- 1Business School, Hohai University, Nanjing, China
- 2College of Economics and Management, Nanjing Forestry University, Nanjing, China
- 3Jiangsu Culture Innovation Development Co., Ltd., Nanjing, China
- 4Jiangsu Province Science and Technology System Reform Think Tank, Nanjing, China
Introduction: The recycling and repurposing of industrial solid waste is a crucial element of sustainable development in growing industrialized nations.
Methods: An advanced two-stage Data Envelopment Analysis (DEA) model was employed to assess the effectiveness of solid waste management across all 31 provinces in China throughout the period spanning from 2016 to 2022.
Results: The research findings suggest the following: (1) The circular economy has shown varying degrees of improvement in efficiency across most regions. (2) The average efficiency of the resource reuse stage is not higher than 0.4, indicating significant room for development that requires serious attention. (3) The western region has a higher average solid waste treatment efficiency of 0.65 compared to 0.53 in the eastern region, while the central region falls at a moderate level. Furthermore, this work employed sensitivity analysis to examine the resilience of regional efficiency research and discovered that the overall findings remain statistically significant. Hence, it is imperative to simultaneously enhance the internal administration of businesses and enhance governmental environmental legislation and regulations, with the aim of attaining the utmost optimization of resource recycling.
Discussion: This paper presents policy suggestions for enhancing the solid waste recycling system within provincial government departments, while also establishing the foundation for the expansion of the solid waste treatment industry, which is necessary to accomplish the goal of “double carbon”.
1 Introduction
The combination of industrial development and population growth has presented established and rising nations with a twofold challenge of limited resources and the need for pollution management. Addressing these issues necessitates global collaboration and collective action (Li et al., 2020). Hence, the establishment of an efficient and eco-conscious supply chain is a widely shared objective on a global scale. China’s fast industrialization in recent decades has resulted in climate change, disruption of ecosystems, and shortage of natural resources (Albores et al., 2016). Efficient waste disposal and recycling are crucial for addressing the environmental issues that arise from industrial activity. The waste generated by economic development is mainly industrial solid waste, which includes smelting waste, slag, and electronic waste. These wastes mainly come from heavily polluting industries such as the power industry, iron and steel industry, and manufacturing industry. Among them, electronic waste is currently one of the fastest growing types of waste (Quan et al., 2024). Industrial wastes have the potential to be partially recycled and used as a valuable resource after undergoing treatment. Hence, effective waste recycling management is particularly crucial for the sustainability of China.
To effectively manage and recycle the different types of waste generated throughout industrial production operations, it is essential to accurately categorize them. According to the characteristics of pollution, solid waste can be divided into general industrial solid waste (GISW) and hazardous waste. GISW refers to industrial solid waste that is not listed in the National Catalogue of Hazardous Wastes1 and does not have hazardous characteristics according to the national identification standards and methods. The main source is GISW. GISW encompasses non-hazardous waste generated by several industries, including industrial production, transportation, post and telecommunications. Hazardous waste typically exhibits one or more hazardous traits, including toxicity, corrosiveness, flammability, reactivity, or infectivity2 (Tang et al., 2024). Details on the types and industrial sources of industrial solid waste are shown in Supplementary Appendix SA1. Based on the most recent data from the Ministry of Ecology and Environment of China, the output of conventional industrial solid waste rose from 3.97 billion tons to 4.11 billion tons between 2021 and 2022. However, the comprehensive utilization rate experienced a decline, dropping from 61% to 57.66%. This suggests that the issue of ineffective processing remains present.
In 2019, the output of industrial hazardous waste increased to 81.26 million tons, up from 39.761 million tons in 2015. However, it decreased to 72.818 million tons for the first time in 2020. In 2020, it is important to mention that the usage and disposal of a certain product or resource surpassed its production for the first time. This could be attributed to the higher level of hazard associated with HISW (Guan et al., 2019), which has led to a growing focus on its appropriate disposal.
Countries worldwide have implemented environmental rules in response to the environmental issues caused by economic development and industrial output. These policies are based on the principles of circular economy. China’s developmental plan and environmental laws largely address pollution, waste management, and resource usage by adopting a circular economy approach (Li et al., 2010). Additionally, they embrace the practical business strategy of industrial symbiosis (Li et al., 2020). Despite the continuous efforts of the ecological environment department to enhance the legal system and innovate the environmental service mode, and the development of the environmental protection industry through collaboration with the science and technology department, the government still lacks appropriate methods to evaluate the efficiency and effectiveness of environmental policy. This is primarily due to the imperfect method (Sueyoshi et al., 2017), regional variations in circular economy efficiency (Li et al., 2010), and the scarcity of real-time data.
Regarding the solid waste treatment situation in different regions and cities in China. The Ministry of Ecology and Environment of China’s 2022 China Eco-environmental Statistics Annual Report reveals that Shanxi, Inner Mongolia, Hebei, Liaoning, and Shandong are the top five regions in terms of GISW production. These regions collectively produce 1.79 billion tons of waste, which accounts for 43.4% of the national GISW production. The provinces of Hebei, Shandong, Shanxi, Inner Mongolia, and Henan were the top five performers in the comprehensive usage of GISW. Together, they utilized a total of 910 million tons, which accounted for 38.2% of China’s total comprehensive utilization of GISW. Shanxi, Inner Mongolia, Liaoning, Hebei, and Shaanxi were the top five regions in terms of GISW disposal. Together, they had a total disposal capacity of 590 million tons, which accounted for 67.0% of the national capacity for GISW disposal.
The key to addressing environmental problems rests in waste disposal and recycling. Despite efforts to maximize resource efficiency and minimize pollutant output, pollution remediation remains crucial (Li et al., 2020). In the realm of circular economy, the effective usage of resources is intricately connected to the management of pollution. The purpose of this initiative is to efficiently utilize resources throughout the production phase, actively reduce environmental degradation, and attain the highest possible environmental advantages (Mardani et al., 2017). During the recycling stage, the goal is to optimize the use of resources and minimize the negative environmental effects of manufacturing by reusing industrial waste. However, most literature put forward suggestions on solid waste treatment from the perspective of solid waste treatment means (Su et al., 2021; Tang et al., 2020a; Wang et al., 2023; Wang and Cheng, 2024), and the research on efficiency in the recycling and reuse process is not yet fully mature. Especially, there is a lack of internal structural research to evaluate the efficiency of industrial waste treatment systems and the decomposition efficiency of pollution treatment and recovery subsystems.
In recent years, with the accelerated industrialization process and the deep-rooted concept of a circular carbon economy, environmental research in the field of industrial solid waste recycling has become a focus of global attention, attracting the attention of governments, international organizations and academia in many countries (Nogueira, 2023; Abdullah and Abedin, 2024; Ke et al., 2023; Neves et al., 2024). This trend is particularly pronounced in China, where the resource utilization rate of industrial solid waste has increased significantly, showing good momentum. However, the continuous improvement of the industrialization level of society is also accompanied by the aggravation of resource constraints, posing a severe challenge to the safe disposal strategy of industrial waste.
Kirchherr et al. (2017) pointed out that in the face of increasing waste production and limited natural resource reserves, a deeper understanding and balance between economic needs and environmental protection has become a key research topic that needs to be urgently addressed. The continuous and large-scale generation of industrial solid waste not only aggravates environmental damage, but also accelerates the depletion of natural resources. At present, most countries still rely on traditional methods of solid waste treatment, including open burning, landfilling, direct incineration, composting, and pyrolysis (Maturi et al., 2022). Although these methods have alleviated the problem of waste accumulation to some extent, their side effects should not be ignored. In Europe, for example, according to 2017 data, 23% of municipal solid waste was sent to landfills for disposal, which not only led to the generation and spread of harmful gases and leachate, but also caused serious environmental and health risks (Sauve and Van Acker, 2020).
It is worth noting that the current technology for the reuse and recycling of industrial solid waste faces many limitations, making it difficult to effectively curb the trend of environmental degradation. At the same time, traditional treatment methods are also unable to meet the long-term needs of sustainable development. Therefore, it is particularly urgent to conduct a comprehensive and in-depth exploration of the mechanism for the reuse and recycling of industrial solid waste. This process involves converting waste into reusable resources or energy. The evaluation of its efficiency has become a core indicator for measuring the development level in this field, which is directly related to the improvement of both economic benefits and environmental quality (Majchrowska et al., 2022). By optimizing the recycling process, we can not only significantly reduce the amount of solid waste produced and effectively alleviate the pressure on the environment, but also improve the efficiency of resource recycling, contributing to the harmonious coexistence of economy, society and environment.
At present, there have been many research results on efficiency evaluation at home and abroad. Kundariya et al. (2021) examined emerging strategies and monitoring tools for municipal solid waste treatment, discussed advanced technologies and innovations that cover the environmental and economic aspects of waste management technologies. Tsai et al. (2020) applied the entropy weight method to conduct systematic data-driven literature metrology analysis on municipal solid waste management, determine effective improvement indicators, and provide comparative data between regions. Bui et al. (2022) supplemented the knowledge of sustainable solid waste management and established an effective hierarchical model. It also provides a direction for practice. Chien et al. (2023) devised a structured hierarchy outlining sustainable solid waste interdependencies, transforming ambiguous and intricate features into quantifiable characteristics. Rodrigues et al. (2021) innovatively proposed a four-stage approach based on the AESA theory, aiming at accurately assessing its critical impact on systemic and Joule scales. Although scholars have recognized the importance of solid waste management for environmental protection, most of them focus on solid waste treatment and neglect the evaluation of its recycling and reuse process. In particular, the analysis of the internal structure of industrial waste treatment system is still immature in existing research, and further excavation is urgently needed.
