- 1Instituto de Microbiologia Paulo de Góes, Universidade Federal do Rio de Janeiro, Rio de Janeiro, Brazil
- 2Multiuser Unit of Environmental Analysis, Institute of Biology, Federal University of Rio de Janeiro, Rio de Janeiro, Brazil
- 3Department of Thematic Studies – Environmental Change and Biogas Solutions Research Center (BSRC), Linköping University, Linköping, Sweden
- 4Institute of Marine Science, Federal University of São Paulo (IMar/UNIFESP), Santos, Brazil
Endocrine-disrupting compounds (EDCs) are a group of emergent pollutants that affect the endocrine system. EDCs can cause harm to humans and animals and unbalance the aquatic ecosystem even at low concentrations. Several methods are applied to remove EDCs, and recently, magnetic nanoparticles (MNPs), a low-cost and magnetically-responsive approach, have been introduced as modern innovative tools for this purpose. Thus, we conducted a systematic analysis to determine the characteristics of MNPs required to optimize EDCs removal in water/waste treatments. Most studies used magnetite (Fe3O4) nanoparticles, a low-cost and easily acquired material, followed by other metallic oxides nanoparticles (MOPs) like zinc, copper, and nickel oxides. Short adsorption time (0.5–15 min) and small MNP (10–80 nm) were the most prominent and successful in EDCs recovery approaches. After analyzing data published in the last decade, results showed that, in general, processes that apply lower concentrations of MNP seem to achieve a significant higher removal rate. For instance, MNPs formulations are able to successfully remove >90% of bisphenols and phthalates. However, depending on the EDCs type, the concentration of MNPs have to be adjusted to increase EDCs recovery rates, possibly due to their different chemical compositions and properties. Our results indicate that MNPs are an efficient, with potential to be a relatively cheap way to extract or eliminate EDCs from wastewater and should be considered an innovative tool to be included in waste treatment protocols.
1 Introduction
Endocrine-disrupting compounds (EDCs) are a group of pollutants with estrogenic and androgenic activity that unbalance the endocrine system (Andersson et al., 2018). EDCs are present in several products consumed by humans, such as pharmaceuticals, pesticides, cosmetics, self-care products, and food packaging. These contaminants can be natural or synthetic and are related to reproductive abnormalities, such as infertility and fetal deformation, different types of cancer, such as ovarian, testicular, and breast cancer, and neurological and immunological disturbs (Martínez-Gómez et al., 2013). Several substances have been associated to endocrine interference, such as bisphenol A (BPA), estrogens and androgens, phenols, phthalates, parabens, and many others (Ahn and Jeung, 2023). Natural estrogens include hormones such as estrone (E1), 17β-estradiol (E2), and estriol (E3) (da Silva et al., 2018; Rosenfeld and Trainor, 2014). Natural androgens include testosterone and androsterone, for instance. Some examples of synthetic hormones are 17β-ethinylestradiol (EE2), norgestrel, and trenbolone found in birth control pills and hormone supplements. However, EE2 and E2, in particular, have high environmental risks, due to their abundance in treated and untreated water (López-Velázquez et al., 2020; López-Velázquez et al., 2022; Latif et al., 2020). Two of the best-known EDCs are BPA, found in plastic packaging, and nonylphenol, a compound generated in the production of detergents, which have high binding affinity for G protein-coupled estrogen receptors (GPER) (Cimmino et al., 2019; Kowalska et al., 2020; Xie et al., 2019; López-Velázquez et al., 2023).
Some EDCs can be degraded into more complex and toxic components, becoming a more significant threat to public health (Nicole, 2013). Furthermore, EDCs banned decades ago are still found in significant concentrations in the environment due to their incomplete degradation increasing the importance of developing efficient methods for removing these substances from the aquatic environment (Čelić, 2020).
In fish, EDCs can cause abnormalities in the reproductive system, reducing the production of fertile eggs by up to 90% (Da Silva et al., 2018). Fetal exposure in humans to EDCs can generate neurobehavioral diseases. Early exposure to BPA, for example, can generate stress-related neurobehavioral disorders earlier in men than in women (Rosenfeld and Trainor, 2014).
Furthermore, phthalates are a group of relevant EDC contaminants and include compounds such as di-2-ethylhexyl phthalate (DEHP), which is used as a plasticizer in products such as poly (vinyl chloride) (PVC) film. In contact with fatty foods, such as meats and cheeses, phthalates like DEHP, dibutyl phthalate (DBP), and diisononyl phthalate (DINP), can migrate from the film to the actual product (Alp and Yerlikaya, 2019).
Perfluorochemicals (PFCs), such as perfluorooctanoic acid (PFOA) and perfluorooctanesulfonic acid (PFOS), also known as C-8, are components used as surfactants in the emulsion polymerization of fluoropolymers in the textile industry and polytetrafluoroethylene (PTFE) cookware that are also known as EDCs. C-8 components have been a controversial topic for many years since the American scandal involving the release of PFCs in their industrial effluents and the lack of toxicity studies (Nicole, 2013). PFCs can induce peroxisome proliferation, affect mitochondrial and reproductive functioning and lipid metabolism (Hyötyläinen et al., 2021), in addition to generating triglyceride accumulation in the liver, pancreatic and testicular cancer, and reduced circulation of thyroid hormone (thyroxine) (Feng et al., 2017; Coperchini et al., 2021; Jensen, 2022).
In Brazilian law, BPA is not seen as a major threat since the number of studies of its effect on humans in smaller doses is considered low by the Brazilian government. However, as a precaution, in Brazil and other countries, the import of baby bottles that contain BPA in their composition is prohibited (resolution RDC n. 41/2011; ANVISA, Brazil). Nevertheless, E2, EE2, BPA, 4NP, and 4NOP were added to the list of priority substances that could be dangerous to the aquatic environment (European Commission, 2018) due to these compounds’ potential to mimic the natural hormone E2 (Vega-Morales et al., 2013; Lopez-Velazquez et al., 2020). Regarding phthalates, DEHP has been added to the EU’s hazardous chemicals list due to their toxicity to the reproductive system. In 2010, the EU stipulated that all medical supplies containing phthalates must be labeled so that healthcare professionals can make informed decisions about the products they use [COMMISSION REGULATION (EU), 2021]. In the United States, the National Food and Drug Administration (FDA) issued a Public Health Notice that directs healthcare professionals to use alternatives to products containing DEHP for certain vulnerable patients (Food and Drug Administration, 2012). In Canada, a Department of Health expert advisory group recommended that healthcare professionals not use DEHP-containing products for the treatment of pregnant women, nursing women, male children before puberty, patients undergoing hemodialysis, and patients who went through heart surgery (Health Canada. 2021).
As much as they are released into the environment in low concentrations (µg/L and ng/L), the continuous disposal of wastes containing EDCs or products that generate EDCs leads to the accumulation of these pollutants over time, especially in aquatic environments (Darbre, 2022). Contaminated waters and wastes are usually directed to wastewater treatment plants (WWTP) and treated to remove EDCs and other pollutants, such as pesticides and pharmaceuticals. However, there is no currently efficient process for removing all potential EDCs. For instance, nonylphenol monoethoxylate has a recovery rate of 31% through low loading/nitrifying process and a 3% of degradation product produced through high loading/non-nitrifying process, differently from other pollutants like nonylphenol diethoxylate that can be removed more efficiently through low loading/nitrifying process (Gadupudi et al., 2021).
