1900–1930: pre-big government projects
Approval of new inter-basin water transfer projects was usually rapid unless the proposed project was technically or economically infeasible. A week after its official establishment in 1902, the Reclamation authorized the Milk River Project to divert water from the Saint Mary River. Projects built before the enactment of the National Environmental Policy Act (NEPA) were not required to conduct any environmental impact review or consider environmental impacts in their planning. However, substantial changes in the project features or operation after 1969 (e.g., lining canals, repairing features, adding new infrastructure) can trigger an environmental review. For example, in 1972, a State Court of Appeal ordered Los Angeles to file an environmental impact report for the First Los Angeles Aqueduct (completed in 1913). It took three attempts (1976, 1979, and 1990) to address the environmental impact of the aqueduct.
4.2 1930–1970: free-for-all project construction
The next-generation of water transfer projects was generally large, with complex technical problems and high implementation costs. Since the mid-1960s, the opposition to mass water transfers has increased significantly on environmental grounds in the US. Californians have started to recognize the damage to the Delta ecosystem and native fish by water diversions of the State Water Project (Castillo et al., 2012). The construction of the Central Valley Project dams on the Sacramento and San Joaquin Rivers reduced salmon populations (Fisher, 1994). The Canadian River Project encounters reduced inflow and storage in Lake Meredith, increasing the salinity of the Lake’s waters (Wurbs and Lee, 2011). Constructing the Second Los Angeles Aqueduct faced controversy from the beginning, as water diversions to Los Angeles completely dried up the Owens Valley (Kahrl, 1983).
Before any environmental assessment or statement was a requirement prior to the new inter-basin water transfer project, some of the initial hypotheses on negative environmental impacts were decreasing salmon runs, degrading scenic beauty (Quinn, 1968), changes in fish species and populations, destructing bird habitat (Thomas and Box, 1969), and altering river channels (LeCren, 1972; Stanford et al., 1979). Despite growing interest in this topic, there was still a lack of knowledge of monitoring systems, planning, and management (Davies et al., 1992). Conceptual and empirical work was undertaken (Snaddon et al., 1999), but fieldwork and pre- and post-environmental work are rarely available (Herrmann, 1983). After prolonged efforts spanning decades, considering the environmental impacts of inter-basin water transfer projects become a determining factor in the planning and operating stages.
4.3 1970–2020: post-national environmental policy act
The 1970s was when the management of the world’s water resources was undergoing a momentous shift (White, 1977). Since its enactment in 1969, NEPA has required environmental assessments before undertaking any major federal action that could impact the environment, ensuring that potential environmental effects are considered (Karkkainen, 2002). The NEPA process includes Environmental Assessments (EA) and more detailed Environmental Impact Statements (EIS), which identify significant effects, explore alternatives, and involve public participation (Friesema, H. P., & Culhane, 1976). While these regulations were initially limited in their impact on large inter-basin transfer plans, they gradually influenced the approach to water infrastructure development. The requirement to provide mitigation documentation in environmental statements facilitated project approvals, though the increasing complexity of NEPA regulations led to extended review times. For instance, the Central Arizona Project’s first environmental statement took 2 years, while more complex projects, like the Red River Water Supply Project and Navajo-Gallup Water Supply Project, took eight to 9 years for EIS and EIA approvals.
In some cases, the environmental review led to major changes in design infrastructure or significantly scaled down the proposed projects. The California Water Fix and Eco Restore Project first proposed two large tunnels to convey water south along the eastern margin of the Sacramento Delta. This proposal was withdrawn and scaled down to the Single Delta Tunnel Project mainly due to environmental criticism. Future projects now require long periods of thorough study, comprehensive planning, and careful design, with assessments detailing studies of all transfer aspects, including environmental impacts (Yevjevich, 2001). The environmental evaluation results can be a major driving factor in decision making, even overriding political, social, or economic considerations (Thomas and Box, 1969).
As the design structure and the EIS requirement became strict, documentation compliance slowed plan approvals and construction processes. The US Bureau of Land Management dismissed the Yampa River Pump Back Project and focused on a planning framework that prioritized environmental needs. After 6 years of investigation, the US government also dismissed the Flaming Gorge Pipeline as it could potentially harm the Green River and the Colorado River downstream. The water agencies shelved Eastern Nevada to Las Vegas Pipeline Plan after 30 years of environmental investigation. The assessment concluded that this project could potentially dry springs, harm wildlife habitats, and alter ecosystems.
