Field efficacy of urease inhibitors for mitigation of ammonia emissions in agricultural field settings: a systematic review
- 1Teagasc, Environment, Soils and Land Use Department, Crops, Environment and Land Use Programme, Wexford, Ireland
- 2Teagasc, Food Safety Department, Food Research Centre, Dublin, Ireland
- 3School of Biosystems and Food Engineering, University College Dublin, Dublin, Ireland
Globally, ammonia (NH3) is one of the key air pollutants and reducing NH3 emissions and the associated indirect emission of the greenhouse gas nitrous oxide remains challenging for the agricultural sector. During the past three decades, a number of urease inhibitors have been placed on the market with the goal of reducing NH3 loss from urea containing fertilisers. N–(n-butyl) thiophosphoric triamide (NBPT), N–(2-nitrophenyl) phosphoric triamide (2-NPT), a 3:1 ratio of NBPT + N-(n-propyl) thiophosphoric triamide (NPPT) and the maleic and itaconic acid co-polymer (MIP) are registered urease inhibitors under the European Commission Fertilising Products Regulation (FPR). However, the availability of several inhibitor options has raised questions from farmers, policymakers and emissions inventory compiling authorities regarding the field efficacy of the different options available for reducing NH3 loss. Despite many disparate NH3 field studies existing for NBPT, 2-NPT, NBPT + NPPT and MIP there is presently no review that brings these results together, a significant and important knowledge gap. This review addresses the gap by summarising the published field trial literature on NH3 volatilisation mitigation offered by NBPT, 2-NPT, NBPT + NPPT and MIP. Our review identified 48 peer reviewed studies where NH3 loss mitigation was measured in a field setting, giving 256 replicated comparisons. The synthesised literature results revealed that NBPT + NPPT reduced NH3 loss by 75% (95% CI = 58–82% n = 32), 2-NPT reduced NH3 loss by 70% (95% CI = 63–76% n = 19) and NBPT reduced NH3 loss by 61% (95% CI = 57–64% n = 165), giving on average a 69% reduction by these three urease inhibitors. In contrast, MIP increased NH3 loss by 0.3% on average (95% CI = −8–9% n = 40). The results presented in this review broaden the understanding of urease inhibitor efficacy in field conditions and demonstrate that not all products behave the same in terms of field NH3 reduction efficacy. This review is important for farmers, policymakers, emission inventory compilers and other stakeholders.
1 Introduction
The growing world population has increased food demand, resulting in increased use of organic and inorganic fertilisers to ensure sufficient crop productivity (Gojon et al., 2023; Guo et al., 2023). For example, it is estimated that mineral nitrogen (N) fertiliser supports approximately 46% of global food/feed production (Oita et al., 2016) and global N demand is expected to increase by 5% between 2021 and 2027 (Statista, 2024), such increases would drive further breaches of the safe planetary boundaries for N use set out by Schulte-Uebbing et al. (2022) and Campbell et al. (2017). Strategies to make better use of the N currently in circulation are urgently needed to stem the need for increases in N usage. A major challenge associated with N fertiliser is that on average less than 50% of applied fertiliser N is recovered by plant systems (Tilman et al., 2011) and less by animal systems (5%–30%) (Oenema et al., 2005). Fertiliser N usage is associated with ammonia (NH3) volatilization, denitrification loss including emission of the potent greenhouse gas nitrous oxide (N2O) along with nitrate (NO3−) leaching to waterbodies (Scheer et al., 2023) as illustrated in Figure 1. Pan et al. (2016) estimated that at global scale, an average of 18% applied urea N is lost through NH3 volatilisation. However, losses ranged greatly with between 0.9% and 64% loss being reported (Pan et al., 2016). In 2021, global urea N fertiliser usage was 53.8 million tonnes N (IFA, 2024) as outlined in Table 1. Based on the NH3 loss value of 18% (Pan et al., 2016) in 2021 approximately 9.7 million tonnes of N was lost through NH3 volatilisation from urea fertiliser usage globally (Table 1). If this N was retained through NH3 loss mitigation the projected increases in global N demand could be provided for without increasing N usage. Hence, implementation of NH3 loss mitigation strategies in farming systems is crucial to stem increases in N fertilisers’ usage further beyond the safe planetary boundaries set out by Schulte-Uebbing et al. (2022) and Campbell et al. (2017).
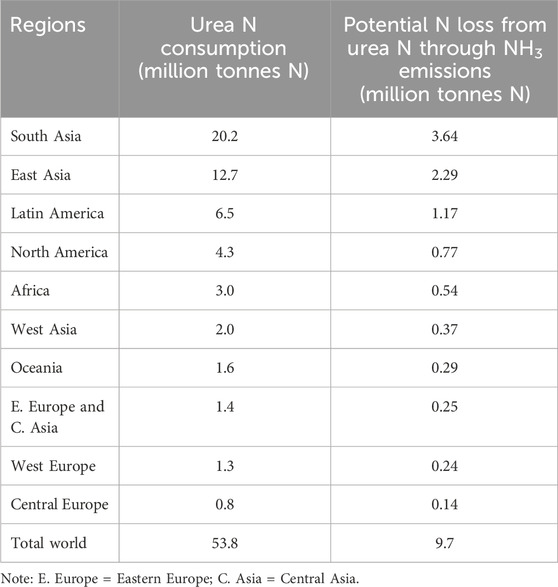
Table 1. Global N consumption from urea fertilisers during the 2021 period (IFA, 2024) and potential N losses through NH3 emissions. Potential NH3 emissions are calculated based on global meta-analysis by Pan et al. (2016) which reported that an average of 18% of N applied is lost through NH3 emissions.
1.1 Effects of NH3 volatilisation
Ammonia emissions pose a threat to human health worldwide. For example, in Europe and the United States, NH3 emissions from agriculture contribute to 50% and 30%, respectively of all PM2.5 (Erisman and Schaap, 2004). Literature evidence has shown that exposure to PM2.5 can cause serious health problems such as lung cancer, chronic obstructive pulmonary disease (COPD) and in some cases PM2.5 is associated with asthma and premature mortality (Burnett et al., 2014; Lim et al., 2012). Holst et al. (2018), investigated the association of atmospheric NH3, particulate NH4+ and the total concentration of PM2.5 with incidences of asthma in Danish preschool children (n = 335,629) during the period 2006–2012. The authors reported that exposure to high concentrations of NH3 and its components such as PM2.5 may increase the risk for the onset of asthma in preschool children. Furthermore, Lelieveld et al. (2015) estimated that the global PM2.5 related mortality in 2010 was 3.15 million people (95% CI = 1.52–4.60 million people). Given that exposure to NH3 and PM2.5 of which NH3 is a precursor poses a human health threat therefore, it is important to mitigate NH3 loss from a human health perspective. Previous studies have concluded that reducing NH3 emissions is the most effective control strategy for mitigation of PM2.5 and its associated adverse health effects (Pozzer et al., 2017; Tsimpidi et al., 2007).
Ammonia loss has financial implications for farmers. For example, globally we estimate (Table 1) that approximately 9.7 million tonnes of N was lost from urea fertiliser through NH3 volatilisation, and this equates to about US$6.8 billion (based on Statistica 2023 urea price of US$707 dollars per tonne N) in direct financial loss of fertiliser. There is a clear rationale for mitigation of NH3 volatilisation even purely from an on-farm economic perspective.
Ammonia emitted to the atmosphere reacts with aerosols containing sulphuric and nitric acids to create particulates such as ammonium nitrate, ammonium sulphate, and ammonium chloride (Gong et al., 2013; Wyer et al., 2022). These particulates travel and are deposited over short (4–5 km) or long distances (100–1,000 km) from the source (Asman et al., 1998; Krupa, 2003) as either dry particles (dry deposition at velocity of 14 cm s-1) or wet particles (wet deposition during rainfall events at rate of 0.2 cm s-1) (Krupa, 2003; Phillips et al., 2004; Wen et al., 2020) as illustrated in Figure 1. Both wet and dry N deposition processes have been strongly linked with serious environmental concerns such as accelerating eutrophication of water bodies (Draaijers et al., 1989; Kelleghan et al., 2019). Eutrophication can result in declines in aquatic life thus reducing aquatic biodiversity. Furthermore, deposition of NH3-N on soils and water bodies can cause acidification, which has detrimental effects on the availability of plant nutrient essential elements such as phosphorus, calcium, potassium, and magnesium (Pearson and Stewart, 1993), particularly in low N and sensitive ecosystems including those not under agricultural management. Ammonia volatilisation is regulated by EU laws. Member states must report NH3 emissions under the National Reduction Commitments Directive and monitor NH3 concentrations in sensitive areas under, the Habitats Directive (92/43/EEC) (Habitat Council Directive, 1992). The magnitude of reported NH3 effects on the environment indicates that there is an urgent need for research to find ways to mitigate NH3 losses from fertiliser use.
