- 1Qingyang Prefectural Center for Disease Control and Prevention, Qingyang, Gansu, China
- 2Qingyang People’s Hospital, Qingyang, Gansu, China
In this research, graphene oxide (GO) as an adsorbent was used to remove norfloxacin and ceftriaxone antibiotics from aqueous solutions. All environmental factors affecting removal (e.g., pH, adsorbent mass, contact time, and concentration) were optimized in a discontinuous system. The design of experiments and the optimization of variables were carried out using the response surface method (RSM). The results of the analysis of variance (ANOVA) and the regression coefficients of the quadratic terms indicated that the responses were significantly affected by all the studied variables (P < 0.05). Also, the quadratic polynomial model results corresponded to empirical data with a high coefficient of determination (i.e., R2 ˃ 0.99 for both antibiotics). Besides, the adjusted R2 (R2-adj ˃ 0.98 for both antibiotics) was close to R2 values, indicating a good and acceptable statistical model. According to the results, the optimal removal of antibiotics by GO occurs in conditions of pH of 8, 0.034 g of the adsorbent mass, a sonication time of 20 min, and a concentration of 25 mg L−1. In these conditions, the maximum removal efficiencies of ceftriaxone and norfloxacin were equal to 95.87% and 98.64%, respectively. GO was recovered in five adsorption/desorption processes, and the removal efficiency declined very slightly after using the adsorbent for five cycles. Therefore, it is concluded that GO is an efficient and acceptable adsorbent for removing ceftriaxone and norfloxacin from aqueous environments.
1 Introduction
The presence of pollutants of organic origin in water resources is considered a serious threat to the environment and human health (Singh et al., 2022). Pharmaceutical compounds account for a type of these pollutants entering surface and underground water sources, mainly through sewage and urban/industrial effluents. Due to their high stability, the presence of pharmaceutical substances in the environment not only causes disturbances in the standard wastewater treatment processes in treatment systems but also exerts toxic effects on humans and other living organisms; hence, their removal is of interest to researchers (Jäger et al., 2018; Farhat et al., 2022).
Among the wide range of pharmaceutical pollutants, antibiotics are widely used in medicine and veterinary medicine because of their antimicrobial effects (Bilal et al., 2023). These organic compounds enter the environment not only through effluent from the pharmaceutical industry but also due to an incomplete metabolism mechanism during the treatment period. In this respect, the persistent presence of antibiotics in the aquatic environment and their bioaccumulation leads to microbial and bacterial resistance (Wydro et al., 2023; Wysowska et al., 2024).
As an antibiotic of the third generation of cephalosporins, ceftriaxone (C18H18N8O7S3) is used to kill many bacteria and to treat bacterial infections such as meningitis, gonorrhea-induced infections, pneumonia, and orthopedic surgeries (Nguyen et al., 2022). The main side effects of ceftriaxone include skin and digestive complications, dyspnea, increased blood platelet levels, anaphylactic shock, anaphylactoid reactions, and cardiac arrest. These effects have severe effects on patient’s life-threatening in many cases. Unfortunately, the excessive use of ceftriaxone in unnecessary cases (e.g., colds) is an important factor for the increased occurrence of the drug’s side effects. Ceftriaxone may also cause severe intestinal disorders such as diarrhea or abdominal cramps (Tao et al., 2024). Research has shown that some of these highly consumed antibiotics enter the wastewater treatment systems in their original form daily through the renal biliary excretion system of the patients under treatment (Noman et al., 2022).
As the second generation of fluoroquinolones, the antibiotic norfloxacin (C16H18FN3O3) is used to treat various infections, particularly urinary infections (Liu et al., 2022). This antibiotic prevents the function of a bacterial enzyme involved in DNA replication called DNA gyrase and topoisomerase II and inhibits the multiplication of bacterial DNA. The side effects of norfloxacin include nausea, headache, fatigue, arthritis, gastrointestinal bleeding, depression, and indigestion (Swidan and Rubin, 2020). This drug can cause severe side effects such as tendon irritation or rupture and nervous system problems. Tendon problems may appear a long time after treatment and can lead to lifelong disability in severe cases (Sharma et al., 2008). Multiple fluoroquinolone family antibiotics and their modified by-products in drinking water, surface and underground water, and wastewater have currently caused major concerns (Hiasa, 2018; Bhatt and Chatterjee, 2022).
These pollutants not only do not vanish in wastewater treatment systems but also kill effective microbes in biological treatment, leading to the ineffectiveness of wastewater treatment systems (Pei et al., 2019; Madhusoodanan, 2022). Due to their resistance to biological decomposition, these compounds enter the environment (particularly water sources) through wastewater treatment plants (Kim et al., 2023). Researchers have frequently addressed the entry of these antibiotics into the environment in the concentration range of ng L−1 to μg L−1 (Dorival-García et al., 2013). Therefore, it is necessary to adopt appropriate treatment methods to eliminate or reduce such antibiotics before entering the environmental resources.
Various methods, such as chemical precipitation, biological processes, ion exchange, membrane filtration, and electrochemical techniques, are available for antibiotic-containing wastewater treatment (Molinari et al., 2020; Uluseker et al., 2021; Spit et al., 2022; Li J. et al., 2023; Vinayagam et al., 2023; da Silva Júnior et al., 2024). The efficiency of these methods is limited due to the high cost, long treatment time, toxicity of chemicals, prohibition in wide applications, and limited access in developing countries, making it necessary to use alternative techniques (Ahmed et al., 2021). Adsorption is among the low-cost methods of high interest used for reducing some pollutants for various reasons such as low cost, flexibility, simplicity of design, ease of operation, insensitivity to toxic pollutants, and the availability of a wide range of inexpensive adsorbents compared to other treatment methods (Tony, 2022; Dutta et al., 2024).
Different adsorbents, including natural and synthetic adsorbents, are used in adsorption method. A group of synthetic adsorbents used for this purpose is graphene oxide (GO). The identified unique properties of this adsorbent (e.g., a high surface area, special functional groups on the surface, and high absorption capacity) have nowadays directed attention toward using this material as an adsorbent (Wang et al., 2018; Krishna et al., 2023).