EDCs’ treatment can be categorized into physical, chemical, and biological methods. Physical methods, which are commonly employed, include activated carbon adsorption and membrane removal. Activated carbon absorption, in the form of powdered activated carbon or granular activated carbon, represents a well-established process for removing organic components from wastewater, demonstrating efficiency on both laboratory and large-scale plants (Kovalova et al., 2013; Werkneh et al., 2022). Furthermore, the process of isolating and removing the carbon-activated sorbent particles in WWTP can be quite inconvenient. If the approach is based on water filtration, membrane fouling is likely to be an issue. The main chemical method used for the removal of EDCs is advanced chemical oxidation (CAO), which is characterized by the generation of an OH- radical and the mineralization of pollutants in wastewater to CO2 or transfer of pollutants to other metabolic products by strong oxidants through oxidation-reduction reactions. Combined with UV/O3, UV/H2O2 and UV/Fenton, the EDC removal effect is enhanced (Gao et al., 2020). Chlorination is also a chemical method that can be applied for EDCs’ removal. However, studies have shown that the chlorination reaction is incomplete in the removal of BPA and the estrogenic activity slightly decreased after the process (Gao et al., 2020). Compared to chlorination, the use of O3, UV/H2O2 and other methods are more efficient (Werkneh et al., 2022). The biological treatment method is the most used in the extraction of EDCs in WWTPs. The removal of EDCs by biodegradation in wastewater, such as the use of activated sludge, generates the highest rate of estrogen removal (Lange et al., 2013).
Using magnetic nanoparticles (MNPs) offers a convenient solution to mitigate the inefficiency of pollutant removal in current water treatment technologies. MNPs can be easily added to water tanks and recovered and removed by applying an external magnetic field, potentially reducing the labor and time. Several successful applications of MNPs for EDCs removal and degradation have already been developed, and the results from these studies will be collected, analyzed, and discussed in conjunction with this review. An additional approach in which MNPs have also been applied is the development of EDCs preconcentration before detection or quantification in environmental monitoring. Chromatography is the most used technique to detect EDCs. Both gas (GC) and liquid (HPLC) chromatography associated with mass spectrometry (MS) are highly sensitive and commonly used tools for quantitative and qualitative analysis, but they are laborious and time-consuming. Several methods have already been developed to improve chromatography analysis, including those based on applying MNPs (Capriotti et al., 2016; Zhang et al., 2020). Magnetic preconcentration is a technique with the advantage of the easy recovery of nanoparticles through an external magnetic field and the possibility of reuse, bringing an economic benefit to the product. Also, MNPs can be used as catalyst agents to remove or degrade EDCs. Therefore, we used statistics tools to assemble a review based on studies involving magnetic tools to identify and remove EDCs from wastewater.
2 Methods
The systematic review was performed on the Web of Science database from 2013 to 2023 based on the Preferred Reporting Items for Systematic Reviews and Meta-Analyses (PRISMA) (Page et al., 2021). The selected studies were filtered to only articles (document type) and only English (language). The search was performed using the following keywords combined with the Boolean operators and wildcards to create a command line used in the Web Of Science advanced search:
TS=(“endocrine-disrupting” OR “EDC” OR “estrogen*”) AND TS=(“magnetic*” OR “magnetic nanoparticles” OR “magnetic nanosorbent” OR “magnetic sorbent” OR “magnetite”) AND TS=(“removal” OR “removing” OR “extraction” OR “extracting” OR “monitoring” OR “identification” OR “identifying” OR “determination” OR “determining” OR “preconcentration”) AND TS=(“wastewater” OR “effluent*” OR “water”).
The eligibility criteria to include the articles in the systematic review were as follows: EDCs’ type, adsorption time, extraction, degradation, and detection methods, recovery rate, MNPs’ composition and functionalization, particle size, MNPs’ synthesis method, MNPs’ concentration, and reusability.
Data normality was assessed by Shapiro-Wilk test and Gaussian fitting using GraphPad Prism v. 8 software along with statistical inference performed by Kruskal–Wallis test followed by Dunn’s multiple comparison test. Hence, p-value for inference level was set for: 0.0332 (*), 0.0021 (**), 0.0002 (***) and <0.0001 (****).
GraphPad Prism v. 8 software was used for Pearson’s correlation analysis with a 95% confidence interval and a two-tailed p-value approach. Principal component analysis (PCA) and Kernel density estimator density plot (KDE) were performed using Past4 (Hammer, 2001) and OriginPro 2024 (Origin Lab Corporation, Northampton, Massachusetts, United States) and plotted on GraphPad Prism v. 8 for (GraphPad, Boston, Massachusetts United States).
3 Results
3.1 Screening trials
The search for nanoparticles applications in the database retrieved 262 articles, and only 69 remained after the first selection step, in which, in addition to the data mentioned above, reviews, papers outside our research scope, and conference abstracts were eliminated. All selected studies are listed in Supplementary Table S1. Frequency analysis showed that natural hormones (23%), bisphenols (20%), and synthetic hormones (14%) are the most studied EDCs, which may be related to the rising awareness of how impactful these pollutants can potentially become. For instance, magnetic nanotools used to remove some classes of disinfectants and antiseptics (e.g., triclocarban) have only been recently developed, starting in 2020, as they are recent EDCs pollutant models that need to be dealt with. Estrone and 17β-estradiol are the most studied natural hormones, which may be due to their importance in human and other mammals’ health. BPA seems to be the most studied bisphenol since it has been a widely used polymer in plastic composition.
Regarding the MNPs’ composition for EDCs’ removal, magnetite is the most used (65.2%), followed by other MOPs (Supplementary Table S1). Most studies produce their own MNP to evaluate their efficiency in EDCs’ removal. Except in studies that apply magnetic polymers, the co-precipitation method is the most used method of synthesis of MNPs, followed by commercially acquired and hydrothermal method (Supplementary Figure S1). Co-precipitation is a simple and low-cost method, which may be the reason for its broad application, also allowing the combination of different functionalization techniques.
Magnetite MNPs emerge as the most widely used nanoparticle due to their cost-effectiveness and simple production process, such as chemical co-precipitation and hydrothermal method (Supplementary Figure S1). Besides, magnetite nanoparticles have a distinctive surface charge, allowing them to be coated with silica or organic matrices, making them promising candidates for various molecule or enzyme-functionalization. Magnetite was found to be functionalized with different molecules, such as polypyrrole, chitosan, cyclodextrin, oleic and stearic acid, carbon-based structures, polydopamine, and many others, which allows MNPs to interact with components of interest, especially as an adsorption tool and keep their magnetic properties.
Other MNPs’ compositions were used, such as maghemite, the smallest nanoparticle retrieved in our research (11 nm). Ordering them by the increase in average diameter, we detected the use of a hybrid nanoparticle of alginate/magnetite (80 nm), magnetite nanoparticles (82.5 nm ± 93.3), other MOPs (116.1 nm ± 145.4), carbon-based magnetic biopolymers (158.6), and iron nanowires (175 nm). Functionalized molecule size was also considered in the particle size analysis.
3.2 Global tendencies
Regarding recovery rates, there is significant difference between all data sets (****p < 0.0001). Furthermore, MOPs (95.50% ± 5.45%) present significant higher recovery percentages compared to magnetite (82.61% ± 22.80%; ****p < 0.0001) and other nanoparticles (90.62% ± 3.64%; **p = 0.0017) (Figure 1; Supplementary Table S1). Additionally, an important parameter considering EDCs’ removal processes is MNPs’ concentration, which ranges from 4 × 10−7 mg/mL to 200 mg/mL (Figure 1A) (*p = 0.0182). Hence, even though magnetite nanoparticles display a lower recovery rate, this effect could be supported by the largest number of studies using them (n = 437) compared to MOPs (n = 226). Thus, magnetite nanoparticles recover EDCs in a wider percentage area, but also displays good efficiency in reduced concentrations (10.89 ± 34.37 mg/mL) along with MOPs (1.67 ± 3.47 mg/mL), exhibiting no statistical difference between them (ns; p = 0.1896). However, MOPs (1.67 ± 3.40 mg/mL) concentration employed is significantly higher than other nanoparticles (11.78 ± 17.55 mg/mL; *p = 0.0323) and there is a weak positive correlation between their concentration and the recovery rate (r = 0.34; ****p=<0.0001), which is not observed for magnetite or other nanoparticles.