While NH3 volatilization is an international problem, in the European Union (EU) member states have committed to reduce NH3 losses under the National Emissions Reduction Commitments Directive (2016/2284/EU). Therefore, to meet commitments, NH3 mitigation practices are urgently needed to reduce NH3 volatilization in agricultural systems to reduce the economic, environmental, and human health impacts of NH3 loss.
1.2 Urease inhibitors
To improve the N use efficiency of synthetic mineral fertilisers and to reduce NH3 volatilisation, several mitigation strategies have been studied and implemented worldwide. The use of specific urease inhibitors have been reported to efficiently minimise NH3 volatilisation by slowing down urea hydrolysis (Kim et al., 2012; Sha et al., 2023; Watson et al., 1990). Several different inhibitors are commercially available globally. The most widely researched urease inhibitors are phosphorodiamide and phosphorotriamide derivatives (Singh et al., 2023). These include N–(n-butyl) thiophosphoric triamide (NBPT), N–(2-nitrophenyl) phosphoric triamide (2-NPT), and N-(n-propyl) thiophosphoric triamide (NPPT) (Modolo et al., 2018; Song et al., 2022). These phosphoramide inhibitors, NBPT, 2-NPT and a 3:1 ratio of NBPT + NPPT have been shown to be effective and practically applicable in agricultural field systems. For example, in a field study conducted in New Zealand by Dawar et al. (2011), the authors demonstrated that urea coated with NBPT at 0.1% (w/w) of urea N reduced NH3 volatilisation loss by 67% on average compared to standard urea in a spring application to a perennial ryegrass (Lolium perenne L.) and white clover (Trifolium repens L.) sward. Forrestal et al. (2016) reported that application of urea coated with urease inhibitor NBPT (0.066% (w/w) at two grassland experimental sites in Ireland decreased NH3 volatilisation by 79% on average across the two sites. Similarly, Krol et al. (2020) reported that NBPT and NBPT + NPPT reduced NH3 volatilisation by 68% on average relative to standard urea. In a 3 year study in winter-wheat conducted by Ni et al. (2014), urea coated with 2-NPT at 0.07 g N kg-1 decreased NH3 volatilisation by 62, 72, and 65% in 2011, 2012, and 2013, respectively. The inhibitory effect of these phosphoramide urease inhibitors is linked with these inhibitors being a structural analogue of urea as reported in several studies (Byrne et al., 2020; Font et al., 2008; Kot et al., 2001; Mazzei et al., 2017). In research reports, the use of these urease inhibitors has shown promising results, however their widespread application in farm systems has encountered challenges. There is limited information in literature that depicts which is the most effective urease inhibitor under various soil and climatic conditions.
1.3 Regulation of urease inhibitors in EU
In Europe, NBPT entered the Fertilising Products Regulation (FPR) of the European Commission (EC) as a urease inhibitor under Regulation EC No 1107/2008 of 7 November 2008, 2-NPT under Regulation No 223/2012 of 14 March 2012 and NBPT + NPPT trade name: Limus® under Regulation EC No 1257/2014 of 24 November 2014. The former EU Fertiliser Directive (EU 2003/2003) minimum and maximum augmentation of each of these three urease inhibitors by mass of total N present as urea N is outlined in Table 2. However, recently the criteria for entry into the EU fertiliser regulations were changed to provide a route via a European Conformity (CE) mark. Briefly, a CE mark is a marking by which the manufacturer indicates that the EU fertilising product is in conformity with set out requirements. The current (2024) CE criteria for a urease inhibitor outline that “a urease inhibitor shall inhibit hydrolytic action on urea (CH4N2O) by the urease enzyme, primarily targeted to reduce NH3 volatilisation” this is to be tested by comparing to a control sample where the urease inhibitor has not been added, “an in vitro test containing the urease inhibitor shall show a 20% reduction in the rate of hydrolysis of urea (CH4N2O) based on an analysis carried out 14 days after application at the 95% confidence level”. Under the CE mark testing NH3 loss mitigation is not measured (European Commission, 2019). Under the CE mark criteria, the maleic and itaconic acid co-polymer (MIP) was recently granted a CE mark as a urease inhibitor.
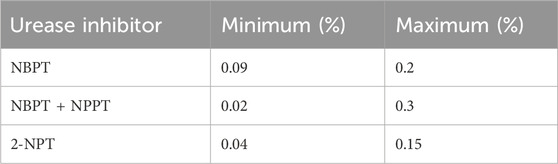
Table 2. Former EU Fertiliser Directive (EU 2003/2003) minimum and maximum urease inhibitor levels by mass of total N present as urea N.
However, despite many disparate NH3 field studies existing for NBPT, 2-NPT, NPPT + NBPT and MIP there is presently no review that compares them, which is an important gap given the pressure to provide NH3 loss mitigation options to the agriculture sector. Existing reviews focus on NBPT (Silva et al., 2017) and at most one additional inhibitor (e.g., NBPT + NPPT) (Fan et al., 2022; Pan et al., 2016), meaning that 2-NPT and MIP are omitted, a significant gap in literature. Therefore, a broader inter-comparison of all four compounds which focuses on efficacy under field conditions is urgently needed. The focus of the current review on field studies only is a further unique aspect of this review.
2 Materials and methods
2.1 Data search and selection criteria
The present review was conducted to outline the NH3 loss mitigation effect of adding NBPT, 2-NPT, NBPT + NPPT and MIP to solid urea or urea ammonium nitrate (UAN) liquid. The data synthesised in this study were sourced from peer-reviewed studies collected from the Web of science and Scopus databases. The keywords used during data search were “urease inhibitor/s” OR “NBPT” OR “2-NPT” OR “NBPT + NPPT” OR “Limus” OR “Nutrisphere” OR “MIP” AND “crop systems” AND“pasture systems” AND “ammonia” AND “volatilisation” OR “Volatilization”.
To evaluate the efficacy of the urease inhibitors, the data was selected based on the following criteria: (a) field study, (b) using either granular urea N or UAN, and (c) the study must include a urea or UAN reference that was not treated with a urease inhibitor. The PRISMA (Preferred Reporting Items for Systematic Reviews and Meta-analysis) statement methodology was used to ensure scientific quality (Figure 2). The review considered only peer-reviewed publications. The initial search of literature from Scopus and Web of science generated a total of 1,310 articles. After screening based on tittle and abstract, a total of 880 articles were excluded leaving 430 articles. Following the review objective which was to compare the efficacy of NBPT, 2-NPT, NBPT + NPPT and MIP for reducing NH3 volatilisation under field conditions, 332 studies failed to meet the criteria, leaving 98 articles. The full text of these 98 articles were thoroughly evaluated with a total of 50 found not to meet the review criteria. Forty eight (48) articles satisfied the criteria for this review (Figure 2). These 48 peer reviewed papers provided 256 comparisons and were used to evaluate the field efficacy of NBPT, 2-NPT, NBPT + NPPT and MIP. From the individual data sets NBPT had 165 comparisons (from 33 articles), 2-NPT had 19 comparisons (from 2 articles), NBPT + NPPT had 32 comparisons (from 7 articles) and MIP had 40 comparisons (from 6 articles). The data used originated from field testing at experimental sites in 14 countries across Europe, America, Asia and Oceania totalling 79 individual experimental sites. In this review, most of these field studies used the enclosure methods, micrometeorological and wind tunnels methods for NH3 measurement (Supplementary Table S1). Synthesized data was extracted from published study tables. Data from graphs were extracted using WebPlotDigitizer, https://automeris.io/WebPlotDigitizer/as used by Fan et al. (2022) into a database in Microsoft Excel software. All studies summarised in this review are presented in Figures 3A–D.