Yang et al. (2022) used the GO adsorbent to remove enrofloxacin and rhodamine B. They obtained the optimum conditions as a pH of 7, optimal contact time of 23 min, and removal of ˃92.5% for both analytes. The GO adsorbent performed well in enrofloxacin and rhodamine B removal from an aqueous solution with maximum adsorption capacities of 45.035 and 107.230 mg g−1 for enrofloxacin and rhodamine B, respectively (Yang et al., 2022).
Kadhim et al. (2020) modified the polyether sulfone (PES) membrane using the GO adsorbent. They used the PES/GO adsorbent to remove Acid Black and Rose Bengal. They presented evidence that this adsorbent could efficiently remove the mentioned dyes. The performance of the PES/GO adsorbent showed a removal rate of >99% for both dyes (Kadhim et al., 2020).
Madadrang et al. (2012) removed Pb (II) through the silanization reaction between N-(trimethoxysilylpropyl) ethylenediamine triacetic acid (EDTA-silane) and hydroxyl groups on the GO surface to improve the removal technique. The results showed that the adsorption capacity of the adsorbent to remove Pb (II) was equal to 479 mg g−1 (Madadrang et al., 2012).
Yang et al. (2011) used GO to remove methylene blue from aqueous solutions. They reported that using the hematite powder adsorbent could remove more than 99% of methylene blue from aqueous solutions, with an adsorption capacity of 714 mg g−1 (Yang et al., 2011).
This study reports using GO as an adsorbent to remove ceftriaxone and norfloxacin from aqueous solutions. The surface characteristics of the adsorbent and operating variables in the adsorption process, including pH of the solution, concentration, time, and mass of the adsorbent, were investigated in this research.
2 Materials and methods
2.1 Chemicals, reagents, and instrumentation
The materials used in this study include laboratory-grade chemicals without the need for further purification. A stock solution of the antibiotics (1,000 mg L−1) was prepared in double distilled water (DDW). Working solutions were prepared by diluting the stock solution. In all experiments, DDW was used to prepare solutions and reagents. The pH of the solution was adjusted using 0.1 M HCl and 0.1 M NaOH. The pH was measured by a pH meter (Metrohm 632). The adsorbent was separated from the mixture with an Andreas Hettich D72 centrifuge. The residual concentration of antibiotics was quantified using a (DR6000) UV-VIS spectrophotometer. The GO adsorbent was characterized with a scanning electron microscope (SEM, Cambridge Stereoscan S360), a Fourier transform infrared spectrophotometer (FTIR, Thermo Nicolet Avatar 370), x-ray powder diffraction (XRD, Philips XPERT MPD), and Brunauer-Emmett-Teller (BET, NOVA 2000e) techniques.
2.2 Synthesis of GO
GO was synthesized using the Hummers method. According to this method, 23 mL of concentrated sulfuric acid was added to 1 g of graphite powder, and the resulting mixture was stirred at 5°C for 20 min. The color of the solution is black due to the presence of graphite. Then, 0.5 g of sodium nitrate was added to the solution and stirred at 20°C for 30 min. Next, 3 g of potassium permanganate was added to the mixture gradually during 1 h. The graphite oxidation process was completed by stirring the mixture on a stirrer for 3 h. At this stage, the color of the solution turned dark green. Afterward, 100 mL of DDW was added to the solution and stirred for some time, followed by adding about 5 mL of hydrogen peroxide to neutralize the excess permanganate. Finally, the GO obtained from the solution was separated using a centrifuge at 2,500 rpm. The separated GO was washed with DDW several times. Graphite oxide was converted to graphene oxide by heating it in an ultrasonic bath with a power of 400 W for 30 min. Next, the precipitate was separated from the liquid through centrifugation. Finally, GO powder was obtained by drying the resulting precipitate completely in an oven with a maximum temperature of 80°C for 24 h (Omar et al., 2023).
2.3 Experimental design
Using design methods in any research helps manage the order and number of required tests before starting the tests such that the least number of tests are needed in the shortest time. Using this method, in turn, saves time, reduces material consumption, and improves accuracy (Li X. et al., 2023).
Statistical and multivariate engineering techniques such as the response surface method (RSM) are among the widely used design methods in wastewater treatment. Studies employ RSM to build models free from problems existing in the method of changing one factor at a time (OFAT) and can simultaneously examine and optimize the effect of multiple factors (Maldonado et al., 2023). Compared to OFAT, the advantages of RSM include prioritizing the importance of factors, reducing the number of tests, determining the interaction between factors, providing a mathematical relationship between factors to reach the response, and predicting new answers by changing the factors (Shojaei et al., 2021a; He et al., 2023).
The built models may also be applied to access the linear or quadratic effects of the tested factors and various graphs, including the interaction graphs between the factors. RSM includes factors, levels, and responses (Veza et al., 2023).
Factors are actually the variables affecting the results of tests, namely environmental factors such as pH, contact time, adsorbent mass, and adsorbent concentration in adsorption processes. The levels refer to the range of factor changes, including the minimum, maximum, and average values of the factors. The responses deal with the necessary values for the outputs.
Among the RSM design methods, the central composite design (CCD) method was chosen in the present study due to the greater number of levels. In this design, the factors have five levels defined as -α, −1, 0, +1, and +α. Here, +α and -α are the maximum and minimum values of each factor, respectively (Amin et al., 2023). In this method, four important and influential factors (i.e., pH, adsorbent mass, contact time, and concentration) were simultaneously optimized using Design-Expert V.12 software. This design allows the responses to be modeled by fitting a second-order polynomial, which can be expressed as (Equation 1).
where β0, βi, βii, and βij are the constant, linear, square, and interaction coefficients of the factors, respectively. Xi and Xj are independent variables, and e is the unpredicted error. Y (R%) is the response. In the CCD design, the number of tests is calculated using Equation 2.
where k is the number of independent variables, and nc is the number of central points. According to the mentioned inputs, the model determined 30 tests for each antibiotic. Table 1 shows the test design matrix of CCD to remove antibiotics. The number of responses defined for the Design-Expert software included the removal percentages of ceftriaxone and norfloxacin (Table 2).