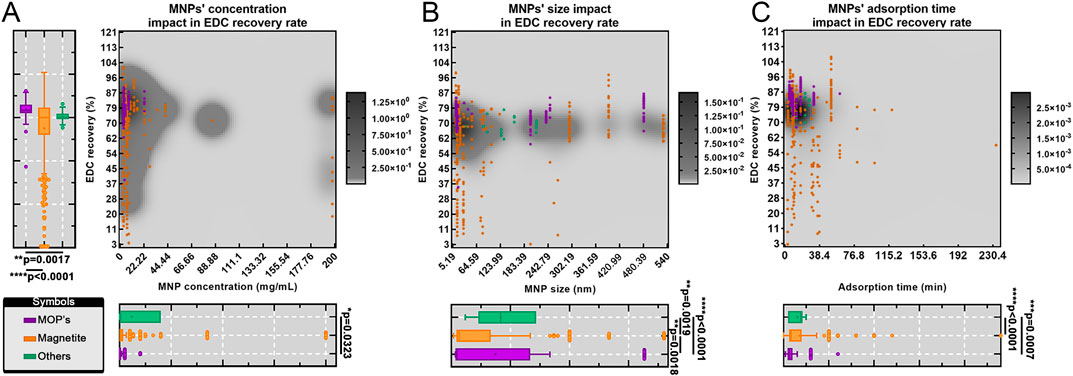
Figure 1. KDE density plot fitting against MNPs and EDCs recovery rates regarding all the contaminants. Different parameters such as concentration (A), size (B), and adsorption time (C) are shown. (A) MOPs (95.50% ± 5.45%; n = 226) present significant higher recovery rates compared to magnetite (82.61% ± 22.80%; ****p < 0.0001; n = 437) and other nanoparticles (90.62% ± 3.64%; **p = 0.0017; n = 26). MOPs (1.67 ± 3.40 mg/mL) concentration employed is significantly higher than other nanoparticles (11.78 ± 17.55 mg/mL; *p = 0.0323). (B) Regarding size, other nanoparticles usually presented significantly higher particle size (125.00 ± 68.54 nm) compared to MOPs (111.6 ± 157.00 nm; **p = 0.0019) and magnetite (99.60 ± 150.40 nm; **p = 0.0018). (C) Regarding adsorption time, significantly lower interaction time for EDC removal was found amongst MOPs (8.88 ± 8.46 min) compared to magnetite (17.84 ± 24.05 min; ****p < 0.0001) and other nanoparticles (15.38 ± 7.47 min; ***p = 0.0007). Legend: (i) MNPs (orange dots); (ii) MOPs (purple dots); and (iii) other nanoparticles (green dots).
Regarding MNPs’ size, it ranges from 5 to 540 nm (Figure 1B), exhibiting significant global difference between groups (****p < 0.0001). Hence, other nanoparticles usually presented significantly higher particle size (125.00 ± 68.54 nm) compared to MOPs (111.6 ± 157.00 nm; **p = 0.0019) and magnetite (99.60 ± 150.40 nm; **p = 0.0018). Additionally, EDCs and MNPs’ adsorption time also showed a wide variance among data, ranging from 30 s to 240 min (Figure 1C), exhibiting significant global difference between groups (****p < 0.0001). Thus, significantly lower interaction time for EDCs removal was found amongst MOPs (8.88 ± 8.46 min) compared to magnetite (17.84 ± 24.05 min; ****p < 0.0001) and other nanoparticles (15.38 ± 7.47 min; ***p = 0.0007). At the same time, adsorption time (Figure 1C) and MNPs’ size (Figure 1B) does not seem to be related to EDCs’ removal on a global scale (Figure 1B). MNPs of the same composition and size presented discrepancies in results (Figure 1B), as well particles with the same composition and dimensions of different magnitudes showed similar results. The shape and crystallographic features of these particles could probably reveal factors associated with high EDCs’ recovery rates. The same rationale appears to be valid for global results considering adsorption time. Moreover, only a few studies reported MNPs reusability, varying through 2 to 60 cycles of use. This would be a critical point in developing an approach to remove EDCs based on particles that can be recovered by magnetism.
Both magnetite and other MOPs require similar and low concentrations (<22 mg/mL) to achieve a high removal rate (Figure 1A). Nevertheless, the systematic analysis suggests that magnetite requires a shorter time than other MOPs to recover EDCs (Figure 1C), which is advantageous since it could accelerate the water treatment process on a large scale.
3.3 Impact of conditions according to EDCs’ composition
To understand the impact of EDCs’ composition on the efficiency of the recovery process, a KDE analysis was performed, focusing on six main EDCs’ categories: parabens, phenols, natural and synthetic hormones, phthalates, and bisphenols (Figure 2). MNPs’ concentration does not seem to directly influence the recovery efficiency of natural hormones (83.04% ± 18.22%) (Figure 2C), including estrogens, mycoestrogens and phytoestrogens, as the application of a similar range of concentrations of MNPs resulted in different recovery rates. This tendency can also be observed in phenols and synthetic hormones (90.11% ± 10.18%), but only in a few studies (Figures 2B, E).
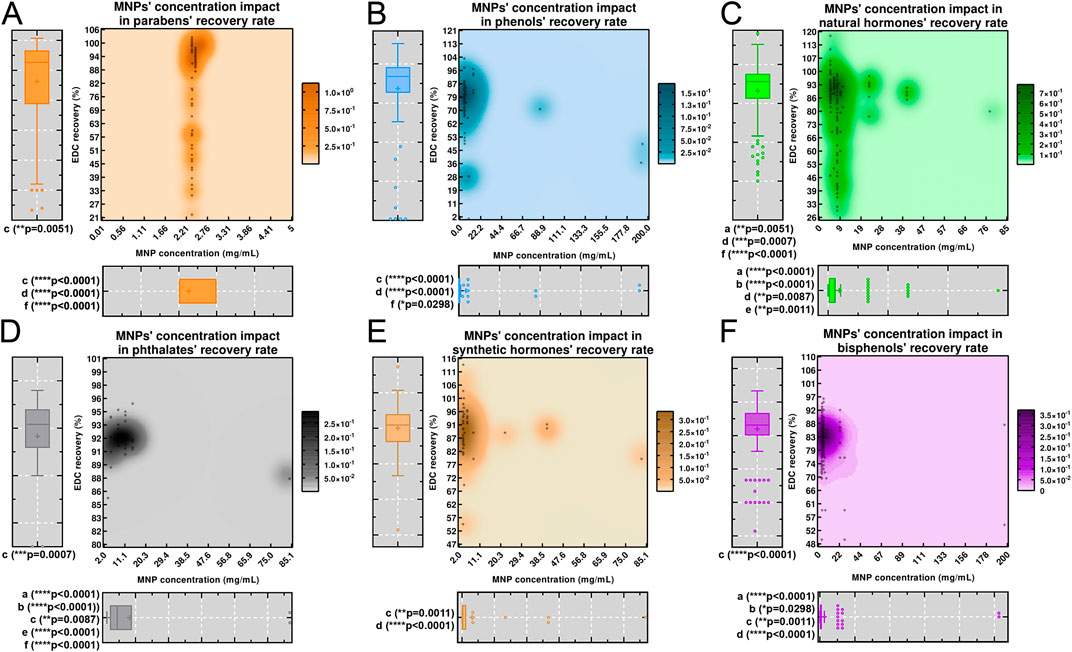
Figure 2. KDE density plot comparing the influence of MNP’ concentration (mg/mL) on different types of EDC on the pollutants classes: (i) parabens (A); (ii) phenols (B); (iii) natural hormones (C); (iv) phthalates (D); (v) synthetic hormones (E); and (vi) bisphenols (F). Regarding efficiency recovery, statistical difference is found among: (i) parabens (84.40% ± 22.32%) and natural hormones (83.04% ± 18.22%; **p = 0.0051); (ii) natural hormones and phthalates (94.99% ± 5.09%; ***p = 0.0007); (iii) natural hormones and bisphenols (92.97% ± 11.03%; ****p < 0.0001). Additionally, regarding MNP concentration usage, parabens (1.33 ± 2.81 mg/mL) display significantly lower values relative to natural hormones (5.57 ± 11.10 mg/mL; ****p < 0.0001), phthalates (12.26 ± 19.87 mg/mL; ****p < 0.0001) and bisphenols (5.07 ± 22.73 mg/mL; ****p < 0.0001).