Maximizing economic value with minimal investment and waste emissions is critical for economic systems, also known as ISW efficiency. Eco-efficiency is defined as the ability to produce products and services with little use of natural resources and negative environmental repercussions (Long et al., 2017; Oggioni et al., 2011). Previous studies have primarily assessed ecological efficiency at a single stage, with researchers focused solely on the industrial sectors that directly generate carbon emissions, frequently overlooking government services (Li et al., 2017). Despite the efforts of several researchers. Due to the need for new approaches, assessing the efficacy of these government practical solutions remains difficult (Sueyoshi and Goto, 2019), as do short implementation cycles and a lack of instant data. The influence of government supply of public goods and services on industry and overall efficiency warrants more investigation (De Souza et al., 2021). Many scholars investigated eco-efficiency in China, at the provincial, regional, and national levels (Liou and Wu, 2011).
Undoubtedly, the current research on solid waste treatment exhibits a clear inclination towards specific provinces. While the research object provides a comprehensive analysis, it fails to consider the interplay between economic zones. Furthermore, the aforementioned documents have a limited range of cut-in angles, primarily focusing on the management of solid waste while disregarding the comprehensive industrial waste treatment system. Although early study has been undertaken on the effectiveness of industrial solid waste treatment, there is a lack of in-depth research on regional disparities in China. Given the significant differences in economic development levels and resource conditions across China, it is especially important to conduct a thorough analysis of the efficiency of industrial solid waste treatment in each region, as this is critical for the precise formulation and implementation of appropriate policies. This also improves the precision of policy design and implementation.
The importance of effective management of solid waste for improving overall efficiency is self-evident. However, previous research has been limited to the evaluation of the efficiency of recycling and reuse processes, with a particular focus on the effectiveness of treatment technologies used at the beginning of industrial solid waste generation (De Souza et al., 2022; Luo et al., 2022). This research paradigm faces two core limitations: a single perspective and a lack of breadth; and it ignores the inherent connections and interactions between research objects. Specifically, current research generally exhibits a regional focus, that is, it is mainly concentrated on independent analysis within each province, ignoring the potential synergies and interactions between urban agglomerations and economic zones in solid waste management. In fact, due to close economic and geographical ties, the strategies and practices of solid waste management in provinces within the same economic zone often complement each other, learn from each other, and jointly improve management efficiency. Therefore, broadening the research perspective and considering urban agglomerations and economic zones as a whole is crucial for a deeper understanding and optimization of the solid waste management system. In addition, the existing literature on the effectiveness of industrial solid waste treatment is often limited to micro-level discussions of technical aspects, neglecting the integrity and coordination of the entire waste management process from a macro-system perspective. This one-sidedness has led to an inadequate assessment of the overall management efficiency and environmental impact.
This paper conducts an analysis using 31 regions from 2016 to 2022 as research samples. It employs a two-stage DEA model that takes into account unexpected output and sensitivity analysis. The objective is to evaluate the efficiency of industrial waste treatment in different regions of mainland China, focusing on the circular economy perspective. The two-stage DEA model is employed to assess and compare the effectiveness of solid waste treatment and reuse in different regions. This research examines the variability in efficiency among urban agglomerations by reviewing relevant literature and doing a sensitivity analysis using the one-by-one elimination technique to test the reliability of the findings. By evaluating the efficiency of industrial solid waste disposal in each province, this paper provides a reference for the development of environmental policies in China and other emerging countries. In addition, by analyzing the differences in efficiency among provinces, this paper helps to promote the exchange of experience and technology transfer in waste management between regions, and thus promotes the construction of waste recycling systems.
Compared with the limitations of previous literature in this field, the innovations of this paper are as follows. On the one hand, this research employs a two-stage DEA model to analyze efficiency in the context of circular economy, taking into account the two aspects of waste: pollution treatment and resource reuse. The assessment is decomposed into a pollution treatment stage (PDS) and a resource reuse stage (RRS). The current body of literature on assessing the effectiveness of industrial waste treatment primarily focuses on the treatment of solid waste from a singular standpoint (Tang et al., 2020a; Wang et al., 2023; Tang et al., 2020b; Wang and Cheng, 2024). However, this approach overlooks the reuse phase of solid waste, which hinders the development of appropriate policies and advancements in the waste treatment industry. Conversely, this article adopts a circular economy perspective and thoroughly takes into account the integrity of the industrial waste treatment system. Integrating resource reuse into the efficiency assessment system can greatly enhance resource use.
On the other hand, the research examines the variations in efficiency across the central, western, and eastern areas and conducts a dynamic analysis of the disparity in levels between provinces. Unlike prior studies that just examines disparities among provinces (Ji et al., 2023; Zhang et al., 2021). This paper examines many urban agglomerations simultaneously, considering both the individual components and the collective entity as a whole. The development disparities among the eastern, central, and western areas of China are distinct and representative. Evaluating efficiency based on this classification method helps each region choose a more appropriate approach to maximize resource usage. The effectiveness of solid waste management in specific provinces is not applicable to provinces in various economic zones due to significant disparities in resource allocation and developmental progress across areas. Hence, it is crucial to evaluate the efficacy of solid waste management across the eastern, central, and western regions of China. I Furthermore, sensitivity analysis, commonly employed in the medical domain to assess the reliability of research results, is infrequently implemented in this particular discipline (Tang et al., 2020a; Ji et al., 2023; Wang and Feng, 2020). This research employs sensitivity analysis to further confirm the veracity of the conclusions based on statistical methodologies.
The remainder of this paper is arranged as follows: Part 2 is a Research Methods and Models, Part 3 is an empirical analysis, Part 4 is a discussion, and Part 5 is a conclusion and recommendations.
2 Research methods and models
2.1 Research methodology on industrial solid waste
There are many methods to evaluate efficiency, mainly including parametric method and non-parametric method. The former includes stochastic frontier analysis, distribution-free method, life cycle method, SFA, TFA, DFA, etc., which is mainly used in the performance analysis of financial institutions, while the latter includes data envelopment analysis, DEA, etc. To measure eco-efficiency, data envelopment analysis (DEA) based on total factor production processes is often used to assess the relative efficiency of decision units (DMU) (Charnes et al., 1978).
The utilization of DEA technology in investigating solid waste efficiency has gained widespread application across numerous countries and regions (Simões and Marques, 2012). For example, Alizadeh et al. (2023) compared the eco-efficiency index of urban solid waste management systems in Iran. Pérez-López et al. (2018) studied the long-term scale efficiency of solid waste treatment services. Efficiency score in DEA is the ratio of output and input, and the weight of input and output will be generated automatically, so large samples or predictive parameter functions are not required. Due to its impartiality, this evaluation method has become the most favored approach in the realm of environmental issues (Lampe and Hilgers, 2015).
After about 40 years of development, Seiford and Zhu (1999), a key DEA model analyzed from two stages was introduced, which deals with the evaluation of system efficiency and its inherent structure. The traditional DEA models are limited to production assessment, ignoring the efficiency of waste treatment and resource reuse, while the two-stage network DEA framework reveals the inadequacy of management within the government and the industry. Managers can implement improved dimensional analysis methods or organizational strategies to improve recycling and reuse efficiency, thereby strengthening cooperation among them to improve ecological efficiency. Utilizing the enhanced DEA model, Zhou and Zhang (2019) examined the disparities and determinants influencing the efficiency of industrial solid waste resource utilization across 31 regions of China in 2017. Li et al. (2020) based on the Environmental Research Protection Institute (EPRA), a two-stage circular economy DEA model was established to accurately evaluate the efficiency of industrial waste recycling, treatment, and reuse in China.
It can be seen that the use of DEA method for solid waste efficiency has been widely recognized by scholars (Tang et al., 2024; Liu et al., 2023; Yang and Li, 2018). The method has been continuously improved. This study aims to address the limits of the standard DEA model and focuses on the circular economy. To do this, focused improvements and optimizations are made to the traditional DEA model framework. Furthermore, to prevent any distortion of the findings due to certain factors, this study used the one-by-one elimination approach to conduct sensitivity analysis and assess the reliability of the conclusion on regional efficiency. Hence, this study employs a two-stage DEA model to assess the effectiveness of solid waste utilization in different locations of China. Additionally, it ingeniously integrates the resource reuse stage into the model to account for its recycling value. Simultaneously, the trustworthiness of the finding is also confirmed using sensitivity analysis.
2.2 DEA model
Data Envelopment Analysis (DEA) was proposed by Charnes et al. (1978), which is used to evaluate the effectiveness of multiple decision-making units (DMUs) with multiple inputs and multiple outputs. The DEA method does not need to assume the form of the production function between the inputs and outputs, and is a non-parametric method of analysis. Since then, scholars have also derived a variety of models such as Slack-Based Measure (SBM) mode (Tone, 2001), Network DEA model (Färe et al., 2007) and Dynamic Network DEA model based on weighted relaxation measures (Tone and Tsutsui, 2014) among many others.
This research introduces a novel approach by incorporating the reuse stage of solid waste into the DEA evaluation system, which is a departure from earlier studies that mostly focused on the treatment of solid waste. Additionally, the paper takes into account the overall integrity of the solid waste treatment system. This research employs a two-stage circular DEA model to evaluate the management level of solid waste in different locations, focusing on solid waste treatment and reuse, from the standpoint of circular economy.
Considering the complete industrial solid waste treatment system, this paper refers to Zhang et al. (2021), which utilizes a two-stage dynamic recycling DDF model, and the specific flow framework diagram is shown in Figure 1.
