- 1Fredericton Research and Development Centre, Agriculture and Agri-Food Canada, Fredericton, NB, Canada
- 2Department of Plant, Food, and Environmental Sciences, Faculty of Agriculture, Dalhousie University, Truro, NS, Canada
- 3Envirem Organics Inc., Fredericton, NB, Canada
As organic waste is converted to usable amendments via composting, there are large CO2 emissions associated with the decomposition of organic matter via microorganisms. While the active composting phase produces the largest emissions over a short duration, compost can often be stored during and after the maturation phase for much longer periods of time, increasing cumulative emissions. As such, the objectives of this study were to examine the spatial and temporal variability associated with in situ emissions sampling while identifying the environmental and chemical controls on emissions in windrow composting facilities during and after the maturation phase. A total of 665 flux measurements were taken from four windrows representing different ages and compositions between June and November 2020. Factorial analysis of covariance (ANOVA) was used to determine the variability between sampling locations, while multiple linear regression was used to identify those parameters which had the most influence on CO2 flux. Emissions showed significant variability over time that were attributed to ambient temperatures. During the summer, each windrow reached peak emissions between 5.0 and 32.3 g CO2 m-2 hr-1. As temperatures cooled, the windrows saw a 62%–86% decline in emissions, generally falling below 2 g CO2 m-2 hr-1. Significant differences occurred between the top-most sampling location and all others on the windrow, emitting between 33%–100% more CO2. The environmental controls of surface temperature, moisture content, and internal temperature showed the highest influence on emissions (R2 = 0.62). Chemical properties including organic nitrogen, carbon, pH, magnesium, and nitrate also showed significant influence (R2 = 0.43). This research has shown that environmental factors including temperature and moisture show the strongest influence over emission rates in mature compost. A significant negative effect of organic nitrogen on CO2 flux was found, indicating that increased presence of organic nitrogen would aid in the retention of carbon after the maturation phase, acting to lower total emissions.
1 Introduction
Globally, waste generation and waste diversion strategies have increased in developed nations since the early 2000s (Cao et al., 2023). In Canada, for instance, organic and paper fibre waste accounted for two-thirds of all diverted waste in 2020 (Statistics Canada, 2022). As populations continue to grow and countries continue to develop, it is expected that waste generation will increase and create a greater need for diversion strategies (Kaza et al., 2018). Composting is an effective diversion method to convert organic waste into by-products that predominately take the form of organic soil amendments and biofertilizers (Gallardo-Lara and Nogales, 1987; NiChualain and Prasad, 2009; Ozores-Hampton et al., 2022). The demand for more intensive agricultural outputs, as well as uses of organic soil amendments in remediation efforts, is further driving a demand for more composted products (Bennett et al., 2012; Scotti et al., 2015).
The composting process is carried out via the degradation and transformation of organic matter by microorganisms. There are four phases of aerobic composting characterized by variations in temperature and microbial activity (Vergara and Silver, 2019). During the first mesophilic phase, temperatures range from ambient temperatures to 45°C as microorganisms begin to degrade readily available carbon. The thermophilic phase begins when temperatures exceed 45°C, driven by the rapidly increasing microbial activity and biomass. The second mesophilic stage is marked by significant reductions in CO2 emissions as microbial activity reduces in response to an exhaustion of readily available carbon and nitrogen sources. Finally, the maturation phase is the longest phase in many composting processes as the organic matter stabilizes.
During each phase, the organic materials are continuously used by microorganisms within the compost as an energy source. In aerobic conditions, facilitated by regular aeration of the compost, carbon within the organic waste is oxidized to CO2 (Martín et al., 2015). The oxidization via microbial respiration generates heat, hence the interconnectedness of the compost phases, CO2 generation, and microbial activity. Cellulose and lignocellulose are the predominant compounds in mature compost, which facilitate a microbial community of slow growing, mesophilic bacteria and fungi (Danon et al., 2007; Zahra et al., 2023). At this stage, available carbon will limit the microbial activity (López-González et al., 2015), however the microbial community will continue to change in response to reduced supply (Danon et al., 2008), therefore it should not be assumed that respiration is stable.
Significant work has been carried out to characterize the emissions of compost, however much of this research is conducted during the active composting phase and does not extend to the storage of mature, stable compost (e.g., Bonifacio, Rotz, and Richard, 2016; Williams et al., 2019). For instance, Swati and Hait (2018) reviewed 16 publications studying GHG emissions from compost, only two of which measured a single source of compost for 12 months or greater durations. Andersen, et al. (2010a) measured small scale home compost emissions over a near 2-year period finding that while CO2 emissions declined after the active phase, peaks occurred in response to increasing ambient temperatures and, unexpectedly, near the 450-day mark. Amlinger, Peyr, and Cuhls (2008) monitored emissions over a 64-week period from small-scale composting with weekly additions of fresh household organic waste. Measured emissions showed that CO2 and NH3 remained heightened throughout the 64-weeks, which was attributed to the regular additions of fresh waste, however there was no comparison to compost without continuous additions. Andersen, et al. (2010b) measured windrowed garden waste emissions over a near 2-year period and saw continual increases in CO2 within the pore space reaching a peak near the 350-day mark. While many studies of compost emissions treat the maturation phase as relatively stable, these findings indicate that there may be more variability than normally anticipated.
Large-scale composting can be accomplished using several methods including static piles, forced aeration which use fans and piping to deliver air to the inside of a windrow or pile, or in-vessel composting where feedstocks are contained within buildings or containers which are regularly turned or agitated. A popular form of large-scale composting is the use of manually turned windrows. Continual piles are created which can range from a few to over a hundred meters in length placed in a triangular or elongated dome shape (Zhu-Barker et al., 2017). This is a preferred method for large compost producers due to the minimal requirement of manual intervention, efficient use of space, and inexpensive nature. Challenges of windrow composting could include a limited ability to control and monitor the composting process, and the requirement for deliberate moisture management to avoid anaerobic conditions (Michel et al., 2022). Once established, the windrows are then left to compost, being periodically turned for aeration during the active composting phases. During and after the maturation phase, windrows may be left unaltered for long periods of time in a form of storage. Due to the volume of material, these windrows can remain stored for much longer than smaller-scale composting applications.
A knowledge gap exists in the changes occurring during the maturation phase and subsequent storage of industrial-scale windrows, in-situ, and their impact on CO2 emissions. The influence of physical and chemical properties on CO2 flux during this storage period also presents a knowledge gap—particularly on the scale at which industrial producers compost. Composted material can remain at the facility after the active and maturation phases for long durations, therefore an oversight of these impacts will lead to an underestimation of the actual climate impacts. Site-specific emissions calculations are preferred for compost producers, rather than using literature-based estimates, to account for influences beyond just the feedstock type (Lou and Nair, 2009; Nordahl et al., 2023); therefore, producers must understand both the controls and the methodologies to quantify emissions.
The goal of this research was to quantify the CO2 flux produced following the maturation and subsequent storage of compost windrows. Total CO2 emissions were calculated over an annual growing period, representing the number of annual frost-free days, to determine the impact of long-term storage frequently practiced at commercial composting facilities. Differences in CO2 emissions attributed to composts of differing feedstocks were examined to reveal the impact of compost origin material. Our hypothesis is that compost feedstocks will continue to influence the CO2 flux of the selected windrows due to remnant carbon and nitrogen levels, while age will not significantly affect emissions as the selected windrows are mature and the carbon should be stable. To address the need for site-specific emission measurements, this research examined the impact of spatiotemporal variability on windrow sampling positions. A secondary objective was to identify the drivers of CO2 flux during the storage period. Physical and chemical parameters were identified independently to better understand the continued effect on emissions in stabilized material. Comparisons between windrows of differing ages with similar feedstocks indicate whether these parameters change during the storage period.
2 Methods
2.1 Study area
The study was conducted at an industrial compost facility near Clarendon, New Brunswick, Canada (45.4688630N, 66.4773800W). The site has been operated by Envirem Organics Inc. since 2004, who specialize in converting organic waste, biological waste, and organic municipal solid waste products into merchantable topsoil, potting soils, organic soil amendments, and biofuels. Envirem Organics Inc. is one of the largest compost producers in Canada with an average annual operating capacity of approximately 100,000 tonnes of composted product at the studied site. The facility is located in the Southern New Brunswick Uplands Ecoregion with monthly average temperatures from 4.0°C to 20.1°C and mean annual precipitation between 9.0 mm and 58.5 mm per month over the study period (Table 1). Compost windrows were formed and stored outside, exposed to the changing seasons. The windrows were arranged parallel to one another on a sloped surface to aid in drainage.
