- 1Manaaki Whenua—Landcare Research, Lincoln, New Zealand
- 2Manaaki Whenua—Landcare Research, Hamilton, New Zealand
- 3Waikato Regional Council, Hamilton, New Zealand
Mitigation practices for nitrogen leaching losses from livestock agriculture are needed to protect freshwater quality and increase the efficiency of agricultural production. Within New Zealand, the most common pasture type is a two-species mix of perennial ryegrass (Lolium perenne) and white clover (Trifolium repens). Ecological theory suggests that increasing species and functional diversity improves ecosystem function, including nitrogen (N) retention. Use of more diverse pasture types, including a mix of pasture grasses, legumes and other forbs, particularly plantain (Plantago lanceolata), with functional traits, including winter activity, deep-rooting, N fixation, and biological inhibition of nitrification in the soil, is a potential mitigation practice that requires further verification with long-term field measurements. Here we utilize a network of large lysimeters to make field-based measurements of N leaching from 5–8 species diverse pasture, including plantain, under a range of soil, climate and management conditions, for comparison with losses from traditional ryegrass-clover pasture. Over 3 years of measurements, leaching from fully established diverse pasture was 2–80 kg N ha−1 y−1. No differences were observed in dry matter production or N leaching of diverse pasture compared to ryegrass-clover lysimeters. Large losses, up to 120 kg N ha−1, were observed during periods when pasture was not fully established, including cultivation and sowing of new pasture, depending on season. Timing of management activities could be optimized to minimize these losses. These data provide critical assessment of diverse pasture as a mitigation approach for reducing N losses. Further work on diverse pastures should include higher diversity mixes as well as consideration of animal mediated effects of diverse pasture diets on N inputs.
1 Introduction
Leaching of nitrogen (N) from livestock agriculture negatively affects surface and groundwater quality, contributing to both ecological and human health consequences. Management practices to mitigate leaching losses are needed to minimize the environmental impact of livestock grazing, as well as increase the efficiency of agricultural practices, as nutrients lost to leaching could otherwise support production. Increasing species diversity of pastures is a management practice with the potential to reduce N losses. However, there is an insufficient number of long-term field studies of leaching losses from more diverse pastures to verify a mitigation effect.
There is a positive relationship between plant species diversity, productivity, and N retention of grassland ecosystems established in the ecological literature (Tilman et al., 1996; Hector et al., 1999; Hooper et al., 2005). Such effects arise from complementarity amongst functional traits affecting resource acquisition. Multispecies mixes, compared to monocultures, lose less N to leaching, although there can be complex changes in the processes contributing to N loss (Dijkstra et al., 2007). In addition to reducing N leaching, other benefits of increasing diversity include enhanced soil carbon stocks (Lange et al., 2015), increased soil water infiltration (Fischer et al., 2015), and increased resistance to drought (Isbell et al., 2015). The diversity-ecosystem function concepts from the ecological literature can be applied to grazing landscapes, where increasing diversity of pastures has the potential to increase productivity and environmental sustainability of grazing land (Sanderson et al., 2004; Vibart et al., 2016).
Pastures in New Zealand are most often a two species mix of perennial ryegrass (Lolium perenne) and white clover (Trifolium repens), henceforth “ryegrass-clover.” However, there is increasing adoption of more diverse pastures with four or more species, including a mixture of grasses, legumes, and forbs, henceforth “diverse pasture.” The species mixtures of diverse pasture are generally selected to possess beneficial functional traits, such as greater cool-season activity to increase N uptake during winter drainage seasons (Malcolm et al., 2014), deep-rooting species that contribute to higher belowground biomass (McNally et al., 2015) and greater legume content to enhance N fixation, decreasing reliance on chemical fertilizers (Peoples et al., 2009). Species such as plantain (Plantago lanceolata) are known to affect N transformations in the soil, so-called biological nitrification inhibitors, reducing both N leaching and nitrous oxide (N2O) emissions (Di et al., 2016; Gardiner et al., 2018; Podolyan et al., 2020). Animal mediated effects have been documented, including changes in amounts and concentrations of N in excreta of livestock fed on diverse pasture and plantain diets (Vibart et al., 2016; Pinxterhuis et al., 2024). Such changes to amounts and concentrations of N inputs have implications for N leaching losses.
Malcolm et al. (2014) made direct measurements of N leaching from lysimeters with mixes of up to four species. Following application of a simulated urine patch, leaching from 4-species diverse pasture was not different from perennial ryegrass-clover lysimeters. However, lysimeters containing winter-active Italian ryegrass (Lolium multiflorum) leached 24%–54% less than perennial ryegrass-clover due to higher dry matter production and N uptake, demonstrating the importance of seasonal activity for reducing N losses. While this single study did not find a mitigation effect of diverse pasture, further field measurements across different soil types and management intensities are needed to identify the mitigation potential of diverse pastures for reducing N losses.
The objective of this study was to provide field-scale measurements of N leaching losses from diverse pasture on contrasting soil types and for a range of management intensities, from low-input, non-irrigated sites to high-intensity effluent irrigated sites, for comparison with N losses from ryegrass-clover pasture. We employed a network of large lysimeters to make direct measurements of leaching losses from diverse and ryegrass-clover pastures. These measurements provide critical assessment of diverse pastures as a mitigation approach, reducing N losses from livestock agriculture. Our dataset will likewise contribute to process-based modelling of N leaching in these systems and inform decision support tools for farmers, regulators, and land managers.
2 Methods
2.1 Lysimeter sites
2.1.1 Ashley Dene Research and Development Station
The Lincoln University Ashley Dene Research and Development Station (ADRDS) is a 190-ha commercial dairy farm in Canterbury, South Island, New Zealand (43°38′56.2″S, 172°21′04.1″E, 32 m a.s.l., Supplementary Figure S1). The mean annual air temperature is 12°C, with maximum and minimum temperatures of 34° and −4°C respectively. The site receives c. 780 mm y−1 of precipitation. Much of the farm receives 200–400 mm y−1 irrigation during the growing season (October – April). Dairy effluent (henceforth “effluent”), collected from the milking shed into storage ponds, is applied by irrigator to a portion of the pasture area. The ADRDS is on flat land and the soil is derived from glacial alluvium. Stony silt loam topsoil overlies sandy subsoil, with stone contents of up to 70%. The soil classification is Typic Orthic Brown Soil (Hewitt, 2010).
Lysimeters were installed at three sites across ADRDS: one receiving irrigation and effluent from a central pivot irrigator (EI), one receiving irrigation with water only from a linear irrigator (OI) and one non-irrigated site (NI). All measurement sites are within 500–700 m of each other. Six large lysimeters, 2 m diameter and 1.5 m depth, were constructed in situ from an undisturbed column of soil at the EI and NI site in 2016. Following 4 years of measurements of drainage and leaching from lucerne (Medicago sativa), these lysimeters were sprayed off with glyphosate, the soil inside the lysimeters was cultivated to 250 mm depth, and re-sown with 5–8 species diverse pasture, including plantain (Table 1). A subset at EI were converted in a similar fashion to ryegrass-clover pasture for comparison. Three other lysimeters, 2 m diameter and 1.2 m depth, had been constructed at OI in 2014. Following a crop cycle in maize, these lysimeters were likewise sprayed off and converted to diverse pasture. The pasture species mixes and sowing rates were selected based on commonly used mixes and advice from commercial seed suppliers. EI was converted in autumn (April) 2020, while OI and NI were converted in spring (October) 2020. Further details of the ADRDS lysimeter sites and construction can be found in Carrick et al. (2017), Graham et al. (2019) and Graham et al. (2022).