Regarding parabens, different recovery rates were obtained at the same MNPs’ concentrations, potentially influenced by adsorption time and MNPs’ size (see discussion below, Figures 3, 4). Studies using bisphenols and phthalates (Figures 2D, F) seem to have a more consistent protocol, based on the use of the same MNPs’ concentration range and these studies were able to achieve the same EDCs’ recovery rate. For phthalates highest-recovery rates (94.99% ± 5.09%) were achieved in processes using 2–11 mg/L; the increase in this MNPs’ concentration did not reflect in better efficiency (Figure 2D). Additionally, usage of MNPs to remove EDCs presented significantly different recovery rates between: (i) parabens (84.40% ± 22.32%) and natural hormones (83.04% ± 18.22%; **p = 0.0051); (ii) natural hormones and phthalates (94.99% ± 5.09%; ***p = 0.0007); and (iii) natural hormones and bisphenols (92.97% ± 11.03%; ****p < 0.0001). The same pattern of efficiency in MNPs low concentration was also identified for bisphenol’s recovery processes, in which concentrations below 10 mg/mL showed more than 90% of the EDCs’ recovery. Interestingly, there is a moderate negative correlation found among phthalates’ recovery percentage and concentration (r = −0.70; ****p < 0.0001), thus, indicating smaller MNPs’ concentration has the highest removal rates. The same pattern is found among phenols, but yields a weak negative and statistically significant correlation against MNPs’ concentration (r = −0.30; **p = 0.0024).
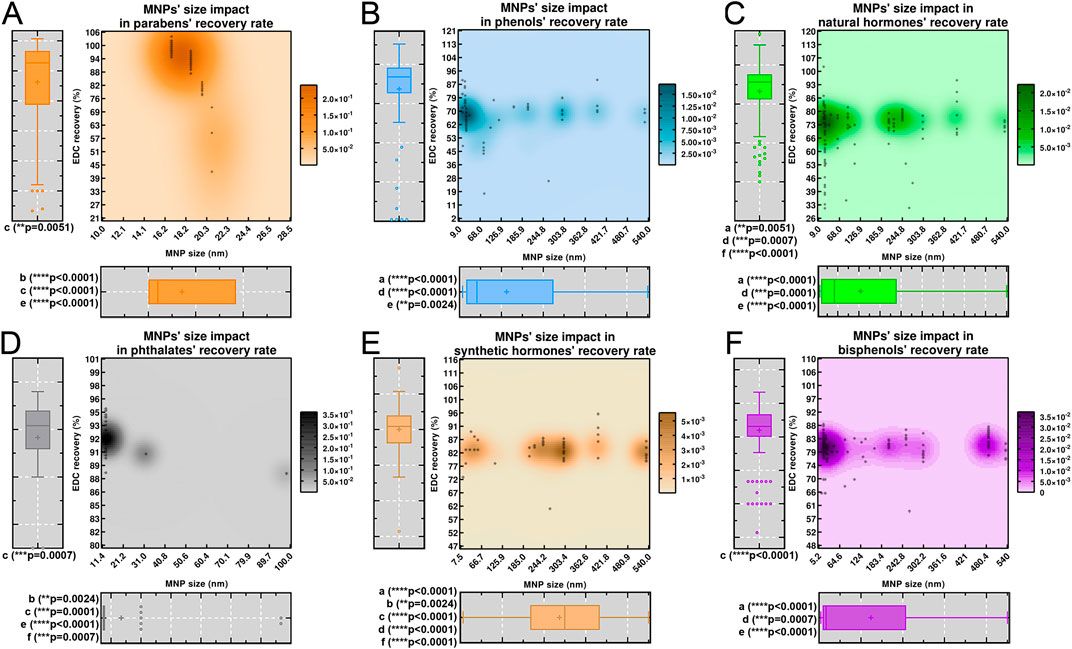
Figure 3. KDE density plot comparing the influence of MNP size (nm) on different types of EDC on the pollutants classes: (i) parabens (A); (ii) phenols (B); (iii) natural hormones (C); (iv) phthalates (D); (v) synthetic hormones (E); and (vi) bisphenols (F). Statistical significantly smaller MNPs were used in parabens (17.11 ± 7.69 nm) recovery compared to phenols (135.20 ± 155.20 nm; ****p < 0.0001), natural hormones (124.40 ± 144.90 nm; ****p < 0.0001), synthetic hormones (284.40 ± 168.80 nm; ****p < 0.0001) and bisphenols (148.80 ± 187.10 nm; ****p < 0.0001).
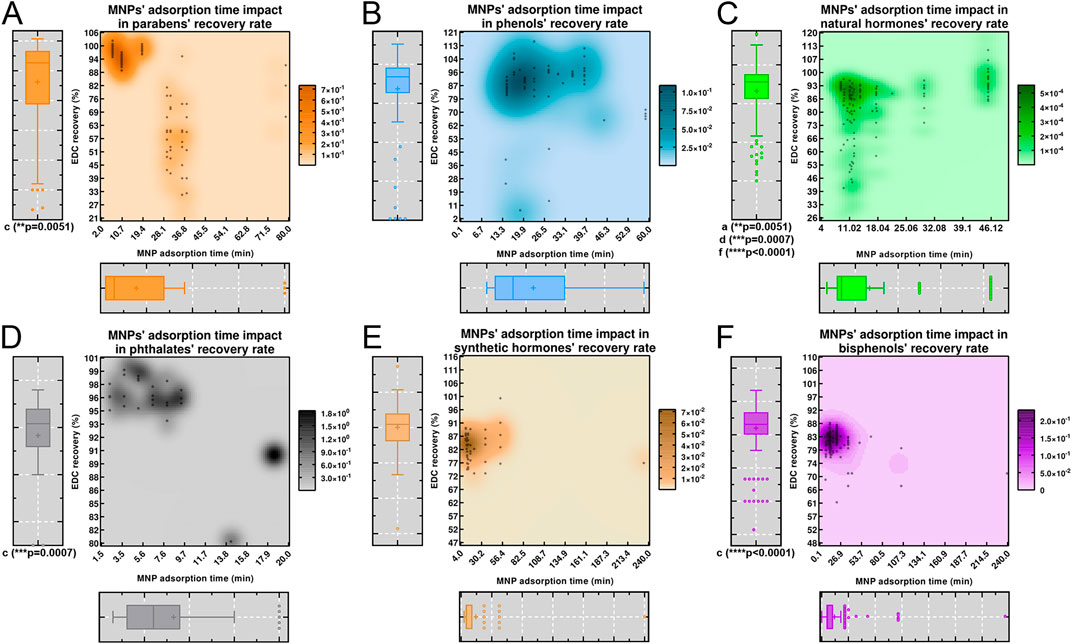
Figure 4. KDE density plot comparing the influence of adsorption time (min) on different types of EDC on the pollutants classes: (i) parabens (A); (ii) phenols (B); (iii) natural hormones (C); (iv) phthalates (D); (v) synthetic hormones (E); and (vi) bisphenols (F). Although phthalates display lower values for adsorption time than others (8.25 ± 6.20 min), no statistical difference was found among the different EDCs.