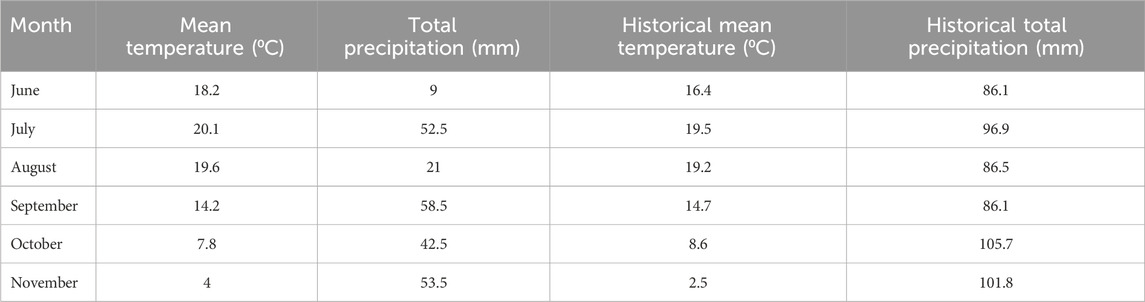
Table 1. Monthly mean temperatures and total precipitation for the study period and historical averages calculated from 1981–2010 (Environment and Climate Change Canada, 2020).
2.2 Sampling methodology
A total of 665 CO2 flux measurements were taken between June 2020 and November 2020 on a weekly schedule across four compost windrows. Each windrow was approximately 3 m tall, therefore five measurement locations were selected on the surface of the windrows following the shape of the pile. Measurement locations were selected at an approximate height of 1 m on each side from ground level, 2 m on each side from ground level, and one on top (Figure 1). The five points are labelled throughout according to location and orientation: east bottom (EB), east mid (EM), top (T), west mid (WM), and west bottom (WB). The five measurements were replicated three times on each windrow for a total of 15 samples per windrow, per day of sampling. Each replicate was separated by a minimum of 2 m. Similar gas sampling methodologies have been used for actively composting windrows by applying static gas chambers to the surface by Sánchez-Monedero et al. (2010), Sommer et al. (2004), and Zhu-Barker et al. (2017). The specific locations were determined by the height and width of the windrows with the top sampling points being critical due to the larger expected emissions channelled through the top of compost windrows. Physical composite samples were collected for each replication at a 30 cm depth for chemical analyses.
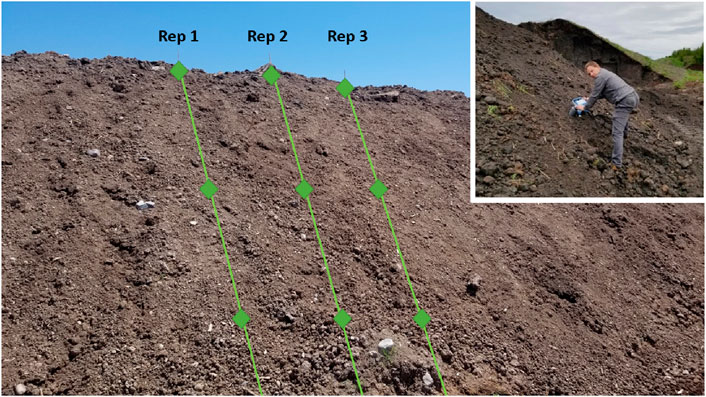
Figure 1. Each replicate is presented as a straight line from the base to the top of the windrow, with sampling points occurring at the green diamonds. The lower two sampling points in each replication were repeated on the other side of the windrow. Markers can be seen at the top of the windrow to ensure consistency throughout the study period. The inset image shows the EGM-5 Portable CO2 Gas Analyzer and SRC-2 Soil Respiration Chamber in use.
Of the four windrows sampled, two were comprised of municipal solid waste (MSW) and two were a mixed industrial waste (IW) composition. The MSW windrows contained household compostable waste, including both kitchen and garden waste material from residential areas, collected by local waste management commissions. The IW windrows were directly managed by Envirem Organics Inc. to produce a homogenous composition, using approximately 70% pulp and paper refuse by weight, with the other 30% being primarily made up of waste from aquaculture operations and fish processing facilities. The IW windrows included small amounts of sawdust and cardboard waste (<5%) incorporated opportunistically as they were received.
The windrows were also selected to represent various ages of compost. For each composition (i.e., MSW and IW), one windrow was composted for 12 months, while the other was composted for 18 months. Envirem Organics Inc. had identified the windrows as stable and mature using a combination of in-field tests including colour, odour, and temperature measurements, and laboratory analyses. Chemical parameters collected during the course of the research, such as C:N and pH, fell within acceptable ranges to indicate stability and maturity, therefore all windrows in the study are considered mature. Normally, the windrows would be regularly turned using a front-end loader for aeration; however, they were not altered during the course of the study. Each windrow was positioned in a similar orientation to best avoid possible biases arising from extended and uneven sun exposure, forced aeration via strong prevailing winds, or other environmental concerns exterior to the windrows.
Gas measurements were conducted using an EGM-5 Portable CO2 Gas Analyzer with an SRC-2 Soil Respiration Chamber and a soil moisture and temperature sensor (PP Systems Inc). Linear respiration rate (g m-2 hr-1), air temperature (⁰C), surface soil temperature (⁰C), and volumetric soil moisture content were recorded on the EGM-5 Portable CO2 Gas Analyzer. The EGM-5 Portable CO2 Gas Analyzer and SRC-2 Soil Respiration Chamber have a 99% accuracy when used within the ranges of CO2 measurements recorded during the research. To ensure accuracy was consistent throughout the research, a zeroing function was performed prior to each measurement by passing the gas sample through a CO2 scrubbing desiccant and measuring the concentration (i.e. 0). The EGM-5 Portable CO2 Gas Analyzer can then ensure that internal equipment deviations and drifts can be corrected without manual intervention. Additionally, a temperature probe was used to measure subsurface soil temperature at a 90 cm depth, a suggested depth for best practices when monitoring internal windrow temperatures (The Compost Council of Canada, 2016).
2.3 Respiration measurement and calculation
Respiration measurements were made in a portable, closed chamber system, which measured the rate of increase of CO2 concentration within the chamber over a 60 s period (Parkinson, 1981). The measurements were made between 9:00 a.m. and 11:00 a.m. to reduce the influence of diurnal temperature changes (Parkin and Kasper, 2003). Once the SRC-2 was applied to the soil surface, the soil respiration rates (
where
Using the five CO2 flux values along the surface of the windrow, site specific estimates of total CO2 flux were calculated. Total hourly and total annual estimates of CO2 emissions per volume of soil were calculated over a 166-day period to reflect the growing degree days in New Brunswick. Flux values were standardized to a cubic metre of compost material to facilitate scalability and applications to windrows of differing sizes.
2.4 Chemical analysis
Concentrations of organic carbon and nitrogen values were generated as percentages of mass using the dry combustion method on an Elementar analyzer (Vario MAX Cube, elemental combustion analyzer, Hanau, Germany). Compost pH was measured using a 1:1 ratio of compost to water (Hendershot, Lalande, and Duquette, 2007). Phosphate, potassium, calcium, magnesium, and aluminum were extracted using the Mehlich 3 extraction method (Mehlich, 1984). Nitrate and ammonia were measured using flow injection analysis colorimetry (Lachat Instruments, 2003; 2008).
2.5 Statistical analysis
Factorial three-way ANOVA was used to compare the mean CO2 flux values between windrow composition and age, sampling positions, and replicates. By identifying significant differences related to windrow composition and age it can be determined whether CO2 flux is variable over the duration of storage (12 and 18 months since windrow establishment) and whether it varies between compositions of the same age. Comparison of sampling positions determines whether CO2 emissions experience the chimney effect seen in windrow composting, whereby heat causes air to rise from the center of the pile while drawing cool air into the pile from the sides via convective heat transfer, concentrating emissions at the top of the windrow (Andersen, et al., 2010b; Stegenta et al., 2019). Finally, a comparison of replicates is required to ensure that the collected data were representative of thoroughly mixed, homogenous windrows.
The assumptions of normality and homogeneity of variance were confirmed with the (Shapiro and Wilko, 1965) and Levene’s test (Levene, 1960), respectively. Pearson’s correlation coefficients were calculated to determine the relationship between each independent variable and the linear respiration rate of the windrows. Then, a stepwise, multiple linear regression model was used to identify the combinations of variables which best explained the observed variability in linear respiration rates. The stepwise approach retained all variables which showed a significant effect, as measured by a p-value of <0.05. Both linear regression and Pearson’s correlation have been successfully applied to explore emissions in active composting (Williams et al., 2019), and in determining the relationship between compost age and CO2 evolution (Hutchinson and Griffin, 2008).
