- 1Research Unit Comparative Microbiome Analysis, Helmholtz Zentrum München, Neuherberg, Germany
- 2Terrestrial Ecology Research Group, Department of Ecology and Ecosystem Management, School of Life Sciences Weihenstephan, Technische Universität München, Freising, Germany
- 3Chair for Environmental Microbiology, Department of Ecology and Ecosystem Management, TUM School of Life Sciences, Technical University of Munich, Freising, Germany
- 4Department of Earth, Environment and Life Sciences, University of Genoa, Genoa, Italy
Introduction
Urban biodiversity faces major obstacles resulting from simultaneous reductions in green space, habitat fragmentation, and environmental changes (Beninde et al., 2015). All of these affects biodiversity in urban areas as well as the provision of the associated ecosystem services with direct negative consequences for all living organisms in urban areas including humans (Cardinale et al., 2012). Nature-based solutions (NbS), which involve sustainable management and restoration of ecosystems to address societal challenges and enhance human wellbeing and biodiversity (Cohen-Shacham et al., 2016), are crucial for improving urban biodiversity. These solutions include green roofs and facades, urban parks, gardens and urban agriculture, all of which becomes increasingly important (Dempsey and Jenks, 2010; Filazzola et al., 2019). The successful implementation of these elements into urban landscapes strongly depends on the quality of the soils or substrates used. Recent studies have shown that multifunctionality and soil resilience, strongly depend on their biotic properties, including biomass, diversity of micro- and macro-organisms along with their activity (Zak et al., 2003; Lange et al., 2015; Weisser et al., 2017; Prommer et al., 2020). However, the microbiome of urban soils and substrates is often in a dysbiotic state, i.e., both the identity and diversity of microbiota is different from natural soils (Clayton et al., 2021). Therefore, improving the diversity and activity of the soil microbiome may result in several advantages for the respective soils or substrates. However, strategies on how to manage low microbial diversity in urban soils and substrates are still not well established (Fulthorpe et al., 2018). Here we discuss if strategies from agriculture or restoration ecology can be implemented in urban settings to manage microbial diversity.
Technosols – what is special about soils and substrates in urban settings?
As a result of construction activities in urban areas, such as excavations, compression from heavy machinery, and calcium accumulation from cement, gypsum, and irrigation, urban soils are characterized by increased pH values and numerous disturbances (Pouyat et al., 2010). These result in compaction, poor soil structure, including a lack of aggregates and soil pores of all size classes, reduced nutrient and organic matter contents, and increased concentrations of organic and inorganic pollutants (Pouyat et al., 2010). The consequences of these disturbances for life in the soil are exacerbated by additional stress factors such as the urban heat island effect (Buzzard et al., 2021) and prolonged periods of drought mainly in summer (Changnon, 2000). Subsequently negative effects for water permeability, gas exchange, and the ability of plant roots to grow in such environments are frequently observed (Craul, 1985; Byrne, 2007; Pouyat et al., 2010; Faeth et al., 2011). Urban soils range from semi-natural soils (e.g., in parks) to Technosols and in worst case to Ekranic Technosols. According to the WRB classification of soils, Technosols are characterized by a strong human influence and contain a large proportion of artefacts (IUSS Working Group WRB, 2022), where the Ekranic Technosols are referring to sealed surfaces, which however will be out of scope as they likely need a very special treatment. Importantly, Technosols are not a soil class with clearly defined genesis and chemical properties as other soils in the soil classification but vary dramatically in their chemical and physical properties (Schad, 2018). Another special case of urban Technosols are substrates used for green roofs or facades. Their physical properties like weight and water holding capacity are optimized for the built environment. For example, the mixture of artificially modified and recycled materials used for green roofs is much lighter than finite natural soils and requires minimal irrigation and fertilization due to the materials it contains (Ampim et al., 2010). However, biotic properties supporting ecosystem functions and plant microbe interactions are often hampered (Ondoño et al., 2014; Fulthorpe et al., 2018), but are beneficial for plant performance under the relatively harsh abiotic conditions with shallow soil depth and direct sunlight on green roofs (Ampim et al., 2010).