As the USGS had to prepare and submit a required EIS, they sought the help of Luna Leopold to create a preliminary environmental consideration framework for any water infrastructure projects (Luna et al., 1971). In his framework, Leopold used the California State Water Project to illustrate potential types of environmental impacts, but it was too broad as the individual transfer is unique. Apart from the framework devised by Luna Leopold, Asit K. Biswas was part of a task force to create another framework for compiling an EIA (Biswas, 1979). Though general, this guideline was meaningful, designed solely for water transfers, while Leopold’s applied to all types of water infrastructure projects. Later, Biswas and Golubev formulated a framework to focus on a project’s environmental impact at the proposal stage. The previous guidelines were based on EIA on a proposed project’s environmental effects after completion (Golubev and Biswas, 1984). A series of journal articles used these frameworks to assess the environmental impacts of transfers (Pazoki et al., 2015; Zhuang, 2016).
4.4 Post-2020: climate change
The government has been mandating EIS for the US inter-basin water transfer projects for almost half a Century. While fewer new proposals are being suggested, old projects, once proposed and rejected, are coming back to the table (Gleick et al., 2014). These proposals are outdated and need revision by counting in the EIA, especially those proposed before the 1970s. Many of these projects are technically feasible; however, they may cause environmental damages and may not be sustainable in the rapidly changing climate. Climate change is the next critical component to extend and sustain the life of century-old inter-basin water transfer projects (Yan et al., 2012; Yi and Yi, 2024). Over most of the continental US, both annual precipitation and the frequency of extreme events (e.g., floods, droughts, and melting glaciers) are gradually increasing. This may diminish water flows in many rivers and make water supplied by transfer projects unreliable. This suggests that new inter-basin water transfer projects should be implemented only after comprehensive environmental impact and climate change vulnerability assessments. The climate change vulnerability assessment for the inter-basin water transfer project is an initial step in the adaptation planning process that identifies and addresses the climate change impacts on the recipient basin, donor basin, routes of conveyance, and infrastructure.
Not many cases in the US were adopting any form of a comprehensive climate change vulnerability assessment during the planning stages, and in many instances, prior to construction. It was only recently that the changing climate impacts of inter-basin water transfers have become apparent. In the post-2000s, publications highlighted the importance of assessing changing climate conditions of water transfers to sustain water supplies and freshwater ecosystems (Pittock et al., 2007). Studies have started to acknowledge that climate change was a factor that influenced water availability in the donor and recipient basins. Numerous studies have developed a vulnerability assessment framework for identifying water transfer strategies to mitigate the potential climate change impact on the operation of a water transfer project (Zhang et al., 2014). Other studies took it further by illustrating the framework and modeling the effects of climate change on water quantity (Maknoon et al., 2012) and biodiversity (Pittock and Finlayson, 2011) in the river basin with different climate scenarios. The impact of climate change on inter-basin water transfers slowly gained rising significance. The withdrawn California Water Fix and Eco Restore Project recognized climate change as one of the possible threats to the water system. A few more projects mentioned climate change in their initial planning framework, which did not adequately consider the impacts of climate change, but this topic is becoming progressively significant.
To sufficiently address the climate change impact on current and future projects, Federal law could require climate change vulnerability assessments as part of the planning process. The traditional planning frameworks are poorly equipped to address climate effects on water transfer projects. The US Department of Agriculture provides a climate change vulnerability assessment that lays out critical features for the natural environment, including water resources (Joyce and Janowiak, 2011). However, no specific climate change framework is dedicated to water transfer projects. The climate change vulnerability assessment designated for the inter-basin water transfer project should comprehensively address the effect of climate change on the water supply and demand system, recipient basin, donor basin, and conveyance routes. Given the importance of proactively managing these risks, it would be advisable for Congress to consider mandating such assessments during the initial review process to ensure that climate change impacts are adequately addressed for both aging and proposed projects.