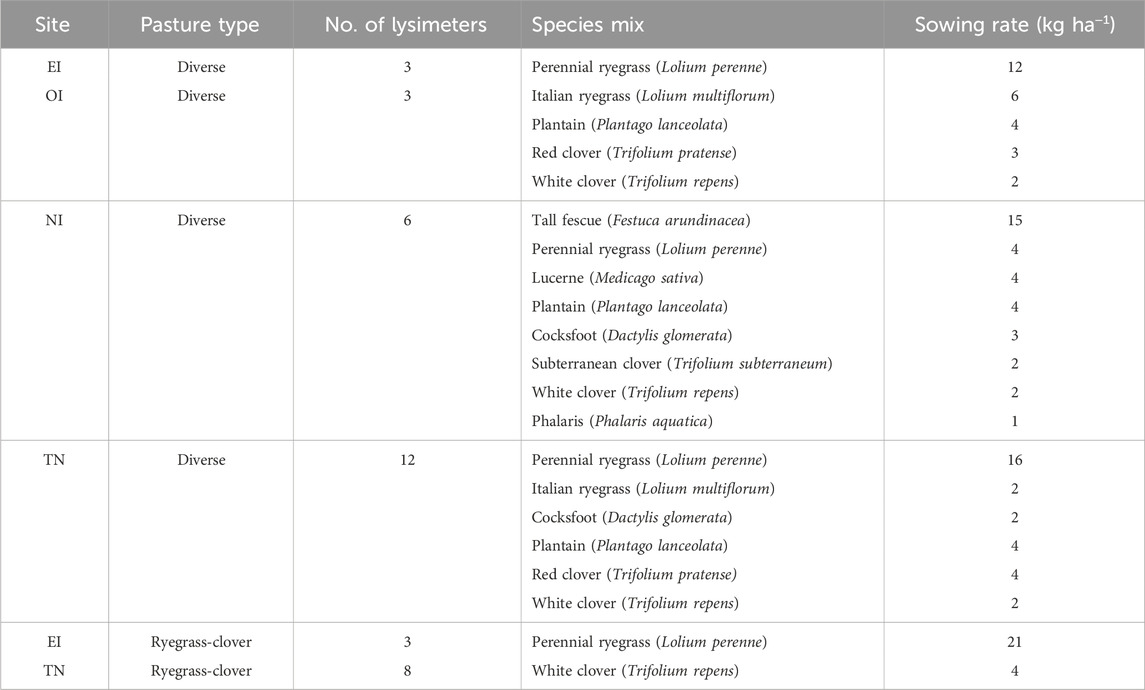
Table 1. Species mixes planted in diverse and ryegrass-clover pasture lysimeters at the Ashley Dene Research and Development Station effluent irrigated (EI) site, irrigated only (OI) site, non-irrigated site, and the Tihoi non-irrigated (TN) site.
2.1.2 Tihoi
The Tihoi lysimeter site (TN) is on a commercially operated sheep and beef farm in the hill country of Waikato, North Island, New Zealand (38°39′51.1″S, 175°44′43.8″E, 564 m a.s.l., Supplementary Figure S1). The mean annual air temperature is 11°C, with maximum and minimum temperatures of 30° and −5°C. The site receives c. 1,400 mm y−1 of precipitation distributed through the year, although summer droughts do occur. The soil is a deep, relatively stone-free volcanic pumice classified as Typic Orthic Pumice Soil (Hewitt, 2010).
In 2016, twenty non-irrigated lysimeters, 0.9 m diameter and 1.5 m depth, were constructed from undisturbed soil and arranged around a central drainage collection caisson. Following 5 years of measurement of drainage and leaching from lucerne and ryegrass-clover, in spring (October) 2020, the lysimeters were sprayed off with glyphosate, cultivated to 150 mm and re-sown with 6 species diverse pasture (12 lysimeters) and ryegrass-clover pasture (8 lysimeters) (Table 1), evenly distributed across the previous lucerne and ryegrass-clover histories. Further details of lysimeter construction can be found in McLeod (2020).
2.2 Drainage volume and leachate collection
At EI, OI and NI, drainage volume from the lysimeters was measured by tipping bucket gauges (0.03 mm drainage resolution), logged continuously by a datalogger. A subsample of leachate was accumulated continuously and was periodically collected for analysis of total N, NO3− (including NO2−) and ammoniacal N (NH3 and NH4+) concentrations by an accredited commercial laboratory following each large drainage event. At TN, lysimeter drainage was into 80 L reservoirs and the drainage volumes since the previous sampling event were manually recorded and a subsample was collected for analysis. Sampling occurred approximately every 20–60 mm of drainage.
2.3 Dry matter harvest
Dry matter production on the lysimeters was measured 5–7 times per year, simulating a grazing cycle appropriate to pasture growth for each site. At EI, OI, and NI, biomass was clipped to a simulated grazing height of 50 mm within a 0.25 m2 quadrat. Subsequently biomass was cut and removed on the remainder of the lysimeter. At TN, biomass was sampled from the entire lysimeter area. The samples were dried at 60°C for 48 h and weighed for dry mass. Seasonally, the clipped biomass was sorted into grasses, legumes, plantain, and other forbs. A subset of the samples was ground and analyzed for analysis of N content of dry matter.
2.4 N inputs
Additions of excreta from grazing animals were simulated in a manner appropriate to each management system at EI, OI and NI. Approximately every second dry matter harvest event, once establishment of pasture was complete, fresh dung (1.1–2.5 kg) was added to the lysimeter in a 300 mm diameter circular patch and synthetic urine (1.1–2.5 L) with a N content of 6 g N L−1 (Clough et al., 1998) was applied to a separate location in a 300 mm diameter circular patch. Amounts and timing were varied to reflect dry matter production and assumed supplemental feed according to the intensity of each management system (Graham et al., 2022). No assumptions were made on the effect of a diet of the different pasture types on N inputs and ryegrass-clover and diverse pasture lysimeters at a given site received the same inputs of dung and urine.
Due to the smaller area of the TN lysimeters, inputs from excreta were simulated though a single application of 2.1 L synthetic urine (McLeod, 2020) each year. Dung was not applied to the TN lysimeters, as it is long-lasting on the soil surface and repeated application would negatively affect dry matter production. Applications of urine occurred in late-summer or autumn each year.
2.5 N uptake and surplus calculations
N uptake by plants (NU) as a component of the lysimeter N balance was calculated from Equation 1:
where MH is the harvested dry matter from each lysimeter, pG, pL, pP, and pO are the proportions of harvested dry matter represented by grass, legumes, plantain and other forbs, respectively, and cG, cL, cP, and cO are the N concentration of grass, legumes, plantain and other forbs. Since a portion of N in legumes comes from fixation of atmospheric N (NF), and not uptake from the soil, this amount was estimated from Equation 2:
where fF is the fraction of N coming from fixation. For the effluent irrigated site, fF was estimated as 0.55, based on previous measurements of lucerne under this management (Graham et al., 2022). For the lower intensity sites, fF was estimated as 0.80, based on measurements from low-input, non-irrigated lucerne.
As a metric of the balance of N inputs and uptake and removal by harvest, surplus N (NS) was calculated by Equation 3:
where NI is the total input of N from fertilizer, effluent and animal excreta.
2.6 Statistical analysis
The statistical significance of differences in the annual total of soil drainage, N leaching and dry matter production between ryegrass-clover and diverse pasture was tested at the EI and TN sites with a Welch’s t-test. This was applied separately for each full year of measurement: Year 1 (October 2020-September 2021), Year 2 (October 2021-September 2022) and Year 3 (October 2022-September 2023). The test was also applied to the cumulative totals for 3 years of measurement.