The importance of positive feedback loops between above- and below-ground biodiversity for the resilience and multifunctionality of soils
Under optimal conditions soils promote plant growth by providing nutrients, water and diverse ecological niches (Breure, 2004; Guilland et al., 2018). This enables the growth of different plants, which in turn increases carbon transfer to the soil, leading to higher carbon stocks (Barrios, 2007) and greater microbial diversity and activity (Ehrenfeld et al., 2005). These interactions between soil, plants, and microbes influence each other’s functions positively and have an impact on the ecosystem services provided (Ehrenfeld et al., 2005). Based on the classification by Adhikari and Hartemink (2016), we posit that the disturbed properties of urban soils, which are centrally considered due to their significant influence, adversely affect the quality of ecosystem services (Figure 1). Nutrient-rich soils with diverse microbial communities promote higher above-ground biomass, while diverse plant communities support below-ground biodiversity (Morel et al., 2015; Saccá et al., 2017; Prommer et al., 2020). These beneficial interactions, particularly with plant growth-promoting bacteria (PGPB) and fungi (PGPF) improve soil colonization (Cecchi et al., 2021; Singh et al., 2023). Positive feedbacks between above- and below-ground biodiversity in natural and seminatural areas are well-documented (Eisenhauer, 2012; Jing et al., 2015). A wide cropping sequence or catch crops, for example, improve soil biodiversity and crop yields through increased nutrient mobilization and pathogen control (Pattnaik et al., 2021). However, such concepts are missing for urban soils, where management strategies for Technosols must consider their unique properties and constraints.
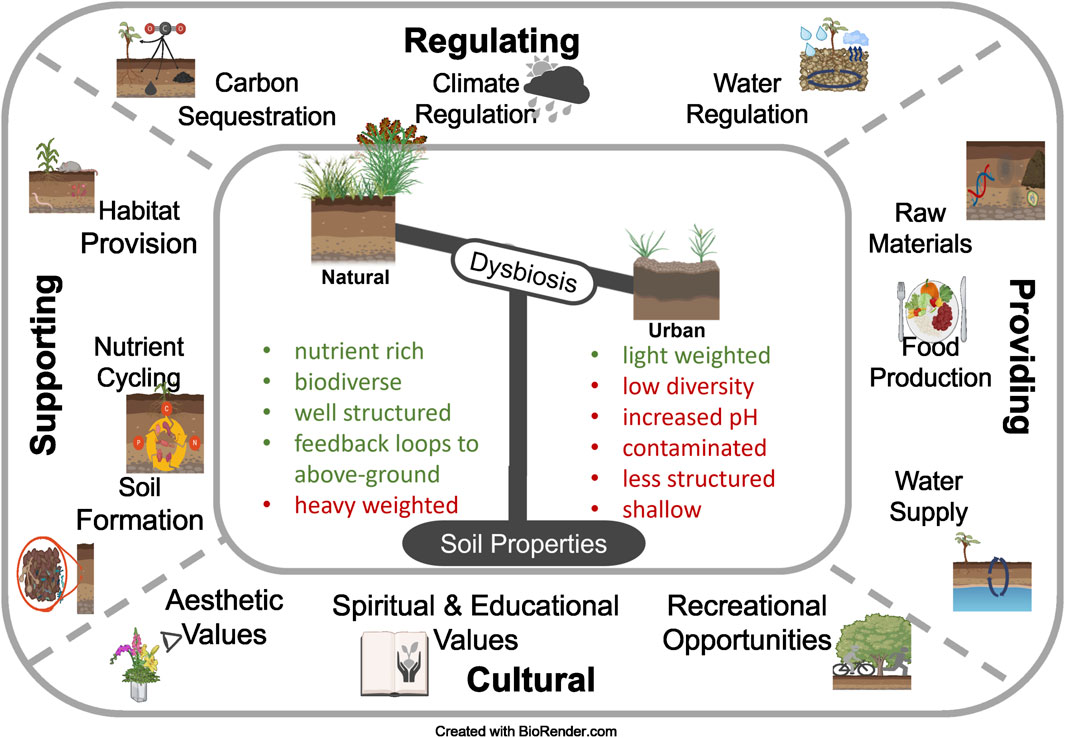
Figure 1. Ecosystem services of urban soil - a direct correlation exists between the various soil properties (pH value, organic matter content, fragment composition, bulk density, etc.) and the ecosystem services provided. Properties marked in green show the positive and those marked in red the negative effects on biodiversity and the functionality of the ecosystem due to the differences of natural and urban soils. The dysbiosis shown affects the four areas of ecosystem services provided (supporting, regulating, providing and cultural services, respectively). The figure was created with BioRender.com.
How to improve and maintain microbial biodiversity in Technosols?
