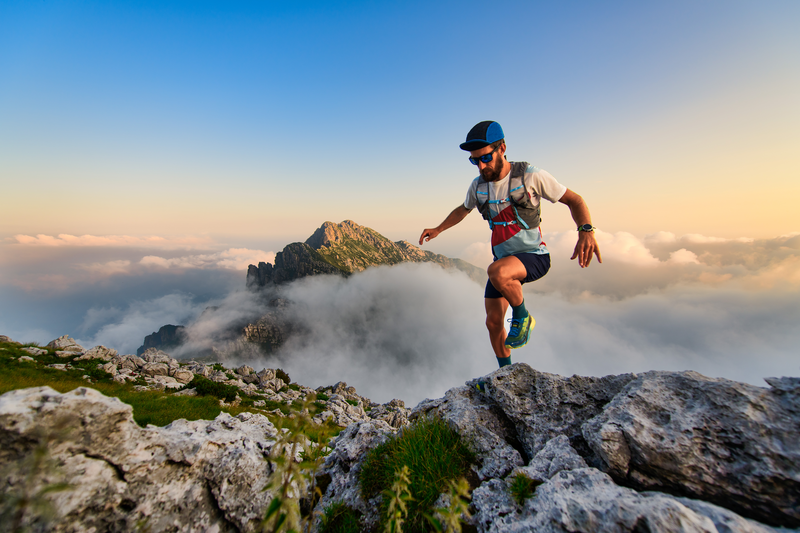
95% of researchers rate our articles as excellent or good
Learn more about the work of our research integrity team to safeguard the quality of each article we publish.
Find out more
ORIGINAL RESEARCH article
Front. Environ. Sci. , 17 September 2024
Sec. Toxicology, Pollution and the Environment
Volume 12 - 2024 | https://doi.org/10.3389/fenvs.2024.1457970
This article is part of the Research Topic Legacy & Emerging Contaminants in the Aquatic Environment View all 11 articles
Farm roadway runoff is a high-risk source of pollution when connectivity with waters occurs. Nutrients in this runoff are dominated by fresh animal deposits, but recent dairy and beef farm studies showed that available phosphorus (P) accumulates in roadway surface material and can be lost in runoff. A current knowledge gap is to examine available P concentrations in unsealed roadway and trackway (non-maintained) network of a lowland sheep farm. The present study focused on a 45 ha farm stocked with 544 sheep in south-east Ireland. Ten locations were sampled along with the adjacent fields for available P (i.e., Morgan’s P) and ancillary parameters (e.g., pH, total P and heavy metals) in December 2022. The first sampling location was on an aggregate roadway and the other nine were on trackways representing an older aggregate roadway network used by the flock but now covered with soil and grass. Results showed a distinct difference in surface material pH between roadway and trackway locations. Trackways had a pH that mimicked adjacent fields around the agronomic optimum for grassland of ∼6.2. All sampling locations had elevated available P concentrations, ranging from 26.3 to 111.0 mg L−1 (mean 62.8 mg L−1), similar to the spatial distribution for dairy farms but above those found at beef farms previously studied. The highest available P concentrations were found in roadway and trackway sections adjacent to the farmyard. Other elevated sampling areas were on trackways (87.3 or 97.7 mg P L−1) away from the farmyard where sheep are funnelled to pasture, stop to seek shade, urinate and defecate but do not graze. By contrast the average available P concentration for the surrounding fields was 8.4 mg L−1 with a range of 2.7–15.9 mg L−1. Two sampling areas combine to create a critical source area where a high available P source becomes visibly mobilised as runoff during rainfall, discharges into an open drainage ditch, which is then connected to a local stream. Breaking the pathway before runoff enters the open ditch could be a cheap and effective way of mitigating nutrient losses at these two locations.
Previous international research on roadway runoff and its impacts on connected waters has largely focused on planning, building, and operating roadway networks in urban and rural environments (Gillis et al., 2022). Other research on characterization and mitigation of forest road and trackways has been well developed (Wemple and Jones, 2003). It is known that farm roadways and tracks contribute sediments and particulates to waterways (Adams et al., 2014). Some recent research has focused on roadway runoff from internal farm roadways (called laneways in New Zealand (Monaghan and Smith, 2012); farm tracks Australia (Adams et al., 2014) or stock lanes in EU (Lucci et al., 2010) trafficked by machinery and livestock (Sifundza et al., 2024). In all cases, contaminants originating from roadway runoff can be deposited to surfaces and may be bioaccumulated directly or partitioned to sediments, or dissolve in surface waters (Gillis et al., 2022). In temperate regions, nutrient loads lost in farm roadway runoff can have significant negative impacts on water quality and this has led to regulation of soiled water runoff. For example, in Ireland soiled roadway runoff is strictly regulated since 2021 and is prohibited from entering waters (e.g., dry ditches and streams). Article 17.20 states “There shall be no direct runoff of soiled water from farm roadways to waters from 1 January 2021. The occupier of such a holding shall comply with the minimum specification for farm roadways.” (Current specification S199 July 2020).