When data was evaluated according to the different EDCs types, MNPs’ larger size seemed to have a negative impact on parabens and phthalates recovery rate (Figures 3A, D), but not on phenols (Figure 3B), and natural (Figure 3C) and synthetic hormones (Figure 3E), and bisphenols (Figure 3F). Thus, adsorption time seems related to increased parabens (Figure 4A), phenols (Figure 4B), and phthalate (Figure 4D) removal rate. Furthermore, statistically significant negative and strong correlations can be observed regarding MNPs’ size and removal rate for parabens (r = −0.84; ****p < 0.0001) and phthalates (r = −0.91; ****p < 0.0001).
However, elevated adsorption times does not seem to influence the removal rate from natural (Figure 4C) and synthetic hormones (Figure 4E), and bisphenols (Figure 4F), since a same range of time promotes a same range of EDCs’ recovery. It is interesting to point out that some pollutants showed quicker removal rate than others. For instance, MNPs can achieve over 90% recovery of parabens and phthalates in less than 10 min (Figures 4A, D), whereas the recovery of phenols requires 20–40 min of adsorption time (Figure 4B), recovery (Figure 4B), which may be due to the difference on the physical-chemical properties of each molecule. Additionally, a moderate negative and statistically significant correlation was found between adsorption time employed in recovering parabens (r = −0.67; ****p < 0.0001) and phthalates (r = −0.72; ****p < 0.0001) and a weak negative and statistically significant correlation was found among bisphenols (r = −0.44; ****p < 0.0001). Thus, this data corroborates that short period of incubation could be used for recovering these pollutants with high efficiency.
Pearson’s correlation analysis (r) showed a weak statistically significant positive correlation between MNPs’ size and recovery (r = 0.12; **p = 0.0018), which may be due to data heterogeneity. Hence, the positive statistically significant correlation was observed in MOPs (r = 0.29; ****p < 0.0001) and magnetite’s (r = 0.10; *p = 0.0300) group. Furthermore, globally, there is weak negative statistically significant correlation between MNPs’ concentration and recovery (r = −0.11; **p = 0.0032) and a weak positive statistically significant correlation in the MOPs group (r = 0.34; ****p < 0.0001) (Supplementary Figure S2B). Thus, PCA and correlation analysis showed a statistically non-significant negative correlation between adsorption time and recovery rate (r = −0.05; ns; p = 0.1334) (Supplementary Figure S2A), which is also confirmed by a correlation analysis regarding different types of EDCs (Supplementary Table S2). However, linear regression showed that the gathered data is not linear due to data heterogeneity, which was the main reason that KDE was the approach to this study (Supplementary Table S3). Nevertheless, it was possible to observe a tendency regarding MNPs’ concentrations and EDCs’ recovery rates, especially between magnetite and other MOPs.
4 Discussion
EDCs are a major threat in wastewater management and have been increasingly acknowledged in the past few years. Compounds like natural and synthetic hormones comprise 34% of the EDCs studied in the past 10 years. For instance, exposure to diethylstilbestrol, a phytoestrogen, can induce structural and functional alterations in the development of the reproductive system of males (Santti et al., 1998). Moreover, other phytoestrogens like isoflavones such as genistein and daidzein have similar molecular weight and structure characteristics as 17β-estradiol, being able to disrupt the action of this hormone. Furthermore, daidzein can be metabolized to equol, which can specifically bind 5 alpha-dihydrotestosterone and inhibit testosterone (Lephart et al., 2004). Thus, the concern regarding these hormones has been for a long time and still must be dealt with to this day.
However, 20% of all the studies address the removal of bisphenols in general since they are a persistent problem in wastewater, causing several diseases in humans and marine life. In fish, it can cause fish feminization, lower reproductive fitness and sperm quantity, and other reproductivity alterations (Carnevali et al., 2018). In humans, BPA is related to ovulation disorders, brain injury, breast cancer, endometriosis, cardiovascular diseases, obesity, pregnancy and fertility problems, sex and growth hormones disturbances, prostate cancer, and so many others (Engler-Chiurazzi et al., 2017; Sifakis et al., 2017; Giulivo et al., 2016; Wang et al., 2016).
Thus, bisphenols were the most addressed EDC in the last 5 years (29.17%), which may also be due to the rise of toxicity studies regarding implementing alternatives to BPA in the industry, such as BPS, BPF, and others. For instance, BPS is structurally similar to BPA and is stable regarding heat and light (Herrero et al., 2018). Nonetheless, BPS has a longer half-life when compared to BPA, is less likely to be biodegraded, and can generate bioaccumulation (Qiu et al., 2019). Besides bisphenols, other phenols and phthalates have also been in the spotlight in recent years. Phthalates are the most used plasticizers in polymer products and can cause testicular and ovarian dysfunction and reduction in steroidogenesis in humans and other animals (Sree et al., 2022). Phthalates are widely used in the pharmaceutical industry as a drug coating agent. However, it has already been advised to limit the use of components like DEHP and DBP since it has been a concern addressed by different countries (Conforti et al., 2021).
MNPs can be an interesting technology to remove EDCs from water since it is possible to isolate them with an external magnetic field. Most of the MNPs used in literature studies are composed of magnetite and produced through a chemical co-precipitation method (Supplementary Figure S1). In the last 5 years, approximately 45% of the studies used magnetite as their chosen MNP (Supplementary Table S1). Magnetite is an easily acquired and low-cost MNPs with a sufficiently large surface area for analyte binding, obtaining rapid mass transfer, which can be beneficial for a rapid equilibrium (Han et al., 2012). Most of the studies (58 articles) indicated that adsorption is the primary mechanism for removing EDCs, but the increase in concentration and size of MNPs did not influence the process on a global scale (Figure 1). This relatively surprising result could be masked by factors such as MNPs type, as shown in Figure 1B, and EDC type, but also MNPs shape and crystallographic properties, which are usually not considered in studies. Thus, since most studies use magnetite as the MNPs of choice, these studies may be biasing the global analysis, which reinforces the importance of deeper studies. An essential characteristic of MNPs that defines its application and efficiency in processes is its shape (Ali et al., 2016). This may explain the varied effect of MNPs concentration on EDCs recovery (Figure 1A). For instance, similar magnetite concentrations generate different EDC recovery rates, which may implicate an influence of this parameter on EDCs’ recovery (Figure 1A). That may be due to other MNPs’ characteristics besides their composition, such as synthesis method, crystalline structure, and chemical purity, which can affect EDCs’ recovery.
However, when analyzed depending on the EDCs’ type, the conditions studied seem to impact the pollutants’ removal differently. This could assist future research in identifying the optimal concentration conditions depending on the type of contaminant. For instance, point distribution analysis showed that the lower efficiency of parabens recovery is related to the pH effect of the process (Figure 2A) (Rostamifasih et al., 2019), as pH appears to be the most critical parameter in methylparaben removal, which is higher in acidic environments (pH 3–6). For example, reduced MNPs’ concentrations seem to be more relevant for achieving better recovery rates for parabens and phthalates (Figure 2). Moreover, MNPs’ size also impacts differently depending on the EDCs’ composition. For instance, smaller MNPs’ seem to be more efficient in removing parabens and phthalates (Figure 3). Nevertheless, MNPs’ morphology, as mentioned above, and molecule functionalization may also influence the EDCs recovery rate, depending on the available surface for EDC adsorption and the functionalized molecules’ affinity to certain types of EDCs. For example, several studies already used polymers molecularly imprinted magnetic nanoparticles for BPA removal (Ji et al., 2009; Hiratsuka et al., 2013; Lin et al., 2018), using molecules such as 2,2′-bis(4-hydroxyphenyl)-hexafluoropropane, that has high affinity to BPA. Yet, no studies compared the efficiency of different MNPs’ sizes on EDCs’ recovery, which could be interesting to better comprehend the effect of this parameter in water treatment.