According to the definition given by the National Bureau of Statistics of China3, the amount of hazardous waste generated refers to the actual amount of hazardous waste generated by the survey target throughout the year. The amount of comprehensive utilization of GISW refers to the amount of solid waste that enterprises have extracted from solid waste or converted into useable resources, energy and other raw materials through recycling, processing, circulation, exchange and other means during the reporting period. The amount of GISW disposal refers to the amount of industrial solid waste that an enterprise incinerates or uses other methods to change the physical, chemical, or biological properties of industrial solid waste to reduce or eliminate its hazardous components during the reporting period. It also refers to the amount of solid waste that is finally placed in a landfill that meets the requirements of environmental protection regulations. The amount of hazardous waste utilized for disposal refers to the sum of the comprehensive utilization and disposal of hazardous waste. Among them, the comprehensive utilization of hazardous waste refers to the amount of hazardous waste consumed in the activities of the survey subjects in the current year that extract substances from hazardous waste as raw materials or fuels. The amount of hazardous waste disposed of refers to the activities of enterprises that incinerate hazardous waste and use other methods that change the physical, chemical, and biological properties of industrial solid waste during the reporting period to reduce or eliminate its hazardous components.
Suppose there are
Referring to Zhang et al. (2021), the efficiency of
Here,
S.T.
Here,
The efficiencies of inputs, desirable output and undesirable output are shown in Equations 2–4 respectively (Hu and Wang, 2006).
Input:
Desirable output:
Undesirable output:
To test the robustness of regional efficiency analysis, this article uses a one-by-one exclusion method for sensitivity analysis.
2.3 Sensitivity analysis
To test the robustness of regional efficiency analysis, this article uses a one-by-one exclusion method for sensitivity analysis. The Method is as follows.
Research object: The efficiency level of solid waste treatment in the eastern and western regions of China.
Exclusion criteria: ① Research with incomplete or unavailable data; ② Research with unclear experimental methods or unreliable data; ③ Repeated published research.
Literature retrieval strategy: By searching databases such as Web of Science, ScienceDirect, and Spring Link, publicly published research on the efficiency or ecological efficiency of solid waste management in eastern and western China was conducted from 2015 to May 2024, with search terms including China solid waste, industrial sector, ecological efficiency, regional efficiency, etc.
Literature screening: The researchers in this article independently screen based on the inclusion and exclusion criteria of literature, excluding non-regional experiments and literature reviews. They search the full text of positive and negative literature before screening. If there are differences, they should be discussed and resolved, and other researchers should assist in resolving them if necessary.
3 Empirical analysis
3.1 Data description and statistical analysis
The dataset in this article covers 31 regions from 2016 to 2022 (including 22 provinces, 5 autonomous regions, and 4 municipalities in Chinese Mainland), and uses 7 years of industrial waste panel data to test the efficiency of waste treatment. Due to insufficient data in some regions, these areas were omitted from the analysis. This article follows the basic classification of industrial solid waste by the Chinese Ministry of Ecology and Environment: GISW and hazardous solid waste, and then selects the corresponding data from the China Environmental Statistics Yearbook. The data mainly comes from the China Environmental Statistical Yearbook, China Population and Employment Statistical Yearbook and China Statistical Yearbook.
Solid waste generated by enterprises during industrial production processes that does not fall into the category of hazardous waste is collectively referred to as GISW. This type of waste covers a wide range, such as tailings, gangue, fly ash, slag generated during the smelting process, slag, and desulfurization gypsum, etc., and there are many different types, each with very different properties (Wang and Cheng, 2024). The main methods of dealing with these wastes are divided into four categories: comprehensive utilization, storage, disposal, and dumping and discarding. According to the China National Statistical Yearbook, dumping and discarding waste accounts for a very small proportion, and comprehensive utilization is the main way to deal with GISW, followed by storage and disposal.
Hazardous waste refers to solid and liquid waste that is included in the national list or identified according to national standards and methods as having hazardous characteristics such as corrosivity, toxicity, flammability, reactivity, infectivity, etc., or that is not clearly specified but may be harmful to the environment and human health (Wu et al., 2024). The scope is very broad, including a wide range of wastes from the medical industry (such as infectious, injurious, pathological, chemical and pharmaceutical wastes), oil sludge and oil feet produced by the petroleum industry, and fly ash generated by the incineration of domestic waste. Given their diverse sources and different natures, the China Statistical Yearbook shows that China’s treatment of hazardous waste is dominated by the strategy of comprehensive utilization as a resource.
More specific classification of solid waste and industrial sources is shown in Supplementary Appendix SA1.
Table 1 shows the descriptive statistical data of the research indicators. The comprehensive utilization of industrial solid waste remains the core approach for its treatment. From 2016 to 2022, the average production, utilization, and disposal of GISW in 31 regions were 38.6 million tons, 21.4 million tons, and 920 million tons, respectively.
Figure 2 shows the trend of the production and utilization of industrial solid waste in China from 2016 to 2022. The results showed that the utilization and disposal of GISW remained relatively stable, and there was a slight increase in 2020. Since 2016, the amount of industrial solid waste produced has been rising slowly but steadily. The production of solid waste has been increasing year by year and peaked at approximately 4.41 billion tons in 2020. But that figure then dropped to 3.68 billion tons in 2021, a decline of about 16.6 percent. In 2022, the output of solid waste affected by the environment rose again to 3.97 billion tons, but only increased by about 7.9%, and the production and treatment capacity of GISW still needs to be improved compared with the growth volume.
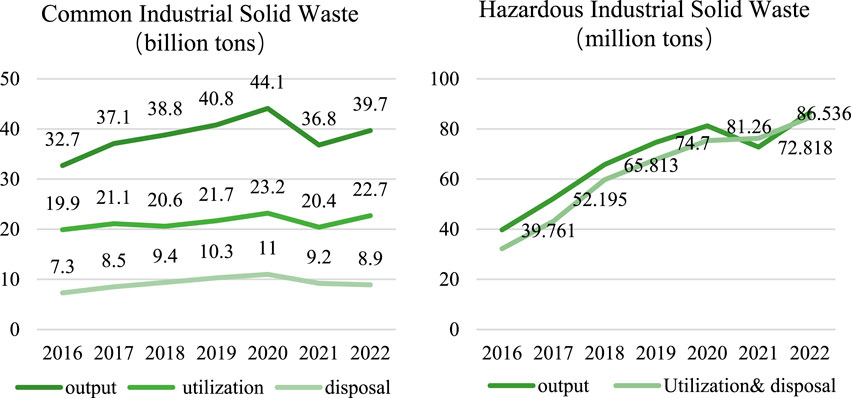
Figure 2. The trend of the production and utilization of industrial solid waste. Source: own analysis.
In contrast, the production and utilization rate of hazardous waste disposal showed a rapid growth trend. In 2021, the amount of utilization and disposal of hazardous waste exceeded the amount of production for the first time, reaching 59.12 million tons. This indicates that the disposal efficiency of hazardous waste in China shows a favorable development trend and further proves the important value of this paper’s analysis of China’s industrial solid waste.
3.2 Total efficiency score of solid waste
Figure 3 shows the overall efficiency score for each provincial administrative region. See Supplementary Appendix SA2 for the values. Among them, Beijing, Qinghai and Xinjiang ranked top with a total efficiency close to 1, indicating that these three regions attach great importance to industrial solid waste disposal and environmental protection, and have achieved good results in solid waste disposal. At the same time, Inner Mongolia, Shanxi and other regions tend to perform well at 0.9 points, maintaining a high efficiency of solid waste treatment. On the contrary, Chongqing, Fujian, Jiangsu and other regions have poor efficiency scores of about 0.38, reflecting the need for further improvement in solid waste management and pollution prevention.
Among the sample regions, Beijing and Qinghai have the highest solid waste management efficiency, at 1. Taking Beijing as an example, the Beijing Economic-Technological Development Area has established a resource exchange for the disposal of solid waste. This not only contributes to the improvement of the integrated solid waste management platform, but also explores a digital management model that serves the entire life cycle of industrial solid waste. In addition, strict environmental supervision, strong policy support and high public awareness of environmental governance have greatly contributed to the improvement of solid waste management efficiency in Beijing.
Among the sample regions, the bottom five are Tianjin, Guangdong (Ji et al., 2023), Chongqing, Fujian and Jiangsu. Take Jiangsu Province and Guangdong Province as examples. Guangdong Province and Jiangsu Province are two of the leading regions in terms of China’s economic development. In 2022, the GDP of the two regions will be 12,911.858 billion yuan and 12,287.6 billion yuan respectively. The rapid economic development has led to a continuous increase in the amount of solid waste generated. Therefore, the contradiction between supply and demand caused by the rapid increase in the treatment capacity of solid waste and the amount generated in Guangdong Province and Jiangsu Province is becoming increasingly prominent. In particular, there is a lack of regional treatment facilities for industrial solid waste and hazardous waste, resulting in an overall low efficiency of solid waste management. In addition, there are historical solid waste stockpiles in some areas of Guangdong Province, and it will take time and resources to solve these problems.
China does face many challenges in solid waste management, and some regions in China have low efficiency in solid waste treatment due to a lack of advanced technology (Li et al., 2020). According to the 2021 Classification Catalogue of Solid Waste and the National Catalogue of Hazardous Waste released by the Ministry of Ecology and Environment of China, there are 209 types of general industrial waste and 467 types of hazardous waste in China, each with a different main component. The Chinese Solid Waste Classification Catalogue and Hazardous Waste Catalogue have a wide variety of waste types, each with different components, which increases the challenge of solid waste treatment. The overly complex and diverse industrial solid waste makes management and treatment more difficult.