5 Conclusion
This study provided a comprehensive inventory of inter-basin water transfer projects (built, incomplete, proposed) in the US, identified patterns of these projects’ characteristics, analyzed the growing role of environmental planning in these projects, and drew lessons to inform future proposals. The key findings were that early projects, mostly gravity-driven and smaller-in-scale, grew in scale and required more pumping stations (energy-intensive) to lift the water over high elevations. California and Colorado were the most active in using these transfers, while other states have begun to implement them. Federal agencies stepped back from funding most transfers due to the severity of impacts documented through the EIS process. The environmental impacts have become a primary factor determining whether inter-basin water transfer projects are now implemented, and if built, how they are operated. We suggest that the climate change vulnerability assessment become the next determinant for future proposals.
This study is limited to assessing the projects in the US. Data availability is another limitation. Information is unavailable for all data elements for some transfers. Some data formerly available online was taken down during our study, such as data for the Southern Delivery System project of Colorado Springs Utilities, whose webpage was retired upon completion of project construction. The California Water Fix and Eco Restore Project webpage was taken down at the end of 2019, when the proposed project was withdrawn, and the state regrouped to develop a new proposal.
Pressures will continue to transport water from river basins where the water is relatively less economically valuable to expanding urban areas or irrigated agriculture areas, reflecting political and economic power imbalances between donor and recipient basins. Even with the inclusion of new data, the fundamental drivers of water transfers—economic valuation and power dynamics—are likely to persist. However, additional data could help refine the understanding of specific local conditions, variations in power dynamics, and emerging trends in inter-basin water transfers. Unless less-impactful alternatives can be shown to economically meet the water demands of the recipient basin, it is likely that pressure for water transfers will persist. The next step should be integrating climate change issues and examining the problem in its present form. Climate change introduces uncertainty in the future viability of projects by affecting water availability in donor basins and water demand in recipient basins, thus exacerbating environmental impacts and growing the risk posed by such projects. However, just as environmental documents were prepared only after legislation required them, we recommend that climate change vulnerability assessments be required for future water projects, including transfer schemes. We regard it as imperative that a formal climate change vulnerability assessment and research infrastructure be drawn up nationally, as a matter of priority, to minimize the climate change impact of future schemes. We suggest that specific climate change regulations and guidelines are needed in each step of the EIA process. Working toward implementing climate change vulnerability assessments and guidance appear to be an essential first step for the next-generation of inter-basin water transfers.
Data availability statement
The original contributions presented in the study are included in the article/supplementary material, further inquiries can be directed to the corresponding author.
Author contributions
SY: Conceptualization, Data curation, Formal Analysis, Methodology, Resources, Validation, Visualization, Writing–original draft, Writing–review and editing. GMK: Conceptualization, Project administration, Resources, Supervision, Writing–original draft, Writing–review and editing.
Funding
The author(s) declare financial support was received for the research, authorship, and/or publication of this article. Publication made possible in part by support from the Berkeley Research Impact Initiative (BRII) sponsored by the UC Berkeley Library.
Conflict of interest
The authors declare that the research was conducted in the absence of any commercial or financial relationships that could be construed as a potential conflict of interest.
Publisher’s note
All claims expressed in this article are solely those of the authors and do not necessarily represent those of their affiliated organizations, or those of the publisher, the editors and the reviewers. Any product that may be evaluated in this article, or claim that may be made by its manufacturer, is not guaranteed or endorsed by the publisher.
References
Ashoori, N., Dzombak, D. A., and Small, M. J. (2015). Sustainability review of water-supply options in the Los Angeles region. J. Water Resour. Plan. Manag. 141 (12), A4015005. doi:10.1061/(asce)wr.1943-5452.0000541
Autobee, R. (1996). Colorado-Big Thompson Project. Available at: https://www.usbr.gov/projects/pdf.php?id=97.
Bharati, L., Anand, B. K., and Smakhtin, V. (2008). Analysis of the Inter-basin water transfer scheme in India: a case study of the godavari – krishna link. Strategic Analyses Natl. River Link. Proj. (NRLP) India Ser. 2, Proc. Workshop Analyses Hydrological, Soc. Ecol. Issues NRLP, 63–78. Available at: http://ageconsearch.umn.edu/bitstream/118387/2/H041793.pdf.