The low adsorption time of EDC treatment using MNPs is beneficial since these contaminants are laborious to remove from water, such as observed in correlations for parabens, phthalates and bisphenols (Figure 4). Their removal efficiency highly depends on physicochemical properties such as electrostatic properties, Kow (i.e., n-octanol/water partition coefficient), water solubility, and molecule weight. It is known that molecules with higher Kow are more easily removed through activated carbon, while organic pollutants are usually removed through membranes (Abe, 1999). However, one of the significant advantages of using MNPs to remove EDCs, is the possibility of MNPs’ reusability, decreasing the costs of water treatment.
An important observation is the lack of a consistent pattern in reporting MNPs’ information such as size, reusability, synthesis, concentrations, and removal protocols in publications, leading to the absence of clear patterns. However, despite these data gaps, the information gathered in this review provides valuable insights for optimizing EDCs’ recovery protocols regarding the class of pollutant and MNPs’ parameters (e.g., size, adsorption time, MNPs’ concentration). Furthermore, more in-depth reports of MNPs’ characteristics, such as MNPs’ shape and process conditions, are needed so that comparison of results and simulation of process efficiency are more feasible, especially on large scale.
Although using MNPs to remove EDCs on a large scale has several advantages, it also presents different limitations. MNPs tend to aggregate in aqueous environments due to the loss of colloidal stability due to dipole-dipole interactions. This is one of the main challenges of using this tool since it reduces their effective surface area and, consequently, their adsorption capacity for different molecules. A solution would be to modify the MNP to optimize their effectiveness, which adds an extra step and cost to the production of MNPs (Yeap et al., 2017).
Another important characteristic of MNPs is their reusability. Even though the ability to reuse MNPs is a crucial advantage for their economic feasibility, MNPs may lose their adsorption efficiency after multiple cycles due to surface fouling or structural degradation (Mohammed et al., 2016). Thus, developing more effective and sustainable regeneration techniques is essential for MNPs to be economically viable in large-scale applications. Research into methods that minimize nanoparticle degradation, such as low-temperature regeneration processes or enzymatic treatments, could significantly enhance the reusability of MNPs (Shukla et al., 2021). Moreover, the energy costs of magnetic recovery can limit the economic feasibility of large-scale applications, which is also a parameter that should be optimized.
Nonetheless, MNPs show promise for EDC removal, but their selectivity for specific contaminants is often limited. The presence of competing organic matter and ions in real wastewater environments can affect the performance of MNPs, which indicates that further work is needed to enhance the selectivity of MNPs (Shukla, Khan and Daverey, 2021).
Combining MNPs with other treatment technologies, such as membrane filtration or advanced oxidation processes, may provide a more robust solution for EDC removal. Hybrid systems could exploit the strengths of multiple techniques to improve efficiency and reduce operational costs (Mpongwana and Rathilal, 2022).
5 Conclusion
Most of the nanoparticles used in EDCs’ recovery in the literature are composed of magnetite and produced through a chemical co-precipitation method. MNPs’ concentration seems to be directly related to EDCs’ recovery, in which a low concentration of the tool could achieve significant removal rates. Studies using MNPs with sizes ranging from 10 to 80 nm, in low concentrations (0.071–20 mg/mL) and short adsorption time (0.5–15 min) showed high EDCs’ recovery rates (e.g., >90%), which allows these conditions to be applied on new technology development in water treatment units. Thus, this strategy could represent a low-cost solution since it does not require high-end infrastructure. Since magnetite is an easily-acquired and low-cost nanomaterial and can be coated with different molecules, the usage of MNPs in future studies to optimize EDCs’ recovery in water is a relevant model. Thus, this study shows that each parameter may impact differently each EDC type, which can assist new studies in optimizing experimental designs.
Data availability statement
The original contributions presented in the study are included in the article/Supplementary Material, further inquiries can be directed to the corresponding authors.
Author contributions
JG: Conceptualization, Writing–original draft, Writing–review and editing, Data curation, Investigation. IT: Data curation, Writing–original draft, Writing–review and editing, Methodology. TA: Methodology, Writing–original draft, Writing–review and editing. AE-P: Methodology, Writing–original draft, Writing–review and editing, Conceptualization, Funding acquisition, Project administration. FA: Conceptualization, Funding acquisition, Project administration, Writing–original draft, Writing–review and editing, Supervision.
Funding
The author(s) declare that financial support was received for the research, authorship, and/or publication of this article. Financial support was provided by the Brazilian agencies CNPq, CAPES, and FAPERJ. This study was financed in part by the Coordenação de Aperfeiçoamento de Pessoal de Nível Superior–Brasil (CAPES)—Finance Code 001. Financial support was also provided by the Swedish Energy Agency (project number P2023-00827) and the funding agency Formas (Grant number: 2021-02429). AE-P received funds from the Swedish Energy Agency (Grant number: 35624-2) at the Biogas Research Solution Center hosted by Linköping University, Sweden.
Conflict of interest
The authors declare that the research was conducted in the absence of any commercial or financial relationships that could be construed as a potential conflict of interest.
Publisher’s note
All claims expressed in this article are solely those of the authors and do not necessarily represent those of their affiliated organizations, or those of the publisher, the editors and the reviewers. Any product that may be evaluated in this article, or claim that may be made by its manufacturer, is not guaranteed or endorsed by the publisher.