Overall, solid waste management in most regions of China still needs significant improvement, with only seven regions achieving an efficiency of over 0.7. In order to solve this problem, the Chinese government has continuously increased investment and issued a series of policies and regulations, such as the Solid Waste Pollution Prevention and Control Law of the People’s Republic of China, to comprehensively strengthen management and governance measures.
3.3 Staged efficiency analysis
Figure 4 shows the efficiency distribution of industrial solid waste in pollution treatment stage (PDS) and resource reuse stage (RRS) in different regions of China from 2019 to 2022. See Supplementary Appendix SA3 for the values.
3.3.1 Stage efficiency score of solid waste pollution treatment stage (PDS)
During the overall study period, the resource reuse stage of most provinces in China has been actively improved, but the performance of industrial solid waste pollution treatment stage is somewhat mixed. Among them, Beijing and Qinghai performed best, and the scores from 2019 to 2022 were 1, continuing to maintain the first place. With the exception of 2019, the efficiency scores of Shanghai and Xinjiang in all years were 1, and Beijing and Shanghai reflected better solid waste treatment efficiency due to their advanced industrial structure. However, Jiangsu, Chongqing, Guangdong and other provinces, due to their special geographical location or high degree of industrialization, are basically lagging behind in the annual ranking, reflecting that these regions have insufficient investment in solid waste treatment technology and facilities while developing the economy, and industrial solid waste management and pollution prevention and control need to be improved.
It can be clearly seen from Figure 4 that the score and ranking of Hebei Province have significantly decreased, and the efficiency of waste treatment and utilization has significantly decreased. In 2019, Hebei Province ranked first in solid waste treatment efficiency and ninth in utilization efficiency. However, it has been experiencing a decline ever since, falling to 22nd place by 2022. These regions accumulate substantial industrial solid waste, yet frequent floods wash about a quarter of it into farmlands, leading to persistent heavy metal contamination that is challenging to break down, thereby diminishing the efficiency of industrial solid waste management. Tianjin faces a similar scenario; its industrial solid waste disposal efficiency score remained high from 2019 to 2021, but plummeted to the lowest rank in 2022 with an efficiency value of 0.2274.
With its unique geographical advantages, Hainan Province has developed into a water-saving city (Zhuang et al., 2022), and its efficiency score has improved significantly. Starting in 2021, Hainan Province will continue to improve its solid waste management, and the efficiency of both stages will remain stable at 1 for the next 2 years. At the same time, Taipei City also remained stable in terms of industrial solid waste disposal efficiency, ranking first from 2019 to 2022, and the utilization efficiency score showed a fluctuating but generally positive trend. This shows that these regions have always attached importance to the management and disposal of industrial solid waste, and efficient and environmentally friendly governance has played an important role. Meanwhile, the government’s policy support and industrial transformation have enabled their solid waste treatment efficiency to increase year by year.
It is also worth noting that there are significant differences in the efficiency of industrial solid waste treatment and utilization in many regions. For example, the efficiency score of industrial solid waste utilization in Hunan and Ningxia from 2019 to 2022 were both 1 point, and the performance was good. However, their industrial solid waste treatment efficiency scores lagged behind in China, ranking behind 25 points. This may be due to the fact that although this area of our country has relatively advanced solid waste recycling technology and means, and has achieved certain results in waste recycling, some industrial areas are basically not close to the city. Under these circumstances, it is difficult for local industries to consume such large quantities of products. Due to higher transportation costs and increasingly stringent environmental protection measures in cities along the river, producers in these areas can only be required to dispose of as much product as possible locally. The huge output and high treatment costs have also produced greater pressure on the treatment of industrial solid waste in these areas.
3.3.2 Stage efficiency score of solid waste resource reuse stage (RRS)
Table 2 shows the efficiency of solid waste treatment investment in the recycling stage from 2019 to 2022.
As can be seen from Table 2, from 2019 to 2022, Significant improvement in solid waste treatment efficiency across China, signifying positive outcomes in the reuse stage of solid waste (Guo et al., 2021). The average investment efficiency in reuse rose from 0.438 in 2019 to 0.719 in 2022. This surge is primarily attributed to technological innovation, supportive government policies, and heightened public concern for the environment.
From the perspective of individual differences, the efficiency of solid waste reuse among different regions shows a polarization phenomenon (Li et al., 2023). This is mainly related to the investment environment, government support and technical level. Developed regions may have more resources and funds to invest in solid waste reuse, while less developed regions may struggle to attract sufficient investment due to limited resources and technology. In high-efficiency regions such as Beijing, Hunan, Ningxia, Qinghai and Xinjiang, the solid waste reuse efficiency has been maintained at the highest level of 1 for 4 years. However, in some areas, such as Fujian and Jiangsu, the efficiency value was always below 0.4 during the study period, which caused a large gap with other regions. The inefficiency of investment in such developed regions may be due to the unhealthy competitive landscape within the solid waste recycling industry. The emergency of over-motivation will lead to the dispersion of resources, which is not conducive to the formation of effective investment concentration. Several cities in the lower ranking, such as Shandong, Zhejiang, Anhui, have highly developed industrial zones and industrial bases. These developed provinces or cities have rapid economic development, high urbanization rate, and substantial increase in solid waste generation. This is also a significant reason for its inefficiency.
When exploring the regional differences in the efficiency of solid waste reuse, a significant polarization trend is evident, which profoundly reflects the combined impact of core factors such as the investment environment, government policy direction, and technological level. Specifically, some regions have demonstrated significant advantages in the recycling of solid waste and have maintained near-optimal or optimal efficiency levels for many years (e.g., Beijing, Hunan, Ningxia, Qinghai, and Xinjiang, where efficiency indicators have remained near-optimal or optimal for four consecutive years). In contrast, some economically developed regions, such as Fujian and Jiangsu, have long hovered at a relatively low level of solid waste recycling efficiency (with efficiency values consistently below 0.4), creating a significant gap with the high-efficiency regions. Further analysis shows that even in some traditionally developed regions, the investment efficiency of the solid waste recycling industry is unsatisfactory, which may be attributed to the unreasonable competitive structure within the industry. Excessive competition and distorted incentives may lead to ineffective integration and concentration of resources, but instead promote their decentralized allocation, which in turn hinders the formation of efficient investment models and the realization of economies of scale. The lower-ranking cities such as Shandong, Zhejiang and Anhui have highly developed industrial bases. Specifically, the concentration of economic activity and the increase in the level of urbanization have not only promoted socio-economic prosperity, but have also posed more severe challenges to the solid waste management system, including but not limited to the surge in the amount of solid waste generated, the upgrading of the demand for treatment technologies, and the improvement of the resource recovery and recycling system.
According to De Oliveira et al. (2022), Dou et al. (2024) and Iqbal et al. (2024), the investment efficiency of solid waste management refers to the ratio of economic, environmental and social benefits that can be obtained when a certain amount of funds and resources are invested in waste treatment and management. Simply put, it is the relationship between the cost of the input and the benefit obtained in the treatment of solid waste. High investment efficiency means that under the same investment, more benefits can be obtained, including resource recovery, environmental protection, social benefits and other benefits. In order to improve the investment efficiency of solid waste management, comprehensive optimization in technology, management and policy is needed to achieve maximum benefits.
Under the framework of the 2019 investment efficiency assessment of solid waste governance, the results show that only 11 regions have achieved an efficiency level of more than 0.8, while 8 regions have an efficiency level below 0.1. This also shows that most regions nationwide still need to increase their efforts in terms of investment in solid waste governance in order to maximize resource recycling. Efficient investment efficiency in solid waste is not only a key driver of environmental protection and resource conservation, but also an effective way to enhance the environmental image and market competitiveness of enterprises or organizations. The three regions at the bottom of the list are Hainan, Fujian and Guangxi. Entering 2020, the investment efficiency in the field of solid waste treatment has shown a positive trend, with the number of regions with an efficiency of more than 0.7 increasing to 12, accounting for 38.7% of the total sample, indicating an improvement in the overall effectiveness of solid waste management. Although the investment efficiency in solid waste management in some regions such as Sichuan, Inner Mongolia and Tibet is still relatively low, these regions have shown significant signs of improvement compared to the previous year.
However, the 2021 data reveals an unexpected turn of events, with the national average solid waste treatment efficiency slightly decreasing from 0.611 in 2020 to a level close to 0.584 in 2018. Despite fluctuations in the overall trend, it is worth noting that some cities, such as Inner Mongolia, have shown a steady increase in solid waste reuse (Li et al., 2023). In particular, the Inner Mongolia Autonomous Region, which has been among the top producers of GISW in China since 2019, has continued to innovate and optimize its solid waste treatment methods (Guo et al., 2021). Its transformation from relative backwardness to gradually approaching the leading level not only marks the Inner Mongolia Autonomous Region’s remarkable achievements and progress in the field of solid waste treatment, but also verifies the effective use and return of its investment funds. Inner Mongolia has quickly adapted and found a solid waste treatment path that suits the province’s actual situation, successfully promoting a regional green transformation. By 2022, the average investment efficiency of solid waste treatment across the country finally crossed the threshold of 0.7, reaching 0.719. Compared with 2019, the overall resource reuse level has achieved a significant improvement of 28.1%. This achievement not only affirms the efforts and investment made in the early stages, but also lays a solid foundation for future solid waste management. In addition, since 2019, China has also made significant progress in the field of hazardous waste management. The hazardous waste treatment and e-waste dismantling capabilities of some provinces and cities have achieved a qualitative leap, far exceeding historical levels (Guo et al., 2021), which indicates that China is steadily moving towards new heights in environmental protection and resource recycling.
