- School of Earth and Sustainability, Northern Arizona University, Flagstaff, AZ, United States
The Colorado River is a vital water source for the western United States, yet the river is governed by disjointed and outdated policies that have left water management fragmented and water quantities overallocated. Groundwater is an overlooked component of Colorado River Basin (CRB) water supplies, making it vulnerable to overuse from disparities in uncoordinated protective management strategies. In this study, we analyzed state level groundwater policies to reveal the diversity and efficacy of groundwater governance mechanisms. The existing groundwater management plans for each state throughout the basin are fragmented and limited in scope. We found that with policies only covering 22% of the basin, they do not provide adequate protection at the basin scale for the sustainable use of groundwater resources in the face of increasing demands, creating a positive feedback loop that reinforces the scarcity issue. We conclude that a comprehensive management plan that can fully address resource use throughout the CRB is necessary for the sustainable use of groundwater and its contribution to base flow in the Colorado River. We suggest that such a plan could be derived through an interstate compact like the Colorado River Compact that is used for surface water management.
1 Introduction
The Colorado River is the seventh largest river in the United States based on its 2,334-km length and 630,000 square kilometer area stretching across seven United States and two Mexican states, making it a transboundary and transnational river (Kammerer, 1990; Figure 1). Annually, the Colorado River discharge ranges between 5.4 km3 and 29.6 km3, with an annual average of 16.7 km3 (Best, 2019; Christensen and Lettenmaier, 2007; Gelt, 1997a). Today, this flow supports over 50 million people, and by 2030, that population is expected to increase by 23 million (Gleick, 2010; Richter et al., 2020).
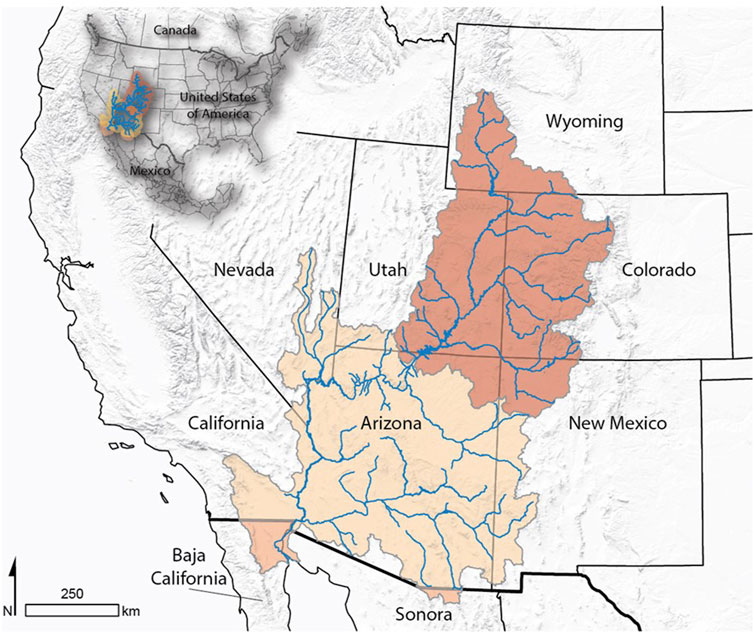
Figure 1. The Colorado River is divided into the Upper Basin (red) and Lower Basin (tan), and Mexico’s portion (orange).
The Fourth National Climate Assessment suggests the region is likely to become drier and experience more severe droughts than those already observed. If current flow of the Colorado River is not sufficient to meet demand, then drying climate will complicate the strain on the river water supply further. The Southwest is in a period of aridification (Overpeck and Udall, 2020). Trend analyses of springs in the Grand Canyon document the decline of base flow since the 1990s (Burch, 2021) and how aridification in the aquifers adjacent to the Grand Canyon has had a larger impact on aquifer storage than groundwater pumping (Nuyttens, 2022). The increasing global temperatures that reduce spring snowpack and soil moisture content are consequently lowering river discharge (Cayan et al., 2010; Lukas and Payton, 2020). In the Colorado River, these hydrologic changes are decreasing the annual mean discharge by an estimated 9.3% for every degree Celsius of atmospheric warming (Milly and Dunne, 2020).
Groundwater throughout the Colorado River Basin (CRB) is also at risk, with a total loss of 50.1 km3 of storage from 2004–2013, whereas the surface water loss was only 14.7 km3 (Castle et al., 2014). This trend is forecasted to continue with increasing rates of water storage decline (Castle et al., 2014; Rahaman et al., 2019). These kinds of water loss trends merit attention, especially groundwater as it has experienced the most decline. Groundwater storage decline has direct and proportional effects on groundwater discharge, or base flow, to rivers (Brutsaert, 2008). Groundwater storage is a shared resource–its decline has both local and basin scale effects. Recharge rates for the local aquifers are often very slow and do not show the signs of overuse immediately (Meixner et al., 2016; Swanson et al., 2020; Tillman et al., 2016). The base-flow response to the decline in groundwater storage has not been observed in the Grand Canyon region, but the effects could be delayed and unprecedented (Swanson et al., 2020), especially with a shift from snow-dominated to rain-dominated recharge processes (Donovan et al., 2023). Miller et al. (2021) projected that the base-flow contribution in the Upper Colorado River Basin could decline by up to 33% by the 2080s. The base flow is contributed from two principal aquifer systems in the CRB; the Colorado Plateau Aquifers that generally consist of water bearing permeable sedimentary rock units in the Colorado Plateaus Physiographic Province, and the Basin and Range Aquifers that consist of water-bearing alluvium filled basins separated by mountain ranges (Miller, 1999; Figure 2).
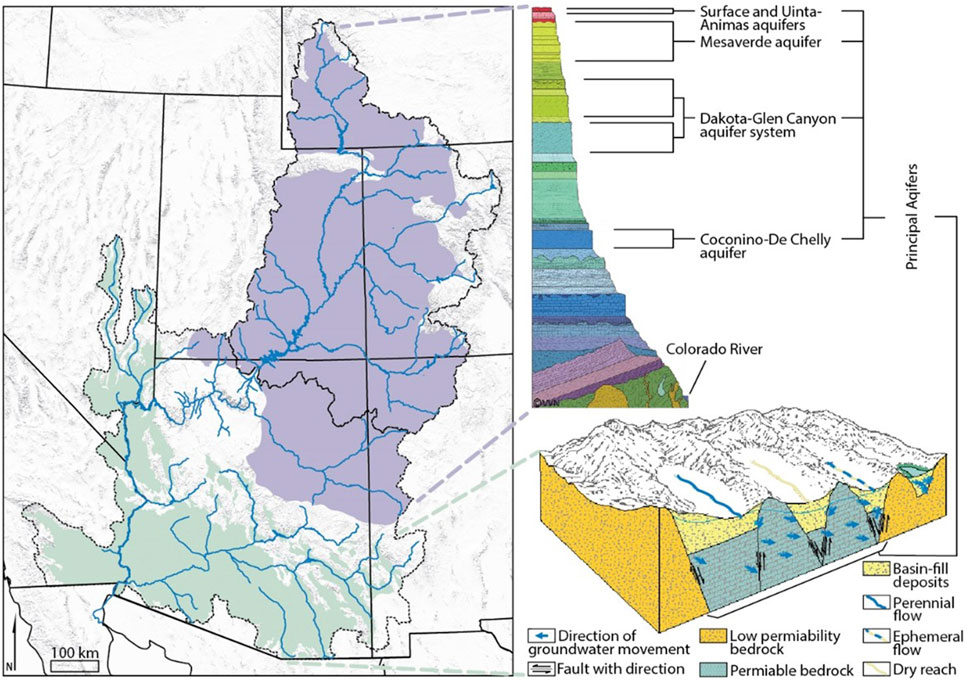
Figure 2. Extent of the principal aquifer systems within the Colorado River Basin including the Colorado Plateau Aquifers (purple) and the Basin and Range Aquifers (green) (Modified from Artiola and Uhlman, 2009 and Miller, 1999).
