- 1Department of Earth and Environmental Sciences, University of Manchester, Manchester, United Kingdom
- 2Department of Biotechnology, College of Life Science and Biotechnology, Yonsei University, Seoul, Republic of Korea
The management of landfill leachate presents a significant environmental challenge, necessitating a comprehensive and dynamic treatment approach. This comprehensive review delves into the critical issue of landfill leachate treatment, exploring its environmental impact, treatment technologies, regulatory frameworks, and the path towards sustainable management practices. This review explores the complexities of landfill leachate, emphasizing the need for sustainable waste management practices to safeguard environmental health. Our analysis highlights the evolution of conventional and advanced treatment technologies designed to mitigate these risks, focusing on membrane technologies, advanced oxidation processes, and the promising potential of emerging techniques such as adsorption and biological nutrient removal. These technologies are evaluated for their efficiency, cost implications, and sustainability impacts, underscoring the challenges and opportunities within the current landscape of leachate treatment. The review aims to provide insights into designing efficient and effective treatment systems through a detailed analysis of conventional and advanced treatment methods. By examining a case study in Changsha City, the effectiveness of a comprehensive treatment system integrating various technologies is demonstrated. The review underscores the interconnectedness of human activities, environmental health, and waste management, emphasizing the importance of a holistic approach. It stresses the continuous improvement of leachate treatment technologies and the adoption of sustainable practices to reduce the environmental footprint of landfills. Ultimately, it calls for integrating multiple treatment processes, economic considerations, and readiness to address future challenges in landfill leachate treatment, contributing to the advancement of sustainable waste management practices.
1 Introduction
Landfill leachate, a liquid byproduct of the decomposition process in landfills, represents a significant environmental challenge that encapsulates the complexity of modern waste management and the imperative of safeguarding environmental health (Teng et al., 2021). As rainfall or other sources of moisture percolate through the waste material accumulated in landfills, they dissolve and suspend a myriad of substances present in the waste, creating a potent mixture known as leachate (Mor and Ravindra, 2023). This concoction’s composition can vary widely but typically includes dissolved organic matter, inorganic macro-components like salts and metals, and a range of xenobiotic organic compounds, reflecting the diverse nature of disposed materials (Kumar et al., 2023). Organic components, measured in terms of biochemical oxygen demand (BOD) and chemical oxygen demand (COD), indicate the leachate’s potential impact on water bodies, while inorganic macro-components, such as ammonia, chlorides, sulfates, and heavy metals like arsenic, cadmium, chromium, lead, and mercury, underline the toxicological concerns associated with leachate (Al-Yaqout and Hamoda, 2020). Furthermore, the presence of xenobiotic compounds, which include pesticides, pharmaceuticals, and industrial chemicals, adds to the complexity of leachate, given their resistance to degradation and potential for bioaccumulation (de Oliveira et al., 2020). The environmental impacts of uncontrolled landfill leachate are profound and far-reaching. Leachate can seep into groundwater and surface water, contaminating aquatic ecosystems, wildlife, and human health (Essien et al., 2022). The infiltration of pollutants into water supplies can disrupt aquatic habitats, harm species diversity, and bioaccumulate the food chain, presenting significant risks to public health through consuming contaminated water and food (Sharma et al., 2023). Moreover, the heavy metals and persistent organic pollutants found in leachate are particularly concerning due to their toxicity, environmental longevity, and potential to cause long-term ecological damage (Hussein et al., 2021).
Recognizing the environmental hazards of landfill leachate, its treatment and management have become critical components of sustainable waste management practices (El-Saadony et al., 2023). Effective leachate treatment aims to neutralize its harmful components, making it safe for discharge or further use (Kumar et al., 2023). This necessitates a multifaceted approach that combines physical, chemical, and biological treatment processes tailored to the specific composition of the leachate and local regulatory requirements (Torretta et al., 2017). Physical treatments, such as sedimentation and filtration, remove suspended solids and particulate matter (Siddiqi et al., 2022). Chemical treatments, including precipitation, coagulation, and advanced oxidation, target dissolved pollutants, reducing their concentration to safer levels (Kurniawan et al., 2006). Biological aerobic and anaerobic treatments further degrade organic substances, transforming them into less harmful compounds (Kurniawan et al., 2010). The importance of landfill leachate treatment extends beyond the immediate goal of pollution control. It embodies the broader principles of environmental protection and sustainable waste management (Mukherjee et al., 2015). By mitigating the adverse impacts of leachate on ecosystems and human health, effective treatment practices contribute to the preservation of water quality, protect biodiversity, and ensure the wellbeing of communities (Senathirajah and Palanisami, 2023). Furthermore, advancements in leachate treatment technologies offer opportunities for resource recovery, such as the extraction of valuable materials or the reuse of treated water, aligning with the principles of the circular economy and resource efficiency (Puyol et al., 2017).
Treating landfill leachate is not just a technical challenge but a fundamental aspect of environmental stewardship and public health protection (Nath and Debnath, 2022). It reflects the evolving understanding of waste not merely as a byproduct to be disposed of but as a potential source of pollution that must be managed with foresight and responsibility. As such, the continuous improvement of leachate treatment technologies, alongside adopting more sustainable waste management practices, is essential to reducing the environmental footprint of landfills. This includes the development of more efficient and cost-effective treatment solutions and the implementation of waste reduction, reuse, and recycling initiatives that can minimize leachate generation in the first place (Kamaruddin et al., 2017).
Ultimately, the comprehensive management and treatment of landfill leachate highlight the interconnectedness of human activities, environmental health, and the need for a holistic approach to waste management (Torretta et al., 2017). This review aims to provides an integrated analysis of both conventional and advanced treatment technologies, emphasizing recent advancements and emerging techniques such as adsorption and biological nutrient removal. Unlike previous reviews that may focus solely on specific technologies or methodologies, this review evaluates a broad spectrum of treatment methods, offering a practical guidance for designing and optimizing treatment systems by evaluating the efficiency, cost implications, and sustainability impacts of various methods. Additionally, we incorporate a detailed case study from Changsha City, demonstrating the practical application and effectiveness of a comprehensive treatment system that integrates various technologies. This real-world example bridges the gap between theoretical discussions and practical implementation, providing actionable insights for practitioners and policymakers. By addressing the interconnectedness of human activities, environmental health, and waste management, our review advocates for a holistic approach that emphasizes continuous improvement of leachate treatment technologies and the adoption of sustainable practices. This comprehensive perspective makes our review an invaluable resource for engineers, environmental scientists, and waste management professionals seeking to implement effective and sustainable treatment solutions in diverse contexts.
2 Characteristics of landfill leachate
2.1 Physical and chemical properties of landfill leachate
Leachate embodies a dynamic concoction of substances, each contributing to its hazardous nature. Landfill leachate’s physical, chemical, and biological characteristics delineate a complex and multifaceted environmental challenge that requires a nuanced understanding for effective management and treatment (Table 1) (Andreottola and Cannas, 2024; Castrillón et al., 2010; Naveen et al., 2017; Luo et al., 2020; Subiza-Pérez et al., 2023).

Table 1. Characteristics of landfill leachate and implications for treatment (Andreottola and Cannas, 2024; Castrillón et al., 2010; Naveen et al., 2017; Luo et al., 2020; Subiza-Pérez et al., 2023).
Physically, leachate is primarily a liquid matrix. However, its appearance, viscosity, and other physical properties can vary greatly depending on the landfill’s age, the composition of waste, and the amount of precipitation the landfill receives (Anjum et al., 2023). Typically, young leachate is characterized by a high organic load, evident in its dark color and strong odor (Wijekoon et al., 2022). In contrast, older leachate may appear clearer but contain higher concentrations of dissolved inorganic compounds (Abdel-Shafy et al., 2024). The physical properties of leachate, such as pH, temperature, and conductivity, are critical indicators of its overall composition and the potential challenges it presents for treatment (Abdel-Shafy et al., 2024). For instance, high conductivity in leachate signifies a high concentration of dissolved ions such as chlorides, sulfates, and ammonia, which can affect the efficiency of certain treatment processes. Similarly, pH levels can influence the solubility of metals and the effectiveness of chemical treatments, with acidic or alkaline conditions necessitating specific adjustments in the treatment strategy (Deng et al., 2020).
Chemically, leachate is a cocktail of dissolved organic and inorganic matter, heavy metals, and xenobiotic compounds (Sil and Kumar, 2017). The organic fraction is typically dominated by biodegradable substances like carbohydrates, fats, proteins, and persistent organic pollutants that resist natural degradation processes (Dignac et al., 2000). COD and BOD are key parameters used to quantify the organic strength of leachate, reflecting its potential impact on receiving environments and treatment systems (Naveen et al., 2017). Inorganic components include a range of salts, metals, and nutrients, with ammonia being particularly prevalent due to the nitrogenous decomposition of organic waste (Borah et al., 2020). Heavy metals such as arsenic, cadmium, chromium, lead, and mercury are of significant concern due to their toxicity and propensity to accumulate in ecosystems (Rahman and Singh, 2019). Moreover, the presence of xenobiotics—synthetic chemicals like pharmaceuticals, pesticides, and industrial chemicals—poses a unique challenge, as these compounds often exhibit resistance to degradation and can disrupt biological processes in treatment systems and natural environments (Priya et al., 2024). Table 2 presents a detailed analysis of the physical and chemical properties of landfill leachate from various regions around the world, showing significant variability across different regions (Abunama et al., 2021a). These variations highlight the importance of regional assessments in understanding leachate pollution potential and tailoring appropriate management strategies.
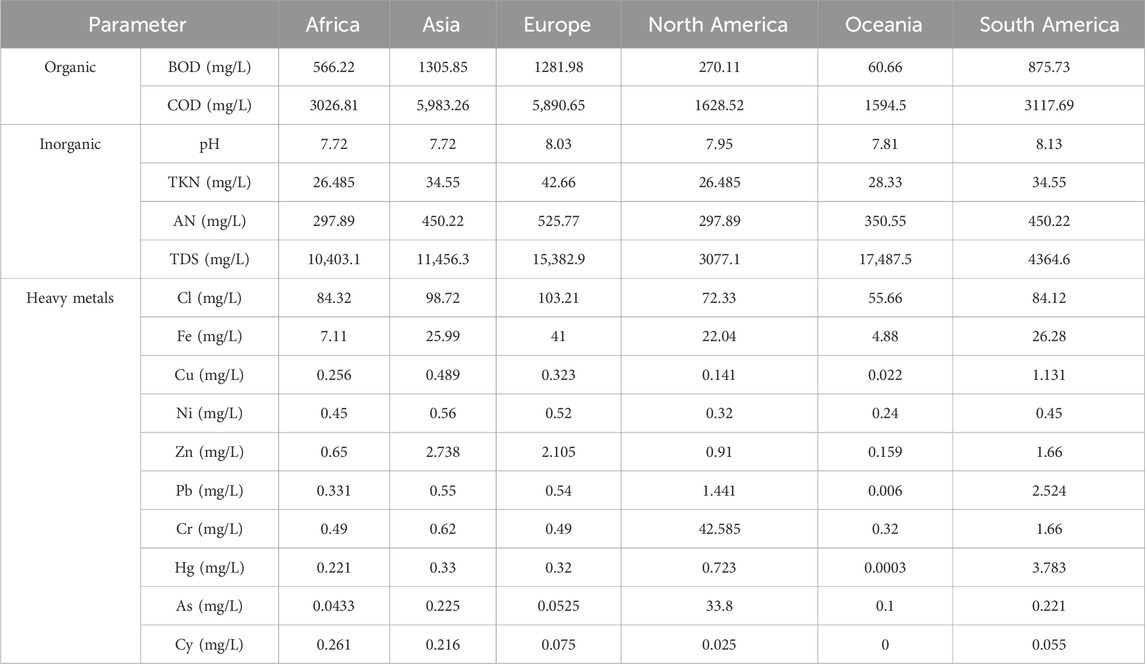
Table 2. Physical and chemical properties of landfill leachate across different continents (Abunama et al., 2021a).
Biologically, leachate is a living, evolving entity, teeming with microorganisms, such as bacteria, fungi and protozoa, which are adapted to its unique chemical milieu. The microbial communities within leachate play a pivotal role in the biodegradation of organic matter, facilitating the natural attenuation of some pollutants (Qi et al., 2023). However, the diversity and activity of these microbial populations can be both a boon and a bane for leachate treatment (Dave et al., 2020). Bacteria such as Pseudomonas and Clostridium spp., and fungi like Aspergillus and Penicillium spp., are integral to breaking down organic pollutants and transforming hazardous substances. While certain bacteria and fungi are harnessed in biological treatment processes to degrade organic pollutants, the presence of pathogenic organisms or those capable of generating harmful byproducts necessitates careful management to prevent environmental and health risks (Mani et al., 2020). The methanogenic archaea, including Methanobacterium and Methanosarcina spp., also play a crucial role by converting waste into methane, highlighting the importance of microbial activity in both waste reduction and potential energy recovery.
The interplay between the physical, chemical, and biological characteristics of leachate significantly influences the selection and design of treatment processes. Physical attributes like suspended solids and temperature necessitate pre-treatment steps such as sedimentation and filtration to prevent system clogging and optimize subsequent treatment stages. Chemical properties, including pH, organic load, and the presence of toxic compounds, determine the need for neutralization, biological treatment, or advanced oxidation processes to ensure effective contaminant removal. Biological factors, such as microbial content and biodegradability, guide the choice of biological treatment methods, which might require nutrient supplementation for optimal microbial activity. Consequently, tailored and integrated treatment strategies are developed to address the specific challenges presented by leachate’s complex composition, ensuring efficient and sustainable management to mitigate its environmental impact.
2.2 Factors on the composition of landfill leachate
The composition of landfill leachate is influenced by a myriad of factors, including the age of the landfill, the types of waste it contains, climatic conditions, and the specific landfill operating technologies employed (Mor and Ravindra, 2023). Each of these factors plays a critical role in determining the leachate’s physical, chemical, and biological characteristics, thus influencing its potential environmental impact and the strategies required for its effective management and treatment (Table 2) (Cano et al., 2020; Golwala et al., 2022; Ma et al., 2022; Abdel-Shafy et al., 2024).
The age of a landfill significantly affects the composition of the leachate produced. In the initial stages of landfilling, leachate tends to have a high organic content, characterized by a high BOD and COD, due to the abundance of readily degradable organic matter (Lindamulla et al., 2022). As the landfill matures, the rate of organic matter decomposition decreases, leading to a reduction in BOD levels (Kulikowska and Klimiuk, 2008). However, the concentration of inorganic compounds, such as ammonia, chlorides, and heavy metals, may increase over time due to the continued degradation and leaching processes (Prieto-Espinoza et al., 2022). This temporal evolution in leachate composition necessitates adaptable treatment approaches that effectively address the shifting balance between organic and inorganic constituents (Saxena et al., 2022) (Table 3). In 2022, Liu et al. conducted a study on the molecular differences between young landfill leachate (YL) and mature landfill leachate (ML) (Liu J. et al., 2022). The results indicated that the COD of YL was 74,853 mg/L, while the COD of ML was 3,098 mg/L, suggesting a higher concentration of organic matter in young leachate. Regarding molecular composition, YL contained lower molecular weight organic compounds, primarily consisting of CHO compounds and aliphatic compounds. In contrast, ML predominantly contained CHON compounds and high oxygen highly unsaturated and phenolic compounds. The molecular structure of YL was relatively simple, with higher bioavailability and more straight-chain structures. ML, however, featured more oxygen-containing functional groups and benzene-ring structures, indicating a more complex molecular structure. In terms of chemical properties, YL exhibited lower nominal oxidation state of carbon and modified aromaticity index, indicating that its organic matter was in a more reduced state. Conversely, ML’s organic matter had higher oxidation states and aromaticity, reflecting that over time, the organic matter in mature leachate had undergone extensive biochemical reactions, leading to more complex structures (Table 4).
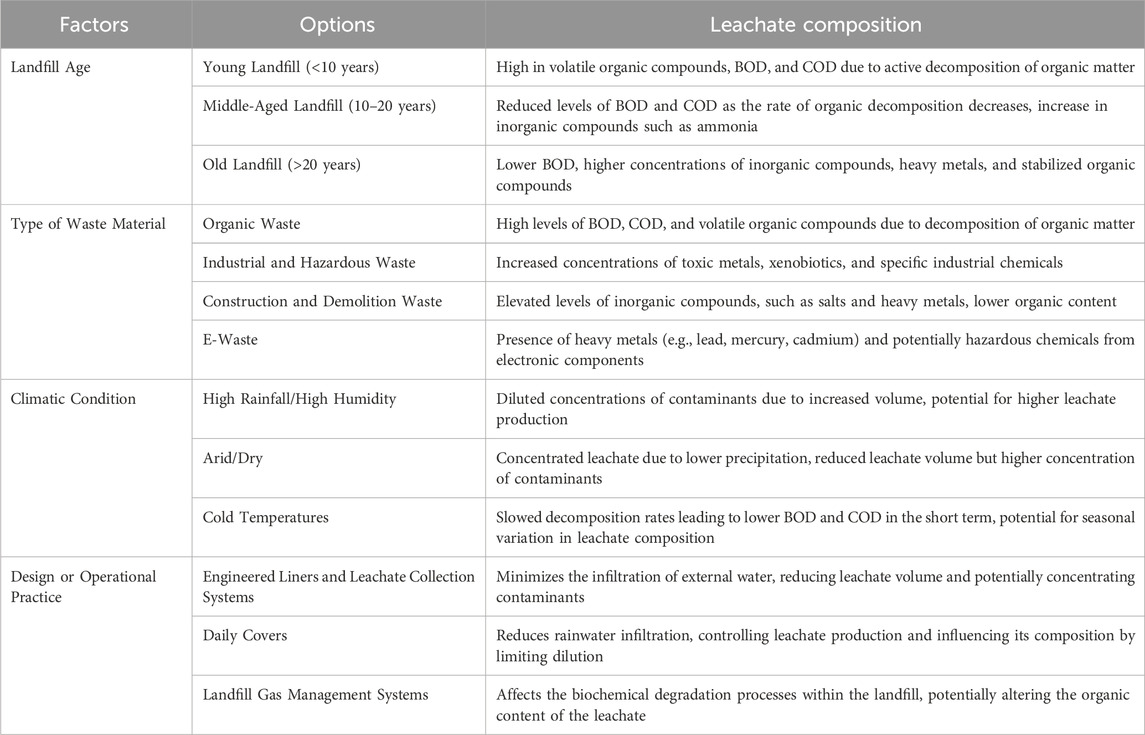
Table 3. Impact of various factors on the composition of landfill leachate (Kulikowska and Klimiuk, 2008; Lindamulla et al., 2022; Prieto-Espinoza et al., 2022; Saxena et al., 2022).
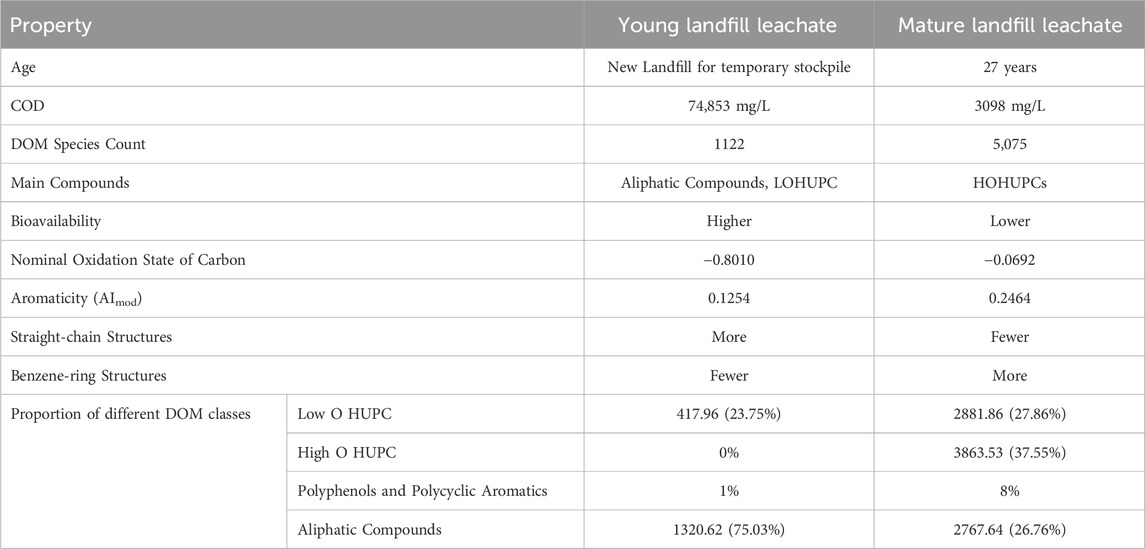
Table 4. Comparative Molecular Characteristics of Young and Mature Landfill Leachates in the example landfills in southwest China (Liu J. et al., 2022).
Moreover, the nature of the waste material disposed of in a landfill is another critical determinant of leachate composition. Landfills containing a high proportion of organic waste, such as food scraps and yard waste, tend to produce leachate with high levels of organic pollutants and a greater potential for biological activity (Kjeldsen et al., 2002). Conversely, landfills with significant industrial or hazardous waste may generate leachate with higher concentrations of toxic metals and xenobiotic organic compounds (El-Saadony et al., 2023). The diversity of waste types, including e-waste, pharmaceuticals, and plastics, contributes to the complexity of leachate, requiring targeted treatment solutions capable of addressing a broad spectrum of contaminants (Srivastava et al., 2021).
Also, climatic conditions, including precipitation, temperature, and humidity, play a pivotal role in the generation and composition of leachate. High rainfall areas will likely produce larger volumes of leachate, potentially diluting its contaminant concentrations but increasing the overall load of pollutants that must be managed (Wijekoon et al., 2022). Temperature affects the rate of biochemical reactions within the landfill, with warmer conditions typically accelerating organic matter decomposition and, consequently, influencing the rate of leachate generation and its organic content (Mohammad et al., 2022). Humidity can also impact the moisture content within the landfill, affecting leachate production rates and composition (Abunama et al., 2021b).
Finally, a landfill’s design and operational practices can significantly influence leachate composition (Ma et al., 2022). Modern landfills equipped with engineered liners and leachate collection systems are designed to minimize the direct contact of waste with groundwater, thereby reducing leachate generation and its potential contamination (Abdel-Shafy et al., 2024). Additionally, using daily covers can limit rainwater infiltration, further controlling leachate volume and composition (Khasawneh et al., 2022). Landfill gas management systems, which capture and treat or utilize the methane produced during organic decomposition, can also impact the biochemical processes within the landfill, indirectly affecting leachate composition (Japperi et al., 2021).
In sum, the composition of landfill leachate results from complex interactions between the age of the landfill, the types of waste it contains, prevailing climatic conditions, and the technologies employed in landfill operation. Understanding these factors is crucial for the development of effective leachate management strategies, as they directly influence the environmental impact of landfills and the technical and economic feasibility of leachate treatment solutions.
2.3 Typical values of pollutants in leachate from different regions
To provide a global perspective, typical values for different classes of contaminants in leachate reported from around the world are described below. These values highlight the variability in leachate composition and typical values for different classes of contaminants in leachate from different regions are critical for designing effective treatment strategies.