Variable reduction was then accomplished using variance inflation factor (VIF) analysis to address multicollinearity amongst the independent variables. Failure to remove correlated independent variables for use in regression can lead to difficulties in interpretation and inaccurate coefficient values (James et al., 2021). The VIF analysis operates by performing ordinary least squares (OLS) regression, whereby one independent variable is fitted to all other independent variables and R2 is calculated, as shown in Equation 2. Here, the VIF is calculated for each variable and the variable with the highest value is removed. The process of calculating the VIF value and subsequent removal of variables with the highest value is repeated until all variables have VIF values that are lower than a selected threshold (VIF <4). The VIF analysis was performed using the car package in R (Fox and Weisberg, 2019). All statistical analysis was completed using the R programming language.
where R2 is the coefficient of determination for independent variable,
3 Results
3.1 Characterizing the compost windrows
3.1.1 Temporal variability
Mean CO2 flux values over the entire study period were 11.4 g CO2 m-2 hr-1 for IW 12 months, 9.9 g CO2 m-2 hr-1 for IW 18 months, 2.1 g CO2 m-2 hr-1 for MSW 12 months, and 2.45 g CO2 m-2 hr-1 for MSW 18 months, however measurements varied considerably between the windrows over time (Figure 2). As expected, measurements showed a general trend of seasonal responses to ambient air temperatures, peaking during the summer, and reducing as daily temperatures cooled. Each windrow showed the highest CO2 flux during July and August with daily peaks of 32.3 g CO2 m-2 hr-1 for IW 12 months, 16.8 g CO2 m-2 hr-1 for IW 18 months, 5.3 g CO2 m-2 hr-1 for MSW 12 months, and 5.0 g CO2 m-2 hr-1 for MSW 18 months. As ambient temperatures dropped below 20°C near the surface of the windrow, emissions from all windrows dropped, generally falling below 2 g CO2 m-2 hr-1. During October, IW windrows showed an 86% decline in CO2 flux values compared to those collected before October, while MSW windrows showed a 62% decline during the same period.
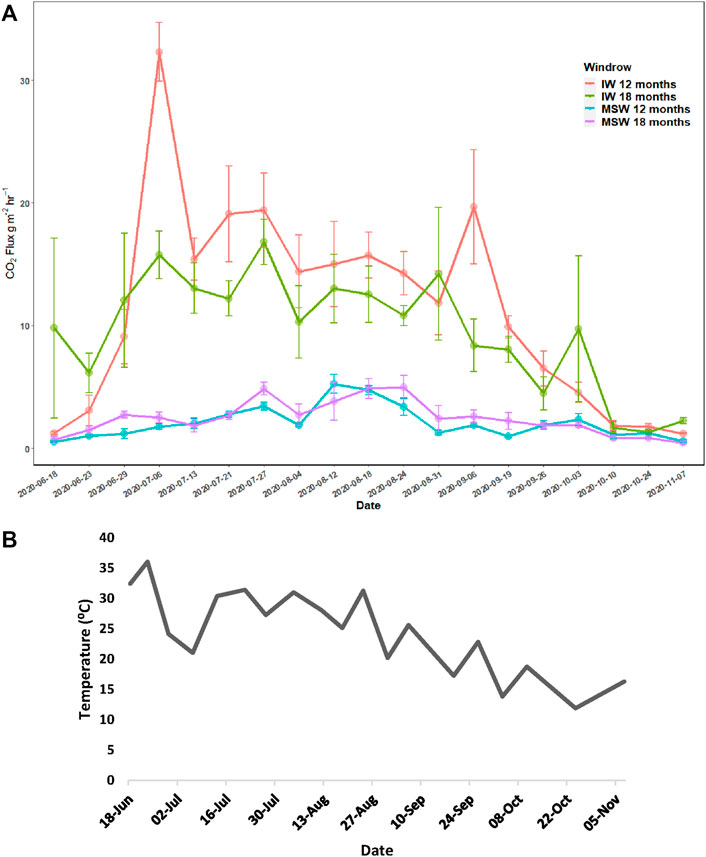
Figure 2. (A) Mean daily CO₂ measured over the duration of the study, separated by each windrow. Error bars represent the standard error of CO₂ flux measurements. MSW, municipal solid waste; IW, mixed industrial waste. (B) Mean daily air temperature (°C) measured near the surface of the windrows during each emissions measurement.
3.1.2 Spatial variability
Significant differences in CO2 flux occurred at different sampling points on the windrows (Figure 3). Using a factorial ANOVA to compare all values collected at each sampling point, a statistically significant difference in mean CO2 flux by sampling position (p = 0.003) and compost composition (p < 0.001) was found. Tukey’s honestly significant difference (HSD) results revealed that the top sampling point of the windrows emitted significantly more CO2 when compared to all other sampling positions, confirming the chimney effect. In both the MSW and IW windrows aged 12-months, the top sampling point emitted 33%–50% more than the other sampling positions. In each windrow aged 18-months, the top sampling point emitted 100% more than other sampling positions on the same windrow. The remaining four sampling positions did not show a significant difference amongst each other. Windrows compromised of IW showed significantly higher CO2 flux compared to windrows of MSW—emitting four to six times more CO2 throughout the study period. Significant differences did not occur between the different ages of the windrows or between sampling replicates on the same windrows.
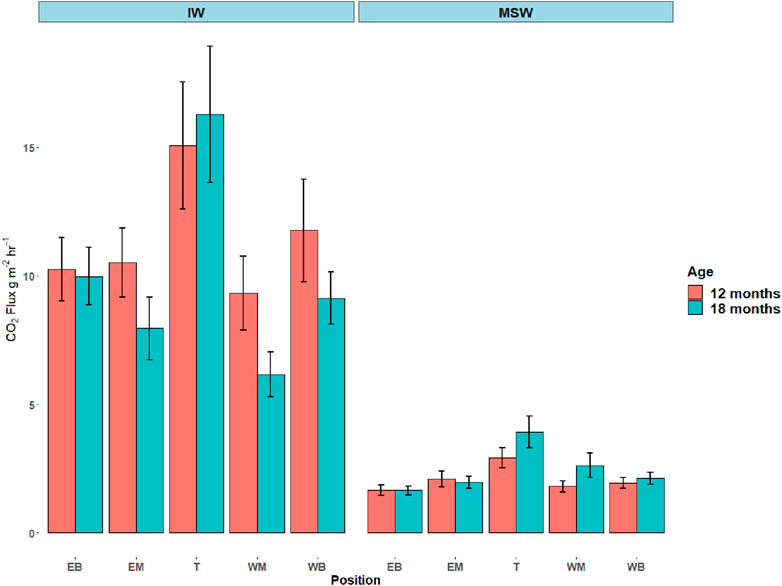
Figure 3. Mean CO2 flux from each sampling position on the windrow averaged over the entire study length. The top sampling position of each windrow had significantly higher emissions, while industrial waste compost emitted significantly higher over the entire windrow. No significant difference was observed due to windrow age. Error bars represent the standard error of CO₂ flux measurements. MSW, municipal solid waste; IW, mixed industrial waste; EB, east bottom; EM, east mid; T, top; WM, west mid; WB, west bottom.
3.2 Chemical properties influencing respiration
Laboratory analyses of compost properties were conducted on composite samples representing each replicate; therefore, the mean field measured data over the replicate was used to explore respiration interactions (Table 2). Pearson’s correlation values were calculated for all variables to identify the strength and directionality of relationships between CO2 flux and the individual measured variables (Figure 4). Internal windrow temperature shows the strongest relationship with CO2 flux (r = 0.67). Three additional variables showed moderate negative relationships with CO2 flux: Nitrogen (r = −0.56), C:N ratio (r = 0.56), and phosphate (r = −0.55). Four variables showed weak relationships with CO2 flux: Potassium (r = −0.45), surface soil temperature (r = 0.39), aluminum (r = 0.35), and carbon (r = 0.33).
