- State Key Laboratory of Nutrient Use and Management, Shandong Key Laboratory of Bulk Open-field Vegetable Breeding, Ministry of Agriculture and Rural Affairs Key Laboratory of Huang Huai Protected Horticulture Engineering, Institute of Vegetables, Shandong Academy of Agricultural Sciences, Jinan, China
The accumulation of cadmium (Cd) in facility soil is attracting increasing attention. Reductive soil disinfestation (RSD) is an effective soil disinfection method, while also having a certain passivating effect on Cd. The application of organic matter is crucial for the success of RSD, but the reduction efficiencies of different organic materials vary. Here, five treatments, namely untreated soil (CK), bean dregs (BD), peanut dregs (PD), sesame dregs (SD), and apple dregs (AD) were applied, and their performances in Cd immobilization in Cd-contaminated soil were assessed. Changes in soil properties and microbial communities were monitored. The results showed that the overall soil pH following RSD treatment decreased significantly, while organic matter, hydrolyzed nitrogen, available phosphorus, available potassium, exchangeable calcium, and exchangeable magnesium increased significantly. Compared to CK, the exchangeable (EX)-Cd contents after treatments SD and AD significantly decreased by 25.4% and 23.7%, respectively. RSD led to significant changes in the composition of soil microbial communities. SD treatment significantly increased the number of soil fungal operational taxonomic units (OTU), while BD, PD, and SD significantly increased the relative abundances of Bacteroidetes, Chloroflexi, Deinococus Thermus, and Basidiomycota in bacterial communities. The Gemmatimonadetes phylum showed a highly significant negative correlation with EX-Cd, indicating that it might have a positive effect on the fixation of Cd in polluted soil. SD significantly reduced the Cd content in the above-ground parts of lettuce by 74.76%, and had the least impact on lettuce biomass. It can be inferred that RSD is an ecologically effective method for the remediation of Cd pollution in facility soil by improving soil characteristics and altering microbial community composition to reduce Cd activity. However, further in-depth research is needed to optimize the types and amounts of organic materials applied.
1 Introduction
Cadmium (Cd) is a heavy metal element that seriously endangers the health of the food chain and is listed among the most dangerous metals by the United States Environmental Protection Agency (Hussain et al., 2020; Shi et al., 2018). According to a survey, 7% of the surveyed farmland was found to be polluted by Cd, most of which was moderately polluted (Ministry of Environmental Protection, Ministry of Land and Resources of the People’s Republic of China, 2014). Excessive Cd can inhibit cell elongation and change root morphological characteristics, thereby seriously affecting plant photosynthesis, respiration, transpiration, mineral absorption, and other physiological processes, resulting in a decline in crop yield and quality (Sun et al., 2019; Huang et al., 2020; Ogunkunle et al., 2020).
The commonly used remediation technologies for Cd-contaminated soil include physical and chemical remediation (soil washing, electroremediation, chemical immobilization, etc.) and bioremediation (phytoremediation and microbial precipitation) (Mulligan et al., 2001). The former include the application of strong chelating agents to clean Cd-contaminated soil (Dermont et al., 2008) and the use of high-surface-area adsorbents to reduce the mobility of Cd ions (Guo et al., 2006). It has been reported that the combined deployment of limestone and sepiolite could reduce the Cd content in rice by 26.1%–56.5% (Yang et al., 2022). Among bioremediation methods, strain B7 was recently isolated from Cd-contaminated soil, which was shown to significantly reduce the Cd content in the upper part of Chinese cabbage by 21.3%–32.9% (Han et al., 2022). However, physical and chemical methods usually have high energy requirements, while biological methods are time-consuming, and heavy metals accumulated in biomass may cause secondary pollution (Martin and Ruby, 2010; Nareshkumar et al., 2008). Therefore, the development of eco-friendly and low-cost remediation methods suitable for reducing Cd pollution in agricultural land has received significant attention.
Reductive soil disinfestation (RSD), originally developed by researchers in the Netherlands and Japan, is an effective method for eradicating soil-borne pathogens during the continuous cropping of crops (such as flowers and strawberries) (Butler et al., 2012). Earlier, researchers had created anaerobic environments by using easily degradable organic matter such as sugarcane bagasse (SB) and bean dregs (BD), and achieved strongly reducing soil environments by irrigating to saturation and laying plastic film, thereby achieving a good bactericidal effect (Blok et al., 2000; Momma et al., 2013). RSD can significantly improve soil chemical properties and microbial community composition and structure, which is beneficial to crop seedling growth (Li et al., 2021). Studies have shown that RSD can increase the pH of the soil environment (Liu et al., 2019), and should have a significant effect on the solubility and morphological composition of metals (Zhao et al., 2010). RSD treatment has the potential to alter the composition of the soil microbial community, which could ultimately impact the morphological distribution and bioavailability of Cd. For example, RSD treatment has been shown to increase the relative abundances of Plantomycetes and Gemmatimonadetes and to decrease the exchangeable Cd content in soil (Li et al., 2021). In a recent study, the key genera Blastococcus and Gemmaticonas were found to play important ecological roles in triggering resistance to Cd toxicity (Wang Y. et al., 2019). Cheng et al. (2020) proved that the strain Serratia liquefaciens CL-1 could effectively reduce the available Cd and Pb contents in metal-contaminated soil under anaerobic conditions (similar to the environmental conditions imparted by RSD treatment).
However, to the best of our knowledge, few studies have explored the effects of RSD technology on Cd speciation and microbial community changes in continuous cropping facility soil through microbiome sequencing. The change of soil microbial community caused by RSD may be another factor affecting Cd immobilization. In our study, the effects of five RSD treatments [untreated soil (CK), bean dregs (BD), peanut dregs (PD), sesame dregs (SD), and apple dregs (AD)] were studied by soil culture experiments. The effects of RSD on Cd immobilization by linking microbial communities and microbial metabolites in Cd-contaminated soil were probed. We assessed whether the RSD method could limit the bioavailability of Cd by changing the composition of microbial communities and the physical and chemical properties of soil.
The objectives of this study were as follows: 1) to explore the effect of RSD treatment on the characteristics of Cd-contaminated soil in continuous cropping facilities; 2) to determine the distributions of Cd fractions in Cd-contaminated soil following RSD treatment; 3) to determine the differences in soil microbial compositions between different treatments; and 4) to delineate the correlation between soil properties, microbial community, and Cd immobilization. The results of this study are expected to further deepen our understanding of RSD-mediated soil Cd fixation mechanisms and to provide a potential biotechnological approach for the healthy utilization of Cd-contaminated soil in continuous cropping facilities.
2 Materials and methods
2.1 Site description, sample collection, and experimental design
Experiments were conducted in a greenhouse at the core experimental base of the Vegetable Institute of the Shandong Academy of Agricultural Sciences. The tested soil (0–20 cm) was taken from a tomato greenhouse in Changqing District, Jinan City, Shandong Province, China (116° 51′ 44″ E, 36° 33′ 54″ N). The properties of the soil were analyzed. Specifically, its pH was 7.93, its organic matter content was 42.8 g kg−1, its total nitrogen content was 1.84 g kg−1, its available phosphorus was 102.6 mg kg−1, and its available potassium was 1.53 g kg−1.