Organic pollutants like BOD and COD are critical for assessing the pollution levels. For instance, in tropical regions, average BOD values reported were 2435.16 mg/L in Africa, 3455.77 mg/L in America, and 2127.79 mg/L in Asia, while COD values were 7985.1 mg/L in Africa, 6185.17 mg/L in America, and 7504.32 mg/L in Asia (Lindamulla et al., 2022). In China, BOD5 concentrations varied from 750 to 25,000 mg/L, with an average of 8,500 mg/L, and COD levels ranged from 2,000 to 62,000 mg/L, averaging 19,250 mg/L (Ma et al., 2022).
Inorganic pollutants like Total Dissolved Solids (TDS) and Ammonium Nitrogen (NH4+-N) also show significant variability. Polish landfills reported TDS values from 2,225 mg/L to 7,830 mg/L, while NH4+-N concentrations in tropical regions varied widely, with higher concentrations in younger leachates (Wdowczyk and Szymańska-Pulikowska, 2021). China reported NH4+-N levels ranging from 100 to 3,100 mg/L, with a mean of 1,300 mg/L (Ma et al., 2022). Brazil’s ammonium nitrogen levels ranged between 0.4 and 1,800 mg/L, reflecting the diversity of landfill conditions and waste compositions across the country (Lindamulla et al., 2022).
Heavy metals, another major pollutant category, had varied concentrations with iron ranging from 0.019 mg/L in inactive Polish landfills to 38.73 mg/L in active ones, and zinc reaching up to 2,560 mg/L in Polish active landfills (Wdowczyk and Szymańska-Pulikowska, 2021). In Thailand, heavy metals like cadmium, chromium, copper, nickel, lead, and zinc were generally below 1 mg/L, though still posing potential ecological and health risks (Ma et al., 2022). This highlights the need for careful monitoring and tailored remediation strategies to address potential environmental and health impacts.
The Leachate Pollution Index (LPI) further highlights the pollution potential of leachate, being higher in open dumps compared to engineered landfills (Lindamulla et al., 2022). Young leachates typically show higher LPI values than older leachates, indicating a higher pollution potential in newer landfills. This global variability in leachate composition underscores the importance of site-specific assessments and tailored treatment approaches for effective leachate management.
3 Regulations and standards
Countries around the world are increasingly recognizing the importance of effective landfill leachate treatment as a critical component of waste management and environmental protection. The significant number of research publications on this topic, particularly from nations with large populations, underscores their commitment to addressing the challenges posed by waste and pollutants. Figure 1 shows the research on “landfill leachate treatment” collected from the Scopus database from 2014 to 2023. The top five countries are China (1,675 articles), India (500 articles), the United States (498 articles), Malaysia (418 articles), and Brazil (302 articles). These all reflect a positive stance in mitigating the impact of rapid industrial and urban development on the environment, and countries have also invested heavily in research to develop efficient landfill leachate treatment technologies, further highlighting the global recognition of the need for advanced and sustainable waste management practices. This joint effort is not only aimed at improving public health and environmental quality, but also at promoting global sustainable development.
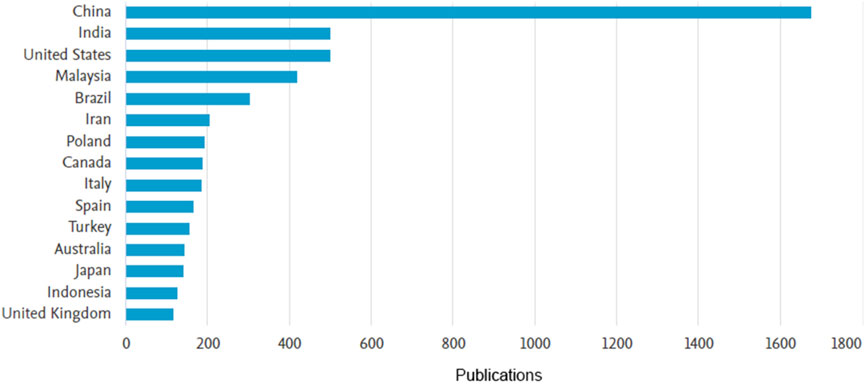
Figure 1. Number of publications from the top 15 countries on the topic “Landfill leachate treatment” collected in the Scopus database from 2014 to 2023.
3.1 Global regulations on landfill leachate management
International and national regulations governing the disposal and treatment of landfill leachate are crucial for protecting the environment and public health from the potential hazards posed by waste management practices (Daniel et al., 2021). These regulations are designed to set minimum standards for leachate management, ensure the safe handling of this potentially toxic byproduct, and encourage sustainable practices in the waste management industry (Table 5) (Oakes and Shank, 1979; Directive, 2003; Protection, 2003; Obradović et al., 2010; Pollutants, 2011; Gas, 2017; Bergesen et al., 2018; Bhawan and Nagar, 2020; Lin et al., 2022; Santana et al., 2022).
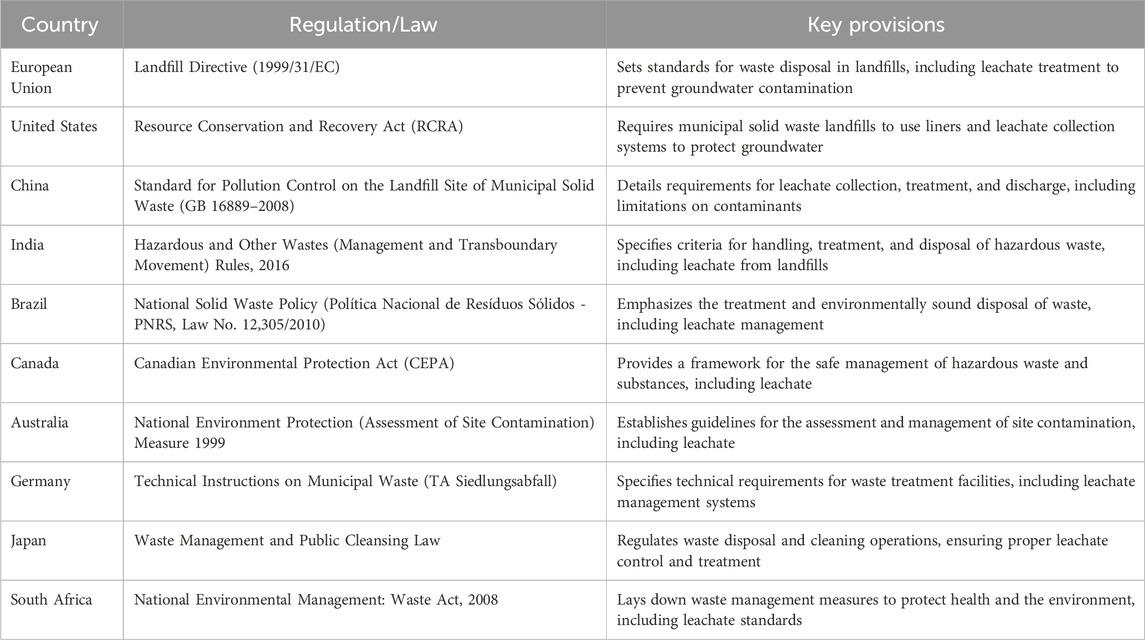
Table 5. Global laws and regulations on landfill leachate management (Oakes and Shank, 1979; Directive, 2003; Protection, 2003; Obradović et al., 2010; Pollutants, 2011; Gas, 2017; Bergesen et al., 2018; Bhawan and Nagar, 2020; Lin et al., 2022; Santana et al., 2022).
At the international level, the Basel Convention on the Control of Transboundary Movements of Hazardous Wastes and their disposal is one of the most significant treaties (Ahmed, 2019). It impacts leachate management by restricting the international movement of hazardous wastes, which includes leachate, and encouraging proper disposal (Amos et al., 2024). Another important piece of international legislation is the Stockholm Convention on Persistent Organic Pollutants, which addresses the treatment and disposal of leachate due to its potential to carry organic pollutants that can persist in the environment (Omoto, 2014). The European Union (EU) has established stringent directives for waste management, including the Landfill Directive (1999/31/EC), which sets the standards for waste disposal in landfills, including the collection and treatment of leachate (Schiopu and Gavrilescu, 2010). It specifies that landfills must be designed and operated to collect and treat leachate to prevent groundwater and environmental contamination. Furthermore, the EU’s Water Framework Directive (2000/60/EC) also affects leachate management by setting out a framework for the protection of inland surface waters, transitional waters, coastal waters, and groundwater, requiring that leachate be treated to prevent the pollution of water bodies (Kyriakopoulos, 2021).
The Resource Conservation and Recovery Act (RCRA) provides the framework for properly managing solid and hazardous waste in the United States (Hinds, 2022). Under Subtitle of RCRA, the Environmental Protection Agency (EPA) has established regulations that require municipal solid waste landfills to use composite liners and leachate collection systems to protect groundwater (Bonaparte et al., 2024). The National Pollutant Discharge Elimination System (NPDES), part of the Clean Water Act, regulates the discharge of leachate into surface waters, requiring permits and setting treatment standards (Hudiburgh, 2020). The EPA’s treatment standards for hazardous wastes subject to land disposal restrictions include several key parameters that must be monitored under applicable regulations (Agency, 2024). These standards specify regulated hazardous constituents, which are chemicals or compounds that need to be treated to meet specific numerical limits before disposal. For instance, chloroform must be reduced to a concentration of 0.046 mg/L for wastewaters and 6.0 mg/kg for nonwastewaters. Characteristic wastes, such as those exhibiting ignitability, corrosivity, reactivity, or toxicity, require deactivation of these characteristics and treatment of any underlying hazardous constituents (UHCs) to meet universal treatment standards (UTS). Ignitable wastes must be treated by methods such as combustion or deactivation, depending on their total organic carbon content, to remove the ignitability characteristic. Corrosive wastes need deactivation to eliminate corrosivity, with high-level radioactive corrosive wastes requiring vitrification. Reactive wastes are generally treated by deactivation, with reactive cyanide wastes having specific concentration-based standards, such as 590 mg/kg total cyanide and 30 mg/kg amenable cyanide for nonwastewaters, and 0.86 mg/L amenable cyanide for wastewaters. Toxicity characteristic wastes must be treated to meet numerical standards for metals, pesticides, and organics, as well as the UTS for UHCs. Additionally, alternative treatment standards are available for certain waste types like contaminated soil and debris, allowing for a reduction in hazardous constituent concentration by 90% or to levels not exceeding 10 times the UTS. These parameters ensure that hazardous wastes are adequately treated to minimize their environmental impact before land disposal.
In China, the Environmental Protection Ministry released the “Standard for Pollution Control on the Landfill Site of Municipal Solid Waste” (GB 16889–2008), which sets out leachate collection, treatment, and discharge requirements (Liu Q. et al., 2022). These include specific limitations on contaminants such as BOD, COD, ammonia nitrogen, total phosphorus, and heavy metals in the discharged leachate (Shi et al., 2021). India has enacted the Hazardous and Other Wastes (Management and Transboundary Movement) Rules, 2016, which set forth the criteria for identifying hazardous waste and its handling, treatment, and disposal standards, including leachate from landfills (Sadala et al., 2023). The Central Pollution Control Board (CPCB) of India provides technical guidelines for the secure landfill of hazardous waste, emphasizing the need for a comprehensive leachate management system (Kumar et al., 2007). In Brazil, the National Solid Waste Policy (Política et al. - PNRS) established under Law No. 12,305/2010 requires proper leachate management and emphasizes the treatment and environmentally sound disposal of waste (Galavote et al., 2022).
These examples demonstrate the common goal across different regulatory frameworks: minimizing environmental and health risks associated with leachate from landfills. National regulations often incorporate or build upon international guidelines, tailoring requirements to local conditions and available technologies. However, the effectiveness of these regulations often depends on the robustness of enforcement mechanisms and the commitment of local authorities to uphold standards. The significance of these regulations extends beyond environmental protection. They also have profound economic and social implications. Strict regulatory standards drive innovation in the waste management sector, leading to the development of new treatment technologies and practices that can reduce the volume and toxicity of leachate. Moreover, by requiring the safe disposal of leachate, regulations also prevent the long-term social costs associated with environmental cleanup and healthcare for communities affected by pollution (Fang et al., 2021).
3.2 Regulatory influence on landfill leachate treatment technology selection
Regulatory standards significantly influence the selection of landfill leachate treatment technologies. These regulations protect the environment and public health from the potential hazards posed by untreated or inadequately treated leachate (Tenodi et al., 2020). As such, they set specific benchmarks that leachate must meet before it can be released into the environment or treated further for potential reuse.
The impact of regulatory standards on the choice of treatment technology is multifaceted. Initially, these standards determine the permissible levels of various pollutants, such as organic matter, heavy metals, and nitrogen compounds, that can be present in the treated leachate (Salem et al., 2008). This directly affects the technology selection, as different processes vary in their ability to remove or neutralize specific contaminants (Ahmed et al., 2017). For instance, high BOD and COD levels might necessitate biological treatment processes, such as activated sludge systems (Haydar et al., 2007). At the same time, the presence of heavy metals may require chemical precipitation or ion exchange technologies (Saleh et al., 2022). Furthermore, regulations often specify the methods that must be used for testing and monitoring leachate quality (Brennan et al., 2016). These requirements can influence the choice of technology by necessitating systems that treat leachate effectively and allow for easy sampling and monitoring. Technologies that offer more control and predictability in their operation, such as membrane bioreactors, can become preferred options under stringent monitoring requirements (Galinha et al., 2018). Advanced treatment technologies like reverse osmosis, nanofiltration, and advanced oxidation processes become more prevalent in jurisdictions with strict environmental standards (Valdés et al., 2021). These technologies can achieve the low contaminant levels required for discharge into sensitive environments or for meeting the high-quality standards necessary for leachate reuse in irrigation or industrial processes (Torretta et al., 2017).
Moreover, regulations also impact the economic aspects of technology choice. Compliance with stringent standards often comes with higher operational and maintenance costs (Siddiqi et al., 2022). As a result, regulatory frameworks can indirectly incentivize the development and adoption of more cost-effective and energy-efficient technologies (Pan et al., 2015). For example, the push to meet low nitrogen limits could encourage the adoption of innovative nitrogen removal processes that consume less energy than traditional methods (Nourmohammadi et al., 2013). Regulatory standards also influence the long-term planning and scalability of treatment solutions. Facilities must consider current regulations and potential future tightening of standards (Bunce et al., 2018). This foresight leads to the selection of technologies that are compliant now and adaptable to future regulatory changes, thereby protecting the investment in treatment infrastructure over time. Environmental policies and regulatory standards can also foster the integration of multiple treatment technologies. To meet comprehensive standards that cover a wide range of contaminants, treatment plants may combine physical, chemical, and biological processes in a tiered treatment strategy. Such integration can achieve higher levels of purification than standalone systems.
In addition to these factors, regulations may promote sustainable practices by advocating for treatment technologies that minimize waste generation or allow for resource recovery (Capodaglio, 2017). Techniques that enable the recovery of byproducts, such as biogas production or the retrieval of precious metals, might be preferred in regions where sustainability is a regulatory goal (Chrispim et al., 2021).
In conclusion, the impact of regulatory standards on the choice of landfill leachate treatment technology is profound, driving innovation and influencing every aspect from the design phase to operational strategy. By setting the bar for environmental compliance, regulations ensure that the selected technologies not only mitigate the potential impact of leachate on the environment but also align with the broader goals of sustainability and public health protection.
4 Conventional landfill leachate treatment technologies
Landfill leachate treatment is a crucial component of modern waste management systems, aiming to mitigate the environmental impact of leachate discharge from landfills. As leachate comprises a complex mixture of dissolved and suspended contaminants, its treatment requires a multifaceted approach involving various conventional technologies. These include physical, chemical, and biological methods, each with principles, advantages, limitations, and practical considerations (Table 6) (Torretta et al., 2017; Dogaris et al., 2020; Ahmed et al., 2021; Younas et al., 2021; Siddiqi et al., 2022).
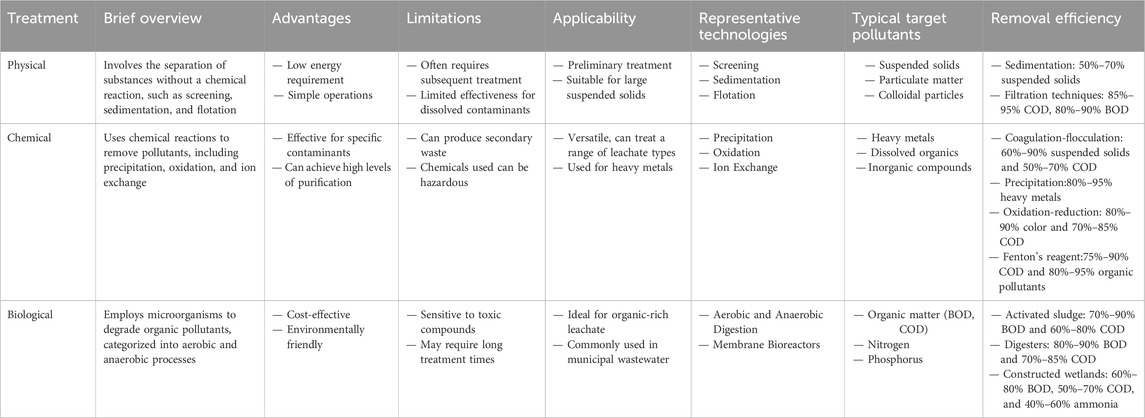
Table 6. Conventional landfill leachate treatment technologies (Torretta et al., 2017; Dogaris et al., 2020; Ahmed et al., 2021; Younas et al., 2021; Siddiqi et al., 2022).
4.1 Physical methods
Physical methods for landfill leachate treatment involve processes that primarily rely on physical separation or filtration mechanisms to remove suspended solids and particulate matter (Renou et al., 2008). These methods typically include sedimentation, filtration, and membrane technologies (Bashir et al., 2016). Sedimentation involves allowing suspended solids to settle out of the leachate under the influence of gravity, facilitating their removal from the liquid phase (Wang et al., 2021). Filtration techniques, such as sand or activated carbon filtration, employ porous media to trap suspended solids and organic compounds as the leachate passes through the filter bed (Loh et al., 2021). Membrane technologies, including microfiltration, ultrafiltration, nanofiltration, and reverse osmosis, utilize semi-permeable membranes to remove contaminants based on their molecular size and charge selectively (Rai and Shrivastav, 2022). Air stripping is a physical treatment process that removes volatile contaminants, such as VOCs and ammonia, from landfill leachate by passing air through the liquid (De et al., 2019a; 2022). The process relies on Henry’s Law to transfer contaminants from the liquid to the gas phase, using packed towers or aeration tanks to maximize contact surface area. Air stripping is effective, simple to operate, and can achieve high removal efficiencies (up to 90% for ammonia). However, it produces off-gas that requires further treatment to prevent air pollution and can be energy-intensive. Despite these challenges, air stripping is an essential part of leachate treatment, often used alongside other technologies to improve overall performance.
In terms of efficiency, sedimentation can remove 50%–70% of suspended solids. Filtration techniques, such as activated carbon filtration, can achieve removal efficiencies of 85%–95% for COD and 80%–90% for BOD (De et al., 2019b; Mojiri et al., 2019). Membrane technologies, including microfiltration, ultrafiltration, nanofiltration, and reverse osmosis, utilize semi-permeable membranes to selectively remove contaminants based on their molecular size and charge. Microfiltration and ultrafiltration can remove 90%–99% of suspended solids and bacteria, while nanofiltration is effective in removing 70%–90% of divalent ions and 50%–70% of monovalent ions (Peng, 2017). Reverse osmosis offers the highest removal efficiencies, capable of removing 95%–99% of dissolved solids, heavy metals, and organics (Peng, 2017).
The principal advantage of physical treatment methods lies in their ability to achieve high levels of contaminant removal, particularly for suspended solids and larger organic molecules (Saravanan et al., 2021). These methods are often effective in reducing turbidity and removing colloidal particles, thereby improving the treated leachate’s visual clarity and overall quality (Zakaria et al., 2023). Additionally, physical treatment processes are generally straightforward to implement and operate, requiring minimal chemical additives and relatively low energy inputs compared to some chemical and biological methods (Nyabadza et al., 2023). However, physical treatment methods also have certain limitations and practical considerations. The potential for membrane fouling or clogging, particularly in membrane-based processes, can reduce treatment efficiency and increase operational costs (Asif and Zhang, 2021). Moreover, physical methods may not effectively remove dissolved contaminants such as heavy metals or organic compounds, which may require additional treatment steps (Saleh et al., 2022). Furthermore, membrane technologies’ high capital and operational costs can limit their widespread adoption, particularly in regions with limited financial resources (Othman et al., 2022).
4.2 Chemical methods
Chemical methods for landfill leachate treatment involve the addition of chemical reagents or agents to facilitate the precipitation, coagulation, flocculation, oxidation, or neutralization of contaminants present in the leachate (Aziz et al., 2022). These methods aim to enhance the removal of dissolved metals, organic compounds, and other pollutants through chemical reactions that transform them into insoluble precipitates or aggregates that can be separated from the liquid phase (El-Saadony et al., 2023; Chen et al., 2024). Common chemical treatment processes include coagulation-flocculation, precipitation, oxidation-reduction, and advanced oxidation processes (AOPs) (Sengupta and Pal, 2021).
Coagulation-flocculation involves the addition of coagulants, such as ferric chloride or aluminum sulfate, followed by the addition of flocculants, such as polymers, to promote the aggregation of suspended solids and colloidal particles into larger flocs that can be easily separated from the leachate (Abujazar et al., 2022). Precipitation methods rely on adding chemicals that react with dissolved contaminants to form insoluble precipitates, which can then be removed by sedimentation or filtration (Pohl, 2020). Oxidation-reduction processes, such as ozonation or chlorination, involve adding oxidizing agents to degrade organic pollutants or reduce the concentration of toxic compounds (Wang and Chen, 2020). AOPs utilize powerful oxidants such as hydrogen peroxide or ozone, combined with UV radiation or catalysts, to generate highly reactive hydroxyl radicals that can mineralize organic contaminants into simpler, less harmful compounds (Pandis et al., 2022).
Regarding removal efficiency, coagulation-flocculation can remove 60%–90% of suspended solids and 50%–70% of COD (Teh et al., 2016). Precipitation is highly effective in removing heavy metals, achieving removal efficiencies of 80%–95% for metals such as lead, cadmium, and chromium (Fu and Wang, 2011). Oxidation-reduction processes like ozonation can remove 80%–90% of color and 70%–85% of COD (Miklos et al., 2018). Advanced oxidation processes, such as the use of Fenton’s reagent, can remove 75%–90% of COD and 80%–95% of organic pollutants (Babuponnusami and Muthukumar, 2014).
The primary advantage of chemical treatment methods lies in their versatility and effectiveness in targeting a wide range of contaminants present in leachate (Pisharody et al., 2022). Chemical processes can achieve high removal efficiencies for dissolved metals, organic compounds, and recalcitrant pollutants that may not be effectively treated by physical or biological methods alone (Nidheesh et al., 2022). Additionally, chemical treatment processes can often be tailored to specific leachate compositions and treatment objectives by adjusting the type and dosage of chemical additives used (Khoo et al., 2020). One of the common disadvantages of chemical treatment methods, particularly coagulation-flocculation and precipitation, is the generation of sludge. This sludge, a byproduct of the treatment process, consists of the aggregated contaminants and excess chemical reagents, posing a significant disposal challenge (Sharma et al., 2022). Using chemical reagents can also increase operational costs and pose health and safety risks to operators if improperly handled. The management of this sludge is crucial as it requires appropriate handling, treatment, and disposal to avoid secondary pollution. Chemical sludge typically needs to be dewatered and may require further stabilization or treatment before disposal in landfills or use in other applications. The disposal costs and environmental impacts associated with sludge management must be considered when evaluating the overall feasibility and sustainability of chemical treatment methods (Hou et al., 2023).