Studies of natural chronosequences like glacier forefields demonstrated that microbial succession to a climax ecosystem can take hundreds of years (Baer et al., 2002; Schulz et al., 2013). To accelerate this process, restoration ecology uses targeted actions to restore disturbed ecosystems faster (Waterhouse et al., 2014; Luna et al., 2016; Perkins and Hatfield, 2016). Here we outline options inspired by strategies from agriculture and restoration ecology to increase and maintain microbial biodiversity in urban Technosols.
Introducing microbial inoculants has been successfully applied to increase soil microbial diversity (Calvo et al., 2014; Delmont et al., 2014), making it a popular strategy for restoration and agriculture (Luna et al., 2016; Schmid et al., 2020). Single strain inoculation used as biofertilizer (Wong et al., 2014) or biopesticide (Müller and Berg, 2008), can also be used to generally improve ecosystem services (Dwivedi and Soni, 2011). The effectiveness of inoculation depends on parameters like organic matter (Farrell et al., 2020), available nutrients like phosphorus (Rooney and Clipson, 2009), and pH (Ferguson et al., 2013), as well as the competitiveness of the existing microbial community (O’Callaghan et al., 2022). In urban settings, successful strains must improve soil quality and initiate positive feedback loops with above-ground biodiversity, while being resilient to urban soil constraints like pollution and poor structure. Although single strains can enhance plant stress tolerance, for example against heavy metals, the numerous stressors in Technosols makes it unlikely that a single strain can provide all needed functional traits. Instead, defined microbial consortia with complementary functions and synchronized interactions may be more effective. The development and application of such synthetic communities (SynComs) (Shayanthan et al., 2022), can be tailored to address the different environmental stressors like drought, heat, salinity, and pollutants. This novel approach thus enables the customized use of microbial inocula depending on the conditions in urban environments. Recent high-throughput strain isolations provide numerous microbes with known physiology for such strategies (Valliere et al., 2020) like microbes which induce stress-resistance, e.g., by forming exopolysaccharides or by degrading pollutants.
Another approach to improve urban soil microbiomes is soil inoculation, which uses a small amount of natural soil as inoculum instead of poor surface capping materials available in huge amounts (Wubs et al., 2016). Similar approaches were already used to improve other soil properties like bulk density by adding compost (Kranz et al., 2020). The inoculation of microbes by using small amounts of soil has been already successfully used in restoring degraded terrestrial ecosystems, such as German post-mining areas, where loess material is mixed with 10% original soil and improves soil quality within a few years (Pihlap et al., 2019; Schmid et al., 2020; Vuko et al., 2020). Although cost-efficient and easy to implement, this black box approach lacks detailed knowledge of the inoculum composition (Allison and Martiny, 2008), making a targeted use difficult. Additionally, the original soil must be preserved during construction work, which requires advanced planning. If the original soil is lost or contaminated, it can be difficult to find similar soils with suitable abiotic properties for transplanting (Boivin et al., 2002). Metalliferous or soils with high organic matter content (e.g., naturally occurring phenols), where the corresponding soil microbiome had centuries to adapt to the prevailing conditions and could be considered ‘extremophilic’, may serve as valuable sources for the inoculating urban soils.
Whether the establishment of a plant beneficial microbiome is successful strongly relies on the plant community as well as plants provide the essential ecological niches and carbon sources for the plant-associated microbiota (Tsiknia et al., 2021). Thus, a successful inoculation approach requires a combined application of PGPB and the target plant species that benefits from the functional properties of the introduced microbes. Legumes (e.g., plants from the Fabaceae family) and their symbionts are well-researched in this regard and can be used on green roofs to improve soil structure and nutrient contents. However, green roofs are extreme habitats with challenges like substrate depth, temperature fluctuations, water availability, and wind speed (Lundholm, 2006; Madre et al., 2014). Thus, current plant selection focuses on adaptability to harsh conditions and drought resilience (Lundholm and Walker, 2018), favoring drought-tolerant species like Crassulaceae succulents, which have low cooling performance, because of their CAM metabolism which enables them to reduce their transpiration during the day/summer (Blanusa et al., 2013). However, relying solely on Crassulaceae does not improve soil structure, microbial communities, or above-ground biodiversity. In contrast to roofs dominated by a single plant species, diverse plant communities can be used, which are characterized by high species richness and thus provide more and temporally stable ecosystem function than less diverse communities (Allan et al., 2011). At the same time, the number of ecological niches for the microbiota increases, which reduces competition for nutrients and space for the plants (Ashton et al., 2010). In this regard, Hoch et al. (2019) showed that green roofs hosting diverse plant species of wildflowers and grasses exhibited elevated diversity among root-associated microorganisms along with reduced pathogen diversity compared to green roofs consisting of sedum species only. Diverse plant mixtures with grasses also retain rainwater better compared to classical succulents (Dunnett et al., 2008). Moreover, the use of different plant species on green roofs can reduce urban fragmentation and serves as a green corridor in an urban green network with higher seed exchange and vegetative propagation (Beninde et al., 2015). Thus, increased plant diversity on green roofs has the potential to improve both the diversity of microbiomes in the substrate as well as the overall performance of the ecosystem inducing the described positive feedback loops between above-ground performance and below-ground processes and activities. Anyhow in many areas we are still lacking the right plant communities for such approaches. In addition, usually plants are chosen because of their aesthetic value, even if they are exotic and sometimes invasive, with pronounced consequences for microbial life in soil, as many of those plants are capable to produce phytoalexins and other toxins, which are exuded into soil (Kourtev et al., 2002).