To determine the drivers of annual N leaching, a linear regression approach was used. A maximal model of annual N leaching loss was constructed, including total annual water input (precipitation and irrigation), drainage volume, grass fraction, plantain fraction, clover fraction, nitrogen surplus and pasture type were included as explanatory factors. Interactions between drainage and N surplus and water input and N surplus were also investigated. This model was then minimized by dropping factors in a stepwise fashion to minimize Akaike’s Information Criterion. Analyses were conducted in R v4.2.1 (R Core Team, 2022). Since there was potential for non-linearity in the responses of N leaching to the explanatory variables, as well as correlations amongst the explanatory variables, an alternative Random Forest modelling approach was conducted using the “randomForest” package in R (Liaw and Wiener, 2002). Additional, and likely correlated, explanatory variables (including dry matter harvest, N input, N uptake) were included in this approach. The importance of each explanatory variable in the Random Forest analysis was estimated based on the percentage change in mean square error associated with each variable. Both modelling approaches were assessed based on a test subset made up of 30% of the original dataset, held out during model fitting.
3 Results
3.1 Drainage and N leaching
The EI lysimeters were the first to be converted from lucerne to diverse or ryegrass–clover pasture in autumn 2020. N losses in the first 6 months following conversion exceeded 120 kg N/ha, higher than the annual loss recorded for any of the sites and measurement years (Figure 1). This high loss could be attributable to low N uptake by the pasture early in establishment, coinciding with high drainage volumes with the onset of winter rain. Sources of N driving this loss are likely to involve mineralization of soil organic matter and dead lucerne biomass, in combination with residual N from animal excreta inputs preceding conversion, and the continued input of 25 kg N ha−1 as effluent while the soil was actively draining.
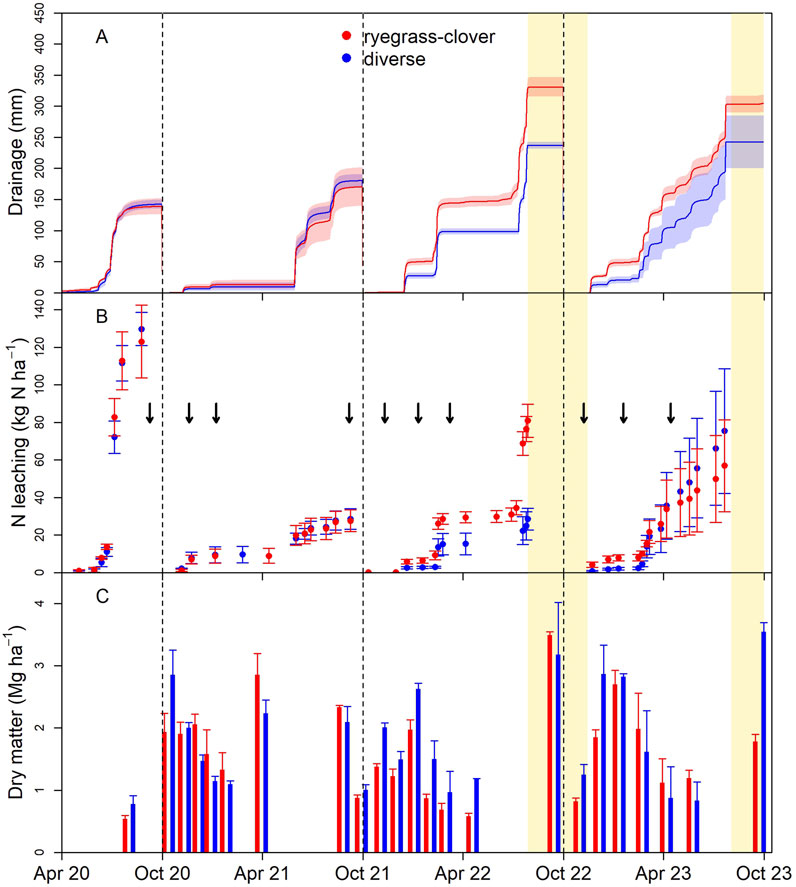
Figure 1. Lysimeter drainage volume (±SEM) (A), cumulative leached nitrogen (B), and dry matter harvest mass (C) following conversion to diverse (blue) or ryegrass–clover (red) pasture in April 2020 and for three subsequent leaching years (October to September) at a site on the Ashley Dene Research and Development Station irrigated with water and effluent (EI); arrows indicate the timing of additions of dung and urine to the lysimeters and the gold-shaded regions indicate periods of lysimeter inoperability due to high groundwater levels.
By the start of Year 1 (October 2020), the pasture was well established at EI. Leaching losses for ryegrass–clover and diverse pasture lysimeters were similar at 27 and 28 kg N ha−1 y−1, respectively (Figure 1; Table 2). In Year 2, a wet winter led to cumulative N leaching from ryegrass-clover of 80 kg N ha−1 y−1, 2.8 times greater than diverse pasture (p < 0.05). However, lysimeter measurements were interrupted between late-July to November 2022 due to unusually high groundwater levels and inundation of lysimeter instrumentation, introducing some uncertainty into the annual total. The losses during this period were likely low, as few drainage events were recorded at the OI site, which remained operational. In Year 3, N losses from diverse pasture were not statistically different from ryegrass-clover. Once again, the measurements were interrupted by high groundwater levels between August and September 2023 introducing uncertainty into the annual sum.
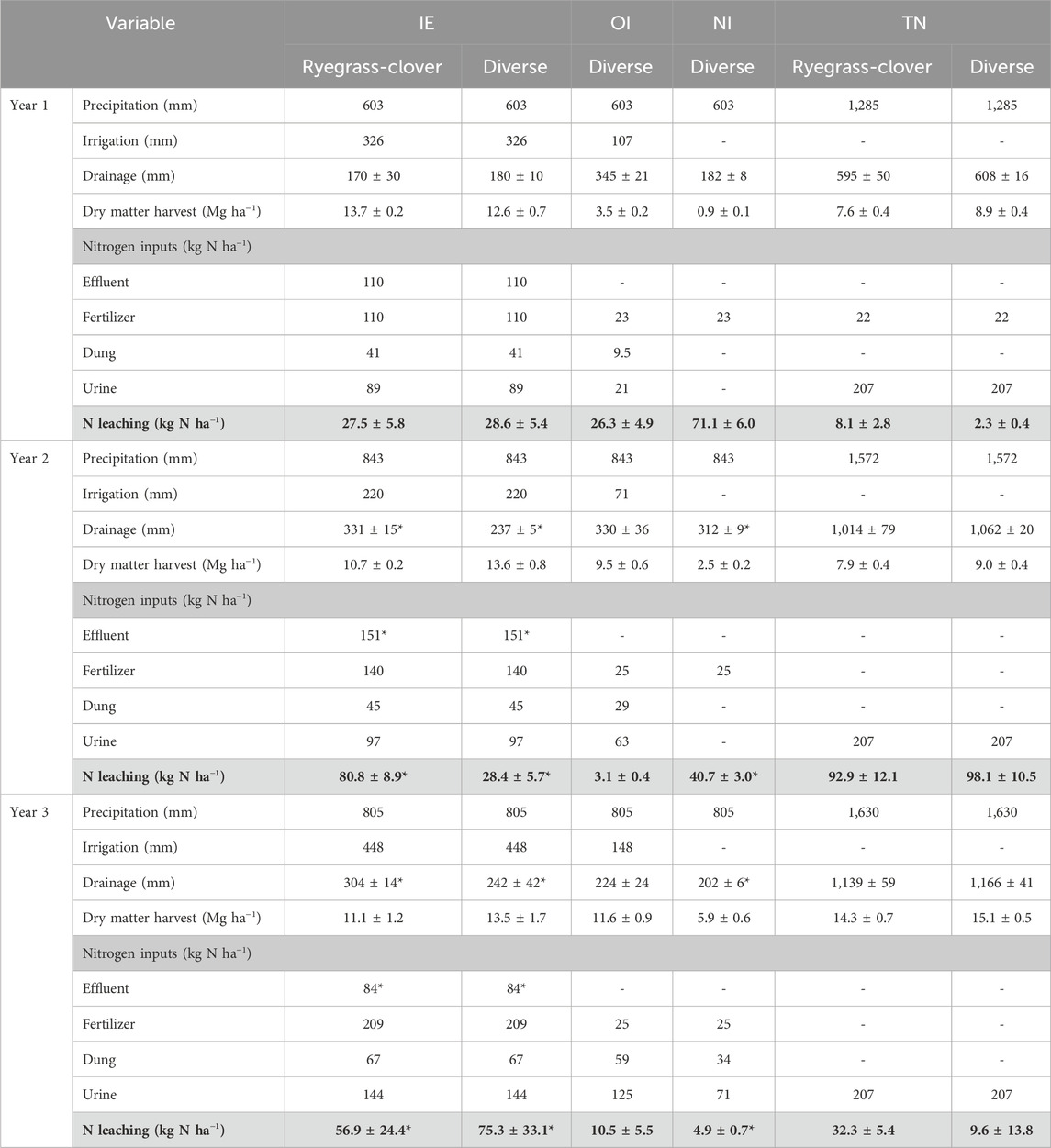
Table 2. Annual totals (±SE) for site variables, nitrogen inputs, and nitrogen losses for diverse and ryegrass–clover pasture lysimeters at effluent irrigated (IE), irrigated only (OI) and non-irrigated sites (NI) at the Ashley Dene Research and Development Station and the Tihoi non-irrigated (TN) site for Year 1 (October 2020 – September 2021), Year 2 (October 2021 – September 2022) and Year 3 (October 2022 – September 2023) since conversion to pasture; values affected by periods of lysimeter inoperability are indicated with an asterisk.