Experiments were carried out in pots. The total Cd content in the soil was set at 5 mg kg–1 as the target value. The requisite amount of CdCl2 was calculated according to the dry weight and Cd content of the soil. The accurately weighed CdCl2 was evenly mixed with the soil sample, which was then watered. The soil moisture content was adjusted to 60% of the maximum field capacity. All treatments were performed in polyethylene pots of 17 cm × 24.8 cm × 17.1 cm, with 4 kg of soil per pot (water content 13.5%, w/w). 1) Untreated soil (CK), 2) bean dregs (BD), 3) peanut dregs (PD), 4) sesame dregs (SD), and 5) apple dregs (AD) were selected and mixed with the balanced soil as appropriate. Each material was mixed with the balanced soil at a loading of 150 g (dry weight) per pot, and the final mixture was irrigated and covered with plastic film (moisture content 12.5%, w/w). A randomized block design was used, with four replicates per treatment. Anaerobic soil conditions at 30°C–35°C were maintained for 30 days. Subsequently, the lettuce seedlings were transplanted into pots, five plants per pot, and grown in a solar greenhouse environment for 60 days. Lettuce plants and soil samples were then collected. Some lettuce plants and fresh soil samples were treated with liquid nitrogen and kept at −80°C prior to determination. Some lettuce plants and soil samples were dried and air-dried, respectively, and sieved prior to further testing.
2.2 Analysis of soil characteristics and Cd components
Soil pH was measured using a pH meter (Mettler-Toledo International, Inc., China) at a soil/water ratio of 1:5 (v/v). Available phosphorus (AP) was determined on an automated colorimeter (Zhong et al., 2010) after extraction with 0.5 m NaHCO3 solution at a 1:10 soil-to-NaHCO3 ratio. Available potassium (AK) was extracted with NH4OAc solution and determined by a flame photometric method. Exchangeable calcium (ECa) and exchangeable magnesium (EMg) were extracted with 1 m NH4OAc solution at pH 7.0 and analyzed by means of an atomic absorption spectrophotometer (AAS, TAS-990, China). Hydrolyzable nitrogen (AN) was measured by UV/Vis spectrophotometry. Tessier’s sequential extraction method was used to measure the contents of five different forms of Cd, namely the exchangeable component (EX), the carbonate-bound Cd fraction (CB), the Fe/Mn oxide-bound Cd fraction (OX), the organic-matter-bound Cd fraction (OM), and the residual Cd fraction (RS) in soil (Tessier et al., 1979). After centrifugation and filtration of the suspension at each extraction stage, the extract was examined by AAS (ContrAA800, Analytik Jena AG, Germany) and inductively coupled plasma mass spectrometry (ICPMS; ICAP RQ, United States). The correlation coefficient of the standard plot was >0.999, and three parallel measurements were performed on each soil sample.
2.3 DNA extraction, PCR assay, and high-throughput illumina sequencing
Total genomic DNA was extracted from approximately 5 g of soil using the PowerSoil DNA Isolation Kit (MoBio Laboratories Inc., United States), following the manufacturer’s specifications and stored at −70°C until further use. Samples were sent to Biomarker Co., Ltd. (Biomarks, Beijing, China) and sequenced with reference to the Illumina HiSeq platform (Illumina, San Diego, CA, United States). The raw data were classified and analyzed according to the standard process. Primers 338F/806R and ITS1F/ITS2R were used to amplify the V3-V4 and ITS1 regions of bacterial and fungal DNA, respectively. The polymerase chain reaction (PCR) amplification procedure was performed as follows: initial denaturation at 95°C for 5 min, followed by 35 cycles of denaturation at 95°C for 30 s, annealing at 55°C for 30 s, extension at 72°C for 1 min, 35 cycles, and finally cooling to 4°C. Thereafter, 4 μL of 5× TransStart FastPfu buffer, 2 μL of 2.5 mm dNTPs (5 μm per primer), 0.4 μL of TransStart FastPfu DNA polymerase, template DNA (10 ng), and doubly distilled H2O (up to 20 μL in three PCR runs) were added. The PCR product was extracted from 2% agarose gel, purified with an AxyPrep DNA gel extraction kit (Axygen Biosciences, Union City, CA, United States) according to the manufacturer’s instructions, and then purified with Quantus™. Quantification was performed with a fluorescence analyzer (Promega, United States). MiSeq sequencing was conducted at Majorbio Biomedical Technology Co., Ltd., in Shanghai, using the Illumina MiSeq PE300 platform. Sequence data were stored in the NCBI Sequence Read Archive (SRA) database.
2.4 Statistical analyses
Coverage, richness (Chao and ACE indices), and diversity (Shannon and Simpson indices) were used to estimate the alpha diversity of each sample. Principal component analysis (PCA) and redundancy analysis (RDA) were performed using Canoco 5.0. To explore co-occurrence patterns of bacterial and fungal phyla and five different Cd fractions, Spearman’s correlations were analyzed through an online website (https://www.omicstudio.cn). The data of soil physiochemical properties are presented as mean ± standard deviation. Analysis of variance and Pearson’s correction analysis were performed using SPSS statistical software (version 17.0, IBM SPSS Inc.).
3 Results
3.1 Changes in soil physical and chemical properties and soil cadmium fractions
Each treatment had significant effects on soil physicochemical indices (Table 1). Compared with CK, the pH after treatments BD, PD, and SD decreased by 6.52%, 7.82%, and 7.94%, respectively. EC, OM, AN, AP, AK, ECa, and EMg were significantly increased following treatments BD, PD, SD, and AD. In particular, BD significantly increased EC, AK, and ECa, while SD significantly increased AN, AP, EMg, and CEC.
The EX-Cd contents following treatments SD and AD were significantly lower than that in the case of CK by 25.4% and 23.7%, respectively, and the OX-Cd content following SD was 30.0% higher than that in the case of CK (P < 0.05). Treatment PD significantly increased the EX-Cd content compared with CK (P < 0.05). There were no significant differences in the contents of CB-Cd, OM-Cd, and RS-Cd components among the treatments (P > 0.05) (Figure 1).
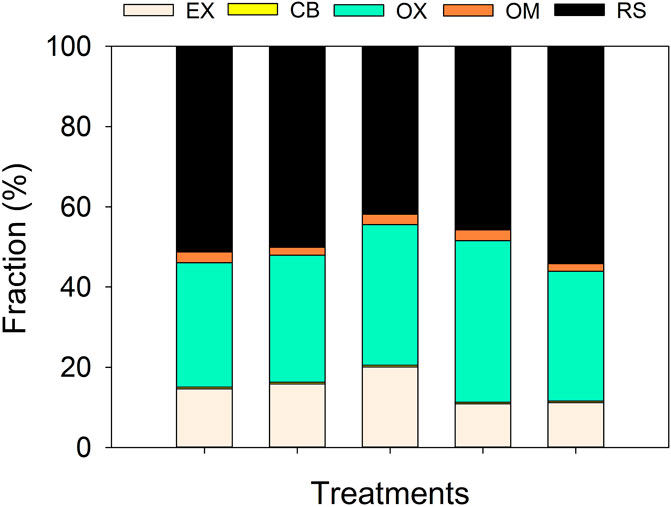
Figure 1. Variation of five Cd fractions in all treatments. EX, exchangeable Cd fraction; CB, carbonate-bound Cd fraction; OX, Fe/Mn oxide-bound Cd fraction; OM, organic-matter-bound Cd fraction; RS, residual Cd fraction.