Discussion
Soil microorganisms act as a linchpin between soil and plants, that grow in it. In this publication, we have presented two options for improving urban soils or substrates. Recently, the understanding of mutual plant-microbe interactions has improved, and focus has shifted to the important effects of soil microorganisms. We agree with O’Callaghan et al. (2022) on the importance of understanding the ecology and mode of action of vaccinated strains in order to optimize their efficacy and targeted use. Therefore, careful consideration of site-specific conditions and further research into microbial community dynamics and potential unintended consequences are essential for successful implementation. Stressors differ temporally and spatially in an urban environment and the interactions caused by multiple stressors also need to be better understood. Particularly in urban settings, consequences for human health must be considered. This includes the possibility to introduce potential human pathogens or microbes, which may carry antibiotic resistance genes as a matter of co-selection, which they can transfer to human pathogenic bacteria, but also issues like allergens which might be introduced by plant species used for maintaining microbial diversity in soil. Additionally, the importance of soil conservation in urban areas must be considered. Many of these soils can be considered at least as semi natural and may not be real Technosols. A careful treatment of these soils during construction, avoiding compaction and pollution would improve the situation of urban soils (Kumar and Hundal, 2016). Preserved soil can also be used as soil inoculant in already degraded urban areas leading to self-regeneration cycles of those soils. Despite the challenges described, we believe that there are implementable opportunities to strengthen urban ecosystem services based on an improvement of the soil microbiome. Here combined approaches of microbial inoculation of single strains, SynComs or the transplantation of soils together with the best fitting plant species might open a window of new opportunities.
Author contributions
AS: Writing–original draft, Writing–review and editing. MS: Conceptualization, Funding acquisition, Supervision, Writing–original draft, Writing–review and editing. ER: Funding acquisition, Writing–review and editing. WW: Funding acquisition, Project administration, Writing–review and editing. SS: Supervision, Writing–original draft, Writing–review and editing, Conceptualization.
Funding
The author(s) declare that financial support was received for the research, authorship, and/or publication of this article. This work was funded by ECOLOPES project [H2020-EU.1.2.1 - FET Open (Grant Agreement ID 964414)].
Conflict of interest
The authors declare that the research was conducted in the absence of any commercial or financial relationships that could be construed as a potential conflict of interest.
MS declared that they were an editorial board member of Frontiers, at the time of submission. This had no impact on the peer review process and the final decision.
Publisher’s note
All claims expressed in this article are solely those of the authors and do not necessarily represent those of their affiliated organizations, or those of the publisher, the editors and the reviewers. Any product that may be evaluated in this article, or claim that may be made by its manufacturer, is not guaranteed or endorsed by the publisher.