Temporal and spatial concentrations of phosphorus (P) stored in farm roadway surface materials as a source distinct from fresh urine and faeces deposited on the roadways is a new area of research. It has been shown that this source of P can be lost throughout the year (i.e., in open and closed periods when animals are outside and housed, respectively). These materials act as storage reservoirs for P, which can become available in runoff during rainfall events with (i.e., soiled runoff) or without (i.e., non-soiled runoff) the presence of animal wastes (Fenton et al., 2022).
Recent work on dairy and beef farms in the south-east of Ireland, investigated the spatial and temporal distribution of available P in surface roadway material (Fenton et al., 2022; Sifundza et al., 2024). In Fenton et al. (2022), the range of available P in roadway surface materials (to 1 cm depth and excludes fresh excreta) on a dairy farm during a spatial survey conducted at the end of the open period (i.e., period where livestock actively used the roadway) was 10–110 mg L−1. The highest concentrations were found near farmyards, before underpasses and wherever animals slowed down due to roadway design and roadway features. In Sifundza et al. (2024), a temporal survey showed available P concentrations ranged from 15.9–101.4 mg L−1 (includes open and closed periods) which was higher than on two neighbouring beef farms (4.1–24.4 mg L−1). Temporal results showed that mean available P concentrations (5.4, 14.9, 13.4 mg L−1 for dairy, beef farm 1 and beef farm 2, respectively) increased in the open period (from February to September), whereas mean concentrations (40.3, 10.4, 9.8 mg L−1 for dairy, beef farm 1 and beef farm 2, respectively) declined over the closed period (October to January). On all farms examined, approximately 3 roadway locations were considered connected to surface waters forming a critical source area (CSA) (McDowell et al., 2024; Shore et al., 2014) and therefore needed mitigation intervention. Typically, the installation of low cost diversion bars to divert runoff into a vegetated buffer (>3 m) or swale, changing the slope of the roadway away from waters towards a buffer or swale and minimising the nutrient source through stock management (e.g., increasing the flow of animal traffic minimises deposits) were all noted as possible ways to break the pathway thereby minimising chemical and ecological impacts (Boger et al., 2018; Fenton et al., 2021).
Consideration of available P concentrations of roadways and trackways within different farms accessed by animals is important as fresh faeces and urine patches are deposited from large numbers of animals both spatially and temporally on these pervious unsealed surfaces. To date, research has focused on the loads of nutrients in runoff delivered to waters from roadways, while presenting the P source stemming only from fresh materials, e.g., McDowell et al. (2020).This approach limits losses to the open period and does not consider losses from the storage of P in the roadway itself, which can be lost during the closed period in addition to the open period. It is therefore important to consider the spatial distribution of available P in roadway and trackway materials across the major farming types where animals access roadways on a regular or ad hoc basis. An understanding of this available P store will help with the management of this source and mitigation of this source in both open and closed periods in the farming calendar. Such knowledge will also be important for consideration of the contribution of roadway runoff at catchment scale.
In previous studies (Fenton et al., 2022; Sifundza et al., 2024), it was hypothesised for temperate climates that the mean spatial and temporal available P roadway material concentration would be greatest during the open period when animals trafficked the roadway network and highest where animal movement was impeded (e.g., at bends, at entrance or exit to farmyard, before underpasses). In addition roadway condition and width have an influence on cow flow, e.g., farm roadways should be at least 3.5 m wide (for a 50 cow herd) with another 0.5 m allowed for each additional 50 cows (Maher et al., 2023). Also is was hypothesised that the mean available P concentration would be larger on a dairy farm than beef farms due to the amount of time animals traffic roadways due to milking operations (morning and evening). The temperate climate allows for high-quality pasture production over a long growing season and the efficient utilisation of pasture is critical for profitability, and farm roadways are essential for rotational grazing systems (Maher et al., 2023). Results from Sifundza et al. (2024) which compared dairy and beef farms, showed that the mean available P concentration on dairy farms was up to 4 times higher than that of beef farms. Overall, the P concentrations on both dairy and beef farms followed the same trend over the months, whereby the P concentration declined over the winter months, i.e., December and January and increased in the open period. The present study examines for the first time the spatial nature of available P on a lowland sheep farm roadway and trackway network. It is often the case in the south east of Ireland that dairy, beef and sheep farms are in close proximity to each other and could be within the same catchment area. There is a similar number of dairy and sheep farms in Co. Wexford where the present study site is located, e.g., 836 sheep farms versus 1048 dairy farms (Central Statistics Office, 2024; Teagasc, 2024). It is hypothesised as the sheep rarely use the roadway network but use the trackway system more frequently the available P concentration on the trackway system will be higher than that of the roadway. As the animal numbers can greatly exceed those of a dairy or beef system it is hypothesised that the mean and available P range spatially on the sheep farm in the closed period sampling event could be similar to a beef farm for the roadways and similar to a dairy farm for the trackways.