Supplementary material
The Supplementary Material for this article can be found online at: https://www.frontiersin.org/articles/10.3389/fenvs.2024.1488895/full#supplementary-material
References
Ahn, C., and Jeung, E.-B. (2023). Endocrine-disrupting chemicals and disease endpoints. Int. J. Mol. Sci. 24 (6), 5342. doi:10.3390/ijms24065342
Abe, I. (1999). Adsorption properties of endocrine disruptors onto activated carbon. J. Water Waste 41, 43–47. Available at: https://cir.nii.ac.jp/crid/1571980075526719616
Ali, A., Zafar, H., Zia, M., ul Haq, I., Phull, A. R., Ali, J. S., et al. (2016). Synthesis, characterization, applications, and challenges of iron oxide nanoparticles. Nanotechnol. Sci. Appl. 9, 49–67. doi:10.2147/nsa.s99986
Alp, A. C., and Yerlikaya, P. (2019). Phthalate ester migration into food: effect of packaging material and time. Eur. Food Res. Technol. 246 (3), 425–435. doi:10.1007/s00217-019-03412-y
Andersson, N., Arena, M., Auteri, D., Barmaz, S., Grignard, E., Kienzler, A., et al. (2018). Guidance for the identification of endocrine disruptors in the context of Regulations (EU) No 528/2012 and (EC) No 1107/2009. EFSA J. 16 (6), e05311. doi:10.2903/j.efsa.2018.5311
Capriotti, A. L., Cavaliere, C., La Barbera, G., Piovesana, S., Samperi, R., Zenezini Chiozzi, R., et al. (2016). Polydopamine-coated magnetic nanoparticles for isolation and enrichment of estrogenic compounds from surface water samples followed by liquid chromatography-tandem mass spectrometry determination. Anal. Bioanal. Chem. 408, 4011–4020. doi:10.1007/s00216-016-9489-9
Carnevali, O., Santangeli, S., Forner-Piquer, I., Basili, D., and Maradonna, F. (2018). Endocrine-disrupting chemicals in aquatic environment: what are the risks for fish gametes? Fish Physiology Biochem. 44 (6), 1561–1576. doi:10.1007/s10695-018-0507-z
Čelić, M. (2020). Occurrence and assessment of environmental risks of endocrine disrupting compounds in drinking, surface and wastewaters in Serbia. Environ. Pollution. 262, 114344. doi:10.1016/j.envpol.2020.114344
Cimmino, I., Oriente, F., D’Esposito, V., Liguoro, D., Liguoro, P., Ambrosio, M. R., et al. (2019). Low-dose Bisphenol-A regulates inflammatory cytokines through GPR30 in mammary adipose cells. J. Mol. Endocrinol. 63 (4), 273–283. doi:10.1530/jme-18-0265
Conforti, A., Carbone, L., Simeon, V., Chiodini, P., Marrone, V., Bagnulo, F., et al. (2021). Unravelling the link between phthalate exposure and endometriosis in humans: a systematic review and meta-analysis of the literature. J. Assisted Reproduction Genet. 38 (10), 2543–2557. doi:10.1007/s10815-021-02265-3
Coperchini, F., Croce, L., Ricci, G., Magri, F., Rotondi, M., Imbriani, M., et al. (2021). Thyroid disrupting effects of old and new generation PFAS. Front. Endocrinol. 11, 612320. doi:10.3389/fendo.2020.612320
Darbre, P. D. (2022). What are endocrine disrupters and where are they found? in: Endocrine disruption and human health (Academic Press), 3–29. doi:10.1016/B978-0-12-821985-0.00001-3
da Silva, A. P. A., De Oliveira, C. D. L., Quirino, A. M. S., Da Silva, F. D. M., de Aquino Saraiva, R., and Silva-Cavalcanti, J. S. (2018). Endocrine disruptors in aquatic environment: effects and consequences on the biodiversity of fish and amphibian species. Aquat. Sci. Technol. 6, 35–51. doi:10.5296/ast.v6i1.12565
Engler-Chiurazzi, E. B., Brown, C. M., Povroznik, J. M., and Simpkins, J. W. (2017). Estrogens as neuroprotectants: estrogenic actions in the context of cognitive aging and brain injury. Prog. Neurobiol. 157, 188–211. doi:10.1016/j.pneurobio.2015.12.008
European Commission (2018). Proposal for a DIRECTIVE of the EUROPEAN PARLIAMENT and of the COUNCIL on the quality of water intended for human consumption. Brussels: Recast. Available at: https://ec.europa.eu/transparency/regdoc/rep/2/2017/EN/SEC-2017-527-F1-EN-MAIN-PART-1.PDF (Accessed October, 2024).
Feng, X., Cao, X., Zhao, S., Hua, X., Chen, L., Chen, L., et al. (2017). Exposure of pregnant mice to perfluorobutanesulfonate causes hypothyroxinemia and developmental abnormalities in female offspring. Toxicol. Sci. 155 (2), 409–419. doi:10.1093/toxsci/kfw219
Food and Drug Administration (FDA) (2012). Guidance for industry limiting the use of certain phthalates as Excipients in CDER-regulated products. Available at: https://www.fda.gov/files/drugs/published/Limiting-the-Use-of-Certain-Phthalates-as-Excipients-in-CDER-Regulated-Products_pdf.pdf (Accessed October, 2024).
Gao, X., Shuang, K., Rongwei, X., and Ming, C. (2020). Environment-friendly removal methods for endocrine disrupting chemicals. Sustain. 12, 7615. doi:10.3390/su12187615
Gadupudi, C. K. (2021). Endocrine Disrupting Compounds Removal Methods from Wastewater in the United Kingdom: A Review. Sci 3 (1), 11. doi:10.3390/sci3010011
Giulivo, M., de Alda, M. L., Capri, E., and Barceló, D. (2016). Human exposure to endocrine disrupting compounds: their role in reproductive systems, metabolic syndrome and breast cancer. A Rev. Environ. Res. 151, 251–264. doi:10.1016/j.envres.2016.07.011
Hammer, O. (2001). PAST: paleontological statistics software package for education and data analysis. Palaeontol. Electron 4, 9. Available at: https://palaeo-electronica.org/2001_1/past/issue1_01.htm
Han, Q., Wang, Z., Xia, J., Chen, S., Zhang, X., and Ding, M. (2012). Facile and tunable fabrication of Fe3O4/graphene oxide nanocomposites and their application in the magnetic solid-phase extraction of polycyclic aromatic hydrocarbons from environmental water samples. Talanta 101, 388–395. doi:10.1016/j.talanta.2012.09.046
Health Canada (2021). Di(2-ethylhexyl) phthalate (DEHP) in Canadians. Available at: https://www.canada.ca/content/dam/hc-sc/documents/services/environmental-workplace-health/reports-publications/environmental-contaminants/human-biomonitoring-resources/2-ethylhexyl-phthalate-canadians/dehp-eng.pdf (Accessed October, 2024).
Herrero, Ó., Aquilino, M., Sánchez-Argüello, P., and Planelló, R. (2018). The BPA-substitute bisphenol S alters the transcription of genes related to endocrine, stress response and biotransformation pathways in the aquatic midge Chironomus riparius (Diptera, Chironomidae). PLoS One 13 (2), e0193387. doi:10.1371/journal.pone.0193387
Hiratsuka, Y., Funaya, N., Matsunaga, H., and Haginaka, J. (2013). Preparation of magnetic molecularly imprinted polymers for bisphenol A and its analogues and their application to the assay of bisphenol A in river water. J. Pharm. Biomed. analysis 75, 180–185. doi:10.1016/j.jpba.2012.11.030
Hyötyläinen, T., Bodin, J., Duberg, D., Dirven, H., Nygaard, U. C., and Orešič, M. (2021). Lipidomic analyses reveal modulation of lipid metabolism by the PFAS perfluoroundecanoic acid (PFUNDA) in Non-Obese diabetic mice. Front. Genet. 12, 721507. doi:10.3389/fgene.2021.721507
Jensen, R. C. (2022). Higher free thyroxine associated with PFAS exposure in first trimester. The odense child cohort. Environ. Rese. 212, 113492. doi:10.1016/j.envres.2022.113492
Ji, Y., Yin, J., Xu, Z., Zhao, C., Huang, H., Zhang, H., et al. (2009). Preparation of magnetic molecularly imprinted polymer for rapid determination of bisphenol A in environmental water and milk samples. Anal. Bioanal. Chem. 395, 1125–1133. doi:10.1007/s00216-009-3020-5
Kovalova, L., Siegrist, H., Von Gunten, U., Eugster, J., Hagenbuch, M., Wittmer, A., et al. (2013). Elimination of micropollutants during post-treatment of hospital wastewater with powdered activated carbon, ozone, and UV. Environ. Sci. and Technol. 47 (14), 7899–7908. doi:10.1021/es400708w