References
Adhikari, K., and Hartemink, A. E. (2016). Linking soils to ecosystem services—a global review. Geoderma 262, 101–111. doi:10.1016/j.geoderma.2015.08.009
Allan, E., Weisser, W., Weigelt, A., Roscher, C., Fischer, M., and Hillebrand, H. (2011). More diverse plant communities have higher functioning over time due to turnover in complementary dominant species. Proc. Natl. Acad. Sci. 108 (41), 17034–17039. doi:10.1073/pnas.1104015108
Allison, S. D., and Martiny, J. B. H. (2008). Resistance, resilience, and redundancy in microbial communities. Proc Natl Acad Sci U S A. 105 (Suppl. 1), 11512–11519. doi:10.1073/pnas.0801925105
Ampim, P. A. Y., Sloan, J. J., Cabrera, R. I., Harp, D. A., and Jaber, F. H. (2010). Green roof growing substrates: types, ingredients, composition and properties. J. Environ. Hortic. 28 (4), 244–252. doi:10.24266/0738-2898-28.4.244
Ashton, I. W., Miller, A. E., Bowman, W. D., and Suding, K. N. (2010). Niche complementarity due to plasticity in resource use: plant partitioning of chemical N forms. Ecology. 91 (11), 3252–3260. doi:10.1890/09-1849.1
Baer, S. G., Kitchen, D. J., Blair, J. M., and Rice, C. W. (2002). Changes in ecosystem structure and function along a chronosequence of restored grasslands. Ecol. Appl. 12 (6), 1688–1701. doi:10.1890/1051-0761(2002)012[1688:CIESAF]2.0.CO;2
Barrios, E. (2007). Soil biota, ecosystem services and land productivity. Ecol. Econ. 64 (2), 269–285. doi:10.1016/j.ecolecon.2007.03.004
Beninde, J., Veith, M., and Hochkirch, A. (2015). Biodiversity in cities needs space: a meta-analysis of factors determining intra-urban biodiversity variation. Ecol. Lett. 18 (6), 581–592. doi:10.1111/ele.12427
Blanusa, T., Vaz Monteiro, M. M., Fantozzi, F., Vysini, E., Li, Y., and Cameron, R. W. F. (2013). Alternatives to sedum on green roofs: can broad leaf perennial plants offer better ‘cooling service’?. Build. Environ. 59, 99–106. doi:10.1016/j.buildenv.2012.08.011
Boivin, M.-E. Y., Breure, A. M., Posthuma, L., and Rutgers, M. (2002). Determination of field effects of contaminants—significance of pollution-induced community tolerance. Hum. Ecol. Risk Assess. An Int. J. 8 (5), 1035–1055. doi:10.1080/1080-700291905800
Breure, A. M. (2004). “Soil biodiversity: measurements, indicators, threats and soil functions,” in International conference soil and compost eco-biology, León, Spain, September 15–17, 2004. Available at: http://www.fao.org/fileadmin/templates/soilbiodiversity/Downloadable_files/8.Breure.pdf, (accessed September 17, 2004).
Buzzard, V., Gil-Loaiza, J., Graf Grachet, N., Talkington, H., Youngerman, C., Tfaily, M. M., et al. (2021). Green infrastructure influences soil health: biological divergence one year after installation. Sci. Total Environ. 801, 149644. doi:10.1016/j.scitotenv.2021.149644
Byrne, L. B. (2007). Habitat structure: a fundamental concept and framework for urban soil ecology. Urban Ecosyst. 10 (3), 255–274. doi:10.1007/s11252-007-0027-6
Calvo, P., Nelson, L., and Kloepper, J. W. (2014). Agricultural uses of plant biostimulants. Plant Soil 383 (1–2), 3–41. doi:10.1007/s11104-014-2131-8
Cardinale, B. J., Duffy, J. E., Gonzalez, A., Hooper, D. U., Perrings, C., Venail, P., et al. (2012). Biodiversity loss and its impact on humanity. Nature 486 (7401), 59–67. doi:10.1038/nature11148
Cecchi, G., Di Piazza, S., Rosatto, S., Mariotti, M. G., Roccotiello, E., and Zotti, M. (2021). A mini-review on the Co-growth and interactions among microorganisms (fungi and bacteria) from rhizosphere of metal-hyperaccumulators. Front. Fungal Biol. 2, 787381. doi:10.3389/ffunb.2021.787381
Changnon, S. A. (2000). Reactions and respones to recent urban drought. Phys. Geogr. 21 (1), 1–20. doi:10.1080/02723646.2000.10642695
Clayton, J., Lemanski, K., and Bonkowski, M. (2021). Shifts in soil microbial stoichiometry and metabolic quotient provide evidence for a critical tipping point at 1% soil organic carbon in an agricultural post-mining chronosequence. Biol. Fertil. Soils 57 (3), 435–446. doi:10.1007/s00374-020-01532-2
Cohen-Shacham E., Walters G., Janzen C., and Maginnis S. (Editors) (2016). Nature-based solutions to address global societal challenges. Gland, Switzerland: IUCN International Union for Conservation of Nature.
