- 1School of Ecological Engineering, Guizhou University of Engineering Science, Bijie, China
- 2Guizhou Province Key Laboratory of Ecological Protection and Restoration of Typical Plateau Wetlands, Bijie, China
- 3School of Civil and Surveying Engineering, Jiangxi University of Science and Technology, Ganzhou, China
- 4Ministry of Education Key Laboratory for Biodiversity Science and Ecological Engineering, School of Life Sciences, Fudan University, Shanghai, China
Promoting the construction of ecological civilization and sustainable development in karst mountainous areas by analyzing the spatial and temporal changes of landscape ecological risks is critical in karst mountainous watersheds. In this study, the land use transfer matrix, landscape ecological risk evaluation model, ecological contribution rate of land use change, and spatial autocorrelation analysis were combined to quantitatively analyze the land use and landscape ecological risk of a typical karst watershed, Liuchong River Basin, over the past 20 years. The results revealed that: 1) From 2000 to 2020, the functional classification of land use in the Liuchong River Basin was dominated by the woodland ecological space, and the most significant shifting characteristics were the increase in the area of watershed ecological space and industrial production space and the decrease in woodland ecological space, with shifts in the middle reaches of the Liuchong River being the most drastic; 2) Generally, the change of the regional landscape pattern was related to the transformation of the land use function type of “production-life-ecological space,” and the spatial aggregation of ecological risk level showed a gradual weakening trend. 3) The conversion of the watershed ecological space to the grassland ecological and agricultural production spaces, the conversion of urban living space to the agricultural production space, and the conversion of the rural living space to the agricultural production space were the dominant factors affecting ecological improvement, whereas the conversion of the woodland ecological space to the grassland ecological space, the woodland ecological space to the agricultural production space, and the grassland ecological space to the agricultural production space contributed to ecological degradation. The study findings can be used as a reference for the coordinated development of “production-life-ecological space” in karst watersheds and provide a scientific basis for ecological environmental protection and sustainable utilization.
1 Introduction
Ecological risk is the amount of risk an ecosystem and its components are exposed under natural or anthropogenic disturbances (Yu et al., 2022). Landscape ecological risk assessment can reflect the effect of landscape patterns on ecological processes and functions (Liu et al., 2022; Ran et al., 2022). Urban growth patterns in China exhibit a trend of sprawling expansion, which has led to a considerable increase in the level of urbanization and a drastic expansion in the scale of land use. However, urbanization has caused several problems, including encroachment on productive agricultural and ecological land, deepening landscape fragmentation, and ecological pollution. These problems have resulted in an imbalance in the ratio of the production-life-ecology spatial structure (Bai et al., 2019; Qi, 2020; Zhang et al., 2021; Lu et al., 2022). Especially in the karst mountainous areas of southwest China, the frequent natural and human activities and the increasing level of economic development and urbanization have exerted a considerable ecological and environmental pressure on the ecologically fragile and environmentally change-sensitive karst mountain belt. In this context, the 18th Party Congress proposed ecological civilization construction to control the development intensity and adjust the spatial structure and balance population, resources and environment, and unify economic, social and ecological benefits, for promoting intensive and efficient production space, livable and moderate living space, and beautiful ecological space, with nature restoration, good land for agriculture, and a beautiful home with blue sky, green land, and clean water for future generations. Thus, the construction of ecological civilization has gradually become the prime focus of national land space development.
By providing novel perspectives for regional ecological risk research, the landscape pattern index method has become a research hotspot (Guo and Guo, 2022; Li et al., 2023). To construct landscape ecological risk evaluation models, corresponding parameters are chosen according to the specificity of landscape patterns in various research regions. This approach has been applied in many risk control regions, yielding excellent results. For example, Hayes et al. used a relative risk model to assess regional ecological risk in the near-coastal marine environment of northwest Washington and identified ship traffic, mountainous urban, and agricultural land use, and shoreline recreational activities as the ecological risk factors in the marine nearshore area (Hayes and Landis, 2004). Ayre analyzed (Ayre and Landis, 2012) a forested landscape in northeastern Oregon from a landscape disturbance, habitat, and ecological resource perspective based on a Bayesian network model with an ecological risk assessment framework. Paukert conducted a landscape-scale ecological risk assessment of land use, waterway development and diversion, and human development in the lower Colorado River basin at four watershed scales (Paukert et al., 2011). Studies in China have investigated the factors affecting landscape ecological risk (LER) from the perspective of landscape ecology and elucidated the dynamics of LER and its spatial and temporal patterns. Kang constructed a LER index for the Manas River basin from the proportion of landscape components during 2000–2015 and then compared the distribution of various levels of LER and spatial and temporal distribution in the region (Kang et al., 2020). Based on the landscape ecological risk index and geographically weighted regression model, Wang revealed the interconnection between the levels of LER and urban expansion in Yuanzhou district from 2000 to 2018 (Wang et al., 2021). Liu constructed an ecological risk evaluation model from the watershed scale and water source protection zone scale to examine the spatial and temporal changes of ecological risk in the Miyun Reservoir watershed during 1990–2018 (Liu et al., 2023). Lan evaluated the spatial and temporal evolution characteristics of ecological risk in Guilin city at the overall and county scales based on the spatial correlation between land use and ecological risk in the city during 2000–2020 (Lan et al., 2023). Although studies on LER evaluation are relatively mature, limited research has been conducted on LER in karst mountains (Wang et al., 2022a). In addition, most studies have focused on a single land use type, and fewer studies have analyzed LER from the perspective of “production-life-ecological space” (Su et al., 2020; Wang et al., 2022b).