Correlation analysis showed that EX-Cd was significantly positively correlated with EC and negatively correlated with AP (P < 0.05, Figure 2). RS-Cd was significantly positively correlated with ECa. CB-Cd was significantly negatively correlated with OM, AP, CEC, AN, and EMg, while significantly positively correlated with pH. OM-Cd was significantly negatively correlated with ECa, AK, and EC. OX-Cd was significantly negatively correlated with ECa, Cd and EC, but significantly positively correlated with AP.
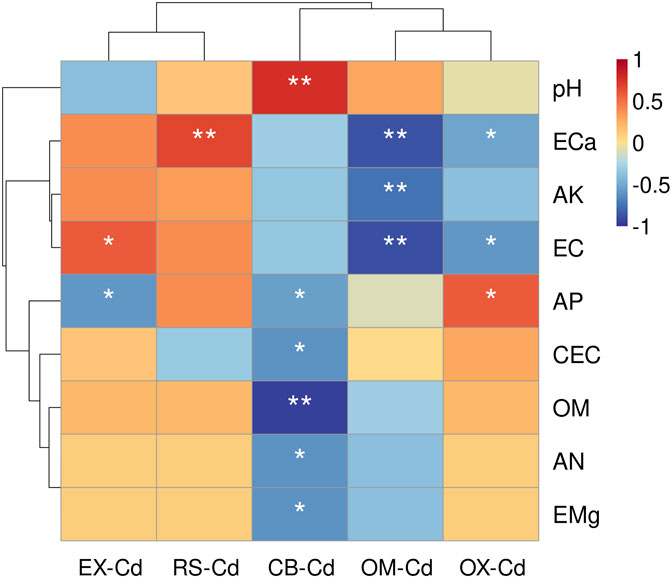
Figure 2. Pearson’s correlation analysis between environmental parameters and the Cd of five fractions. *Correlation is significant at the 0.05 level, **Correlation is significant at the 0.01 level. EX, exchangeable Cd fraction; CB, carbonate-bound Cd fraction; OX, Fe/Mn oxide-bound Cd fraction; OM, organic-matter-bound Cd fraction; RS, residual Cd fraction.
3.2 Effects of different RSD treatments on soil microbial communities
Totals of 1,600,693 and 1,600,225 high-quality sequences of bacterial 16S rRNA and fungal ITS were obtained by Illumina NovaSeq sequencing. The alpha diversity index of each treatment was statistically analyzed. At the 97% similarity level, the coverage of each treatment was above 99.7%, indicating sufficient sequencing depth. The operational taxonomic units (OTU) and the ACE and Chao1 indices of soil bacterial communities following BD, PD, and SD treatments were significantly lower than those in the case of CK (p > 0.05) (Table 2). SD was the highest in fungal community (Table 3).
Compared with CK, different organic material treatments significantly regulated the composition of soil bacterial and fungal communities at the phylum and genus levels (Figures 3, 4). At the bacterial phylum level (Figure 3A), BD, PD, and SD significantly increased the relative abundances of Bacteroidetes, Chloroflexi, and Deinococcus Thermus, while those of Actinobacteria, Acidobacteria, and Patescibactera were significantly decreased. At the genus level (Figure 3B), BD, PD, and SD significantly increased uncultured-bacterium_f-anaerobic-bacterium-MO-CFX2, uncultured-bacterium_f-Pseudomonadaceae, uncultured-bacterium_f-Rhodothermaceae, Luteimonas (Abyssal Vine Yellow Monascus), and uncultured-bacterium_o-SBR1031, while Chryseolinea, uncultured-bacterium_cSubsgroup_6, and uncultured-bacterium_cSubsgroup_6 um_cAlphaproteobject were slightly decreased. At the phylum level (Figure 4A), the relative abundances of Basidiomycota following BD, PD, and SD treatments were increased, while the relative abundances of Ascomycota and Rozellomycota were decreased. In terms of fungal genus level (Figure 4B), the relative abundances of Acremonium and Mortierella following BD, PD, and SD treatments increased, while the relative abundances of Botryotinia and Remersonia decreased.
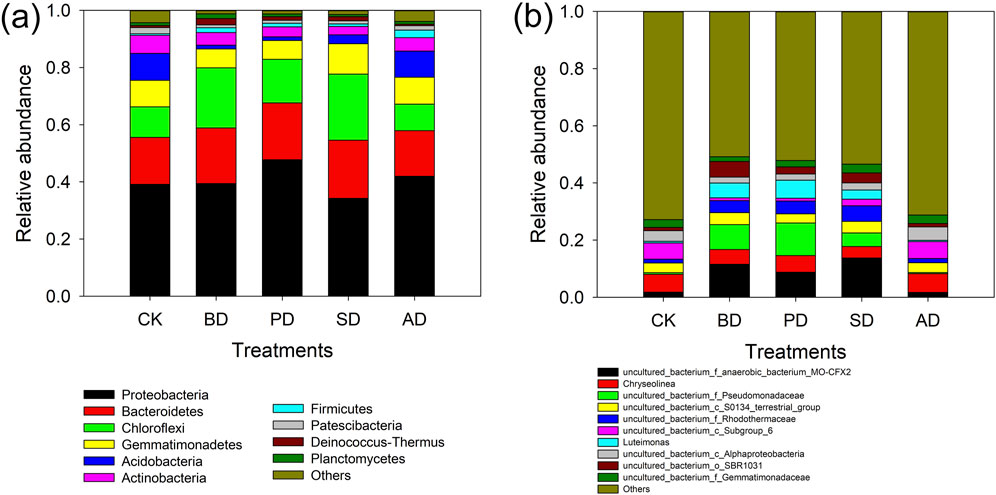
Figure 3. Taxonomic composition of bacterial communities. (A) Classification composition of soil bacterial communities at phylum level under different treatments; (B) taxonomic composition of soil bacterial communities at genus level under different treatments.