At OI, the lysimeters leached 26 kg N ha−1 y−1 in Year 1. The largest losses were in the period immediately following conversion in October 2020 (Figure 2; Table 2). Excess irrigation during this period contributed to drainage during the period when pasture establishment was incomplete and N uptake was low. In Year 2 and Year 3, when pasture was fully established, the losses were lower than Year 1 and the EI site, at 3 and 11 kg N ha−1 y−1, respectively. This reflects the lower N input relative to EI.
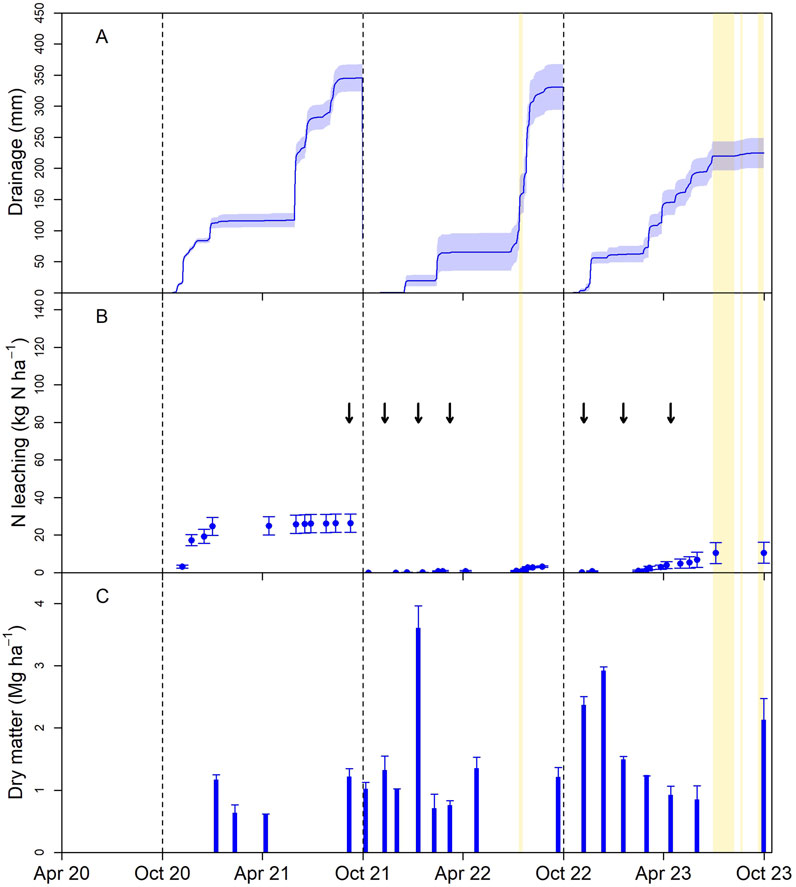
Figure 2. Cumulative drainage volume (±SEM) (A), cumulative leached nitrogen (B), and dry matter harvest mass (C) for diverse pasture in an area of the Ashley Dene Research and Development Station irrigated with water-only (OI) for 3 years following conversion to diverse pasture in October 2020; arrows indicate the timing of additions of dung and urine to the lysimeters, and gold-shaded regions indicate periods of lysimeter inoperability due to high groundwater levels.
At NI, N leaching was 71 kg N ha−1 y−1 in Year 1, larger than those of any of the other sites in Year 1, despite lower N inputs (Figure 3; Table 2). This was likely due to low pasture production and N uptake resulting from poor germination and establishment. In Year 2, the site was re-sown, but losses were 40 kg N ha−1 y−1, corresponding with continued low dry matter production. By Year 3, when pasture establishment was complete, the losses were low, 5 kg N ha−1 y−1. Drainage volumes at NI were similar to EI and OI, reflecting the predominant winter drainage season. However, both EI and OI had growing season drainage events that were not observed at NI (Figures 1–3). These drainage events were the direct effect of irrigation and could have been prevented through improved irrigation management.
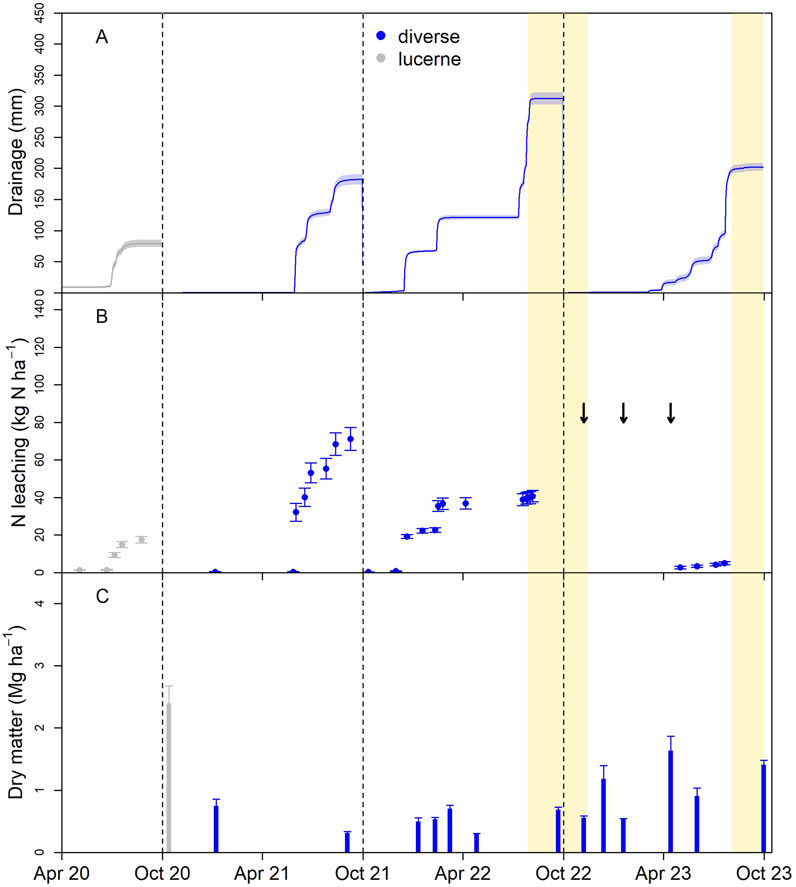
Figure 3. Lysimeter drainage volume (±SEM) (A), cumulative leached nitrogen (B), and dry matter harvest mass (C) following conversion to diverse pasture in a non-irrigated (NI) area of the Ashley Dene Research and Development Station; arrows indicate the timing of additions of dung and urine to the lysimeters, and the gold-shaded regions indicate periods of lysimeter inoperability due to high groundwater levels.