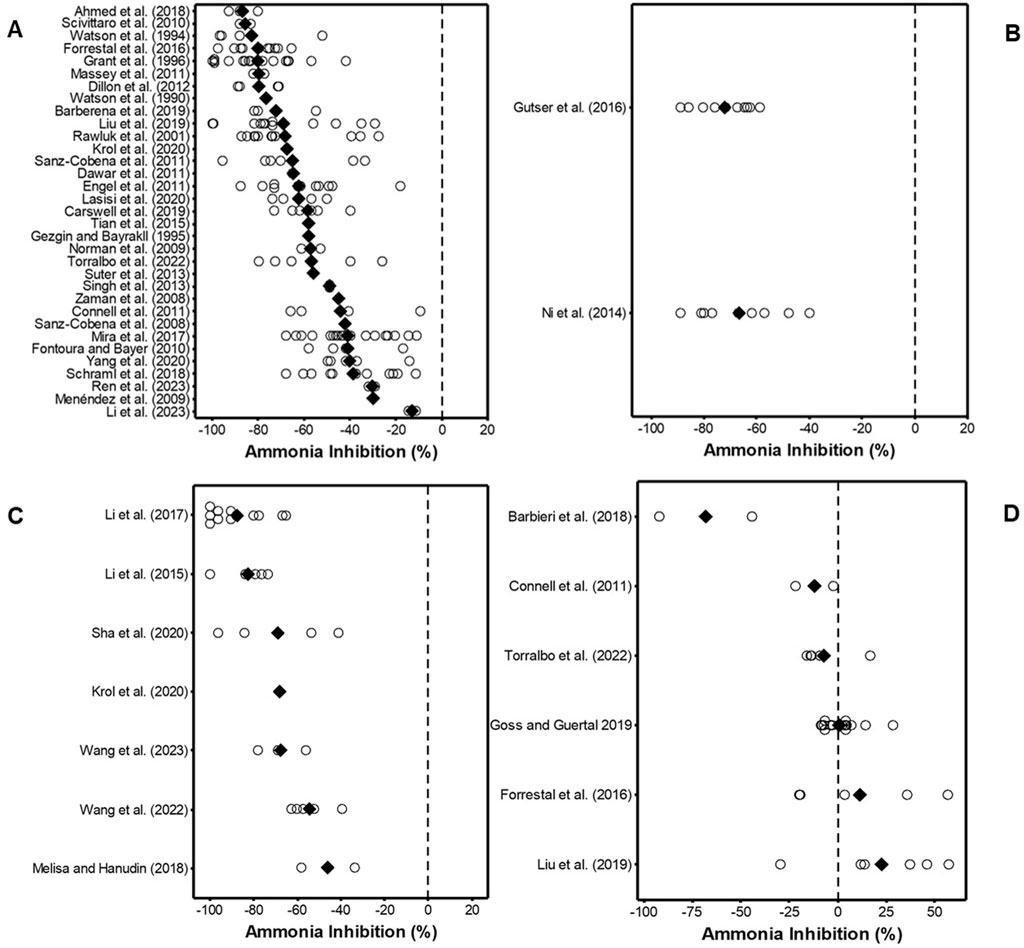
Figure 3. Effect of (A) NBPT, (B) 2-NPT (C) NBPT + NPPT and (D) MIP on NH3 inhibition relative to untreated urea N or UAN. White hollow points indicate individual data points for each study and solid back diamond (♦) points indicate mean value for each study. Negative values indicate reduced emissions and positive values indicate increased emissions. Studies in each graph are arranged by efficacy relative to either urea or UAN. All authors in the figures are included in the reference list.
2.2 Statistical description
In this review, NH3 mitigation values are presented as a percentage (%) calculated using Equation 1. Following an approach similar to Zheng et al. (2019), in this review a negative value mitigation % in Figures 3, 4 represents decreased emissions from the inhibited fertiliser and a positive percentage indicates increased emissions for the fertiliser with inhibitor relative to the untreated fertiliser.
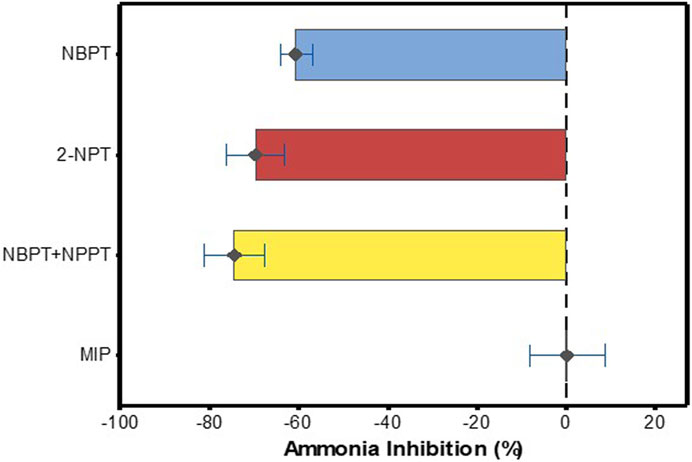
Figure 4. Effect of NBPT, 2-NPT, NBPT + NPPT and MIP on NH3 volatilisation relative to untreated urea N or UAN. Error bars show 95% CIs. Black diamonds (♦) points indicate mean value for each inhibitor from the synthesised data. 95% CIs that overlap with zero indicate no significant difference from the control (urea or UAN without inhibitor) treatment. Negative values indicate reduced emissions and positive values indicate increased emissions.
Mean values of each urease inhibitor were considered significantly different from the untreated control fertiliser when the 95% confidence intervals (95% CI) did not overlap zero. Mean values of each urease inhibitor with 95% CI that overlap each other are considered not significantly different from one another, and vice versa as used by Wall et al. (2024) and Fan et al. (2022). All data analysis in this current review was conducted using MiniTab (Version 19. Minitab Inc., United States).
3 Results and discussion
3.1 Mitigation of NH3 emissions through the use of urease inhibitors
The data displayed in this review (Table 1) shows that urea based N fertilisers are widely used globally for agricultural production thus measures to protect urea based fertilisers from NH3 losses have broad applicability globally as well as in the EU.
The data showed that, relative to standard urea or UAN, the phosphoramide inhibitors NBPT, decreased NH3 volatilisation by 13%–87% (range of the mean reduction) (Figure 3A), averaging a 61% reduction (95% CI = 57–64%, n = 165) (Figure 4). For 2-NPT, NH3 emissions were reduced by 67%–72% (range of the mean reduction) (Figure 3B), averaging a 70% reduction (95% CI = 63–76%, n = 19) (Figure 4). The use of NBPT + NPPT with either urea or UAN decreased NH3 volatilisation by 46%–88% (range of the mean reduction) (Figure 3C), averaging a 75% reduction (95% CI = 68–82%, n = 32) (Figure 4). In contrast, fertiliser N treated with MIP was not significantly different to the untreated fertiliser in the overall analysis, showing data range of −68 to 23 (range of the mean reduction) (Figure 3D), averaging an increase of 0.3% (95% CI = −8–9%, n = 40) in NH3 loss (Figure 4).
The data synthesised in this review clearly demonstrated that urease inhibitors can potentially reduce NH3 emissions in field agricultural systems however their efficacy differs. Among the different urease inhibitors, NBPT is the most widely researched urease inhibitor. NBPT has shown successful results ever since its introduction in the mid-1990s up-to-date (Ren et al., 2023; Watson et al., 1990; Zhengping et al., 1991) hence the greater number of available field studies. Results from the current review of global field studies demonstrates that NBPT is effective in decreasing urea fertiliser N losses through NH3 volatilization in field conditions across a range of soils, crops and environments. Compared to urea or UAN only, NBPT inclusion decreased NH3 volatilization by an average of 61%, n = 165. These mitigation results are lower than the values reported in a meta-analysis by Pan et al. (2016) who found that the use of urea treated with NBPT decreased NH3 volatilization by 69% on average. The difference between the results may be due to the fact that the current analysis focuses only in field efficacy while Pan et al. (2016) included laboratory incubations. Clough et al. (2007) reported the inclusion of incubation studies has often been associated with greater inhibitor effect than is observed in field studies and highlights the need for a field efficacy focused review where in field effects are needed by farmers, the environment and emissions inventory compliers. The current findings support the report of Clough et al., 2007 which suggested that field studies are associated with differing and reduced efficacy compared with laboratory studies on urease inhibitor efficacy. Thus, while laboratory incubation trials provide a useful proof of concept and mitigation potential, field testing is important to establish efficacy under in-vivo conditions.
The reduction in NH3 loss with the incorporation of NBPT has been shown to improve plant N recovery. For example, Krol et al. (2020) reported that urea plus NBPT significantly increased N recovery in perennial ryegrass (L. perenne. L) by 16% (31 kg N ha-1) relative to standard urea. Similarly, Dawar et al. (2011) reported that urea treated with NBPT significantly increased N recovery by 22% compared to standard urea. Furthermore, Harty et al. (2017) reported that application of urea coated with NBPT increased N uptake by of 9.8 kg N ha-1 across three locations over 2 years covering a range of soils and climatic conditions.