2.4 Removal of ceftriaxone and norfloxacin from aqueous solution by GO
This empirical study was conducted discontinuously on a laboratory scale in 250-mL Erlenmeyer flasks. Operating factors, including pH (3–11), contact time (5–25 min), concentration (20–100 mg L−1), and the adsorbent mass (0.01–0.05 g), were investigated in this research. The removal process of antibiotics was implemented with GO in an ultrasonic bath. For each test, 150 mL of the sample with an antibiotic concentration of 25 mg L−1 was first poured into the Erlenmeyer flask. If needed, 0.1 M NaOH or 0.1 M HCl solutions were used to adjust the pH of the samples. Next, a determined volume of the GO adsorbent was weighed and added to the sample in an Erlenmeyer flask, and the suspension was heated in an ultrasonic bath for a defined period. After the determined contact time, centrifugation was applied to separate adsorbent particles from the sample. The antibiotic concentration in the final solution was measured by a spectrophotometer. The antibiotic removal percentage was calculated using Equation 3.
where C0 and Ce are the initial and final concentrations of the antibiotic (after the adsorption process), respectively.
3 Results and discussion
3.1 Characterization of the GO adsorbent
The FT-IR technique is a powerful characterization method used to determine functional groups in a substance. Supplementary Figure S1 A shows the FT-IR spectrum of GO. The FT-IR pattern recorded at room temperature was measured in the range of 4,000–500 cm−1. The peak at 3,400 cm−1 belongs to the presence of the hydroxyl group, 1730 cm−1 corresponds to carboxyl groups, 1,650 cm−1 is assigned to the carbon-carbon double bond (C = C), and 1,220 cm−1 refers to epoxy rings on the surface of GO. The carbon-oxygen bond also creates the 1,050 cm−1 peak. SEM was applied to determine the morphology of the GO adsorbent. According to the SEM image of GO (Supplementary Figure S1 B), the adsorbent surface is layered with the layers overlapped on each other. Due to the π-π bond between the benzene rings on the surface of GO, bonding is possible between different adsorbent layers, forming multilayer GO in this case. Supplementary Figure S1 C represents the N2 adsorption and desorption isotherm for GO. The surface area of GO and the total volume of its cavities were measured using the BET method. The obtained surface area of GO, the holes’ total volume, and the holes’ average diameter were equal to 260.8 m2 g−1, 0.254 cm3 g−1, and 3.85 nm, respectively. According to the IOPAC, adsorbents with pore sizes between 2 and 50 nm are called mesoporous. Therefore, GO falls under the mesoporous adsorbents. The structure of GO was identified using the XRD analysis. The obtained results of the XRD pattern of GO (Supplementary Figure S1 D) show that the main peak at 2θ = 11.3° belongs to the GO (002) plane, and a weak peak at 2θ = 43° results from the un-laminated graphite.
3.2 Statistical analysis
Table 3 represents the ANOVA data for ceftriaxone and norfloxacin removal. After entering the required information into the software and running the model, the usefulness and appropriateness of the model should be evaluated in terms of application in the removal process of antibiotics. These assessments can be done using the ANOVA table data provided by the model. In this table, the P-value shows the effect of the factors on the response; the higher its value, the lower the effect of the factor. Therefore, if the P-value is >0.05 in a model, it means a very low impact of the factors on the obtained response, thereby rendering the model inappropriate. On the other hand, when the P-value is <0.05, the confidence level of the model is >95%, meaning favorable conditions (Di Leo and Sardanelli, 2020; Li Z. et al., 2023). According to Table 3, the P-value of the model is <0.0001 for both antibiotics, suggesting that the model is acceptable. The F-value represents a measure of the significance or validity of the model, and a higher value indicates more significance of the model. The F-value was high for both models, suggesting that the model is generally significant and acceptable.
Lack of fit (LOF) means the degree of compatibility of the model with real data, which should not be significant. The most ideal model is the one in which the model’s fit with the laboratory data is statistically significant, and its incompatibility is not significant. Since such an ideal state can be seen for both antibiotics, it can be concluded that the models produced for both antibiotics and the responses provided by these models are in good agreement with the laboratory data. The coefficient of determination (R2) should also be controlled in addition to examining the P-value and F-value. The closer R2 is to 1, the better the model corresponds to the laboratory data. In addition to the R2, this software provides two other coefficients of determination: 1) one belonging to the laboratory data, known as the adjusted R2 (R2-Adj) and 2) the prediction of the model responses, called the prediction R2 (R2-Pred). The difference between these two values should be less than 0.2 for the model to be acceptable (Yin et al., 2023). It can also be seen that this agreement is established in Table 3.
In addition to examining the numbers in the ANOVA table, the obtained graphs should also be verified to visually evaluate the appropriate agreement of the model results with the laboratory data. Supplementary Figure S2 shows the real and predicted values for the response of the removal percentage of norfloxacin and ceftriaxone. Obviously, a good agreement exists between the predicted values of the model and the laboratory data because the laboratory-obtained points are distributed near the normal line. Among the useful outputs provided by the software is a mathematical equation between the factors affecting the removal (i.e., pH, contact time, adsorbent mass, and concentration) to reach the response. Equations 4, 5 are polynomial functions illustrating significant relationships between experimental variables and responses in the experimental design.
where A, B, C, and D were concentration, pH, adsorbent mass, and sonication time, respectively.
3.3 Three-dimensional plots
Design-Expert software can also provide three-dimensional levels of the influence of factors on the response and exhibit the influence level of each factor on the response in colored forms. Figures 1A–D present the three-dimensional response surface for the effect of two factors simultaneously on the antibiotic removal rate. Accordingly, all four factors have a maximum area, indicating the influence of each factor on the response.