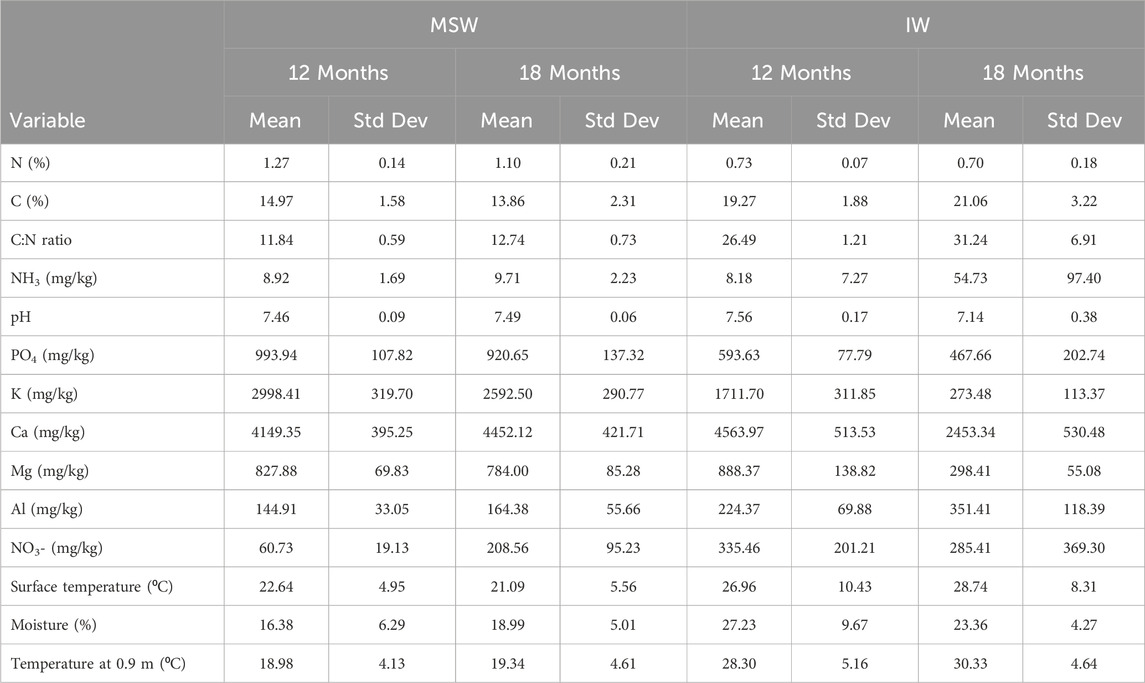
Table 2. Mean and standard deviation of all values used in regression analysis separated by composition and age (n = 127).
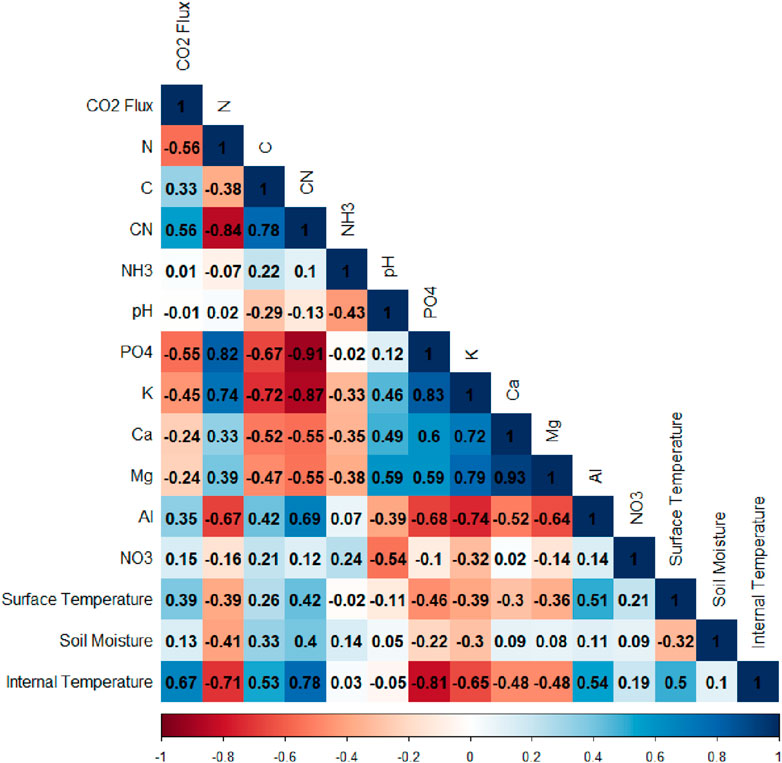
Figure 4. Correlation matrix of all variables used in the regression analysis of CO2 flux. Internal temperature of the windrow was the most influential variable in the generation of CO2. Numbers within the matrix are the calculated Pearson r values of the two intersecting variables.
Multiple linear regression models were generated to determine the combination of variables that most explained the observed variability in CO2 flux. C:N ratio was removed prior to the regression analysis to avoid redundancy as it is a calculated value of total carbon and total nitrogen. Using all other variables, significant relationships were found between surface soil temperature ⁰C (p < 0.001), soil moisture content (p = 0.005), and soil temperature at 0.9 m ⁰C (p < 0.001). The resulting full model is shown in Equation 3, with standard errors of the coefficients shown in square parenthesis.
where
Further multiple linear regression models were generated to investigate the relationships between CO₂ flux values and the chemical properties of the compost by removing surface soil temperature ⁰C, soil moisture content, and soil temperature at 0.9 m ⁰C as predictors. Significant relationships were found between nitrogen (p < 0.001), carbon (p = 0.049), pH (p < 0.001), magnesium (p < 0.001), nitrate (p < 0.001), and potassium (p = 0.08). Potassium was found to be highly correlated with the other selected variables, with a VIF value of 17.4, therefore it was removed from the regression equation. The resulting full model is shown in Equation 4, with standard errors of the coefficients shown in square parenthesis.
where
3.3 Total site emissions
Total hourly and total annual estimates of CO2 emissions per volume of compost were calculated for site-specific emissions estimates (Table 3). Annual emissions were calculated over 166 days to capture the growing degree days in New Brunswick. Annual estimates showed a greater climate impact associated with storing IW compost as emissions were 74%–83% higher than the MSW compost. As indicated by the change from 12 months to 18 months, it is unlikely values will remain static over the entire year. Instead, dynamic estimates should be made by producers as the emissions change over time.

Table 3. Hourly and annual estimates of CO2 flux from the four windrows standardized to a cubic metre of compost material. Annual flux values were calculated over 166-days to capture the growing degree days in New Brunswick, Canada.
4 Discussion
4.1 Compost windrows
Comparing the sampling points spatially over the windrow revealed direct patterns associated with windrow shape. The top (T) sampling point of each windrow emitted significantly more CO2 than the other positions, while the four sampling positions along the sides of the windrow showed no statistical difference (Figure 3). This was expected due to the chimney effect acting to concentrate airflow towards the centre of the pile and away from the bottom and sides (Poulsen, 2010; Stegenta et al., 2019). The spatial variances within the windrows were assessed by the comparison between replicates. The lack of significant variation in the replicates indicates that the compost was a thoroughly mixed, homogeneous pile.
No significant difference was found between the age of the windrows in CO2 flux, indicating that both ages were at a similar stage of stability regarding carbon. This was expected due to the age of the windrows, being formed 12 and 18 months prior to the measurements being taken. Therefore, total emissions generated following the maturation phase can most easily be reduced by ensuring compost producers utilize the product once the material reaches stability. Excessive storage time may lead to additional climate impacts beyond the necessary carbon turnover during the composting process.
The IW windrows showed significantly higher CO2 flux, surface temperature, temperature at 0.9 m, moisture, carbon, C:N ratio, and nitrate. The MSW windrows were significantly higher in organic nitrogen, pH, and magnesium. One study comparing MSW and pulp compost from olive milling, similar to forestry and pulp refuse, also found that MSW had lower comparative carbon, organic nitrogen, and C:N ratio values (Komilis and Tziouvaras, 2009). The C:N ratio of pulp and paper mill compost can have a wide range relative to composting method and other feedstocks. With a mean C:N ratio of 29, the IW windrow in this study were lower than comparable composts using pulp and paper mill waste (Turner, Wheeler, and Oliver, 2022), while still indicating stability. This was likely due to the addition of other wastes, such as N-rich inputs derived from fish processing. In comparisons of MSW and pulp and paper composts, MSW has been considered more nutrient rich while pulp and paper is considered more carbon rich (Atkinson, Jones, and Gauthier, 1996; Quintern, 2014), which supports the findings of this study when comparing the two compositions in chemical analysis. Compost created from MSW provides an opportunity for a high quality product with a lessened climate impact compared to those created from pulp and paper refuse.