The rights to the limited water supply in the CRB have long been a contentious issue. In the early 1920s, tensions between the basin states grew in response to fears over the rapid population growth in California that gave the state disproportionate priority rights to the water compared to the slower-growing upriver states (Gelt, 1997a). Confirming these fears, the United States Supreme Court ruled that the Doctrine of Prior Appropriation applied regardless of state lines. Prior Appropriation water law, known colloquially as the “First in Time, First in Right” law, is the doctrine that dominates water allocation in the CRB. This doctrine gives the senior water right to the first user who puts the water to a beneficial use, giving that user priority over all subsequent rights holders. These rights for the quantity of water that was initially put to use remain with the rights holder regardless of location in the basin.
In 1922, the basin states along with the federal government, developed an agreement to share water resources, known as the Colorado River Compact. This compact determined the allocation of Colorado River water and divided the basin into two parts: the Upper Basin and the Lower Basin (USBR, 1922; USBR, 2012). The Upper Basin comprises Arizona (AZ), Colorado (CO), New Mexico (NM), Utah (UT), and Wyoming (WY); and the Lower Basin with Arizona (AZ), California (CA), and Nevada (NV) (Figure 1). This Compact is the most central piece of legislation for the CRB. Following this law, a series of interjurisdictional compacts, acts, treaties, and other resource management policies from the international, federal, state, Tribal, and private level have been devised to contend with allocation challenges forming the “Law of the River” (Davis, 2001).
However, the Colorado River Compact naively overallocated the water based on hydrologic data from abnormally wet years that were producing annual flows 3.7 km3 higher than average at Lees Ferry (Castle et al., 2014; Kuhn and Fleck, 2019; Wang et al., 2020). Lees Ferry marks the divide between the Upper and Lower Colorado River basin and has the longest-term record of flow that was used for developing the Colorado River Compact. The initial allocation of 9.3 km3 given to both the Upper and Lower basins was made despite warnings from researchers who found evidence of average conditions well below the then current, above average flows. This decision was made to help the political goals of early policymakers to develop the Lower Basin states (USBR, 1922; Kuhn and Fleck, 2019). Then in 1944 Mexico was allocated water rights as part of a United States-Mexico Treaty (Gelt, 1997b).
Complicating the overallocation of the river, federally recognized Tribes within the basin have secured Federal Reserved Water Rights to an estimated 3.6 km3 since the passage of the Colorado River Compact and continue seeking their rightful allocations through ongoing state adjudications, pushing the states closer to using their full allocated amounts (Anderson, 2015; CRS, 2019; Ellis and Perry, 2020; USBR, 2018). Over time, the clear overallocation of water resources has engendered a need for ongoing negotiations and policy changes from the original Colorado River Compact of 1922, such as the re-consultation process for reservoir operations after 2026 when current management guidelines are due to expire. Moreover, of these 30 Tribes, some are being granted the development of groundwater resources as part of their water rights adjudication settlements with the states containing their reservations (WTI, 2021).
Similar groundwater shortages and associated management problems that have occurred in the High Plains of the United States and in South America can be used as comparative cases. In the United States, the Ogallala Aquifer is a highly used shared water resource for the states of Colorado, Kansas, Oklahoma, Nebraska, New Mexico, Texas, South Dakota, and Wyoming (Frankel, 2018). To address shortages, this aquifer has received a comprehensive set of groundwater conservation practices from state and federal cooperation with the Ogallala Aquifer Initiative (OAI; USDA, 2017). In South America, Argentina, Brazil, Paraguay, and Uruguay share the Guarani Aquifer System, a transboundary groundwater resource with shared monitoring and management that receives both national and global support (Hussein, 2018; Walter, 2015). Santarosa et al. (2022) show a focused study of the base flow within the Guarani Aquifer System that spans two water resource management units in Sao Paulo state (Brazil). They advocate for adaptive management strategies at the local level due to the high fluctuations in the base-flow contributions ranging from 40%–75%. The base-flow conditions seen in these sub-basins studied in Brazil are comparable to locations in the CRB with similar varied aquifer conditions and human uses. The management of the CRB aquifers, however, does not include basin-wide protections. The CRB policies remain fragmented and interstate agreements remain focused on surface water supplies.
With both groundwater and surface water storage declining in the CRB, shortages are occurring. The declines are most visible in the notably low surface water levels of the Lake Mead and Lake Powell reservoirs as well as in the ground subsidence and fissures from groundwater mining in the Lower Basin (Annin, 2019; Castle et al., 2014; Davis, 2017; Morelle, 2016). State populations are continuing to grow rapidly and are still basing their water resource outlook on the outdated and inaccurate allocations from the original Colorado River Compact (Kuhn and Fleck, 2019). To mitigate some of these shortages, the Colorado River Drought Contingency Plan (DCP) was initiated in 2019. The DCP was designed to address some of this surface water usage in shortage times by cutting allocated surface water rights to the Lower Basin states in set tiers based on designated surface elevation of water levels of Lake Mead (Table 1). In 2022 and 2023, Lake Mead water elevations dropped significantly low, triggering a Tier 2a shortage for 2023 due to the lake levels being below 1,050 feet (USDOI, 2022; USBR, 2024). Lake Mead’s water levels in 2024 allowed for the operating level to return to a Tier 1 shortage (USBR, 2024). This plan, however, neglects major aspects of the CRB hydrologic cycle (including groundwater) by focusing only on visible surface water storage levels of a single reservoir (Lake Mead). The management strategy for the entire basin is based solely on the artificially controlled level of Lake Mead (USBR, 2012). Additionally, this lake level is used to manage the entire CRB regardless of other ongoing hydrologic conditions.
Surface water remains the focus for basin-wide initiatives, despite recent studies throughout the basin showing groundwater declines to be 75% of the basin loss and the base flow of the Colorado River to be 56% (Castle et al., 2014; Christensen and Lettenmaier, 2007; Maupin et al., 2018; Miller et al., 2016; Rahaman et al., 2019; Tague and Grant 2009). Due to its “occult” hard to know nature, groundwater was historically governed separately from surface water (Dellapenna, 2009; Mace et al., 2004; Mace, 2016). Notwithstanding scientific advances since the late nineteenth century, groundwater is still all too often seen as an invisible resource that will never be understood (Alley and Alley, 2017; Mace et al., 2004). Hydrologic studies are still caught in the old paradigm that catchments function like “Teflon basins” where surface water is the most important factor, receiving no influence from geologic and biologic materials, soils processes, or groundwater flow (Clow et al., 2003; Williams et al., 1993). For instance, Rosenberg et al. (2013) and Xiao et al. (2018) continue with the “Teflon basins” concept in the CRB, ruling out the importance of groundwater and assuming the effects are negligible. This incongruence between current science and literature signals the need for further studies on groundwater and surface water interaction in the CRB. Moreover, it highlights the potential for misguided studies to influence policy in Colorado River governance. Groundwater is often viewed as a buffer for water supply in drought conditions even when it is adversely affected and needs to be managed conjunctively with the drier conditions (Langridge and Daniels, 2017; Petersen-Perlman et al., 2022). Environmental flow regimes for both surface water and groundwater must also be considered in CRB management to address all ecological aspects, including maintaining all aspects of water flow and quality at high enough standards to sustain the freshwater and estuarine ecosystems, as well as humans and their wellbeing (Acreman, 2016; Bair et al., 2019; de Graaf et al., 2019; Kreamer et al., 2015; Mott LaCroix et al., 2016; Nelson, 2022).