Overall, the level of solid waste reuse in China has shown a significant improvement trend in most regions. With the continuous upgrading of pollution control technology and the enhancement of environmental awareness among citizens, solid waste management has also received more investment. During this period, China’s total investment in environmental pollution control skyrocketed from 101.49 billion yuan (about 14.138 billion dollars) to 903.72 billion yuan (about 125.888 billion dollars). The investment in provincial and municipal environmental infrastructure has increased from 51.55 billion yuan (about 7.181 billion dollars) to 522.299 billion yuan (about 72.756 billion dollars). Provincial and municipal environmental sanitation investment increased from 8.43 billion yuan (about 1.174 billion dollars) to 50.575 billion yuan (about 7.045 billion dollars). These factors collectively promote the recycling and reuse of industrial solid waste, driving investment in related infrastructure construction and economic growth.
In terms of the comprehensive solid waste treatment rates of each region in China from 2019 to 2022, the integrated treatment rate of solid waste is selected in this paper as the ratio of production to comprehensive utilization. See Supplementary Appendix SA4 for details. The closer the treatment rate is to 100%, the higher the efficiency of the province’s comprehensive solid waste disposal and the higher the efficiency of resource reuse. In the event of the value is upwards 100%, it means that the province has effectively treated not only the solid waste of the current year, but also the amount of solid waste stored in the previous year.
The average governance rate of China’s 31 provinces in 2019 was about 62.928%, indicating that the comprehensive governance of China’s provinces in 2019 was in the medium efficiency range. After the “12th Five-Year Plan” (2011–2015), China’s measures in the field of environmentally sustainable development have achieved initial results. Seven provinces and cities, including Tianjin, Shanghai, Zhejiang and Jiangsu, have a processing rate of more than 80%. However, this result is exactly the opposite of the overall efficiency score ranking of the provinces mentioned above, indicating that although these provinces and cities do a good job in the recycling treatment of industrial solid waste, the cost of resources invested in the treatment of solid waste is also high, resulting in a low overall efficiency. In 2020, the average treatment rate across the country’s 31 provinces was about 59.929%. Compared with the previous year, the total amount of solid waste increased and the treatment rate decreased, indicating that the existing solid waste treatment facilities in the year were unable to effectively handle the increased volume of solid waste, which may lead to environmental problems caused by the backlog of solid waste. However, it is gratifying to see that the treatment rate in Shanghai exceeds 100%, reaching 100.17%, and the investment in solid waste treatment this year is in line with the forecast value.
In 2021, the average processing rate of the country’s 31 provinces was about 60.300%. Compared with the previous year, the summation of solid waste is still growing steadily, but the average treatment rate has improved. The average disposal rate in 2022 was only 0.191% higher than in 2021, but the growth rate of industrial solid waste generation increased from 5.426% in 2021 to 8.088%, which indicates that China’s solid waste treatment processing power has significantly improved in 2022.
Although the enterprise information voluntarily released by some provinces has changed every year, to a certain extent, it will affect the accuracy of industrial solid waste generation. But overall, the efficiency of industrial solid waste reuse stage in all provinces and cities has improved to varying degrees, and the efficiency of industrial solid waste treatment in the top 10 cities with industrial solid waste production is also improving.
3.4 Sub-regional analysis
This article divides China into three parts for analysis, namely, the eastern, central, and western regions. The eastern region includes Beijing, Fujian, Guangdong, Hebei, Hainan, Jiangsu, Liaoning, Shandong, Shanghai, Tianjin, and Zhejiang; The central region includes Anhui, Hubei, Henan, Hunan, Heilongjiang, Jilin, Jiangxi, and Shanxi; The west includes Chongqing, Gansu, Guangxi, Guizhou, Inner Mongolia, Ningxia, Qinghai, Sichuan, Shaanxi, Yunnan, Xinjiang, and Tibet. Combine the total solid waste efficiency score with the map of China to obtain Figure 5.
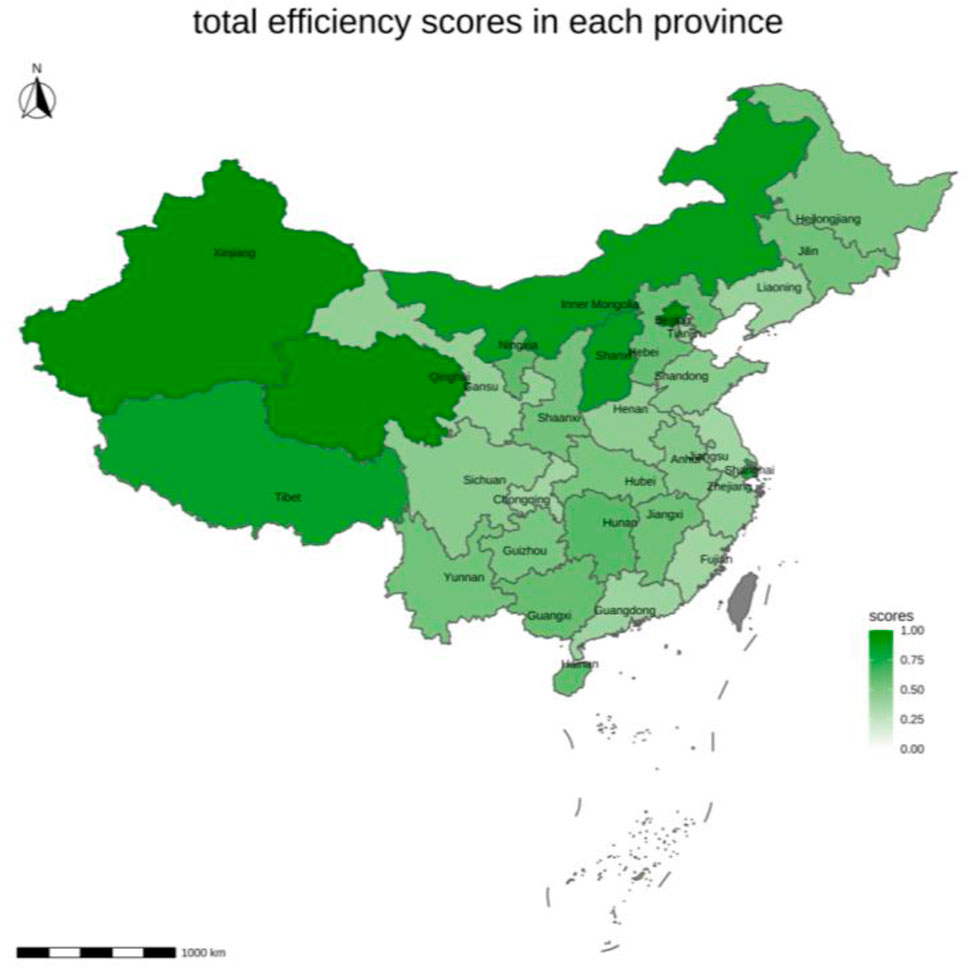
Figure 5. Distribution of the provincial total efficiency scores across the map. Source: The data results were plotted by ArcGIS 10.8 software.
As can be seen from Figure 5, the average score of 0.6493 in the western region is better than the average score of 0.5694 in the central region, and the average score of 0.5275 in the eastern region is the worst. Ten provinces scored an average of 0.6 or more (six in the west, one in the central region and three in the east). Twelve provinces (2 in western China, 4 in central China, and 7 in eastern China) had average efficiencies below 0.5. In terms of provincial rankings, Beijing and Qinghai performed best, tying for first place, followed by Xinjiang with a score of 0.9867 and Inner Mongolia with a score of 0.8832, with three of the top four in the west. The three worst-performing cities were western Chongqing (0.3875), Fujian (0.3827) and Jiangsu (0.3755), all of which are located in eastern China.
China’s industrial base is mainly concentrated in the eastern coastal areas, especially the Pearl River Delta (the lower Pearl River in Guangdong Province), the Yangtze River Delta (Shanghai, Jiangsu, Zhejiang, and Anhui provinces), and the Beijing-Tianjin-Hebei region (Beijing and Tianjin, two municipalities directly under the central government, and parts of Hebei and Henan provinces). These areas are coastal and have a good level of economic development, with developed transportation networks, rich human resources and a complete industrial chain.
In addition, China’s western regions also have some industrial bases, especially in Xinjiang, Gansu and Qinghai provinces, which have rich and unique natural resources and convenient policy support, attracting some heavy industry and resource processing enterprises. Compared with the eastern region and the central region, the number of industrial bases in the western region is far less than these two regions, but its scale is much larger than the central and eastern regions, and the western region still has great development potential.
According to the list of the top ten provinces in terms of gross domestic product (GDP) for 2022 released by the Chinese government, they are Guangdong, Jiangsu, Shandong, Zhejiang, Henan, Sichuan, Hubei, Fujian, Hunan and Anhui. This distribution pattern clearly reflects the uneven development of China’s regional economy, with the proportion of provinces from the western, central and eastern regions being 10%, 40% and 50% respectively. Further analysis reveals that there is a close and complex correlation between the efficiency of economic development in these provinces and their industrial base layout and overall economic performance. Specifically, the difference in the score of industrial solid waste treatment efficiency, an important indicator for measuring the coordinated development of the economy and the environment, directly reflects the intrinsic relationship between the layout of industrial facilities and the level of economic development. On the one hand, the efficiency of industrial solid waste treatment may be affected by both the uneven geographical distribution of industrial bases and the differences in the level of economic development between regions. Large industrial clusters are often accompanied by high solid waste generation, which to some extent exacerbates the volatility of efficiency scores. On the other hand, there is also a mutually restrictive relationship between industrial solid waste treatment efficiency and the layout of industrial bases and economic development. In highly developed regions, although high productivity promotes rapid economic growth, it may also generate large amounts of solid waste due to intensive industrial activities. If the speed of technological innovation and the waste recycling system fail to keep pace, it may lead to inefficient waste treatment, which in turn poses challenges to environmental protection and resource recycling.