Craul, P. J. (1985). A description of urban soils and their desired characteristics. Urban soils. 10, 330–339. doi:10.48044/jauf.1985.071
Delmont, T. O., Francioli, D., Jacquesson, S., Laoudi, S., Mathieu, A., Nesme, J., et al. (2014). Microbial community development and unseen diversity recovery in inoculated sterile soil. Biol. Fertil. Soils 50 (7), 1069–1076. doi:10.1007/s00374-014-0925-8
Dempsey, N., and Jenks, M. (2010). The future of the compact city. Built Environ. 36 (1), 116–121. doi:10.2148/benv.36.1.116
Dunnett, N., Nagase, A., Booth, R., and Grime, P. (2008). Influence of vegetation composition on runoff in two simulated green roof experiments. Urban Ecosyst. 11 (4), 385–398. doi:10.1007/s11252-008-0064-9
Dwivedi, V., and Soni, P. (2011). A review on the role of soil microbial biomass in eco-restoration of degraded ecosystem with special reference to mining areas. J. Appl. Nat. Sci. 3 (1), 151–158. doi:10.31018/jans.v3i1.173
Ehrenfeld, J. G., Ravit, B., and Elgersma, K. (2005). Feedback in the plant-soil-system. Annu. Rev. Environ. Resour. 30 (1), 75–115. doi:10.1146/annurev.energy.30.050504.144212
Eisenhauer, N. (2012). Aboveground–below-ground interactions as a source of complementarity effects in biodiversity experiments. Plant Soil 351 (1–2), 1–22. doi:10.1007/s11104-011-1027-0
Faeth, S. H., Bang, C., and Saari, S. (2011). Urban biodiversity: patterns and mechanisms. Ann. N.Y. Acad. Sci. 14, 69–81. doi:10.1111/j.1749-6632.2010.05925.x
Farrell, H. L., Léger, A., Breed, M. F., and Gornish, E. S. (2020). Restoration, soil organisms, and soil processes: emerging approaches. Restor. Ecol. 28 (S4), S307–S310. doi:10.1111/rec.13237
Ferguson, B., Lin, M.-H., and Gresshoff, P. M. (2013). Regulation of legume nodulation by acidic growth conditions. Plant Signal. and Behav. 8 (3), e23426. doi:10.4161/psb.23426
Filazzola, A., Shrestha, N., and MacIvor, J. S. (2019). The contribution of constructed green infrastructure to urban biodiversity: a synthesis and meta-analysis. J. Appl. Ecol. 56 (9), 2131–2143. doi:10.1111/1365-2664.13475
Fulthorpe, R., MacIvor, J. S., Jia, P., and Yasui, S.-L. E. (2018). The green roof microbiome: improving plant survival for ecosystem service delivery. Front. Ecol. Evol. 6, 5. doi:10.3389/fevo.2018.00005
Guilland, C., Maron, P. A., Damas, O., and Ranjard, L. (2018). Biodiversity of urban soils for sustainable cities. Environ. Chem. Lett. 16 (4), 1267–1282. doi:10.1007/s10311-018-0751-6
Hoch, J. M. K., Rhodes, M. E., Shek, K. L., Dinwiddie, D., Hiebert, T. C., Gill, A. S., et al. (2019). Soil microbial assemblages are linked to plant community composition and contribute to ecosystem services on urban green roofs. Front. Ecol. Evol. 7, 1–14. doi:10.3389/fevo.2019.00198
Jing, X., Sanders, N. J., Shi, Y., Chu, H., Classen, A. T., Zhao, K., et al. (2015). The links between ecosystem multifunctionality and above- and below-ground biodiversity are mediated by climate. Nat. Commun. 6 (1), 8159. doi:10.1038/ncomms9159
Kourtev, P. S., Ehrenfeld, J. G., and Ggblom, M. H. (2002). Exotic plant species alter the microbial community structure and function in the soil. Ecology 83 (11), 3152–3166. doi:10.1890/0012-9658(2002)083[3152:epsatm]2.0.co;2
Kranz, C. N., McLaughlin, R. A., Johnson, A., Miller, G., and Heitman, J. L. (2020). The effects of compost incorporation on soil physical properties in urban soils – a concise review. J. Environ. Manag. 261, 110209. doi:10.1016/j.jenvman.2020.110209
Kumar, K., and Hundal, L. S. (2016). Soil in the city: sustainably improving urban soils. J. Environ. Qual. 45 (1), 2–8. doi:10.2134/jeq2015.11.0589
Lange, M., Eisenhauer, N., Sierra, C. A., Bessler, H., Engels, C., Griffiths, R. I., et al. (2015). Plant diversity increases soil microbial activity and soil carbon storage. Nat. Commun. 6 (1), 6707. doi:10.1038/ncomms7707
Luna, L., Pastorelli, R., Bastida, F., Hernández, T., García, C., Miralles, I., et al. (2016). The combination of quarry restoration strategies in semiarid climate induces different responses in biochemical and microbiological soil properties. Appl. Soil Ecol. 107, 33–47. doi:10.1016/j.apsoil.2016.05.006
Lundholm, J. T., and Walker, E. A. (2018). Evaluating the habitat-template approach applied to green roofs. Urban Naturalist. 1 (1), 39–59.