At TN, N leaching losses in Year 1 from diverse pasture lysimeters were not significantly different from ryegrass-clover lysimeters and were small relative to the ADRDS sites (Figure 4; Table 2). There was a trend for enhanced losses from ryegrass-clover pasture throughout the summer of Year 2, despite similar drainage volumes. However, following a second addition of synthetic urine patch in May 2022, and a wet winter, which lead to high drainage volumes, cumulative N losses from ryegrass-clover and diverse pasture were similar by the end of Year 2, 92 and 98 kg N ha−1 y−1, respectively. Throughout Year 3, drainage volumes and N losses continued to be similar for both ryegrass-clover and diverse pasture. The third urine patch was added to the lysimeters in March 2023. Year 3 leaching losses from diverse pasture were 51 kg N ha−1 y−1 compared to 32 kg N ha−1 y−1 from ryegrass-cover. However, again this difference was not significant.
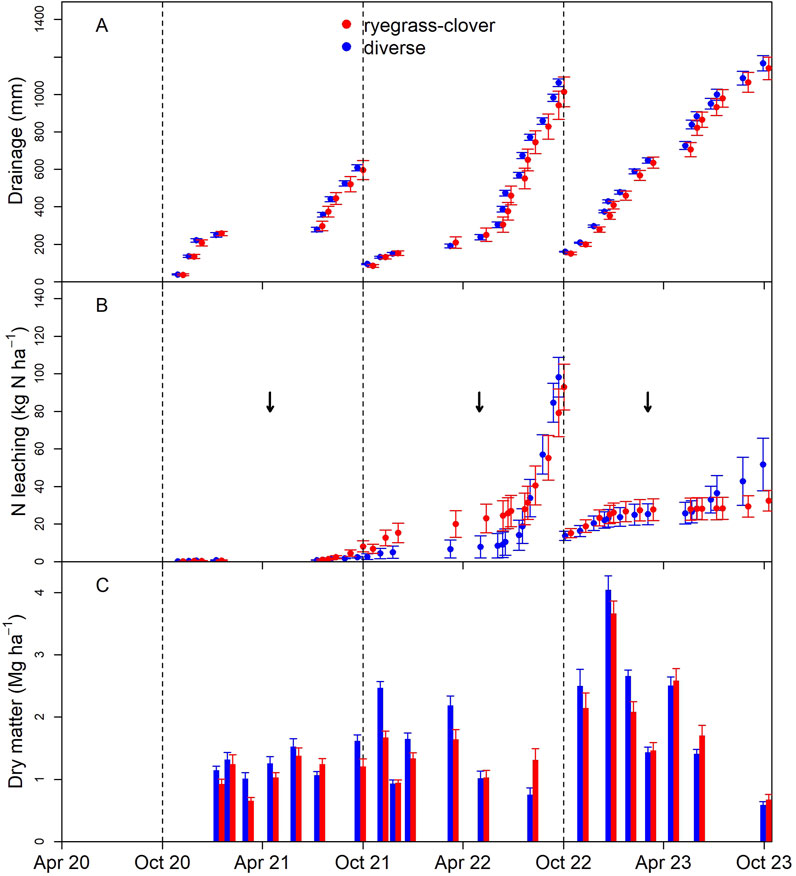
Figure 4. Cumulative drainage volume (±SEM) (A), cumulative leached nitrogen (B), and dry matter harvest mass (C) from lysimeters planted with diverse and ryegrass–clover pasture during Year 1 (October 2020 – September 2021), Year 2 (October 2021 – September 2022) and Year 3 (October 2022 – September 2023) since conversion from lucerne at the Tihoi non-irrigated site (TN); arrows indicate the timing of additions of synthetic urine to the lysimeters.
Due to the treatment of the entire lysimeter as a urine patch at TN, the losses from the lysimeters are not representative of the whole field. A single grazing event leads to 1%–3% of the area receiving urine patches (Haynes and Williams, 1993; Moir et al., 2011). On an annual basis, the assumption is that 25% of the area receives urine patches (Cameron et al., 2013). Lysimeter measurements can be scaled up to the entire field using this assumption, and the finding that losses from pasture not receiving urine are generally low, ∼2 kg N ha−1 y−1 (McLeod, 2020). Thus, the field-scale losses at TN were 2–26 kg N ha−1 y−1 across the measurement years.
3.2 Dry matter production and N uptake
At EI, pasture dry matter production during Year 1 was similar for ryegrass–clover and diverse pasture types under effluent irrigation, at 13.7 and 12.6 Mg dry matter ha−1 y−1 (Figure 1; Table 2). In Year 2, production by diverse pasture was 27% greater than that of ryegrass-clover. In Year 3, biomass production by diverse pasture lysimeters was again statistically similar to ryegrass-clover, at 13.5 and 11.1 Mg ha−1 y−1, respectively. Cumulative production for the entire 3-year measurement period was also similar for the two pasture types. As with dry matter production, N uptake only differed by pasture type in Year 2, when N uptake by diverse pasture was 15% greater than ryegrass-clover. The additional 41 kg N ha−1 y−1 uptake explains the lower leaching losses in Year 2 at EI.
The increase in biomass production by diverse pasture relative to ryegrass–clover in Year 2 at EI also corresponded with a change in the functional group representation. In Year 1 the biomass of diverse pasture was 79% grass (Figure 5). In Year 2, the plantain and clover proportions increased to 32% and 21%, respectively, with the grass proportion decreasing to 44%. However, in Year 3, the plantain content was reduced to 5% while clover content increased to 27%.