This review of the published field trial data on the urease inhibitor 2-NPT, introduced in the market in the 2000s, indicates a mean loss mitigation of 70%, n = 19 (Figure 4) compared to untreated fertiliser. Worth noting is that the 2-NPT data was extracted from two published manuscripts. Limited field studies have been conducted with 2-NPT and the lack of more field studies on 2-NPT is associated with more focus on NBPT which was introduced in late 90s. Nevertheless, in these synthesized studies, the use of 2-NPT was shown to be effective. Adhikari et al. (2019) showed that 2-NPT has greater longevity than NBPT. The stability of 2-NPT in soil relative to NBPT has been reported in other studies that have shown similar effects. Domínguez et al. (2008) found that the application of 2-NPT at 0.5% (w/w of N) inhibited urease activity by 65% and 12% after 10 and 30 days, respectively, while NBPT applied at the same rate inhibited urease activity by 40% and 0% after 10 and 30 days, respectively. The use of 2-NPT in conjunction with urea in field studies has consistently shown effective inhibition of NH3 loss. Furthermore, the use of 2-NPT has given NH3 loss mitigation from dairy cow urine patches under field conditions. Adhikari et al. (2020) used 2-NPT with 589.7 kg N ha-1 dairy cow urine during late-autumn (New Zealand) in Manawatu fine sandy loam soil (pH 5.5) in a perennial ryegrass (L. perenne L.) and white clover (T. repens L.) sward. Adhikari et al. (2020) reported that the application of 0.25% of 2-NPT reduced NH3 loss by 73% when 2-NPT was applied immediately (0 h) with urine and by 41% when 2-NPT was applied 3 hours after urine application. In the same study, the authors demonstrated that adding urine-N (730 kg N ha-1) 28 days after 0.21% 2-NPT application in summer (New Zealand) led to a 20% reduction in NH3 loss. Furthermore, Adhikari et al. (2020) reported that the application of urine + 2-NPT increased N recovery by an average of 1.3% (8 kg N ha-1) and 0.6% (4 kg N ha-1) in New Zealand summer and autumn, respectively compared to the urine only treatment across the different application periods studied.
The synthesised field studies data showed that the use of the urease inhibitor NBPT + NPPT decreased NH3 losses in field studies by an average of 75%, n = 32 (Figure 4). The reduction in NH3 volatilisation induced by the use of NBPT + NPPT has been shown to increase N use efficiency in crops. For example, Wang et al. (2023) demonstrated that the use of urea with NBPT + NPPT at 0.1% (w/w) increased N recovery in a 2 years field (2019–2021) maize-wheat rotation in China by more than 20% compared to standard urea treatment. Krol et al. (2020) reported that the N recovery in perennial ryegrass (L. perenne. L) was increased by 8% (16 kg N ha-1) by use of NBPT + NPPT urea relative to standard urea. These findings are further supported by Li et al. (2015) who showed that plant N recovery increased by 10%–16% when urea was treated with NBPT + NPPT.
In this current review, the overall analysis of field trials showed that MIP gave a mean NH3 emission increase from urea containing fertilisers of 0.3% (95% CI -8 to +9%), n = 40. MIP has recently acquired a CE mark as a urease inhibitor in Europe. The proposed mode of action of MIP in reducing NH3 volatilisation is associated with the maleic-itaconic acid co-polymer binding directly to the active site of the nickel ions found in the urease enzyme (Chien et al., 2014). Mazzei et al. (2018) tested the mode of action of MIP in an in-vitro study using purified urease at pH 7.5 and pH 5.0 in a pure environment with no soil or plants present. The study found that urease was not affected by MIP at pH 7.5 but the enzyme was completely inhibited at pH 5.0 by using MIP at the rate 0.4–2.4 μmol L-1. Mazzei et al. (2018) state that “MIPs have no significant inhibitory effect on urease activity at pH 7.5, a condition in which, on the other hand, NBPT inhibits the enzyme”. They reported that NBPT inhibited urease at both pH levels tested. Furthermore, Mazzei et al. (2018) state that their results “Confirms previous reports stating that MIP has no effect on urease activity in urea-fertilized soils in the pH range 5.9–7.3 (Franzen et al., 2011) or 6.5 to 8.1 (Goos, 2013)”. Goos (2018) conducted a study to determine if the findings of Mazzei et al. (2018) could be replicated when soil was included (three soils were used with pH levels of 4.5, 5.2, and 5.7). NBPT was also tested on the same soils. Goos (2018) reported that application of 5 mg kg-1 of NBPT inhibited urease activity by an average of 49% across all three soils, compared to 3% and 1% inhibition induced by 5 and 50 mg kg-1 of MIP across all three soils. Goos (2018) states that “The observation that MIP inhibits purified urease at a pH of 5.0 could not be repeated with soils with pH values near 5.0”. In the current review in Figure 3D, two points −90% and −44.4% are seen. Both points come from a field study by Barbieri et al. (2018) who reported that application of MIP at 0.25% w/w in a maize field significantly reduced total NH3 losses and the average 2 year reduction was 65% relative to standard urea. In contrast, Connell et al. (2011) reported that urea treated with MIP did not show significant differences in NH3 loss relative to standard urea. Furthermore, in a field study conducted by Forrestal et al. (2016) in Ireland, the authors found that application of urea 40 kg N ha-1 coated with MIP applied on five separate applications in a moderately drained loam soil did not show a significant reduction in NH3 loss relative to standard urea. Liu et al. (2019) reported that use of MIP at 0.1% (w/w) in a maize field significantly increased NH3 emissions by an average of 24.5% relative to standard urea across six experimental sites between 2013 and 2015. In controlled laboratory incubations, the use of MIP has shown some contrasting results. For example, in an incubation study conducted on one soil by Harty et al. (2023) urea coated with MIP (2.1 L Nutrisphere-N® tonne−1 urea) significantly reduced NH3 fluxes by 86% relative to the urea only treatment. Harty et al. (2023) conclude that “In controlled conditions, Nutrisphere-N® successfully reduced NH3 emissions compared to urea, and a field assessment of the NH3 emissions from urea, Nutrisphere-N® and other N inhibitors compared to urea is recommended.” Again emphasising the importance of field studies or reviews such as the present review that focus on field studies. In marked contrast to Harty et al. (2023) in a much larger laboratory incubation study using 79 soils representing a wide range of soil properties, Sunderlage and Cook (2018) reported that application of urea + MIP did not significantly (P = 0.9707) reduce NH3 loss across soils. In the same study NBPT + NPPT significantly reduced NH3 loss. Sunderlage and Cook (2018) concluded that “Although NBPT + NPPT significantly reduced NH3-N loss compared with untreated urea, MIP did not and should not be used as protection against volatilization”. Goos and Guertal (2019) reported NH3 inhibition of 6.8% (not significant) on average using MIP conducted in North Dakota, United States using sandy loam soil, pH = 7.3. The current review of field studies indicates that MIP, on average increased field NH3 loss by 0.3% (95% CI = −8 to +9%). In contrast, the phosphoramide inhibitors gave an NH3 reduction of 61% (95% CI = 57–64%), (NBPT), 70% (95% CI = 63–76%) (2-NPT) and 75% (95% CI = 68–82%) (NBPT + NPPT) under field conditions.
The use of urease inhibitors such as NBPT, 2-NPT and NBPT + NPPT has shown effectiveness in reducing NH3 volatilisation. However, previous studies have highlighted that their efficacy is influenced by soil, environmental and management factors. For example, Engel et al. (2015) investigated the effect of soil pH on NBPT degradation, they tested 10 mg NBPT kg-1 soil at pH 5.1, 6.1, 7.6 and 8.2. The study reported that the calculated half-life of NBPT was 0.07, 0.59, 2.70 and 3.43 days at soil pH 5.1, 6.1, 7.6 and 8.2, respectively. The authors concluded that degradation of NBPT is affected by soil pH, with faster degradation under the more acidic conditions. Goos (2018) explored the effect of NBPT on urea hydrolysis using three soils on a pH gradient, i.e. 4.5, 5.2 and 5.7 and they found that simultaneous application of urea (2.5 mg of urea per 5 g soil) and 5 mg NBPT kg-1 soil inhibited urea hydrolyses by 39, 63 and 75% at soil pH 4.5, 5.2 and 5.7, respectively. Watson et al. (1995) investigated the effectiveness of NBPT following application to 16 grassland soils and reported that the inhibition of NH3 loss was affected by soil type. The authors found that at 0.28% (w/w) NBPT rate, NH3 loss inhibition ranged from 54.4% to 99.4% in the different soils. Watson et al. (1995) also reported that the efficacy of NBPT was significantly and positively correlated with soil pH-H2O (r = 0.826, P < 0.001).