The continuous development of the global economy, technology, and population has further strengthened the effect of human activities on the natural environment. The karst region has considerably higher ecosystem fragility and environmental vulnerability than other regions because of its unique geological and climatic conditions. Being an important region, effectively promoting the healthy development of ecological environment in the watershed is a concern. Therefore, this study analyzed the spatial and temporal evolution patterns of ecological risk in the Liuchong River Basin from the spatial perspective of “three lives,” based on the land use data of 2000, 2010, and 2020. Furthermore, by integrating the results with geographical information system (GIS) spatial analysis and LER index, the spatial evolution of production-ecology in the context of rapid socio-economic development was clarified. The spatial evolution process of life ecology and LER in the context of rapid socio-economic development was considered to provide a reference for ecological risk management and landscape pattern optimization in typical karst mountainous basins.
2 Materials and methods
2.1 Study area
Liuchong River is the largest first-order tributary of the Wujiang River system, with a total length of 273.4 km and a natural drop of 1,243 m. Because of geomorphology and hydrogeology constraints, the river is tortuous, with deep valley, narrow surface and large drop. The Liuchong River Basin is located in Bijie City, northwestern Guizhou Province and southwestern Zhenxiong County, Yunnan Province, with longitude and latitude ranges of 104°20′-160°07′E and 26°31′-27°30′N, respectively. The total area of the basin is 10,874 km2 (Figure 1). The basin is a typical karst mountainous watershed with karst landform development and complex topography. The watershed belongs to a subtropical cool and humid monsoon climate, with moderate water and heat resources. The annual average temperature is approximately 18 °C, and the precipitation is concentrated in May-September, with an annual average precipitation of 848.6–1394.4 mm. Because of the fragile ecological environment in the watershed, the ecological landscape security in the watershed is has attracted considerable research attention because of the continuous urbanization and accelerated comprehensive development and utilization of the watershed in the past 20 a.
2.2 Data
Land use data of the Liuchong River Basin for three periods from 2000 to 2020 (2000, 2010, 2020) were obtained from the Resource and Environment Science Data Center of the Chinese Academy of Sciences (http://wwwresdc.cn), with the spatial resolution of 30 m. Using ArcGIS 10.6, the land use data were projected and transformed, spliced, and cropped. Based on the development objective of building the efficient production space (PS), livable space (LS), and beautiful ecological space proposed by the government and the actual situation of the watershed and according to the land use classification system, PLES was classified into eight secondary categories, namely agricultural production space, industrial production space, urban living space, rural living space, forest ecological space, grassland ecological space, water ecological space, and other ecological spaces (Table 1).
2.3 Methods
2.3.1 Division of the LER assessment unit
To make the LER index reflect the ecological risk status caused by landscape changes in a certain area, a quantitative expression was used to portray the degree of spatial ecological risk, according to the basic requirements and principles of landscape ecology, and using 2–5 times the average patch area for the grid is appropriate, considering the study area and landscape spatial heterogeneity. After repeated debugging, a square grid of 3 km × 3 km was selected as the study area. The center point of each grid was considered to be the sampling point, totaling 1240 sampling points. The ecological risk index was calculated for each of the 1240 cells in the study area based on this grid division and used as the ecological risk value of the sample area center point for spatial interpolation analysis.
2.3.2 The LER assessment model
To examine the spatial and temporal variability and characteristics of landscape ecological risk in the Liuchong River Basin, a landscape ecological risk evaluation model was constructed using the calculation of the LER from previous studies (Su et al., 2020).
Here,
2.3.3 Ecological contribution rate of PLES land use transformation (LEI)
LEI refers to the land use types leading to ecological risk changes. Quantifying the impact of land use type shifts on the ecological environment from both positive and negative aspects can help in discriminating between the land use types that affect changes in regional ecological quality and identifying the dominant factors for changes in the regional ecological environment (Liang et al., 2022). It is calculated using the following formula:
where LEI indicates the ecological contribution of the regional land use transformation type. The value of LEI ranges from −1 to 1, with a positive number indicating a positive contribution that increases the ecological risk, and a negative number indicating a negative contribution that decreases the ecological risk. LEt1 and LEt0 refer to the ecological risk index of a specific land type before and after transformation, respectively; LA is the area of that change type; TA is the total area of the study area.