On sheep farms, internal roadways enable animals to access gazing areas but are less common and indeed less commonly used by animals when compared to other farming systems such as beef and dairy farms. After using the roadway network, sheep tend to stick to defined routes within fields to reach grazing areas where they spread out. However, original roadway networks on sheep farms can become covered by soil and grass over time, can slump and become degraded. Such trackways are still obvious linear features on the landscape and can be fenced off from the surrounding paddock or field. Farm roadways or trackways within these systems still can provide runoff connectivity with waters, thus, information pertaining to the pollutant concentrations needs to be collated. To date no study has investigated surface material available P concentrations on sheep farms along internal roadways or trackways. The present farm scale study examines available P concentrations in surface materials on a sheep farm to assess if a temporal survey is warranted. For that purpose, a sheep farm in south-east Ireland was selected, the roadway and trackway network was mapped and ten representative locations were sampled to 1 cm and tested for available P, pH and metals. In addition the adjacent fields were sampled as a comparison. Each sampling point was assessed with respect to its connectivity to surface waters (i.e., open drainage ditch or surface streams in the vicinity).
The 45 ha sheep farm used in the study is located in a lowland area in the south-east of Ireland, with average annual rainfall of 1,100 mm. The land slopes gently from the farm yard in one direction to a river bounding the farm. At the time of roadway sampling (December 2022) the number of sheep was as follows: breeding Ewes over 12 months of age (n = 370), breeding Rams over 12 months (n = 12), replacement Ewe lambs (n = 162). Average stocking density over the last number of years is 1.44 Livestock Units ha−1. Sheep graze outside for most of the year and are only housed briefly during winter months. Movement around the roadway and trackway network is to access different grazing areas. Sheep also use the roadway or trackways for resting, seek shade by bounding trees but tend not to graze these areas due to the high level of urination and defecation.
In Ireland, typically a farm internal roadway is constructed by first removing the topsoil layer (10–15 cm), before placing stone (shale, limestone or sandstone) aggregate (20–30 cm layer) as the main construction material, overlain by a surface dust or blinding layer (25 mm layer of 0–4 mm in size). Each individual layer is rolled with a heavy vibrating roller to keep it in place. The crossfall on the roadway ensures drainage from the surface and should be either a continuous crossfall of 1:25 or from center to both sides (Teagasc, 2021). Over time as animals and machinery use the roadway, soil from the fields may become incorporated into the dust layer. If not maintained or replaced these roadways become relics of the original network and turn into trackways, which become grassed over. On the farm, only one roadway fits the above description and runs with slope through location 1, 2, 3, 4, and 6 (Figure 1). All other roadways are orientated across slope and can be described as trackways. These trackways, (e.g., location 5, Figure 1) appear sunken from their original positions, are waterlogged and act as overland flow interceptors, which causes sediment to build up on the old roadway surfaces. Some old field boundaries were removed over time to make larger fields. Sample locations are presented in Figure 1 with a detailed description of each sampling location together with a photograph in Table 1.
Figure 1. Sheep farm internal roadway and trackway system with location of shed/yard, sampling locations (1–10), field numbers, position of open drain and stream with critical source area (CSA) delivery point marked.
Table 1. General descriptions of the farm roadway sampling locations on the Sheep Farm with representative images.