Biswas, A. K. (1979). “North American water transfers: an overview,” in Interregional water transfers: problems and prospects. Editors G. N. Golubev, and A. K. Biswas (Great Britan, United Kingdom: Pergamon Press), 79–90.
Biswas, A. K. (1983). Long-distance water transfer: a Chinese case study and international experiences. Editors A. K. Biswas, J. E. Nickum, L. Changming, and D. Zuo (Dublin, Ireland: United Nations University Press).
Bobker, G., Weber-Stover, A., Rosenfield, J., Reis, G., and Vorster, P. (2016). San Francisco Bay: the freshwater – starved estuary. doi:10.13140/RG.2.2.30680.70408
Bui, D. T., Asl, D. T., Ghanavati, E., Al-Ansari, N., Khezri, S., Chapi, K., et al. (2020). Effects of inter-basinwater transfer on water flow condition of destination basin. Sustain. Switz. 12 (1), 338. doi:10.3390/SU12010338
Burkhart, D. (2013). Feature project: lake taxoma pipeline. Available at: https://www.garney.com/wp/wp-content/uploads/2014/01/Winter-2013.pdf.
Byron, L., and Wiley, E. (2015). William Shatner’s fix for Cali. drought? A pipeline to Seattle. USA Today. Available at: https://www.google.com/amp/s/amp.usatoday.com/amp/26111213.
Castillo, G., Morinaka, J., Lindberg, J., Baskerville-Bridges, B., and Hobbs, J. (2012). Pre-screen loss and fish facility efficiency for delta smelt at the South Deltas State Water Project, California. San Franc. Estuary Watershed Sci. 10 (Issue 4). doi:10.15447/sfews.2012v10iss4art4
Chawaga, P. (2019). Initial funding for billion-dollar Missouri River Pipeline secured. Water Online. Available at: https://www.wateronline.com/doc/initial-funding-for-missouri-river-pipeline-secured-0001.
Choi, Y., Ahn, J., Ji, J., Lee, E., and Yi, J. (2020). Effects of inter-basin water transfer project operation for emergency water supply. Water Resour. Manag. 34 (8), 2535–2548. doi:10.1007/s11269-020-02574-9
Cox, W. E. (1999). “Interbasin water transfer,” in Proceedings of the international workshop. Paris: UNESCO, 173–178. 25-27 April 1999.
Cox, W. E. (2007). North Carolina - Virginia conflict: the Lake Gaston water transfer. J. Water Resour. Plan. Manag. 133 (5), 456–461. doi:10.1061/(ASCE)0733-9496(2007)133:5(456)
Das, D. K., Otani, H., and Maulik, N. (2006). Environmental impact of inter-basin water transfer projects: some evidence from Canada. J. Mol. Cell. Cardiol. 41 (Issue 17), 248–255. doi:10.1016/j.yjmcc.2006.03.009
Davies, B. R., Thoms, M., and Meador, M. (1992). An assessment of the ecological impacts of inter-basin water transfers, and their threats to river basin integrity and conservation. Aquatic Conservation Mar. Freshw. Ecosyst. 2 (4), 325–349. doi:10.1002/aqc.3270020404
Dobbs, G. R., Liu, N., Caldwell, P. V., Miniat, C. F., Sun, G., Duan, K., et al. (2023). Inter-basin surface water transfers database for public water supplies in conterminous United States, 1986–2015. Sci. Data 10 (1), 255. doi:10.1038/s41597-023-02148-5
Donnelly, P. (2020). Nevada water officials to abandon appeal of massive Las Vegas Pipeline Project. Center of Biological Diversity. Available at: https://biologicaldiversity.org/w/news/press-releases/nevada-water-officials-abandon-appeal-massive-las-vegas-pipeline-project-2020-04-16/.
Duan, K., Caldwell, P. V., Sun, G., McNulty, S. G., Qin, Y., Chen, X., et al. (2022). Climate change challenges efficiency of inter-basin water transfers in alleviating water stress. Environ. Res. Lett. 17 (4), 044050. doi:10.1088/1748-9326/ac5e68
Duan, K., Qu, S., Liu, N., Dobbs, G. R., Caldwell, P. V., and Sun, G. (2023). Evolving efficiency of inter-basin water transfers in regional water stress alleviation. Resour. Conservation Recycl. 191, 106878. doi:10.1016/j.resconrec.2023.106878
DWR (1957). The California water plan. State Calif. Dep. Water Resour. Div. Resour. Plan. Available at: https://cawaterlibrary.net/document/bulletin-no-3-the-california-water-plan/.