4.2 Factors contributing to CO2 flux
As expected, temperature and moisture were found to be the main controls of CO2 flux. Internal windrow temperature, measured at 0.9 m below the surface, appeared to be the most effective variable at explaining the observed CO2 flux. This may have been due to the heat generated as part of the microbial activity—an explanation that has been well established previously (e.g., Bernal et al., 2017; Chang, Chen, and Yang, 2009; Hellmann et al., 1997), as well as the use of temperature in assessing compost stability as a reflection of microbial activity (Jiménez and Garcia, 1989; Mahapatra, Ali, and Samal, 2022). Our results indicated that even when the compost was stable, CO2 flux was predominately influenced by internal windrow temperatures.
Moisture and surface temperature have also been shown to influence total respiration in previous research (Lei and Han, 2020; Raich and Tufekcioglu, 2000). Moisture has been identified as a possible source of microbial limitation in compost after the thermophilic phase, with approximately 50% moisture content being preferred for active composting (Chroni et al., 2009; Hemidat et al., 2018), reducing to 15%–30% moisture in mature compost relative to the time spent drying (Kong et al., 2023; Temel, Yolcu, and Turan, 2023). In this study, moisture and CO2 flux show a positive relationship even at the relatively low values observed, supporting previous findings. Brempong, Norton, and Norton (2019) found that moisture had a greater impact on soil CO2 flux during dry months in the summer, which may have also been captured in this research as the year in which this study was conducted was much drier than average (Table 1). Given the age of the windrows, precipitation events would be the only continuing source of moisture content. The difference in moisture between the windrows is likely related to the drainage of the site with IW windrows being placed closer together not allowing for regular overland drainage. The reduced moisture in the MSW windrows may be limiting microbial activity and leading to lowered CO2 flux over the study period.
The surface temperature of the windrows is influenced primarily by ambient air temperatures and solar radiation. As such, the close relationship between surface temperature and CO2 flux show the positive influence of temperature on microbial respiration. In a study of soils amended with MSW compost González-Ubierna, de la Cruz, and Casermeiro (2015) found air temperature to be the strongest influencer of soil respiration and that the respiration showed clear seasonal shifts. Within composting practices, studies of seasonality are few due to the short duration of active compost, however, Andersen, et al. (2010b) found an unexpected increase in CO2 emissions after 450 days of household waste composting which may be linked to an increase in ambient air temperature recorded around the same time. While this is not unexpected, the findings show that the seasonal timing of compost storage will influence subsequent emissions by increasing them during warmer months. It may also suggest that indoor compositing facilities may be better equipped to reduce emissions by removing the impact of solar radiation, however further comparison is required.
The final chemical properties of composts are a function of the original inputs (Hameed et al., 2022) and the stability (Grey and Henry, 1999). Nutrient values are under flux during the entire process due to mineralization, leaching, and the addition of inputs during the early stages of composting. As composts age, the impact of total carbon on CO2 flux is expected to reduce as available carbon is mineralized and oxidized (Benito et al., 2005; Bernal et al., 1998; Ekinci et al., 2021) and available nitrogen is reduced (Paré et al., 1998). Therefore, it would be expected that C:N ratio would play a more significant role in CO2 emissions rather than the availability of carbon alone as composts continue to age in storage. Eiland et al. (2001) found that compost with high initial C:N ratios of 47, 50, and 54 lead to higher respiration after a 12-month period compared to compost with lower initial C:N ratios of 11 and 35, which may be attributed to available nitrogen limitations. In a review of monitored compost parameters, Azim et al. (2018) noted that C:N ratios greater than 35 require several lifecycles of microorganisms to oxidize the available carbon, controlled by nitrogen availability. This research has similarly shown a significant negative effect of organic nitrogen on CO2 flux, indicating that increased organic nitrogen would aid in the retention of carbon after the maturation phase, lowering the total emissions from the windrows.
The pH of the compost has been used as an indicator of compost stability when measured values are consistent over time (Jain, Daga, and Kalamdhad, 2019; Tognetti, Mazzarino, and Laos, 2007), and has shown significant influence over soil biology (Duddigan et al., 2021). During the composting process, pH, microbial activity, and CO2 flux reach maximum values during the thermophilic phase and begin to gradually decline as the compost cures (Ge et al., 2022; Levanon and Pluda, 2002). Here, pH was shown to have a weak negative relationship with CO2 flux. The inclusion of sawdust in the IW windrows may play a role in this relationship as sawdust has a high carbon content contributing to increased respiration over long periods (N’Dayegamiye and Isfan, 1991), and has been found to lead to lower pH values in finished compost products (Yousefi, Younesi, and Ghasempoury, 2013). Compost pH in this study was measured using a North American standard 1:1 ratio (Hendershot, Lalande, and Duquette, 2007; U.S. EPA, 2004), while other acceptable standards indicate a 2:1 ratio (e.g., van Reeuwijk, 2002), and studies have used 2.5:1, 5:1 or 10:1 (e.g., Duddigan et al., 2021; Ge et al., 2022; Yousefi, Younesi, and Ghasempoury, 2013). Further research should be undertaken into the comparability of these methods with specific regards to compost.
Magnesium and nitrate appeared to have a weak influence on respiration. Nair and Ngouajio (2012) and Yang et al. (2021) found that magnesium had a positive relationship with total microbial biomass which would impact total respiration. Nitrification in compost predominately occurs during the maturation phase after available carbon levels and temperatures begin to lower below 40°C (Cáceres, Malińska, and Marfà, 2018). The ratio of nitrate to ammonium has been indicated as a possible maturity index (Meng et al., 2017; Sciubba et al., 2015) as nitrates will increase in mature compost. Previous studies have shown that reductions in available carbon allow nitrifying bacteria to outcompete the heterotrophic bacteria responsible for the active composting process (Cáceres, Flotats, and Marfà, 2006; Gao et al., 2010). Respiration measured during this research may be attributable to the presence of nitrifying bacteria, however further research regarding the microbial community composition would be required to determine the origin.
Ammonia, phosphate, calcium, and aluminum did not appear to have significant influence on compost respiration during this period. The appearance of nitrate as a significant influence may be the reason for the exclusion of ammonia. As ammonia is converted to nitrate during the maturation phase, it may become an increasingly predominant indicator of microbial activity. Phosphate values were near 1,000 ppm for the MSW compost and nearly half that value for both IW windrows. Potassium was found to be much higher in the MSW with significantly reduced values in the 18-month IW windrow. Previous studies have shown that MSW compost is a viable source of potassium in cropping applications due to the retention during the composting process (Bhattacharyya et al., 2007). Jamroz et al. (2020) found that potassium in MSW compost steadily declined to 3, 130 mg kg-1 after 279 days of composting as soluble forms were leached. It is likely that soluble forms of potassium continue to leach from the windrows in this study leading to further reductions. Calcium values for both MSW and the 12-month IW windrows were similar, however the 18-month IW windrow showed significantly reduced calcium content. Aluminum was higher in the IW windrows, however the impact on respiration was not significant.
The C:N ratio, while not used in the regression analysis, did appear to have the second highest correlation values amongst all variables with CO2 flux, sharing the same value as total organic nitrogen. C:N ratios have been proposed in previous research as indicators of maturity and stability (Basak, 2018), therefore a stabilization of CO2 generation. Our research indicates similarly that C:N ratio, total carbon, and total nitrogen each contribute to the dynamics of CO2 flux. C:N ratios were much higher for the IW windrows due to the inclusion of pulp and paper mill waste in these windrows, while the MSW had higher organic nitrogen values lowering the C:N ratio.
The results of both the correlation analysis and multiple linear regression models indicate that environmental factors (moisture and temperature) had the strongest influence on CO2 flux, each contributing positively to the total carbon emissions observed. While internal temperatures are likely to be a result of slow decomposition of original feedstocks, surface temperature and moisture may be influenced by solar radiation and drainage that are not unique to the compost type. This did not support our original hypothesis that remnant carbon and nitrogen from the original feedstock would be influential in longer-term emissions. However, when removing environmental controls, linear models could still achieve a moderate relationship relying only on compost chemical properties. Further research in environmentally controlled compost facilities may show the properties most critical in original feedstocks to avoid prolonged elevated levels of CO2 emissions.