At each location a duplicate roadway or trackway material sample of 200 g was taken from the top 1 cm, bagged and transported to the laboratory for analysis. Fresh animal deposits were avoided during the sampling process. Each field was sampled using a zig-zag sampling pattern covering the entire area as outlined in Teagasc (2020). All samples (i.e., roadway, trackway and field samples) were oven dried at 40°C, sieved through 2 mm sieve to remove debris and stored at room temperature before analysis. To determine available P, soil samples (3 g) were shaken for 30 min at room temperature with 15 mL Morgan’s solution (sodium acetate and acetic acid and buffered at pH 4.8) at a ratio of 1:5 and then filtered (Morgan, 1941). The filtrates were then analysed for P concentration on the Lachat system using the principle of flow injection analysis. Two-gram (2 g) samples were also shaken on a reciprocating shaker for 5 min and the supernatant was filtered (0.45 μm) and tested for aluminium (Al), iron (Fe), calcium (Ca), boron (B), cobalt (Co), copper (Cu), manganese (Mn), and zinc (Zn) using a soil solution ratio of 1:10 in Mehlich 3 reagent (0.2 M CH3COOH + 0.25MNH4NO3 + 0.015 M NH4F + 0.13 M HNO3 + 0.001 M EDTA). Analyses was conducted on an Agilent 5100 ICP-OES.
The pH across the roadway and trackway locations is at or near the agronomic target for grassland of 6.2 (Table 2). Only the pH at the first roadway location (Figure 1) beside the farm yard and associated housing shed is elevated (i.e., pH 7.54 ± 0.09). These are similar for farm roadways as found by Sifundza et al. (2024) on a dairy and two beef farms in the south-east of Ireland (7.56 ± 0.30, 7.89 ± 0.43 and 7.71 ± 0.49, respectively). The mean pH at locations 2–10 (pH 6.30 ± 0.28) are similar to fields found within the current farm (6.04 ± 0.26).
Available P (Morgan’s P) for roadways and trackways ranged from 26.3 to 111.0 mg L−1 (Table 2; Figure 2 presents available P mean and standard deviation per location). The highest P concentrations were at location 1 (adjacent to the farmyard) and two locations 400 and 500 m away from the farmyard, respectively, i.e., location 7 (connected to open drainage ditch) and 10 (receives run-on from up-gradient areas) (See Table 1 for specific details). Another elevated area is at location 3 where sheep are slowed as they channel through a narrow fence gap. In contrast the average available P concentration for the surrounding fields was 8.4 mg L−1 (Index 4) with a range 2.7–15.9 mg L−1.
Figure 2. Mean available phosphorus (P) concentrations and standard deviation (stdev) for each sampling location.
The pH values found were similar to fields with mineral soils found on the farms studied by Sifundza et al. (2024) i.e., dairy, beef farm 1 and beef farm 2 with 6.2 ± 0.28, 6.3 ± 0.35, and 6.4 ± 0.27, respectively. These results indicate that over time the older roadway network and trackways have become subsumed (as the landowner suggests) into the field network on the farm. The soil test results for the field samples are similar to locations 2–10. Metal concentrations for roadway and trackway locations are within similar ranges for dairy and beef farms studied in the south-east of Ireland and are indicative of an aggregate and soil mix. However, mean field soil metal concentrations are all lower than adjacent roadway and trackways, except for Fe.
The spatial concentration range of available P was similar to that of a dairy farm roadway network (i.e. 15.9 ± 13.0 to 101.4 ± 61.1) but considerably higher than surface materials on roadways within the two beef farms (e.g., 6.31 ± 1.7 to 23.2 ± 9.6 mg L−1) as reported by Sifundza et al. (2024). The lowest values here represent highest values on the beef farms. Source factors will be determined by the concentration of animals on the roadway as influenced by stocking rate, roadway density and animal type. For example, Marsden et al. (2020) document that sheep urinate 8–11 times a day (mean urine event volume recorded was 289 ± 14 mL) with an estimated daily urine production value of 2.77 ± 0.15 L urine sheep−1 d−1. By way of comparison, Ravera et al. (2015) document that cows (wintering system in New Zealand) urinate 8–12 times a day with 2.37 L per event indicating an estimated daily urine production value of between 18–30 L urine cow−1 d−1. The concentration of P in faeces and urine for cows and sheep is largely a product of the diet. It can vary across studies, e.g., McDowell (2006) tested cattle and sheep dung and found 5.5 and 8.0 g TP kg−1 dry matter, respectively. Scott et al. (1972) examined the P content of faeces and urine in sheep fed with different diets and found 5.6 ± 0.3 g d−1 in faeces and 0.3 ± 0.2 g d−1 in urine (fed with roughage), which can increase if concentrates are introduced. In cows, dung P concentration ranges between 4 and 8 g P kg−1 (McDowell, 2006; McDowell et al., 2020; Vadas et al., 2015) and dairy cows defecate 10.5 times cow−1 d−1, with only a fraction of this likely to be on roadways (Oudshoorn et al., 2008). Vebriyanti et al. (2022) showed that with varied diets the P concentration in cow urine ranged from 130.94 mg L−1 ± 4.11–156.67 mg L−1 ± 2.49. Using the numbers above for sheep urine the concentration of P could be as high as 108 mg L−1 (0.3 g P d−1/2.77 L d−1). The number of sheep (Ewe, Rams and Lambs) on this farm at time of sampling was 544. This number coupled with the concentration of P and volumes in urine and faeces documented above gives an indication of daily loads deposited on the farm.