3 Results
3.1 Quantitative change and type shift of land use in the PLES
The changes of PLES area and the proportion of PLES in the Liuchong River Basin for 3 years are presented in Table 3, revealing that the area of “production-life-ecological space” in the Liuchong River Basin varied greatly from 2000 to 2020, and the land use function classification in 2000–2020 is woodland ecological space, followed by an agricultural production space. During the study period, the area of woodland and grassland ecological spaces exhibited an overall decrease, with the woodland ecological space of 93629.73 km2 in 2000 decreasing to 93202.41 km2 in 2020, grassland ecological space shrinking from 31878.41 km2 in 2000–31331.05 km2 in 2020, and the agricultural production space shrinking from 49624.98 km2 in 2000–48403.52 km2 in 2020. The area of the water ecological space showed an increasing trend, from 406.02 km2 in 2000 to 1046.64 km2 in 2020, whereas the area of other ecological spaces did not significantly change. From the perspective of the living space, the area of urban living space and rural living space increased from 251.95 to 240.57 km2 in 2000 to 681.24 and 310.16 km2 in 2020, respectively. From the perspective of the production space, the area of the industrial production space continued to increase from 106.62 km2 in 2000 to 1167.96 km2 in 2020, whereas the area of the agricultural production space exhibited a slightly decreasing trend from 49,624.98 km2 in 2000 to 48,403.52 km2 in 2020.
According to the change patterns in the distribution of PLES in the study area from 2000 to 2020 (Figure 2), the industrial production space underwent the most rapid expansion, the area of urban living, rural living, and watershed ecological spaces exhibited a gradual increase, corresponding to a gradual reduction in the area of agricultural production space, and the woodland ecological space and grassland ecological space exhibited a slight change. Although the area of industrial production space decreased in 2000, which was only sporadically distributed around the urban living space, by 2020, a large expansion was observed along the perimeter of the city, with significant changes in the area.
PLES land use area, in addition to the quantitative increase or decrease, also shows distinct types of transfer. Figure 3 reveals that during the 20-year period, the area transferred out of woodland ecological space was 3667.46 km2, reaching the historical maximum, of which 655.33 km2 was transformed into the grassland ecological space, and 160.90 km2 was transformed into the agricultural production space. The amount of transfer in was only 319.34 km2, whereas the amount of transfer out was considerably larger than the amount of transfer in, which indicated that the area of woodland ecological space decreased during the study period. Second, the amount of the agricultural production space transferred out was 3459.94 km2, mainly to the woodland ecological and grassland ecological spaces, with an area of 222.91 km2, and the amount of transfer in was 280.11 km2, converted from the grassland ecological and woodland ecological spaces, with an area of 279.30 km2. The grassland ecological space was the type with the most amount of land transfer in, and the area converted from woodland ecological and grassland. The area transformed from woodland ecological space and grassland ecological space amounted to 750.09 km2.
3.2 Temporal variation in ecological risk in PLES landscapes
Based on the PLES land use raster data of 2000, 2010, and 2020 in the Liuchong River Basin, the ecological risk indexes of each landscape were calculated using Fragstats 4.2 software and combined with the formula 1 and formulas in Table 2; subsequently, the results were compiled (Table 4). As depicted, for over 20 years, the index of fragmentation of agricultural production space remained unchanged; the degree of separation first decreased and subsequently increased, indicating that the aggregation of its landscape type has increased; the fragmentation and separation of industrial production space and rural living space were high, and the values decreased year by year, indicating that their distribution in space is highly dispersed; and the ecological stability increased considerably with the increase in the area. The increase in the area of the urban living space covered the surrounding small patches, resulting in a decrease in fragmentation and separation of the urban living space annually. The fragmentation of the woodland ecological space increased, whereas the fragmentation and separation of grassland, water, and other ecological spaces continued to decline, with the decline for other ecological spaces being linear. Second, the values of the sub-dimension of each landscape type in the Liuchong River Basin were low and exhibited a decreasing trend, indicating that the shape of the landscape types in the study area tended to be simple and the intensity of disturbance by human activities was decreasing. The change trend of the disturbance index of each landscape type was similar to that of the sub-dimension; however, the disturbance index of rural living space and other ecological space was large, with both reached the historical peak at the beginning of the study, indicating that the disturbance index of human activities on rural living space and other ecological space was large in 2000, which reached the historical minimum in 2020, indicating a decrease in the disturbance of human activities on the landscape.