Madre, F., Vergnes, A., Machon, N., and Clergeau, P. (2014). Green roofs as habitats for wild plant species in urban landscapes: first insights from a large-scale sampling. Landsc. Urban Plan. 122, 100–107. doi:10.1016/j.landurbplan.2013.11.012
Morel, J. L., Chenu, C., and Lorenz, K. (2015). Ecosystem services provided by soils of urban, industrial, traffic, mining, and military areas (Suitmas). J. Soils Sediments 15 (8), 1659–1666. doi:10.1007/s11368-014-0926-0
Müller, H., and Berg, G. (2008). Impact of formulation procedures on the effect of the biocontrol agent Serratia plymuthica HRO-C48 on Verticillium wilt in oilseed rape. BioControl 53 (6), 905–916. doi:10.1007/s10526-007-9111-3
O’Callaghan, M., Ballard, R. A., and Wright, D. (2022). Soil microbial inoculants for sustainable agriculture: limitations and opportunities. Soil Use Manag. 38 (3), 1340–1369. doi:10.1111/sum.12811
Ondoño, S., Bastida, F., and Moreno, J. L. (2014). Microbiological and biochemical properties of artificial substrates: a preliminary study of its application as technosols or as a basis in green roof systems. Ecol. Eng. 70, 189–199. doi:10.1016/j.ecoleng.2014.05.003
Pattnaik, S., Mohapatra, B., and Gupta, A. (2021). Plant growth-promoting microbe mediated uptake of essential nutrients (Fe, P, K) for crop stress management: microbe–soil–plant continuum. Front. Agron. 3, 689972. doi:10.3389/fagro.2021.689972
Perkins, L. B., and Hatfield, G. (2016). Can commercial soil microbial treatments remediate plant–soil feedbacks to improve restoration seedling performance?. Restor. Ecol. 24 (2), 194–201. doi:10.1111/rec.12302
Pihlap, E., Vuko, M., Lucas, M., Steffens, M., Schloter, M., Vetterlein, D., et al. (2019). Initial soil formation in an agriculturally reclaimed open-cast mining area—the role of management and loess parent material. Soil Tillage Res. 191, 224–237. doi:10.1016/j.still.2019.03.023
Pouyat, R. V., Szlavecz, K., Yesilonis, I. D., Groffman, P. M., and Schwarz, K. (2010). “Chemical, physical, and biological characteristics of urban soils,” in Agronomy monographs. Editors J. Aitkenhead-Peterson, and A. Volder (Madison, Wisconsin: American Society of Agronomy, Crop Science Society of America, Soil Science Society of America), 119–152.
Prommer, J., Walker, T. W. N., Wanek, W., Braun, J., Zezula, D., Hu, Y., et al. (2020). Increased microbial growth, biomass, and turnover drive soil organic carbon accumulation at higher plant diversity. Glob. Change Biol. 26 (2), 669–681. doi:10.1111/gcb.14777
Rooney, D. C., and Clipson, N. J. W. (2009). Phosphate addition and plant species alters microbial community structure in acidic upland grassland soil. Microb. Ecol. 57 (1), 4–13. doi:10.1007/s00248-008-9399-2
Saccá, M. L., Barra Caracciolo, A., Di Lenola, M., and Grenni, P. (2017). “Ecosystem services provided by soil microorganisms,” in Soil biological communities and ecosystem resilience. Editors M. Lukac, P. Grenni, and M. Gamboni (New York: Springer international publishing AG 2017), 9–24.