Soil texture has been shown to also influence the efficacy of urease inhibitors in field studies, showing lower efficacy in fine textured soils than in course textured soils (Fan et al., 2022; Gioacchini et al., 2002). This reduced effectiveness of urease inhibitors on fine textured soil has been discussed by Gioacchini et al. (2002), who linked the soil texture effect with the potential strong adsorption of inhibitors to clay fractions in fine textured soil thus reducing their efficacy. Chakraborty et al. (2023) suggested that the high water content in fine textured soil due to their higher water holding capacity can accelerate the degradation of inhibitors in these soils compared with course-textured soils.
Furthermore, the inhibitor rate used has been shown to influence the efficacy of urease inhibitors. Watson et al. (1994) reported that field application of urea amended with NBPT at 0.01, 0.05, 0.1, 0.25% and 0.5% (w/w) urea weight basis gave NH3 loss reductions of 52, 83, 88, 96% and 97%, respectively. Their findings demonstrated that increasing the rate of NBPT increased efficacy. In a later incubation study, Watson et al. (2008) evaluated the effect of NBPT addition rates (0.01, 0.025, 0.05, 0.075% and 0.1% (w/w)) urea weight basis to either coated urea or adding to UAN solutions with four contrasting soil types. The authors found that the average NH3 reduction over all soils and formulations was 61, 70, 74, 79% and 80% at 0.01, 0.25, 0.05, 0.075% and 0.1%, respectively, again highlighting the importance of inhibitor rate. Indeed, the EU FPR set-outs minimum and maximum inhibitor augmentation percentage by mass of the total N in Europe (Table 2). In Ireland, the application of urea coated with NBPT 0.066% (w/w) was shown to be effective in reducing NH3 loss by 79% on average across 10 applications at two grassland sites (Forrestal et al., 2016).
More studies of N treated with 2-NPT, NBPT + NPPT and MIP are still needed to better assess the effect of each soil property, environmental conditions and management factors on the effectiveness of these urease inhibitors in field conditions. Based on this review, researchers should ensure that the inhibitor rate, soil pH and texture are reported when publishing work as this information is not always provided.
4 Conclusion and future prospects
The present review of published field trials found that the addition of the phosphoramide urease inhibitors NBPT, 2-NPT and NBPT + NPPT to both solid urea and liquid urea containing fertilisers such as UAN reduced NH3 volatilisation. For these three inhibitors on average the NH3 reduction was 69%, n = 216. However, from the available field studies the effect of MIP on NH3 volatilisation was not different to zero, giving on average a 0.3% (95% CI -8 to +9%) increase. These results demonstrate that not all products behave the same in terms of field NH3 reduction efficacy, which is important for farmers, policymakers, emission inventory compilers and other stakeholders. Even-though NBPT, 2-NPT and NBPT + NPPT showed NH3 loss mitigation results across a range of field studies there is evidence from literature that their efficacy is influenced by soil properties, environmental factors and management practices. The type and rate of inhibitor chosen is one of the key factors affecting efficacy that can be controlled and should be considered carefully. The field trial literature data compiled in this study can be used to inform industry, policy makes, farmers and other stakeholders on the current evidence regarding urease inhibitor efficacy in agricultural field settings. The use of urease inhibitors can help to reduce N loss through NH3 volatilisation. If the average reduction of 69% was achieved for all urea there is potential to reduce the global NH3-N losses from urea alone from 9.7 to 3.0 million tonnes of urea-N. Such savings could be used to offset projected increased demands for total fertiliser N usage globally.
To achieve a better understanding and adoption of the urease inhibitors in agriculture field settings, the following area of research need to be strengthened.
1. The degradation of urease inhibitors during storage is an important factor that will affect their ultimate field efficacy. Therefore, more studies are needed on the degradation characteristics of these urease inhibitors during fertiliser product storage. Some studies have been done with NBPT (Lasisi et al., 2020; Sha et al., 2020) but limited or no previous studies have been published showing degradation rates with 2-NPT, NBPT + NPPT and MIP. A layer of confidence is provided in Europe where a regulatory minimum levels exist which define the inhibitor levels that must be present on the fertiliser at the point of sale. Assurance regarding inhibitor content is important for farmers and highlights the importance of inhibitor degradation studies and regulation in the sector.
2. Field research studies are needed to understand the effect of soil, environmental and management factors on the efficacy of 2-NPT, NBPT + NPPT and MIP as there is more limited published information for these compared to NBPT due to their more recent availability.
3. More research is needed to develop advanced models that can simulate the efficacy of urease inhibitors at global scale based on environmental factors, management practices and soil properties.
Data availability statement
The original contributions presented in the study are included in the article/Supplementary Material, further inquiries can be directed to the corresponding author.
Author contributions
DM: Data curation, Formal Analysis, Methodology, Software, Validation, Writing–original draft, Writing–review and editing. DK: Conceptualization, Funding acquisition, Validation, Writing–original draft, Writing–review and editing. KR: Conceptualization, Funding acquisition, Resources, Validation, Writing–original draft, Writing–review and editing. MD: Conceptualization, Funding acquisition, Investigation, Validation, Writing–original draft, Writing–review and editing. EC: Conceptualization, Funding acquisition, Investigation, Methodology, Writing–original draft, Writing–review and editing. XW: Data curation, Investigation, Validation, Writing–original draft, Writing–review and editing. PF: Conceptualization, Data curation, Funding acquisition, Methodology, Validation, Writing–original draft, Writing–review and editing.
Funding
The author(s) declare that financial support was received for the research, authorship, and/or publication of this article. This study was supported by Irish Department of Agriculture Food and Marine grant number 2021R447.
Acknowledgments
The authors would like to extend their sincere thanks to the Irish Department of Agriculture Food and Marine for funding support.
Conflict of interest
The authors declare that the research was conducted in the absence of any commercial or financial relationships that could be construed as a potential conflict of interest.
Publisher’s note
All claims expressed in this article are solely those of the authors and do not necessarily represent those of their affiliated organizations, or those of the publisher, the editors and the reviewers. Any product that may be evaluated in this article, or claim that may be made by its manufacturer, is not guaranteed or endorsed by the publisher.
Supplementary material
The Supplementary Material for this article can be found online at: https://www.frontiersin.org/articles/10.3389/fenvs.2024.1462098/full#supplementary-material
References
Adhikari, K. P., Saggar, S., Hanly, J. A., and Guinto, D. F. (2019). Comparing the effectiveness and longevity of the urease inhibitor N-(2-nitrophenyl) phosphoric triamide (2-NPT) with N-(n-butyl) thiophosphoric triamide (nBTPT) in reducing ammonia emissions from cattle urine applied to dairy-grazed pasture soils. Soil Res. 57 (7), 719–728. doi:10.1071/SR18337
Adhikari, K. P., Saggar, S., Hanly, J. A., and Guinto, D. F. (2020). Urease inhibitors reduced ammonia emissions from cattle urine applied to pasture soil. Nutr. Cycl. Agroecosyst. 117 (3), 317–335. doi:10.1007/s10705-020-10070-0
Ahmed, M., Yu, W., Lei, M., Raza, S., and Zhou, J. (2018). Mitigation of ammonia volatilization with application of urease and nitrification inhibitors from summer maize at the Loess Plateau. Plant, Soil and Environ. 64 (4), 164–172. doi:10.17221/46/2018-pse
Asman, W. A. H., Schjørring, J. K., and Sutton, M. A. (1998). Ammonia: emission, atmospheric transport and deposition. New Phytol. 139 (1), 27–48. doi:10.1046/j.1469-8137.1998.00180.x