As the sheep are outside nearly all year (i.e., some housing in Winter months to protect fields from treading damage) and have access to all the roadway and trackway networks when grazing different parts of the farm, the concentrations found during the testing period herein (i.e., December) may be conservative and warrants a temporal study. For example, in the dairy and beef unit farm study of Sifundza et al. (2024) the mean available P concentration for December was 32.4 mg L−1 (min: 6.5 and max: 67.7), and 10.8 mg L−1 (min: 3.1 and max: 37.1), respectively. This month compares to the mean available P concentration for May was 64.5 mg L−1 (min: 14.2 and max: 149.5) and 15.3 (min: 3.1 and max: 71.2), respectively. In reality for the dairy farm all locations except one were higher in available P concentration in May than in December. For the beef farm the vast majority of locations were higher in May with some staying the same. It could be hypothesized that sheep farm locations will also follow seasonal trends as with dairy and beef farms.
It appears that P losses in runoff (both surface and subsurface) from pasture-based livestock (cattle and sheep) farming areas will generally not exceed 2 kg TP ha−1 year−1 (Hooda et al., 2000). The problem here is that such losses do not have a negative economic consequence to the landowner (equate to < 5% of nutrients applied) but can have large impacts on water quality. To put these numbers into perspective, phosphate impacts on water quality even at very low concentrations. The Environmental Quality Standard (EQS) for good status water bodies is 0.035 mg P L−1 and 0.025 mg P L−1 for high status water bodies. Therefore, as little as 1 kg P when present as phosphate, lost in roadway runoff will pollute approximately 29 million litres of water (NFGWS, 2020). As the wetting and drying cycle impacts the P concentration in sediments and soil and their subsequent release (Smith et al., 2023) a temporal survey of the road and trackway network is advised. This would match the approach of Fenton et al. (2022) whereby a spatial survey established the range of available P concentrations on a dairy roadway network and a follow-up study by Sifundza et al. (2024) established both the spatial and temporal trends of available P on the same roadway network.
Future work should consider other farm and animal types (e.g., pigs) that have access to roadways or trackways and examine the spatial and temporal nature of available P on those networks. In addition the influence of soil type may be important, e.g., mineral soils versus high organic soils. This could be a large difference for upland sheep farms where grazing occurs in upland areas on peat soils with little or no capacity to hold onto P. In addition an examination of the sampling and laboratory techniques would be beneficial. For example, the sampling depth of 1 cm may capture the highest concentrations of available P but other stores may be present below this 1 cm layer. At present an agronomic test is being used to examine available P in a mixed material (aggregate and soil) and there could be other appropriate tests to examine P storage and loss dynamics in these materials. For example, Ezzati et al. (2020) examined source-sink P dynamics of sediment along an agricultural ditch network using equilibrium P concentrations (EPC0) and developed sorption isotherms to examine the binding strength in these materials.
One area (combines locations 6–7) on the farm was identified as a CSA (Table 2). These CSAs are small areas of the farm and suitable for targeted mitigation (Doody et al., 2012). The delivery point to the open ditch is presented in Figure 1 and identified in the image of location 6 in Table 1 (yellow dot). The first contributing area is at location 7 where a high source in the surface materials could be mobilised during rainfall towards a connected open drainage ditch. Another area that contributes to the CSA is location 6, where connectivity exists with the open ditch and the available P source in the roadway materials is 41 mg L−1.