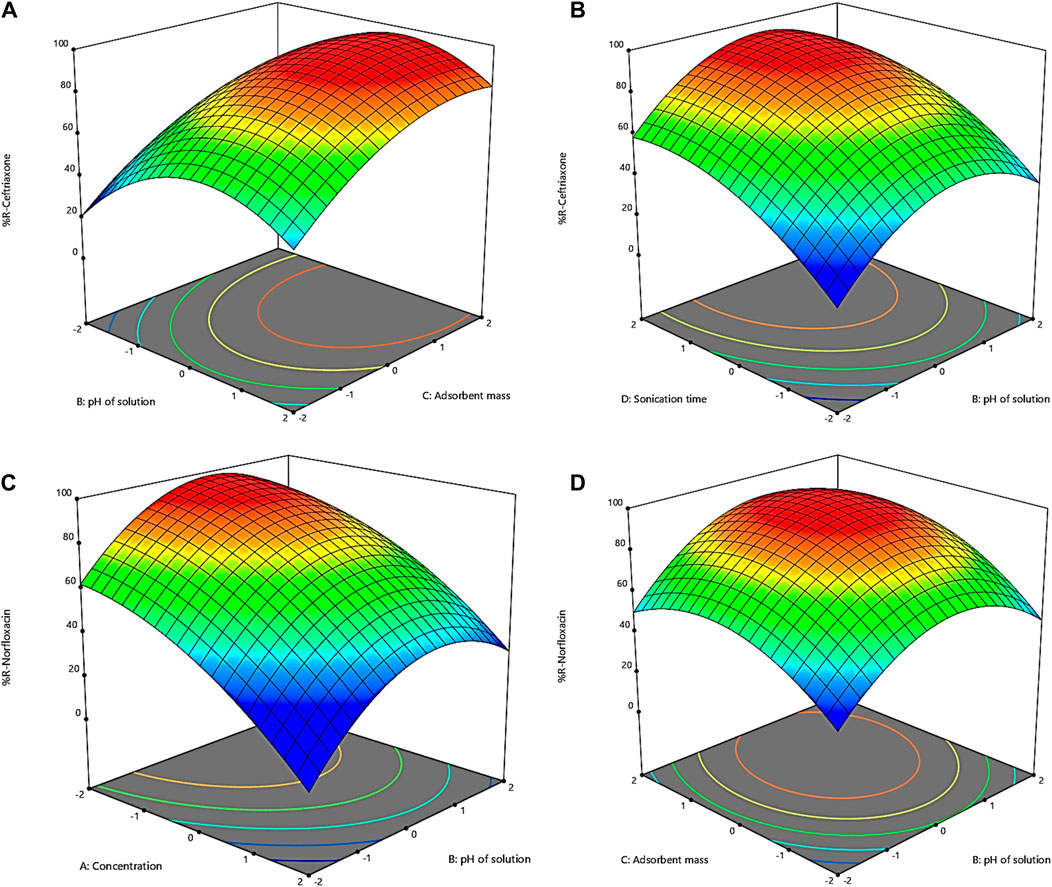
Figure 1. Three-dimensional plots of interactions for removal of (A) and (B) ceftriaxone; (C,D) norfloxacin.
Figure 1A shows the effect of pH changes on ceftriaxone removal by the GO adsorbent. The range of pH changes was chosen to be from 3 to 11. Figure 1A shows that ceftriaxone has a low removal percentage in the acidic range. In other words, the reactions between the adsorbent and the adsorbate are low in these conditions. The removal percentage increases with rising pH toward neutral; however, the removal percentage declines with the continued ascending trend of pH. These observations can be attributed to the type of bond between ceftriaxone and the adsorbent, which is related to the surface charge of the adsorbent because this bond is electrostatic. The lowest negative charge for the GO adsorbent exists at acidic pHs. Therefore, an increase in both pH and the negative charge of the adsorbent allows for more bonds between the cationic molecule and GO, leading to increased adsorption (Zhang et al., 2016). The decrease in adsorption at pHs >7 is due to water ionization in the basic environment. Since the adsorbent is a hydrophilic substance, it shows a greater reaction affinity to water than ceftriaxone molecules. Indeed, water molecules and ceftriaxone molecules compete to seize the negative charges of the adsorbent surface in basic conditions, and the presence of this competitor lowers antibiotic adsorption (Olusegun et al., 2023).
To investigate the effect of the adsorbent mass on the removal of ceftriaxone, values of 0.01 to 0.05 g were chosen for the adsorbent. Figure 1A illustrates the effect of changes in the adsorbent mass on the ceftriaxone removal rate by the GO adsorbent. According to Figure 1A, the removal efficiency of ceftriaxone increased with increasing the adsorbent from 0.01 g to 0.05 g. The elevated efficiency with increasing the adsorbent mass can be attributed to the expanded surface of the adsorbent in contact with GO. This increased surface, in turn, amplifies the number of available adsorption sites for pollutant adsorption (Shojaei et al., 2021b). As can be inferred from Figure 1A, an amount of 0.034 g was optimal for the GO adsorbent. Moreover, this part of the study showed that the removal efficiency was not significantly affected by increasing the GO mass by more than 0.034 g.
Figure 1B shows the effect of contact time on the ceftriaxone removal rate by the GO adsorbent. The contact time was examined in the range of 5–25 min. According to Figure 1B, the contact time changes have two slopes in times <20 min and >20 min. The first part has a high slope, indicating the rapid adsorption process in this period. From the beginning to the first 20 min, the surface sites of the adsorbent are filled with ceftriaxone, and a rapid connection is established between ceftriaxone molecules and the GO adsorbent. After 20 min, the removal rate decreases because the sites are occupied on the adsorbent surface, making it necessary for ceftriaxone molecules to penetrate the adsorbent and fill the internal sites and pores (Anjum et al., 2019). Since GO is a mesoporous adsorbent with a pore diameter of about 3.8 nm and relatively large ceftriaxone molecules, penetration into the pores may be difficult. Therefore, a very low slope of the graphs can be seen in the second part. This slope suggests that a contact time of more than 20 min does not significantly affect removal, and it is not economical to use longer times. As mentioned above, an optimal contact time of 20 min was estimated for ceftriaxone.
Figure 1C represents the effect of norfloxacin concentrations on the GO adsorbent’s removal efficiency. The impact of concentration on the removal efficiency of norfloxacin was investigated in the range of 20–100 mg L−1. According to Figure 1C, the removal efficiency decreased due to insufficient surface to adsorb higher concentrations of norfloxacin by increasing the norfloxacin concentration from 20 to 100 mg L−1. Based on the results, norfloxacin molecules at low concentrations interacted with the adsorption sites on the surface of the adsorbent, resulting in a higher removal percentage. At higher concentrations, the removal efficiency decreases due to the saturation of the active sites of the adsorbent (Ali et al., 2016). As shown in Figures 1A, C concentration of 25 mg L−1 was determined as the optimal dose for norfloxacin.