4.3 Biological methods
Biological methods for landfill leachate treatment harness the metabolic activity of microorganisms to degrade organic pollutants and remove nutrients from the leachate (Wu D. et al., 2015). These methods typically involve aerobic treatment, anaerobic treatment, and constructed wetlands, which utilize different microbial communities and environmental conditions to facilitate the leachate’s biodegradation and transformation of contaminants (Kurniawan et al., 2010). Aerobic treatment involves exposing the leachate to oxygen-rich conditions, allowing aerobic bacteria to metabolize organic compounds and oxidize them into simpler, less harmful substances (Bhambore and Suresh Kumar, 2022). On the other hand, anaerobic treatment processes operate under oxygen-free conditions and rely on anaerobic bacteria to degrade organic matter through fermentation and methanogenesis, producing methane gas as a byproduct (Zamri et al., 2021). Constructed wetlands are engineered systems that mimic natural wetland ecosystems and utilize wetland vegetation, soil, and microbial communities to remove contaminants from the leachate through physical, chemical, and biological processes (Hassan et al., 2021). These systems provide an ideal habitat for the growth of wetland plants and microorganisms, which can absorb, adsorb, and metabolize pollutants in the leachate, improving its quality (Bakhshoodeh et al., 2020). Additionally, constructed wetlands can enhance nutrient removal and promote groundwater recharge by facilitating water infiltration and retention in the soil (Chand et al., 2022).
When it comes to biological methods for landfill leachate treatment, aerobic treatment processes, such as activated sludge, can remove 70%–90% of BOD and 60%–80% of COD (Peng, 2017). Anaerobic treatment using digesters can achieve removal efficiencies of 80%–90% of BOD and 70%–85% of COD, with the added benefit of producing biogas as a byproduct (Mukherjee et al., 2015). Constructed wetlands offer another effective solution, capable of removing 60%–80% of BOD, 50%–70% of COD, and 40%–60% of ammonia (Wu S. et al., 2015).
Here we need to mention a mature biological treatment technology that has been widely used in the field of wastewater treatment for many years, biological nutrient removal (BNR). BNR harnesses the metabolism of microorganisms to remove nutrients, notably nitrogen and phosphorus, from wastewater (Deng et al., 2023). BNR is crucial for preventing eutrophication in aquatic environments caused by nutrient overloads (Kabuba et al., 2022). BNR operates through various biological mechanisms, including nitrification, denitrification, and phosphorus uptake by specific bacteria, under different operational conditions to remove these nutrients (Rout et al., 2021a). Factors influencing the efficiency of BNR include biomass concentration, hydraulic retention time, temperature, and pH, which need to be optimized to enhance microorganism activity and nutrient removal (Mishra et al., 2022). The complexity of BNR systems, which can include multiple tanks and stages for different phases of nutrient removal, requires careful design and operation to achieve the desired outcomes (Al-Hazmi et al., 2022). BNR offers a cost-effective and environmentally friendly approach to nutrient removal, avoiding using chemicals and leveraging natural biological processes (Al-Hazmi et al., 2024). This technology requires for a large footprint due to the extensive infrastructure needed and the complexity of operation and maintenance, which demands skilled personnel and continuous monitoring (Abyar and Nowrouzi, 2023).
Biological treatment methods can achieve sustainable and cost-effective leachate treatment through natural processes (Chand et al., 2022). Biological processes are highly efficient in removing organic pollutants and nutrients from the leachate, often achieving high removal efficiencies under favorable environmental conditions (Li et al., 2021). Moreover, biological treatment methods are generally environmentally friendly and produce minimal secondary waste or harmful byproducts compared to chemical or physical methods (Sathya et al., 2023). Nevertheless, compared with chemical or physical methods, the required processing time is longer because the microbial degradation process can be relatively slow, especially under certain environmental conditions (Sharma, 2020). Additionally, biological treatment systems may be sensitive to fluctuations in temperature, pH, and other environmental parameters, which can affect treatment performance and reliability (Sundui et al., 2021). Moreover, toxic or inhibitory substances in the leachate, such as heavy metals or recalcitrant organic compounds, may limit the effectiveness of biological treatment processes and require additional pre-treatment or post-treatment steps to achieve desired treatment objectives (Ilmasari et al., 2022).
In conclusion, conventional landfill leachate treatment technologies encompass diverse physical, chemical, and biological methods, each offering unique advantages, limitations, and practical considerations. Physical methods rely on separation or filtration mechanisms to remove suspended solids and colloidal particles from the leachate. In contrast, chemical methods involve the addition of chemical reagents to facilitate the precipitation, coagulation, or oxidation of contaminants. Biological methods harness the metabolic activity of microorganisms to degrade organic pollutants and remove nutrients from the leachate through natural processes. While each treatment method has its strengths and weaknesses, selecting an appropriate treatment technology depends on factors such as leachate composition, treatment objectives, regulatory requirements, and site-specific considerations. By understanding each treatment method’s principles, advantages, limitations, and practicality, stakeholders can make informed decisions to design and implement effective leachate treatment systems that meet environmental standards and protect human health and the environment.
5 Advanced landfill leachate treatment technologies
5.1 Common technology
5.1.1 Membrane technology
Membrane technology has emerged as a pivotal solution in treating landfill leachate (Chen et al., 2021). This technology offers a versatile and efficient approach to removing contaminants, including dissolved salts, heavy metals, organic compounds, and particulates (El Batouti et al., 2021). The application of membrane technology in leachate treatment primarily involves reverse osmosis, nanofiltration, ultrafiltration, and membrane bioreactors, each serving unique roles in the purification process (Table 7) (de Almeida et al., 2020; Magalhães et al., 2020; Yusuf et al., 2020; El Batouti et al., 2022).
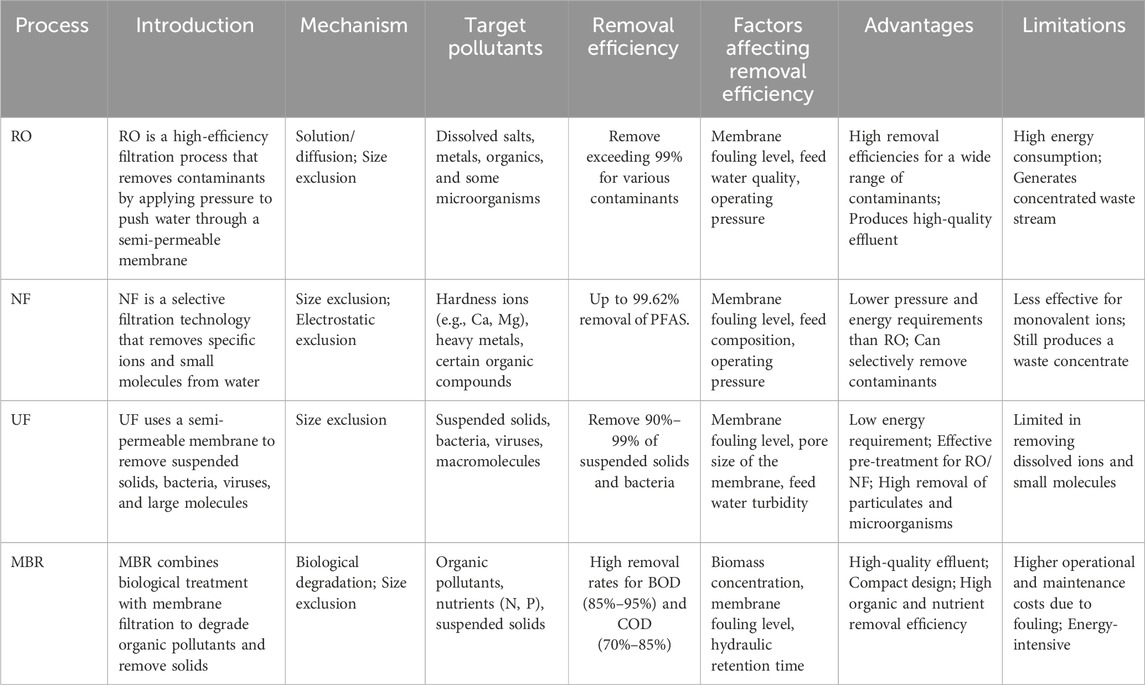
Table 7. Membrane technology in landfill leachate treatment (de Almeida et al., 2020; Magalhães et al., 2020; Yusuf et al., 2020; El Batouti et al., 2022).
5.1.2 Reverse osmosis (RO)
Reverse osmosis is a cornerstone of membrane technology for leachate treatment, renowned for its ability to remove a wide array of contaminants, including dissolved salts and organic molecules (Feria-Díaz et al., 2021). The principle of RO involves applying pressure to the leachate across a semi-permeable membrane, which allows water molecules to pass while retaining the majority of dissolved solids (Mengesha and Sahu, 2022). This process is particularly effective in reducing leachate conductivity, making it a critical step in compliance with stringent discharge regulations (Keyikoglu et al., 2021). RO systems can achieve removal efficiencies exceeding 99% for various contaminants, rendering them indispensable in scenarios requiring high-quality effluent (Srivastava et al., 2022). However, the performance of RO systems is significantly influenced by factors such as membrane fouling, feed water quality, and operating pressure (Odabaşı et al., 2022). Membrane fouling, caused by the accumulation of particles and microorganisms on the membrane surface, can lead to decreased efficiency and increased operational costs (Sengar and Vijayanandan, 2022). To mitigate these effects, pre-treatment processes and regular maintenance are crucial. Additionally, generates a concentrated brine that requires further management or treatment, presenting an environmental and operational challenge (Sengar and Vijayanandan, 2022). RO is particularly suitable for final polishing of treated leachate to meet discharge standards.
5.1.3 Nanofiltration (NF)
Nanofiltration occupies a niche between reverse osmosis and ultrafiltration, with a pore size that allows it to selectively remove divalent and multivalent ions, some monovalent ions, and organic molecules (Zhao et al., 2021). NF is particularly valued for its ability to soften water by removing hardness ions, such as calcium and magnesium, alongside a significant reduction in organic content and color (Patel et al., 2023). NF’s selectivity allows it to target pollutants with lower pressure and energy requirements than RO, making it a cost-effective option for many treatment scenarios (Yadav et al., 2022). The process is well-suited for pre-treatment in RO systems or as a standalone treatment for leachates with moderate contamination levels (Ali, 2021). Like RO, NF also produces concentrate streams that require careful disposal or further treatment (Song et al., 2020). The efficiency of NF is contingent upon membrane fouling, feed composition, and operating pressure (Yang et al., 2024). Similar to RO, the presence of pre-treatment processes is vital to prevent fouling and ensure consistent performance. While NF offers the advantage of selective contaminant removal with reduced energy consumption, its limitations include less effectiveness for monovalent ions and the production of a waste concentrate that requires further treatment (Tian et al., 2021).
5.1.4 Ultrafiltration (UF)
Ultrafiltration is a membrane filtration process employing larger pore sizes than NF and RO, making it ideal for removing suspended solids, bacteria, viruses, and high molecular weight solutes (Urošević and Trivunac, 2020). Factors like membrane fouling, pore size, and feed water turbidity can impact UF’s removal efficiency (Peters et al., 2021). Nonetheless, UF systems require lower energy to operate and serve as an excellent pre-treatment step for RO and NF processes by reducing the load of suspended solids and microbial content, thereby extending the lifespan of downstream membranes (El Batouti et al., 2022). UF is often used as a pre-treatment step to protect downstream RO or NF systems from fouling. It can typically remove 90%–99% of suspended solids and bacteria. The primary advantage of UF lies in its ability to operate at lower pressures, thereby reducing energy costs (Fan et al., 2020). It is also less susceptible to chemical damage, allowing for a broader range of chemical cleaning agents. Although UF is highly effective in removing particulates and microorganisms, its limitations lie in its inability to address dissolved ions and small molecules, underscoring the need for a comprehensive treatment approach that may include subsequent NF or RO stages (Castro-Muñoz, 2020).
5.1.5 Membrane bioreactors (MBR)
The membrane bioreactor technology integrates biological treatment processes with membrane filtration, offering a compact and efficient solution for degrading organic pollutants and removing solids (Neoh et al., 2016). In an MBR system, a bioreactor degrades organic pollutants through microbial activity, while a membrane filtration unit separates treated water from biomass and suspended solids (Deng et al., 2022). MBRs can achieve high levels of organic and nutrient removal, with the added benefit of producing a clarified, disinfected effluent (Hai et al., 2014). Integrating biological treatment and membrane separation enhances system stability and reduces the footprint compared to conventional treatment setups (Waqas et al., 2020). MBRs are suitable for leachate with high organic loads and in locations where space is limited. They can achieve high removal rates for BOD (85%–95%) and COD (70%–85%), along with effective solids separation. The performance of MBRs is influenced by factors such as biomass concentration, membrane fouling, and hydraulic retention time. Despite the challenges of operational and maintenance costs associated with fouling and energy consumption, MBRs provide significant advantages, including high-quality effluent, compact design, and efficient removal of organic matter and nutrients (Meng et al., 2007).
5.2 Advanced oxidation processes
The treatment of landfill leachate using AOPs has garnered significant attention due to the complexity and variability of leachate composition (Fang et al., 2021). AOPs are characterized by their ability to generate highly reactive species, particularly hydroxyl radicals, which can non-selectively degrade a wide range of pollutants (Anandan et al., 2020). Usually there are four primary AOPs: the Fenton process, ozonation, photocatalysis, and electrochemical oxidation (Table 8) (Rekhate and Srivastava, 2020; Wang and Zhuan, 2020; Pandis et al., 2022).
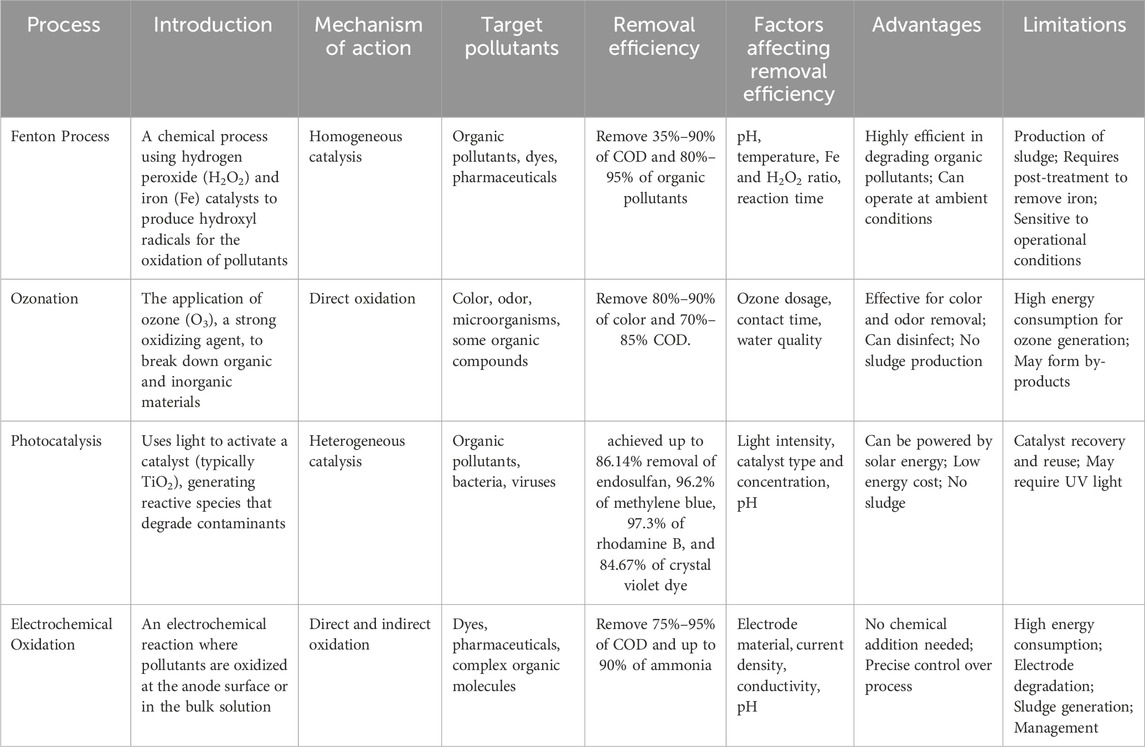
Table 8. AOPs in landfill leachate treatment (Rekhate and Srivastava, 2020; Wang and Zhuan, 2020; Pandis et al., 2022).
5.2.1 Fenton process
The Fenton process is a chemical treatment method that involves the reaction of hydrogen peroxide (H2O2) with ferrous iron (Fe2+) to produce hydroxyl radicals (·OH·) (Benassi et al., 2021). These radicals are extremely reactive and can break down various organic pollutants, including dyes, pharmaceuticals, and other complex organic compounds in landfill leachate (Ateş and Argun, 2021). The mechanism of action in the Fenton process is homogeneous catalysis, where the reaction occurs in solution, allowing for the widespread generation of hydroxyl radicals (Liu et al., 2021). This process is particularly effective for treating organic-rich leachate, offering several advantages, such as high efficiency in degrading organic pollutants and operating under ambient conditions (Soltani et al., 2022). The Fenton process is effective for treating leachate with high concentrations of refractory organic compounds. It can remove 75%–90% of COD and 80%–95% of organic pollutants. However, the process is highly sensitive to operational conditions such as pH, temperature, and the ratios of Fe to H2O2 and reaction time (O’Dowd and Pillai, 2020). A major limitation of the Fenton process is sludge production from iron salts, necessitating further treatment steps (Ziembowicz and Kida, 2022). Additionally, precise control over the reaction conditions is crucial to prevent the consumption of hydrogen peroxide by excessive iron, which would otherwise reduce the efficiency of pollutant degradation (Liu et al., 2024).
5.2.2 Ozonation
Ozonation employs ozone (O3), a potent oxidizing agent, to oxidize pollutants directly (Tripathi and Hussain, 2022). This process is highly effective for removing color, odor, and microorganisms from landfill leachate and degrading some organic compounds (Yang C. et al., 2021). The direct oxidation mechanism of ozonation allows for rapid reaction rates, making it a valuable option for enhancing the biodegradability of recalcitrant organic compounds in leachate (Yang Y. et al., 2021). Ozonation can remove 80%–90% of color and 70%–85% of COD. The efficiency of ozonation is influenced by ozone dosage, contact time, and the quality of water being treated. Its advantages include effective color and odor removal, disinfection capabilities, and the absence of sludge production (Hussain et al., 2022). However, ozonation requires significant energy to generate ozone gas, and the potential formation of by-products during the oxidation of certain pollutants can be a concern (Wang and Chen, 2020). Despite these limitations, ozonation remains a favored choice for specific applications within leachate treatment, particularly when targeting pollutants susceptible to oxidation by ozone.
5.2.3 Photocatalysis
Photocatalysis involves using light (typically UV) and a catalyst (commonly titanium dioxide, TiO2) to produce reactive species capable of degrading pollutants (Gopinath et al., 2020). This process is governed by heterogeneous catalysis, where the catalyst remains in a solid phase, distinct from the liquid phase in which the pollutants are dissolved (Iervolino et al., 2020). Photocatalysis is effective against many contaminants, including organic pollutants, bacteria, and viruses, making it an attractive option for treating landfill leachate (Han et al., 2020). Factors affecting the efficiency of photocatalysis include light intensity, catalyst type and concentration, and the pH of the solution (Gusain et al., 2020). Photocatalysis offers the potential for low energy costs, especially when powered by solar energy, and the absence of sludge production (Ahmad et al., 2020). Catalyst recovery and reuse and dependence on UV light for optimal performance limit its widespread application (Kefeni and Mamba, 2020). Despite these challenges, photocatalysis presents a sustainable option for leachate treatment, especially in sunny climates where solar radiation can be effectively harnessed.
5.2.4 Electrochemical oxidation
Electrochemical oxidation utilizes an electrical current to facilitate the oxidation of pollutants at an electrode surface or within the bulk solution (Fitch et al., 2022). Through direct and indirect oxidation mechanisms, this method can target a broad spectrum of pollutants, including dyes, pharmaceuticals, and complex organic molecules (da Silva et al., 2021). This process is effective for treating leachate with a high load of organic pollutants and ammonia. Electrochemical oxidation can remove 75%–95% of COD and up to 90% of ammonia. One of the primary advantages of electrochemical oxidation is the absence of chemical additives coupled with precise control over the treatment process (Yang L. et al., 2021). This method offers an environmentally friendly approach to leachate treatment, albeit with considerations for high energy consumption and potential electrode degradation over time (Deng et al., 2020).
5.3 Other technologies
As environmental standards become increasingly stringent, adopting innovative and efficient treatment technologies is essential. Emerging technologies for treating landfill leachate are pivotal in addressing the environmental challenges posed by the complex and variable composition. These technologies, including adsorption, ion exchange, and biological nutrient removal (BNR), offer innovative solutions to remove many pollutants, from organic compounds and heavy metals to nitrogen and phosphorus (Table 9) (Bhambri and Karn, 2020; Deng et al., 2020; Hasan et al., 2021; Rajesh Banu et al., 2021).
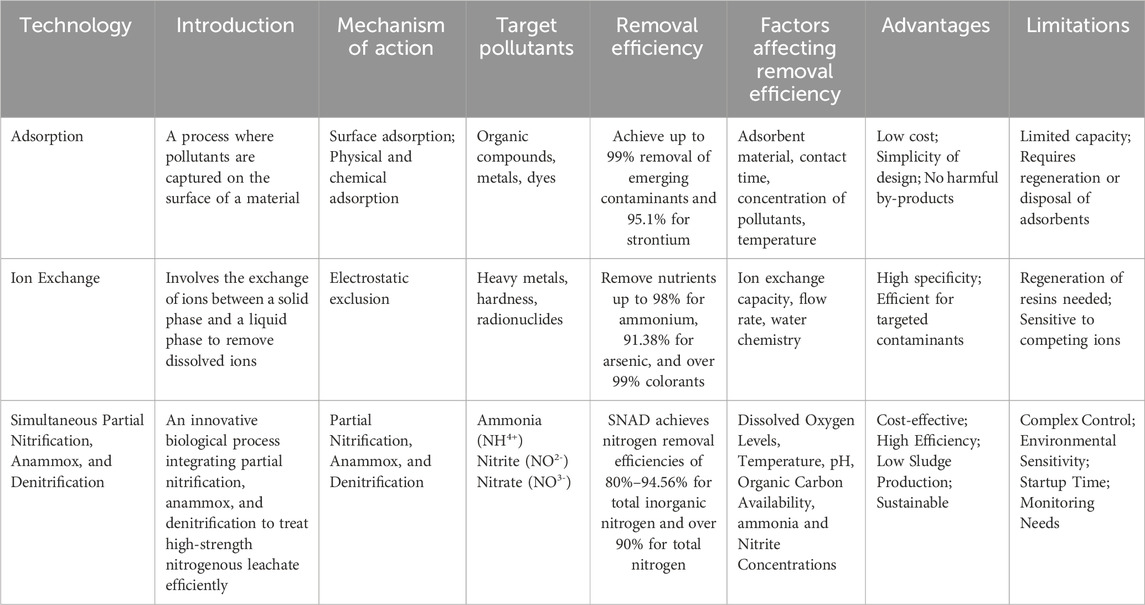
Table 9. Emerging technologies in landfill leachate treatment (Bhambri and Karn, 2020; Deng et al., 2020; Hasan et al., 2021; Rajesh Banu et al., 2021).