4.3 Total site emissions
Total CO2 flux values were calculated per cubic meter of cured compost to facilitate application by producers to windrows of varying sizes. Comparison within the literature is challenging due to a lack of standardized units and a general focus on shorter duration composting. Our findings generally agree with those of Andersen, et al. (2010a) with values of organic household waste observed to decrease to below 2–3 g CO2 hr-1 during maturation with few peaks above 5 g CO2 hr-1. Further comparison to soils amended with compost show that while the MSW declined to near the same rate as amended soils, the emissions of the IW windrows remain comparatively high. For example, Andrés et al. (2012) measured CO2 dynamics in a soil amended with varying applications of treated biosolids finding a peak of 41.89 kg CO2 ha-1 day-1, roughly equating to 1.7 g CO2 m-2 hr-1. Shahzad et al. (2019) utilized a wood chip amendment finding that respiration peaked at 0.83 g CO2 m-2 hr-1 at the initial day of mixture. CO2 flux of cured MSW compost and amended soils appear much lower than that of the IW windrows, therefore further research is needed to corroborate the sustained heightened emissions from IW waste. Additionally, to fully understand the total climate impacts of compost storage further research on N2O and CH4 dynamics are required.
5 Conclusion
In situ comparison between windrows of mixed IW and MSW representing two different ages showed that composition and original feedstocks were the primary driver of CO2 emissions during extended storage at a commercial compost facility. In this research, IW windrows stood to emit 3.9–5.8 times more CO2 over a growing season than windrows of MSW. To accurately calculate cumulative emissions, these extended storage times must be accounted for. The results of this study showed that CO2 flux in windrow composting was highly influenced by environmental factors such as surface temperature, internal temperature, and moisture. These parameters, however, are often outside of the control of the producer or are difficult to manage during the composting process. Of the measured chemical parameters, organic nitrogen, carbon, pH, magnesium, and nitrate were the primary controls on CO2 flux. Efforts to control for these parameters using original feedstocks may then have an effect on lowering total emissions during storage at a large-scale composting facility.
Data availability statement
The original contributions presented in the study are included in the article/supplementary material, further inquiries can be directed to the corresponding author.
Author contributions
TP: Formal Analysis, Writing–original draft. L-PC: Conceptualization, Data curation, Funding acquisition, Methodology, Resources, Supervision, Writing–review and editing. KM: Writing–review and editing. SH: Writing–review and editing. BH: Writing–review and editing. BK: Writing–review and editing.
Funding
The authors declare that financial support was received for the research, authorship, and/or publication of this article. Funding for this study was provided by the project PSS3235 of Agriculture and Agri-Food Canada.
Acknowledgments
The authors are thankful to Tim Rice and the Envirem Organics Inc. crew for assisting and facilitating field work throughout the duration of the study.
Conflict of interest
Author BK was employed by Envirem Organics Inc.
The remaining authors declare that the research was conducted in the absence of any commercial or financial relationships that could be construed as a potential conflict of interest.
Publisher’s note
All claims expressed in this article are solely those of the authors and do not necessarily represent those of their affiliated organizations, or those of the publisher, the editors and the reviewers. Any product that may be evaluated in this article, or claim that may be made by its manufacturer, is not guaranteed or endorsed by the publisher.
References
Amlinger, F., Peyr, S., and Cuhls, C. (2008). Green house gas emissions from composting and mechanical biological treatment. Waste Manag. and Res. J. a Sustain. Circular Econ. 26 (1), 47–60. doi:10.1177/0734242X07088432
Andersen, J. K., Boldrin, A., Christensen, T. H., and Scheutz, C. (2010b). Greenhouse gas emissions from home composting of organic household waste. Waste Manag. 30 (12), 2475–2482. doi:10.1016/j.wasman.2010.07.004
Andersen, J. K., Boldrin, A., Samuelsson, J., Christensen, T. H., and Scheutz, C. (2010a). Quantification of greenhouse gas emissions from windrow composting of garden waste. J. Environ. Qual. 39 (2), 713–724. doi:10.2134/jeq2009.0329
Andrés, E. F. d., Tenorio, J. L., del Mar Albarran, M., and Walter, I. (2012). Carbon dioxide flux in a soil treated with biosolids under semiarid conditions. Compost Sci. and Util. 20 (1), 43–48. doi:10.1080/1065657X.2012.10737021
Atkinson, C. F., Jones, D. D., and Gauthier, J. J. (1996). Putative anaerobic activity in aerated composts. J. Industrial Microbiol. 16 (3), 182–188. doi:10.1007/BF01570002
Azim, K., Soudi, B., Boukhari, S., Perissol, C., Roussos, S., and Thami Alami, I. (2018). Composting parameters and compost quality: a literature review. Org. Agric. 8, 141–158. doi:10.1007/s13165-017-0180-z
Basak, B. B. (2018). Recycling of waste biomass and mineral powder for preparation of potassium-enriched compost. J. Material Cycles Waste Manag. 20 (3), 1409–1415. doi:10.1007/s10163-018-0699-4
Benito, M., Masaguer, A., Moliner, A., and De Antonio, R. (2005). Carbon mineralization of pruning wastes compost at different stages of composting. Compost Sci. Util. 13 (3), 203–207. doi:10.1080/1065657X.2005.10702241
Bennett, A. J., Bending, G. D., Chandler, D., Hilton, S., and Mills, P. (2012). Meeting the demand for crop production: the challenge of yield decline in crops grown in short rotations. Biol. Rev. 87 (1), 52–71. doi:10.1111/j.1469-185X.2011.00184.x
Bernal, M. P., Sánchez-Monedero, M. A., Paredes, C., and Roig, A. (1998). Carbon mineralization from organic wastes at different composting stages during their incubation with soil. Agric. Ecosyst. Environ. 69 (3), 175–189. doi:10.1016/S0167-8809(98)00106-6
Bernal, M. P., Sommer, S. G., Chadwick, D., Qing, C., Guoxue, L., and Michel, F. C. (2017). “Chapter three – current approaches and future trends in compost quality criteria for agronomic, environmental, and human health benefits,” in Advances in agronomy. Editor D. L. Sparkes (Academic Press), 144, 143–223.
Bhattacharyya, P., Chakrabarti, K., Chakraborty, A., Nayak, D. C., Tripathy, S., and Powell, M. A. (2007). Municipal waste compost as an alternative to cattle manure for supplying potassium to lowland rice. Chemosphere 66 (9), 1789–1793. doi:10.1016/j.chemosphere.2006.07.062
Bonifacio, H., Rotz, C., and Richard, T. (2016) “Modeling of carbon and nitrogen gaseous emissions from cattle manure compost windrows,” in Paper presented at 2016 American society of agricultural and biological engineers annual international meeting. Orlando, FL. doi:10.13031/aim.20162459669
Brempong, M. B., Norton, U., and Norton, J. B. (2019). Compost and soil moisture effects on seasonal carbon and nitrogen dynamics, greenhouse gas fluxes and global warming potential of semi-arid soils. Int. J. Recycl. Org. Waste Agric. 8, 367–376. doi:10.1007/s40093-019-00309-4
Cáceres, R., Flotats, X., and Marfà, O. (2006). Changes in the chemical and physicochemical properties of the solid fraction of cattle slurry during composting using different aeration strategies. Waste Manag. 26 (10), 1081–1091. doi:10.1016/j.wasman.2005.06.013
Cáceres, R., Malińska, K., and Marfà, O. (2018). Nitrification within composting: a review. Waste Manag. 72, 119–137. doi:10.1016/j.wasman.2017.10.049
Cao, X., Williams, P. N., Zhan, Y., Coughlin, S. A., McGrath, J. W., Chin, J. P., et al. (2023). Municipal solid waste compost: global trends and biogeochemical cycling. Soil and Environ. Health 1 (4), 100038. doi:10.1016/j.seh.2023.100038
Chang, C.-H., Chen, I.-C., and Yang, S.-S. (2009). Methane and carbon dioxide emissions from different composting periods. Terr. Atmos. Ocean. Sci. 20 (3), 511–520. doi:10.3319/TAO.2008.05.20.01(A)
Chroni, C., Kyriacou, A., Manios, T., and Lasaridi, K.-E. (2009). Investigation of the microbial community structure and activity as indicators of compost stability and composting process evolution. Bioresour. Technol. 100 (15), 3745–3750. doi:10.1016/j.biortech.2008.12.016
Danon, M., Franke-Whittle, I. H., Insam, H., Chen, Y., and Hadar, Y. (2008). Molecular analysis of bacterial community succession during prolonged compost curing. FEMS Microbiol. Ecol. 65 (1), 133–144. doi:10.1111/j.1574-6941.2008.00506.x
Danon, M., Zmora-Nahum, S., Chen, Y., and Hadar, Y. (2007). Prolonged compost curing reduces suppression of sclerotium rolfsii. Soil Biol. Biochem. 39 (8), 1936–1946. doi:10.1016/j.soilbio.2007.02.012
Duddigan, S., Fraser, T., Green, I., Diaz, A., Sizmur, T., and Tibbett, M. (2021). Plant, soil and faunal responses to a contrived pH gradient. Plant Soil 462 (1–2), 505–524. doi:10.1007/s11104-021-04879-z
Eiland, F., Klamer, M., Lind, A.-M., Leth, M., and Bååth, E. (2001). Influence of initial C/N ratio on chemical and microbial composition during long term composting of straw. Microb. Ecol. 41 (3), 272–280. doi:10.1007/s002480000071
Ekinci, K., Tosun, I., Şevik, F., Bitrak, N. B., Sülük, K., and Kumbul, B. S. (2021). Effects of initial C/N ratio on maturity and stability of compost produced from two-phase olive mill pomace, poultry and dairy manure and straw. Compost Sci. and Util. 29 (3-4), 95–106. doi:10.1080/1065657X.2023.2270972
Environment and Climate Change Canada (2020) “Monthly climate summaries,” in Historical climate data. Available at: https://climate.weather.gc.ca/prods_servs/cdn_climate_summary_e.html (Accessed November 3, 2022).