Schad, P. (2018). Technosols in the world reference base for soil resources – history and definitions. Soil Sci. Plant Nutr. 64 (2), 138–144. doi:10.1080/00380768.2018.1432973
Schmid, C. A. O., Reichel, R., Schröder, P., Brüggemann, N., and Schloter, M. (2020). 52 years of ecological restoration following a major disturbance by opencast lignite mining does not reassemble microbiome structures of the original arable soils. Sci. Total Environ. 745, 140955. doi:10.1016/j.scitotenv.2020.140955
Schulz, S., Brankatschk, R., Dümig, A., Kögel-Knabner, I., Schloter, M., and Zeyer, J. (2013). The role of microorganisms at different stages of ecosystem development for soil formation. Biogeosciences. 10 (6), 3983–3996. doi:10.5194/bg-10-3983-2013
Shayanthan, A., Ordoñez, P. A. C., and Oresnik, I. J. (2022). The role of synthetic microbial communities (SynCom) in sustainable agriculture. Front. Agron. 4, 896307. doi:10.3389/fagro.2022.896307
Singh, A., Yadav, V. K., Chundawat, R. S., Soltane, R., Awwad, N. S., Ibrahium, H. A., et al. (2023). Enhancing plant growth promoting rhizobacterial activities through consortium exposure: a review. Front. Bioeng. Biotechnol. 11, 1099999. doi:10.3389/fbioe.2023.1099999
Tsiknia, M., Tsikou, D., Papadopoulou, K. K., and Ehaliotis, C. (2021). Multi-species relationships in legume roots: from pairwise legume-symbiont interactions to the plant – microbiome – soil continuum. FEMS Microbiol. Ecol. 97 (2), fiaa222. doi:10.1093/femsec/fiaa222
Valliere, J. M., Wong, W. S., Nevill, P. G., Zhong, H., and Dixon, K. W. (2020). Preparing for the worst: utilizing stress-tolerant soil microbial communities to aid ecological restoration in the anthropocene. Ecol. Solutions Evid. 1 (2), e12027. doi:10.1002/2688-8319.12027
Vuko, M., Cania, B., Vogel, C., Kublik, S., Schloter, M., and Schulz, S. (2020). Shifts in reclamation management strategies shape the role of exopolysaccharide and lipopolysaccharide-producing bacteria during soil formation. Microb. Biotechnol. 13 (2), 584–598. doi:10.1111/1751-7915.13532
Waterhouse, B. R., Adair, K. L., Boyer, S., and Wratten, S. D. (2014). Advanced mine restoration protocols facilitate early recovery of soil microbial biomass, activity and functional diversity. Basic Appl. Ecol. 15 (7), 599–606. doi:10.1016/j.baae.2014.09.001
Weisser, W. W., Roscher, C., Meyer, S. T., Ebeling, A., Luo, G., Allan, E., et al. (2017). Biodiversity effects on ecosystem functioning in a 15-year grassland experiment: patterns, mechanisms, and open questions. Basic Appl. Ecol. 23, 1–73. doi:10.1016/j.baae.2017.06.002
Wong, W.-T., Tseng, C.-H., Hsu, S.-H., Lur, H.-S., Mo, C.-W., Huang, C.-N., et al. (2014). Promoting effects of a single Rhodopseudomonas palustris inoculant on plant growth by Brassica rapa chinensis under low fertilizer input. Microbes Environ. 29 (3), 303–313. doi:10.1264/jsme2.ME14056
Wubs, E. R. J., Van Der Putten, W. H., Bosch, M., and Bezemer, T. M. (2016). Soil inoculation steers restoration of terrestrial ecosystems. Nat. Plants 2 (8), 16107. doi:10.1038/nplants.2016.107
Keywords: Technosol, soil microbiome, soil quality, synthetic microbial communities, soil transplantation, soil plant (feedback loops)
Citation: Schröder A, Schloter M, Roccotiello E, Weisser WW and Schulz S (2024) Improving ecosystem services of urban soils – how to manage the microbiome of Technosols?. Front. Environ. Sci. 12:1460099. doi: 10.3389/fenvs.2024.1460099
Received: 05 July 2024; Accepted: 31 July 2024;
Published: 16 August 2024.
Edited by:
Vinicius Londe, University of Georgia, United StatesReviewed by:
Remigio Paradelo Núñez, University of Santiago de Compostela, SpainCopyright © 2024 Schröder, Schloter, Roccotiello, Weisser and Schulz. This is an open-access article distributed under the terms of the Creative Commons Attribution License (CC BY). The use, distribution or reproduction in other forums is permitted, provided the original author(s) and the copyright owner(s) are credited and that the original publication in this journal is cited, in accordance with accepted academic practice. No use, distribution or reproduction is permitted which does not comply with these terms.
*Correspondence: Stefanie Schulz, c3RlZmFuaWUuc2NodWx6QGhlbG1ob2x0ei1tdWVuY2hlbi5kZQ==