- 1Department of Soil Science and Agricultural Chemistry, College of Agriculture, Odisha University of Agriculture and Technology, Bhubaneswar, Odisha, India
- 2Department of Soil Science and Agricultural Chemistry, Institute of Agricultural Sciences, Siksha ‘O’ Anusandhan (Deemed to be) University, Bhubaneswar, Odisha, India
- 3Department of Plant Pathology, Institute of Agricultural Sciences, Siksha ‘O’ Anusandhan (Deemed to be) University, Bhubaneswar, Odisha, India
- 4Divisions of Plant Pathology, ICAR-Indian Agricultural Research Institute, New Delhi, India
Conventional fertilizers, although widely available, are relatively low in nutrient use efficiency and cause serious environmental concerns like eutrophication, greenhouse gas (GHG) emissions, nitrate poisoning, and soil pollution. With this in mind, a randomized block design (RBD) experiment was conducted in an experimental field taking 10 treatments and 3 replications to investigate the effects of integrated approaches of nano-formulated DAP fertilizer applications on rice growth, yield, and nutrient use efficiency over conventional approaches. The result revealed that plant height, panicle length, number of tillers hill−1, total number of grain panicle−1, and root length were maximum in T10 treatment (50% of the soil test recommended doses (STD) for N and P + seedling root dipping (SRD) with nano DAP at 5 mL L−1 + twice foliar sprays (FS) with nano DAP at 4 mL L−1 at 25 and 45 DAT (date after transplanting). The highest mean grain yields (4.12 and 4.05 Mg ha−1) and nutrient uptake were recorded in the T2 treatment (100% STD), but this was at par with T10 treatment. The highest benefit–cost ratio (2.26) was recorded in T10, which was 3.5% higher than T2. N and P agronomic use efficiency (AUE) ranged from 7.5% to 31.5% and 15% to 63%, and recovery efficiency (RE) ranged from 30% to 94.2% and 11.2% to 90.4%. The highest nutrient use efficiency was recorded with T10, followed by T9, and lowest in T4. Post-harvest soil pH and available N and P were significantly highest in T10. Soil MBC, MBN, MBP, urease, and phosphatase activity were found significantly higher in T2, followed by T10. The integrated application of 50% STD for N and P + SRD with nano DAP at 5 mL L-1 + twice FS with nano DAP at 4 mL L−1 at 25 and 45 DAT application can be a suitable substitute for conventional DAP and urea for rice in climate-smart agriculture as it possibly reduces environmental pollution while undisturbing crop yield over the 100% STD.
1 Introduction
Rice (Oryza sativa L.) is the oldest domesticated grain cultivated around the world, with the largest growing area, which covers nearly 9% of earth’s arable land, i.e., 160 million hectares (Mha) and 493 million tons (Mt) of milled rice production (FAOSTAT, 2016). It plays an important role in the cultural history of most Asian countries since ancient times, despite a vast range of environmental circumstances. Irrigated rice areas account for 78% of all rice production and 55% of the total harvested rice area, mostly concentrated in alluvial floodplains, terraces, inland valleys, and deltas in the sub-humid and humid tropics of Asia. India grows rice in 43 Mha with the production of 112 million tons (Mt) of milled rice and the average productivity of 2.6 t ha−1. The crop is grown mostly in the wet season in highly diverse conditions, ranging from hills to coasts with unpredictable rainfall distribution (FAOSTAT, 2016; Bal et al., 2023). Hence, the tagline “Rice is Life” highlights rice’s role as a way to eradicate hunger, fight poverty, and improve people’s diets across Asia and the rest of the world.
Nitrogen (N) and phosphorous (P) are essential macronutrients that play a crucial role in promoting optimal growth and yield in rice (Zhu et al., 2008). India consumed 19.44 and 7.83 mt of total N and P2O5 fertilizers, respectively, during 2021–22 (FAI, 2022). Farmers usually apply excess N in the form of granular urea and DAP to ensure higher grain yield (Kaufman et al., 2013). Although the application greatly increases the grain yield, still the low N and P use efficiency of 35%–40% and 15%–20%, respectively, possesses a major constraint in rice cultivation as huge amounts of nutrients are lost (Alam et al., 2023; Qiao et al., 2022; Tarafdar, 2020). The indiscriminate use of nitrogenous fertilizers causes serious environmental issues like eutrophication, intensified ammonia (NH3) volatilization, and increased nitrous oxide (N2O) and methane emissions from transplanted paddy fields (Mboyerwa et al., 2022; Zhang et al., 2023). In addition, P adsorption in tropical soil is a major limitation, hindering plant uptake and polluting aquatic ecosystems through acidification, toxic algal bloom formation, fish mortality, and increased pollution of nearby rivers and wells (Hanyabui et al., 2020). The frequent use of inorganic fertilizers in paddy fields leads plant tissues to assemble heavy metals, which therefore affects their nutritional quality (Maqbool et al., 2020). Therefore, we should adopt alternative types and techniques of fertilizer application to maintain adequate plant nutrition without causing pollution to the ecosystem (Mejias et al., 2021).