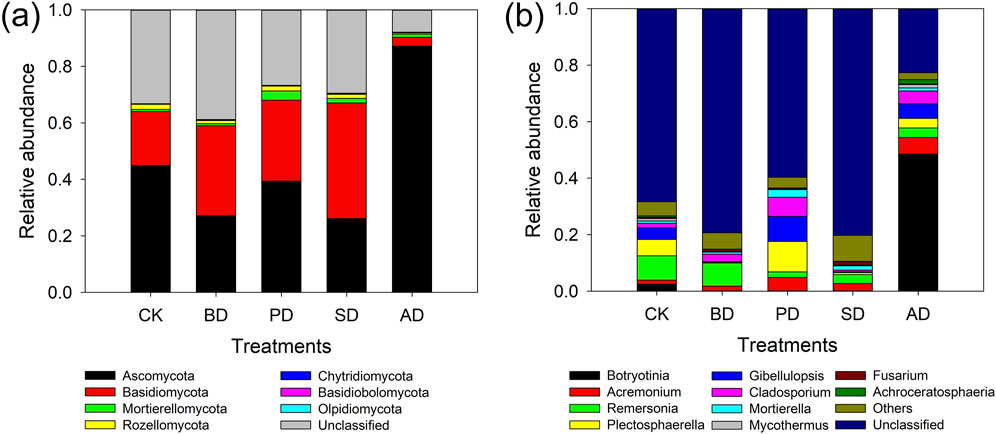
Figure 4. Taxonomic composition of fungal community. (A) Taxonomic composition of soil fungal communities at phylum level under different treatments; (B) taxonomic composition of soil fungal communities at genus level under different treatments.
3.3 Correlation between soil physicochemical composition or Cd fraction and soil microbial community composition at the phylum and genus levels
RDA was used to analyze the correlations between soil environmental variables and microbial community structure (Figure 5). In terms of environmental factors, pH was negatively correlated with other environmental factors. In terms of soil bacterial communities (Figure 5A), OM, EC, AK, and ECa were positively correlated with Planctomycetes, Firmicutes, and Deinococcus Thermus. CEC, AN, AP, and EMg were positively correlated with Bacteroidetes and Chloroflexi. There was a positive correlation between pH and Proteobacteria, Gemmatimonadetes, Acidobacteria, Actinobacteria, and Patescibacteria. In terms of soil fungal communities (Figure 5B), EC, OM, and ECa showed highly positive correlations with Basidiomycota, Mortierellomycota, and Rozellomycota. AP, AN, AK, EMg, and CEC were positively correlated with Basidiomycota and Olpidiomycota, but negatively correlated with Ascomycota. pH was positively correlated with Ascomycota and Chytridiomycota, and negatively correlated with other phylum species.
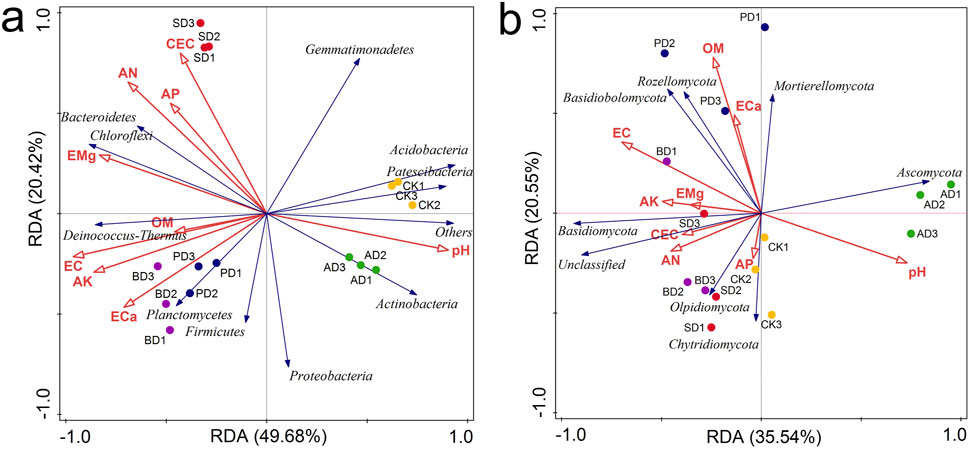
Figure 5. Redundancy analysis (RDA) at the phylum level. (A) RDA of bacterial communities based on relative abundances at phylum level and soil properties of individual samples; (B) RDA of fungal communities based on relative abundances at phylum level and soil properties of individual samples. Each value represents the mean of three replicates.
The 11 phyla of Bacteriae were divided into four subclasses (Figure 6A), among which subclass 1 was negatively correlated with RS-Cd, while subclasses 2 and 4 were positively correlated with RS-Cd. Gemmatimonadetes showed a significant negative correlation with EX-Cd, while Proteobacteria showed a significant positive correlation with EX-Cd. Firmicutes were negatively correlated with OM-Cd. OX-Cd was significantly positively correlated with Chloroflexi and negatively correlated with Actinobacteria. As regards the phyla (Figure 6B), RS-Cd was significantly negatively correlated with Basidiomycota and positively correlated with Ascomycota. EX-Cd was positively correlated with Basidiobolomycota. OX-Cd was significantly positively correlated with Basidiomycota and negatively correlated with Ascomycota.
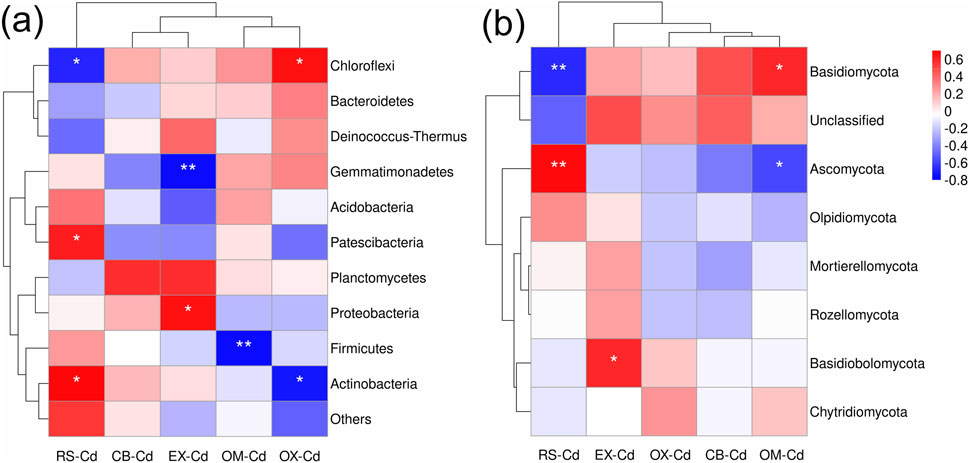
Figure 6. Heat maps of the Spearman correlation coefficient. (A) Heat map of the Spearman correlation coefficient between Cd fractions and abundant bacterial phyla; (B) heat map of the Spearman correlation coefficient between Cd fraction and abundant fungal phyla. UC denotes unclassified.
3.4 Plant biomass and element uptake status
As can be seen from Figures 7, 8, the addition of all organic materials significantly decreased the biomass of lettuce (P < 0.05), with decreases in the range 14.31%–83.77%. Treatment SD showed the smallest decline.