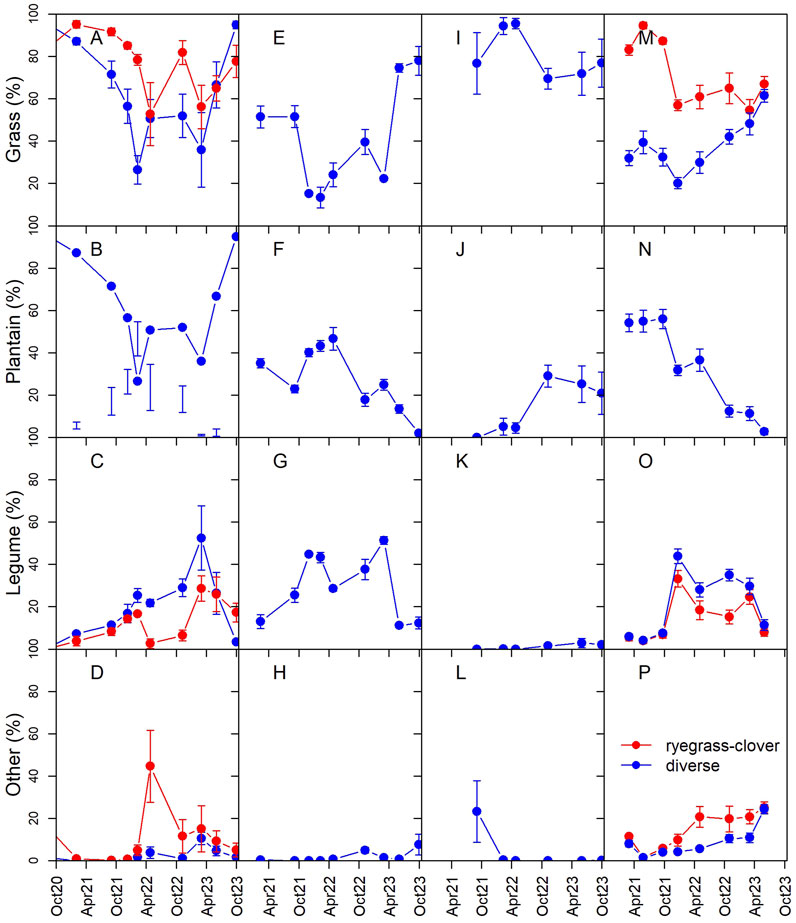
Figure 5. Seasonal and interannual variation in the percentage of dry matter harvested from diverse and ryegrass-clover pasture lysimeters represented by each functional group, including grass, plantain, legumes and other forbs for the Ashley Dene Research and Development Station effluent irrigated (EI) site (A–D), irrigated only (OI) site (E–H), non-irrigated (NI) site (I–L) and the Tihoi (TN) site (M–P).
Despite lower N inputs, dry matter production at OI was 70%–85% of that at EI in Year 2 and Year 3, at 9.5 and 11.6 Mg ha−1 y−1 (Figure 2; Table 2). The OI lysimeters also had the highest plantain content amongst the ADRDS sites, with 43% plantain, 38% clover and 17% grass in Year 2 (Figure 5).
Dry matter production at NI was low in Year 1 due to the failed pasture establishment (Figure 3; Table 2). In Year 2 the site was re-sown, but production remained only 18%–27% of that at other sites. Production increased in Year 3 and harvested dry matter was 5.9 Mg ha−1 y−1, 44% of that by diverse pasture at EI.
Dry matter production of the diverse pasture lysimeters at TN was not statistically different from the ryegrass-clover lysimeters (Figure 4; Table 2). Likewise, there were no significant differences in N uptake. Annual dry matter production at TN was responsive to seasonal water availability, as biomass production was 67%–80% greater in Year 3 compared to Year 2, due to high summer rainfall which contributed to year-round soil drainage, in contrast to the relatively dry summer in Year 2. Uptake of N associated with the high dry matter production also explains the lower leaching losses in Year 3 relative to Year 2, despite similar drainage volumes and N input. Plantain was the primary contributor to harvest biomass in Year 1, representing 55% of dry matter (Figure 5). This dropped to 8% in Year 3, with a commensurate increase in grass fraction.
Nitrogen content of the different functional groups varied, particularly for legumes. However, there was no difference in the N content of bulk, unsorted dry matter harvested from different pasture types at either EI or TN. The N contents of dry matter were 25.5 g N kg−1 for grass, 39.8 g N kg−1 for clovers, 22.5 g N kg−1 for plantain and 29.9 g N kg−1 for other forbs.
3.3 Drivers of annual N leaching
The minimized linear model of annual N leaching included pasture type, water input, drainage volume, grass fraction, plantain fraction, N surplus and an interaction between N surplus and water input. This model explained 51% of variability in lysimeter N leaching in the test dataset across the sites, years of measurement, and pasture types. Although pasture type was included in the minimal model, an analysis of variance associated with the different factors revealed that only site, N surplus, drainage volume, plantain fraction and the interaction between drainage and N surplus were significant (p < 0.05) (Supplementary Figure S2; Table 1).
The Random Forest approach explained 71% of the variability in the test dataset. Analysis of the importance of each explanatory variable, as estimated from percentage increase in mean square error, confirmed the importance of drainage volume and water input, followed by N input and surplus. Plantain fraction and other vegetation-related variables followed with pasture type occupying the lowest level of importance (Supplementary Figures S3, S4).
4 Discussion
Across the measurement sites, which represented contrasting soils, climatic conditions and a range of management intensities, field-scale N leaching losses from established diverse pasture were 2–80 kg N ha−1 y−1, well within the range of values previously measured for grazed pastures (Cameron et al., 2013). Although there were isolated instances in which significant effects of pasture type were observed (e.g., Year 2 at EI), these effects were inconsistent, and none contributed to a change in cumulative leaching loss over the three measurement years. This is similar to a previous study in New Zealand that found no difference between ryegrass-clover and diverse pasture lysimeter leaching (Malcolm et al., 2014). Neither drainage volume nor pasture production differed consistently for diverse and ryegrass-clover lysimeters, contributing to the absence of an effect of pasture type on N leaching.
The result that leaching losses from diverse pasture were not different from ryegrass-clover would appear to be at odds with the ecological literature, which suggests that production and nutrient retention are increased with increasing diversity in grasslands (Tilman et al., 1996; Dijkstra et al., 2007). However, some studies suggest that it is the presence of specific functional groups, and not diversity, that effects productivity and N losses (Hooper and Vitousek, 1998). In the current study, we investigated minimally diverse mixes of 5–8 species, where in some cases only one species filled a functional role. The proportional representation of different functional groups (grass, clover, plantain, other forbs) was also variable through time and, by the end of the study tended to be similar for the different pasture types. Thus, our results may not represent the full potential of increased pasture diversity.
Empirical modelling confirmed the importance of water input, drainage volume and N input and surplus as drivers of N loss, as has been shown previously (Cameron et al., 2013). However, plantain fraction was also identified as a significant driver of N leaching by both the linear regression and Random Forest modelling approaches. This may be an artefact arising from the distribution of plantain across measurement sites and measurement years. N leaching from diverse pasture lysimeters was similar to ryegrass-clover lysimeters in most cases at the comparison sites. However, across the entire dataset, the highest plantain fraction was observed at TN in Year 1, when N losses from both pasture types were low. The plantain fraction subsequently decreased during years 2 and 3 when drainage amounts were higher and subsequent urine patches had been applied. At Ashley Dene, the highest plantain contents were observed at the irrigated only site, which had low N losses due to a low N input compared to the effluent irrigated site. However, the lower losses from diverse pasture in Year 2 at the effluent irrigated site also coincided with the highest plantain content. Hence, it is difficult to draw a definitive conclusion on the effect of plantain on N leaching losses from our data alone.
The inclusion of plantain fraction as an important driver of N leaching is supported by the literature from grazed grasslands, which has shown that including plantain in pastures at moderate rates (30%–40%) can decrease N leaching losses by 20%–60% (Pinxterhuis et al., 2024). However, in contrast to the current study, this result included animal mediated effects of diet on N excretion by animals. Plantain content was variable across sites and through time, despite identical sowing rates. Use of plantain as a mitigation approach will depend on its persistence in the pasture. Regular over-sowing of plantain has been investigated as a management intervention which would reduce N losses without affecting farm profitability (Robertson et al., 2023).
Another potential pathway to realizing the benefits of diversity for mitigating N losses may be through sowing highly diverse pastures. In contrast to the minimally diverse mixes investigated here, highly diverse mixes may have better outcomes due to the inclusion of functional redundancy in the initial species mix and a wider variation in functional traits (Orwin et al., 2022). Such mixes may have a lower risk of degradation over time, and their impact on ecosystem function is likely to improve over time as complementarity amongst species increases (Cardinale et al., 2007; Zheng et al., 2024).