Barbieri, P. A., Sainz Rozas, H. R., and Echeverría, H. E. (2018). Nitrogen recovery efficiency from urea treated with NSN Co-polymer applied to No-till corn. Agron 8 (8), 154. doi:10.3390/agronomy8080154
Burnett, R. T., Pope, C. A., Ezzati, M., Olives, C., Lim Stephen, S., Mehta, S., et al. (2014). An integrated risk function for estimating the global burden of disease attributable to ambient fine particulate matter exposure. Environ. Health Perspect. 122 (4), 397–403. doi:10.1289/ehp.1307049
Byrne, M. P., Tobin, J. T., Forrestal, P. J., Danaher, M., Nkwonta, C. G., Richards, K., et al. (2020). Urease and nitrification inhibitors—as mitigation tools for greenhouse gas emissions in sustainable dairy systems: a review. Sustainability 12 (15), 6018. doi:10.3390/su12156018
Campbell, B. M., Beare, D. J., Bennett, E. M., Hall-Spencer, J. M., Ingram, J. S. I., Jaramillo, F., et al. (2017). Agriculture production as a major driver of the Earth system exceeding planetary boundaries. Ecol. Soc. 22 (4), art8. Available at:. doi:10.5751/es-09595-220408
Carswell, A., Shaw, R., Hunt, J., Sánchez-Rodríguez, A. R., Saunders, K., Cotton, J., et al. (2019). Assessing the benefits and wider costs of different N fertilisers for grassland agriculture. Archives Agron. Soil Sci. 65 (5), 625–639. doi:10.1080/03650340.2018.1519251
Chakraborty, R., Purakayastha, T. J., Pendall, E., Dey, S., Jain, N., and Kumar, S., 2023. Nitrification and urease inhibitors mitigate global warming potential and ammonia volatilization from urea in rice-wheat system in India: a field to lab experiment. Sci. Total Environ., 898, 165479. doi:10.1016/j.scitotenv.2023.165479
Chien, S. H., Edmeades, D., McBride, R., and Sahrawat, K. L. (2014). Review of maleic–itaconic acid copolymer purported as urease inhibitor and phosphorus enhancer in soils. Agron. J. 106 (2), 423–430. doi:10.2134/agronj2013.0214
Clough, T. J., Di, H. J., Cameron, K. C., Sherlock, R. R., Metherell, A. K., Clark, H., et al. (2007). Accounting for the utilization of a N2O mitigation tool in the IPCC inventory methodology for agricultural soils. Nutr. Cycl. Agroecosyst. 78 (1), 1–14. doi:10.1007/s10705-006-9069-z
Connell, J. A., Hancock, D. W., Durham, R. G., Cabrera, M. L., and Harris, G. H., (2011). Comparison of enhanced-efficiency nitrogen fertilizers for reducing ammonia loss and improving bermudagrass forage production. Crop Sci., 51(5), 2237–2248. doi:10.2135/cropsci2011.01.0052
Dawar, K., Zaman, M., Rowarth, J. S., Blennerhassett, J., and Turnbull, M. H., (2011). Urease inhibitor reduces N losses and improves plant-bioavailability of urea applied in fine particle and granular forms under field conditions. Agric. Ecosyst. Environ., 144(1), 41–50. doi:10.1016/j.agee.2011.08.007
Dillon, K. A., Walker, T. W., Harrell, D. L., Krutz, L. J., Varco, J. J., Koger, C. H., et al. (2012). Nitrogen sources and timing effects on nitrogen loss and uptake in delayed flood rice. Agron. J., 104(2), 466–472. doi:10.2134/agronj2011.0336
Domínguez, M. J., Sanmartín, C., Font, M., Palop, J. A., San Francisco, S., Urrutia, O., et al. (2008). Design, synthesis, and biological evaluation of phosphoramide derivatives as urease inhibitors. J. Agric. Food Chem. 56 (10), 3721–3731. doi:10.1021/jf072901y
Draaijers, G. P. J., Ivens, W. P. M. F., Bos, M. M., and Bleuten, W. (1989). The contribution of ammonia emissions from agriculture to the deposition of acidifying and eutrophying compounds onto forests. Environ. Pollut. 60 (1), 55–66. doi:10.1016/0269-7491(89)90220-0
Engel, R. E., Towey, B. D., and Gravens, E., (2015). Degradation of the urease inhibitor NBPT as affected by soil pH. Soil Sci. Soc. Am. J., 79(6), 1674–1683. doi:10.2136/sssaj2015.05.0169
Erisman, J. W., and Schaap, M. (2004). The need for ammonia abatement with respect to secondary PM reductions in Europe. Environ. Pollut. 129 (1), 159–163. doi:10.1016/j.envpol.2003.08.042
European Commissions (2019). Regulation (EU) 2019/842 of the European Parliament and of the council of 30 May 2018 on binding annual greenhouse gas emission reductions by Member States from 2021 to 2030 contributing to climate action to meet commitments under the Paris agreement and amending regulation EU No 525/2013. Available at: https://eur-lex.europa.eu/legal-content/en/ALL/?uri=CELEX%3A32019R1009https://eur-lex.europa.eu/legal-content/en/ALL/?uri=CELEX%3A32019R1009 (Accessed December 12, 2023).
Fan, D., He, W., Smith, W. N., Drury, C. F., Jiang, R., Grant, B. B., et al. (2022). Global evaluation of inhibitor impacts on ammonia and nitrous oxide emissions from agricultural soils: a meta-analysis. Glob. Change Biol., 28(17), 5121–5141. doi:10.1111/gcb.16294
Font, M., Domínguez, M.-J., Sanmartín, C., Palop, J. A., San-Francisco, S., Urrutia, O., et al. (2008). Structural characteristics of phosphoramide derivatives as urease inhibitors. Requirements for activity. J. Agric. Food Chem. 56 (18), 8451–8460. doi:10.1021/jf801786d
Forrestal, P. J., Harty, M., Carolan, R., Lanigan, G. J., Watson, C. J., Laughlin, R. J., et al. (2016). Ammonia emissions from urea, stabilized urea and calcium ammonium nitrate: insights into loss abatement in temperate grassland. Soil Use Manage 32 (S1), 92–100. doi:10.1111/sum.12232
Franzen, D., Goos, R. J., Norman, R. J., Walker, T. W., Roberts, T. L., Slaton, N. A., et al. (2011). Field and laboratory studies comparing Nutrispher-nitrogen urea with urea in North Dakota, Arkansas, and Mississippi. J. Plant Nutr. 34 (8), 1198–1222. doi:10.1080/01904167.2011.558162
Gioacchini, P., Nastri, A., Marzadori, C., Giovannini, C., Vittori Antisari, L., and Gessa, C. (2002). Influence of urease and nitrification inhibitors on N losses from soils fertilized with urea. Biol. Fertil. Soils 36 (2), 129–135. doi:10.1007/s00374-002-0521-1
Gojon, A., Nussaume, L., Luu, D. T., Murchie, E. H., Baekelandt, A., Rodrigues Saltenis, V. L., et al. (2023). Approaches and determinants to sustainably improve crop production. Food Energy Secur 12 (1), e369. doi:10.1002/fes3.369
Gong, L., Lewicki, R., Griffin, R. J., Tittel, F. K., Lonsdale, C. R., Stevens, R. G., et al. (2013). Role of atmospheric ammonia in particulate matter formation in Houston during summertime. Atmos. Environ. 77, 893–900. doi:10.1016/j.atmosenv.2013.04.079
Goos, R. J. (2013). Effects of fertilizer additives on ammonia loss after surface application of urea–ammonium nitrate fertilizer. Commun. Soil Sci. Plant Anal. 44 (12), 1909–1917. doi:10.1080/00103624.2013.783061
Goos, R. J. (2018). Evaluation of Nutrisphere-N as an inhibitor of urease in soils with pH values near five. Soil Sci. Soc. Am. J. 82 (6), 1568–1571. doi:10.2136/sssaj2018.07.0258
Goos, R. J., and Guertal, E., (2019). Evaluation of commercial additives used with granular urea for nitrogen conservation. Agron. J., 111(3), 1441–1447. doi:10.2134/agronj2018.08.0493
Grant, C. A., Brown, K. R., Bailey, L. D., and Jia, S. (1996). Short Communication: volatile losses of NH3 from surface-applied urea and urea ammonium nitrate with and without the urease inhibitors NBPT or ammonium thiosulphate. Can. J. Soil Sci. 76 (3), 417–419. doi:10.4141/cjss96-050
Guo, T., Bai, S. H., Omidvar, N., Wang, Y., Chen, F., and Zhang, M. (2023). Insight into the functional mechanisms of nitrogen-cycling inhibitors in decreasing yield-scaled ammonia volatilization and nitrous oxide emission: a global meta-analysis. Chemosphere 338, 139611. doi:10.1016/j.chemosphere.2023.139611
Gutser, R., Maier, H., Schmidhalter, U., and Schraml, M. (2016). Ammonia loss from urea in grassland and its mitigation by the new urease inhibitor 2-NPT. J. Agric. Sci. 154 (8), 1453–1462. doi:10.1017/S0021859616000022
Habitat Council Directive. 1992. Habitat Council directtive 92/43/EEC of 21 May 1992 on the conservation of natural Habitats and of wild fauna and flora Accessed: 01 February 2024
Harty, M. A., Forrestal, P. J., Carolan, R., Watson, C. J., Hennessy, D., Lanigan, G. J., et al. (2017). Temperate grassland yields and nitrogen uptake are influenced by fertilizer nitrogen source. Agron. J. 109 (1), 71–79. doi:10.2134/agronj2016.06.0362
Harty, M. A., McDonnell, K. P., Whetton, R., Gillespie, G., and Burke, J. I. (2023). Comparison of ammonia-N volatilization losses from untreated granular urea and granular urea treated with NutriSphere-N®. Soil Use Manage., n/a 40 (n/a). doi:10.1111/sum.12891
Holst, G., Thygesen, M., Pedersen, C. B., Peel, R. G., Brandt, J., Christensen, J. H., et al. (2018). Ammonia, ammonium, and the risk of asthma: a register-based case–control study in Danish children. Environ. Epidemiol. 2 (3), e019. doi:10.1097/EE9.0000000000000019
IFA (2024). Global consumption of synthetic nitrogen fertilisers. Available at: http://www.ifastat.org/graph/1_3 (Accessed June 15, 2024).