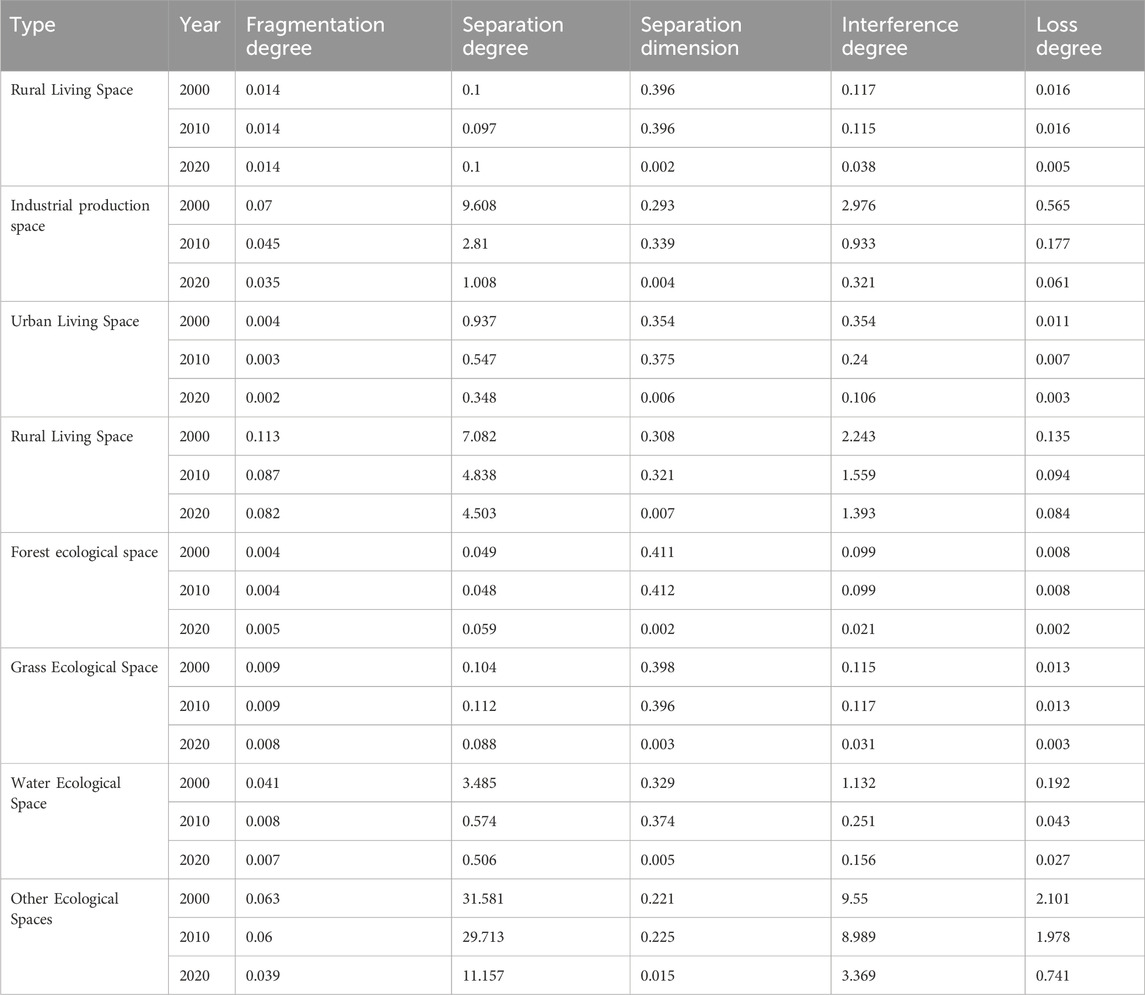
Table 4. Land Use Transfer Matrix for production-life-ecological space in the Liuchong River Basin (Unit: km2).
3.3 Analysis of spatial and temporal changes in LER
The ecological risk distribution maps of the study area in 2000, 2010, and 2020 were obtained through kriging interpolation. According to the natural breakpoint method, the ecological risk of the study area landscape was classified into low, lower, medium, higher, and high ecological risk zones (Figure 4).
The distribution of the ecological risk level of the landscape strongly correlated with the distribution of PLES land types, with high-risk and medium-high-risk areas in the northeast and southeast regions exhibiting a trend of “scattering-clustering” from 2000 to 2020, and expanding outward by 2020. The aforementioned areas are dominated by the industrial production and urban living space, and the strong human activities lead to the instability of the ecosystem. High landscape separation and sub-dimension number considerably influence the formation of the landscape pattern and are sensitive to external disturbances. For the medium-risk areas, the aggregation areas located in the central-western and south-central regions gradually declined. Furthermore, lower-risk areas were concentrated in the periphery of medium-risk areas, including the woodland ecological and grassland ecological spaces, with low landscape fragmentation and weak human activities. During 2010–2020, this space increased considerably, and most medium-risk areas converted into lower-risk areas. The low ecological risk areas were concentrated in the peripheral areas, and in 2020, a large distribution in the northwest of the watershed, mostly the woodland ecological space, with low population density and complex and diverse topography, was observed. These low-risk areas are not easily disturbed by human activities, leading to the predominance of these areas.