As states attempt to meet continued water resource demands with allocation reductions of surface water via the DCP, it is likely that states will utilize more groundwater. Groundwater will become collateral damage with increasing withdrawals used as a stopgap, ultimately leading to overuse of the resource (Swanson et al., 2020; USDOI, 2019). The absence of a basin-wide policy to govern groundwater enables such exploitation. Unlike basin surface water controlled by an interstate compact that is congressionally funded and monitored with high priority by the United States Bureau of Reclamation (USBR), groundwater is left to the state and/or local level for management, often with little consideration or funding. The lack of attention to groundwater resources as a major contributor to base flow in the Colorado River and its tributaries creates a huge disconnect in water policy for a crucial, interconnected resource. The variation in management of surface water compared to groundwater complicates the ability to sustainably govern these interconnected resources. Against this backdrop, we ask: how effective are state policies at sustainably governing groundwater in the CRB?
2 Methods
To understand the scope of state groundwater governance, we analyzed extant policies in the seven United States basin states. We then summarized the ways that each state allocates groundwater resources, the year the first groundwater policies were enacted, the year of the most recent policy update, the groundwater well permitting agency, the major governing entity, and the areas of protection for each state. These differences in policies were then examined spatially to see the extent of governance across the CRB.
3 Results
Every CRB state has a unique approach to the allocation and management of groundwater rights. Table 2 provides a summary for each state based on the groundwater allocation policy type, when the first and most recent groundwater policy was enacted, whether groundwater is managed in conjunction with surface water, what agency permits well development, the entity governing groundwater regulations, and the areas that have higher protection management in place.
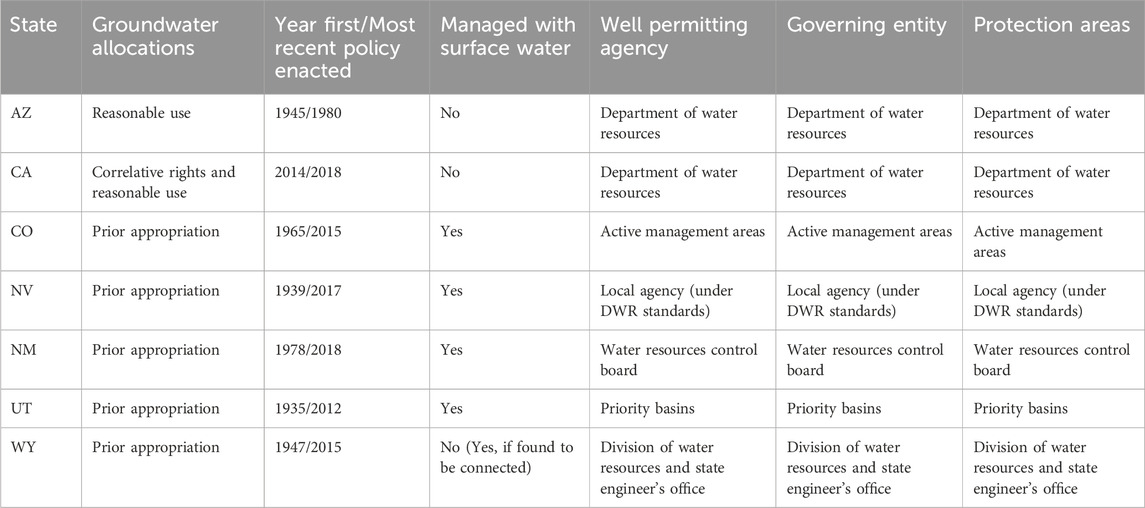
Table 2. A summary for each state based on the groundwater allocation policy type, when the first and most recent groundwater policy was enacted, whether groundwater is managed in conjunction with surface water, what agency permits well development, the entity governing groundwater regulations, and the areas that have higher protection management in place.
Groundwater allocation in the CRB falls into three main policy categories, Prior Appropriation, Correlative Rights, and Reasonable Use. Prior Appropriation allocates water to the first user who puts the resource to beneficial use. This user has priority over all subsequent rights holders, for the quantity that was initially put to use. Prior Appropriation is the dominant policy governing allocation of groundwater in the CRB states with five of the seven states following this doctrine (Table 2). The upper basin states are completely governed by Prior Appropriation (excluding Arizona’s upper basin rights), while the lower basin states have a more diverse policy base of Correlative Rights and Reasonable Use (Figure 3A). Correlative Rights allocates water based on the parcel size.
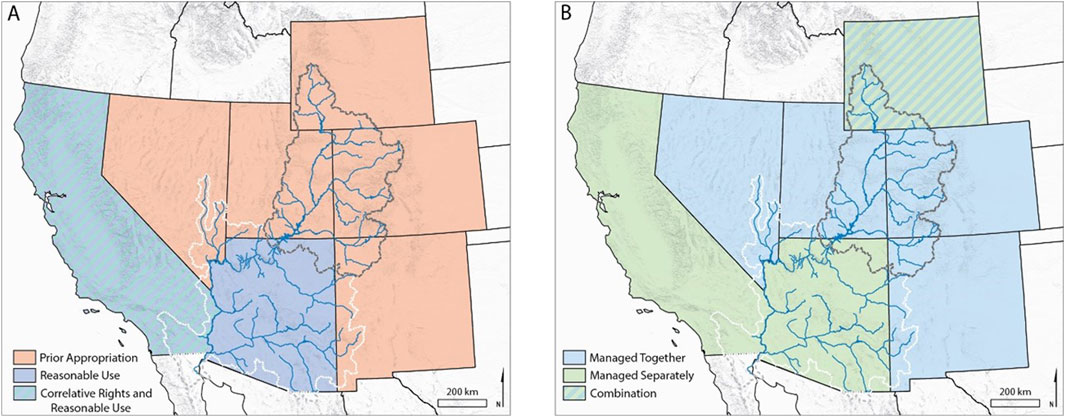
Figure 3. (A) Colorado River Basin states showing the governing policy of groundwater and (B) management of groundwater and surface water connection.
The landowner can use the groundwater from the underlying aquifer equitably based on the amount of land owned. The amount of water allocated with this doctrine also requires the water pumped to be limited to reasonable use, rather than an unlimited supply. Reasonable Use, also known as the American Rule, allocates water to the user as long as the use is reasonably utilized. Arguably a subjective term, reasonable use thus falls into a very broad definition that allows almost any use of water. However, there are restrictions on wasteful use and exportation of water from the location of extraction.