3.4 Optimal conditions of removal
In addition to modeling the removal process, the removal conditions can be optimized using the RSM. The optimal removal conditions were assessed using the numerical software optimization technique in RSM. If the goal is to maximize the removal rate, the presented model with a utility of 1 will reach this goal. In this case, the removal efficiencies of ceftriaxone and norfloxacin will be 93.50% and 97.44%, respectively, with the adsorbent mass, contact time, concentration, and pH values of 0.034 g, 20 min, 25 mg L−1, and 8, respectively (Supplementary Table S1). The prediction of the model was validated by conducting the experimental tests under optimal conditions. The experimental results of ceftriaxone and norfloxacin removal were 95.87% and 98.64%, respectively, confirming the model’s accuracy.
3.5 Regeneration of GO adsorbent
A major aspect of an ideal adsorbent is that it can be used frequently. In fact, the reuse ability of an adsorbent in the adsorption process is valuable when a significant decrease is not observed in its efficiency. In this respect, an adsorbent with the possibility of recovery can significantly reduce the total costs of the removal process. In this research, the reusability of the GO adsorbent was examined by preparing some solutions containing ceftriaxone and norfloxacin. After using the GO adsorbent to remove antibiotics, it was separated from the solutions by centrifugation. The separated adsorbent was washed with 2 mL of methanol and finally dried in an oven at 85°C. This reuse cycle of GO in adsorption/desorption processes was repeated 5 times. Figure 2 presents the changes in the removal percentages of ceftriaxone and norfloxacin using recovered GO. In the first reuse cycle of the GO adsorbent, the removal efficiency decreased very slightly for both antibiotics. Although the removal efficiency declined by about 30% in the fifth reuse cycle of adsorbent, a significant removal rate with removal percentages of >64% was obtained for both antibiotics. Therefore, this adsorbent could well remove ceftriaxone and norfloxacin in five cycles of use.
3.6 Interference studies
The effect of possible interferences of various cations and anions in the removal of antibiotics was investigated to evaluate the method’s applicability in removing norfloxacin and ceftriaxone in the presence of external ions. For this purpose, the signal of the sample solution was first measured in the absence of interfering species under optimal conditions. The obtained analytical signal was measured three times to calculate the average of the signals and the standard deviation. The possible interfering effect of each species was assessed by adding the target ion to the initial solution with a w/w ratio of 1,000 times compared to the antibiotics. Next, the corresponding analytical signal was obtained by performing the removal process on this solution. If the decomposition signal obtained in the presence of interfering species was not in the range of ±5%, it meant that the target ion was interfering with the existing ratio. Otherwise, the weight ratio of the interfering species would be reduced to the extent that the decomposition signal would be in the range of ±5%. The results (Table 4) show that when the species are present in the sample as much as 1,000 times of antibiotics, they do not interfere seriously in removing antibiotics.
3.7 Application to real samples
A synthetic sample was used to evaluate the proposed method’s efficiency in removing ceftriaxone and norfloxacin. These samples were prepared using tap water, wastewater, and groundwater as fixed textures. In this procedure, specific doses of the antibiotics were added to a fixed volume of water samples to prepare synthetic samples. The resulting samples were examined using the proposed method under optimal conditions. Each measurement was performed in triplicate. The standard increase method was used in the measurements. The results in Table 5 indicate the ability of GO to remove ceftriaxone and norfloxacin from water samples.
3.8 Comparison with other adsorbents
Table 6 compares the performance of the GO adsorbent with other adsorbents for ceftriaxone and norfloxacin removal. The experimental results revealed that the GO adsorbent was comparable with the other adsorbents for ceftriaxone and norfloxacin removal. The adsorbent consumption rate of the proposed method was considerably lower than that of other studies. Moreover, the duration of the current study on antibiotic removal was shorter than the other ones because the sonication process significantly increases the process efficiency. Additionally, using the RSM method dramatically reduces the number of tests, thereby saving time and the adsorbent amount. Therefore, the GO adsorbent performed well in ceftriaxone and norfloxacin removal from water samples.
4 Conclusion
In this research, the effectiveness of GO adsorbent in removing ceftriaxone and norfloxacin was investigated in laboratory conditions. The CCD-based RSM was used to model and optimize ceftriaxone and norfloxacin removal. The effects of adsorbent mass (0.01, 0.02, 0.03, 0.04, and 0.005 g), pH (3, 5, 7, 9, and 11), concentration (20, 40, 60, 80, and 100 mg L−1), and sonication time (5, 10, 15, 20, and 25 min) were evaluated as independent variables and the removal rate as the dependent variable (response). Significant process variables were obtained as quadratic regression models for the response (p < 0.0001). An R2 >0.99 was calculated for removal modeling by the CCD method for ceftriaxone and norfloxacin. The results showed that the antibiotic concentration and the adsorbent mass in the removal process had the most negative and positive effects. The best process conditions were obtained as a pH of 8, an adsorbent mass of 0.034 g, a sonication time of 20 min, and a concentration of 25 mg L−1. The removal efficiencies were equal to 95.87% and 98.64% for ceftriaxone and norfloxacin, respectively, under optimal conditions. The interference studies revealed that external ions did not seriously interfere with antibiotics removal when they were present in the sample as much as 1,000 times the antibiotics. The removal rates of ceftriaxone and norfloxacin were in the range of 89.95% to 98.55% for environmental samples under optimum conditions. The results of this study demonstrated that the GO adsorbent has a good ability to remove ceftriaxone and norfloxacin from aqueous solutions. Moreover, CCD and RSM proved a good ability in modeling, predicting, and optimizing the behavior of parameters affecting the process.
Data availability statement
The original contributions presented in the study are included in the article/Supplementary Material, further inquiries can be directed to the corresponding author.
Author contributions
ZL: Data curation, Formal Analysis, Writing–original draft. SZ: Supervision, Writing–review and editing. GZ: Conceptualization, Data curation, Formal Analysis, Writing–original draft. JX: Data curation, Formal Analysis, Writing–original draft.
Funding
The author(s) declare that no financial support was received for the research, authorship, and/or publication of this article.
Acknowledgments
The authors are grateful to Qingyang Prefectural Center for Disease Control and Prevention for their kind support.