The government of the People’s Republic of China has released information on the top ten provinces in terms of GDP ranking in 2022. These provinces are Guangdong, Jiangsu, Shandong, Zhejiang, Henan, Sichuan, Hubei, Fujian, Hunan, and Anhui. It is worth noting that one province is located in the west, four in the central region, and five in the east. Interestingly, the efficiency scores of these provinces are in direct contrast to the distribution of industrial bases and economic development. This suggests that there is an intricate correlation between the efficiency rating of industrial solid waste and the allocation of industrial facilities and economic progress. On the one hand, the efficiency score of industrial solid waste may be affected by uneven distribution of industrial bases or differences in economic development levels. Large industrial production enterprises usually generate more solid waste, which may lead to fluctuations in the efficiency score of industrial solid waste. On the other hand, there may also be a mutually restrictive relationship between the efficiency score of industrial solid waste and the distribution of industrial bases and economic development. In some areas with high levels of economic development, due to high productivity, a large amount of industrial solid waste may be generated, and the technological level and reuse enterprises at that time may not be able to timely recover and utilize these wastes, thereby reducing environmental protection and resource utilization efficiency. Therefore, the efficiency score of industrial solid waste in these provinces may decrease.
Overall, there is indeed a negative correlation between the efficiency score of industrial solid waste and the distribution of industrial bases and economic development, but this result is also influenced by various factors. In promoting industrial solid waste management and resource utilization, it is necessary to comprehensively consider the industrial structure, economic development level, and technical support of different regions to achieve more efficient solid waste treatment and reuse. Furthermore, to mitigate the impact of any oversight on the outcomes of this section, this study also conducted a sensitivity analysis subsequently. By reviewing relevant literature and analyzing its data, this work aims to assess the reliability of its subregional efficiency findings.
3.5 Sensitivity analysis
3.5.1 Literature search results
After screening out cross references, duplicate articles, and reading titles, abstracts, and full texts from various databases, 7 articles were ultimately included. The basic information included in the literature is shown in Table 3.
Among them, W(N), W (mean), and W (sd) represent the regional sample size, efficiency mean, and efficiency standard deviation of each literature in studying the workpiece ratio of solid waste management in the western region. Similarly, E(N), E (mean), and E (sd) represent the regional sample size, mean efficiency, and standard deviation efficiency of each literature in studying the workpiece ratio of solid waste management in the eastern region.
3.5.2 Sensitivity analysis results
This article selects the above 7 references (Tang et al., 2020a; Ji et al., 2023; Wang and Feng, 2020; Li et al., 2020; Matsumoto and Chen, 2021; Zhang et al., 2017; Tang et al., 2020b) and uses a fixed effects model to conduct sensitivity analysis on the included studies using a stepwise exclusion method. Using Stata17 software, one article was sequentially excluded and the remaining six articles were merged for meta-analysis. The changes in the merged results were observed to evaluate the stability of the analysis results. The sensitivity analysis results are shown in Figure 6.
The merge result shows a 95% CI of (−1.298, −0.5395), excluding 0. From this, it can be seen that excluding any one study, the combined results of the remaining six studies still have statistical significance, indicating the robustness of the research results in this article.
4 Discussion
Compared with the results of other literature, this study found some similarities and differences.
First, this paper presents a two-stage DEA model that has been modified to evaluate the efficacy of industrial waste treatment in Chinese provinces from a more comprehensive and systematic perspective. In contrast to the single-stage DEA model (Albores et al., 2016; Zhang et al., 2022; Hernández-Sancho and Sala-Garrido, 2009), the two-stage DEA model offers advantages in the areas of internal structure consideration, more precise efficiency evaluation, and subtler evaluation (lo Storto, 2024). In addition, the circular economy perspective completely considers the entire production and consumption system when evaluating the efficiency of waste treatment, resulting in a more precise measure of efficiency.
Second, the results of this paper are in agreement with Yang et al. (2024) in terms of the efficacy of the circular economy in certain provinces, including Sichuan and Guangxi. The circular economy efficiency in Sichuan and Guangxi has experienced varying degrees of development as a result of the growing emphasis on waste management and the continuous advancements in pollution control technology. The findings of this paper also corroborate Zhuang et al. (2022) and Guo et al. (2021) that the circular economy performance in certain cities, such as Heilongjiang and Hainan, has been improving over the study period regarding waste management.
This paper concludes that the western region is more efficient than the eastern region in terms of solid refuse management. This differs from certain literature (Ji et al., 2023), which is primarily concerned with the research method, time duration, and study sample. Furthermore, sensitivity analysis is more frequently employed in the medical field and less frequently in environmental fields, such as solid waste treatment, as an essential component of meta-analysis (Tang et al., 2020a; Ji et al., 2023; Wang and Feng, 2020). The robustness of the findings of this paper is also entirely demonstrated by the results of the sensitivity analysis of the included literature, which was conducted using the one-by-one exclusion procedure.
The research presented in this article is of significant theoretical and practical importance in the assessment of the efficacy of industrial solid waste recycling and the investigation of viable circular economy models. The applicability of the findings must be enhanced due to the constraints of the research topic and data acquisition, despite the fact that this study concentrates on the efficiency of the treatment and reuse stages of industrial solid refuse. The research conclusions are restricted by the fact that the study was conducted between 2019 and 2022. The circular economy of China has been significantly affected by the downward pressure on the economy since 2020, and these changes warrant additional in-depth research. In order to conduct a more thorough and standardized evaluation of the efficacy of industrial solid waste treatment and reuse, future research should consider expanding the time frame, increasing data samples, and incorporating additional factors. This will contribute to the comprehensive and standardized development of waste recycling systems.
5 Conclusions and suggestions
5.1 Conclusions
This paper utilizes a two-stage DEA model considering non-desired inputs and a sensitivity analysis method to analyze the efficiency of solid waste disposal in each region of China from 2019 to 2022, and draws the following conclusions:
First, from the perspective of the total efficiency of solid waste treatment, the solid waste treatment efficiency in most parts of China has been improved to varying degrees. Due to the large amount of production of industrial solid waste in China, the unbalanced regional development of production and comprehensive utilization of industrial solid waste. Only Beijing, Qinghai and Xinjiang have higher overall efficiency.
Second, from the perspective of the circular economy. The solid waste treatment stage exhibits substantial regional disparities, although the efficiency of each location in the reuse stage has notably increased. Out of all the regions, Beijing and Qinghai achieved the highest performance. Their scores for industrial solid waste treatment and utilization efficiency remained at 1 from 2019 to 2022, consistently securing the top position. Nevertheless, Jiangsu, Chongqing, Guangdong, and other provinces are generally falling behind in the yearly ranking because of their unique geographical position or significant level of industrialization.
In terms of investment efficiency of solid waste management, the performance of different regions in China shows a relatively obvious two-stage differentiation phenomenon. The investment efficiency of most regions is still on the upward trend during the study period. However, in some regions, such as Fujian Province, the investment efficiency was always below 0.2 during the study period. In economically developed areas, there is often a significant generation of industrial solid waste due to high productivity. However, the capacity of technology and recycling enterprises is limited, making it challenging to recycle the waste promptly. This situation has a negative impact on environmental protection and the efficiency of resource utilization.
Thirdly, the efficiency of solid waste management in various regions. In contrast to prior rankings of provincial efficiency, certain provinces and cities, such as Jiangsu and Tianjin, have demonstrated commendable performance in the comprehensive utilization of industrial solid waste. China’s capability for treating solid waste has steadily increased throughout time. The mean score in the western region surpasses that in the central region, whilst the mean score in the eastern zone is the most inferior. The efficiency of industrial solid waste is inversely correlated with the dispersion of bases and economic development. However, this relationship is also influenced by several factors.
In addition, in the sensitivity analysis section, this paper applies the fixed-effects model to merge the results, and the merged results show that the 95% CI is (−1.298, −0.5395) excluding 0. Therefore, excluding any one study, the merged results of the remaining studies are still statistically significant, which further validates the robustness of the conclusions of this paper.
5.2 Suggestion
From an enterprise standpoint, enhancing the effectiveness of industrial solid waste recycling necessitates bolstering internal administration and implementing a systematic approach to classify and recycle solid waste. Enterprises must enhance employees’ environmental consciousness and provide training to ensure they understand the significance of solid waste categorization and recycling. This will help raise their knowledge and passion for solid waste recycling. However, it is essential for businesses to create robust waste classification and recycling facilities to enable efficient separation and recycling of solid waste.
The government bears significant duties and assumes crucial functions in facilitating the optimization of industrial solid waste recycling. To enhance oversight and control over the recycling of industrial solid waste, the government can implement appropriate legislation and regulations to guarantee that firms adhere closely to the applicable guidelines. The government can incentivize firms to enhance solid waste recycling and use by implementing tax policies and incentive systems. This will facilitate investment, building, and operation of solid waste recycling facilities. Simultaneously, the government can enhance the guidance and oversight of the solid waste recycling market, facilitating the efficient exploitation and recycling of solid waste resources.