Some states started management plans as early as the 1930s and ‘40s (e.g.: Arizona - 1945, Utah - 1935, and Wyoming - 1947), however, these plans were limited in scope, focusing only on legally defining groundwater and developing registration systems (Bryner and Purcell, 2003). It was not until 2014 that all the CRB states had implemented the newest versions of their management plans that made improvements to managing and restricting the use of groundwater (Table 2).
Despite improvements, these states still lack an integrated approach to water governance that includes the connection of groundwater and surface water. Currently, there is a split in whether states manage surface and groundwater separately or together. Two states manage them separately, four together, and Wyoming separates them unless the resources are found to be connected. This split, however, again aligns generally between the upper and lower basin division (Figure 3B). The upper basin tends to manage the resource together, while the lower basin separates surface and groundwater management. This interaction between groundwater and surface water cannot be ignored in the management of the CRB and the allocations of water. Groundwater’s substantial contribution to Colorado River flow needs to be included in a basin-wide management plan.
States’ regulations on well permitting are mostly the responsibility of the State Engineer or the state Division of Water Resources (Table 2). These agencies oversee issuing well permits and maintaining groundwater records. However, when it comes to the governing entity of groundwater, the states differ from the permitting agency. The differences are seen with half of the states, where either a different state office was involved, or the court system was involved in governing the resource. The existence of similar well permitting (five of the seven states permit from the state engineer’s office) and the governance of the resource (four of the seven states are based in the Department of Water Resources), show potential for a cooperative interstate agreement.
The total area of protection is limited within the CRB states with only 139,000 square kilometers or 22% of the CRB covered. The actual areas of protection are titled differently, but are generally small, highlighted areas of use for each state. Arizona has five Active Management Areas (AMA) and three Irrigation Non-Expansion Areas (INA) all except for one INA are within the CRB. AMAs are the strictest management for Arizona, with each area having different management focused to meet the area’s individual goals (ADWR, 2022). INAs are less strictly managed areas with policies to slow the expanse of groundwater irrigated cropland (ADWR, 2022). The AMAs and INAs total 38,100 km2. California has 515 Priority Basins with 48 of them within the CRB totaling 17,613 km2. California’s Sustainable Groundwater Management Act classifies these basins into high, medium, low, or very low priority levels to determine the level of management for each individual basin (CADWR, 2014). Of the priority basins within the CRB, three are at the medium and one is at the high and critically overdrafted prioritization level (CADWR, 2022). Colorado has eight Designated Basins, but none of them fall within the CRB. These basins are on the eastern plains of Colorado and are defined based on the extent of disconnect from surface water sources and receive stricter management (CODWR, 2022). Nevada also utilizes Designated Basins with 256 defined hydrographic regions that can be designated by the State Engineer for heightened management at different levels including denying irrigation, limiting pumping to preferred users, or general heightened advisory (NVDWR, 2022a). The CRB within Nevada contains 27 hydrographic regions with 16 Designated Basins, two with irrigation denied (one partial), seven with preferred use and irrigation denied, two with preferred use (one partial), and five at the general level (NVDWR, 2022a; 2022b). The protected area of these basins totals 23,143 km2. New Mexico has 39 Declared Basins covering the entire state and 7 are within the CRB totaling 60,143 km2 (NMOSE, 2022). Each of these basins is under the jurisdiction of the State Engineer to manage the source based on the needs of each basin (NMOSE, 2022). Utah uses Groundwater Management Plan areas to incite stricter groundwater management and has implemented 18 plans, none of which fall into the CRB (UDWR, 2022). These areas are under the control of the State Engineer to limit the groundwater withdrawals to a safe yield (USL, 2022). Wyoming has three Groundwater Control Areas, all that fall outside the CRB. These areas add a Groundwater Control Area Advisory Board to help the State Engineer to create stricter policies for the specific area (WSEO, 2022).
4 Discussion
4.1 Patchwork of policies
Because the governance of water rights is largely devolved to states in the United States federal system (CRS, 2019), the CRB is left with a patchwork of policies lacking interchangeable management capacity across borders. Without a central governance structure, states have created a wide variety of policies to deal with their individual groundwater losses (Nelson and Perrone, 2016). However, states have only implemented sustainable management policies recently, once large shortages had already developed (Molle et al., 2018; Rahaman et al., 2019). State policies currently only address the areas of highest concern, not the impacts for the entire basin and the Colorado River. The steps taken to mitigate the overuse of groundwater resources are small in the overall scope of the drainage basin. Molle et al. (2018) found that uncoordinated management solutions at the state level rarely work, as the governing entity does not have the jurisdiction to address the entire issue. The changes needed to fix the issue, at a scale like the CRB, must come from the federal level or an interstate agreement (Warner et al., 2014). None of the states individually have the power to govern the greater CRB area.
There are examples of this kind of federal and interstate cooperation working toward sustainable management. The Ogallala aquifer is a direct comparison case of groundwater management with beneficial intervention from the federal level in an interstate agreement. The Ogallala Aquifer Initiative (OAI) is an agreement between eight states where federal aid supports local management efforts for the greater benefit of the aquifer (USDA, 2017). The OAI has the jurisdiction to lay out guidelines at the interstate scale, creating a more efficient management system. However, the OAI is also flexible enough to maintain local control where adequate management is in place. This is seen in one of the states where conservation of the aquifer is left to the state’s farmers who can voluntarily follow guidelines of Local Enhanced Management Areas (LEMA), a program where the farmers can create and utilize their own conservation plans for the aquifer once approved by the state (Frankel, 2018). Federal influence is also seen in the management of groundwater for allocation to Tribes and reservations (CRS, 2019). Lessons can be learned from the federal allocation of water rights to Tribes, as finalizing the water rights may reduce conflict and promote sustainable management of the resource (Anderson, 2015; Womble et al., 2018). On a smaller scale, California has adopted a new groundwater management plan to promote more sustainable groundwater use that allows management to remain at the local level with periodic reporting to the state (Milman et al., 2018). The success has not been evaluated because management plans were not due until 2020–2022 and the agencies in charge have until 2040 to achieve sustainability (CADWR, 2014).
The existing assortment of policies in the CRB remain surface water focused. Mandated surface water cuts have already begun in the Lower Basin with the designated shortages of the DCP. Arizona and Nevada curtailed their annual Colorado River Apportionment by 592,000 acre-feet (0.73 km3) and 25,000 acre-feet (0.03 km3), respectively, as well as Mexico curtailing its allotment by 104,000 acre-feet (0.13 km3) due to the projected elevation of Lake Mead falling to a record low below 1,050 ft (320 m) and triggering the Tier 2a shortage level of the DCP (USDOI, 2019; USBR, 2022; Table 1). Without a change in demand, these cuts will push people to find water from different sources, resulting in many people turning to groundwater (Brown et al., 2019; Hughes et al., 2012; Womble et al., 2018). Newer surface water policies and the resulting restrictions have developed faster than the corresponding groundwater policies, as was seen with the addition of the DCP for water shortages. The DCP focuses on maintaining the water supply in Lake Mead for times of shortage but does not address groundwater. This asymmetrical management of water sources has created a disconnect in protection. With surface water prioritized in management, groundwater is less protected and therefore at risk. Turning to groundwater to supplement the water that was lost due to surface supply cuts mandated by the DCP will only exacerbate the problem. It has been shown that increases in the water supply alone are often incongruent with environmental needs and inadequate for mitigating shortages, and thus, demand-based changes are needed as well (Perry and Praskievicz, 2017). The changes in sources of water due to drying and warming climate, including decreases in snowpack, are not included in planning or policies such as the DCP.