Conflict of interest
The authors declare that the research was conducted in the absence of any commercial or financial relationships that could be construed as a potential conflict of interest.
Publisher’s note
All claims expressed in this article are solely those of the authors and do not necessarily represent those of their affiliated organizations, or those of the publisher, the editors and the reviewers. Any product that may be evaluated in this article, or claim that may be made by its manufacturer, is not guaranteed or endorsed by the publisher.
Supplementary material
The Supplementary Material for this article can be found online at: https://www.frontiersin.org/articles/10.3389/fenvs.2024.1436848/full#supplementary-material
References
Ahmed, S. F., Mofijur, M., Nuzhat, S., Chowdhury, A. T., Rafa, N., Uddin, M. A., et al. (2021). Recent developments in physical, biological, chemical, and hybrid treatment techniques for removing emerging contaminants from wastewater. J. Hazard. Mater. 416, 125912. doi:10.1016/j.jhazmat.2021.125912
Ali, R. M., Hamad, H. A., Hussein, M. M., and Malash, G. F. (2016). Potential of using green adsorbent of heavy metal removal from aqueous solutions: adsorption kinetics, isotherm, thermodynamic, mechanism and economic analysis. Ecol. Eng. 91, 317–332. doi:10.1016/j.ecoleng.2016.03.015
Amin, I. S., Neysari, A. N., Althomali, R. H., Musad Saleh, E. A., Baymakov, S., Radie Alawady, A. H., et al. (2023). Development of microextraction methods for the determination of sulfamethoxazole in water and biological samples: modelling, optimization and verification by central composite design. Front. Environ. Sci. 11, 1242730. doi:10.3389/fenvs.2023.1242730
Amiri, S., Reza Sohrabi, M., and Motiee, F. (2020). Optimization removal of the ceftriaxone drug from aqueous media with novel zero-valent iron supported on doped strontium hexaferrite nanoparticles by response surface methodology. ChemistrySelect 5 (19), 5831–5840. doi:10.1002/slct.202000285
Anjum, H., Johari, K., Gnanasundaram, N., Appusamy, A., and Thanabalan, M. (2019). Impact of surface modification on adsorptive removal of BTX onto activated carbon. J. Mol. Liq. 280, 238–251. doi:10.1016/j.molliq.2019.02.046
Bhatt, S., and Chatterjee, S. (2022). Fluoroquinolone antibiotics: occurrence, mode of action, resistance, environmental detection, and remediation–A comprehensive review. Environ. Pollut. 315, 120440. doi:10.1016/j.envpol.2022.120440
Bilal, H., Li, X., Iqbal, M. S., Mu, Y., Tulcan, R. X. S., and Ghufran, M. A. (2023). Surface water quality, public health, and ecological risks in Bangladesh—a systematic review and meta-analysis over the last two decades. Environ. Sci. Pollut. Res. 30 (40), 91710–91728. doi:10.1007/s11356-023-28879-x
da Silva Júnior, A. H., de Oliveira, C. R. S., Wolff Leal, T., Pellenz, L., de Souza, S. M. D. A. G. U., de Souza, A. A. U., et al. (2024). Reviewing perovskite oxide-based materials for the effective treatment of antibiotic-polluted environments: challenges, trends, and new insights. Surfaces 7 (1), 54–78. doi:10.3390/surfaces7010005
Di Leo, G., and Sardanelli, F. (2020). Statistical significance: p value, 0.05 threshold, and applications to radiomics—reasons for a conservative approach. Eur. Radiol. Exp. 4, 18–8. doi:10.1186/s41747-020-0145-y
Dorival-García, N., Zafra-Gómez, A., Navalón, A., González, J., and Vílchez, J. L. (2013). Removal of quinolone antibiotics from wastewaters by sorption and biological degradation in laboratory-scale membrane bioreactors. Sci. Total Environ. 442, 317–328. doi:10.1016/j.scitotenv.2012.10.026
Dutta, L., Sethi, G. K., and Dey, S. (2024). A comprehensive and critical assessment on the efficiency of natural and synthetic adsorbents for the removal of recalcitrant malachite green from water: present level and future perspectives. Korean J. Chem. Eng. 1-19. doi:10.1007/s11814-024-00114-4
Fang, L., Miao, Y., Wei, D., Zhang, Y., and Zhou, Y. (2021). Efficient removal of norfloxacin in water using magnetic molecularly imprinted polymer. Chemosphere 262, 128032. doi:10.1016/j.chemosphere.2020.128032
Farhat, B., Chrigui, R., Rebai, N., and Sebei, A. (2023). Analysis of hydrochemical characteristics and assessment of organic pollutants (PAH and PCB) in El Fahs plain aquifer, northeast of Tunisia. Environ. Sci. Pollut. Res. 30 (35), 84334–84356. doi:10.1007/s11356-023-28216-2
Hashemi, S. Y., Yegane Badi, M., Pasalari, H., Azari, A., Arfaeinia, H., and Kiani, A. (2022). Degradation of Ceftriaxone from aquatic solution using a heterogeneous and reusable O3/UV/Fe3O4@ TiO2 systems: operational factors, kinetics and mineralisation. Int. J. Environ. Anal. Chem. 102 (18), 6904–6920. doi:10.1080/03067319.2020.1817909
He, X., Chen, X., Wang, X., and Jiang, L. (2023). Optimization of activated carbon production from corn cob using response surface methodology. Front. Environ. Sci. 11, 1105408. doi:10.3389/fenvs.2023.1105408
Hiasa, H. (2018). DNA topoisomerases as targets for antibacterial agents. DNA topoisomerases Methods Protoc. 1703, 47–62. doi:10.1007/978-1-4939-7459-7_3
Jäger, T., Hembach, N., Elpers, C., Wieland, A., Alexander, J., Hiller, C., et al. (2018). Reduction of antibiotic resistant bacteria during conventional and advanced wastewater treatment, and the disseminated loads released to the environment. Front. Microbiol. 9, 2599. doi:10.3389/fmicb.2018.02599