In terms of environmental management across dairy versus beef versus sheep farms, as the regulation pertaining to roadway runoff on farms in Ireland covers all farm types, the onus is on the landowner to identify where runoff enters waters and to come up with a mitigation solution to “break the pathway” the soiled roadway runoff delivery point and waters. The number of connectivity points on a dairy farm seems to be consistent with approx. 10% of roadway sections in connectivity with waters (Maher et al., 2023; Rice et al., 2022). A similar number of connectivity points was also found by Sifundza et al. (2024) on both dairy and beef farms studied. So although the source concentration may be different (combination of available P from fresh and roadway materials) within a CSA across dairy, beef and sheep farms, environmental management through mitigation will still be needed. The CSA concept refers to small areas of fields, farms or catchments. The best application of CSAs to target mitigation actions should be at such small areas but only where they account for most contaminant loss (McDowell et al., 2024). With respect to roadway and trackway runoff the contribution to overall P losses at farm and catchment scales has not been established and this needs further investigation and indeed consideration in future regulation and policy. At present all CSAs within the roadway and trackway network regardless of their contribution to overall catchment losses require environmental management through source minimization and mitigation.
Possible mitigation solutions for the present farm would be to prevent runoff from these areas entering the open ditch (considered water under current regulations in Ireland) by breaking the pathway. A suggestion would be installation of a diversion bar or to change the camber of the trackway towards the field or to bund the open drainage ditch. Options and costs of these mitigation measures are detailed in Fenton et al. (2021). It is important to note that waterlogged soils have the potential to release available P to a greater extent than the surrounding soils. This is the case along flow pathways, e.g., roadway or trackway runoff pathways which are constantly wetting and drying. The work of Smith et al. (2023) indicate that high soil profile saturation, low nitrate (NO3−), and increased temperature induce P release, potentially via reductive dissolution. Without the buffering influence of NO3− in a soil profile, anaerobic conditions caused by inundation could cause a soil profile to become Fe- and Mn-reducing and release associated P.Therefore, the period of greatest risk is likely to be when saturation (i.e., anaerobia and reduction) overlaps with increasing temperatures. This established winter to spring as risky periods for P loss, due to the combination of: existing moisture; warmer temperatures; minimal rainfall quantities required to saturate the soil profile; and, NO3− is likely to be depleted via leaching from the soil. This again highlights why it is important to understand where saturation and wetting and drying cycles occurs on a given farm. For instance: in typically poorly-drained soils; in low-lying areas where flow from upslope would accumulate (e.g., locations 5 and 10); or on soils or roadways over poorly-permeable layers.
Roadways and trackways used by sheep exhibited high concentrations of available P across the ten locations sampled and exhibited a different signature to that of field soil. Concentrations were above previously studied beef farms but on a par with dairy farms studied. One location was considered critical source areas (combination of locations 6–7), where a source from a soiled roadway runoff entered a dry open ditch connecting directly with a nearby stream. Mobilisation of roadway and trackway runoff to these delivery points occurs during rainfall events. These locations are in need of mitigation, e.g., installation of a diversion bar or establishment of a buffer area to break the pathway and block nutrients and sediment entering waters. As sheep have access to the entire roadway and trackway system throughout the year (apart for a brief winter housing period) the temporal distribution of available P on sheep farm roadways and trackways should be investigated and compared in lowland and upland areas.
The original contributions presented in the study are included in the article/supplementary material, further inquiries can be directed to the corresponding author.
OF: Visualization, Validation, Supervision, Software, Resources, Project administration, Writing–review and editing, Writing–original draft, Methodology, Investigation, Funding acquisition, Formal Analysis, Data curation, Conceptualization. SL: Writing–review and editing, Visualization, Investigation, Formal Analysis, Data curation. KD: Funding acquisition, Writing–review and editing, Resources, Formal Analysis. PT: Funding acquisition, Writing–review and editing, Methodology, Formal Analysis. JC: Investigation, Data curation, Writing–review and editing. LS: Writing–review and editing. JM: Writing–review and editing, Visualization, Methodology, Investigation, Formal Analysis.
The author(s) declare that financial support was received for the research, authorship, and/or publication of this article. The funding for the present project was under the Teagasc core-funded ROAD-READY project.
Special thanks goes to farm and laboratory staff members for their time, advice and patience and to those who helped with training and testing of samples.
The authors declare that the research was conducted in the absence of any commercial or financial relationships that could be construed as a potential conflict of interest.
All claims expressed in this article are solely those of the authors and do not necessarily represent those of their affiliated organizations, or those of the publisher, the editors and the reviewers. Any product that may be evaluated in this article, or claim that may be made by its manufacturer, is not guaranteed or endorsed by the publisher.