The strongest effect on N loss observed in this study was that of management. The highest level of N input (363–505 kg N/ha/y) at the EI site resulted in the highest field-scale losses, 27–80 kg N ha−1 y−1. At the remaining sites, where N input was generally less than 200 kg N ha−1 y−1, the losses were 2–26 kg N ha−1 y−1 (excluding years with conversion-related N losses). In addition to N input, irrigation contributed to the effects of management intensity on N leaching. At Ashley Dene, drainage events were observed at the EI and OI lysimeters which were not present in the NI lysimeters. The drainage and associated N losses in these events were a direct result of irrigation management, and thus could be avoided through improved irrigation practices. However, these intensive management practices also lead to 15%–205% more dry matter production by the EI site, relative to NI and OI sites.
Pasture conversion was another important source of management-driven N losses. Losses of over 120 kg N ha−1 followed an autumn cultivation and re-sowing at the EI site. This large loss was likely driven by the combination of winter drainage, high residual N in the soil from the previous lucerne crop, and low N uptake by the recently sown pasture. Drainage triggered by irrigation at the OI lysimeters before pasture establishment was complete contributed substantially to the 26 kg N ha−1 y−1 loss in Year 1. Conversely, no large loss was observed for the TN site following successful spring establishment of diverse and ryegrass-clover pasture. These results suggest that conversion activities should be carried out in a manner that prioritizes complete ground coverage and establishment before the winter drainage season.
Empirical modelling of lysimeter drainage could only explain 51%–71% of the variability in lysimeter N leaching, highlighting the need for process-based modelling to disentangle the effects of seasonal dynamics of drainage, N uptake by plants and amounts and timing of N inputs on N leaching losses. Process-based modelling will allow for optimization of the production benefits of intensive practices, such as irrigation, effluent application, and high stocking rates, versus environmental impacts, such as N leaching. Additional co-benefits and trade-offs with other environmental impacts of mitigation practices, such as use of more diverse pasture, need to be considered. For instance, measurements of net greenhouse gas emissions from more diverse pasture demonstrated that a mitigation effect can be shown for N2O emissions, which were 39% lower for diverse pasture relative to ryegrass-clover (Laubach et al., 2023). However, the net emissions, considering all greenhouse gasses, were higher from diverse pasture due to the less favorable CO2 balance. This demonstrates the need for considering a wide range of environmental impacts through process-based modelling. Process-based modelling will likewise facilitate investigation of a wide range of climate and management scenarios, making the results more generalizable for use by decision makers and in the development of decision support tools.
5 Conclusion
Nitrogen leaching losses measured for fully established diverse pasture were 2–80 kg N ha−1 y−1, varying with plant production, volume and timing of drainage, and quantity and timing of N inputs. Harvested dry matter, drainage volume and leaching losses from 5–8 species diverse pasture were not consistently different from ryegrass-clover pasture. Although no mitigation effect of diverse pasture was observed in this case, these results should not be extrapolated to high diversity pasture mixes, which should be verified separately. As well, further work is needed to account for animal mediated effects of diverse pasture diets on N inputs to the system. In this study, the most important driver of N leaching losses was management intensity, with higher losses being associated with higher N inputs. Timing of management activities should also be optimized to ensure full pasture cover leading into the drainage season, as high losses of 120 kg N ha−1 y−1 were observed during the winter drainage season following autumn cultivation and sowing of new pasture in this study. This dataset will be used, along with process-based modelling, to inform decision support tools support farmers and regulators to reduce leaching losses from pasture-based livestock grazing systems.
Data availability statement
The datasets presented in this study can be found in online repositories. The names of the repository/repositories and accession number(s) can be found below: Manaaki Whenua - Landcare Research DataStore (https://doi.org/10.7931/AFSM-BC10).
Author contributions
SG: Writing–review and editing, Writing–original draft, Investigation, Funding acquisition, Formal Analysis, Conceptualization. JP: Writing–review and editing, Investigation, Conceptualization. JL: Writing–review and editing, Data curation, Conceptualization. JH: Writing–review and editing, Methodology, Investigation. GR: Writing–review and editing, Methodology, Investigation. SC: Writing–review and editing, Funding acquisition, Conceptualization. DW: Writing–review and editing, Funding acquisition, Conceptualization. MM: Writing–review and editing, Methodology, Investigation. GM: Writing–review and editing, Investigation. PM: Writing–review and editing, Funding acquisition, Conceptualization.
Funding
The author(s) declare that financial support was received for the research, authorship, and/or publication of this article. This work was funded by the Ministry for Primary Industries Sustainable Land Management and Climate Change Freshwater Mitigation Fund (project 406345).
Acknowledgments
We are grateful to the Lincoln University Ashley Dene Research and Development Station and Tihoi landowners for use of their farms for this study. We thank Brendon Malcolm for helpful comments on an early draft of the manuscript.
Conflict of interest
The authors declare that the research was conducted in the absence of any commercial or financial relationships that could be construed as a potential conflict of interest.
Publisher’s note
All claims expressed in this article are solely those of the authors and do not necessarily represent those of their affiliated organizations, or those of the publisher, the editors and the reviewers. Any product that may be evaluated in this article, or claim that may be made by its manufacturer, is not guaranteed or endorsed by the publisher.
Supplementary material
The Supplementary Material for this article can be found online at: https://www.frontiersin.org/articles/10.3389/fenvs.2024.1445212/full#supplementary-material
References
Cameron, K. C., Di, H. J., and Moir, J. L. (2013). Nitrogen losses from the soil/plant system: a review. Ann. Appl. Biol. 162, 145–173. doi:10.1111/aab.12014
Cardinale, B. J., Wright, J. P., Cadotte, M. W., Carroll, I. T., Hector, A., Srivastava, D. S., et al. (2007). Impacts of plant diversity on biomass production increase through time because of species complementarity. Proc. Natl. Acad. Sci. 104, 18123–18128. doi:10.1073/pnas.0709069104
Carrick, S., Rogers, G., Cameron, K., Malcolm, B., and Payne, J. (2017). Testing large area lysimeter designs to measure leaching under multiple urine patches. N. Z. J. Agric. Res. 60, 205–215. doi:10.1080/00288233.2017.1291527
Clough, T. J., Ledgard, S. F., Sprosen, M. S., and Kear, M. J. (1998). Fate of 15N labelled urine on four soil types. Plant Soil 199, 195–203. doi:10.1023/a:1004361009708
Di, H. J., Cameron, K. C., Podolyan, A., Edwards, G. R., De Klein, C. A., Dynes, R., et al. (2016). The potential of using alternative pastures, forage crops and gibberellic acid to mitigate nitrous oxide emissions. J. Soils Sediments 16, 2252–2262. doi:10.1007/s11368-016-1442-1
Dijkstra, F. A., West, J. B., Hobbie, S. E., Reich, P. B., and Trost, J. (2007). Plant diversity, CO2, and N influence inorganic and organic N leaching in grasslands. Ecology 88, 490–500. doi:10.1890/06-0733
Fischer, C., Tischer, J., Roscher, C., Eisenhauer, N., Ravenek, J., Gleixner, G., et al. (2015). Plant species diversity affects infiltration capacity in an experimental grassland through changes in soil properties. Plant Soil 397, 1–16. doi:10.1007/s11104-014-2373-5
Gardiner, C. A., Clough, T. J., Cameron, K. C., Di, H. J., Edwards, G. R., and De Klein, C. a.M. (2018). Potential inhibition of urine patch nitrous oxide emissions by Plantago lanceolata and its metabolite aucubin. N. Z. J. Agric. Res. 61, 495–503. doi:10.1080/00288233.2017.1411953
Graham, S. L., Laubach, J., Hunt, J. E., Eger, A., Carrick, S., and Whitehead, D. (2019). Predicting soil water balance for irrigated and non-irrigated lucerne on stony, alluvial soils. Agric. Water Manag. 226, 105790. doi:10.1016/j.agwat.2019.105790
Graham, S. L., Laubach, J., Hunt, J. E., Mudge, P. L., Nuñez, J., Rogers, G. N. D., et al. (2022). Irrigation and grazing management affect leaching losses and soil nitrogen balance of lucerne. Agric. Water Manag. 259, 107233. doi:10.1016/j.agwat.2021.107233
Haynes, R. J., and Williams, P. H. (1993). “Nutrient cycling and soil fertility in the grazed pasture ecosystem,” in Advances in agronomy. Editor D. L. Sparks (Academic Press), 119–199.