The most restrictive policies within the basin are only found in the specific protection areas where overuse of groundwater resources is the highest. All the states have implemented a version of these protection areas that are designed to stop the overuse of groundwater withdrawals and return the aquifers to a balance of use and natural recharge. These protection areas, however, are only created once the problem already exists. Little is done to prevent the overuse of the resource, and heavy use is often continued, as seen with some basin states developing management strategies of individual groundwater basins that include the planned exhaustion of the aquifer but at a “controlled” rate. This type of management is explicitly choosing to not protect the resource, even when the problem is well known. Human consumption is still exceeding any natural balance of recharge to the aquifer. Furthermore, each state only has a limited number of protected areas in their groundwater management plans, and the areas themselves are very small scale. For instance, Arizona, the state almost entirely within the CRB boundary, has five AMAs. These management areas only account for around 38,100 km2 or 13% of the state of Arizona but contain 82% of the population and covers 50% of the total water use (Jacobs and Megdal, 2005). The protections of each area only have control over small areas, leaving the rest of the state and drainage basin without the additional regulations.
The basin-wide groundwater declines are not addressed in any of the states’ groundwater policies. Without a plan that can extend beyond the small protection areas of concern, the declining trends will continue. Groundwater storage changes and groundwater discharge also typically have a delayed response compared to surface water declines. With the CRB groundwater resources already greatly tapped, there may be some unseen and unprecedented effects already in progress, such as a delayed decline in base flow once groundwater storage is greatly compromised. Swanson et al. (2020) estimated a 0.5 km3 per year loss from the Grand Canyon region alone, a total close to the Lower Basin DCP water cut from reaching the Tier 1 shortage point at the 1,075 ft (327.66 m) water level of Lake Mead, determined by the Colorado River Compact. Making the problem worse is the continued population growth in the CRB and the changing climate. Population growth cannot be supported by current water resources in the basin with the current management system. Shortages are already present with both surface and groundwater; with a growing population and drying climate, the outlook for the resource is becoming bleaker (Cayan et al., 2010; Lukas and Payton, 2020; USGCRP, 2018).
4.2 Connection of groundwater and surface water
There is a large misconception that the Colorado River flow is dominantly surface water derived, when most of the water source is coming from groundwater (Miller et al., 2016; Swanson et al., 2020). Groundwater is generally still conceptualized as an additional water source to the states and the general public, rather than the interconnected extension of surface water. Water needs to be viewed as a single supply for better management (De Stefano et al., 2018; Sugg et al., 2016). The loss of groundwater storage negatively affects the amount of base-flow (groundwater) contribution to the total Colorado River discharge. Rahaman et al. (2019) determined that 85% of the basin displayed a decreasing trend in groundwater storage from 2002–2016. Brutsaert (2008) showed that there is a strong connection between groundwater storage and base-flow discharge to a stream; the loss of groundwater storage will decrease the amount of surface water flow, reducing the surface water resource.
Some instances of local and sub-regional projects aimed at actively managing groundwater for conservation purposes do exist such as the Coachella Valley Groundwater Basin (CVWD, 2024) and those managed by the Arizona Water Banking Authority (AWBA, 2024). Nonetheless, the connected contribution of groundwater to surface water is not reflected in coordinated policies governing water resources at the state level in the CRB. Rather, new policies at this scale are solely focused on one part of the issue, surface water storage conservation, as seen with the DCP.
The DCP agreement is designed to delay inevitable shortages by declaring water supply cuts triggered with a drop in predetermined lake levels in Lake Mead at the start of the year (USDOI, 2019). When each of the predetermined lake levels are reached, more and more cutbacks of surface water supply from the Colorado River are declared (Table 1). However, without a change in the demand for water, states will ultimately turn to groundwater supply to supplement the cuts seen in the surface water supply (Brown et al., 2019; Perry and Praskievicz, 2017). Pumping groundwater will increase to offset shortages in surface water supplies, causing even more groundwater loss. This decrease in groundwater will proportionally reduce the base-flow contribution to the Colorado River. The reduction of surface flows will then decrease the amount of water in Lake Mead, prompting more cutbacks in Colorado River water allocation. This creates a positive feedback loop that directly affects millions of people using the Colorado River as a resource (Figure 4). The emergence of a feedback loop highlights the need for policies and regulations to consider management of groundwater along with surface water in the CRB.
Acknowledging the connection in surface and groundwater resources is a major step in addressing the foundational issue of overallocation rooted in the original Colorado River Compact that allocates the entirety of the river as long as it “shall be beneficially applied” (USBR, 1922, page 1). The vague wording of the original documentation accentuates the need for basin-wide policies that address the structural deficit (Kuhn and Fleck, 2019). Without a change in policy, groundwater pumping effects are likely to increase with the DCP, as it does not address the structural deficit of the system (Brown et al., 2019; Hughes et al., 2012; Womble et al., 2018).
Water rights are often at the forefront of people’s attention in the West, however, water is often fought over as individual sources such as lake levels and depth to water in a well, rather than at the hydrologic cycle level with all aspects connected. The interconnectedness of the resources suggests the need for integrated policies governing all sources of water and including environmental flow regimes and upland forests. Principally, the essential connection between groundwater and surface water of the Colorado River needs to be recognized. Land use and groundwater pumping has a direct negative impact on the adjacent surface water, yet current regulations do little to manage these aspects of the hydrologic cycle. Current governance lacks sufficient, basin encompassing regulation aimed at reducing overuse, rather than proactively stopping and preventing unsustainable use before it occurs.
The issues observed in the basin, from decreasing surface runoff to rapidly declining groundwater levels, need to be addressed. These issues are occurring with the present number of people currently supported by the Colorado River. With the rapidly growing population and a changing climate, there is an imminent need for improvement in the inadequate policies and management that have resulted in the shortages of water resources in the CRB. These changes need to include an adjustment for water availability based on a warming and drying climate that has less snow available for recharge and base flow.
The current CRB policies are not conducive to proper management. The lack of basin-wide groundwater management is a huge oversight and has forced the CRB states to create a patchwork of independent policies to try and address the underlying issues, which are not efficient for managing the basin-wide shortages. The development of a Colorado River Groundwater Compact is needed. Management should mirror the existing infrastructure that is used for surface water in the Colorado River Compact of 1922. This interstate compact has successfully brought together the basin states in surface water management, allowing for negotiation and planning of a shared fugitive resource. There is still time to develop a new coordinated groundwater basin management plan during the re-consultation process and implement it in 2027 after the current management guidelines expire.
An interstate groundwater compact is possible; federal guidance has already occurred in the management of interstate and international groundwater resources. The allocation and management of Tribal water rights in the United States has seen federal influence that interacts positively with states. The federal influence in finalizing the water rights can reduce conflict and promote sustainable management of the resource. Management of the Ogallala aquifer incorporates a comprehensive set of conservation practices from state and federal cooperation (USDA, 2017). This federal aid, with greater jurisdiction, has been utilized to support local efforts and goals for the benefit of the entire system by laying out guidelines at the interstate scale, creating a more efficient management system. Additionally, inclusive management in South America has brought together epistemic communities that have furthered the quality and extent of resource monitoring and cooperation. Applying the lessons learned from other examples like these will help the prospect of successful policy development in the Colorado River Basin.