Feng, T., Wang, C., Hou, J., Wang, P., Liu, Y., Dai, Q., et al. (2017). Effect of inter-basin water transfer on water quality in an urban lake: a combined water quality index algorithm and biophysical modelling approach. Ecol. Indic. 1, 61–71. doi:10.1016/j.ecolind.2017.06.044
Fisher, F. W. (1994). “Past and present status of Central Valley chinook salmon,”. Editors L. Bergere Leopold, F. Eldridge Clarke, B. B. Hanshaw, and J. R. Balsley, 8, 870–873. doi:10.1046/j.1523-1739.1994.08030863-5.xConserv. Biol.3
Friesema, H. P., and Culhane, P. J. (1976). Social impacts, politics, and the environmental impact statement process. Nat. Resour. J. 16 (2), 339–356.
Glaser, L. S. (2001). San juan-Chama transfer. Available at: https://www.usbr.gov/projects/pdf.php?id=186.
Gleick, P., Heberger, M., and Donnely, K. (2014). “Zombie water projects,” in The world’s water volume 8: the biennial report on freshwater resources. Editor P. Gleick, 123–146.
Golubev, G. N., and Biswas, A. K. (1979). “Interregional water transfers: problems and prospects (Pergamon Press), 59–65.
Golubev, G. N., and Biswas, A. K. (1984). Large-scale water transfers: emerging environmental and social issues. Int. J. Water Resour. Dev. 2 (2–3), 1–5. doi:10.1080/07900628408722312
Greer, C. (1983). “The Texas water system: implications for environmental assessment in palnningfor interbasin water transfers,” in Long-distance water transfer: a Chinese case study and international experiences. Editors A. K. Biswas, Z. Dakang, J. E. Nickum, and L. Changming (Dublin, Ireland: United Nations University Press).
Hagan, R. M., and Roberts, E. B. (1972). Ecological impacts of water projects in California. J. Irrigation Drainage Div. 98 (1), 25–48. doi:10.1061/JRCEA4.0000856
Herrmann, R. (1983). “Environmental implications of water transfer,” in Long-distance water transfer: a Chinese case study and international experiences. Editors A. K. Biswas, Z. Dakang, J. E. Nickum, and L. Changming (Dublin, Ireland: United Nations University).
IID (2020). All-American canal. Imp. Irrig. Dist. Available at: https://www.iid.com/water/water-transportation-system/colorado-river-facilities/all-american-canal.
Joyce, L. A., and Janowiak, M. K. (2011). Climate change assessments. U.S. Available at: www.fs.usda.gov/ccrc/topics/impact-assessments.
Kahrl, W. L. (1983). Water and power: the conflict over Los Angeles water supply in the Owens Valley. University of California Press.
Karamouz, M., Mojahedi, S. A., and Ahmadi, A. (2010). Interbasin water transfer: economic water quality-based model. J. Irrigation Drainage Eng. 136 (2), 90–98. doi:10.1061/(ASCE)IR.1943-4774.0000140
Karkkainen, B. C. (2002). Toward a smarter NEPA: monitoring and managing government’s environmental performance. Columbia Law Rev. 102 (4), 903. doi:10.2307/1123648
Katirtzidou, M., Skoulikaris, C., Makris, C., Baltikas, V., Latinopoulos, D., and Krestenitis, Y. (2023). Modeling stakeholders’ perceptions in participatory multi-risk assessment on a deltaic environment under climate change conditions. Environ. Model. and Assess. 28 (3), 367–388. doi:10.1007/s10666-023-09890-5
Krug, J. A. (1949). Central Valley Basin: a comprehensive report on the development of the water and related resources of the Central Valley Basin for irrigation, power production and other beneficial uses in California. 1st ed. Department of the Interior.