Fox, J., and Weisberg, S. (2019). An R companion to applied regression. 3rd edn. Thousand Oaks, CA: Sage.
Gallardo-Lara, F., and Nogales, R. (1987). Effect of the application of town refuse compost on the soil-plant system: a review. Biol. Wastes 19 (1), 35–62. doi:10.1016/0269-7483(87)90035-8
Gao, M., Liang, F., Yu, A., Li, B., and Yang, L. (2010). Evaluation of stability and maturity during forced-aeration composting of chicken manure and sawdust at different C/N ratios. Chemosphere 78 (5), 614–619. doi:10.1016/j.chemosphere.2009.10.056
Ge, M., Shen, Y., Ding, J., Meng, H., Zhou, H., Zhou, J., et al. (2022). New insight into the impact of moisture content and pH on dissolved organic matter and microbial dynamics during cattle manure composting. Bioresour. Technol. 344, 126236. doi:10.1016/j.biortech.2021.126236
González-Ubierna, S., de la Cruz, M. T., and Casermeiro, M. A. (2015). How do biodegradable organic residues affect soil CO2 emissions? Case study of a mediterranean agro-ecosystem. Soil Tillage Res. 153, 48–58. doi:10.1016/j.still.2015.05.002
Grey, M., and Henry, C. (1999). Nutrient retention and release characteristics from municipal solid waste compost. Compost Sci. Util. 7 (1), 42–50. doi:10.1080/1065657X.1999.10701951
Hameed, M., Bhat, R. A., Pandit, B. A., Ramzan, S., Dijoo, Z. K., and Wani, M. A. (2022). Qualitative assessment of compost engendered from municipal solid waste and green waste by indexing method. J. Air Waste Manag. Assoc. 72 (2), 210–219. doi:10.1080/10962247.2021.1959466
Hellmann, B., Zelles, L., Palojärvi, A., and Bai, Q. (1997). Emission of climate-relevant trace gases and succession of microbial communities during open-windrow composting. Appl. Environ. Microbiol. 63 (3), 1011–1018. doi:10.1128/aem.63.3.1011-1018.1997
Hemidat, S., Jaar, M., Nassour, A., and Nelles, M. (2018). Monitoring of composting process parameters: a case study in Jordan. Waste Biomass Valorization 9 (12), 2257–2274. doi:10.1007/s12649-018-0197-x
Hendershot, W. H., Lalande, H., and Duquette, M. (2007). “Soil reaction and exchangeable acidity,” in Soil sampling and methods of analysis. 2nd Edn, Editors M. R. Carter,, and E. G. Gregorich (Abingdon, UK: Taylor and Francis), 173–178.
Hutchinson, M., and Griffin, T. S. (2008). Evaluation of fiber content relative to other measures of compost stability. Compost Sci. and Util. 16 (1), 6–11. doi:10.1080/1065657X.2008.10702349
Jain, M. S., Daga, M., and Kalamdhad, A. S. (2019). Variation in the key indicators during composting of municipal solid organic wastes. Sustain. Environ. Res. 29, 9. doi:10.1186/s42834-019-0012-9
James, G., Witten, D., Hastie, T., and Tibshirani, R. (2021) “An introduction to statistical learning,” in Springer texts in statistics. 2nd Edn. New York, NY: Springer US. doi:10.1007/978-1-0716-1418-1
Jamroz, E., Bekier, J., Medynska-Juraszek, A., Kaluza-Haladyn, A., Cwielag-Piasecka, I., and Bednik, M. (2020). The contribution of water extractable forms of plant nutrients to evaluate MSW compost maturity: a case study. Sci. Rep. 10 (1), 12842. doi:10.1038/s41598-020-69860-9
Jiménez, E. I., and Garcia, V. P. (1989). Evaluation of city refuse compost maturity: a review. Biol. Wastes 27 (2), 115–142. doi:10.1016/0269-7483(89)90039-6
Kaza, S., Yao, L. C., Bhada-Tata, P., and Woerden, F. V. (2018). What a waste 2.0: a global snapshot of solid waste management to 2050. Washington, DC: World Bank.
Komilis, D. P., and Tziouvaras, I. S. (2009). A statistical analysis to assess the maturity and stability of six composts. Waste Manag. 29 (5), 1504–1513. doi:10.1016/j.wasman.2008.10.016
Kong, Y., Zhang, J., Yang, Y., Liu, Y., Zhang, L., Wang, G., et al. (2023). Determining the extraction conditions and phytotoxicity threshold for compost maturity evaluation using the seed germination index method. Waste Manag. 171, 502–511. doi:10.1016/j.wasman.2023.09.040
Lachat Instruments (2003). Determination of nitrate/nitrite in surface and wastewaters by flow injection analysis. QuickChem Method 10-107-04-1-J.
Lachat Instruments (2008). Determination of ammonia in brackish or seawater by flow injection analysis quikchem method 31-107-06-1-B.
Lei, N., and Han, J. (2020). Effect of precipitation on respiration of different reconstructed soils. Sci. Rep. 10 (1), 7328. doi:10.1038/s41598-020-63420-x
Levanon, D., and Pluda, D. (2002). Chemical, physical and biological criteria for maturity in composts for organic farming. Compost Sci. Util. 10 (4), 339–346. doi:10.1080/1065657X.2002.10702096
Levene, H. (1960). “Robust tests for equality of variances,” in Contributions to probability and statistics. Editor I. Olkin (Stanford University Press), 278–292.
López-González, J. A., Suárez-Estrella, F., Vargas-García, M. C., López, M. J., Jurado, M. M., and Moreno, J. (2015). Dynamics of bacterial microbiota during lignocellulosic waste composting: studies upon its structure, functionality and biodiversity. Bioresour. Technol. 175, 406–416. doi:10.1016/j.biortech.2014.10.123
Lou, X. F., and Nair, J. (2009). The impact of landfilling and composting on greenhouse gas emissions - a review. Bioresour. Technol. 100 (16), 3792–3798. doi:10.1016/j.biortech.2008.12.006
Mahapatra, S., Ali, M. H., and Samal, K. (2022). Assessment of compost maturity-stability indices and recent development of composting bin. Energy Nexus 6 (16), 100062. doi:10.1016/j.nexus.2022.100062
Martín, J., Santos, J. L., Aparicio, I., and Alonso, E. (2015). Pharmaceutically active compounds in sludge stabilization treatments: anaerobic and aerobic digestion, wastewater stabilization ponds and composting. Sci. Total Environ. 503 (4), 97–104. doi:10.1016/j.scitotenv.2014.05.089
Mehlich, A. (1984). Mehlich 3 soil test extractant: a modification of Mehlich 2 extractant. Commun. Soil Sci. Plant Analysis 15 (12), 1409–1416. doi:10.1080/00103628409367568
Meng, L., Li, W., Zhang, S., Wu, C., and Lv, L. (2017). Feasibility of co-composting of sewage sludge, spent mushroom substrate and wheat straw. Bioresour. Technol. 226, 39–45. doi:10.1016/j.biortech.2016.11.054
Michel, F., O’Neil, T., Rynk, R., Gilbert, J., Wisbaum, S., and Halbach, T. (2022). “Chapter 5 - passively aerated composting methods, including turned windrows,” in The Composting Handbook: a how-to and why manual for farm, municipal, institutional and commercial composters. Editor R. Rynk (Academic Press), 159–196.