Data availability statement
The data analyzed in this study is subject to the following licenses/restrictions: Data will be made available upon request. Requests to access these datasets should be directed to Denielle Perry, denielle.perry@nau.edu.
Author contributions
DP: Conceptualization, Formal Analysis, Methodology, Project administration, Supervision, Visualization, Writing–original draft, Writing–review and editing. RS: Conceptualization, Data curation, Formal Analysis, Investigation, Methodology, Project administration, Visualization, Writing–original draft, Writing–review and editing. AS: Conceptualization, Methodology, Supervision, Writing–review and editing.
Funding
The author(s) declare that no financial support was received for the research, authorship, and/or publication of this article.
Acknowledgments
We would like to thank: NAU School of Earth and Sustainability, Springs Stewardship Institute, Grand Canyon Trust, Grand Canyon National Park, Kaibab National Forest, and Grand Staircase Escalante National Monument. We would like to acknowledge Benjamin Tobin for his support throughout this study, Matthew Roderick for his invaluable support in the literature review of this article, Katelyn LaPine for her assistance in geospatial analysis and calculations, and Macie Wagner for her attention to detail in editing and formatting.
Conflict of interest
The authors declare that the research was conducted in the absence of any commercial or financial relationships that could be construed as a potential conflict of interest.
Publisher’s note
All claims expressed in this article are solely those of the authors and do not necessarily represent those of their affiliated organizations, or those of the publisher, the editors and the reviewers. Any product that may be evaluated in this article, or claim that may be made by its manufacturer, is not guaranteed or endorsed by the publisher.
References
ADWR (Arizona Department of Water Resources) (2022). Active management areas. Available at: https://www.azwater.gov/ama/active-management-area-overview (Accessed March 30, 2022).
Acreman, M. (2016). Environmental flows-basics for novices. WIREs Water 3, 622–628. doi:10.1002/wat2.1160
Alley, W. M., and Alley, R. (2017). High and dry: meeting the challenges of the world’s growing dependence on groundwater. Yale University Press.
Anderson, R. T. (2015). Water rights, water quality, and regulatory jurisdiction in Indian country. 34 Stanf. Environ. Law J., 195. Available at: https://digitalcommons.law.uw.edu/faculty-articles/384.
Annin, P. (2019). Tough times along the Colorado river. N. Y. Times. Available at: https://www.nytimes.com/2019/01/30/opinion/tough-times-along-the-colorado-river.html.
Artiola, J., and Uhlman, K. (2009). Arizona well owner’s guide to water supply. The University of Arizona. Available at: https://www.researchgate.net/publication/255685781.
AWBA (Arizona Water Banking Authority) (2024). Water Bank storage facilities. Available at: https://waterbank.az.gov/water-storage/storage-facilities (Accessed July 24, 2024).
Best, A. (2019). Hydraulic empire: sharing a legacy, carving a future for the Colorado river. Land Lines, 14–25.
Brown, T. C., Mahat, V., and Ramirez, J. A. (2019). Adaptation to future water shortages in the United States caused by population growth and climate change. Earth’s Future 7, 219–234. doi:10.1029/2018ef001091
Brutsaert, W. (2008). Long-term groundwater storage trends estimated from streamflow records: climatic perspective. Water Resour. Res. 44, W02409. doi:10.1029/2007WR006518
Bryner, G., and Purcell, E. (2003). Groundwater law Sourcebook of the western United States. Boulder, CO, United States: Natural Resources Law Center University of Colorado School of law.
Burch, S. J. (2021). Assessing fire impacts to a spring-fed riparian ecosystem during a dry climate cycle in Grand Canyon National Park, USA, 136.
CADWR (California Department of Water Resources) (2014). California’s sustainable groundwater management act: recommendations for preventing and resolving groundwater conflicts. Sacramento, CA: California Department of Water Resources.
CADWR (California Department of Water Resources) (2022). Critically overdrafted basins. Available at: thttps://water.ca.gov/programs/groundwater-management/bulletin-118/critically-overdrafted-basins (Accessed March 30, 2022).
Castle, S. L., Thomas, B. F., Reager, J. T., Rodell, M., Swenson, S. C., and Famiglietti, J. S. (2014). Groundwater depletion during drought threatens future water security of the Colorado River Basin. Geophys. Res. Lett. 41, 5904–5911. doi:10.1002/2014GL061055
Cayan, D. R., Das, T., Pierce, D. W., Barnett, T. P., Tyree, M., and Gershunov, A. (2010). Future dryness in the southwest US and the hydrology of the early 21st century drought. Proc. Natl. Acad. Sci. U. S. A. 107 (21), 21271–21276. doi:10.1073/pnas.0912391107
Christensen, N. S., and Lettenmaier, D. P. (2007). A multimodel ensemble approach to assessment of climate change impacts on the hydrology and water resources of the Colorado River basin. Hydrology Earth Syst. Sci. 3, 3727–3770. doi:10.5194/hess-11-1417-2007
Clow, D., Schrott, L., Webb, R., Campbell, D. H., Torizzo, A., and Dornblaser, M. (2003). Ground water occurrence and contributions to streamflow in an alpine catchment, Colorado Front Range. Ground Water 41 (7), 937–950. doi:10.1111/j.1745-6584.2003.tb02436.x
CRS (Congressional Research Service) (2019). Indian water rights settlements. R44148. Available at: https://crsreports.congress.gov (Accessed March 30, 2022).
CVWD (Coachella Valley Water District) (2024). Sustainable groundwater management act. Available at: http://www.cvwd.org/357/Sustainable-Groundwater-Management-Act (Accessed July 24, 2024).
Davis, S. K. (2001). The politics of water scarcity in the western states. Soc. Sci. J. 38 (4), 527–542. doi:10.1016/S0362-3319(01)00148-3
Davis, T. (2017). Record Pinal County fissure shows Arizona is still prone to shifting earth levels, 11 February 2017, Updated 14 February 2018. Ariz. Dly. Star. Available at: https://tucson.com/news/local/record-pinal-county-fissure-shows-arizona-is-still-prone-to/article_7746322e-e4a4-55f7-bdd3-5ff9bed82662.html.
de Graaf, I. M. E., Gleeson, T., van Beek, L. P. H., Sutanudjaja, E. H., and Bierkens, M. F. P. (2019). Environmental flow limits to global groundwater pumping. Nature 574 (4), 90–94. doi:10.5683/SP2/D7I7CC
Dellapenna, J. W. (2009). “United States: the allocation of surface waters,” in The evolution of the law and politics of water. Editors J. W. Dellapenna, and J. Gupta (Netherlands: Springer), 189–204. Available at: http://link.springer.com/chapter/10.1007/978-1-4020-9867-3_12.
De Stefano, L., Welch, C., Urquijo, J., and Garrick, D. E. (2018). Groundwater governance in the rio grande: Co-evolution of local and intergovernmental management. Water Altern. 11 (3), 824–846.
Donovan, K. M., Springer, A. E., Tobin, B. W., and Parnell, R. A. (2023). “Karst spring processes and storage implications in high elevation, semi-arid southwestern United States,” in Threats to springs in a changing world: science and policies for protection, geophysical monograph 275. Editors B. Katz, and M. Currell (Wiley/AGU). doi:10.1002/9781119818625.ch04
Ellis, R., and Perry, D. (2020). A confluence of anticolonial pathways for indigenous sacred site protection. J. Contemp. Water Res. Educ. 169, 8–26. doi:10.1111/j.1936-704x.2020.03329.x
Frankel, J. (2018). Crisis on the high plains: the loss of America’s largest aquifer–the Ogallala. Denver, Colorado: University of Denver Water Law Review. Available at: http://duwaterlawreview.com/crisis-on-the-high-plains-the-loss-of-americas-largest-aquifer-the-ogallala/.