Laassilia, O., Ouazar, D., Bouziane, A., and Hasnaoui, M. D. (2021). Justification criteria for Inter-Basin water transfer projects. E3S Web Conf. 314 (06001), 06001–06006. doi:10.1051/e3sconf/202131406001
Lasserre, F. (2005). Massive water diversion schemes in North America: a solution to water scarcity. Water Resour. Manag. 80 (10), 385–394. doi:10.2495/WRM050391
LeCren, E. D. (1972). “A commentary on uses of a river: past and present,” in River ecology and man. Editors J. A. M. R. T. Oglesby, and C. A. Carlson (American Press), 251–260.
Li, Y., Xiong, W., Zhang, W., Wang, C., and Wang, P. (2016). Life cycle assessment of water supply alternatives in water-receiving areas of the South-to-North Water Diversion Project in China. Water Res. 89, 9–19. doi:10.1016/j.watres.2015.11.030
Libecap, G. D. (2007). Owens valley revisited: a reassessment of the west’s first great water transfer. Stanford University Press.
Liu, Y., Xin, Z., Sun, S., Zhang, C., and Fu, G. (2023). Assessing environmental, economic, and social impacts of inter-basin water transfer in China. J. Hydrology 625, 130008. doi:10.1016/j.jhydrol.2023.130008
Luna, B. L., Clarke, F. E., Hanshaw, B. B., and Balsley, J. R. (1971). Procedure for evaluating environmental impact. USGS. doi:10.3133/cir645
Maknoon, R., Kazem, M., and Hasanzadeh, M. (2012). Inter-basin water transfer projects and climate change: the role of allocation protocols in economic efficiency of the project. Case study: dez to Qomrood inter-basin water transmission project (Iran). J. Water Ressources Prot., 4, 750–758. doi:10.4236/jwarp.2012.49085
Manitoba Government (2020). “Red River Valley Water supply project,” in Potential transboundary water projects. Available at: https://www.gov.mb.ca/sd/waterstewardship/water_info/transboundary/potential.html#a4.
Mccammon, L. B., and Lee, F. C. (1966). Undersea aqueduct system. J. Am. Water Works Assoc. 58 (7), 885–892. doi:10.1002/j.1551-8833.1966.tb01650.x
Meader, M. R. (1992). Inter-basin water transfer: ecological concerns. Fisheries 17 (2), 17–22. doi:10.1577/1548-8446(1992)017<0017:iwtec>2.0.co;2
Micklin, P. P. (1984). Inter-basin water transfers in the United States. Int. J. Water Resour. Dev. 2 (2–3), 37–65. doi:10.1080/07900628408722314
MWDSC (2017). California Waterfix physical infrastructure. Available at: http://www.californiawaterfix.com.
NDWC. (2019). North Dakota’s Northwest area water supply project. Office of technology assessment (1992). Alaska water for California? The Subsea Pipeline option.
O’Shaughnessy, M. M. (1931). The Hetch Hetchy water supply of the city of san Francisco. J. Am. Water Works Assoc. 9 (5), 743–765. doi:10.1002/j.1551-8833.1922.tb12416.x
Pazoki, M., Ahmadi, P. M., Dalaei, P., and Ghasemzadeh, R. (2015). Environmental impact assessment of a water transfer project. Jundishapur J. Health Sci. 7 (3), 9–14. doi:10.17795/jjhs-27238
Petitjean, M. O. G., and Davies, B. R. (1988). Ecological impacts of inter-basin water transfers: some case studies, research requirements and assessment procedures in Southern Africa. South Afr. J. Sci. 84 (10), 819–828.
Pittock, J., and Finlayson, C. M. (2011). Australia’s MurrayDarling Basin: freshwater ecosystem conservation options in an era of climate change. Mar. Freshw. Res. 62 (3), 232–243. doi:10.1071/MF09319
Pittock, J., Meng, J., and Geiger, M. (2007). Pipedream? Interbasin water transfers and water shortages. Available at: https://www.wwf.or.jp/activities/lib/pdf_freshwater/freshwater/pipedreams_27_june_2007_1.pdf.
Quinn, F. (1968). Water transfers: must the American west Be won again? Geogr. Rev. 58 (1), 108. doi:10.2307/212834
Reza, H., Manshadi, D., Niksokhan, M. H., and Ardestani, M. (2013). Water allocation in inter basin water transfer with the virtual water approach, 2510–2521.