Adams, R., Arafat, Y., Eate, V., Grace, M. R., Saffarpour, Sh., Weatherley, A. J., et al. (2014). A catchment study of sources and sinks of nutrients and sediments in south-east Australia. J. Hydrology 515, 166–179. doi:10.1016/j.jhydrol.2014.04.034
Boger, A. R., Ahiablame, L., Mosase, E., and Beck, D. (2018). Effectiveness of roadside vegetated filter strips and swales at treating roadway runoff: a tutorial review. Environ. Sci. Water Res. & Technol. 4 (4), 478–486. doi:10.1039/c7ew00230k
Central Statistics Office (2024). Census of agriculture 2020. Available at: https://www.cso.ie/en/releasesandpublications/ep/p-coa/censusofagriculture2020-preliminaryresults/livestock/.
Doody, D. G., Archbold, M., Foy, R. H., and Flynn, R. (2012). Approaches to the implementation of the Water Framework Directive: targeting mitigation measures at critical source areas of diffuse phosphorus in Irish catchments. J. Environ. Manage 93 (1), 225–234. doi:10.1016/j.jenvman.2011.09.002
Ezzati, G., Fenton, O., Healy, M. G., Christianson, L., Feyereisen, G. W., and Thornton, S. (2020). Impact of P inputs on source-sink P dynamics of sediment along an agricultural ditch network. J. Environmen. Manage. 257, 109988.
Fenton, O., Rice, P., Murnane, J., Tuohy, P., and Daly, K. (2022). Dairy farm roadway surface materials as a P-source within the nutrient transfer continuum framework. Front. Environ. Sci. 10, 878166. doi:10.3389/fenvs.2022.878166
Fenton, O., Tuohy, P., Daly, K., Moloney, T., Rice, P., and Murnane, J. G. (2021). A review of on-farm roadway runoff characterisation and potential management options for Ireland. Water Air Soil Pollut. 232, 89. doi:10.1007/s11270-021-05027-0
Gillis, P. L., Parrott, J. L., and Helm, P. (2022). Environmental fate and effects of road run-off. Arch. Environ. Contam. Toxicol. 82 (2), 159–161. doi:10.1007/s00244-021-00906-3
Hooda, P. S., Edwards, A. C., Anderson, H. A., and Miller, A. (2000). A review of water quality concerns in livestock farming areas. Sci. Total Environ. 250, 143–167. doi:10.1016/s0048-9697(00)00373-9
Lucci, G. M., McDowell, R. W., and Condron, L. M. (2010). Potential phosphorus and sediment loads from sources within a dairy farmed catchment. Soil Use Manag. 26, 44–52. doi:10.1111/j.1475-2743.2009.00247.x
Maher, P., Egan, M., Murphy, M. D., and Tuohy, P. (2023). Assessment of the current performance of grazing infrastructure across Irish dairy farms. J. Dairy Sci. 106, 4759–4772. doi:10.3168/jds.2022-22799
Marsden, K. A., Lush, L., Holmberg, J. A., Whelan, M. J., King, A. J., Wilson, R. P., et al. (2020). Sheep urination frequency, volume, N excretion and chemical composition: implications for subsequent agricultural N losses. Agric. Ecosyst. Environ. 302 (2020), 107073. doi:10.1016/j.agee.2020.107073
McDowell, R. W. (2006). Contaminant losses in overland flow from cattle, deer and sheep dung. Water, Air, Soil Pollut. 174, 211–222. doi:10.1007/s11270-006-9098-x
McDowell, R. W., Daly, K., and Fenton, O. (2020). Mitigation of phosphorus, sediment and Escherichia coli losses in runoff from a dairy farm roadway. Ir. J. Agric. Food Res. 59. doi:10.15212/ijafr-2020-0117
McDowell, R. W., Kleinman, P. J. A., Haygarth, P., McGrath, J. M., Smith, D., Heathwaite, L., et al. (2024). A review of the development and implementation of the critical source area concept: a reflection of Andrew Sharpley's role in improving water quality. J. Environ. Qual. doi:10.1002/jeq2.20551
Monaghan, R. M., and Smith, L. C. (2012). Contaminant losses in overland flow from dairy farm laneways in southern New Zealand. Agric. Ecosyst. Environ. 159, 170–175. doi:10.1016/j.agee.2012.07.022
Morgan, M. F. (1941). Chemical soil diagnosis by the Universal soil testing system. Connecticut, USA: Connecticut Agricultural Experimental Station. Bulletin 450.
NFGWS (2020). A handbook for source protection and mitigation actions for farming. Dublin, Ireland : Published by the National Federation of Group Water Schemes. Available at: www.nfgws.ie.