Gelt, J. (1997a). Sharing Colorado River Water: history, public policy and the Colorado River Compact. Working Paper. Tucson, Arizona: Water Resources Research Center, University of Arizona, 12. Arroyo.
Gelt, J. (1997b). Sharing Colorado River Water: history, public policy and the Colorado River Compact, Water Resources Research Center, Tucson, AZ, August 01, 1997. Arroyo 10 (1). Available at: https://wrrc.arizona.edu/publication/sharing-colorado-river-water-history-public-policy-and-colorado-river-compact.
Gleick, P. H. (2010). Roadmap for sustainable water resources in southwestern North America. Proc. Natl. Acad. Sci. U. S. A. 107 (21), 21300–21305. doi:10.1073/pnas.1005473107
Hughes, J. D., Petrone, K. C., and Silberstein, R. P. (2012). Drought, groundwater storage and stream flow decline in southwestern Australia. Geophys. Res. Lett. 39 (3), 6. doi:10.1029/2011GL050797
Hussein, H. (2018). The Guarani Aquifer System, highly present but not high profile: a hydropolitical analysis of transboundary groundwater governance. Environ. Sci. Policy 83, 54–62. doi:10.1016/j.envsci.2018.02.005
Jacobs, K., and Megdal, S. (2005). Water management in the active management areas. Water Resour. Res. Cent. 6, 71–91.
Kammerer, J. C. (1990). “Largest rivers in the United States,” in Water fact sheet (Reston, Virginia: U.S. Geological Survey, Department of the Interior). Available at: https://pubs.usgs.gov/of/1987/ofr87-242/pdf/ofr87242.pdf.
Kreamer, D. K., Stevens, L. E., and Ledbetter, J. D. (2015). “Groundwater dependent ecosystems – policy challenges and technical solutions, groundwater, hydrochemistry, environmental impacts and management impacts,” in Groundwater: hydrochemistry, environmental impacts, and management practices. Chapter 9, Editor A. Segun (New York: Nova Publishers), 205–230.
Kuhn, E., and Fleck, J. (2019). Science Be dammed: how ignoring inconvenient science drained the Colorado river. Tucson, Arizona: University of Arizona Press.
Langridge, R., and Daniels, B. (2017). Accounting for climate change and drought in implementing sustainable groundwater management. Water Resour. Manag. 31, 3287–3298. doi:10.1007/s11269-017-1607-8
Lukas, J., and Payton, E. (2020). Colorado River Basin climate and hydrology: state of the science. Western water assessment (Boulder, CO: University of Colorado). doi:10.25810/3hcv-w477
Mace, R. E. (2016). “So secret, occult, and concealed—an overview of groundwater management in Texas,” in Proceedings of law of the rio grande, water law Institute, CLE international, Sante Fe, NM, April 2016.
Mace, R. E., Ridgeway, C., and Sharp, J. M. (2004). “Groundwater is no longer secret and occult–a historical and hydrogeologic analysis of the east case,” in Proceedings of the 100 Years of Rule of capture: from east to groundwater management, report 361, Austin, TX, 63–88.
Maupin, M. A., Ivahnenko, T., and Bruce, B. (2018). Estimates of water use and trends in the Colorado River Basin, southwestern United States, 1985–2010. U.S. Geol. Surv. Sci. Investig. Rep. 2018 (5049), 61. doi:10.3133/sir20185049
Meixner, T., Manning, A. H., Stonestrom, D. A., Allen, D. M., Ajami, H., Blasch, K. W., et al. (2016). Implications of projected climate change for groundwater recharge in the western United States. J. Hydrology 534, 124–138. doi:10.1016/j.jhydrol.2015.12.027
Miller, J. A. (1999). Ground water atlas of the United States, introduction and national summary. HA-730 (and volumes within the HA-730 series). Reston, Virginia: U.S. Geological Survey. Available at: http://capp.water.usgs.gov/gwa/index.html.
Miller, M. P., Buto, S. G., Susong, D. D., and Rumsey, C. A. (2016). The importance of base flow in sustaining surface water flow in the Upper Colorado River Basin Water Resour. Res. 52, 3547–3562. doi:10.1002/2015WR017963
Miller, O. L., Miller, M. P., Longley, P. C., Alder, J. R., Bearup, L. A., Pruitt, T., et al. (2021). How will baseflow respond to climate change in the Upper Colorado River Basin? Geophys. Res. Lett. 48, e2021GL095085. doi:10.1029/2021GL095085
Milly, P. C. D., and Dunne, K. A. (2020). Colorado River flow dwindles as warming-driven loss of reflective snow energizes evaporation. Science 367 (6483), 1252–1255. doi:10.1126/science.aay9187
Milman, A., Galindo, L., Blomquist, W., and Conrad, E. (2018). Establishment of agencies for local groundwater governance under California’s Sustainable Groundwater Management Act. Water Altern. 11 (3), 458–480.
Molle, F., López-Gunn, E., and van Steenbergen, F. (2018). The local and national politics of groundwater overexploitation. Water Altern. 11 (3), 445–457.
Morelle, R. (2016). Surface water shifting around the Earth. London, United Kingdom: British Broadcasting Corporation. Available at: https://www.bbc.com/news/science-environment-37187100.
Nelson, R. L. (2022). Water rights for groundwater environments as an enabling condition for adaptive water governance. Ecol. Soc. 27 (2), 28. doi:10.5751/ES-13123-270228
Nelson, R. L., and Perrone, D. (2016). Local groundwater withdrawal permitting laws in the south-western U.S.: California in comparative context. Groundwater 54 (6), 747–753. doi:10.1111/gwat.12469
NMOSE (New Mexico Office of the State Engineer) (2022). Declared groundwater basins. Available at: https://www.ose.state.nm.us/WR/groundWater.php.Cited (Accessed March 30, 2022).
Nuyttens, K. (2022). Ecohydrogeology of springs below the Grand canyon’s South rim, Grand Canyon National Park, Arizona. Masters thesis. Santa Fe, New Mexico: Northern Arizona University. Available at: https://openknowledge.nau.edu/id/eprint/5914/ (Accessed March 30, 2022).
NVDWR (Nevada Division of Water Resources) (2022b). State engineer designated groundwater basins of Nevada. Available at: http://water.nv.gov/mapping/maps/designated_basinmap.pdf (Accessed March 30, 2022).
NVDWR (Nevada Division of Water Resources) (2022a). Water law overview. Available at: http://water.nv.gov/waterlaw.aspx (Accessed March 30, 2022).
Overpeck, J., and Udall, B. (2020). Climate change and the aridification of North America. Proc. Natl. Acad. Sci. U. S. A. 117 (22), 11856–11858. doi:10.1073/pnas.2006323117
Perry, D. M., and Praskievicz, S. J. (2017). A new era of big infrastructure? (Re)developing water storage in the U.S. West in the context of climate change and environmental regulation. Water Altern. 10 (2), 437–454.