The area and proportion of each risk level area were counted to analyze the changes in increase and decrease of the ecological risk (Figure 5). From the temporal perspective, in 2000, the ecological risk was dominated by low-risk, lower-risk, and medium-risk areas, among which the lower-risk area occupied the largest area, reaching 4078.91 km2, which was 41.25% of the total study area. The ecological risk situation deteriorated from 2000 to 2010 and improved considerably from 2010 to 2020. This phenomenon indicates that the ecological environment quality improved and the ERI level decreased during the study period.
3.4 Spatial autocorrelation analysis of ecological risk in the landscape
The global Moran’s I value of ERI in the study area in 2000, 2010, and 2020 were 0.3881, 0.3456, and 0.3100, respectively, all of which are greater than 0, indicating that ERI is positively correlated in space and exhibits a certain spatial convergence. Furthermore, the global Moran’s I value from 2000 to 2020 exhibited an overall decreasing trend, reflecting the weakening of the ERI and spatial convergence. Compared with the global Moran’s I, the local Moran’s I accurately reflects the spatial distribution of ecological risk values in the landscape. As displayed in Figure 6, high-high (HH) and low-low (LL) clustering dominated ecological risk values in the three periods, as presented in the LISA clustering of ecological risk indices in the Liuchong River Basin from 2000 to 2020 (Figure 6), with the high concentration exhibiting a northeast-southeast trend. By 2020, the HH agglomeration declined and part of the internal grid became nonsignificant. By contrast, LL agglomerations were distributed around the study area and were dispersed. The agglomerated areas exhibited a gradual convergence, whereas the dispersed areas showed gradual dispersion. High-low (HL) and low-high (LH) phenomena are rare and discrete in distribution. Quantitatively, both the spatial autocorrelation and the number of grids in the HH clustered areas declined over time, and the number of positively correlated grids that passed the significance test (p > 1) decreased. Therefore, the spatial clustering characteristics of ERI in the Sixchon River basin are weakening.
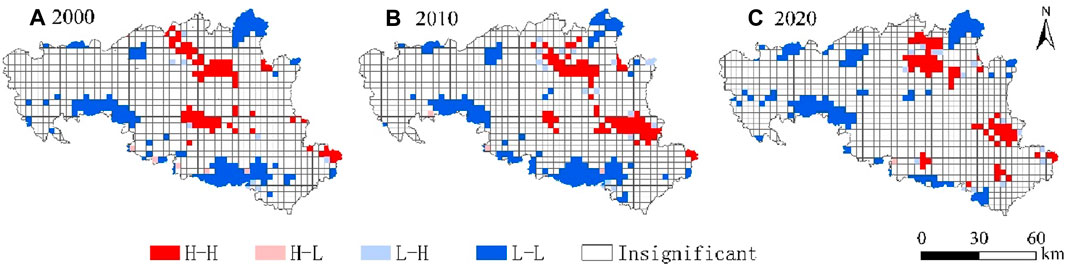
Figure 6. LISA cluster map of land use ecological risk index in the Liuchong river basin in 2000 (A), 2010 (B), 2020 (C).
3.5 Effect of PLES land use conversion on ecological risk
In terms of the contribution of the dominant PLES land use conversion (Table 5), the dominant factors affecting ecological improvement are the conversion of the watershed ecological space to the grassland ecological and agricultural production spaces, the conversion of the urban living space to the agricultural production space, and the conversion of the rural living space to the agricultural production space in the Liuchong River Basin from 2000 to 2020, with a combined contribution of 83.37%. By contrast, the conversion of the woodland ecological space to the grassland ecological space, the woodland ecological space to the agricultural production space, and the grassland ecological space to the agricultural production space were the dominant factors leading to ecological degradation, with a combined contribution of 63.26%. The expansion of the urban living space during the study period was attributed mainly to the reduction of the agricultural production space. The transformation of the agricultural production space to the urban living space indirectly changes landscape fragmentation, landscape separateness, and landscape fractional dimension index, eventually increasing the regional ecological risk.