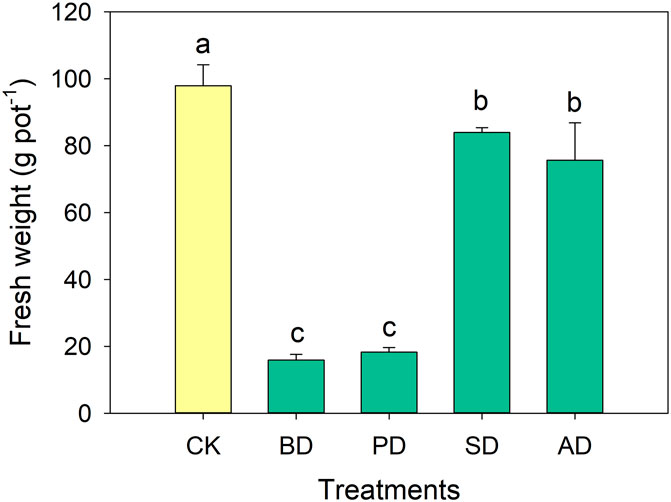
Figure 7. Comparison of lettuce biomass among different treatments. Error bars indicate the standard deviations of the means of four replicates. Different letters represent significant differences at P < 0.05 according to Duncan’s multiple range test.
The contents of the main elements in the ground parts following each treatment were analyzed (Figure 9). For Cd (Figure 9A), the contents after treatment were: AD (2.65 g kg–1) > CK (2.33 g kg–1) > SD (0.59 g kg–1) > BD (0.24 g kg–1) > PD (0.33 g kg–1). Thus, treatments BD, PD, and SD reduced the Cd content by 89.75%, 85.91%, and 74.76%, respectively, compared with CK. Each treatment significantly increased the P content in lettuce compared with CK (Figure 9B), by 46.71%, 79.05%, 33.84%, and 38.41% with BD, PD, SD, and AD, respectively. The Fe content following AD treatment increased compared with that in the case of CK (Figure 9C), whereas the other treatments decreased it by 20.09%–55.02%. Compared with CK, treatments BD, PD, and SD significantly increased the Ca contents by 338.02%, 363.02%, and 92.19% (P < 0.05), respectively (Figure 9D). The trends in Mg and Ca contents following each treatment were similar (Figure 9E). Treatments BD, PD, and SD significantly increased the Mg contents by 212.26%, 226.32%, and 117.53%, respectively. The contents of Fe and Cu following each treatment showed no significant changes (Figures 9F, G). There was no significant difference between BD and CK, except that Zn content was increased following BD treatment (Figure 9F).
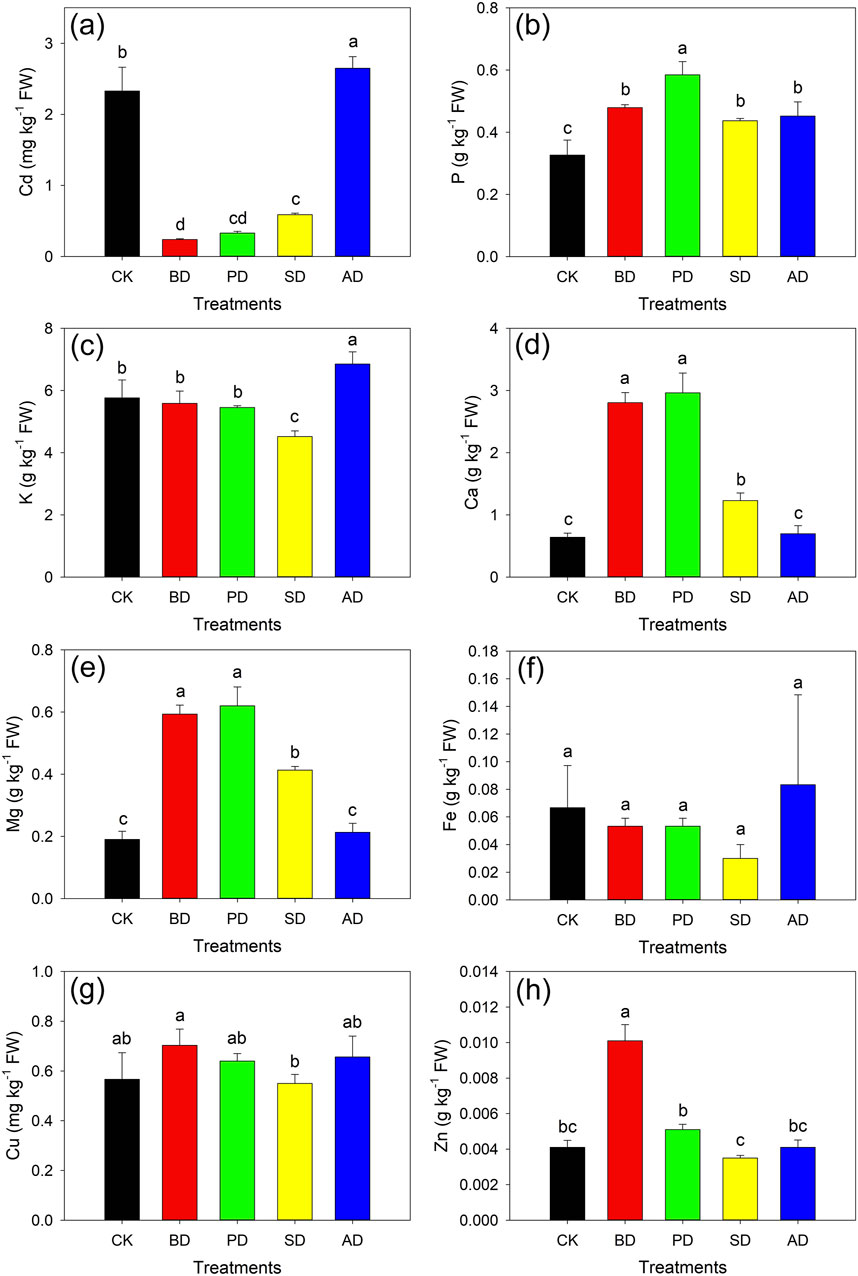
Figure 9. Contents of main elements in lettuce. (A) Cd; (B) P; (C) K; (D) Ca; (E) Mg; (F) Fe; (G) Cu; (H) Zn. Different letters represent significant differences at P < 0.05 according to Duncan’s multiple range test.