Kowalska, K., Habrowska-Górczyńska, D. E., Domińska, K., Urbanek, K. A., and Piastowska-Ciesielska, A. W. (2020). ERβ and nfκb—modulators of zearalenone-induced oxidative stress in human prostate cancer cells. Toxins 12 (3), 199. doi:10.3390/toxins12030199
Lange, C., Kuch, B., and Metzger, J. W. (2013). Determination of the occurrence and elimination of endocrine disrupting compounds (EDCs) in Municipal Wastewater Treatment Plants (WWTP). Comput. Water Energy Environ. Eng. 03 (01), 1–7. doi:10.4236/cweee.2014.31001
Latif, A., Sheng, D., Sun, K., Si, Y., Azeem, M., Abbas, A., et al. (2020). Remediation of heavy metals polluted environment using Fe-based nanoparticles: mechanisms, influencing factors, and environmental implications. Environ. Pollut. 264, 114728. doi:10.1016/j.envpol.2020.114728
Lephart, E. D., Setchell, K. D., Handa, R. J., and Lund, T. D. (2004). Behavioral effects of endocrine-disrupting substances: phytoestrogens. ILAR J. 45 (4), 443–454. doi:10.1093/ilar.45.4.443
Lin, Z., Zhang, Y., Su, Y., Qi, J., Jia, Y., Huang, C., et al. (2018). Selective extraction of bisphenol A from water by one-monomer molecularly imprinted magnetic nanoparticles. J. Sep. Sci. 41 (9), 2029–2036. doi:10.1002/jssc.201701162
López-Velázquez, K., Guzmán-Mar, J. L., Saldarriaga-Noreña, H. A., Murillo-Tovar, M. A., Hinojosa-Reyes, L., and Villanueva-Rodríguez, M. (2020). Occurrence and seasonal distribution of five selected endocrine-disrupting compounds in wastewater treatment plants of the Metropolitan Area of Monterrey, Mexico: the role of water quality parameters. Environ. Pollut. 269, 116223. doi:10.1016/j.envpol.2020.116223
López-Velázquez, K., Guzmán-Mar, J. L., Saldarriaga-Noreña, H. A., Murillo-Tovar, M. A., and Villanueva-Rodríguez, M. (2022). Ecological risk assessment associated with five endocrine-disrupting compounds in wastewater treatment plants of Northeast Mexico. Environ. Sci. Pollut. Res. 30 (11), 30714–30726. doi:10.1007/s11356-022-24322-9
Martínez-Gómez, C., Lamoree, M., Hamers, T., Van Velzen, M., Kamstra, J. H., Fernández, B., et al. (2013). Integrated chemical and biological analysis to explain estrogenic potency in bile extracts of red mullet (Mullus barbatus). Aquat. Toxicol. 134, 1–10. doi:10.1016/j.aquatox.2013.02.015
Mohammed, L. G., Ragab, D., and Zhu, J. (2016). Magnetic nanoparticles for environmental and biomedical applications: a review. Particuology 30, 1–14. doi:10.1016/j.partic.2016.06.001
Mpongwana, N., and Rathilal, S. (2022). A review of the Techno-Economic feasibility of nanoparticle application for wastewater treatment. Water 14 (10), 1550. doi:10.3390/w14101550
Nicole, W. (2013). PFOA and cancer in a highly exposed community: new findings from the C8 science panel. Environ. Health Perspect. 121, A340. doi:10.1289/ehp.121-A340
Page, M. J. (2021). PRISMA 2020 explanation and elaboration: updated guidance and exemplars for reporting systematic reviews. BMJ, 160. doi:10.1136/bmj.n160
Qiu, W., Zhan, H., Hu, J., Zhang, T., Xu, H., Wang, M., et al. (2019). The occurrence, potential toxicity, and toxicity mechanism of bisphenol S, a substitute of bisphenol A: a critical review of recent progress. Ecotoxicol. Environ. Saf. 173, 192–202. doi:10.1016/j.ecoenv.2019.01.114
Rosenfeld, C. S., and Trainor, B. C. (2014). Environmental health factors and sexually dimorphic differences in behavioral disruptions. Curr. Environ. Health Rep. 1, 287–301. doi:10.1007/s40572-014-0027-7
Rostamifasih, Z., Pasalari, H., Mohammadi, F., and Esrafili, A. (2019). Heterogeneous catalytic degradation of methylparaben using persulfate activated by natural magnetite; optimization and modeling by response surface methodology. J. Chem. Technol. and Biotechnol. 94 (6), 1880–1892. doi:10.1002/jctb.5964
Santti, R., Mäkelä, S., Strauss, L., Korkman, J., and Kostian, M. L. (1998). Phytoestrogens: potential endocrine disruptors in males. Toxicol. Industrial Health 14 (1-2), 223–237. doi:10.1177/074823379801400114
Shukla, S., Khan, R., and Daverey, A. (2021). Synthesis and characterization of magnetic nanoparticles, and their applications in wastewater treatment: A review. Environ. Techno. Innovat. 24, 101924. doi:10.1016/j.eti.2021.101924
Sifakis, S., Androutsopoulos, V. P., Tsatsakis, A. M., and Spandidos, D. A. (2017). Human exposure to endocrine disrupting chemicals: effects on the male and female reproductive systems. Environ. Toxicol. Pharmacol. 51, 56–70. doi:10.1016/j.etap.2017.02.024
Sree, C. G., Buddolla, V., Lakshmi, B. A., and Kim, Y. J. (2022). Phthalate toxicity mechanisms: an update. Comp. Biochem. Physiology Part C Toxicol. and Pharmacol. 263, 109498. doi:10.1016/j.cbpc.2022.109498
Vega-Morales, T., Sosa-Ferrera, Z., and Santana-Rodríguez, J. J. (2013). Evaluation of the presence of Endocrine-Disrupting compounds in dissolved and solid wastewater treatment plant samples of Gran Canaria Island (Spain). BioMed Res. Int. 2013, 1–15. doi:10.1155/2013/790570
Wang, Q., Trevino, L. S., Wong, R. L. Y., Medvedovic, M., Chen, J., Ho, S. M., et al. (2016). Reprogramming of the epigenome by MLL1 links Early-Life environmental exposures to prostate cancer risk. Mol. Endocrinol. 30 (8), 856–871. doi:10.1210/me.2015-1310
Werkneh, A. A., Gebru, S. B., Redae, G. H., and Tsige, A. G. (2022). Removal of endocrine disrupters from the contaminated environment: public health concerns, treatment strategies and future perspectives - a review. Heliyon 8 (4), e09206. doi:10.1016/j.heliyon.2022.e09206
Xie, M., Liang, J. L., Huang, H. D., Wang, M. J., Zhang, T., and Yang, X. F. (2019). Low doses of nonylphenol promote growth of colon cancer cells through activation of ERK1/2 via G protein‒coupled receptor 30. Cancer Res. Treat. 51 (4), 1620–1631. doi:10.4143/crt.2018.340
Yeap, S. P., Lim, J., Ooi, B. S., and Ahmad, A. L. (2017). Agglomeration, colloidal stability, and magnetic separation of magnetic nanoparticles: collective influences on environmental engineering applications. J. Nanoparticle Res. 19 (11), 368. doi:10.1007/s11051-017-4065-6
Keywords: endocrine disrupting compounds, nanotechnology, water treatment, water pollution, wastewater treatment
Citation: Guimarães J, Taveira I, Anacleto TM, Enrich-Prast A and Abreu F (2024) Magnetic nanoparticles for eliminating endocrine-disrupting compounds in water treatment – a quantitative systematic analysis. Front. Environ. Sci. 12:1488895. doi: 10.3389/fenvs.2024.1488895
Received: 30 August 2024; Accepted: 07 November 2024;
Published: 28 November 2024.
Edited by:
Carmen Teodosiu, Gheorghe Asachi Technical University of Iași, RomaniaReviewed by:
Khirbet López Velázquez, Polytechnic University of Tapachula, MexicoŞana Sungur, Mustafa Kemal University, Türkiye
Copyright © 2024 Guimarães, Taveira, Anacleto, Enrich-Prast and Abreu. This is an open-access article distributed under the terms of the Creative Commons Attribution License (CC BY). The use, distribution or reproduction in other forums is permitted, provided the original author(s) and the copyright owner(s) are credited and that the original publication in this journal is cited, in accordance with accepted academic practice. No use, distribution or reproduction is permitted which does not comply with these terms.
*Correspondence: Alex Enrich-Prast, YWxleC5lbnJpY2gucHJhc3RAbGl1LnNl; Fernanda Abreu, ZmVybmFuZGFhYWJyZXVAbWljcm8udWZyai5icg==
†These authors share first authorship