4 Discussion
Examining the interactions between PLES land use changes and landscape patterns in the Liuchong River Basin, a typical karst basin, can help analyze the correlation at the macro level. In this study, we investigated the land use and LER changes in the Liuchong River Basin from the perspective of PLES. We constructed a LER assessment model by referring to existing studies (Yang et al., 2018; Qi, 2020; Chen and Shi, 2021; Chen et al., 2022b; Wang et al., 2022c; Guo and Guo, 2022; Li and Wu, 2022; Liang et al., 2022). Furthermore, by using land use change ecological contribution ratio and spatial autocorrelation analysis, we quantitatively analyzed the LER of the Liuchong River Basin in karst areas. By comparing data of different years, we summarized the spatial and temporal change patterns of LER in the region in the past 20 years. The results revealed that, first, the stability of the ecosystem in the karst region was severely disturbed by human activities. In this study, the functional classification of land use in the Liuchong River Basin in the past 20 years was dominated by the woodland ecological space, but a trend of decreasing woodland ecological space was observed, whereas the area of the industrial production space increased. This phenomenon indicates that the impact of current human activities on the karst ecosystem is intensifying, and effective measures are required to protect and restore the ecosystem. Second, the distribution of high and low ecological risk levels in the landscape is strongly correlated with the distribution of PLES land types. The high-risk and medium-high-risk areas are distributed in the northeast and southeast regions, which are dominated by the agricultural production, industrial production, and urban living spaces and are disturbed by human activities. Although the lower and low-risk areas are mostly in the periphery of the medium-risk area, including the woodland ecological and grassland ecological spaces. Therefore, targeted measures should be enacted to protect high-risk and medium-high-risk areas. Moreover, coordinated management with agriculture, industry, and towns should be conducted to ensure environmental quality and ecosystem stability. Finally, the conversion of the watershed ecological space to the grassland ecological and agricultural production spaces, the conversion of the urban living space to the agricultural production space, and the conversion of the rural living space to the agricultural production space are the dominant factors affecting ecological improvement. This phenomenon indicates that the agricultural production and grassland ecological spaces should be protected and restored to ensure ecological environmental protection in karst areas. Further, to promote agricultural modernization and sustainable development, the occupation of land resources by urban and rural living spaces should be reduced and transformed into the agricultural production space as much as possible.
This study has some shortcomings. First, LER assessment is a complex process requiring the consideration of multiple uncertainties. These factors determine the comprehensive evaluation results. When assessing ecological risks, these factors determine the integrated evaluation results. In the LER assessment of complex karst areas, the method and process should be improved. Second, this study selected only the Liuchong River Basin as the study object and did not cover other regions. In the future, more study sites can be selected for cross-sectional comparative analysis to improve the generalizability and reliability of the results. Finally, we did not consider the differences in human activities and influence, especially under different topographic conditions, human production, living, and other activities. These factors considerably influence the evolutionary process of PLES land use, spatial and temporal patterns, and the extent of their effect on the ecosystem. Therefore, these factors were not included to elucidate the complexity and diversity of land use and its ecological environment in the Liuchong River Basin. Future research should use improved methodology and advanced technologies in land use analysis and ecological risk evaluation, and expand the scope of the study area to achieve effective protection and management of karst watershed ecosystems.
5 Conclusion
In this study, the land use classification system was constructed from the perspective of PLES based on the land use cover data in 2000, 2010, and 2020. Using GIS spatial analysis technology and Fragstats 4.2 software, the land use transfer matrix, LER evaluation model, ecological contribution rate of land use flow, and spatial autocorrelation analysis were combined. Furthermore, the spatial and temporal patterns of PLES land use and its LER in the Liuchong River Basin over the past 20 years were quantitatively analyzed. The conclusions are as follows:
(1) From 2000 to 2020, the functional classification of land use in the Liuchong River Basin was dominated by the woodland ecological space, accounting for more than 53% of the total area, and the industrial production space underwent the most rapid expansion. The most significant transfer characteristics of PLES land use were the increases in the ecological space of watershed and the area of industrial production space and a decrease in the woodland ecological space; the transfer was the most drastic in the middle reaches of the main stream of the Liuchong River, whereas the surrounding areas of Hezhang County are stable ecosystems due to higher altitude and less disturbance by human activities.
(2) The distribution of high and low LER levels correlated strongly with the distribution of PLES land types, with high-risk and medium-high-risk areas distributed in the northeast and southeast regions, and medium-risk areas clustered in the west-central and south-central regions of the basin and exhibiting a gradual decrease. Furthermore, the lower and lowest risk areas were concentrated in the periphery of the medium-risk areas, including the woodland ecological and grassland ecological spaces, with low landscape fragmentation and weakly affected by human activities.
(3) The spatial aggregation characteristics of ecological risk levels gradually weakened from 2000 to 2020. HH and LL are concentrated in distribution, HL and LH phenomena are rare and discrete in distribution, and the clustering of HH and LL is obvious in local areas, showing a northeast-southeast trend and a strip-like distribution in space. These areas predominately comprise the agricultural production space, industrial production space, and urban living space and are strongly affected by anthropogenic activities.