5.3.1 Adsorption
Adsorption is a versatile and widely implemented method for contaminant removal, characterized by the adherence of pollutants onto the surface of an adsorbent material (Shen et al., 2023). This process can occur through physical adsorption, driven by weak van der Waals forces, or chemical adsorption, involving stronger covalent bonds (Rathi and Kumar, 2021). The mechanism is influenced by the surface area, porosity, and chemical nature of the adsorbent, as well as the properties of the pollutants (Ambaye et al., 2021). Adsorption is particularly effective for removing organic compounds, heavy metals, and dyes from leachate, making it a valuable tool for improving water quality (Saravanan et al., 2021). The adsorption efficiency is determined by factors such as the type and concentration of pollutants, contact time, adsorbent material, and environmental conditions like temperature (Rathi and Kumar, 2021). Activated carbon is among the most commonly used adsorbents due to its high surface area and porosity, although other materials like biochar, zeolites, and engineered nanomaterials are also employed (Sharma et al., 2022). The primary advantages of adsorption include its simplicity, cost-effectiveness, and the absence of harmful by-products. The finite adsorption capacity of materials, which necessitates their periodic regeneration or replacement (Sharma et al., 2024). Similar to coagulation-flocculation, the adsorption method also generates sludge, which consists of spent adsorbent materials saturated with contaminants (Kurniawan et al., 2021a). The management of this adsorption sludge is a critical issue, as it involves the disposal or regeneration of spent adsorbents. Regeneration can often be achieved through thermal, chemical, or biological methods to restore the adsorbent’s capacity for reuse. However, these processes can be energy-intensive and costly, and not all adsorbents can be regenerated effectively. Disposal of non-regenerable adsorbents must be conducted in an environmentally safe manner, often requiring landfilling or incineration, which introduces additional costs and environmental concerns. The environmental impact and cost of managing adsorption sludge are important factors in assessing the overall sustainability of the adsorption process.
5.3.2 Ion exchange
Ion exchange is a specialized technique designed for removing dissolved ions from wastewater, functioning through the reversible exchange of ions between a liquid and a solid phase (Liu et al., 2023). Ion exchange relies on electrostatic interactions between charged particles, making it particularly suitable for targeting specific ionic contaminants such as heavy metals, hardness-causing ions (e.g., calcium and magnesium), and radionuclides (Liu et al., 2023). The performance of ion exchange systems is influenced by the resin or medium’s ion exchange capacity, the leachate’s flow rate, and the water’s chemical composition, including the presence of competing ions (Xu et al., 2024). Factors such as resin specificity, regeneration requirements, and operational parameters must be carefully managed to optimize performance (Clark et al., 2024). Ion exchange resins, synthetic or natural, are chosen based on their selectivity for certain ions, offering high specificity and efficiency in contaminant removal (Wang et al., 2024). Ion exchange offers the advantage of high specificity and efficiency for targeted contaminants, providing a tailored approach to leachate treatment (Aziz et al., 2023). The need for regular resin regeneration with chemicals like brine, which can generate secondary waste streams (Sahu, 2021). It is sensitive to competing ions, which can reduce the efficiency of targeted ion removal, requiring careful water chemistry management (Chen et al., 2023).
5.3.3 Simultaneous partial nitrification, anammox, and denitrification (SNAD)
SNAD is an emerging biological treatment technology that integrates partial nitritation, anaerobic ammonium oxidation (anammox), and denitrification processes within a single reactor (Singh et al., 2022). This integrated approach shows great potential for treating landfill leachate with high ammonia concentrations. The SNAD process involves three main steps: partial nitritation, which converts part of the ammonia into nitrite; anammox, which utilizes the remaining ammonia and the produced nitrite to generate nitrogen gas; and denitrification, which reduces any remaining nitrate to nitrogen gas. These processes occur simultaneously in the same reactor, achieving efficient nitrogen removal. SNAD achieves nitrogen removal efficiencies of 80%–94.56% for total inorganic nitrogen and over 90% for total nitrogen, while also reducing oxygen demand and sludge production compared to conventional methods (Zheng et al., 2016). SNAD technology offers several advantages for landfill leachate treatment, including high nitrogen removal efficiency, energy savings due to lower oxygen and organic carbon requirements compared to traditional nitrification-denitrification processes, reduced operational costs, smaller footprint due to the integration of three processes in one reactor, and less sludge production, which lowers sludge handling costs (Su et al., 2022). Despite its promising prospects, SNAD technology faces challenges such as the need for precise control of parameters like dissolved oxygen and pH, long startup times due to the slow growth of anammox bacteria, and sensitivity to temperature. Future research will focus on optimizing operational parameters, enhancing system stability, and exploring innovative reactor designs to further improve the application of SNAD technology in landfill leachate treatment.
In summary, when selecting an appropriate landfill leachate treatment technology, it is essential to consider the balance between efficiency, cost, and sustainability (Özdemir et al., 2020; Cherni et al., 2021; Dereli et al., 2021; Saadatlu et al., 2023). Membrane technologies are highly effective, capable of removing up to 99% of dissolved salts and other specific pollutants. However, their application involves significant capital and operational costs, particularly with RO, due to the expenses associated with energy use, membrane replacement, and maintenance. Despite these costs, advancements in technology are gradually improving the sustainability of membrane processes, although energy use and membrane disposal remain concerns. AOPs offer another robust solution, particularly for degrading resistant organic compounds and pathogens. Nevertheless, they come with high operational costs, mainly due to the chemical inputs and energy demands, such as for ozone generation or UV lamps. These factors also contribute to moderate sustainability concerns, especially regarding potential secondary pollution. Emerging technologies provide a more cost-effective and sustainable approach. While their efficiency varies depending on the materials and infrastructure used, they excel in targeting specific contaminants like heavy metals and nutrients. These technologies emphasize the use of recyclable materials and natural processes, thereby enhancing environmental friendliness. The choice of leachate treatment technology should be guided by specific treatment goals, regulatory requirements, and sustainability objectives, balancing efficiency with environmental and economic considerations to achieve effective and responsible leachate management.
6 Comprehensive treatment system
The cornerstone of addressing the leachate challenge lies in implementing a comprehensive treatment system that synergistically combines various treatment methodologies to remove contaminants effectively and meet or exceed regulatory discharge standards (Abdelfattah and El-Shamy, 2024). These systems are designed not only to mitigate the adverse environmental impacts of leachate but also to contribute to the sustainability of landfill operations by enabling the possibility of resource recovery and reuse of treated water (Mojiri et al., 2020).
6.1 Comprehensive treatment system
A comprehensive landfill leachate treatment system is a multi-faceted and dynamic approach that mitigates leachate contaminants’ environmental impacts through a well-orchestrated sequence of processes (Figure 2) (Britz, 2020; Babaei et al., 2021; Fang et al., 2021; Righetto et al., 2021; Teng et al., 2021; Nath and Debnath, 2022). Initiating with pretreatment, the system begins by addressing the more tangible aspects of leachate—easily separable solids are removed, and the pH is carefully adjusted. This foundational step is about preparing the leachate for the complex journey ahead and safeguarding the intricate mechanisms of downstream processes from potential abrasion, blockages, and harmful chemical interactions. As the leachate advances, it encounters the primary treatment phase, a sophisticated interplay between biology and chemistry. Here, living microbes engage in a digestive ballet, utilizing both aerobic and anaerobic pathways to metabolize organic pollutants, thereby reducing the load of substances that demand oxygen in the water. Concurrently, a suite of physical-chemical treatments, each a targeted strike—coagulation, flocculation, and flotation—work in concert to clear out suspended solids, strip away color, and separate particulate organic matter. This systematic approach continues into the secondary treatment, where a more refined attack is launched on dissolved organic compounds that have eluded the grasp of biological processes. This stage employs the precision of AOPs, the fine sieve of membrane filtration technologies like reverse osmosis, and the binding affinities of adsorption techniques, each method meticulously selected to ensure no contaminant is left unchallenged. Nearing the end, the leachate undergoes tertiary treatment—an exemplary display of purification, where the effluent is polished to meet stringent quality standards. Further, membrane filtration stands sentinel against the minutest of pollutants, disinfection processes wage war on pathogenic microorganisms, and additional specific treatments meticulously remove any lingering traces of heavy metals or nitrogen compounds.
The comprehensive landfill leachate treatment system’s responsibility does not end with the effluent; sludge management remains a testament to the ethos of sustainability that underpins the entire operation (Torretta et al., 2017). This critical phase manages the solid byproducts of the leachate’s treatment to minimize further environmental impact, reclaim valuable resources, and embody the cyclic nature of waste transformed into worth (Remmas et al., 2023). Implementing a comprehensive landfill leachate treatment system is a paragon of environmental responsibility and technological ingenuity, offering multifarious benefits that resonate far beyond the confines of waste management. Such systems are critical in preventing the percolation of hazardous contaminants into the groundwater and surface waters, safeguarding the aquatic ecosystems and public health against the potential onslaught of toxic substances (Ravindiran et al., 2023). By utilizing progressively targeted treatments that include biological degradation, chemical transformation, and physical separation, these systems adeptly reduce the concentrations of organic and inorganic pollutants, heavy metals, and pathogens to meet stringent regulatory standards (Rathod et al., 2024). Beyond the immediate environmental benefits, these systems embrace the principles of a circular economy, often allowing for the recovery of resources such as biogas, which can be converted to energy, and treated water, which can be repurposed for industrial or agricultural use (Zarei, 2020). This contributes to the conservation of natural resources and offers economic advantages by offsetting operational costs and generating revenue (Ekins and Zenghelis, 2021). Comprehensive treatment systems are committed to sustainable development as they adapt to the ever-changing waste composition and emerging contaminants, ensuring resilience against future environmental challenges. Thus, these systems’ significance lies in their immediate efficacy in pollution control and their strategic contribution to long-term environmental sustainability and economic viability (Mojiri et al., 2020).
6.2 Example of comprehensive treatment system for landfill leachate
In the bustling urban environment of Changsha City, the capital of Hunan Province, China (28.2282°N, 112.9388°E), located 35 km north in Qiaoyi Town, Wangcheng District, the Changsha Municipal Solid Waste Treatment Site stands as a pivotal solution to the city’s waste management challenges. Operated by Welle Environmental Protection Technology Group Co., Ltd., this project exemplifies a cutting-edge approach to handling landfill leachate treatment (Welle Environmental Protection Technology Group Co.L, 2024). As the sole comprehensive co-processing site for municipal solid waste in Changsha, it is responsible for managing domestic waste from the city’s expansive area, municipal sludge from 13 sewage treatment plants, and all leachate in the reservoir area. The facility is ingeniously divided into two functional units: landfill and incineration plant, with 80% of domestic waste processed through incineration for electricity generation and the remaining 20% allocated for landfilling. The core challenge in managing landfill leachate stems from its highly concentrated and variable composition, which can severely impact local water sources and the broader environment.
To tackle this, Welle Group Co., Ltd has designed a comprehensive leachate treatment system addressing both landfill leachate and domestic/low-concentration sewage (Figure 3). The process for high-concentration leachate regeneration is a testament to engineering excellence, employing a sophisticated combination of “pretreatment + up-flow anaerobic sludge blanket (UASB) + external membrane bioreactors (MBR) + nanofiltration (NF) + reverse osmosis (RO). This multi-stage approach ensures the thorough removal of contaminants, making the leachate safe for discharge or reuse. In addition to the high-concentration leachate treatment, the facility also addresses the treatment of domestic and low-concentration inorganic sewage. The domestic sewage treatment follows a “mixed coagulation and sedimentation + anaerobic-aerobic (A/O) + built-in MBR” process. In contrast, the low-concentration inorganic sewage is treated through “coagulation and air flotation + multi-media filtration + RO.” These processes are designed to efficiently handle the varied waste streams entering the facility, ensuring that all forms of wastewater are effectively managed. The landfill leachate treatment, in particular, employs an “external MBR + NF + RO” process. This specific treatment path is crucial for dealing with the highly contaminated leachate from the landfill, effectively reducing its pollutant load before release or reuse. The concentrated liquid produced from these treatment processes undergoes advanced oxidation/reduction treatment, showcasing the facility’s commitment to minimizing environmental impact. This liquid is then cleverly returned to the factory for consumption, embodying the principles of sustainability and resource recovery. The achievements of this project are both tangible and impactful. After undergoing the comprehensive treatment processes, the landfill leachate meets the stringent emission standards outlined in Table 2 of the “Pollution Control Standards for Domestic Waste Landfills” (GB16889-2008) (Table 10). Similarly, the effluent from the three sewage systems at the incineration plant complies with the “Water Quality for Industrial Water for Recycling of Urban Wastewater” (GB/T19923-2005), which sets the water quality standards for supplementary water in open circulating water systems. The project addresses the immediate need for waste and leachate treatment and contributes to resource conservation and pollution reduction goals. It stands as a testament to the power of technology and strategic planning in solving the complex environmental challenges of our time.
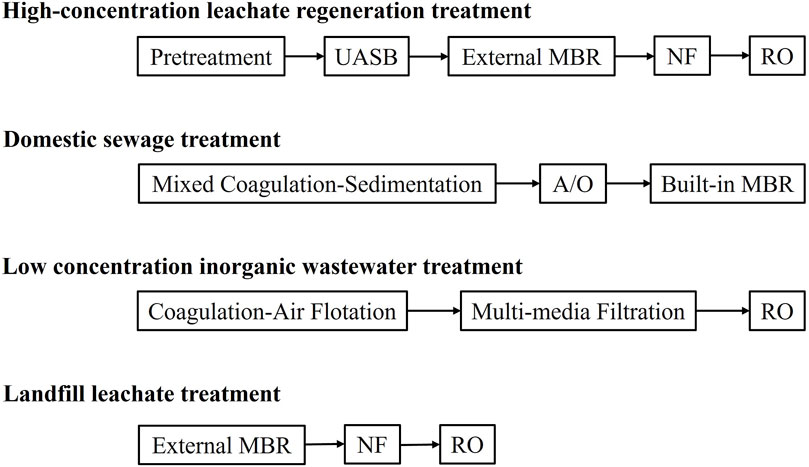
Figure 3. Comprehensive wastewater treatment system by Welle Group Co., Ltd, addressing high-concentration landfill leachate and domestic/low-concentration sewage through advanced multi-stage processes for effective contaminant removal and safe discharge or reuse.
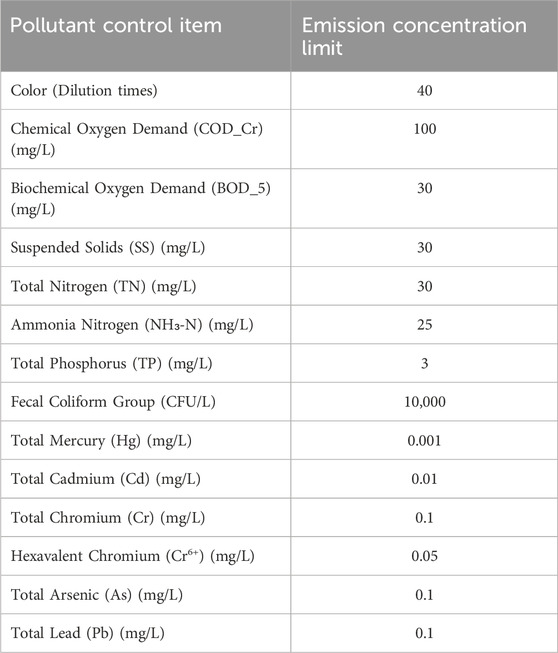
Table 10. Emission standards in Table 2 of the ‘pollution control standards for municipal solid waste landfills’ (GB16889-2008).
6.3 Optimizing integrated landfill leachate treatment systems
In environmental management, optimizing and modeling an integrated system for landfill leachate treatment serve as cornerstones for enhancing system efficiency, curbing expenditures, and adhering to environmental norms (Awewomom et al., 2024). The essence of this optimization lies in crafting a flexible treatment architecture that proficiently manages the intricate and diverse makeup of leachate while remaining nimble to fluctuations in waste inputs and legislative edicts. A synergy of suitable treatment modalities—such as UASB, MBR, NF, and RO—complements the specific leachate profile, ensuring that downstream processing is primed for optimal treatment (Huang et al., 2024). Operational parameters like retention time, aeration rate, and chemical dosing are meticulously fine-tuned to bolster the removal of pollutants, and real-time monitoring systems are deployed for on-the-fly adjustments. The model’s sophistication extends to embodying resource recovery, advocating for repurposing byproducts like biogas into energy and advocating for the reuse of treated water.
The modeling facet delves into the assimilation of comprehensive data, which informs the simulation of the system’s multifaceted treatment dynamics through advanced mathematical constructs (Ergene et al., 2022). These models are enriched with predictive analytics and scenario simulations, courtesy of machine learning algorithms, paving the way for a thorough understanding of potential operational outcomes. Validation through empirical data ensures the model’s fidelity, while optimization algorithms distill the operational sweet spot that marries environmental efficacy with economic prudence (Podlasek, 2023). This ongoing, iterative process is honed by real-world performance feedback and shifting regulatory frameworks, propelling landfill leachate treatment toward unparalleled operational finesse.
7 Challenges and future
In addressing the complex challenges of landfill leachate treatment, it is crucial to consider the economic, operational, and emerging contaminant-related hurdles that impede the efficacy of current systems. This section delves into the multifaceted issues faced by waste management facilities, highlighting the significant financial burdens, operational difficulties due to leachate variability, and the rising threat of emerging contaminants like pharmaceuticals and microplastics. These challenges necessitate innovative solutions and strategic approaches to ensure effective, sustainable leachate management in the future.
7.1 Comprehensive challenges in landfill leachate treatment: Economic, operational, and emerging contaminant
The foremost economic challenge in landfill leachate treatment lies in the high capital and operational costs associated with advanced treatment systems (El-Saadony et al., 2023). Technologies such as RO, NF, and MBRs are effective in removing a wide range of contaminants but require substantial financial investment. These systems are not only expensive to install but also incur ongoing costs related to energy consumption, maintenance, and the need for skilled personnel to operate and monitor the processes. The financial burden is further amplified by the variability in leachate composition, which demands adaptable systems capable of handling fluctuations in pollutant concentrations. This variability necessitates continuous system optimization, leading to additional costs associated with adaptive management and process upgrades (Yadav et al., 2021). Table 11, which outlines the costs of various representative leachate treatment technologies, underscores the financial strain on waste management facilities (Gerald et al., 1998; Randall and Ubay Cokgor, 2000; Mahamuni and Adewuyi, 2010; Ju-Chang Huang, 2017; Ighalo et al., 2022; Pérez et al., 2022; Kurniawan et al., 2023). Moreover, the costs are not merely limited to initial capital outlays but extend to operational expenses that accumulate over the system’s lifecycle (Abdelfattah and El-Shamy, 2024). As such, the economic challenges of landfill leachate treatment call for innovative approaches to reduce costs while maintaining treatment efficacy.
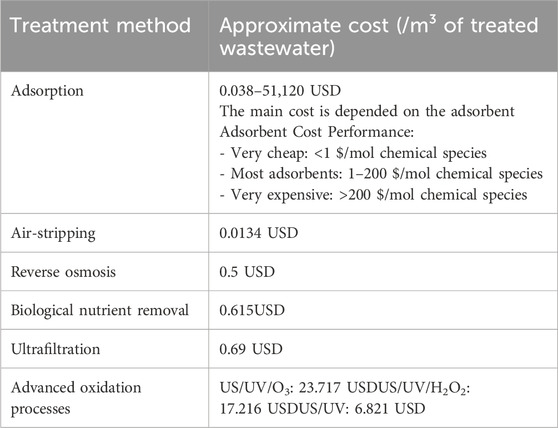
Table 11. Costs of representative leachate treatment technologies (Gerald et al., 1998; Randall and Ubay Cokgor, 2000; Mahamuni and Adewuyi, 2010; Huang, 2017; Ighalo et al., 2022; Pérez et al., 2022; Kurniawan et al., 2023).
Operationally, the treatment of landfill leachate is fraught with difficulties due to the high variability of leachate quality. This variability is influenced by factors such as seasonal changes, waste composition, and the age of the landfill, all of which affect the concentration and types of pollutants present in the leachate. The dynamic nature of leachate composition requires continuous monitoring and adjustment of treatment processes to ensure consistent performance (Upadhyay et al., 2023). Operators must continuously monitor and adjust treatment processes, requiring skilled personnel and sophisticated control systems. The potential for system failure or suboptimal performance poses significant environmental risks, including groundwater contamination and non-compliance with discharge regulations, leading to potential fines and reputational damage. Addressing these operational challenges requires a holistic approach that integrates cost-effective treatment technologies with operational efficiency and environmental stewardship (Kundariya et al., 2021). The development of more energy-efficient membrane technologies or the adoption of natural treatment solutions, such as constructed wetlands, offers promising avenues for reducing operational difficulties and associated costs (Kataki et al., 2021). For instance, the development of more energy-efficient membrane technologies or the adoption of natural treatment solutions, such as constructed wetlands, offers promising avenues for reducing operational difficulties and associated costs. Moreover, embracing circular economy principles, where treated leachate is reused for industrial or agricultural purposes, can help mitigate operational challenges while contributing to sustainability goals. (Bakan et al., 2022; Das et al., 2023).
The emergence of new contaminants, such as pharmaceuticals, personal care products, microplastics, and other micropollutants, presents a significant challenge to traditional wastewater and landfill leachate treatment systems (Kumar et al., 2023). These substances, often called emerging contaminants (ECs), have become a focal point for environmental scientists and engineers due to their potential to bypass conventional treatment processes and enter the aquatic environment, posing risks to wildlife and human health (Ahmed et al., 2021; Morin-Crini et al., 2021). Traditional wastewater and landfill leachate treatment systems were designed to remove organic matter, nutrients, and major pollutants (Lindamulla et al., 2022). However, these systems often lack the specificity to capture and degrade ECs effectively, many of which are designed to be biologically active and resistant to degradation (Teng and Chen, 2023). Pharmaceuticals, for example, are engineered to resist metabolic breakdown to ensure their efficacy, making them persistent in the environment (González-González et al., 2022). Microplastics, conversely, are resistant to biodegradation due to their synthetic polymer composition, accumulating in water bodies and potentially entering the food chain (Huang et al., 2021). The presence of ECs in water and leachate streams can significantly reduce the treatment efficiency of these systems (Rout et al., 2021b). Certain contaminants can inhibit microbial activity in biological treatment processes, reducing the degradation rate of organic pollutants and altering the microbial community structure (Aragaw, 2021). For example, antibiotics in wastewater can inhibit the growth of bacteria critical to the biological treatment processes, leading to a decrease in overall treatment efficiency (Zhu et al., 2021). Moreover, some ECs can form complex compounds with other elements in the wastewater, making them more difficult to remove and requiring additional treatment stages or more advanced treatment technologies (Shahid et al., 2021). Notably, based on the environmental risks and regulatory status of chemicals, and following technical feasibility and socioeconomic assessments, the Ministry of Ecology and Environment of China published the latest list of key controlled emerging contaminants in 2023 (Table 12) (China, 2022). The newly listed contaminants are now subject to environmental risk management measures, including those related to their manufacture, use, import, or export. Some contaminants have transition periods or exemptions. This initiative highlights the government’s commitment to addressing emerging contaminants and urges relevant departments to take action to prevent environmental and public health risks. For landfill leachate treatment, the presence of these new pollutants complicates the process, often requiring upgrades or advanced technologies to effectively manage them. Proactively addressing these contaminants can improve treatment efficiency and reduce environmental contamination risks. It is worth mentioning that, Per- and polyfluoroalkyl substances (PFAS) are synthetic chemicals widely used for their water- and grease-resistant properties, found in various consumer products and industrial applications (Venkatesh Reddy et al., 2024). Their strong carbon-fluorine bonds make them highly persistent and resistant to conventional water treatment methods, posing significant challenges for removal (Jin et al., 2021). Traditional processes like coagulation, sedimentation, and standard filtration are largely ineffective against PFAS, necessitating the use of advanced technologies (Nguyen and Le Tran, 2024). Methods such as granular activated carbon adsorption, ion exchange, and high-pressure membrane filtration (nanofiltration and reverse osmosis) show varying degrees of success but often involve high operational costs and complex waste management (Lee et al., 2022). Emerging technologies like electrochemical treatment, plasma-based processes, and combined treatment approaches offer potential but require further development and investment (Blotevogel et al., 2023). The inclusion of PFAS-specific treatments increases operational complexity and costs for water treatment facilities, which must adapt to stringent regulatory standards. Research continues to focus on more efficient and cost-effective removal technologies to address the persistent nature of PFAS and protect public health and the environment.