Rinaudo, J., and Barraqué, B. (2015). Inter-basin transfers as a supply option: the end of an era? Underst. Manag. Urban Water Transition 15, 175–200. doi:10.1007/978-94-017-9801-3_8
Rogers, J. S. (2006). Fryingpan-Arkansas project. Available at: https://www.usbr.gov/projects/pdf.php?id=120.
Ryan, E. (2015). Public Trust Doctrine, Private Water Allocation, Mono Lake Hist. Saga Natl. Audubon Soc. v. Super. Court. Available at: https://ir.law.fsu.edu/articles/610.
Shiklomanov, I. A., (1999). World Water Resources: modern assessment and outlook for the 21st century. St Petersburg: Federal Service of Russia for Hydrometeorology and Environment Monitoring, State Hydrological Institute UNCSD
Shumilova, O., Tockner, K., Thieme, M., Koska, A., and Zarfl, C. (2018). Global water transfer megaprojects: a potential solution for the water-food-energy nexus? Front. Environ. Sci. 6. doi:10.3389/fenvs.2018.00150
Siddik, M. A. B., Dickson, K. E., Rising, J., Ruddell, B. L., and Marston, L. T. (2023). Interbasin water transfers in the United States and Canada. Sci. Data 10 (1), 27. doi:10.1038/s41597-023-01935-4
Simonds, W. J. (1996). The Newlands project. Available at: https://www.usbr.gov/projects/pdf.php?id=142.
Simonds, W. J. (1998). Milk River project. Available at: https://www.usbr.gov/projects/pdf.php?id=136.
Snaddon, C. D., Davies, B. R., and Wishart, M. J. (1999). A global overview of inter-basin water transfer schemes, with an appraisal of their ecological, socio-economic and socio-political implications, and recommendations for their management.
Snaddon, C. D., Wishart, M. J., and Davies, B. R. (1998). Some implications of inter-basin water transfers for river ecosystem functioning and water resources management in southern africa. Aquatic Ecosyst. Health Manag. 1 (2), 159–182. doi:10.1080/14634989808656912
Socha, M. K. (1965). Construction of the second Los Angeles aqueduct. Journal of American Water Works Association.
Stanford, J. A., and J, V. W. (1979). “Stream regulation in North America,” in The ecology of regulated streams. Editor J. A. Stanford (Plenum Press), 215–236.
Stene, E. A. (1995). Canadian River Project. Available at: https://www.usbr.gov/projects/pdf.php?id=92
Stern, C. V., Sheikh, P. A., and Ward, E. H. (2020). Central Valley Project: issues and legislation.
Thomas, G. W., and Box, T. W. (1969). Social and ecological implications of water importation into arid lands. Arid Lands Perspective, 363–374.
Tockner, K., Bernhardt, E. S., Koska, A., and Zarfl, C. (2016). “A global view on future major water engineering projects,” in Society-water-technology. Water resources development and management. Editors R. Hüttl, O. Bens, and C. Bismuth (Cham: Springer). doi:10.13140/RG.2.1.4442.0887
TRWD (2013). Tarrant regional water district integrated water supply plan. Available at: https://www.trwd.com/wp-content/uploads/2019/04/Integrated-Water-Supply-Plan-1.pdf.
TWDB (1968). The Texas water plan. Available at: http://www.twdb.texas.gov/waterplanning/swp/1968/index.asp.
Unrau, H. D. (1983). Historic structure report, historical data section: the Delaware Aqueduct. New York, Pennsylvania: University of Michigan Library.
USACE (1999). Grand Prairie area demonstration project: environmental impact statement. U.S. Army Corps of Engineers.
USBR (2020). Navajo-gallup water supply project. U.S. Department of the Interior Bureau of Reclamation. Available at: https://www.usbr.gov/projects/index.php?id=580.
Water Resources Group (2010). Charting Our Water Future: Economic Frameworks to Inform Decision-Making. 2030 Water Resources Group.
WCWCD (2019). What is the Lake Powell pipeline? Washington county water conservancy district. Available at: https://lpputah.org/what-is-the-lake-powell-pipeline/.
White, G. F. (1977). Environmental effects of complex river development. Boulder, Colorado: Westview Press.