Kelleghan, D. B., Hayes, E. T., Everard, M., and Curran, T. P. (2019). Mapping ammonia risk on sensitive habitats in Ireland. Sci. Total Environ. 649, 1580–1589. doi:10.1016/j.scitotenv.2018.08.424
Kim, D.-G., Saggar, S., and Roudier, P. (2012). The effect of nitrification inhibitors on soil ammonia emissions in nitrogen managed soils: a meta-analysis. Nutr. Cycl. Agroecosyst. 93 (1), 51–64. doi:10.1007/s10705-012-9498-9
Kot, M., Zaborska, W., and Orlinska, K. (2001). Inhibition of jack bean urease by N-(n-butyl)thiophosphorictriamide and N-(n-butyl)phosphorictriamide: determination of the inhibition mechanism. J. Enzyme Inhibition 16 (6), 507–516. doi:10.1080/14756360127569
Krol, D. J., Forrestal, P. J., Wall, D., Lanigan, G. J., Sanz-Gomez, J., and Richards, K. G. (2020). Nitrogen fertilisers with urease inhibitors reduce nitrous oxide and ammonia losses, while retaining yield in temperate grassland. Sci. Total Environ. 725, 138329. doi:10.1016/j.scitotenv.2020.138329
Krupa, S. V. (2003). Effects of atmospheric ammonia (NH3) on terrestrial vegetation: a review. Environ. Pollut. 124 (2), 179–221. doi:10.1016/S0269-7491(02)00434-7
Lasisi, A. A., Kumaragamage, D., and Akinremi, O. (2020). Stability of stored N-(n-butyl) thiophosphoric triamide (NBPT) treated urea-based fertilizers. Commun. Soil Sci. Plant Anal. 51 (7), 911–918. doi:10.1080/00103624.2020.1744632
Lelieveld, J., Evans, J. S., Fnais, M., Giannadaki, D., and Pozzer, A. (2015). The contribution of outdoor air pollution sources to premature mortality on a global scale. Nature 525 (7569), 367–371. doi:10.1038/nature15371
Li, Q., Cui, X., Liu, X., Roelcke, M., Pasda, G., Zerulla, W., et al. (2017). A new urease-inhibiting formulation decreases ammonia volatilization and improves maize nitrogen utilization in North China Plain. Sci. Rep. 7 (1), 43853. doi:10.1038/srep43853
Li, Q., Yang, A., Wang, Z., Roelcke, M., Chen, X., Zhang, F., et al. (2015). Effect of a new urease inhibitor on ammonia volatilization and nitrogen utilization in wheat in north and northwest China. Field Crops Res. 175, 96–105. doi:10.1016/j.fcr.2015.02.005
Lim, S. S., Vos, T., Flaxman, A. D., Danaei, G., Shibuya, K., Adair-Rohani, H., et al. (2012). A comparative risk assessment of burden of disease and injury attributable to 67 risk factors and risk factor clusters in 21 regions, 1990–2010: a systematic analysis for the Global Burden of Disease Study 2010. lancet 380 (9859), 2224–2260. doi:10.1016/S0140-6736(12)61766-8
Liu, S., Wang, X., Yin, X., Savoy, H. J., McClure, A., and Essington, M. E. (2019). Ammonia volatilization loss and corn nitrogen nutrition and productivity with efficiency enhanced UAN and urea under No-tillage. Sci. Rep. 9 (1), 6610. doi:10.1038/s41598-019-42912-5
Mazzei, L., Broll, V., and Ciurli, S. (2018). An evaluation of maleic-itaconic copolymers as urease inhibitors. Soil Sci. Soc. Am. J. 82 (4), 994–1003. doi:10.2136/sssaj2017.09.0323
Mazzei, L., Cianci, M., Contaldo, U., Musiani, F., and Ciurli, S. (2017). Urease inhibition in the presence of N-(n-Butyl)thiophosphoric triamide, a suicide substrate: structure and kinetics. Biochemistry 56 (40), 5391–5404. doi:10.1021/acs.biochem.7b00750
Melisa, M., and Hanudin, H. (2018). Effects of urease inhibitor and nitrification inhibitor on the nitrogen losses, physiological activity, and oil palm yield on red-yellow podzolic. Ilmu Pertan. Agric. Sci. 3 (3), 127–134. doi:10.22146/ipas.37291
Modolo, L. V., da-Silva, C. J., Brandão, D. S., and Chaves, I. S. (2018). A minireview on what we have learned about urease inhibitors of agricultural interest since mid-2000s. J. Adv. Res. 13, 29–37. doi:10.1016/j.jare.2018.04.001
Ni, K., Pacholski, A., and Kage, H. (2014). Ammonia volatilization after application of urea to winter wheat over 3 years affected by novel urease and nitrification inhibitors. Agric. Ecosyst. Environ. 197, 184–194. doi:10.1016/j.agee.2014.08.007
Oenema, O., Wrage, N., Velthof, G. L., van Groenigen, J. W., Dolfing, J., and Kuikman, P. J. (2005). Trends in global nitrous oxide emissions from animal production systems. Nutr. Cycl. Agroecosyst. 72 (1), 51–65. doi:10.1007/s10705-004-7354-2
Oita, A., Malik, A., Kanemoto, K., Geschke, A., Nishijima, S., and Lenzen, M. (2016). Substantial nitrogen pollution embedded in international trade. Nat. Geosci. 9 (2), 111–115. doi:10.1038/ngeo2635
Pan, B., Lam, S. K., Mosier, A., Luo, Y., and Chen, D. (2016). Ammonia volatilization from synthetic fertilizers and its mitigation strategies: a global synthesis. Ecosyst. Environ. 232, 283–289. doi:10.1016/j.agee.2016.08.019
Pearson, J., and Stewart, G. R. (1993). The deposition of atmospheric ammonia and its effects on plants. New Phytol. 125 (2), 283–305. doi:10.1111/j.1469-8137.1993.tb03882.x
Phillips, S. B., Arya, S. P., and Aneja, V. P. (2004). Ammonia flux and dry deposition velocity from near-surface concentration gradient measurements over a grass surface in North Carolina. Atmos. Environ. 38 (21), 3469–3480. doi:10.1016/j.atmosenv.2004.02.054
Pozzer, A., Tsimpidi, A. P., Karydis, V. A., de Meij, A., and Lelieveld, J. (2017). Impact of agricultural emission reductions on fine-particulate matter and public health. Atmos. Chem. Phys. 17 (20), 12813–12826. doi:10.5194/acp-17-12813-2017
Ren, B., Huang, Z., Liu, P., Zhao, B., and Zhang, J. (2023). Urea ammonium nitrate solution combined with urease and nitrification inhibitors jointly mitigate NH3 and N2O emissions and improves nitrogen efficiency of summer maize under fertigation. Field Crops Res. 296, 108909. doi:10.1016/j.fcr.2023.108909
Sanz-Cobena, A., Misselbrook, T. H., Arce, A., Mingot, J. I., Diez, J. A., and Vallejo, A. (2008). An inhibitor of urease activity effectively reduces ammonia emissions from soil treated with urea under Mediterranean conditions. Agric. Ecosyst. Environ. 126 (3), 243–249. doi:10.1016/j.agee.2008.02.001
Scheer, C., Rowlings, D. W., Antille, D. L., De Antoni Migliorati, M., Fuchs, K., and Grace, P. R. (2023). Improving nitrogen use efficiency in irrigated cotton production. Nutr. Cycl. Agroecosyst. 125 (2), 95–106. doi:10.1007/s10705-022-10204-6
Schraml, M., Weber, A., Heil, K., Gutser, R., and Schmidhalter, U. (2018). Ammonia losses from urea applied to winter wheat over four consecutive years and potential mitigation by urease inhibitors. J. Plant Nutr. Soil Sci. 181 (6), 914–922. doi:10.1002/jpln.201700554
Schulte-Uebbing, L. F., Beusen, A. H. W., Bouwman, A. F., and de Vries, W. (2022). From planetary to regional boundaries for agricultural nitrogen pollution. Nature 610 (7932), 507–512. doi:10.1038/s41586-022-05158-2
Sha, Z., Lv, T., Staal, M., Ma, X., Wen, Z., Li, Q., et al. (2020). Effect of combining urea fertilizer with P and K fertilizers on the efficacy of urease inhibitors under different storage conditions. J. Soils Sed. 20 (4), 2130–2140. doi:10.1007/s11368-019-02534-w
Sha, Z., Wang, J., Ma, X., Lv, T., Liu, X., and Misselbrook, T. (2023). Ammonia loss potential and mitigation options in a wheat-maize rotation system in the North China Plain: a data synthesis and field evaluation. Agric. Ecosyst. Environ. 352, 108512. doi:10.1016/j.agee.2023.108512
Silva, A. G. B., Sequeira, C. H., Sermarini, R. A., and Otto, R. (2017). Urease inhibitor NBPT on ammonia volatilization and crop productivity: a meta-analysis. Agron. J. 109 (1), 1–13. doi:10.2134/agronj2016.04.0200
Singh, R., Kumar, P., Devi, M., Sindhu, J., Kumar, A., Lal, S., et al. (2023). Urease inhibition and structure-activity relationship study of thiazolidinone-triazole-and benzothiazole-based heterocyclic derivatives: a focus review. ChemistrySelect 8 (13), e202300244. doi:10.1002/slct.202300244
Song, W.-Q., Liu, M.-L., Li, S.-Y., and Xiao, Z.-P. (2022). Recent efforts in the discovery of urease inhibitor identifications. Curr. Top. Med. Chem. 22 (2), 95–107. doi:10.2174/1568026621666211129095441
Statista (2024). World Fertilizer demand medium term forecast 2027. Available at: https://www.statista.com/statistics/438964/medium-forecast-fertilizer-demand-globally-by-nutrient/https://www.statista.com/statistics/438964/medium-forecast-fertilizer-demand-globally-by-nutrient/(Accessed March 11, 2024).