Oudshoorn, F. W., Kristensen, T., and Nadimi, E. S. (2008). Dairy cow defecation and urination frequency and spatial distribution in relation to time-limited grazing. Livest. Sci. 113, 62–73. doi:10.1016/j.livsci.2007.02.021
Rice, P., Daly, K., Tuohy, P., Murnane, J. G., Nag, R., and Fenton, O. (2022). Evaluating connectivity risk of farm roadway runoff with waters-Development and sensitivity analysis of a semi quantitative risk model. Scie. Total Environ. 851, 158114.
Ravera, B. L., Bryant, R. H., Cameron, K. C., Di, H. J., Edwards, G. R., and Smith, N. (2015). Use of a urine meter to detect variation in urination behaviour of dairy cows on winter crops. Proc. N. Z. Soc. Animal Prod. 75, 84–88.
Scott, D. (1972). Excretion of phosphorus and acid in the urine of sheep and calves fed either roughage or concentrate diets. Quarter. J. Experimen. Physiol. 57,, 379–392.
Shore, M., Jordan, P., Mellander, P.-E., Kelly-Quinn, M., Wall, D. P., Murphy, P. N. C., et al. (2014). Evaluating the critical source area concept of phosphorus loss from soils to water-bodies in agricultural catchments. Sci. Total Environ. 490, 405–415. doi:10.1016/j.scitotenv.2014.04.122
Sifundza, L. S., Murnane, J., Daly, K., Lopez-Sangil, L., Leach, S., Tuohy, P., et al. (2024). Phosphorus in farm roadway substrates: contrasting spatial and temporal patterns in dairy and beef farms. Front. Environ. Sci. 12, 1405378. doi:10.3389/fenvs.2024.1405378
Smith, G. J., McDowell, R. W., Daly, K., hUallacháin, D. Ó., Condron, L. M., and Owen, F. (2023). Factors controlling shallow subsurface dissolved reactive phosphorus concentration and loss kinetics from poorly drained saturated grassland soils. J. Environ. Qual. 52, 355–366. doi:10.1002/jeq2.20442
Teagasc (2020). in Major & micro nutrient advice for productive agricultural crops Editors D. P. Wall, and M. Plunkett
Teagasc (2021). Building a farm roadway. Available at: https://www.teagasc.ie/news--events/daily/dairy/building-a-farm-roadway.php (Accessed.
Teagasc (2024). National farm survey small farms report 2022. Available at: https://www.teagasc.ie/publications/2024/national-farm-survey-small-farms-report-2022.php (Accessed May, 2024).
Vadas, P. A., Busch, D. L., Powell, J. M., and Brink, G. E. (2015). Monitoring runoff from cattle-grazed pastures for a phosphorus loss quantification tool. Agric. Ecosyst. & Environ. 199, 124–131. doi:10.1016/j.agee.2014.08.026
Vebriyanti, E., Arief, I. I., and Dewi, S. P. (2022). Utilization of cow urine waste for the manufacture of urine as a form of environmentally friendly dairy farming business. IOP Conf. Ser. Earth Environ. Sci. 950, 012028.
Keywords: agriculture, nutrient transfer continuum, critical source area, water quality, soil
Citation: Fenton O, Daly K, Tuohy P, Cardiff J, Leach S, Sifundza LS and Murnane J (2024) Spatial distribution of available phosphorus in surface road and trackway surface materials on a sheep farm in Ireland. Front. Environ. Sci. 12:1457970. doi: 10.3389/fenvs.2024.1457970
Received: 01 July 2024; Accepted: 03 September 2024;
Published: 17 September 2024.
Edited by:
Sanjeeb Mohapatra, Delft University of Technology, NetherlandsReviewed by:
Nitish Venkateswarlu Mogili, National Institute of Technology, IndiaCopyright © 2024 Fenton, Daly, Tuohy, Cardiff, Leach, Sifundza and Murnane. This is an open-access article distributed under the terms of the Creative Commons Attribution License (CC BY). The use, distribution or reproduction in other forums is permitted, provided the original author(s) and the copyright owner(s) are credited and that the original publication in this journal is cited, in accordance with accepted academic practice. No use, distribution or reproduction is permitted which does not comply with these terms.
*Correspondence: Owen Fenton, b3dlbi5mZW50b25AdGVhZ2FzYy5pZQ==
Disclaimer: All claims expressed in this article are solely those of the authors and do not necessarily represent those of their affiliated organizations, or those of the publisher, the editors and the reviewers. Any product that may be evaluated in this article or claim that may be made by its manufacturer is not guaranteed or endorsed by the publisher.
Research integrity at Frontiers
Learn more about the work of our research integrity team to safeguard the quality of each article we publish.