Data availability statement
The original contributions presented in the study are included in the article/Supplementary Material, further inquiries can be directed to the corresponding author.
Author contributions
XG: Conceptualization, Methodology, Resources, Writing–original draft. FR: Formal Analysis, Writing–original draft, Project administration. GF: Investigation, Writing–review and editing. QZ: Data curation, Writing–review and editing. TW: Validation, Writing–review and editing.
Funding
The author(s) declare that financial support was received for the research, authorship, and/or publication of this article. This study was supported by National Social Science Foundation Project (24BJY142), Jiangsu Province Social Science Foundation Project (22GLD019), Major Project of Philosophy and Social Science Research in Universities of Jiangsu Province (2022SJZD053).
Conflict of interest
Author GF was employed by Jiangsu Culture Innovation Development Co., Ltd.
The remaining authors declare that the research was conducted in the absence of any commercial or financial relationships that could be construed as a potential conflict of interest.
Publisher’s note
All claims expressed in this article are solely those of the authors and do not necessarily represent those of their affiliated organizations, or those of the publisher, the editors and the reviewers. Any product that may be evaluated in this article, or claim that may be made by its manufacturer, is not guaranteed or endorsed by the publisher.
Abbreviations
DEA, data envelopment analysis; PDS, pollution treatment stage; RRS, resource reuse stage; GISW, general industrial solid waste.
Footnotes
1https://www.mee.gov.cn/gzk/gz/202112/t20211213_963867.shtml
2https://www.gov.cn/gongbao/content/2021/content_5585231.htm
3https://www.stats.gov.cn/zt_18555/ztsj/hjtjzl/2014/202303/t20230303_1924259.html
References
Abdullah, M., and Abedin, M. Z. (2024). Assessment of plastic waste management in Bangladesh: a comprehensive perspective on sorting, production, separation, and recycling. Res. Surf. Interf. 15, 100221. doi:10.1016/j.rsurfi.2024.100221
Albores, P., Petridis, K., and Dey, P. K. (2016). Analysing efficiency of waste to energy systems: using data envelopment analysis in municipal solid waste management. Procedia Environ. Sci. 35, 265–278. doi:10.1016/j.proenv.2016.07.007
Alizadeh, S., Vali, F., Vatani, Z., and Avami, A. (2023). Sustainable analysis of Waste-to-Energy systems in cities by eco-efficiency assessment using DEA approach: a case study of Iran's municipalities. Sustain. Cities Soc. 98, 104825. doi:10.1016/j.scs.2023.104825
Bui, T. D., Tseng, J. W., Tseng, M. L., and Lim, M. K. (2022). Opportunities and challenges for solid waste reuse and recycling in emerging economies: a hybrid analysis. Resour. Conserv. Recy. 177, 105968. doi:10.1016/j.resconrec.2021.105968
Charnes, A., Cooper, W. W., and Rhodes, E. (1978). Measuring the efficiency of decision making units. Eur. J. Oper. Res. 2 (6), 429–444. doi:10.1016/0377-2217(78)90138-8
Chien, C. F., Aviso, K., Tseng, M. L., Fujii, M., and Lim, M. K. (2023). Solid waste management in emerging economies: opportunities and challenges for reuse and recycling. Resour. Conserv. Recy. 188, 106635. doi:10.1016/j.resconrec.2022.106635
De Oliveira, B. O. S., De Medeiros, G. A., Mancini, S. D., Paes, M. X., and Gianelli, B. F. (2022). Eco-efficiency transition applied to municipal solid waste management in the Amazon. J. Clean. Prod. 373, 133807. doi:10.1016/j.jclepro.2022.133807
De Souza, A. M., De Carvalho, J. M. F., Santos, C. F. R., Ferreira, F. A., Pedroti, L. G., and Peixoto, R. A. F. (2022). On the strategies to improve the eco-efficiency of self-compacting concrete using industrial waste: an analytical review. Constr. Build. Mater. 347, 128634. doi:10.1016/j.conbuildmat.2022.128634
De Souza, V. M., Bloemhof, J., and Borsato, M. (2021). Assessing the eco-effectiveness of a solid waste management plan using agent-based modelling. Waste Manag. 125, 235–248. doi:10.1016/j.wasman.2021.02.019
Dou, W., Li, H., Li, Z., Li, P., Wu, C., and Liu, Y. (2024). Modeling and evaluating costs and greenhouse gas emissions in solid waste management based on system dynamics in a mega-city: the case of Xi'an. J. Clean. Prod. 454, 142325. doi:10.1016/j.jclepro.2024.142325
Färe, R., Grosskopf, S., and Whittaker, G. (2007). Network DEA. Modeling data irregularities and structural complexities in data envelopment analysis. Springer, 209–240.
Guan, Y., Huang, G., Liu, L., Huang, C. Z., and Zhai, M. (2019). Ecological network analysis for an industrial solid waste metabolism system. Environ. Pollut. 244, 279–287. doi:10.1016/j.envpol.2018.10.052
Guo, W., Xi, B., Huang, C., Li, J., Tang, Z., Li, W., et al. (2021). Solid waste management in China: policy and driving factors in 2004–2019. Resour. Conserv. Recy. 173, 105727. doi:10.1016/j.resconrec.2021.105727
Hernández-Sancho, F., and Sala-Garrido, R. (2009). Technical efficiency and cost analysis in wastewater treatment processes: a DEA approach. Desalination 249 (1), 230–234. doi:10.1016/j.desal.2009.01.029
Hu, J. L., and Wang, S. C. (2006). Total-factor energy efficiency of regions in China. Energy pol. 34 (17), 3206–3217. doi:10.1016/j.enpol.2005.06.015
Iqbal, A., Yasar, A., Nizami, A. S., Haider, R., Sultan, I. A., Kedwaii, A. A., et al. (2024). Empirical analysis of cost-effective and equitable solid waste management systems: environmental and economic perspectives. Environ. Res. 244, 117858. doi:10.1016/j.envres.2023.117858
Ji, L., Sun, Y., Liu, J., and Chiu, Y. H. (2023). Analysis of the circular economy efficiency of China's industrial wastewater and solid waste-based on a comparison before and after the 13th Five-Year Plan. Sci. Total Environ. 881, 163435. doi:10.1016/j.scitotenv.2023.163435
Ke, Y., Chen, Y., Liang, S., Hu, J., Hou, H., Quan, J., et al. (2023). Environmentally sound management of industrial solid waste: a paradigm of proposed bi-tetrahedron. Resour. Conserv. Recy. 198, 107212. doi:10.1016/j.resconrec.2023.107212
Kirchherr, J., Reike, D., and Hekkert, M. (2017). Conceptualizing the circular economy: an analysis of 114 definitions. Resour. Conserv. Recy. 127, 221–232. doi:10.1016/j.resconrec.2017.09.005
Kundariya, N., Mohanty, S. S., Varjani, S., Ngo, H. H., Wong, J. W., Taherzadeh, M. J., et al. (2021). A review on integrated approaches for municipal solid waste for environmental and economical relevance: monitoring tools, technologies, and strategic innovations. Bioresour. Technol. 342, 125982. doi:10.1016/j.biortech.2021.125982
Lampe, H. W., and Hilgers, D. (2015). Trajectories of efficiency measurement: a bibliometric analysis of DEA and SFA. Eur. J. Oper. Res. 240 (1), 1–21. doi:10.1016/j.ejor.2014.04.041
Li, D., Wang, M. Q., and Lee, C. (2020). The waste treatment and recycling efficiency of industrial waste processing based on two-stage data envelopment analysis with undesirable inputs. J. Clean. Prod. 242, 118279. doi:10.1016/j.jclepro.2019.118279
Li, H., Bao, W., Xiu, C., Zhang, Y., and Xu, H. (2010). Energy conservation and circular economy in China's process industries. Energy 35 (11), 4273–4281. doi:10.1016/j.energy.2009.04.021
Li, Z., Ouyang, X., Du, K., and Zhao, Y. (2017). Does government transparency contribute to improved eco-efficiency performance? An empirical study of 262 cities in China. Energy Pol. 110, 79–89. doi:10.1016/j.enpol.2017.08.001
Liou, J. L., and Wu, P. I. (2011). Will economic development enhance the energy use efficiency and CO2 emission control efficiency? Expert Syst. Appl. 38 (10), 12379–12387. doi:10.1016/j.eswa.2011.04.017
Liu, F., Li, L., Ye, B., and Qin, Q. (2023). A novel stochastic semi-parametric frontier-based three-stage DEA window model to evaluate China's industrial green economic efficiency. Energy Econ. 119, 106566. doi:10.1016/j.eneco.2023.106566
Long, X., Sun, M., Cheng, F., and Zhang, J. (2017). Convergence analysis of eco-efficiency of China’s cement manufacturers through unit root test of panel data. Energy 134, 709–717. doi:10.1016/j.energy.2017.05.079
lo Storto, C. (2024). Measuring the eco-efficiency of municipal solid waste service: a fuzzy DEA model for handling missing data. Util. Policy. 86, 101706. doi:10.1016/j.jup.2023.101706
Luo, X., Li, X., Wei, C., Deng, Z., and Li, M. (2022). Use of Ca-containing industrial waste brine for CO2 sequestration and recovery of NaCl and Na2SO4. Sep. Purif. Technol. 303, 122208. doi:10.1016/j.seppur.2022.122208