4 Discussion
4.1 Relationship between soil physicochemical parameters changed by RSD treatment and Cd composition
By adding organic matter and subjecting mixtures to anaerobic fermentation, RSD can significantly change the physical and chemical properties of soil in a short time (Zhu et al., 2022). The physical and chemical properties of soil are closely related to the Cd content, and pH has been found to be one of the key influencing factors that seriously affects its immobilization process (Chen et al., 2019; Muhammad et al., 2012). In this study, with the exception of AD, the other three RSD treatments reduced the pH of alkaline soil (Table 1), most notably SD treatment. This is contrary to previous results of a pH increase after RSD treatment of acidic soil (Li et al., 2021; Meng et al., 2019). In accordance with previous studies, it was found that the change of soil pH after RSD treatment depended on its original pH. That is to say, after RSD treatment, the pH of a soil with an initially high value (pH > 6.0) tended to decrease (Momma et al., 2006; Huang et al., 2015), whereas that of a soil with an initially low value (pH < 5.0) tended to increase (Butler et al., 2012; Butler et al., 2014; Meng et al., 2019). Anaerobic decomposition of organic matter to produce organic acids may lead to a decrease in pH, especially in sandy soils with low buffering capacity (Butler et al., 2012). Therefore, RSD plays an important role in regulating soil pH. OM was seen to be significantly negatively correlated with CB-Cd. Shen et al. (2020) also proved that after adding organic fertilizer, the redox state of soil changed, and the Cd contents of EX-Cd, CB-Cd, and other components decreased significantly. It was further found that soil pH significantly affected the differences in soil enzyme activity and bacterial community or function, which eventually led to changes in soil Cd immobilization and Fe or S reaction (Wang et al., 2020). Suleiman et al. (2013) found that changes in soil pH could directly or indirectly affect many soil characteristics (such as nutrient availability, cationic metal solubility, and organic carbon characteristics), and that these factors might further drive changes in microbial community composition. This is consistent with our finding that RSD reduces soil pH while also enhancing the availability of N, P, K, Ca, and Mg. The increase of macronutrients and their availability may stem directly from the anaerobic degradation of organic materials (Meng et al., 2019), or indirectly from the enhancement of nutrient cycling (Yang et al., 2021). Changes in soil chemical properties after RSD treatment may be related to soil background, the type of organic matter applied, and the application amount (Di Gioia et al., 2017). Therefore, we hypothesize that different types of organic materials in these experiments have different effects on soil properties (such as pH) and nutrient availability and total, as well as microbial communities, resulting in differences in lettuce growth and nutrient element contents.
4.2 Effects of microbial community changes on Cd speciation following RSD treatment
Microorganisms are an important part of soil ecosystems and a key factor in the functional stability and integrity of soil (Kennedy and Smith, 1995). In this study, we found that BD, PD, and SD treatments reduced the richness and diversity of bacteria, at variance with the results of previous studies (Yao et al., 2016). According to previous studies, the decrease in bacterial diversity may be caused by an increase in the proliferation of some dominant microorganisms (Huang et al., 2017). For example, some strains can produce compounds that are toxic to pathogens under anaerobic conditions, such as indole, cresol, and some other phenolic compounds (Mowlick et al., 2013). In contrast, the fungal diversity following BD, PD, and SD treatments increased significantly, which may have been due to an increase in some functional fungal populations, such as Basidiomycota members (Messiha et al., 2007). In addition, RSD led to marked changes in microbial community composition, which in this study were driven by changes in soil environmental factors such as pH, EC, TN, and AN. Previous studies have indicated that Fe(III) minerals can be reduced by Fe(III)-reducing bacteria (FeRB) (such as Firmicutes) (Burkhardt et al., 2010; Burkhardt al., 2011; Francis and Dodge, 1990; Muehe et al., 2013). Our study indicated that the abundances of Bacteroidetes, Firmicutes, Gemmatimonadetes, and Chloroflexi following RSD treatment were significantly higher than in the case of CK. Studies have shown that bio-bacterial fertilizer can effectively alleviate Cd toxicity and promote the growth of beneficial groups such as Proteobacteria, Bacteroidetes, Gemmatimonadetes, and Firmicutes (Wang M. et al., 2019), thereby significantly increasing rice biomass and reducing Cd concentration in tissues. These results suggest that microorganisms involved in Firmicutes and Gemmatimonadetes may partake in the fixation of Cd in co-contaminated soil by promoting the reduction of Fe(III) after RSD treatment or resisting Cd, which is similar to the conclusion of Li et al. (2021). Furthermore, RDA showed pH to be an important factor affecting microbial community changes (Figure 5), consistent with the results of Kim et al. (Kim et al., 2016), indicating that soil pH is strongly correlated with microbial richness and diversity. Interestingly, we found Gemmatimonadetes to be significantly negatively correlated with EX-Cd (Figure 6), further suggesting that it may have a positive effect on the fixation of Cd in contaminated soil, which was also surmised by Wang M. et al. (2019).
5 Conclusion
In general, the four organic materials selected for these experiments all reduced the pH of alkaline soil, increased the soil organic matter, and increased the availability of the pertinent elements. SD and AD treatments significantly reduced the EX-Cd contents in soil by 25.4% and 23.7%, respectively. SD treatment significantly increased the number of soil fungal OTU, and Gemmatimonadetes showed a significant negative correlation with EX-Cd, indicating that it may have a positive effect on Cd fixation in contaminated soil. SD treatment significantly reduced the Cd content of lettuce by 74.76%, without affecting the crop yield. This study has shown that RSD can reduce Cd activity by improving soil characteristics and changing microbial community composition. In particular, SD treatment reduced Cd accumulation with no reduction in lettuce crop yield, offering good application prospects.
Data availability statement
The original contributions presented in the study are included in the article/supplementary material, further inquiries can be directed to the corresponding author.
Author contributions
KS: Data curation, Funding acquisition, Investigation, Methodology, Writing–original draft, Writing–review and editing. SZ: Formal Analysis, Resources, Writing–review and editing. JW: Software, Supervision, Writing–review and editing.
Funding
The author(s) declare that financial support was received for the research, authorship, and/or publication of this article. This work was supported by Shandong Province technology innovation guidance program [YDZX2023007]; China Agriculture Research System [CARS-23-B18]; China Agriculture Research System [CARS-23-G13]; Key Research and Development Technologies of Shandong Province (Major Science and Technology Innovation Project) [2023CXGC010702]; Provincial Modern Agricultural Industry Technology System [SDAIT-05].
Conflict of interest
The authors declare that the research was conducted in the absence of any commercial or financial relationships that could be construed as a potential conflict of interest.
Publisher’s note
All claims expressed in this article are solely those of the authors and do not necessarily represent those of their affiliated organizations, or those of the publisher, the editors and the reviewers. Any product that may be evaluated in this article, or claim that may be made by its manufacturer, is not guaranteed or endorsed by the publisher.