Petersen-Perlman, J. D., Aguilar-Barajas, I., and Megdal, S. B. (2022). Drought and groundwater Management: interconnections, challenges, and policy responses. Environ. Sci. and Health 28, 100364. doi:10.1016/j.coesh.2022.100364
Rahaman, M. M., Thakur, B., Kalra, A., and Ahmad, S. (2019). Modeling of GRACE-derived groundwater information in the Colorado River Basin. Hydrology 6, 19. doi:10.3390/hydrology6010019
Richter, B. D., Bartak, D., Caldwell, P., Davis, K. F., Debaere, P., Hoekstra, A. Y., et al. (2020). Water scarcity and fish imperilment driven by beef production. Nat. Sustain. 3, 319–328. doi:10.1038/s41893-020-0483-z
Rosenberg, E. A., Clark, E. A., Steinemann, A. C., and Lettenmaier, D. P. (2013). On the contribution of groundwater storage to interannual streamflow anomalies in the Colorado River basin. Hydrology Earth Syst. Sci. 17 (4), 1475–1491. doi:10.5194/hess-17-1475-2013
Santarosa, L. V., Gastmans, D., and Stager Quaggio, C. (2022). Stable isotope modeling of the groundwater discharge in complex watersheds of the state of São Paulo, Brazil. J. South Am. Earth Sci. 120. doi:10.1016/j.jsames.2022.104063
Sugg, Z. P., Ziaja, S., and Schlager, E. C. (2016). Conjunctive groundwater management as a response to socio-ecological disturbances: a comparison of 4 western U.S. states. Tex. Water J. 7 (1), 1–24. doi:10.21423/twj.v7i1.7019
Swanson, R. K., Springer, A. E., Kreamer, D. K., Tobin, B. W., and Perry, D. M. (2020). Quantifying the base flow of the Colorado River: its importance in sustaining perennial flow in northern Arizona and southern Utah (USA). Hydrogeology J. 29, 723–736. doi:10.1007/s10040-020-02260-5
Tague, C., and Grant, G. E. (2009). Groundwater dynamics mediate low-flow response to global warming in snow-dominated alpine regions. Water Resour. Res. 45 (7). doi:10.1029/2008WR007179
Tillman, F. D., Gangopadhyay, S., and Pruitt, T. (2016). Changes in groundwater recharge under projected climate in the Upper Colorado River basin. Geophys. Res. Lett. 43, 6968–6974. doi:10.1002/2016GL069714
UDWR (Utah Division of Water Resources) (2022). Groundwater management plans. Available at: https://waterrights.utah.gov/groundwater/ManagementReports/ground.asp. March 30, 2022).
USBR (U.S. Bureau of Reclamation) (2012). Colorado River Basin water supply and demand study, study report. Washington, DC: U.S. Department of the Interior, 95. Available at: http://www.usbr.gov/lc/region/programs/crbstudy/finalreport/studyrpt.html.
USBR (U.S. Bureau of Reclamation) (2018). Colorado River Basin ten Tribes partnership tribal water study. Available at: https://www.usbr.gov/lc/region/programs/crbstudy/tws/docs/Ch.%201%20Introduction%2012-13-2018.pdf (Accessed March 30, 2022).
USBR (U.S. Bureau of Reclamation) (2022). Interior department announces actions to protect Colorado river system, sets 2023 operating conditions for Lake Powell and Lake Mead. Available at: https://www.usbr.gov/newsroom/#/news-release/4294.
USBR (U.S. Bureau of Reclamation) (2024). Lower Colorado river daily report. Available at: https://www.usbr.gov/lc/region/g4000/hourly/mead-elv.html (Accessed July 24, 2024).
USDA (U.S. Department of Agriculture) (2017). Ogallala aquifer initiative, 2017 progress report. Available at: https://www.nrcs.usda.gov/Internet/FSE_MEDIA/nrcseprd1407817.pdf.
USDOI (U.S. Department of the Interior) (2019). Agreement concerning Colorado River Drought contingency management and operations.
USDOI (U.S. Department of the Interior) (2022). “Interior department announces actions to protect Colorado river system,” in Sets 2023 operating conditions for Lake Powell and Lake Mead.
USGCRP (U.S. Global Change Research Program) (2018). in Impacts, risks, and adaptation in the United States: Fourth national climate assessment. Editors D. R. Reidmiller, C. W. Avery, D. R. Easterling, K. E. Kunkel, K. L. M. Lewis, T. K. Maycocket al. (Washington, DC), 1515. doi:10.7930/NCA4.2018
USL (Utah State Legislature) (2022). 73-5-15. Groundwater management plan. Available at: https://le.utah.gov/xcode/Title73/Chapter5/73-5-S15.html. (. (March 30, 2022).
Walter, M. (2015). The invention of the Guarani aquifer system: new ideas and new water politics in the southern cone. ReVista 14 (3), 23–25.
Wang, J., Rosenberg, D. E., Wheeler, K. G., and Schmidt, J. C. (2020). “Managing the Colorado river for an uncertain future,” in The future of the Colorado river project center for Colorado river studies quinney college of natural resources (Logan, Utah: Utah State University). White Paper No. 3.
Warner, J. F., Wester, P., and Hoogesteger, J. (2014). Struggling with scales: revisiting the boundaries of river basin management. Water 1 (5), 469–481. doi:10.1002/wat2.1035
Water and Tribes Initiative (2021). The status of tribal water rights in the Colorado River Basin. Policy Brief. Available at: https://www.naturalresourcespolicy.org/publications/policy-brief-4-final-4.9.21-.pdf.
Williams, M. W., Brown, A. D., and Melack, J. M. (1993). Geochemical and hydrologic controls on the composition of surface water in a high-elevation basin, Sierra Nevada, California. Limnol. Oceanogr. 38 (4), 775–797. doi:10.4319/lo.1993.38.4.0775
Womble, P., Perrone, D., Jasechko, S., Nelson, R. L., Szeptycki, L. F., Anderson, R. T., et al. (2018). Indigenous communities, groundwater opportunities. Science 361 (6401), 453–455. doi:10.1126/science.aat6041
WSEO (Wyoming State Engineer’s Office) (2022). Groundwater control areas and advisory boards. Available at: https://seo.wyo.gov/ground-water/groundwater-control-areas-and-advisory-boards.Cited March 30, 2022).
Keywords: groundwater governance, interbasin management, Colorado River Compact, base flow, aquifer management
Citation: Perry D, Swanson RK and Springer AE (2024) Policy deficiencies and contingency plans: groundwater management implications for baseflow contributions to the Colorado River. Front. Environ. Sci. 12:1444015. doi: 10.3389/fenvs.2024.1444015
Received: 04 June 2024; Accepted: 16 August 2024;
Published: 30 August 2024.
Edited by:
Martin Thoms, University of New England, AustraliaReviewed by:
Katharine Dahm, U. S. Geological Survey, United StatesThomas Shahady, University of Lynchburg, United States
Copyright © 2024 Perry, Swanson and Springer. This is an open-access article distributed under the terms of the Creative Commons Attribution License (CC BY). The use, distribution or reproduction in other forums is permitted, provided the original author(s) and the copyright owner(s) are credited and that the original publication in this journal is cited, in accordance with accepted academic practice. No use, distribution or reproduction is permitted which does not comply with these terms.
*Correspondence: Denielle Perry, Denielle.Perry@nau.edu