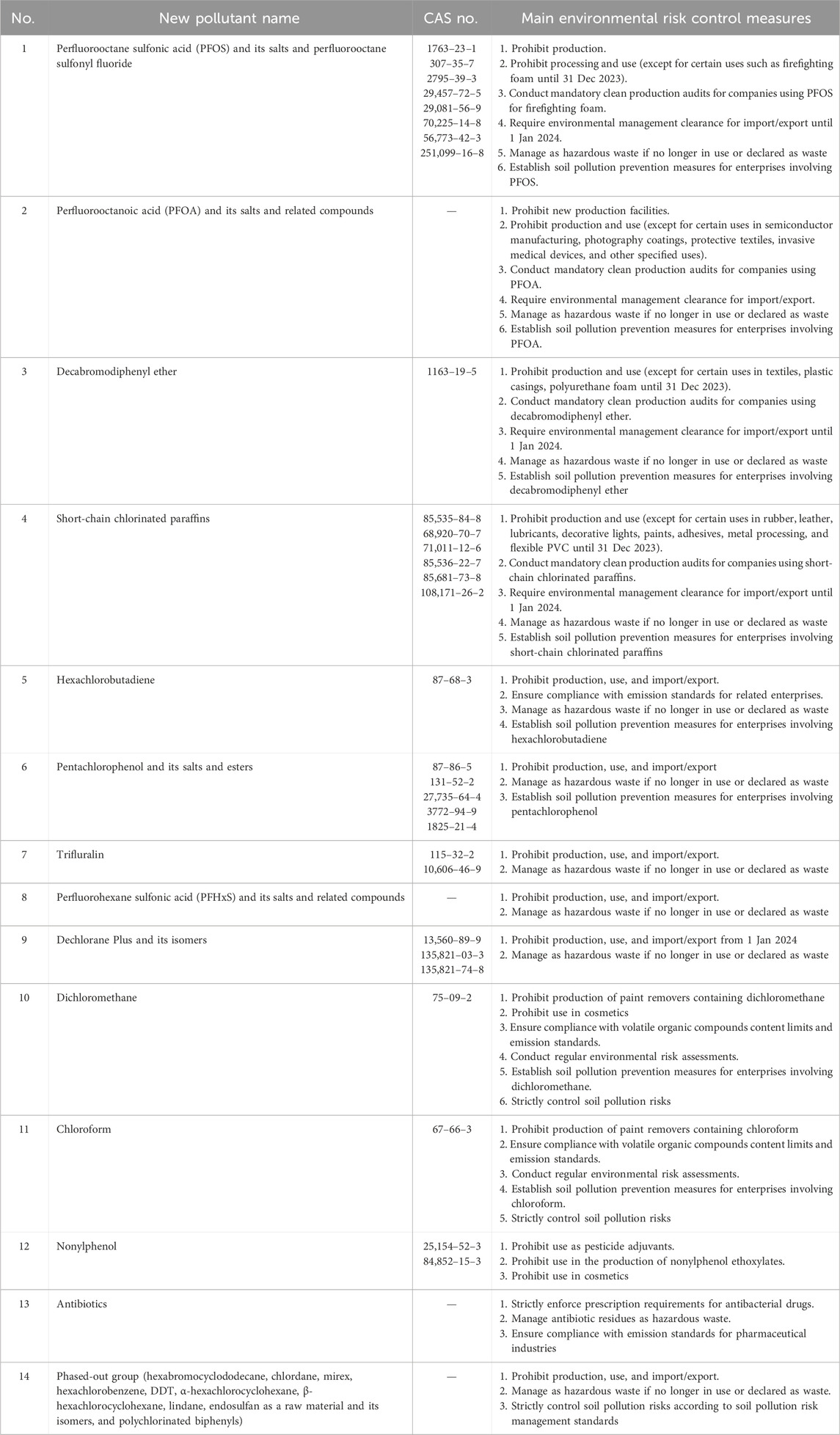
Table 12. 2023 List of Key Controlled New Pollutants posted by Ministry of Ecology and Environment of the People’s Republic of China (China, 2022).
The impact of emerging contaminants on the efficiency of wastewater and landfill leachate treatment systems presents a complex challenge that requires immediate and sustained attention. While these contaminants pose significant environmental and public health risks, they also provide an impetus for innovation in treatment technologies and strategies. Addressing this challenge will require a combination of technological advancement, regulatory action, public education, and international cooperation to ensure the safety and sustainability of water resources in the face of emerging environmental threats.
7.2 Future research
Embracing the future of landfill leachate treatment demands a multi-faceted approach: pioneering new materials for adsorption and catalysis, harnessing microbial genetic engineering for targeted bioremediation, and committing to process intensification for enhanced efficiency. These innovative pathways herald a new era of sustainability and effectiveness in managing complex waste challenges.
7.2.1 New materials for adsorption and catalysis
Future research in landfill leachate treatment increasingly focuses on developing new materials for adsorption and catalysis, driven by the need for more efficient, cost-effective, and sustainable treatment solutions. These materials are at the forefront of addressing complex pollutants, including emerging contaminants that traditional treatment methods struggle to remove. Advanced adsorbents, such as modified biochars, nanocomposites, and metal-organic frameworks (MOFs), are being explored for their high surface area, porosity, and tunable surface chemistry, which can significantly enhance the adsorption of pollutants (Zango et al., 2020). For instance, biochars produced from agricultural waste can be engineered at the nano-scale to improve their adsorption capacity for specific contaminants like pharmaceuticals and heavy metals (Bhandari et al., 2023). Catalytic materials are equally crucial for breaking down pollutants through AOPs. New photocatalysts, including quantum dots and graphitic carbon nitride (g-C3N4), offer the potential for more efficient light absorption and utilization, enabling the degradation of contaminants under visible light and thus reducing energy consumption (Wang et al., 2020). The development of these materials aims to enhance the efficiency and specificity of contaminant removal and minimize secondary pollution and the use of harsh chemicals in the treatment process (Ahmed et al., 2021). Research also focuses on the regeneration and reuse of these materials to ensure the sustainability of the treatment processes (Baskar et al., 2022). Innovative approaches to regenerate spent adsorbents and catalysts, such as thermal, chemical, and ultrasonic methods, are being studied to extend their life and reduce operational costs (Ferella, 2020). Overall, exploring new materials for adsorption and catalysis represents a dynamic and promising area of landfill leachate treatment, offering potential breakthroughs in efficiency, sustainability, and tackling a broader spectrum of pollutants.
7.2.2 Microbial genetic engineering
Microbial genetic engineering stands at the forefront of biotechnological innovations in landfill leachate treatment (Saeed et al., 2022). The natural biodegradation processes employed by microorganisms offer a sustainable and efficient means of removing organic pollutants from leachate (Pisharody et al., 2022). However, leachate’s complex and toxic nature often limits the effectiveness of native microbial communities (Nimonkar et al., 2022). Genetic engineering presents an opportunity to overcome these limitations by enhancing the metabolic capabilities of microorganisms, enabling them to degrade a wider array of contaminants, including recalcitrant and emerging pollutants (Haripriyan et al., 2022). The manipulation of microbial genomes involves introducing genes responsible for producing specific enzymes that can break down complex molecules or modifying existing metabolic pathways to increase their efficiency and specificity (Michalska et al., 2020). This can lead to the creation of microbial strains capable of rapid pollutant degradation, reduced biomass production, and enhanced tolerance to high pollutant concentrations and toxic substances (Maqsood et al., 2023). Ongoing research focuses on identifying key enzymatic pathways for the degradation of specific contaminants, developing genetic tools for efficient genome editing, and integrating genetically engineered microbes into existing treatment processes (Saravanan et al., 2022). Additionally, the ecological safety of releasing genetically modified organisms into environmental treatment systems is a critical consideration, necessitating rigorous containment strategies and risk assessments.
7.2.3 Process enhancement
Process intensification in landfill leachate treatment aims to enhance the efficiency and effectiveness of treatment processes by integrating and optimizing physical, chemical, and biological methods. The goal is to achieve more with less—reducing the footprint, energy consumption, and operational costs of treatment systems while maximizing pollutant removal efficiency (Cardoso et al., 2021). This can involve the development of novel reactor designs that facilitate enhanced contact between the leachate and treatment agents, using AOPs to break down complex organic molecules, and integrating membrane technologies for selective pollutant removal and water recovery (Titchou et al., 2021). Process intensification can leverage the synergies between different treatment modalities, such as combining adsorption processes with biodegradation to handle a broader range of contaminants more effectively.
Future research directions include exploring hybrid treatment systems that integrate emerging materials for adsorption and catalysis with genetically engineered microbial processes, developing energy-efficient AOPs, and optimizing process parameters through advanced modeling and control strategies. The aim is to develop compact, modular treatment systems that can be easily adapted to varying leachate compositions and volumes, ensuring robust and sustainable leachate management strategies for the future.
7.2.4 Circular economy and sustainability in future landfill leachate treatment
The circular economy and sustainability principles are increasingly influencing the development and adoption of future landfill leachate treatment technologies, steering them towards more environmentally friendly and resource-efficient solutions (Bandala et al., 2021). This shift is a response to the growing awareness of the environmental impacts of waste management practices and the urgent need to transition towards more sustainable models of production and consumption. The role of circular economy concepts in shaping future leachate treatment technologies is profound, focusing on waste minimization, resource recovery, and the closed-loop reuse of materials and water (Yusuf et al., 2020).
At the heart of the circular economy is reducing waste and preventing pollution (Dincă et al., 2022). Future landfill leachate treatment technologies are being designed with a preventative approach, aiming to minimize the generation of leachate and its associated contaminants at the source (Bandala et al., 2021). This can be achieved through better landfill design, including impermeable liners and covers and waste pre-treatment to reduce its leachate-generating potential. By addressing the problem at its source, these technologies contribute to a more sustainable and less resource-intensive waste management system. Another cornerstone of the circular economy is the recovery and reuse of resources, which plays a significant role in shaping leachate treatment technologies (Puntillo et al., 2021). Emerging technologies are not only aimed at treating leachate to meet environmental discharge standards but also at recovering valuable resources from it (Li and Liu, 2021). Nutrient recovery, for example, can transform leachate into a source of nitrogen and phosphorus for agricultural use (Kurniawan et al., 2021b). Similarly, advanced treatment processes can recover water for irrigation or industrial applications, contributing to water conservation and reducing the demand for freshwater resources (Meese et al., 2022). The recovery of energy in the form of biogas from the anaerobic treatment of leachate further underscores the potential of these technologies to contribute to a more sustainable and energy-efficient waste management system (An et al., 2023). The circular economy encourages a systemic view of waste management, promoting the integration of leachate treatment technologies within broader waste and water management systems (Smol et al., 2020). Future technologies will likely be characterized by their ability to integrate with other treatment and recovery processes, creating synergies that enhance overall efficiency and sustainability (Kehrein et al., 2020). For example, integrating leachate treatment with solid waste management processes can facilitate the co-treatment of organic waste and leachate, optimizing resource recovery and minimizing environmental impacts (Mishra et al., 2023).
Collectively, the influence of circular economy and sustainability principles on the future of landfill leachate treatment technologies is transformative, driving innovation towards solutions that are effective in treating leachate and minimizing waste, recovering resources, and integrating seamlessly into a circular and sustainable waste management framework. As these principles continue to gain traction, they will undoubtedly shape the development of more sustainable, efficient, and integrated leachate treatment technologies, aligning waste management practices with the broader goals of environmental sustainability and resource conservation.
8 Conclusion
The environmental impact of untreated leachate is significant, with potential contamination of groundwater, surface water, and overall ecosystem health, necessitating robust and dynamic treatment solutions.
This comprehensive review of landfill leachate treatment technologies presents a global perspective, focusing on the physicochemical properties of leachate, regulatory frameworks, and specific country examples. The study highlights the complex composition of landfill leachate, which includes a mix of organic and inorganic pollutants, heavy metals, and xenobiotic compounds. The review also underscores the importance of global regulatory frameworks in shaping landfill leachate management practices. International agreements, such as the Basel Convention and the Stockholm Convention, along with national regulations from countries like the United States, China, India, and Brazil, are setting standards and encouraging the adoption of advanced treatment technologies.
Key findings emphasize the evolution of conventional and advanced treatment technologies. Traditional methods such as physical, chemical, and biological treatments remain fundamental. Physical methods, including sedimentation and membrane filtration, effectively remove suspended solids and larger organic molecules. Chemical treatments like coagulation-flocculation and advanced oxidation processes are crucial for targeting dissolved pollutants and heavy metals. Biological treatments utilize microbial processes to degrade organic pollutants and are particularly effective for nutrient removal. Advanced treatment technologies, including MBRs, NF, RO, and AOPs), provide higher efficiency and are essential for meeting stringent regulatory standards. These technologies can remove a broader range of contaminants, including emerging pollutants such as pharmaceuticals and microplastics, which traditional methods may not adequately address. Economic considerations are also crucial in the treatment process. The high capital and operational costs of advanced leachate treatment systems pose significant challenges. Technologies such as RO, NF, and MBR are effective but require substantial investment in equipment, maintenance, and energy consumption. Addressing these economic challenges necessitates the development of cost-effective, energy-efficient solutions and the integrating of resource recovery practices, such as biogas production and water reuse, to offset operational costs. A case study from Changsha City illustrates the practical application and effectiveness of a comprehensive treatment system that integrates various technologies. This example provides valuable insights into designing integrated systems capable of meeting diverse regulatory requirements and addressing complex environmental challenges.
In conclusion, the continuous improvement of landfill leachate treatment technologies is vital. Adopting innovative and sustainable practices is essential to reduce the environmental footprint of landfills. Future research should focus on developing cost-effective, energy-efficient solutions and exploring emerging technologies for better pollutant removal. By emphasizing a global perspective and integrating multiple treatment processes, this review significantly contributes to advancing sustainable waste management practices and protecting environmental health.
Author contributions
JW: Conceptualization, Data curation, Formal Analysis, Resources, Software, Writing–original draft, Writing–review and editing. ZQ: Conceptualization, Project administration, Supervision, Writing–original draft, Writing–review and editing.
Funding
The author(s) declare that no financial support was received for the research, authorship, and/or publication of this article.
Conflict of interest
The authors declare that the research was conducted in the absence of any commercial or financial relationships that could be construed as a potential conflict of interest.
Publisher’s note
All claims expressed in this article are solely those of the authors and do not necessarily represent those of their affiliated organizations, or those of the publisher, the editors and the reviewers. Any product that may be evaluated in this article, or claim that may be made by its manufacturer, is not guaranteed or endorsed by the publisher.
References
Abdelfattah, I., and El-Shamy, A. M. (2024). Review on the escalating imperative of zero liquid discharge (ZLD) technology for sustainable water management and environmental resilience. J. Environ. Manag. 351, 119614. doi:10.1016/j.jenvman.2023.119614
Abdel-Shafy, H. I., Ibrahim, A. M., Al-Sulaiman, A. M., and Okasha, R. A. (2024). Landfill leachate: sources, nature, organic composition, and treatment: an environmental overview. Ain Shams Eng. J. 15, 102293. doi:10.1016/j.asej.2023.102293
Abujazar, M. S. S., Karaağaç, S. U., Abu Amr, S. S., Alazaiza, M. Y. D., and Bashir, M. J. K. (2022). Recent advancement in the application of hybrid coagulants in coagulation-flocculation of wastewater: a review. J. Clean. Prod. 345, 131133. doi:10.1016/j.jclepro.2022.131133
Abunama, T., Moodley, T., Abualqumboz, M., Kumari, S., and Bux, F. (2021a). Variability of leachate quality and polluting potentials in light of leachate pollution index (LPI) – a global perspective. Chemosphere 282, 131119. doi:10.1016/j.chemosphere.2021.131119
Abunama, T., Othman, F., and Nilam, T. I. T. (2021b). Comparison of landfill leachate generation and pollution potentials in humid and semi-arid climates. Int. J. Environ. Waste Manag. 27, 79–92. doi:10.1504/ijewm.2021.111906
Abyar, H., and Nowrouzi, M. (2023). A comprehensive framework for eco-environmental impact evaluation of wastewater treatment plants: integrating carbon footprint, energy footprint, toxicity, and economic assessments. J. Environ. Manag. 348, 119255. doi:10.1016/j.jenvman.2023.119255
Agency, U. S. E. P. (2024). Treatment standards for hazardous wastes subject to land disposal restrictions. Available at: https://www.epa.gov/hw/treatment-standards-hazardous-wastes-subject-land-disposal-restrictions.
Ahmad, K., Ghatak, H. R., and Ahuja, S. M. (2020). A review on photocatalytic remediation of environmental pollutants and H2 production through water splitting: a sustainable approach. Environ. Technol. and Innovation 19, 100893. doi:10.1016/j.eti.2020.100893
Ahmed, I. (2019). The Basel convention on the control of transboundary movements of hazardous wastes and their disposal: a legal misfit in global ship recycling jurisprudence. Wash. Int'l LJ 29, 411.
Ahmed, M. B., Zhou, J. L., Ngo, H. H., Guo, W., Thomaidis, N. S., and Xu, J. (2017). Progress in the biological and chemical treatment technologies for emerging contaminant removal from wastewater: a critical review. J. Hazard. Mater. 323, 274–298. doi:10.1016/j.jhazmat.2016.04.045
Ahmed, S. F., Mofijur, M., Nuzhat, S., Chowdhury, A. T., Rafa, N., Uddin, M. A., et al. (2021). Recent developments in physical, biological, chemical, and hybrid treatment techniques for removing emerging contaminants from wastewater. J. Hazard. Mater. 416, 125912. doi:10.1016/j.jhazmat.2021.125912
Al-Hazmi, H. E., Hassan, G. K., Kurniawan, T. A., Śniatała, B., Joseph, T. M., Majtacz, J., et al. (2024). Technological solutions to landfill management: towards recovery of biomethane and carbon neutrality. J. Environ. Manag. 354, 120414. doi:10.1016/j.jenvman.2024.120414
Al-Hazmi, H. E., Hassan, G. K., Maktabifard, M., Grubba, D., Majtacz, J., and Mąkinia, J. (2022). Integrating conventional nitrogen removal with anammox in wastewater treatment systems: microbial metabolism, sustainability and challenges. Environ. Res. 215, 114432. doi:10.1016/j.envres.2022.114432
Ali, M. E. A. (2021). Nanofiltration process for enhanced treatment of RO brine discharge. Membranes 11, 212. doi:10.3390/membranes11030212
Al-Yaqout, A., and Hamoda, M. F. (2020). Long-term temporal variations in characteristics of leachates from a closed landfill in an arid region. Water, Air, and Soil Pollut. 231, 319. doi:10.1007/s11270-020-04688-7
Ambaye, T. G., Vaccari, M., Van Hullebusch, E. D., Amrane, A., and Rtimi, S. (2021). Mechanisms and adsorption capacities of biochar for the removal of organic and inorganic pollutants from industrial wastewater. Int. J. Environ. Sci. Technol. 18, 3273–3294. doi:10.1007/s13762-020-03060-w
Amos, O., Abiodun, O. A., Olalekan, O., Opeodu, O. T., and Ademola, A. (2024). Investigation of efforts and problems in implementing the Basel convention on the control of transboundary movements of wastes and their disposal in Nigeria. Asian J. Geogr. Res. 7, 69–84. doi:10.9734/ajgr/2024/v7i1216
An, Z., Zhu, J., Zhang, M., Zhou, Y., Su, X., Lin, H., et al. (2023). Anaerobic membrane bioreactor for the treatment of high-strength waste/wastewater: a critical review and update. Chem. Eng. J. 470, 144322. doi:10.1016/j.cej.2023.144322
Anandan, S., Kumar Ponnusamy, V., and Ashokkumar, M. (2020). A review on hybrid techniques for the degradation of organic pollutants in aqueous environment. Ultrason. Sonochemistry 67, 105130. doi:10.1016/j.ultsonch.2020.105130
Andreottola, G., and Cannas, P. (2024). in Chemical and biological characteristics of landfill leachate. Editor R. C. R. S. T. H. Christensen (Elsevier Applied Science), 65–88.