Kadhim, R. J., Al-Ani, F. H., Al-Shaeli, M., Alsalhy, Q. F., and Figoli, A. (2020). Removal of dyes using graphene oxide (GO) mixed matrix membranes. Membranes 10 (12), 366. doi:10.3390/membranes10120366
Kim, J. W., Hong, Y. K., Ryu, S. H., Kwon, O. K., Lee, Y. B., and Kim, S. C. (2023). Development of analytical method for veterinary antibiotics and monitoring of residuals in agricultural environment. Appl. Biol. Chem. 66 (1), 20. doi:10.1186/s13765-023-00777-3
Krishna, R. H., Chandraprabha, M. N., Samrat, K., Murthy, T. K., Manjunatha, C., and Kumar, S. G. (2023). Carbon nanotubes and graphene-based materials for adsorptive removal of metal ions–a review on surface functionalization and related adsorption mechanism. Appl. Surf. Sci. Adv. 16, 100431. doi:10.1016/j.apsadv.2023.100431
Li, J., Bai, H., Qiao, H., Du, C., Yao, P., Zhang, Y., et al. (2023). Causal effects of COVID-19 on cancer risk: a Mendelian randomization study. J. Med. Virol. 95 (4), e28722. doi:10.1002/jmv.28722
Li, X., Wang, B., Liu, F., and Yu, G. (2023). Occurrence and removal of pharmaceutical contaminants in urine: a review. Water 15 (8), 1517. doi:10.3390/w15081517
Li, Z., Zhao, R., Chen, X., Jiao, Y., and Chen, Z. (2023). Design approach for tuning the hybrid region of 3D-printed heterogeneous structures: modulating mechanics and energy absorption capacity. ACS Appl. Mater. Interfaces 15 (6), 7686–7699. doi:10.1021/acsami.2c17753
Liu, C., Xie, Y., Jiao, Y., Du, Y., Zheng, Q., and Sun, Y. (2022). Visible-light-driven nanoscale zero-valent iron loaded rGO/g-C3N4 for fluoroquinolone antibiotics degradation in water. Front. Environ. Sci. 10, 1065770. doi:10.3389/fenvs.2022.1065770
Madadrang, C. J., Kim, H. Y., Gao, G., Wang, N., Zhu, J., Feng, H., et al. (2012). Adsorption behavior of EDTA-graphene oxide for Pb (II) removal. ACS Appl. Mater. Interfaces. 4 (3), 1186–1193. doi:10.1021/am201645g
Madhusoodanan, J. (2022). How persister bacteria evade antibiotics, prolong infections. Proc. Natl. Acad. Sci. 119 (41), e2215617119. doi:10.1073/pnas.2215617119
Mahmoud, M. E., El-Ghanam, A. M., Mohamed, R. H. A., and Saad, S. R. (2020). Enhanced adsorption of Levofloxacin and Ceftriaxone antibiotics from water by assembled composite of nanotitanium oxide/chitosan/nano-bentonite. Mater. Sci. Eng. 108, 110199. doi:10.1016/j.msec.2019.110199
Maldonado, I., Vega Quispe, A. P., Merma Chacca, D., and Zirena Vilca, F. (2022). Optimization of the elimination of antibiotics by Lemna gibba and Azolla filiculoides using response surface methodology (RSM). Front. Environ. Sci. 10, 940971. doi:10.3389/fenvs.2022.940971
Molinari, R., Lavorato, C., and Argurio, P. (2020). Application of hybrid membrane processes coupling separation and biological or chemical reaction in advanced wastewater treatment. Membranes 10 (10), 281. doi:10.3390/membranes10100281
Nayak, A., Bhushan, B., and Kotnala, S. (2022). Fabrication of chitosan-hydroxyapatite nano-adsorbent for removal of norfloxacin from water: isotherm and kinetic studies. Mater. Today Proc. 61, 143–149. doi:10.1016/j.matpr.2021.07.356
Nazraz, M., Yamini, Y., and Asiabi, H. (2019). Chitosan-based sorbent for efficient removal and extraction of ciprofloxacin and norfloxacin from aqueous solutions. Microchim. Acta. 186, 459–9. doi:10.1007/s00604-019-3563-x
Nguyen, P. V., Aubry, C., Boudaoud, N., Gaubert, A., Langlois, M. H., Marchivie, M., et al. (2022). Oligonucleotide solid nucleolipid nanoparticles against antibiotic resistance of ESBL-producing bacteria. Pharmaceutics 14 (2), 299. doi:10.3390/pharmaceutics14020299
Noman, E. A., Mohamed, R. M. S. R., Al-Gheethi, A. A., Al-Shaibani, M. M., Al-Wrafy, F. A., Al-Maqtari, Q. A., et al. (2022). Antibiotics and antibiotic-resistant bacteria in greywater: challenges of the current treatment situation and predictions of future scenario. Environ. Res. 212, 113380. doi:10.1016/j.envres.2022.113380
Noudeh, G. D., Asdaghi, M., Noudeh, N. D., Dolatabadi, M., and Ahmadzadeh, S. (2023). Response surface modeling of ceftriaxone removal from hospital wastewater. Environ. Monit. Assess. 195 (1), 217. doi:10.1007/s10661-022-10808-z
Olusegun, S. J., Osial, M., Souza, T. G., Krajewski, M., Rodrigues, G. L., Marek, P., et al. (2023). Comparative characteristics and enhanced removal of tetracycline and ceftriaxone by Fe3O4-lignin and Fe3O4-carbon-based lignin: mechanism, thermodynamic evaluation, and DFT calculation. J. Mol. Liq. 371, 121075. doi:10.1016/j.molliq.2022.121075
Omar, H., Malek, N. S. A., Nurfazianawatie, M. Z., Rosman, N. F., Bunyamin, I., Abdullah, S., et al. (2023). A review of synthesis graphene oxide from natural carbon based coconut waste by Hummer’s method. Today Proc. 75, 188–192. doi:10.1016/j.matpr.2022.11.427
Pei, M., Zhang, B., He, Y., Su, J., Gin, K., Lev, O., et al. (2019). State of the art of tertiary treatment technologies for controlling antibiotic resistance in wastewater treatment plants. Environ. Int. 131, 105026. doi:10.1016/j.envint.2019.105026
Sharma, P. C., Saneja, A., and Jain, S. (2008). Norfloxacin: a therapeutic review. Int. J. Chem. Sci. 6 (4), 1702–1713.