References
Blok, W. J., Lamers, J. G., Termorshuizen, A. J., and Bollen, G. J. (2000). Control of soilborne plant pathogens by incorporating fresh organic amendments followed by tarping. Phytopathology 90 (3), 253–259. doi:10.1094/PHYTO.2000.90.3.253
Burkhardt, E. M., Akob, D. M., Bischoff, S., Sitte, J., Kostka, J. E., Banerjee, D., et al. (2010). Impact of biostimulated redox processes on metal dynamics in an iron-rich creek soil of a former uranium mining area. Environ. Sci. Technol. 44, 177–183. doi:10.1021/es902038e
Burkhardt, E. M., Bischoff, S., Akob, D. M., Büchel, G., and Küsel, K. (2011). Heavy metal tolerance of Fe(III)-reducing microbial communities in contaminated creek bank soils. Appl. Environ. Microbiol. 77, 3132–3136. doi:10.1128/AEM.02085-10
Butler, D., Kokalis-Burelle, N., Albano, J., McCollum, T., Muramoto, J., Shennan, C., et al. (2014). Anaerobic soil disinfestation (ASD) combined with soil solarization as a methyl bromide alternative: vegetable crop performance and soil nutrient dynamics. Plant Soil 378, 365–381. doi:10.1007/s11104-014-2030-z
Butler, D. M., Kokalis-Burelle, N., Muramoto, J., Shennan, C., McCollum, T. G., and Rosskopf, E. N. (2012). Impact of anaerobic soil disinfestation combined with soil solarization on plant–parasitic nematodes and introduced inoculum of soilborne plant pathogens in raised-bed vegetable production. Crop Prot. 39, 33–40. doi:10.1016/j.cropro.2012.03.019
Chen, Y., Ma, J., Li, Y., and Weng, L. (2019). Enhanced cadmium immobilization in saturated media by gradual stabilization of goethite in the presence of humic acid with increasing pH. Sci. Total Environ. 648, 358–366. doi:10.1016/j.scitotenv.2018.08.086
Cheng, C., Han, H., Wang, Y., He, L., and Sheng, X. (2020). Metal-immobilizing and urease-producing bacteria increase the biomass and reduce metal accumulation in potato tubers under field conditions. Ecotoxicol. Environ. Saf. 203, 111017. doi:10.1016/j.ecoenv.2020.111017
Dermont, G., Bergeron, M., Mercier, G., and Richer-Lafleche, M. (2008). Soil washing for metal removal: a review of physical/chemical technologies and field applications. J. Hazard Mater. 152, 1e31–31. doi:10.1016/j.jhazmat.2007.10.043
Di Gioia, F., Ozores-Hampton, M., Zhao, X., Thomas, J., Wilson, P., Li, Z. N., et al. (2017). Anaerobic soil disinfestation impact on soil nutrients dynamics and nitrous oxide emissions in fresh-market tomato. Agric. Ecosyst. Environ. 240, 194–205. doi:10.1016/j.agee.2017.02.025
Francis, A. J., and Dodge, C. J. (1990). Anaerobic microbial remobilization of toxic metals coprecipitated with iron oxide. Environ. Sci. Technol. 24, 373–378. doi:10.1021/es00073a013
Guo, G., Zhou, Q., and Ma, L. Q. (2006). Availability and assessment of fixing additives for the in situ remediation of heavy metal contaminated soils: a review. Environ. Monit. Assess. 116, 513–528. doi:10.1007/s10661-006-7668-4
Han, H., Wu, X., Hui, R., Xia, X., Chen, Z., Yao, L., et al. (2022). Synergistic effects of Cd-loving bacillus sp. n3 and iron oxides on immobilizing Cd and reducing wheat uptake of Cd. Environ. Pollut. 305, 119303. doi:10.1016/j.envpol.2022.119303
Huang, F., Zhou, H., Gu, J., Liu, C., Yang, W., Liao, B., et al. (2020). Differences in absorption of cadmium and lead among fourteen sweet potato cultivars and health risk assessment. Ecotoxicol. Environ. Saf. 203, 111012. doi:10.1016/j.ecoenv.2020.111012
Huang, G., Huang, Y., Hu, H., Liu, F., Zhang, Y., and Deng, R. (2015). Remediation of nitrate-nitrogen contaminated groundwater using a pilot-scale two-layer heterotrophic-autotrophic denitrification permeable reactive barrier with spongy iron/pine bark. Chemosphere 130, 8–16. doi:10.1016/j.chemosphere.2015.02.029
Huang, X., Cui, H., Yang, L., Lan, T., Zhang, J., and Cai, Z. (2017). The microbial changes during the biological control of cucumber damping-off disease using biocontrol agents and reductive soil disinfestation. Biocontrol 62 (1), 97–109. doi:10.1007/s10526-016-9768-6
Hussain, B., Lin, Q., Hamid, Y., Sanaullah, M., Di, L., Hashmi, M., et al. (2020). Foliage application of selenium and silicon nanoparticles alleviates Cd and Pb toxicity in rice (Oryza sativa L.). Sci. Total Environ. 712, 136497. doi:10.1016/j.scitotenv.2020.136497
Kennedy, A. C., and Smith, K. L. (1995). Soil microbial diversity and the sustainability of agricultural soils. Plant Soil 170, 75–86. doi:10.1007/BF02183056
Kim, J., Roh, A., Choi, S., Kim, E., Choi, M., Ahn, B., et al. (2016). Soil pH and electrical conductivity are key edaphic factors shaping bacterial communities of greenhouse soils in Korea. J. Microbiol. 54, 838–845. doi:10.1007/s12275-016-6526-5
Li, X., Li, X., Li, Y., Dai, X., Zhang, Q., Zhang, M., et al. (2021). Improved immobilization of soil cadmium by regulating soil characteristics and microbial community through reductive soil disinfestation. Sci. Total. Environ. 778, 146222. doi:10.1016/j.scitotenv.2021.146222
Liu, L., Huang, X., Zhao, J., Zhang, J., and Cai, Z. (2019). Characterizing the key agents in a disease-suppressed soil managed by reductive soil disinfestation. Appl. Environ. Microbiol. 85, 029922–e3018. doi:10.1128/AEM.02992-18
Martin, T. A., and Ruby, M. V. (2010). Review of in situ remediation technologies for lead, zinc, and cadmium in soil. Remediat. J. 14 (3), 35–53. doi:10.1002/rem.20011
Meng, T., Ren, G., Wang, G., and Ma, Y. (2019). Impacts on soil microbial characteristics and their restorability with different soil disinfestation approaches in intensively cropped greenhouse soils. Appl. Microbiol. Biotechnol. 103 (15), 6369–6383. doi:10.1007/s00253-019-09964-z
Messiha, N. A. S., van Diepeningen, A. D., Wenneker, M., van Beuningen, A. R., Janse, J. D., Coenen, T. G. C., et al. (2007). Biological Soil Disinfestation (BSD), a new control method for potato brown rot, caused by Ralstonia solanacearum race 3 biovar 2. Eur. J. Plant Pathol. 117, 403–415. doi:10.1007/s10658-007-9109-9
Ministry of Environmental Protection, Ministry of Land and Resources of the People’s Republic of China (2014). Report on the national general survey of soil contamination. Available at: http://english.mep.gov.cn/ (Accessed April 28, 2014).