Majchrowska, S., Mikołajczyk, A., Ferlin, M., Klawikowska, Z., Plantykow, M. A., Kwasigroch, A., et al. (2022). Deep learning-based waste detection in natural and urban environments. Waste Manag. 138, 274–284. doi:10.1016/j.wasman.2021.12.001
Mardani, A., Zavadskas, E. K., Streimikiene, D., Jusoh, A., and Khoshnoudi, M. (2017). A comprehensive review of data envelopment analysis (DEA) approach in energy efficiency. Renew. Sustain. Energy Rev. 70, 1298–1322. doi:10.1016/j.rser.2016.12.030
Matsumoto, K. I., and Chen, Y. (2021). Industrial eco-efficiency and its determinants in China: a two-stage approach. Ecol. Indic. 130, 108072. doi:10.1016/j.ecolind.2021.108072
Maturi, K. C., Gupta, A., Haq, I., and Kalamdhad, A. S. (2022). A glance over current status of waste management and landfills across the globe: a review. Biodegr. Detoxif. Micro. Ind. Wastewater, 131–144. doi:10.1016/b978-0-323-88507-2.00001-4
Neves, S. A., Marques, A. C., and Silva, I. P. (2024). Promoting the circular economy in the EU: how can the recycling of e-waste be increased? Struct. Change. Econ. Dyn. 70, 192–201. doi:10.1016/j.strueco.2024.02.006
Nogueira, L. A. (2023). Exploring the industrial dynamics of waste management and recycling: a call for research and a proposed agenda. Waste Manag. 170, 33–39. doi:10.1016/j.wasman.2023.07.022
Oggioni, G., Riccardi, R., and Toninelli, R. (2011). Eco-efficiency of the world cement industry: a data envelopment analysis. Energy pol. 39 (5), 2842–2854. doi:10.1016/j.enpol.2011.02.057
Pérez-López, G., Prior, D., and Zafra-Gómez, J. L. (2018). Temporal scale efficiency in DEA panel data estimations. An application to the solid waste disposal service in Spain. Omega 76, 18–27. doi:10.1016/j.omega.2017.03.005
Quan, Z., Xu, X., Wang, W., Jiang, J., and Gao, S. (2024). Do industrial solid waste recycling and technological innovation promote low-carbon development in China? New insights from NARDL approach. Sci. Total Environ. 916, 170446. doi:10.1016/j.scitotenv.2024.170446
Rodrigues, J., Gondran, N., Beziat, A., and Laforest, V. (2021). Application of the absolute environmental sustainability assessment framework to multifunctional systems–The case of municipal solid waste management. J. Clean. Prod. 322, 129034. doi:10.1016/j.jclepro.2021.129034
Sauve, G., and Van Acker, K. (2020). The environmental impacts of municipal solid waste landfills in Europe: a life cycle assessment of proper reference cases to support decision making. J. Environ. Manag. 261, 110216. doi:10.1016/j.jenvman.2020.110216
Seiford, L. M., and Zhu, J. (1999). An investigation of returns to scale in data envelopment analysis. Omega 27 (1), 1–11. doi:10.1016/s0305-0483(98)00025-5
Simões, P., and Marques, R. C. (2012). On the economic performance of the waste sector. A literature review. J. Environ. Manag. 106, 40–47. doi:10.1016/j.jenvman.2012.04.005
Su, F., Peng, H., Yin, H., Luo, C., Zhu, L., Zhong, W., et al. (2021). Biowaste-derived hydrochar microspheres: realizing metal-free visible-light photocatalytic oxidation of amines. J. Catal. 404, 149–162. doi:10.1016/j.jcat.2021.09.019
Sueyoshi, T., and Goto, M. (2019). The intermediate approach to sustainability enhancement and scale-related measures in environmental assessment. Eur. J. Oper. Res. 276 (2), 744–756. doi:10.1016/j.ejor.2019.01.032
Sueyoshi, T., Yuan, Y., and Goto, M. (2017). A literature study for DEA applied to energy and environment. Energy Econ. 62, 104–124. doi:10.1016/j.eneco.2016.11.006
Tang, J., Wang, Q., and Chang, Y. T. (2020a). China’s regional industrial two-stage system–Efficiencies and their influencing factors. J. Clean. Prod. 249, 119420. doi:10.1016/j.jclepro.2019.119420
Tang, J., Wang, Q., and Choi, G. (2020b). Efficiency assessment of industrial solid waste generation and treatment processes with carry-over in China. Sci. Total Environ. 726, 138274. doi:10.1016/j.scitotenv.2020.138274
Tang, J., Wang, Q., Li, Z., Gu, J., and Xu, J. (2024). Coupling coordination degree of industrial solid waste prevention and treatment efficiencies and its driving factors in China. Ecol. Indic. 158, 111395. doi:10.1016/j.ecolind.2023.111395
Tone, K. (2001). A slacks-based measure of efficiency in data envelopment analysis. Eur. J. Oper. Res. 130 (3), 498–509. doi:10.1016/s0377-2217(99)00407-5
Tone, K., and Tsutsui, M. (2014). Dynamic DEA with network structure: a slacks-based measure approach. Omega-Int. J. Manag. Sci. 42 (1), 124–131. doi:10.1016/j.omega.2013.04.002
Tsai, F. M., Bui, T. D., Tseng, M. L., Lim, M. K., and Hu, J. (2020). Municipal solid waste management in a circular economy: a data-driven bibliometric analysis. J. Clean. Prod. 275, 124132. doi:10.1016/j.jclepro.2020.124132
Wang, C. Q., and Cheng, L. X. (2024). Study on general industrial solid waste and carbon reduction in China: coupling coordination model, life cycle assessment and environmental safety control. Sustain. Chem. Pharm. 39, 101557. doi:10.1016/j.scp.2024.101557
Wang, M., and Feng, C. (2020). Regional total-factor productivity and environmental governance efficiency of China’s industrial sectors: a two-stage network-based super DEA approach. J. Clean. Prod. 273, 123110. doi:10.1016/j.jclepro.2020.123110
Wang, Q., Duan, H., Miao, Q., Li, H., Liu, J., Wang, N., et al. (2023). Environmental and economic impact assessment of synergistic organic-waste treatment strategies in eco-industrial parks: a pilot-scale case study in Shenzhen, China. Environ. Impact Assess. Rev. 103, 107250. doi:10.1016/j.eiar.2023.107250
Wu, D., Liu, Y., Meng, L., Chen, Y., and Yang, Z. (2024). Distribution, occurrence, and environmental risks of heavy metals in hazardous waste: a regional study in Beijing, China. Circ. Econ. 3, 100099. doi:10.1016/j.cec.2024.100099
Yang, L., Chen, S., Chiu, Y. H., Chang, T. H., and Wang, Y. (2024). Reassessment of industrial eco-efficiency in China under the sustainable development goals: a meta twostage parallel entropy dynamic DDF-DEA model. J. Clean. Prod. 141275. doi:10.1016/j.jclepro.2024.141275
Yang, W., and Li, L. (2018). Efficiency evaluation of industrial waste gas control in China: a study based on data envelopment analysis (DEA) model. J. Clean. Prod. 179, 1–11. doi:10.1016/j.jclepro.2017.12.277
Zhang, J., Liu, Y., Chang, Y., and Zhang, L. (2017). Industrial eco-efficiency in China: a provincial quantification using three-stage data envelopment analysis. J. Clean. Prod. 143, 238–249. doi:10.1016/j.jclepro.2016.12.123
Zhang, L., Du, X., Chiu, Y. H., Pang, Q., and Yu, Q. (2022). Measuring industrial operational efficiency and factor analysis: a dynamic series-parallel recycling DEA model. Sci. Total Environ. 851, 158084. doi:10.1016/j.scitotenv.2022.158084
Zhang, L., Zhao, L., and Zha, Y. (2021). Efficiency evaluation of Chinese regional industrial systems using a dynamic two-stage DEA approach. Socio-Econo. Plan. Sci. 77, 101031. doi:10.1016/j.seps.2021.101031
Zhou, J., and Zhang, R. (2019). Efficiency evaluation of industrial solid waste recycling utilization based on improved DEA model. IOP Conf. Ser. Earth Environ. Sci. 295 (3), 032024. doi:10.1088/1755-1315/295/3/032024
Keywords: pollution disposal, resource reuse, solid waste management, circular economy, DEA (data envelope analysis)
Citation: Guan X-g, Ren F-r, Fan G, Zhang Q-q and Wu T-f (2024) Dynamic evaluation and sensitivity analysis of China’s industrial solid waste management efficiency based on ecological environment cycle perspective. Front. Environ. Sci. 12:1462975. doi: 10.3389/fenvs.2024.1462975
Received: 11 July 2024; Accepted: 18 November 2024;
Published: 02 December 2024.
Edited by:
Jiachao Peng, Wuhan Institute of Technology, ChinaReviewed by:
Junxue Zhang, Jiangsu University of Science and Technology, ChinaShui-ying Jin, Zhejiang Normal University, China
Shiliang Hu, Nanjing University of Information Science and Technology, China
Jiahui Yi, China University of Geosciences Wuhan, China
Copyright © 2024 Guan, Ren, Fan, Zhang and Wu. This is an open-access article distributed under the terms of the Creative Commons Attribution License (CC BY). The use, distribution or reproduction in other forums is permitted, provided the original author(s) and the copyright owner(s) are credited and that the original publication in this journal is cited, in accordance with accepted academic practice. No use, distribution or reproduction is permitted which does not comply with these terms.
*Correspondence: Gao Fan, Z2ZhbjIwMjRAMTYzLmNvbQ==
†These authors have contributed equally to this work and share first authorship