Anjum, M., Anees, M., Qadeer, S., Khalid, A., Kumar, R., and Barakat, M. A. (2023). A recent progress in the leachate pretreatment methods coupled with anaerobic digestion for enhanced biogas production: feasibility, trends, and techno-economic evaluation. Int. J. Mol. Sci. 24, 763. doi:10.3390/ijms24010763
Aragaw, T. A. (2021). Functions of various bacteria for specific pollutants degradation and their application in wastewater treatment: a review. Int. J. Environ. Sci. Technol. 18, 2063–2076. doi:10.1007/s13762-020-03022-2
Asif, M. B., and Zhang, Z. (2021). Ceramic membrane technology for water and wastewater treatment: a critical review of performance, full-scale applications, membrane fouling and prospects. Chem. Eng. J. 418, 129481. doi:10.1016/j.cej.2021.129481
Ateş, H., and Argun, M. E. (2021). Advanced oxidation of landfill leachate: removal of micropollutants and identification of by-products. J. Hazard. Mater. 413, 125326. doi:10.1016/j.jhazmat.2021.125326
Awewomom, J., Dzeble, F., Takyi, Y. D., Ashie, W. B., Ettey, E. N. Y. O., Afua, P. E., et al. (2024). Addressing global environmental pollution using environmental control techniques: a focus on environmental policy and preventive environmental management. Discov. Environ. 2, 8. doi:10.1007/s44274-024-00033-5
Aziz, H. A., Zahari, M. S. M., Ahmed, Z., Ismail, S., Jaafar, I., Yusoff, M. S., et al. (2022). “Landfill leachate treatment,” in Solid waste engineering and management. Editors L. K. Wang, M.-H. S. Wang, and Y.-T. Hung (Cham: Springer International Publishing), 3, 435–548. doi:10.1007/978-3-030-96989-9_8
Aziz, K. H. H., Mustafa, F. S., Omer, K. M., Hama, S., Hamarawf, R. F., and Rahman, K. O. (2023). Heavy metal pollution in the aquatic environment: efficient and low-cost removal approaches to eliminate their toxicity: a review. RSC Adv. 13, 17595–17610. doi:10.1039/d3ra00723e
Babaei, S., Sabour, M. R., and Moftakhari Anasori Movahed, S. (2021). Combined landfill leachate treatment methods: an overview. Environ. Sci. Pollut. Res. 28, 59594–59607. doi:10.1007/s11356-021-16358-0
Babuponnusami, A., and Muthukumar, K. (2014). A review on Fenton and improvements to the Fenton process for wastewater treatment. J. Environ. Chem. Eng. 2, 557–572. doi:10.1016/j.jece.2013.10.011
Bakan, B., Bernet, N., Bouchez, T., Boutrou, R., Choubert, J.-M., Dabert, P., et al. (2022). Circular economy applied to organic residues and wastewater: research challenges. Waste Biomass Valorization 13, 1267–1276. doi:10.1007/s12649-021-01549-0
Bakhshoodeh, R., Alavi, N., Oldham, C., Santos, R. M., Babaei, A. A., Vymazal, J., et al. (2020). Constructed wetlands for landfill leachate treatment: a review. Ecol. Eng. 146, 105725. doi:10.1016/j.ecoleng.2020.105725
Bandala, E. R., Liu, A., Wijesiri, B., Zeidman, A. B., and Goonetilleke, A. (2021). Emerging materials and technologies for landfill leachate treatment: a critical review. Environ. Pollut. 291, 118133. doi:10.1016/j.envpol.2021.118133
Bashir, M. J. K., Ibrahim, N., Ismail, M. N., and Jaya, M. A. (2016). Physical treatment technologies for landfill leachate: performance and limitation, in Control and Treatment of Landfill Leachate for Sanitary Waste Disposal. H. Aziz & S. Amr (Eds.), IGI Glob., 250–285. doi:10.4018/978-1-4666-9610-5.ch011
Baskar, A. V., Bolan, N., Hoang, S. A., Sooriyakumar, P., Kumar, M., Singh, L., et al. (2022). Recovery, regeneration and sustainable management of spent adsorbents from wastewater treatment streams: a review. Sci. Total Environ. 822, 153555. doi:10.1016/j.scitotenv.2022.153555
Benassi, J. C., Sánchez, A. a.C., Da Costa, S. A., and Da Costa, S. M. (2021). Evaluation of color removal efficiencies and kinetic parameters of Fenton (H2O2/Fe2+) and photo-Fenton (H2O2/Fe2+/UV) processes in the treatment of a textile wastewater containing indigo blue/Avaliação da eficiência de remoção de cor e parâmetros cinéticos dos processos de Fenton (H2O2/Fe2+) e foto-Fenton (H2O2/Fe2+/UV) no tratamento de um efluente têxtil contendo corante azul índigo. Braz. J. Dev. 7, 102327–102347. doi:10.34117/bjdv7n11-044
Bergesen, H. O., Parmann, G., and Thommessen, Ø. B. (2018). “Convention on the control of transboundary movements of hazardous wastes and their disposal (Basel convention),” in Year book of international Co-operation on environment and development (Oxfordshire: Routledge), 87–89. doi:10.4324/9781315066547-15
Bhambore, N., and Suresh Kumar, M. (2022). Municipal solid waste generation, management scenarios, and leachate treatment using sequencing batch biofilter granular reactor. Process Saf. Environ. Prot. 167, 454–468. doi:10.1016/j.psep.2022.09.027
Bhambri, A., and Karn, S. K. (2020). Biotechnique for nitrogen and phosphorus removal: a possible insight. Chem. Ecol. 36, 785–809. doi:10.1080/02757540.2020.1777991
Bhandari, G., Gangola, S., Dhasmana, A., Rajput, V., Gupta, S., Malik, S., et al. (2023). Nano-biochar: recent progress, challenges, and opportunities for sustainable environmental remediation. Front. Microbiol. 14, 1214870. doi:10.3389/fmicb.2023.1214870
Blotevogel, J., Thagard, S. M., and Mahendra, S. (2023). Scaling up water treatment technologies for PFAS destruction: current status and potential for fit-for-purpose application. Curr. Opin. Chem. Eng. 41, 100944. doi:10.1016/j.coche.2023.100944
Bonaparte, R., Gross Beth, A., Gupta, R., Beech John, F., Griffin Leslie, M., and Phillips David, K. (2024). Twenty-year performance of a mixed LLRW/RCRA waste disposal facility.
Borah, P., Kumar, M., and Devi, P. (2020). “Chapter 2 - types of inorganic pollutants: metals/metalloids, acids, and organic forms,” in Inorganic pollutants in water. Editors P. Devi, P. Singh, and S. K. Kansal (Elsevier), 17–31.
Brennan, R. B., Healy, M. G., Morrison, L., Hynes, S., Norton, D., and Clifford, E. (2016). Management of landfill leachate: the legacy of European Union Directives. Waste Manag. 55, 355–363. doi:10.1016/j.wasman.2015.10.010
Britz, T. J. (2020). “Landfill leachate treatment,” in Microbiology of landfill sites (Boca Raton, FL: CRC Press), 131–164. doi:10.1201/9780367813475-5
Bunce, J. T., Ndam, E., Ofiteru, I. D., Moore, A., and Graham, D. W. (2018). A review of phosphorus removal technologies and their applicability to small-scale domestic wastewater treatment systems. Front. Environ. Sci. 6. doi:10.3389/fenvs.2018.00008
Cano, V., Vich, D. V., Andrade, H. H. B., Salinas, D. T. P., and Nolasco, M. A. (2020). Nitrification in multistage horizontal flow treatment wetlands for landfill leachate treatment. Sci. Total Environ. 704, 135376. doi:10.1016/j.scitotenv.2019.135376
Capodaglio, A. G. (2017). Integrated, decentralized wastewater management for resource recovery in rural and peri-urban areas. Resources 6, 22. doi:10.3390/resources6020022
Cardoso, B. J., Rodrigues, E., Gaspar, A. R., and Gomes, Á. (2021). Energy performance factors in wastewater treatment plants: a review. J. Clean. Prod. 322, 129107. doi:10.1016/j.jclepro.2021.129107
Castrillón, L., Fernández-Nava, Y., Ulmanu, M., Anger, I., and Marañón, E. (2010). Physico-chemical and biological treatment of MSW landfill leachate. Waste Manag. 30, 228–235. doi:10.1016/j.wasman.2009.09.013
Castro-Muñoz, R. (2020). The strategy of nanomaterials in polymeric membranes for water treatment: nanocomposite membranes. Tecnol. ciencias del agua 11, 410–436. doi:10.24850/j-tyca-2020-01-11
Chand, N., Kumar, K., and Suthar, S. (2022). Enhanced wastewater nutrients removal in vertical subsurface flow constructed wetland: effect of biochar addition and tidal flow operation. Chemosphere 286, 131742. doi:10.1016/j.chemosphere.2021.131742
Chen, H., Rose, M., Fleming, M., Souizi, S., Shashvatt, U., and Blaney, L. (2023). Recent advances in Donnan dialysis processes for water/wastewater treatment and resource recovery: a critical review. Chem. Eng. J. 455, 140522. doi:10.1016/j.cej.2022.140522
Chen, H., Xu, H., Zhong, C., Liu, M., Yang, L., He, J., et al. (2024). Treatment of landfill leachate by coagulation: a review. Sci. Total Environ. 912, 169294. doi:10.1016/j.scitotenv.2023.169294
Chen, W., Gu, Z., Ran, G., and Li, Q. (2021). Application of membrane separation technology in the treatment of leachate in China: a review. Waste Manag. 121, 127–140. doi:10.1016/j.wasman.2020.12.002
Cherni, Y., Elleuch, L., Messaoud, M., Kasmi, M., Chatti, A., and Trabelsi, I. (2021). Recent technologies for leachate treatment: a review. Euro-Mediterranean J. Environ. Integration 6, 79. doi:10.1007/s41207-021-00286-z
China, M. (2022). 2023 list of key controlled new pollutants. Available at: https://www.mee.gov.cn/xxgk2018/xxgk/xxgk02/202212/t20221230_1009167.html.
Chrispim, M. C., Scholz, M., and Nolasco, M. A. (2021). Biogas recovery for sustainable cities: a critical review of enhancement techniques and key local conditions for implementation. Sustain. Cities Soc. 72, 103033. doi:10.1016/j.scs.2021.103033
Clark, B., Sharma, N., Apraku, E., Dong, H., and Tarpeh, W. A. (2024). Ligand exchange adsorbents for selective phosphate and total ammonia nitrogen recovery from wastewaters. Accounts Mater. Res. 5, 492–504. doi:10.1021/accountsmr.3c00290
Daniel, A. N., Ekeleme, I. K., Onuigbo, C. M., Ikpeazu, V. O., and Obiekezie, S. O. (2021). Review on effect of dumpsite leachate to the environmental and public health implication. GSC Adv. Res. Rev. 7, 051–060. doi:10.30574/gscarr.2021.7.2.0097
Das, S., Singh, C. K., Sodhi, K. K., and Singh, V. K. (2023). Circular economy approaches for water reuse and emerging contaminant mitigation: innovations in water treatment. Environ. Dev. Sustain. doi:10.1007/s10668-023-04183-z
Da Silva, S. W., Welter, J. B., Albornoz, L. L., Heberle, A. N. A., Ferreira, J. Z., and Bernardes, A. M. (2021). Advanced electrochemical oxidation processes in the treatment of pharmaceutical containing water and wastewater: a review. Curr. Pollut. Rep. 7, 146–159. doi:10.1007/s40726-021-00176-6
Dave, D., Sarma, S., Parmar, P., Shukla, A., Goswami, D., Shukla, A., et al. (2020). Microbes as a boon for the bane of heavy metals. Environ. Sustain. 3, 233–255. doi:10.1007/s42398-020-00112-2
De, S., Hazra, T., and Dutta, A. (2019a). Sustainable treatment of municipal landfill leachate by combined association of air stripping, Fenton oxidation, and enhanced coagulation. Environ. Monit. Assess. 191, 49. doi:10.1007/s10661-018-7171-8
De, S., Hazra, T., and Dutta, A. (2019b). Treatment of landfill leachate by integrated sequence of air stripping, coagulation–flocculation and adsorption. Environ. Dev. Sustain. 21, 657–677. doi:10.1007/s10668-017-0053-3
De, S., Hazra, T., and Dutta, A. (2022). Application of integrated sequence of air stripping, coagulation flocculation, electrocoagulation and adsorption for sustainable treatment of municipal landfill leachate. Clean. Waste Syst. 3, 100033. doi:10.1016/j.clwas.2022.100033
De Almeida, R., De Souza Couto, J. M., Gouvea, R. M., De Almeida Oroski, F., Bila, D. M., Quintaes, B. R., et al. (2020). Nanofiltration applied to the landfill leachate treatment and preliminary cost estimation. Waste Manag. Res. 38, 1119–1128. doi:10.1177/0734242x20933333
Deng, L., Guo, W., Ngo, H. H., Zhang, X., Chen, C., Chen, Z., et al. (2022). Recent advances in attached growth membrane bioreactor systems for wastewater treatment. Sci. Total Environ. 808, 152123. doi:10.1016/j.scitotenv.2021.152123
Deng, S., Ngo, H. H., Guo, W., You, N., and Peng, S. (2023). “Chapter 15 - biological nutrient recovery from wastewater for circular economy,” in Current developments in biotechnology and bioengineering. Editors G. Mannina, A. Pandey, and R. Sirohi (Elsevier), 355–412.
Deng, Y., Zhu, X., Chen, N., Feng, C., Wang, H., Kuang, P., et al. (2020). Review on electrochemical system for landfill leachate treatment: performance, mechanism, application, shortcoming, and improvement scheme. Sci. Total Environ. 745, 140768. doi:10.1016/j.scitotenv.2020.140768
De Oliveira, M., Frihling, B. E. F., Velasques, J., Filho, F. J. C. M., Cavalheri, P. S., and Migliolo, L. (2020). Pharmaceuticals residues and xenobiotics contaminants: occurrence, analytical techniques and sustainable alternatives for wastewater treatment. Sci. Total Environ. 705, 135568. doi:10.1016/j.scitotenv.2019.135568
Dereli, R. K., Clifford, E., and Casey, E. (2021). Co-treatment of leachate in municipal wastewater treatment plants: critical issues and emerging technologies. Crit. Rev. Environ. Sci. Technol. 51, 1079–1128. doi:10.1080/10643389.2020.1745014
Dignac, M. F., Ginestet, P., Rybacki, D., Bruchet, A., Urbain, V., and Scribe, P. (2000). Fate of wastewater organic pollution during activated sludge treatment: nature of residual organic matter. Water Res. 34, 4185–4194. doi:10.1016/s0043-1354(00)00195-0
Dincă, G., Milan, A.-A., Andronic, M. L., Pasztori, A.-M., and Dincă, D. (2022). Does circular economy contribute to smart cities’ sustainable development? Int. J. Environ. Res. Public Health 19, 7627. doi:10.3390/ijerph19137627
Directive, W. F. (2003). Common implementation strategy for the water framework directive (2000/60/EC). Guid. Doc. 7.
Dogaris, I., Ammar, E., and Philippidis, G. P. (2020). Prospects of integrating algae technologies into landfill leachate treatment. World J. Microbiol. Biotechnol. 36, 39. doi:10.1007/s11274-020-2810-y
Ekins, P., and Zenghelis, D. (2021). The costs and benefits of environmental sustainability. Sustain. Sci. 16, 949–965. doi:10.1007/s11625-021-00910-5
El Batouti, M., Al-Harby, N. F., and Elewa, M. M. (2021). A review on promising membrane technology approaches for heavy metal removal from water and wastewater to solve water crisis. Water 13, 3241. doi:10.3390/w13223241
El Batouti, M., Alharby, N. F., and Elewa, M. M. (2022). Review of new approaches for fouling mitigation in membrane separation processes in water treatment applications. Separations 9, 1. doi:10.3390/separations9010001
El-Saadony, M. T., Saad, A. M., El-Wafai, N. A., Abou-Aly, H. E., Salem, H. M., Soliman, S. M., et al. (2023). Hazardous wastes and management strategies of landfill leachates: a comprehensive review. Environ. Technol. and Innovation 31, 103150. doi:10.1016/j.eti.2023.103150
Ergene, D., Aksoy, A., and Dilek Sanin, F. (2022). Comprehensive analysis and modeling of landfill leachate. Waste Manag. 145, 48–59. doi:10.1016/j.wasman.2022.04.030
Essien, J. P., Ikpe, D. I., Inam, E. D., Okon, A. O., Ebong, G. A., and Benson, N. U. (2022). Occurrence and spatial distribution of heavy metals in landfill leachates and impacted freshwater ecosystem: an environmental and human health threat. PLOS ONE 17, e0263279. doi:10.1371/journal.pone.0263279
Fan, G., Li, Z., Yan, Z., Wei, Z., Xiao, Y., Chen, S., et al. (2020). Operating parameters optimization of combined UF/NF dual-membrane process for brackish water treatment and its application performance in municipal drinking water treatment plant. J. Water Process Eng. 38, 101547. doi:10.1016/j.jwpe.2020.101547
Fang, D., Wang, J., Cui, D., Dong, X., Tang, C., Zhang, L., et al. (2021). Recent advances of landfill leachate treatment. J. Indian Inst. Sci. 101, 685–724. doi:10.1007/s41745-021-00262-0
Ferella, F. (2020). A review on management and recycling of spent selective catalytic reduction catalysts. J. Clean. Prod. 246, 118990. doi:10.1016/j.jclepro.2019.118990
Feria-Díaz, J. J., Correa-Mahecha, F., López-Méndez, M. C., Rodríguez-Miranda, J. P., and Barrera-Rojas, J. (2021). Recent desalination technologies by hybridization and integration with reverse osmosis: a review. Water 13, 1369. doi:10.3390/w13101369
Fitch, A., Balderas-Hernandez, P., and Ibanez, J. G. (2022). Electrochemical technologies combined with physical, biological, and chemical processes for the treatment of pollutants and wastes: a review. J. Environ. Chem. Eng. 10, 107810. doi:10.1016/j.jece.2022.107810
Fu, F., and Wang, Q. (2011). Removal of heavy metal ions from wastewaters: a review. J. Environ. Manag. 92, 407–418. doi:10.1016/j.jenvman.2010.11.011
Galavote, T., Cerqueira, A. F., Alves, R. B., Ramalho, J. C. M., Yamane, L. H., and Siman, R. R. (2022). Energy recovery technologies from municipal solid waste: enhancing Solid Waste Brazilian Policy. Rev. Bras.28. doi:10.47168/rbe.v28i1.652
Galinha, C. F., Sanches, S., and Crespo, J. G. (2018). “Chapter 6 - membrane bioreactors,” in Fundamental modelling of membrane systems. Editor P. Luis (Elsevier), 209–249.
Gerald, W., Foess, P. S., Williams, K., and Garrett, G. S. (1998). Cost and performance evaluation of BNR processes. Fla. WATER Resour. J.
Golwala, H., Saha, B., Zhang, X., Bolyard, S. C., He, Z., Novak, J. T., et al. (2022). Advancement and challenges in municipal landfill leachate treatment–the path forward. ACS ES&T Water 2, 1289–1300. doi:10.1021/acsestwater.2c00216
González-González, R. B., Sharma, P., Singh, S. P., Américo-Pinheiro, J. H. P., Parra-Saldívar, R., Bilal, M., et al. (2022). Persistence, environmental hazards, and mitigation of pharmaceutically active residual contaminants from water matrices. Sci. Total Environ. 821, 153329. doi:10.1016/j.scitotenv.2022.153329
Gopinath, K. P., Madhav, N. V., Krishnan, A., Malolan, R., and Rangarajan, G. (2020). Present applications of titanium dioxide for the photocatalytic removal of pollutants from water: a review. J. Environ. Manag. 270, 110906. doi:10.1016/j.jenvman.2020.110906
Gusain, R., Kumar, N., and Ray, S. S. (2020). Factors influencing the photocatalytic activity of photocatalysts in wastewater treatment. Photocatal. Adv. Oxid. Process. wastewater Treat., 229–270. doi:10.1002/9781119631422.ch8
Hai, F. I., Riley, T., Shawkat, S., Magram, S. F., and Yamamoto, K. (2014). Removal of pathogens by membrane bioreactors: a review of the mechanisms, influencing factors and reduction in chemical disinfectant dosing. Water 6, 3603–3630. doi:10.3390/w6123603
Han, M., Duan, X., Cao, G., Zhu, S., and Ho, S.-H. (2020). Graphitic nitride-catalyzed advanced oxidation processes (AOPs) for landfill leachate treatment: a mini review. Process Saf. Environ. Prot. 139, 230–240. doi:10.1016/j.psep.2020.04.046
Haripriyan, U., Gopinath, K. P., Arun, J., and Govarthanan, M. (2022). Bioremediation of organic pollutants: a mini review on current and critical strategies for wastewater treatment. Archives Microbiol. 204, 286. doi:10.1007/s00203-022-02907-9
Hasan, M. N., Altaf, M. M., Khan, N. A., Khan, A. H., Khan, A. A., Ahmed, S., et al. (2021). Recent technologies for nutrient removal and recovery from wastewaters: a review. Chemosphere 277, 130328. doi:10.1016/j.chemosphere.2021.130328
Hassan, I., Chowdhury, S. R., Prihartato, P. K., and Razzak, S. A. (2021). Wastewater treatment using constructed wetland: current trends and future potential. Processes 9, 1917. doi:10.3390/pr9111917
Haydar, S., Aziz, J. A., and Ahmad, M. S. (2007). Biological treatment of tannery wastewater using activated sludge process. Pak. J. Eng. Appl. Sci. 1, 61–66.
Hinds, R. D. (2022). “Summary and analysis of the resource conservation and recovery Act of 1976, as amended,” in Hazardous waste management (Boca Raton, FL: CRC Press), 79–97. doi:10.1201/9780429291050-5
Hou, D., Al-Tabbaa, A., O’connor, D., Hu, Q., Zhu, Y.-G., Wang, L., et al. (2023). Sustainable remediation and redevelopment of brownfield sites. Nat. Rev. Earth and Environ. 4, 271–286. doi:10.1038/s43017-023-00404-1
Huang, W., Song, B., Liang, J., Niu, Q., Zeng, G., Shen, M., et al. (2021). Microplastics and associated contaminants in the aquatic environment: a review on their ecotoxicological effects, trophic transfer, and potential impacts to human health. J. Hazard. Mater. 405, 124187. doi:10.1016/j.jhazmat.2020.124187
Huang, Y., Jeffrey, P., and Pidou, M. (2024). Municipal wastewater treatment with anaerobic membrane Bioreactors for non-potable reuse: a review. Crit. Rev. Environ. Sci. Technol. 54, 817–839. doi:10.1080/10643389.2023.2279886
Hudiburgh, G. W. (2020). “The clean water Act,” in Fundamentals of aquatic toxicology (Boca Raton, FL: CRC Press), 717–733. doi:10.1201/9781003075363-28
Hussain, K., Khan, N. A., Vambol, V., Vambol, S., Yeremenko, S., and Sydorenko, V. (2022). Advancement in Ozone base wastewater treatment technologies: brief review. Ecol. Quest. 33, 1–23. doi:10.12775/eq.2022.010
Hussein, M., Yoneda, K., Mohd-Zaki, Z., Amir, A., and Othman, N. (2021). Heavy metals in leachate, impacted soils and natural soils of different landfills in Malaysia: an alarming threat. Chemosphere 267, 128874. doi:10.1016/j.chemosphere.2020.128874
Iervolino, G., Zammit, I., Vaiano, V., and Rizzo, L. (2020). “Limitations and prospects for wastewater treatment by UV and visible-light-active heterogeneous photocatalysis: a critical review,” in Heterogeneous photocatalysis: recent advances. Editors M. J. Muñoz-Batista, A. Navarrete Muñoz, and R. Luque (Cham: Springer International Publishing), 225–264.