Momma, N., Kobara, Y., Uematsu, S., Kita, N., and Shinmura, A. (2013). Development of biological soil disinfestations in Japan. Appl. Microbiol. Biotechnol. 97, 3801–3809. doi:10.1007/s00253-013-4826-9
Momma, N., Yamamoto, K., Simandi, P., and Shishido, M. (2006). Role of organic acids in the mechanisms of biological soil disinfestation (BSD). J. Gen. Plant Pathol. 72 (4), 247–252. doi:10.1007/s10327-006-0274-z
Mowlick, S., Inoue, T., Takehara, T., Kaku, N., Ueki, K., and Ueki, A. (2013). Changes and recovery of soil bacterial communities influenced by biological soil disinfestation as compared with chloropicrin-treatment. Amb. Express 3 (1), 46–12. doi:10.1186/2191-0855-3-46
Muehe, E. M., Adaktylou, I. J., Obst, M., Zeitvogel, F., Behrens, S., Planer-Friedrich, B., et al. (2013). Organic carbon and reducing conditions lead to cadmium immobilization by secondary Fe mineral formation in a pH-neutral soil. Environ. Sci. Technol. 47, 13430–13439. doi:10.1021/es403438n
Muhammad, I., Puschenreiter, M., and Wenzel, W. W. (2012). Cadmium and Zn availability as affected by pH manipulation and its assessment by soil extraction, DGT and indicator plants. Sci. Total Environ. 416, 490–500. doi:10.1016/j.scitotenv.2011.11.029
Mulligan, C. N., Yong, R. N., and Gibbs, B. F. (2001). Remediation technologies for metal-contaminated soils and groundwater: an evaluation. Eng. Geol. 60 (1), 193–207. doi:10.1016/s0013-7952(00)00101-0
Nareshkumar, R., Nagendran, R., and Parvathi, K. (2008). Bioleaching of heavy metals from contaminated soil using Acidithiobacillus thiooxidans: effect of sulfur/soil ratio. World J. Microb. Biot. 24 (8), 1539–1546. doi:10.1007/s11274-007-9639-5
Ogunkunle, C. O., Gambari, H., Agbaje, F., Okoro, H. K., Asogwa, N. T., Vishwakarma, V., et al. (2020). Effect of low-dose nano titanium dioxide intervention on Cd uptake and stress enzymes activity in Cd-stressed cowpea [Vigna unguiculata (L.) Walp] plants. Bull. Environ. Contam. Toxicol. 104, 619–626. doi:10.1007/s00128-020-02824-x
Shen, B., Wang, X., Zhang, Y., Zhang, M., Wang, K., Xie, P., et al. (2020). The optimum pH and Eh for simultaneously minimizing bioavailable cadmium and arsenic contents in soils under the organic fertilizer application. Sci. Total Environ. 711, 135229. doi:10.1016/j.scitotenv.2019.135229
Shi, X., Zhou, G., Liao, S., Shan, S., Wang, G., and Guo, Z. (2018). Immobilization of cadmium by immobilized Alishewanella sp. WH16-1 with alginate-lotus seed pods in pot experiments of Cd-contaminated paddy soil. J. Hazard. Mater. 357, 431–439. doi:10.1016/j.jhazmat.2018.06.027
Suleiman, A. K. A., Manoeli, L., Boldo, J. T., Pereira, M. G., and Roesch, L. F. W. (2013). Shifts in soil bacterial community after eight years of land-use change. Syst. Appl. Microbiol. 36, 137–144. doi:10.1016/j.syapm.2012.10.007
Sun, K. N., Wen, D., Yang, N., Wang, K. A., Li, X. H., and Yu, L. (2019). Heavy metal and soil nutrient accumulation and ecological risk assessment of vegetable fields in representative facilities in Shandong Province, China. Environ. Monit. Assess. 191 (4), 240. doi:10.1007/s10661-019-7396-1
Tessier, A., Campbell, P. G. C., and Bisson, M. (1979). Sequential extraction procedure for the speciation of particulate trace metals. Anal. Chem. 51 (7), 844–851. doi:10.1021/ac50043a017
Wang, M., Chen, S., Chen, L., and Wang, D. (2019b). Responses of soil microbial communities and their network interactions to saline-alkaline stress in Cd-contaminated soils. Environ. Pollut. 252, 1609–1621. doi:10.1016/j.envpol.2019.06.082
Wang, M., Chen, S., Chen, L., and Wang, D. (2020). Microbial mechanisms responsible for the variation of soil Cd availability under different pe+pH environments. Ecotoxicol. Environ. Saf. 206, 111057. doi:10.1016/j.ecoenv.2020.111057
Wang, Y., Yang, R., Zheng, J., Shen, Z., and Xu, X. (2019a). Exogenous foliar application of fulvic acid alleviate cadmium toxicity in lettuce (Lactuca sativa L.). Ecotoxicol. Environ. Saf. 167, 10–19. doi:10.1016/j.ecoenv.2018.08.064
Yang, R., Weiner, J., Shi, X., Wang, Y., Zhang, R., and Zhu, M. (2021). Effect of reductive soil disinfestation on the chemical and microbial characteristics of rhizosphere soils associated with salvia miltiorrhiza production in three cropping systems. Appl. Soil Ecol. 160, 103865. doi:10.1016/j.apsoil.2020.103865
Yang, W., Wang, S., Zhou, H., Zeng, M., Zhang, J., Huang, F., et al. (2022). Combined amendment reduces soil Cd availability and rice Cd accumulation in three consecutive rice planting seasons. J. Environ. Sci. 111 (01), 141–152. doi:10.1016/j.jes.2021.03.027
Yao, Y., Xue, Z., Hong, C., Zhu, F. X., Chen, X., Wang, W., et al. (2016). Efficiency of different solarization-based ecological soil treatments on the control of fusarium wilt and their impacts on the soil microbial community. Appl. Soil Ecol. 108, 341–351. doi:10.1016/j.apsoil.2016.09.015
Zhao, K., Liu, X., Xu, J., and Selim, H. M. (2010). Heavy metal contaminations in a soil-rice system: identification of spatial dependence in relation to soil properties of paddy fields. J. Hazard. Mater. 181 (1-3), 778–787. doi:10.1016/j.jhazmat.2010.05.081
Zhong, W., Gu, T., Wang, W., Zhang, B., Lin, X., Huang, Q., et al. (2010). The effects of mineral fertilizer and organic manure on soil microbial community and diversity. Plant Soil 326, 511–522. doi:10.1007/s11104-009-9988-y
Keywords: lettuce, cadmium, reductive soil disinfestation, soil microbial community, facility soil
Citation: Sun K, Zhang S and Wang J (2024) Regulating facility soil microbial community and reducing cadmium enrichment in lettuce by reductive soil disinfestation. Front. Environ. Sci. 12:1465882. doi: 10.3389/fenvs.2024.1465882
Received: 17 July 2024; Accepted: 02 September 2024;
Published: 08 October 2024.
Edited by:
Jun Zhou, Chinese Academy of Sciences (CAS), ChinaReviewed by:
Guan Bo, Ludong University, ChinaGuangmei Wang, Chinese Academy of Sciences (CAS), China
Zongqiang Wei, Jiangxi Agricultural University, China
Copyright © 2024 Sun, Zhang and Wang. This is an open-access article distributed under the terms of the Creative Commons Attribution License (CC BY). The use, distribution or reproduction in other forums is permitted, provided the original author(s) and the copyright owner(s) are credited and that the original publication in this journal is cited, in accordance with accepted academic practice. No use, distribution or reproduction is permitted which does not comply with these terms.
*Correspondence: Junfeng Wang, bWFudGlkQDE2My5jb20=