Worster, D. (1985). Rivers of Empire: water, aridity and the growth of the American West. Oxford University Press.
Wurbs, R. A., and Lee, C. (2011). Incorporation of salinity in water availability modeling. J. Hydrology 409 (1–2), 451–459. doi:10.1016/j.jhydrol.2011.08.042
Yan, D. H., Wang, H., Li, H. H., Wang, G., Qin, T. L., Wang, D. Y., et al. (2012). Quantitative analysis on the environmental impact of large-scale water transfer project on water resource area in a changing environment. Hydrology Earth Syst. Sci. 16 (8), 2685–2702. doi:10.5194/hess-16-2685-2012
Yevjevich, V. (2001). Water diversions and interbasin transfers. Water Int. 26 (3), 342–348. doi:10.1080/02508060108686926
Yi, S., Kondolf, G. M., Sandoval-Solis, S., and Dale, L. (2022). Application of machine learning-based energy use forecasting for Inter-basin water transfer project. Water Resour. Manag. 36 (14), 5675–5694. doi:10.1007/s11269-022-03326-7
Yi, S., Kondolf, G. M., Sandoval Solis, S., and Dale, L. (2024). Groundwater level forecasting using machine learning: a case study of the baekje weir in four major rivers project, South Korea. Water Resour. Res. 60 (5). doi:10.1029/2022WR032779
Yi, S., and Yi, J. (2024). Reservoir-based flood forecasting and warning: deep learning versus machine learning. Appl. Water Sci. 14 (11), 237. doi:10.1007/s13201-024-02298-w
Zhang, C., Zhu, X., Fu, G., Zhou, H., and Wang, H. (2014). The impacts of climate change on water diversion strategies for a water deficit reservoir. J. Hydroinformatics 16 (4), 872–889. doi:10.2166/hydro.2013.053
Zhang, E., Yin, X., Xu, Z., and Yang, Z. (2016). Bottom-up quantification of inter-basin water transfer vulnerability to climate change. Ecol. Indic. 92, 195, 206. doi:10.1016/j.ecolind.2017.04.019
Zhang, E., Yin, X., Xu, Z., and Yang, Z. (2018). Bottom-up quantification of inter-basin water transfer vulnerability to climate change. Ecol. Indic. 92, 195–206. doi:10.1016/j.ecolind.2017.04.019
Zhou, Y., Guo, S., Hong, X., and Chang, F. J. (2017). Systematic impact assessment on inter-basin water transfer projects of the Hanjiang River Basin in China. J. Hydrology 553, 584–595. doi:10.1016/j.jhydrol.2017.08.039
Zhuang, W. (2016). Eco-environmental impact of inter-basin water transfer projects: a review. Environ. Sci. Pollut. Res. 23 (13), 12867–12879. doi:10.1007/s11356-016-6854-3
Zuniga, J. (2000). The Central Arizona Project. Available at: https://www.usbr.gov/projects/pdf.php?id=94.
Keywords: inter-basin water transfer projects, US environmental planning, environmental impact assessment, environmental impact statement, climate change
Citation: Yi S and Kondolf GM (2024) Environmental planning and the evolution of inter-basin water transfers in the United States. Front. Environ. Sci. 12:1489917. doi: 10.3389/fenvs.2024.1489917
Received: 02 September 2024; Accepted: 25 October 2024;
Published: 12 November 2024.
Edited by:
Buddhi Wijesiri, Queensland University of Technology, AustraliaReviewed by:
Donghoon Lee, University of Manitoba, CanadaCharalampos Skoulikaris, Aristotle University of Thessaloniki, Greece
Copyright © 2024 Yi and Kondolf. This is an open-access article distributed under the terms of the Creative Commons Attribution License (CC BY). The use, distribution or reproduction in other forums is permitted, provided the original author(s) and the copyright owner(s) are credited and that the original publication in this journal is cited, in accordance with accepted academic practice. No use, distribution or reproduction is permitted which does not comply with these terms.
*Correspondence: Sooyeon Yi, c29veWVvbkBiZXJrZWxleS5lZHU=
†ORCID: Sooyeon Yi, orcid.org/0000-0002-3966-4547; G. Mathias Kondolf, orcid.org/0000-0001-5639-9995