Ighalo, J. O., Omoarukhe, F. O., Ojukwu, V. E., Iwuozor, K. O., and Igwegbe, C. A. (2022). Cost of adsorbent preparation and usage in wastewater treatment: a review. Clean. Chem. Eng. 3, 100042. doi:10.1016/j.clce.2022.100042
Ilmasari, D., Kamyab, H., Yuzir, A., Riyadi, F. A., Khademi, T., Al-Qaim, F. F., et al. (2022). A review of the biological treatment of leachate: available technologies and future requirements for the circular economy implementation. Biochem. Eng. J. 187, 108605. doi:10.1016/j.bej.2022.108605
Japperi, N. S., Mohd Asri, Z. Z., Wan Bakar, W. Z., Dollah, A., Ahmad Fuad, M. F. I., and Che Mohamed Hussein, S. N. (2021). Review on landfill gas formation from leachate biodegradation. Malays. J. Chem. Eng. Technol. (MJCET) 4, 39–49. doi:10.24191/mjcet.v4i1.12719
Jin, T., Peydayesh, M., and Mezzenga, R. (2021). Membrane-based technologies for per- and poly-fluoroalkyl substances (PFASs) removal from water: removal mechanisms, applications, challenges and perspectives. Environ. Int. 157, 106876. doi:10.1016/j.envint.2021.106876
Kabuba, J., Lephallo, J., and Rutto, H. (2022). Comparison of various technologies used to eliminate nitrogen from wastewater: a review. J. Water Process Eng. 48, 102885. doi:10.1016/j.jwpe.2022.102885
Kamaruddin, M. A., Yusoff, M. S., Rui, L. M., Isa, A. M., Zawawi, M. H., and Alrozi, R. (2017). An overview of municipal solid waste management and landfill leachate treatment: Malaysia and Asian perspectives. Environ. Sci. Pollut. Res. 24, 26988–27020. doi:10.1007/s11356-017-0303-9
Kataki, S., Chatterjee, S., Vairale, M. G., Sharma, S., Dwivedi, S. K., and Gupta, D. K. (2021). Constructed wetland, an eco-technology for wastewater treatment: a review on various aspects of microbial fuel cell integration, low temperature strategies and life cycle impact of the technology. Renew. Sustain. Energy Rev. 148, 111261. doi:10.1016/j.rser.2021.111261
Kefeni, K. K., and Mamba, B. B. (2020). Photocatalytic application of spinel ferrite nanoparticles and nanocomposites in wastewater treatment: review. Sustain. Mater. Technol. 23, e00140. doi:10.1016/j.susmat.2019.e00140
Kehrein, P., Van Loosdrecht, M., Osseweijer, P., Garfí, M., Dewulf, J., and Posada, J. (2020). A critical review of resource recovery from municipal wastewater treatment plants–market supply potentials, technologies and bottlenecks. Environ. Sci. Water Res. and Technol. 6, 877–910. doi:10.1039/c9ew00905a
Keyikoglu, R., Karatas, O., Rezania, H., Kobya, M., Vatanpour, V., and Khataee, A. (2021). A review on treatment of membrane concentrates generated from landfill leachate treatment processes. Sep. Purif. Technol. 259, 118182. doi:10.1016/j.seppur.2020.118182
Khasawneh, O. F. S., Palaniandy, P., Kamaruddin, M. A., Aziz, H. A., and Hung, Y.-T. (2022). “Landfill leachate collection and characterization,” in Solid waste engineering and management. Editors L. K. Wang, M.-H. S. Wang, and Y.-T. Hung (Cham: Springer International Publishing), 2, 599–657. doi:10.1007/978-3-030-89336-1_9
Khoo, K. S., Tan, X., Show, P. L., Pal, P., Juan, J. C., Ling, T. C., et al. (2020). Treatment for landfill leachate via physicochemical approaches: an overview. Chem. Biochem. Eng. Q. 34, 1–24. doi:10.15255/cabeq.2019.1703
Kjeldsen, P., Barlaz, M. A., Rooker, A. P., Baun, A., Ledin, A., and Christensen, T. H. (2002). Present and long-term composition of msw landfill leachate: a review. Crit. Rev. Environ. Sci. Technol. 32, 297–336. doi:10.1080/10643380290813462
Kulikowska, D., and Klimiuk, E. (2008). The effect of landfill age on municipal leachate composition. Bioresour. Technol. 99, 5981–5985. doi:10.1016/j.biortech.2007.10.015
Kumar, S., Mukherjee, S., Chakrabarti, T., and Devotta, S. (2007). Hazardous waste management system in India: an overview. Crit. Rev. Environ. Sci. Technol. 38, 43–71. doi:10.1080/10643380701590356
Kumar, V., Sharma, N., Umesh, M., Chakraborty, P., Kaur, K., Duhan, L., et al. (2023). Micropollutants characteristics, fate, and sustainable removal technologies for landfill leachate: a technical perspective. J. Water Process Eng. 53, 103649. doi:10.1016/j.jwpe.2023.103649
Kundariya, N., Mohanty, S. S., Varjani, S., Hao Ngo, H., Wong, J., Taherzadeh, M. J., et al. (2021). A review on integrated approaches for municipal solid waste for environmental and economical relevance: monitoring tools, technologies, and strategic innovations. Bioresour. Technol. 342, 125982. doi:10.1016/j.biortech.2021.125982
Kurniawan, S. B., Ahmad, A., Imron, M. F., Abdullah, S. R. S., Hasan, H. A., Othman, A. R., et al. (2023). Performance of chemical-based vs bio-based coagulants in treating aquaculture wastewater and cost-benefit analysis. Pol. J. Environ. Stud. 32, 1177–1187. doi:10.15244/pjoes/156419
Kurniawan, T. A., Lo, W., Chan, G., and Sillanpää, M. E. T. (2010). Biological processes for treatment of landfill leachate. J. Environ. Monit. 12, 2032–2047. doi:10.1039/c0em00076k
Kurniawan, T. A., Lo, W.-H., and Chan, G. Y. S. (2006). Physico-chemical treatments for removal of recalcitrant contaminants from landfill leachate. J. Hazard. Mater. 129, 80–100. doi:10.1016/j.jhazmat.2005.08.010
Kurniawan, T. A., Singh, D., Avtar, R., Othman, M. H. D., Hwang, G. H., Albadarin, A. B., et al. (2021a). Resource recovery from landfill leachate: an experimental investigation and perspectives. Chemosphere 274, 129986. doi:10.1016/j.chemosphere.2021.129986
Kurniawan, T. A., Singh, D., Xue, W., Avtar, R., Othman, M. H. D., Hwang, G. H., et al. (2021b). Resource recovery toward sustainability through nutrient removal from landfill leachate. J. Environ. Manag. 287, 112265. doi:10.1016/j.jenvman.2021.112265
Kyriakopoulos, G. L. (2021). “The effect of immediate treatment for water quality: policies and protection perspectives,” in Chemical Lake restoration: technologies, innovations and economic perspectives. Editors M. G. Zamparas, and G. L. Kyriakopoulos (Cham: Springer International Publishing), 49–67.
Lee, T., Speth, T. F., and Nadagouda, M. N. (2022). High-pressure membrane filtration processes for separation of Per- and polyfluoroalkyl substances (PFAS). Chem. Eng. J. 431, 134023. doi:10.1016/j.cej.2021.134023
Li, H., and Liu, H. (2021). Treatment and recovery methods for leachate concentrate from landfill and incineration: a state-of-the-art review. J. Clean. Prod. 329, 129720. doi:10.1016/j.jclepro.2021.129720
Li, Y., Tang, F., Xu, D., and Xie, B. (2021). Advances in biological nitrogen removal of landfill leachate. Sustainability 13, 6236. doi:10.3390/su13116236
Lin, X., Ma, Y., Chen, T., Wang, L., Takaoka, M., Pan, S., et al. (2022). PCDD/Fs and heavy metals in the vicinity of landfill used for MSWI fly ash disposal: pollutant distribution and environmental impact assessment. Environ. Pollut. 312, 120083. doi:10.1016/j.envpol.2022.120083
Lindamulla, L., Nanayakkara, N., Othman, M., Jinadasa, S., Herath, G., and Jegatheesan, V. (2022). Municipal solid waste landfill leachate characteristics and their treatment options in tropical countries. Curr. Pollut. Rep. 8, 273–287. doi:10.1007/s40726-022-00222-x
Liu, J., Gu, Z., Wang, X., and Li, Q. (2022a). The molecular differences of young and mature landfill leachates: molecular composition, chemical property, and structural characteristic. Chemosphere 287, 132215. doi:10.1016/j.chemosphere.2021.132215
Liu, Q., Wang, X., Gao, M., Guan, Y., Wu, C., Wang, Q., et al. (2022b). Heavy metal leaching behaviour and long-term environmental risk assessment of cement-solidified municipal solid waste incineration fly ash in sanitary landfill. Chemosphere 300, 134571. doi:10.1016/j.chemosphere.2022.134571
Liu, Y., Wang, H., Cui, Y., and Chen, N. (2023). Removal of copper ions from wastewater: a review. Int. J. Environ. Res. Public Health 20, 3885. doi:10.3390/ijerph20053885
Liu, Y., Yuan, Y., Wang, Y., Ngo, H. H., and Wang, J. (2024). Research and application of active species based on high-valent iron for the degradation of pollutants: a critical review. Sci. Total Environ. 924, 171430. doi:10.1016/j.scitotenv.2024.171430
Liu, Y., Zhao, Y., and Wang, J. (2021). Fenton/Fenton-like processes with in-situ production of hydrogen peroxide/hydroxyl radical for degradation of emerging contaminants: advances and prospects. J. Hazard. Mater. 404, 124191. doi:10.1016/j.jhazmat.2020.124191
Loh, Z. Z., Zaidi, N. S., Syafiuddin, A., Yong, E. L., Boopathy, R., Hong Kueh, A. B., et al. (2021). Shifting from conventional to organic filter media in wastewater biofiltration treatment: a review. Appl. Sci. 11, 8650. doi:10.3390/app11188650
Luo, H., Zeng, Y., Cheng, Y., He, D., and Pan, X. (2020). Recent advances in municipal landfill leachate: a review focusing on its characteristics, treatment, and toxicity assessment. Sci. Total Environ. 703, 135468. doi:10.1016/j.scitotenv.2019.135468
Ma, S., Zhou, C., Pan, J., Yang, G., Sun, C., Liu, Y., et al. (2022). Leachate from municipal solid waste landfills in a global perspective: characteristics, influential factors and environmental risks. J. Clean. Prod. 333, 130234. doi:10.1016/j.jclepro.2021.130234
Magalhães, N. C., Silva, A. F. R., Cunha, P. V. M., Drewes, J. E., and Amaral, M. C. S. (2020). Role of nanofiltration or reverse osmosis integrated to ultrafiltration-anaerobic membrane bioreactor treating vinasse for the conservation of water and nutrients in the ethanol industry. J. Water Process Eng. 36, 101338. doi:10.1016/j.jwpe.2020.101338
Mahamuni, N. N., and Adewuyi, Y. G. (2010). Advanced oxidation processes (AOPs) involving ultrasound for waste water treatment: a review with emphasis on cost estimation. Ultrason. Sonochemistry 17, 990–1003. doi:10.1016/j.ultsonch.2009.09.005
Mani, S., Chowdhary, P., and Zainith, S. (2020). 2 - microbes mediated approaches for environmental waste management,” in Microorganisms for sustainable environment and health. Editors P. Chowdhary, A. Raj, D. Verma, and Y. Akhter (Elsevier), 17–36.
Maqsood, Q., Sumrin, A., Waseem, R., Hussain, M., Imtiaz, M., and Hussain, N. (2023). Bioengineered microbial strains for detoxification of toxic environmental pollutants. Environ. Res. 227, 115665. doi:10.1016/j.envres.2023.115665
Meese, A. F., Kim, D. J., Wu, X., Le, L., Napier, C., Hernandez, M. T., et al. (2022). Opportunities and challenges for industrial water treatment and reuse. ACS ES&T Eng. 2, 465–488. doi:10.1021/acsestengg.1c00282
Meng, F., Shi, B., Yang, F., and Zhang, H. (2007). Effect of hydraulic retention time on membrane fouling and biomass characteristics in submerged membrane bioreactors. Bioprocess Biosyst. Eng. 30, 359–367. doi:10.1007/s00449-007-0132-1
Mengesha, A., and Sahu, O. (2022). Sustainability of membrane separation technology on groundwater reverse osmosis process. Clean. Eng. Technol. 7, 100457. doi:10.1016/j.clet.2022.100457
Michalska, J., Piński, A., Żur, J., and Mrozik, A. (2020). Selecting bacteria candidates for the bioaugmentation of activated sludge to improve the aerobic treatment of landfill leachate. Water 12, 140. doi:10.3390/w12010140
Miklos, D. B., Remy, C., Jekel, M., Linden, K. G., Drewes, J. E., and Hübner, U. (2018). Evaluation of advanced oxidation processes for water and wastewater treatment – a critical review. Water Res. 139, 118–131. doi:10.1016/j.watres.2018.03.042
Mishra, S., Singh, V., Cheng, L., Hussain, A., and Ormeci, B. (2022). Nitrogen removal from wastewater: a comprehensive review of biological nitrogen removal processes, critical operation parameters and bioreactor design. J. Environ. Chem. Eng. 10, 107387. doi:10.1016/j.jece.2022.107387
Mishra, S., Singh, V., Ormeci, B., Hussain, A., Cheng, L., and Venkiteshwaran, K. (2023). Anaerobic–aerobic treatment of wastewater and leachate: a review of process integration, system design, performance and associated energy revenue. J. Environ. Manag. 327, 116898. doi:10.1016/j.jenvman.2022.116898
Mohammad, A., Singh, D. N., Podlasek, A., Osinski, P., and Koda, E. (2022). Leachate characteristics: potential indicators for monitoring various phases of municipal solid waste decomposition in a bioreactor landfill. J. Environ. Manag. 309, 114683. doi:10.1016/j.jenvman.2022.114683
Mojiri, A., Zhou, J. L., Ohashi, A., Ozaki, N., and Kindaichi, T. (2019). Comprehensive review of polycyclic aromatic hydrocarbons in water sources, their effects and treatments. Sci. Total Environ. 696, 133971. doi:10.1016/j.scitotenv.2019.133971
Mojiri, A., Zhou, J. L., Ratnaweera, H., Ohashi, A., Ozaki, N., Kindaichi, T., et al. (2020). Treatment of landfill leachate with different techniques: an overview. Water Reuse 11, 66–96. doi:10.2166/wrd.2020.079
Mor, S., and Ravindra, K. (2023). Municipal solid waste landfills in lower- and middle-income countries: environmental impacts, challenges and sustainable management practices. Process Saf. Environ. Prot. 174, 510–530. doi:10.1016/j.psep.2023.04.014
Morin-Crini, N., Lichtfouse, E., Liu, G., Balaram, V., Ribeiro, A. R. L., Lu, Z., et al. (2021). “Emerging contaminants: analysis, aquatic compartments and water pollution,” in Emerging contaminants vol. 1: occurrence and impact. Editors N. Morin-Crini, E. Lichtfouse, and G. Crini (Cham: Springer International Publishing), 1–111.
Mukherjee, S., Mukhopadhyay, S., Hashim, M. A., and Sen Gupta, B. (2015). Contemporary environmental issues of landfill leachate: assessment and remedies. Crit. Rev. Environ. Sci. Technol. 45, 472–590. doi:10.1080/10643389.2013.876524
Nath, A., and Debnath, A. (2022). A short review on landfill leachate treatment technologies. Mater. Today Proc. 67, 1290–1297. doi:10.1016/j.matpr.2022.09.109
Naveen, B. P., Mahapatra, D. M., Sitharam, T. G., Sivapullaiah, P. V., and Ramachandra, T. V. (2017). Physico-chemical and biological characterization of urban municipal landfill leachate. Environ. Pollut. 220, 1–12. doi:10.1016/j.envpol.2016.09.002
Neoh, C. H., Noor, Z. Z., Mutamim, N. S. A., and Lim, C. K. (2016). Green technology in wastewater treatment technologies: integration of membrane bioreactor with various wastewater treatment systems. Chem. Eng. J. 283, 582–594. doi:10.1016/j.cej.2015.07.060
Nguyen, A. T., and Le Tran, L. (2024). A review about the occurrence and effectiveness of conventional and advanced treatment technologies of persistent organic pollutants in surface water. Rev. Environ. Contam. Toxicol. 262, 11. doi:10.1007/s44169-024-00062-4
Nidheesh, P. V., Couras, C., Karim, A. V., and Nadais, H. (2022). A review of integrated advanced oxidation processes and biological processes for organic pollutant removal. Chem. Eng. Commun. 209, 390–432. doi:10.1080/00986445.2020.1864626
Nimonkar, Y. S., Kajale, S., Dake, M., Ranade, D. R., Yadav, K. K., Kumar, R., et al. (2022). A culture-based and culture-independent approach to the study of landfill leachate bacterial and archaeal communities. Anaerobe 77, 102626. doi:10.1016/j.anaerobe.2022.102626
Nourmohammadi, D., Esmaeeli, M. B., Akbarian, H., and Ghasemian, M. (2013). Nitrogen removal in a full-scale domestic wastewater treatment plant with activated sludge and trickling filter. J. Environ. Public Health 2013, 1–6. doi:10.1155/2013/504705
Nyabadza, A., Mccarthy, É., Makhesana, M., Heidarinassab, S., Plouze, A., Vazquez, M., et al. (2023). A review of physical, chemical and biological synthesis methods of bimetallic nanoparticles and applications in sensing, water treatment, biomedicine, catalysis and hydrogen storage. Adv. Colloid Interface Sci. 321, 103010. doi:10.1016/j.cis.2023.103010
Oakes, T. W., and Shank, K. E. (1979). Brief review of the resource conservation and recovery Act (RCRA) 1976.
Obradović, L., Bugarin, M., Stevanović, Z., Ljubojev, M., and Milijić, Z. (2010). Disposal of hazardous waste on the landfill in accordance with the Council Directive of the European Union on the landfill of waste no. 1999/31/EC. Co-Editor, (Bor, Serbia: irmbor.co.rs), 133.
Odabaşı, Ç., Dologlu, P., Gülmez, F., Kuşoğlu, G., and Çağlar, Ö. (2022). Investigation of the factors affecting reverse osmosis membrane performance using machine-learning techniques. Comput. and Chem. Eng. 159, 107669. doi:10.1016/j.compchemeng.2022.107669
O’dowd, K., and Pillai, S. C. (2020). Photo-Fenton disinfection at near neutral pH: process, parameter optimization and recent advances. J. Environ. Chem. Eng. 8, 104063. doi:10.1016/j.jece.2020.104063
Omoto, F. O. (2014). National implementation plan for the Stockholm convention on persistent organic pollutants.
Othman, N. H., Alias, N. H., Fuzil, N. S., Marpani, F., Shahruddin, M. Z., Chew, C. M., et al. (2022). A review on the use of membrane technology systems in developing countries. Membranes 12, 30. doi:10.3390/membranes12010030
Özdemir, A., Özkan, A., Günkaya, Z., and Banar, M. (2020). Decision-making for the selection of different leachate treatment/management methods: the ANP and PROMETHEE approaches. Environ. Sci. Pollut. Res. 27, 19798–19809. doi:10.1007/s11356-020-08524-7
Pan, S.-Y., Du, M. A., Huang, I. T., Liu, I. H., Chang, E. E., and Chiang, P.-C. (2015). Strategies on implementation of waste-to-energy (WTE) supply chain for circular economy system: a review. J. Clean. Prod. 108, 409–421. doi:10.1016/j.jclepro.2015.06.124
Pandis, P. K., Kalogirou, C., Kanellou, E., Vaitsis, C., Savvidou, M. G., Sourkouni, G., et al. (2022). Key points of advanced oxidation processes (AOPs) for wastewater, organic pollutants and pharmaceutical waste treatment: a mini review. ChemEngineering 6, 8. doi:10.3390/chemengineering6010008
Patel, N., Dhasmana, A., Kumari, S., Sharma, R., Nayanam, S., and Malik, S. (2023). “Nanofiltration applications for potable water, treatment, and reuse,” in Advanced and innovative approaches of environmental biotechnology in industrial wastewater treatment. Editor M. P. Shah (Singapore: Springer Nature Singapore), 135–146.
Peng, Y. (2017). Perspectives on technology for landfill leachate treatment. Arabian J. Chem. 10, S2567–S2574. doi:10.1016/j.arabjc.2013.09.031
Pérez, G., Gómez, P., Ortiz, I., and Urtiaga, A. (2022). Techno-economic assessment of a membrane-based wastewater reclamation process. Desalination 522, 115409. doi:10.1016/j.desal.2021.115409
Peters, C. D., Rantissi, T., Gitis, V., and Hankins, N. P. (2021). Retention of natural organic matter by ultrafiltration and the mitigation of membrane fouling through pre-treatment, membrane enhancement, and cleaning - a review. J. Water Process Eng. 44, 102374. doi:10.1016/j.jwpe.2021.102374
Pisharody, L., Gopinath, A., Malhotra, M., Nidheesh, P. V., and Kumar, M. S. (2022). Occurrence of organic micropollutants in municipal landfill leachate and its effective treatment by advanced oxidation processes. Chemosphere 287, 132216. doi:10.1016/j.chemosphere.2021.132216
Podlasek, A. (2023). Modeling leachate generation: practical scenarios for municipal solid waste landfills in Poland. Environ. Sci. Pollut. Res. 30, 13256–13269. doi:10.1007/s11356-022-23092-8
Pohl, A. (2020). Removal of heavy metal ions from water and wastewaters by sulfur-containing precipitation agents. Water, Air, and Soil Pollut. 231, 503. doi:10.1007/s11270-020-04863-w
Prieto-Espinoza, M., Susset, B., and Grathwohl, P. (2022). Long-term leaching behavior of organic and inorganic pollutants after wet processing of solid waste materials. Mater. (Basel) 15, 858. doi:10.3390/ma15030858
Priya, A. K., Muruganandam, M., Kumar, A., Senthilkumar, N., Shkir, M., Pandit, B., et al. (2024). Recent advances in microbial-assisted degradation and remediation of xenobiotic contaminants; challenges and future prospects. J. Water Process Eng. 60, 105106. doi:10.1016/j.jwpe.2024.105106
Puntillo, P., Gulluscio, C., Huisingh, D., and Veltri, S. (2021). Reevaluating waste as a resource under a circular economy approach from a system perspective: findings from a case study. Bus. Strategy Environ. 30, 968–984. doi:10.1002/bse.2664
Puyol, D., Batstone, D. J., Hülsen, T., Astals, S., Peces, M., and Krömer, J. O. (2017). Resource recovery from wastewater by biological technologies: opportunities, challenges, and prospects. Front. Microbiol. 7, 2106. doi:10.3389/fmicb.2016.02106
Qi, Y., Qiu, J., Tao, J., Zeng, J., Liu, R., Liu, H., et al. (2023). Temporal evolution of biogeochemical parameters and microbial communities in a landfill leachate pollution plume. Water, Air, and Soil Pollut. 234, 720. doi:10.1007/s11270-023-06747-1
Rahman, Z., and Singh, V. P. (2019). The relative impact of toxic heavy metals (THMs) (arsenic (As), cadmium (Cd), chromium (Cr)(VI), mercury (Hg), and lead (Pb)) on the total environment: an overview. Environ. Monit. Assess. 191, 419. doi:10.1007/s10661-019-7528-7
Rai, B., and Shrivastav, A. (2022). “Chapter 26 - removal of emerging contaminants in water treatment by nanofiltration and reverse osmosis,” in Development in wastewater treatment research and processes. Editors M. Shah, S. Rodriguez-Couto, and J. Biswas (Elsevier), 605–628.