Sunderlage, B., and Cook, R. L. (2018). Soil property and fertilizer additive effects on ammonia volatilization from urea. Soil Sci. Soc. Am. J. 82 (1), 253–259. doi:10.2136/sssaj2017.05.0151
Suter, H., Sultana, H., Turner, D., Davies, R., Walker, C., and Chen, D. (2013). Influence of urea fertiliser formulation, urease inhibitor and season on ammonia loss from ryegrass. Nutr. Cycl. Agroecosyst. 95 (2), 175–185. doi:10.1007/s10705-013-9556-y
Tian, Z., Wang, J. J., Liu, S., Zhang, Z., Dodla, S. K., and Myers, G. (2015). Application effects of coated urea and urease and nitrification inhibitors on ammonia and greenhouse gas emissions from a subtropical cotton field of the Mississippi delta region. Sci. Total Environ. 533, 329–338. doi:10.1016/j.scitotenv.2015.06.147
Tilman, D., Balzer, C., Hill, J., and Befort, B. L. (2011). Global food demand and the sustainable intensification of agriculture. Proc. Natl. Acad. Sci. 108 (50), 20260–20264. doi:10.1073/pnas.1116437108
Torralbo, F., Boardman, D., Houx, J. H., and Fritschi, F. B. (2022). Distinct enhanced efficiency urea fertilizers differentially influence ammonia volatilization losses and maize yield. Plant Soil 475 (1), 551–563. doi:10.1007/s11104-022-05387-4
Tsimpidi, A. P., Karydis, V. A., and Pandis, S. N. (2007). Response of inorganic fine particulate matter to emission changes of sulfur dioxide and ammonia: the eastern United States as a case study. J. Air Waste Manage. Assoc. 57 (12), 1489–1498. doi:10.3155/1047-3289.57.12.1489
Wall, A. M., Laubach, J., Campbell, D. I., Goodrich, J. P., Graham, S. L., Hunt, J. E., et al. (2024). Effects of dairy farming management practices on carbon balances in New Zealand’s grazed grasslands: synthesis from 68 site-years. Agric. Ecosyst. Environ. 367, 108962. doi:10.1016/j.agee.2024.108962
Wang, J., Kang, J., Sha, Z., Qu, Z., Niu, X., Xu, W., et al. (2022). Mitigation of ammonia volatilization on farm using an N stabilizer – a demonstration in Quzhou, North China Plain. Environ. 336, 108011. doi:10.1016/j.agee.2022.108011
Wang, J., Sha, Z., Zhang, J., Qin, W., Xu, W., Goulding, K., et al. (2023). Improving nitrogen fertilizer use efficiency and minimizing losses and global warming potential by optimizing applications and using nitrogen synergists in a maize-wheat rotation. Agric. Ecosyst. Environ. 353, 108538. doi:10.1016/j.agee.2023.108538
Watson, C. J., Akhonzada, N. A., Hamilton, J. T. G., and Matthews, D. I. (2008). Rate and mode of application of the urease inhibitor N-(n-butyl) thiophosphoric triamide on ammonia volatilization from surface-applied urea. Soil Use Manage 24 (3), 246–253. doi:10.1111/j.1475-2743.2008.00157.x
Watson, C. J., Miller, H., Poland, P., Kilpatrick, D. J., Allen, M. D. B., Garrett, M. K., et al. (1995). Soil properties and the ability of the urease inhibitor N-(n-BUTYL) thiophosphoric triamide (nBTPT) to reduce ammonia volatilization from surface-applied urea. Soil Biol. biochem. 26 (9), 1165–1171. doi:10.1016/0038-0717(94)90139-2
Watson, C. J., Poland, P., Miller, H., Allen, M. B. D., Garrett, M. K., and Christianson, C. B. (1994). Agronomic assessment and 15N recovery of urea amended with the urease inhibitor nBTPT (N-(n-butyl) thiophosphoric triamide) for temperate grassland. Plant Soil 161 (2), 167–177. doi:10.1007/BF00046388
Watson, C. J., Stevens, R. J., and Laughlin, R. J. (1990). Effectiveness of the urease inhibitor NBPT (N-(n-butyl) thiophosphoric triamide) for improving the efficiency of urea for ryegrass production. Fertilizer Res. 24 (1), 11–15. doi:10.1007/BF01073142
Wen, Z., Xu, W., Li, Q., Han, M., Tang, A., Zhang, Y., et al. (2020). Changes of nitrogen deposition in China from 1980 to 2018. Environ. Int. 144, 106022. doi:10.1016/j.envint.2020.106022
Wyer, K. E., Kelleghan, D. B., Blanes-Vidal, V., Schauberger, G., and Curran, T. P. (2022). Ammonia emissions from agriculture and their contribution to fine particulate matter: a review of implications for human health. J. Environ. Manage. 323, 116285. doi:10.1016/j.jenvman.2022.116285
Yang, G., Ji, H., Sheng, J., Zhang, Y., Feng, Y., Guo, Z., et al. (2020). Combining Azolla and urease inhibitor to reduce ammonia volatilization and increase nitrogen use efficiency and grain yield of rice. Sci. Total Environ. 743, 140799. doi:10.1016/j.scitotenv.2020.140799
Zheng, H., Ying, H., Yin, Y., Wang, Y., He, G., Bian, Q., et al. (2019). Irrigation leads to greater maize yield at higher water productivity and lower environmental costs: a global meta-analysis. Agric. Ecosyst. Environ. 273, 62–69. doi:10.1016/j.agee.2018.12.009
Keywords: ammonia volatilisation, urease inhibitors, mitigation, nitrogen, urea
Citation: Matse DT, Krol DJ, Richards KG, Danaher M, Cummins E, Wang X and Forrestal PJ (2024) Field efficacy of urease inhibitors for mitigation of ammonia emissions in agricultural field settings: a systematic review. Front. Environ. Sci. 12:1462098. doi: 10.3389/fenvs.2024.1462098
Received: 09 July 2024; Accepted: 10 September 2024;
Published: 23 September 2024.
Edited by:
Yao Zhang, Colorado State University, United StatesReviewed by:
Yansheng Li, Northeast Institute of Geography and Agroecology, Chinese Academy of Sciences (CAS), ChinaSimon Svane, University of Southern Denmark, Denmark
Copyright © 2024 Matse, Krol, Richards, Danaher, Cummins, Wang and Forrestal. This is an open-access article distributed under the terms of the Creative Commons Attribution License (CC BY). The use, distribution or reproduction in other forums is permitted, provided the original author(s) and the copyright owner(s) are credited and that the original publication in this journal is cited, in accordance with accepted academic practice. No use, distribution or reproduction is permitted which does not comply with these terms.
*Correspondence: Dumsane Themba Matse, ZHVtc2FuZS5tYXRzZUB0ZWFnYXNjLmll