Shojaei, S., Nouri, A., Baharinikoo, L., Farahani, M. D., and Shojaei, S. (2021a). Removal of the hazardous dyes through adsorption over nanozeolite-X: simultaneous model, design and analysis of experiments. Polyhedron. 196, 114995. doi:10.1016/j.poly.2020.114995
Shojaei, S., Shojaei, S., Band, S. S., Farizhandi, A. A. K., Ghoroqi, M., and Mosavi, A. (2021b). Application of Taguchi method and response surface methodology into the removal of malachite green and auramine-O by NaX nanozeolites. Sci. Rep. 11 (1), 16054. doi:10.1038/s41598-021-95649-5
Singh, A. K., Kaur, R., Verma, S., and Singh, S. (2022). Antimicrobials and antibiotic resistance genes in water bodies: pollution, risk, and control. Front. Environ. Sci. 10, 830861. doi:10.3389/fenvs.2022.830861
Spit, T., van der Hoek, J. P., de Jong, C., van Halem, D., de Kreuk, M., and Perez, B. B. (2022). Removal of antibiotic resistance from municipal secondary effluents by ozone-activated carbon filtration. Front. Environ. Sci. 10, 834577. doi:10.3389/fenvs.2022.834577
Swidan, S., and Rubin, M. (2020). “Herbal and supplement use in pain management,” in Advanced therapeutics in pain medicine (CRC Press), 301–322. Available at: https://www.taylorfrancis.com/chapters/edit/10.1201/9780429504891-19/herbal-supplement-use-pain-management-sahar-swidan-mara-rubin.
Tao, E., Zhou, H., Zheng, M., Zhao, Y., Zhou, J., Yuan, J., et al. (2024). Ceftriaxone-induced severe hemolytic anemia, renal calculi, and cholecystolithiasis in a 3-year-old child: a case report and literature review. Front. Pharmacol. 15, 1362668. doi:10.3389/fphar.2024.1362668
Tony, M. A. (2022). Low-cost adsorbents for environmental pollution control: a concise systematic review from the prospective of principles, mechanism and their applications. J. Dispers. Sci. Technol. 43 (11), 1612–1633. doi:10.1080/01932691.2021.1878037
Uluseker, C., Kaster, K. M., Thorsen, K., Basiry, D., Shobana, S., Jain, M., et al. (2021). A review on occurrence and spread of antibiotic resistance in wastewaters and in wastewater treatment plants: mechanisms and perspectives. Front. Microbiol. 12, 717809. doi:10.3389/fmicb.2021.717809
Veza, I., Spraggon, M., Fattah, I. R., and Idris, M. (2023). Response surface methodology (RSM) for optimizing engine performance and emissions fueled with biofuel: review of RSM for sustainability energy transition. Results Eng. 18, 101213. doi:10.1016/j.rineng.2023.101213
Vinayagam, V., Palani, K. N., Ganesh, S., Rajesh, S., Akula, V. V., Avoodaiappan, R., et al. (2023). Recent developments on advanced oxidation processes for degradation of pollutants from wastewater with focus on antibiotics and organic dyes. Environ. Res. 117500. doi:10.1016/j.envres.2023.117500
Wan, Y., Liu, X., Liu, P., Zhao, L., and Zou, W. (2018). Optimization adsorption of norfloxacin onto polydopamine microspheres from aqueous solution: kinetic, equilibrium and adsorption mechanism studies. Sci. Total Environ. 639, 428–437. doi:10.1016/j.scitotenv.2018.05.171
Wang, X., Liu, Y., Pang, H., Yu, S., Ai, Y., Ma, X., et al. (2018). Effect of graphene oxide surface modification on the elimination of Co (II) from aqueous solutions. Chem. Eng. J. 344, 380–390. doi:10.1016/j.cej.2018.03.107
Wydro, U., Wołejko, E., Luarasi, L., Puto, K., Tarasevičienė, Ž., and Jabłońska-Trypuć, A. (2023). A review on pharmaceuticals and personal care products residues in the aquatic environment and possibilities for their remediation. Sustainability 16 (1), 169. doi:10.3390/su16010169
Wysowska, E., Wiewiórska, I., and Kicińska, A. (2024). The problem of health risk resulting from the presence of pharmaceuticals in water used for drinking purposes: a review. J. Ecol. Eng. 25 (5), 244–256. doi:10.12911/22998993/186371
Yang, J., Shojaei, S., and Shojaei, S. (2022). Removal of drug and dye from aqueous solutions by graphene oxide: adsorption studies and chemometrics methods. NPJ Clean. Water. 5 (1), 5. doi:10.1038/s41545-022-00148-3
Yang, S. T., Chen, S., Chang, Y., Cao, A., Liu, Y., and Wang, H. (2011). Removal of methylene blue from aqueous solution by graphene oxide. J. Colloid Interface Sci. 359 (1), 24–29. doi:10.1016/j.jcis.2011.02.064
Yin, S., Yan, Z., Chen, X., Yan, R., Chen, D., and Chen, J. (2023). Mechanical properties of cemented tailings and waste-rock backfill (CTWB) materials: laboratory tests and deep learning modeling. Constr. Build. Mater. 369, 130610. doi:10.1016/j.conbuildmat.2023.130610
Keywords: central composite design, norfloxacin, ceftriaxone, response surface method, ultrasound-assisted removal
Citation: Li Z, Zhang S, Zhu G and Xing J (2024) Use of graphene oxide for the removal of norfloxacin and ceftriaxone antibiotics from aqueous solution: process optimization using response surface approach. Front. Environ. Sci. 12:1436848. doi: 10.3389/fenvs.2024.1436848
Received: 22 May 2024; Accepted: 05 August 2024;
Published: 21 August 2024.
Edited by:
Jahangeer Jahangeer, University of Nebraska-Lincoln, United StatesCopyright © 2024 Li, Zhang, Zhu and Xing. This is an open-access article distributed under the terms of the Creative Commons Attribution License (CC BY). The use, distribution or reproduction in other forums is permitted, provided the original author(s) and the copyright owner(s) are credited and that the original publication in this journal is cited, in accordance with accepted academic practice. No use, distribution or reproduction is permitted which does not comply with these terms.
*Correspondence: Shuhang Zhang, WlNIbWFpbGdvQDE2My5jb20=