Nair, A., and Ngouajio, M. (2012). Soil microbial biomass, functional microbial diversity, and nematode community structure as affected by cover crops and compost in an organic vegetable production system. Appl. Soil Ecol. 58, 45–55. doi:10.1016/j.apsoil.2012.03.008
N’Dayegamiye, A., and Isfan, D. (1991). Chemical and biological changes in compost of wood shavings, sawdust and peat moss. Can. J. Soil Sci. 71 (4), 475–484. doi:10.4141/cjss91-046
NiChualain, D., and Prasad, M. (2009). Evaluation of three methods for determination of stability of composted material destined for use as a component of growing media. Acta Hortic. 819, 303–310. doi:10.17660/ActaHortic.2009.819.35
Nordahl, S. L., Preble, C. V., Kirchstetter, T. W., and Scown, C. D. (2023). Greenhouse gas and air pollutant emissions from composting. Environ. Sci. and Technol. 57 (6), 2235–2247. doi:10.1021/acs.est.2c05846
Ozores-Hampton, M., Biala, J., Evanylo, G., Faucette, B., Cooperband, L., Roe, N., et al. (2022). “Chapter 16 – compost use,” in The Composting Handbook: a how-to and why manual for farm, municipal, institutional and commercial composters. Editor R. Rynk (Academic Press), 777–846.
Paré, T., Dinel, H., Schnitzer, M., and Dumontet, S. (1998). Transformations of carbon and nitrogen during composting of animal manure and shredded paper. Biol. Fertil. Soils 26 (3), 173–178. doi:10.1007/s003740050364
Parkin, T. B., and Kaspar, T. C. (2003). Temperature controls on diurnal carbon dioxide flux. Soil Sci. Soc. Am. J. 67 (6), 1763–1772. doi:10.2136/sssaj2003.1763
Parkinson, K. J. (1981). An improved method for measuring soil respiration in the field. J. Appl. Ecol. 18 (1), 221–228. doi:10.2307/2402491
Poulsen, T. G. (2010). Temperature, pressure, and air flow distribution in passively aerated compost piles. Compost Sci. Util. 18 (2), 127–134. doi:10.1080/1065657X.2010.10736945
Quintern, M. (2014). Full scale vermicomposting and land utilisation of pulpmill solids in combination with municipal biosolids (Sewage Sludge). WIT Trans. Ecol. Environ. 180, 65–76. doi:10.2495/WM140061
Raich, J. W., and Tufekcioglu, A. (2000). Vegetation and soil respiration: correlations and controls. Biogeochemistry 48 (1), 71–90. doi:10.1023/A:1006112000616
Sánchez-Monedero, M. A., Serramiá, N., Civantos, C. G.-O., Fernández-Hernández, A., and Roig, A. (2010). Greenhouse gas emissions during composting of two-phase olive mill wastes with different agroindustrial by-products. Chemosphere 81 (1), 18–25. doi:10.1016/j.chemosphere.2010.07.022
Sciubba, L., Cavani, L., Grigatti, M., Ciavatta, C., and Marzadori, C. (2015). Relationships between stability, maturity, water-extractable organic matter of municipal sewage sludge composts and soil functionality. Environ. Sci. Pollut. Res. 22 (17), 13393–13403. doi:10.1007/s11356-015-4611-7
Scotti, R., Bonanomi, G., Scelza, R., Zoina, A., and Rao, M. A. (2015). Organic amendments as sustainable tool to recovery fertility in intensive agricultural systems. J. Soil Sci. Plant Nutr. 15 (2), 0–52. doi:10.4067/S0718-95162015005000031
Shahzad, K., Bary, A. I., Collins, D. P., Chalker-Scott, L., Abid, M., Sintim, H. Y., et al. (2019). Carbon dioxide and oxygen exchange at the soil-atmosphere boundary as affected by various mulch materials. Soil Tillage Res. 194, 104335. doi:10.1016/j.still.2019.104335
Shapiro, S. S., and Wilk, M. B. (1965). An analysis of variance test for normality (complete samples). Biometrika 52 (3–4), 591–611. doi:10.1093/biomet/52.3-4.591
Sommer, S. G., McGinn, S. M., Hao, X., and Larney, F. J. (2004). Techniques for measuring gas emissions from a composting stockpile of cattle manure. Atmos. Environ. 38 (28), 4643–4652. doi:10.1016/j.atmosenv.2004.05.014
Statistics Canada (2022). Table 38-10-0138-01 Waste materials diverted, by type and by source (dataset). Bienn. waste Manag. Surv. doi:10.25318/3810013801
Stegenta, S., Sobieraj, K., Pilarski, G., Koziel, J. A., and Białowiec, A. (2019). Analysis of the spatial and temporal distribution of process gases within municipal biowaste compost. Sustain. Switz. 11 (8), 2340. doi:10.3390/su11082340
Swati, A., and Hait, S. (2018). Greenhouse gas emission during composting and vermicomposting of organic wastes - a review. Clean. - Soil, Air, Water 46 (6), 1700042. doi:10.1002/clen.201700042
Temel, F. A., Yolcu, O. C., and Turan, N. G. (2023). Artificial intelligence and machine learning approaches in composting process: a review. Bioresour. Technol. 370, 128539. doi:10.1016/j.biortech.2022.128539
The Compost Council of Canada (2016). Best practices for operating an aerated windrow composting facility.
Tognetti, C., Mazzarino, M. J., and Laos, F. (2007). Improving the quality of municipal organic waste compost. Bioresour. Technol. 98 (5), 1067–1076. doi:10.1016/j.biortech.2006.04.025
Turner, T., Wheeler, R., and Oliver, I. W. (2022). Evaluating land application of pulp and paper mill sludge: a review. J. Environ. Manag. 317, 115439. doi:10.1016/j.jenvman.2022.115439
U.S. EPA (2004). Method 9045D: soil and waste pH, part of test methods for evaluating solid waste. Wageningen, Netherlands: Physical/Chemical Methods.
van Reeuwijk, L. P. (2002). Procedures for soil analysis. 6th Edn. International Soil Reference and Information Centre.
Vergara, S. E., and Silver, W. L. (2019). Greenhouse gas emissions from windrow composting of organic wastes: patterns and emissions factors. Environ. Res. Lett. 14 (12), 124027. doi:10.1088/1748-9326/ab5262
Williams, S. R., Zhu-Barker, X., Lew, S., Croze, B. J., Fallan, K. R., and Horwath, W. R. (2019). Impact of composting food waste with green waste on greenhouse gas emissions from compost windrows. Compost Sci. and Util. 27 (1), 35–45. doi:10.1080/1065657X.2018.1550023
Yang, W., Zhang, X., Wu, L., Rensing, C., and Xing, S. (2021). Short-term application of magnesium fertilizer affected soil microbial biomass, activity, and community structure. J. Soil Sci. Plant Nutr. 21 (1), 675–689. doi:10.1007/s42729-020-00392-x
Yousefi, J., Younesi, H., and Ghasempoury, S. M. (2013). Co-composting of municipal solid waste with sawdust: improving compost quality. Clean. - Soil, Air, Water 41 (2), 185–194. doi:10.1002/clen.201100315
Zahra, K., Farhan, M., Kanwal, A., Sharif, F., Hayyat, M. U., Shahzad, L., et al. (2023). Investigating the role of bulking agents in compost maturity. Sci. Rep. 13 (1), 16003. doi:10.1038/s41598-023-41891-y
Keywords: municipal solid waste (MSW), pulp and paper waste, compost chemical properties, maturation phases, carbon dioxide emissions (CO2 emissions)
Citation: Pennell T, Comeau L-P, MacKinley K, Hann S, Heung B and Kiely B (2024) Characterization of carbon dioxide emissions from late stage windrow composting. Front. Environ. Sci. 12:1453306. doi: 10.3389/fenvs.2024.1453306
Received: 22 June 2024; Accepted: 13 August 2024;
Published: 26 August 2024.
Edited by:
Rui Yang, Chinese Academy of Agricultural Sciences, ChinaReviewed by:
Sangeeta Lenka, Indian Institute of Soil Science (ICAR), IndiaCurtis Dell, United States Department of Agriculture, United States
Copyright © 2024 Pennell, Comeau, MacKinley, Hann, Heung and Kiely. This is an open-access article distributed under the terms of the Creative Commons Attribution License (CC BY). The use, distribution or reproduction in other forums is permitted, provided the original author(s) and the copyright owner(s) are credited and that the original publication in this journal is cited, in accordance with accepted academic practice. No use, distribution or reproduction is permitted which does not comply with these terms.
*Correspondence: Louis-Pierre Comeau, louis-pierre.comeau@agr.gc.ca