Hector, A., Schmid, B., Beierkuhnlein, C., Caldeira, M. C., Diemer, M., Dimitrakopoulos, P. G., et al. (1999). Plant diversity and productivity experiments in European grasslands. Science 286, 1123–1127. doi:10.1126/science.286.5442.1123
Hooper, D. U., Chapin Iii, F. S., Ewel, J. J., Hector, A., Inchausti, P., Lavorel, S., et al. (2005). Effects of biodiversity on ecosystem functioning: a consensus of current knowledge. Ecol. Monogr. 75, 3–35. doi:10.1890/04-0922
Hooper, D. U., and Vitousek, P. M. (1998). Effects of plant composition and diversity on nutrient cycling. Ecol. Monogr. 68, 121–149. doi:10.1890/0012-9615(1998)068[0121:eopcad]2.0.co;2
Isbell, F., Craven, D., Connolly, J., Loreau, M., Schmid, B., Beierkuhnlein, C., et al. (2015). Biodiversity increases the resistance of ecosystem productivity to climate extremes. Nature 526, 574–577. doi:10.1038/nature15374
Lange, M., Eisenhauer, N., Sierra, C. A., Bessler, H., Engels, C., Griffiths, R. I., et al. (2015). Plant diversity increases soil microbial activity and soil carbon storage. Nat. Commun. 6, 6707–6708. doi:10.1038/ncomms7707
Laubach, J., Hunt, J. E., Graham, S. L., Buxton, R. P., Rogers, G. N. D., Mudge, P. L., et al. (2023). Mitigation potential and trade-offs for nitrous oxide emissions and carbon balances of irrigated mixed-species and ryegrass-clover pastures. Agric. For. Meteorology 330, 109310. doi:10.1016/j.agrformet.2023.109310
Liaw, A., and Wiener, M. (2002). Classification and regression by randomForest. R. News 2, 18–22. Available at: https://CRAN.R-project.org/doc/Rnews/.
Malcolm, B., Cameron, K., Di, H., Edwards, G., and Moir, J. (2014). The effect of four different pasture species compositions on nitrate leaching losses under high N loading. Soil Use Manag. 30, 58–68. doi:10.1111/sum.12101
Mcleod, M. (2020). Nitrogen leaching under grazed lucerne. Lincoln, New Zealand: Manaaki Whenua - Landcare Research. Contract Report LC3701.
Mcnally, S. R., Laughlin, D. C., Rutledge, S., Dodd, M. B., Six, J., and Schipper, L. A. (2015). Root carbon inputs under moderately diverse sward and conventional ryegrass-clover pasture: implications for soil carbon sequestration. Plant Soil 392, 289–299. doi:10.1007/s11104-015-2463-z
Moir, J. L., Cameron, K. C., Di, H. J., and Fertsak, U. (2011). The spatial coverage of dairy cattle urine patches in an intensively grazed pasture system. J. Agric. Sci. 149, 473–485. doi:10.1017/s0021859610001012
Orwin, K. H., Mason, N. W. H., Berthet, E. T., Grelet, G., Mudge, P., and Lavorel, S. (2022). Integrating design and ecological theory to achieve adaptive diverse pastures. Trends Ecol. and Evol. 37, 861–871. doi:10.1016/j.tree.2022.06.006
Peoples, M. B., Brockwell, J., Herridge, D. F., Rochester, I. J., Alves, B. J. R., Urquiaga, S., et al. (2009). The contributions of nitrogen-fixing crop legumes to the productivity of agricultural systems. Symbiosis 48, 1–17. doi:10.1007/bf03179980
Pinxterhuis, J. B., Judson, H. G., Peterson, M. E., Navarrete, S., Minnée, E., Dodd, M. B., et al. (2024). Implementing plantain (Plantago lanceolata) to mitigate the impact of grazing ruminants on nitrogen losses to the environment: a review. Grass and Forage Sci. 79, 144–157. doi:10.1111/gfs.12649
Podolyan, A., Di, H. J., and Cameron, K. C. (2020). Effect of plantain on nitrous oxide emissions and soil nitrification rate in pasture soil under a simulated urine patch in Canterbury, New Zealand. J. Soils Sediments 20, 1468–1479. doi:10.1007/s11368-019-02505-1
R Core Team (2022). R: a language and environment for statistical computing. 4.2.1 ed. Vienna, Austria: R Foundation for Statistical Computing.
Robertson, C., Schipper, L., Pinxterhuis, I., Edwards, J. P., Doole, G., and Romera, Á. (2023). New Zealand dairy farm system solutions that balance reductions in nitrogen leaching with profitability – a case study. N. Z. J. Agric. Res., 1–21. doi:10.1080/00288233.2023.2269136
Sanderson, M. A., Skinner, R. H., Barker, D. J., Edwards, G. R., Tracy, B. F., and Wedin, D. A. (2004). Plant species diversity and management of temperate forage and grazing land ecosystems. Crop Sci. 44, 1132–1144. doi:10.2135/cropsci2004.1132
Tilman, D., Wedin, D., and Knops, J. (1996). Productivity and sustainability influenced by biodiversity in grassland ecosystems. Nature 379, 718–720. doi:10.1038/379718a0
Vibart, R. E., Vogeler, I., Dodd, M., and Koolaard, J. (2016). Simple versus diverse temperate pastures: aspects of soil–plant–animal interrelationships central to nitrogen leaching losses. Agron. J. 108, 2174–2188. doi:10.2134/agronj2016.04.0193
Keywords: nitrogen leaching, soil hydrology, mitigation, grassland, grazing, plant diversity, Plantago lanceolata
Citation: Graham SL, Pronger J, Laubach J, Hunt JE, Rogers GND, Carrick S, Whitehead D, McLeod M, Mitchell G and Mudge P (2024) Assessing the potential of diverse pastures for reducing nitrogen leaching. Front. Environ. Sci. 12:1445212. doi: 10.3389/fenvs.2024.1445212
Received: 06 June 2024; Accepted: 04 September 2024;
Published: 24 September 2024.
Edited by:
Yefang Jiang, Agriculture and Agri-Food Canada (AAFC), CanadaReviewed by:
Vicki Burgggraaf, AgResearch Ltd., New ZealandSerban Danielescu, Environment and Climate Change Canada (ECCC), Canada
Copyright © 2024 Graham, Pronger, Laubach, Hunt, Rogers, Carrick, Whitehead, McLeod, Mitchell and Mudge. This is an open-access article distributed under the terms of the Creative Commons Attribution License (CC BY). The use, distribution or reproduction in other forums is permitted, provided the original author(s) and the copyright owner(s) are credited and that the original publication in this journal is cited, in accordance with accepted academic practice. No use, distribution or reproduction is permitted which does not comply with these terms.
*Correspondence: Scott L. Graham, grahams@landcareresearch.co.nz