(4) The conversion of the watershed ecological space to the grassland ecological and agricultural production spaces, urban living space to the agricultural production space, and rural living space to agricultural production space were the predominant factors contributing to ecological improvement, with a combined contribution of 83.37%. By contrast, the conversion of woodland ecological space to grassland ecological space, woodland ecological space to agricultural production space, and the grassland ecological space to the agricultural production space were the factors contributing to ecological degradation.
Data availability statement
The original contributions presented in the study are included in the article/supplementary material, further inquiries can be directed to the corresponding author.
Author contributions
JR: Conceptualization, Data curation, Project administration, Writing–original draft, Writing–review and editing. YD: Data curation, Formal Analysis, Methodology, Project administration, Writing–original draft, Writing–review and editing. PH: Methodology, Writing–original draft. HL: Data curation, Methodology, Writing–original draft.
Funding
The author(s) declare that financial support was received for the research, authorship, and/or publication of this article. This study was made possible through financial support from the National Natural Science Foundation of China (42361074), Guizhou Bijie United Fund Project, Bike United [No. (2023)8]; “Karst Plateau Resources and Environment Remote Sensing Talent Team”, Office of the Talent Work Leading Group of the Bijie Municipal Committee of the Communist Party of China, Project No.: BWRLT (2023) No. 14, (2021) No. 12; Discipline Construction Project of Guizhou University of Engineering Science.
Conflict of interest
The authors declare that the research was conducted in the absence of any commercial or financial relationships that could be construed as a potential conflict of interest.
Publisher’s note
All claims expressed in this article are solely those of the authors and do not necessarily represent those of their affiliated organizations, or those of the publisher, the editors and the reviewers. Any product that may be evaluated in this article, or claim that may be made by its manufacturer, is not guaranteed or endorsed by the publisher.
References
Ayre, K. K., and Landis, W. G. (2012). A Bayesian approach to landscape ecological risk assessment applied to the Upper Grande Ronde Watershed, Oregon. Hum. Ecol. Risk Assess. An Int. J. 18, 946–970. doi:10.1080/10807039.2012.707925
Bai, L. M., et al. (2019). Ecological risk assessment for Changchun based on land use change. Ecol. Sci. 38, 26–35. doi:10.14108/j.cnki.1008-8873.2019.03.004
Chen, B., et al. (2022a). Evaluation and coupling coordination analysis of landscape ecological security of Yichang from the perspective of production-life-ecological space. Res. Soil Water Conserv. 29, 344–351. doi:10.13869/j.cnki.rswc.2022.04.011
Chen, D., and Shi, L. (2021). The landscape ecological risk assessment and prediction for xiong’an new area based on land use change. Ecol. Econ. 37, 224–229.
Chen, Z., and Liu, Y. (2022b). Comprehensive eco-environmental effects caused by land use transition from the perspective of production-living-ecological spaces in a typical region: a case study of the guangxi Zhuang autonomous region, China. Land 11, 2160. doi:10.3390/land11122160
Guo, Y., and Guo, W. (2022). Landscape ecological risk analysis of urban agglomeration in the central basin of Shanxi from the perspective of “production-living-ecological spaces”. Chin. J. Ecol. 41, 1813–1824. doi:10.13292/j.1000-4890.202206.015
Hayes, E. H., and Landis, W. G. (2004). Regional ecological risk assessment of a near shore marine environment: cherry Point, WA. Hum. Ecol. Risk Assess. 10, 299–325. doi:10.1080/10807030490438256
Kang, Z., et al. (2020). Landscape ecological risk assessment in Manas River Basin based on land use change. Acta Ecol. Sin. 40, 6472–6485.
Lan, Y., Chen, J., Yang, Y., Ling, M., You, H., and Han, X. (2023). Landscape pattern and ecological risk assessment in Guilin based on land use change. Int. J. Environ. Res. Public Health 20, 2045. doi:10.3390/ijerph20032045
Li, C., and Wu, J. (2022). Land use transformation and eco-environmental effects based on production-living-ecological spatial synergy: evidence from Shaanxi Province, China. Environ. Sci. Pollut. Res. 29, 41492–41504. doi:10.1007/s11356-022-18777-z
Li, S., He, W., Wang, L., Zhang, Z., Chen, X., Lei, T., et al. (2023). Optimization of landscape pattern in China Luojiang Xiaoxi Basin based on landscape ecological risk assessment. Ecol. Indic. 146, 109887. doi:10.1016/j.ecolind.2023.109887
Liang, T., Yang, F., Huang, D., Luo, Y., Wu, Y., and Wen, C. (2022). Land-use transformation and landscape ecological risk assessment in the Three Gorges Reservoir region based on the “production-living-ecological space” Perspective. Land 11, 1234. doi:10.3390/land11081234
Liu, H., Hao, H., Sun, L., and Zhou, T. (2022). Spatial-temporal evolution characteristics of landscape ecological risk in the agro-pastoral region in western China: a case study of ningxia hui autonomous region. Land 11, 1829. doi:10.3390/land11101829
Liu, K., et al. (2023). Optimization of landscape pattern in China Luojiang Xiaoxi Basin based on landscape ecological risk assessment. Acta Ecol. Sin. 43, 105–117.