Rajesh Banu, J., Merrylin, J., Kavitha, S., Yukesh Kannah, R., Selvakumar, P., Gopikumar, S., et al. (2021). Trends in biological nutrient removal for the treatment of low strength organic wastewaters. Curr. Pollut. Rep. 7, 1–30. doi:10.1007/s40726-020-00169-x
Randall, C. W., and Ubay Cokgor, E. (2000). Performance and economics of BNR plants in the chesapeake bay watershed, USA. Water Sci. Technol. 41, 21–28. doi:10.2166/wst.2000.0160
Rathi, B. S., and Kumar, P. S. (2021). Application of adsorption process for effective removal of emerging contaminants from water and wastewater. Environ. Pollut. 280, 116995. doi:10.1016/j.envpol.2021.116995
Rathod, S., Preetam, S., Pandey, C., and Bera, S. P. (2024). Exploring synthesis and applications of green nanoparticles and the role of nanotechnology in wastewater treatment. Biotechnol. Rep. 41, e00830. doi:10.1016/j.btre.2024.e00830
Ravindiran, G., Rajamanickam, S., Sivarethinamohan, S., Karupaiya Sathaiah, B., Ravindran, G., Muniasamy, S. K., et al. (2023). A review of the status, effects, prevention, and remediation of groundwater contamination for sustainable environment. Water 15, 3662. doi:10.3390/w15203662
Rekhate, C. V., and Srivastava, J. K. (2020). Recent advances in ozone-based advanced oxidation processes for treatment of wastewater- A review. Chem. Eng. J. Adv. 3, 100031. doi:10.1016/j.ceja.2020.100031
Remmas, N., Manfe, N., Zerva, I., Melidis, P., Raga, R., and Ntougias, S. (2023). A critical review on the microbial Ecology of landfill leachate treatment systems. Sustainability 15, 949. doi:10.3390/su15020949
Renou, S., Givaudan, J. G., Poulain, S., Dirassouyan, F., and Moulin, P. (2008). Landfill leachate treatment: review and opportunity. J. Hazard. Mater. 150, 468–493. doi:10.1016/j.jhazmat.2007.09.077
Righetto, I., Al-Juboori, R. A., Kaljunen, J. U., and Mikola, A. (2021). Multipurpose treatment of landfill leachate using natural coagulants – pretreatment for nutrient recovery and removal of heavy metals and micropollutants. J. Environ. Chem. Eng. 9, 105213. doi:10.1016/j.jece.2021.105213
Rout, P. R., Shahid, M. K., Dash, R. R., Bhunia, P., Liu, D., Varjani, S., et al. (2021a). Nutrient removal from domestic wastewater: a comprehensive review on conventional and advanced technologies. J. Environ. Manag. 296, 113246. doi:10.1016/j.jenvman.2021.113246
Rout, P. R., Zhang, T. C., Bhunia, P., and Surampalli, R. Y. (2021b). Treatment technologies for emerging contaminants in wastewater treatment plants: a review. Sci. Total Environ. 753, 141990. doi:10.1016/j.scitotenv.2020.141990
Saadatlu, E. A., Barzinpour, F., and Yaghoubi, S. (2023). A sustainable municipal solid waste system under leachate treatment impact along with leakage control and source separation. Process Saf. Environ. Prot. 169, 982–998. doi:10.1016/j.psep.2022.11.065
Sadala, S., Chakradhar, B., and Lakshmi, T. V. (2023). A review on treatment storage and disposal facilities (TSDFS) for effective management of hazardous wastes in India. J. Appl. Geochem. 25, 72–84.
Saeed, M. U., Hussain, N., Sumrin, A., Shahbaz, A., Noor, S., Bilal, M., et al. (2022). Microbial bioremediation strategies with wastewater treatment potentialities – a review. Sci. Total Environ. 818, 151754. doi:10.1016/j.scitotenv.2021.151754
Sahu, P. (2021). A comprehensive review of saline effluent disposal and treatment: conventional practices, emerging technologies, and future potential. Water Reuse 11, 33–65. doi:10.2166/wrd.2020.065
Saleh, T. A., Mustaqeem, M., and Khaled, M. (2022). Water treatment technologies in removing heavy metal ions from wastewater: a review. Environ. Nanotechnol. Monit. and Manag. 17, 100617. doi:10.1016/j.enmm.2021.100617
Salem, Z., Hamouri, K., Djemaa, R., and Allia, K. (2008). Evaluation of landfill leachate pollution and treatment. Desalination 220, 108–114. doi:10.1016/j.desal.2007.01.026
Santana, L. R. B., Walchhütter, S., Slavov, T. N. B., and Russo, P. T. (2022). Municipal solid waste management: analysing the principles of the Brazilian National Solid Waste Policy. Int. J. Environ. Waste Manag. 29, 391–405. doi:10.1504/ijewm.2022.124687
Saravanan, A., Kumar, P. S., Ramesh, B., and Srinivasan, S. (2022). Removal of toxic heavy metals using genetically engineered microbes: molecular tools, risk assessment and management strategies. Chemosphere 298, 134341. doi:10.1016/j.chemosphere.2022.134341
Saravanan, A., Senthil Kumar, P., Jeevanantham, S., Karishma, S., Tajsabreen, B., Yaashikaa, P. R., et al. (2021). Effective water/wastewater treatment methodologies for toxic pollutants removal: processes and applications towards sustainable development. Chemosphere 280, 130595. doi:10.1016/j.chemosphere.2021.130595
Sathya, R., Arasu, M. V., Al-Dhabi, N. A., Vijayaraghavan, P., Ilavenil, S., and Rejiniemon, T. S. (2023). Towards sustainable wastewater treatment by biological methods – a challenges and advantages of recent technologies. Urban Clim. 47, 101378. doi:10.1016/j.uclim.2022.101378
Saxena, V., Kumar Padhi, S., Kumar Dikshit, P., and Pattanaik, L. (2022). Recent developments in landfill leachate treatment: aerobic granular reactor and its future prospects. Environ. Nanotechnol. Monit. and Manag. 18, 100689. doi:10.1016/j.enmm.2022.100689
Schiopu, A.-M., and Gavrilescu, M. (2010). Municipal solid waste landfilling and treatment of resulting liquid effluents. Environ. Eng. and Manag. J. (EEMJ) 9, 993–1019. doi:10.30638/eemj.2010.133
Senathirajah, K., and Palanisami, T. (2023). Strategies to reduce risk and mitigate impacts of disaster: increasing water quality resilience from microplastics in the water supply system. ACS ES&T Water 3, 2816–2834. doi:10.1021/acsestwater.3c00206
Sengar, A., and Vijayanandan, A. (2022). Effects of pharmaceuticals on membrane bioreactor: review on membrane fouling mechanisms and fouling control strategies. Sci. Total Environ. 808, 152132. doi:10.1016/j.scitotenv.2021.152132
Sengupta, S., and Pal, C. K. (2021). “Chemistry in wastewater treatment,” in Advanced materials and technologies for wastewater treatment (Boca Raton, FL: CRC Press), 129–152. doi:10.1201/9781003138303-7
Shahid, M. K., Kashif, A., Fuwad, A., and Choi, Y. (2021). Current advances in treatment technologies for removal of emerging contaminants from water – a critical review. Coord. Chem. Rev. 442, 213993. doi:10.1016/j.ccr.2021.213993
Sharma, A., Grewal, A. S., Sharma, D., and Srivastav, A. L. (2023). “Chapter 3 - heavy metal contamination in water: consequences on human health and environment,” in Metals in water. Editors S. K. Shukla, S. Kumar, S. Madhav, and P. K. Mishra (Amsterdam, Netherlands: Elsevier), 39–52. doi:10.1016/B978-0-323-95919-3.00015-X
Sharma, G., Sharma, S., Kumar, A., Lai, C. W., Naushad, M., Iqbal, J., et al. (2022). Activated carbon as superadsorbent and sustainable material for diverse applications. Adsorpt. Sci. and Technol. 2022, 4184809. doi:10.1155/2022/4184809
Sharma, I. (2020). “Bioremediation techniques for polluted environment: concept, advantages, limitations, and prospects,” in Trace metals in the environment-new approaches and recent advances (IntechOpen).
Sharma, M., Dhiware, P., Laddha, H., Janu, V. C., and Gupta, R. (2024). Harnessing magnetically separable iron based adsorbents for enhanced uranium adsorption. Coord. Chem. Rev. 508, 215766. doi:10.1016/j.ccr.2024.215766
Shen, Z., Kuang, Y., Zhou, S., Zheng, J., and Ouyang, G. (2023). Preparation of magnetic adsorbent and its adsorption removal of pollutants: an overview. TrAC Trends Anal. Chem. 167, 117241. doi:10.1016/j.trac.2023.117241
Shi, L., Chen, H., Meng, H., Cheng, R., Dai, J., Zheng, X., et al. (2021). How environmental policy impacts technology adoption: a case of landfill leachate. J. Clean. Prod. 310, 127484. doi:10.1016/j.jclepro.2021.127484
Siddiqi, S. A., Al-Mamun, A., Baawain, M. S., and Sana, A. (2022). A critical review of the recently developed laboratory-scale municipal solid waste landfill leachate treatment technologies. Sustain. Energy Technol. Assessments 52, 102011. doi:10.1016/j.seta.2022.102011
Sil, A., and Kumar, S. (2017). “17 - landfill leachate treatment,” in Current developments in biotechnology and bioengineering. Editors J. W. C. Wong, R. D. Tyagi, and A. Pandey (Elsevier), 391–406.
Singh, V., Ormeci, B., Mishra, S., and Hussain, A. (2022). Simultaneous partial Nitrification, ANAMMOX and denitrification (SNAD) – a review of critical operating parameters and reactor configurations. Chem. Eng. J. 433, 133677. doi:10.1016/j.cej.2021.133677
Smol, M., Adam, C., and Preisner, M. (2020). Circular economy model framework in the European water and wastewater sector. J. Material Cycles Waste Manag. 22, 682–697. doi:10.1007/s10163-019-00960-z
Soltani, F., Navidjouy, N., and Rahimnejad, M. (2022). A review on bio-electro-Fenton systems as environmentally friendly methods for degradation of environmental organic pollutants in wastewater. RSC Adv. 12, 5184–5213. doi:10.1039/d1ra08825d
Song, W., Lee, L. Y., and Ng, H. Y. (2020). “Chapter 21 - nanofiltration and reverse osmosis processes for the removal of micro-pollutants,” in Current developments in biotechnology and bioengineering. Editors S. Varjani, A. Pandey, R. D. Tyagi, H. H. Ngo, and C. Larroche (Elsevier), 527–552.
Srivastava, A., Dutta, S., Ahuja, S., and Sharma, R. K. (2021). “Chapter 15 - green chemistry: key to reducing waste and improving water quality,” in Handbook of water purity and quality. Editor S. Ahuja 2nd Edn (Amsterdam: Academic Press), 359–407.
Srivastava, A., Singh, R., Rajput, V. D., Minkina, T., Agarwal, S., and Garg, M. C. (2022). A systematic approach towards optimization of brackish groundwater treatment using nanofiltration (NF) and reverse osmosis (RO) hybrid membrane filtration system. Chemosphere 303, 135230. doi:10.1016/j.chemosphere.2022.135230
Su, B., Liu, Q., Liang, H., Zhou, X., Zhang, Y., Liu, G., et al. (2022). Simultaneous partial nitrification, anammox, and denitrification in an upflow microaerobic membrane bioreactor treating middle concentration of ammonia nitrogen wastewater with low COD/TN ratio. Chemosphere 295, 133832. doi:10.1016/j.chemosphere.2022.133832
Subiza-Pérez, M., Zabala, A., Groten, D., Vozmediano, L., Juan, C. S., and Ibarluzea, J. (2023). Waste-to-energy risk perception typology: health, politics and environmental impacts. J. Risk Res. 26, 1101–1118. doi:10.1080/13669877.2023.2259402
Sundui, B., Ramirez Calderon, O. A., Abdeldayem, O. M., Lázaro-Gil, J., Rene, E. R., and Sambuu, U. (2021). Applications of machine learning algorithms for biological wastewater treatment: updates and perspectives. Clean Technol. Environ. Policy 23, 127–143. doi:10.1007/s10098-020-01993-x
Teh, C. Y., Budiman, P. M., Shak, K. P. Y., and Wu, T. Y. (2016). Recent advancement of coagulation–flocculation and its application in wastewater treatment. Industrial and Eng. Chem. Res. 55, 4363–4389. doi:10.1021/acs.iecr.5b04703
Teng, C., and Chen, W. (2023). Technologies for the treatment of emerging contaminants in landfill leachate. Curr. Opin. Environ. Sci. and Health 31, 100409. doi:10.1016/j.coesh.2022.100409
Teng, C., Zhou, K., Peng, C., and Chen, W. (2021). Characterization and treatment of landfill leachate: a review. Water Res. 203, 117525. doi:10.1016/j.watres.2021.117525
Tenodi, S., Krčmar, D., Agbaba, J., Zrnić, K., Radenović, M., Ubavin, D., et al. (2020). Assessment of the environmental impact of sanitary and unsanitary parts of a municipal solid waste landfill. J. Environ. Manag. 258, 110019. doi:10.1016/j.jenvman.2019.110019
Tian, J., Zhao, X., Gao, S., Wang, X., and Zhang, R. (2021). Progress in research and application of nanofiltration (NF) technology for brackish water treatment. Membranes 11, 662. doi:10.3390/membranes11090662
Titchou, F. E., Zazou, H., Afanga, H., El Gaayda, J., Ait Akbour, R., Nidheesh, P. V., et al. (2021). Removal of organic pollutants from wastewater by advanced oxidation processes and its combination with membrane processes. Chem. Eng. Process. - Process Intensif. 169, 108631. doi:10.1016/j.cep.2021.108631
Torretta, V., Ferronato, N., Katsoyiannis, I. A., Tolkou, A. K., and Airoldi, M. (2017). Novel and conventional technologies for landfill leachates treatment: a review. Sustainability 9, 9. doi:10.3390/su9010009
Tripathi, S., and Hussain, T. (2022). “Chapter 7 - water and wastewater treatment through ozone-based technologies,” in Development in wastewater treatment research and processes. Editors M. Shah, S. Rodriguez-Couto, and J. Biswas (Elsevier), 139–172.
Upadhyay, A., Singh, R., Talwar, P., Verma, N., Ahire, P. D., Khatri, H., et al. (2023). Insights into sustainable resource and energy recovery from leachate towards emission mitigation for environmental management: a critical approach. J. Environ. Manag. 343, 118219. doi:10.1016/j.jenvman.2023.118219
Urošević, T., and Trivunac, K. (2020). “Chapter 3 - achievements in low-pressure membrane processes microfiltration (MF) and ultrafiltration (UF) for wastewater and water treatment,” in Current trends and future developments on (bio-) membranes. Editors A. Basile, and K. Ghasemzadeh (Elsevier), 67–107.
Valdés, H., Saavedra, A., Flores, M., Vera-Puerto, I., Aviña, H., and Belmonte, M. (2021). Reverse osmosis concentrate: physicochemical characteristics, environmental impact, and technologies. Membranes 11, 753. doi:10.3390/membranes11100753
Venkatesh Reddy, C., Kumar, R., Chakrabortty, P., Karmakar, B., Pottipati, S., Kundu, A., et al. (2024). A critical science mapping approach on removal mechanism and pathways of per- and poly-fluoroalkyl substances (PFAS) in water and wastewater: a comprehensive review. Chem. Eng. J. 492, 152272. doi:10.1016/j.cej.2024.152272
Wang, D., Zhang, Y., Dong, H., Chen, H., and Sengupta, A. (2024). Ion exchange enabled selective separation from decontamination to desalination to decarbonization: recent advances and opportunities. Environ. Sci. Water Res. and Technol. 10, 1319–1334. doi:10.1039/d4ew00125g
Wang, J., and Chen, H. (2020). Catalytic ozonation for water and wastewater treatment: recent advances and perspective. Sci. Total Environ. 704, 135249. doi:10.1016/j.scitotenv.2019.135249
Wang, J., and Zhuan, R. (2020). Degradation of antibiotics by advanced oxidation processes: an overview. Sci. Total Environ. 701, 135023. doi:10.1016/j.scitotenv.2019.135023
Wang, L. K., Wang, M.-H. S., Shammas, N. K., and Hahn, H. H. (2021). “Physicochemical treatment consisting of chemical coagulation, precipitation, sedimentation, and flotation,” in Integrated natural resources research. Editors L. K. Wang, M.-H. S. Wang, and Y.-T. Hung (Cham: Springer International Publishing), 265–397.
Wang, T., Nie, C., Ao, Z., Wang, S., and An, T. (2020). Recent progress in gC 3 N 4 quantum dots: synthesis, properties and applications in photocatalytic degradation of organic pollutants. J. Mater. Chem. A 8, 485–502. doi:10.1039/c9ta11368a
Waqas, S., Bilad, M. R., Man, Z. B., Klaysom, C., Jaafar, J., and Khan, A. L. (2020). An integrated rotating biological contactor and membrane separation process for domestic wastewater treatment. Alexandria Eng. J. 59, 4257–4265. doi:10.1016/j.aej.2020.07.029
Wdowczyk, A., and Szymańska-Pulikowska, A. (2021). Comparison of landfill leachate properties by LPI and phytotoxicity-A case study. Front. Environ. Sci. 9. doi:10.3389/fenvs.2021.693112
Welle Environmental Protection Technology Group Co., L (2024). Changsha MSW plant leachate treatment project cluster. Available at: https://www.wellegroup.com/cases/detail?id=234.
Wijekoon, P., Koliyabandara, P. A., Cooray, A. T., Lam, S. S., Athapattu, B. C. L., and Vithanage, M. (2022). Progress and prospects in mitigation of landfill leachate pollution: risk, pollution potential, treatment and challenges. J. Hazard. Mater. 421, 126627. doi:10.1016/j.jhazmat.2021.126627
Wu, D., Wang, T., Huang, X., Dolfing, J., and Xie, B. (2015a). Perspective of harnessing energy from landfill leachate via microbial fuel cells: novel biofuels and electrogenic physiologies. Appl. Microbiol. Biotechnol. 99, 7827–7836. doi:10.1007/s00253-015-6857-x
Wu, S., Wallace, S., Brix, H., Kuschk, P., Kirui, W. K., Masi, F., et al. (2015b). Treatment of industrial effluents in constructed wetlands: challenges, operational strategies and overall performance. Environ. Pollut. 201, 107–120. doi:10.1016/j.envpol.2015.03.006
Xu, X., Chen, G., Wei, J., Qin, Y., Tang, R., Wang, L., et al. (2024). Effects of competitive cations and dissolved organic matter on ammonium exchange and up-concentration properties of ion exchangers from domestic wastewater under multicycle exchange - regeneration operation. Sep. Purif. Technol. 345, 127274. doi:10.1016/j.seppur.2024.127274
Yadav, D., Karki, S., and Ingole, P. G. (2022). Current advances and opportunities in the development of nanofiltration (NF) membranes in the area of wastewater treatment, water desalination, biotechnological and pharmaceutical applications. J. Environ. Chem. Eng. 10, 108109. doi:10.1016/j.jece.2022.108109
Yadav, G., Mishra, A., Ghosh, P., Sindhu, R., Vinayak, V., and Pugazhendhi, A. (2021). Technical, economic and environmental feasibility of resource recovery technologies from wastewater. Sci. Total Environ. 796, 149022. doi:10.1016/j.scitotenv.2021.149022
Yang, C., Fu, T., Wang, H., Chen, R., Wang, B., He, T., et al. (2021a). Removal of organic pollutants by effluent recirculation constructed wetlands system treating landfill leachate. Environ. Technol. and Innovation 24, 101843. doi:10.1016/j.eti.2021.101843
Yang, L., Hu, W., Chang, Z., Liu, T., Fang, D., Shao, P., et al. (2021b). Electrochemical recovery and high value-added reutilization of heavy metal ions from wastewater: recent advances and future trends. Environ. Int. 152, 106512. doi:10.1016/j.envint.2021.106512
Yang, Y., Demeestere, K., and Van Hulle, S. (2021c). Ozone-based advanced oxidation of biologically treated landfill leachate: oxidation efficiency, mechanisms, and surrogate-based monitoring for bulk organics. J. Environ. Chem. Eng. 9, 106459. doi:10.1016/j.jece.2021.106459
Yang, Z., Lin, S., Ye, L., Qu, D., Yang, H., Chang, H., et al. (2024). Landfill leachate treatment by direct contact membrane distillation: impacts of landfill age on contaminant removal performance, membrane fouling and scaling. Desalination 577, 117407. doi:10.1016/j.desal.2024.117407
Younas, F., Mustafa, A., Farooqi, Z. U., Wang, X., Younas, S., Mohy-Ud-Din, W., et al. (2021). Current and emerging adsorbent technologies for wastewater treatment: trends, limitations, and environmental implications. Water 13, 215. doi:10.3390/w13020215
Yusuf, A., Sodiq, A., Giwa, A., Eke, J., Pikuda, O., De Luca, G., et al. (2020). A review of emerging trends in membrane science and technology for sustainable water treatment. J. Clean. Prod. 266, 121867. doi:10.1016/j.jclepro.2020.121867
Zakaria, S. N. F., Aziz, H. A., Hung, Y.-T., Wang, M.-H. S., and Wang, L. K. (2023). “Treatment of hazardous sludge from water and wastewater treatment plants,” in Industrial waste engineering. Editors L. K. Wang, M.-H. S. Wang, and Y.-T. Hung (Cham: Springer International Publishing), 1–41.
Zamri, M. F. M. A., Hasmady, S., Akhiar, A., Ideris, F., Shamsuddin, A. H., Mofijur, M., et al. (2021). A comprehensive review on anaerobic digestion of organic fraction of municipal solid waste. Renew. Sustain. Energy Rev. 137, 110637. doi:10.1016/j.rser.2020.110637
Zango, Z. U., Jumbri, K., Sambudi, N. S., Ramli, A., Abu Bakar, N. H., Saad, B., et al. (2020). A critical review on metal-organic frameworks and their composites as advanced materials for adsorption and photocatalytic degradation of emerging organic pollutants from wastewater. Polymers 12, 2648. doi:10.3390/polym12112648
Zarei, M. (2020). Wastewater resources management for energy recovery from circular economy perspective. Water-Energy Nexus 3, 170–185. doi:10.1016/j.wen.2020.11.001
Zhao, Y., Tong, T., Wang, X., Lin, S., Reid, E. M., and Chen, Y. (2021). Differentiating solutes with precise nanofiltration for next generation environmental separations: a review. Environ. Sci. and Technol. 55, 1359–1376. doi:10.1021/acs.est.0c04593
Zheng, Z., Li, J., Ma, J., Du, J., Bian, W., Li, Y., et al. (2016). Nitrogen removal via simultaneous partial nitrification, anammox and denitrification (SNAD) process under high DO condition. Biodegradation 27, 195–208. doi:10.1007/s10532-016-9766-5
Zhu, T.-T., Su, Z.-X., Lai, W.-X., Zhang, Y.-B., and Liu, Y.-W. (2021). Insights into the fate and removal of antibiotics and antibiotic resistance genes using biological wastewater treatment technology. Sci. Total Environ. 776, 145906. doi:10.1016/j.scitotenv.2021.145906
Keywords: landfill leachate, treatment technologies, membrane technologies, advanced oxidation processes, waste treatment
Citation: Wang J and Qiao Z (2024) A comprehensive review of landfill leachate treatment technologies. Front. Environ. Sci. 12:1439128. doi: 10.3389/fenvs.2024.1439128
Received: 27 May 2024; Accepted: 20 August 2024;
Published: 02 September 2024.
Edited by:
Vassilis Inglezakis, University of Strathclyde, United KingdomReviewed by:
Alessandro Abbà, University of Brescia, ItalyAleksandra Wdowczyk, Wroclaw University of Environmental and Life Sciences, Poland
Tumpa Hazra, Jadavpur University, India
Copyright © 2024 Wang and Qiao. This is an open-access article distributed under the terms of the Creative Commons Attribution License (CC BY). The use, distribution or reproduction in other forums is permitted, provided the original author(s) and the copyright owner(s) are credited and that the original publication in this journal is cited, in accordance with accepted academic practice. No use, distribution or reproduction is permitted which does not comply with these terms.
*Correspondence: Zhen Qiao, qiaozhen@yonsei.ac.kr