Lu, C., et al. (2022). Spatial-temporal pattern and influencing factors of the “production-living-ecological” functional space of the Yellow River Basin at county level in Gansu, China. Sci. Geogr. Sin. 42, 579–588.
Paukert, C. P., Pitts, K. L., Whittier, J. B., and Olden, J. D. (2011). Development and assessment of a landscape-scale ecological threat index for the Lower Colorado River Basin. Ecol. Indic. 11, 304–310. doi:10.1016/j.ecolind.2010.05.008
Qi, J. (2020). Spatial function identification and pattern evolution of “production-living-ecology” in fast-going urbanization areas—a sampling case study of Xinzheng City, Henan. J. Saf. Environ. 20, 1588–1595.
Ran, P., Hu, S., Frazier, A. E., Qu, S., Yu, D., and Tong, L. (2022). Exploring changes in landscape ecological risk in the Yangtze River Economic Belt from a spatiotemporal perspective. Ecol. Indic. 137, 108744. doi:10.1016/j.ecolind.2022.108744
Su, Y., et al. (2020). Ecological risk assessment in Yongchuan District of Chongqing city based on landscape pattern. Bull. Soil Water Conserv. 40 (03), 195–201+215. doi:10.13961/j.cnki.stbctb.2020.03.028
Wang, A., Liao, X., Tong, Z., Du, W., Zhang, J., Liu, X., et al. (2022a). Spatial-temporal dynamic evaluation of the ecosystem service value from the perspective of “production-living-ecological” spaces: a case study in Dongliao River Basin, China. J. Clean. Prod. 333, 130218. doi:10.1016/j.jclepro.2021.130218
Wang, H., Liu, X., Zhao, C., Chang, Y., Liu, Y., and Zang, F. (2022b). Spatial-temporal pattern analysis of landscape ecological risk assessment based on land use/land cover change in Baishuijiang National nature reserve in Gansu Province, China. Ecol. Indic. 124, 107454. doi:10.1016/j.ecolind.2021.107454
Wang, J. Y., et al. (2021). Coupling relationship between urban spatial expansion and landscape ecological risk—a case study of Yuanzhou District in Jiangxi Province. Res. Soil Water Conservation 28, 142–151. doi:10.13869/j.cnki.rswc.2021.05.017
Wang, S., et al. (2022c). GIS-based ecological risk assessment and ecological zoning in the Three Gorges Reservoir area. Acta Ecol. Sin. 42, 4654–4664. doi:10.5846/stxb202104080909
Yang, Q. K., et al. (2018). Land use transformation based on ecological-production-living spaces and associated eco-environment effects: a case study in the Yangtze River Delta. Sci. Geogr. Sin. 38, 97–106. doi:10.13249/j.cnki.sgs.2018.01.011
Yu, J., et al. (2022). Landscape ecological risk assessment and ecological security pattern construction in landscape resource-based city: a case study of Zhangjiajie City. Acta Ecol. Sin. 42, 1290–1299. doi:10.5846/stxb202012313341
Zhan, H. X., et al. (2009). Suggestion on reasons and countermeasures for fishery resources to decline of Liuchong River. J. Hydroecology 30, 147–149.
Keywords: landscape ecological risk, production-life-ecological space, spatial and temporal changes, Liuchong river basin, ecological risk
Citation: Ren J, Dong Y, He P and Lu H (2024) Spatial-temporal changes of landscape ecological risk in the Liuchong river basin from the perspective of production-life-ecological space. Front. Environ. Sci. 12:1428058. doi: 10.3389/fenvs.2024.1428058
Received: 06 May 2024; Accepted: 30 May 2024;
Published: 24 June 2024.
Edited by:
Zhiming Han, Northwest A&F University, ChinaReviewed by:
Wang Junjie, Ocean University of China, ChinaXinchen Gu, Tianjin University, China
Dian Yuan, Nanjing University of Information Science and Technology, China
Copyright © 2024 Ren, Dong, He and Lu. This is an open-access article distributed under the terms of the Creative Commons Attribution License (CC BY). The use, distribution or reproduction in other forums is permitted, provided the original author(s) and the copyright owner(s) are credited and that the original publication in this journal is cited, in accordance with accepted academic practice. No use, distribution or reproduction is permitted which does not comply with these terms.
*Correspondence: Hanyu Lu, dGVlc240NjNAZ3Vlcy5lZHUuY24=