The N- and P-based fertilizer management is currently regarded as a highly complex task under field conditions, where nano-based fertilizers used in rice could make a significant contribution by addressing the issue of sustainability and help in unlocking the next revolutionary step in precision farming (Xia et al., 2020; Samreen et al., 2022). Nanotechnology involves the synthesis, design, fabrication, manipulation, and use of materials at the nanometer scale. These materials possess one or more dimensions that are less than 100 nm (Lee and Moon, 2020). Nanomaterials exhibit distinct characteristics compared to their parent materials, resulting in modifications to their physiochemical properties. These adjustments confer remarkable traits, functionalities, and heightened reactivity, which can be attributed to very a high surface-to-volume ratio (Joudeh and Linke, 2022). Its application ensures slow availability, resolves the issue of low fertilizer use efficiency, and allows the release of active ingredients to meet plant nutrient demands (Tarafdar, 2020). Foliar application of nano N and P facilitates optimal management of N (Mejias et al., 2021), as well as P, which minimizes N losses to the environment along with P immobilization in the soil. Astaneh et al. (2021) stated that the use of nano fertilizers has the potential to enhance crop productivity and concurrently ameliorate soil fertility. Furthermore, this innovative approach can mitigate pollution levels and foster a conducive habitat for soil microorganisms. According to a field experiment conducted by Burhan and Al-Hassan (2019), the application of nano fertilizers has been found to significantly enhance the productivity of wheat. The study reported an increase in approximately 48.9% in grain yield, along with notable improvements in various quality parameters. In a recent study conducted by Poudel et al. (2023), it was observed that the use of 100% nitrogen–potassium (NK) along with 75% of the recommended dose of nano P, supplemented by two foliar applications of nano P, resulted in a 25% reduction in the required amount of phosphorus when compared to conventional DAP supplementation. Deo et al. (2022) reported that a combination of 50% phosphorus (P), 100% nitrogen–potassium (NK), and root dipping, along with two foliar sprays of nano-DAP at 20–25 days after transplanting (DAT) and 45–50 DAT, resulted in improved nutrient utilization efficiencies and production responses. With the emerging years and gradual improvement in people’s economic level, research on rice has shifted to the nutritional quality aspect, but the nutrient use efficiency is still an aspect that we cannot ignore. Now, we need to shift our focus to achieve higher-scale of rice yield production with maximum nutrient use efficiency, low eutrophication, greenhouse gas (GHG) emissions, and soil pollution while ensuring our national food security, ecology, and environment.
The novelty of this study is to standardize the rate, methods, and timing of nano fertilizers, which could improve rice productivity and N and P use efficiency with minimal loss, which opens up opportunities to assess the feasibility and advantages of nano N and P fertilizers in the climate change era. With this in mind, the current study was conducted to determine the behavior of nano DAP on yield and yield-attributing rice, as well as the nutritional quality of rice grain and post-harvest soil properties.
2 Materials and methods
2.1 Site specification and characteristics
The present experiment was conducted during consecutive kharif (July to December) seasons of 2021–2022 at the experimental farm, Department of Soil Science and Agricultural Chemistry, OUAT, Bhubaneswar, (20.2961°N, 85.8245°E) in East and South Eastern Coastal Plain agro-climatic zones of Odisha. The climate of the experimental site is characterized by a warm and moist climate distinguished by a hot, humid summer and a mild winter. The monthly weather parameters during the cropping season (kharif 2021–22) were recorded at the meteorological observatory of Central Research Station, OUAT. The monthly mean maximum temperature fluctuated from 26.8°C to 34°C, with an average value of 31.8°C, during the crop growing season, whereas the average weekly mean low temperature was 23.6°C, with a range from 26.3°C to 15.2°C. The monthly mean relative humidity varied from 93.3% to 91% in the morning, with an average of 92.1%, and from 69% to 73% in the afternoon, with an average of 72.6%. Moreover, the mean monthly bright sunshine hours ranged from 4.1 to 4.8 h, and the mean evaporation ranged from 6 to 3 mm. The experimental plot has a sandy loam texture (sand, 71.2%; silt, 14.0%; and clay, 14.8%), moderate acidic in reaction (pH 5.19), slightly alkaline in electrical conductivity (0.28 dS m−1), and medium organic carbon content (6.9 g kg−1). The available nitrogen, phosphorus, and potassium contents of initial soil were 151, 13.3, and 82 kg ha−1, respectively.
2.2 Experiment details
The study was carried out by employing various methodologies and utilizing a combination of traditional fertilizers and nano-formulated DAP on rice (cv. MTU-1001) during its active growth stages. This experiment was done in randomized blocks design (RBD) by taking 10 treatments, with 3 replications conducted consecutively over a span of 2 years (Table 1). MTU 1001 (Vijetha) is a semi-dwarf (115 cm), medium-duration (120–125 days) variety with the potential of 9.7 Mg ha−1 and tolerant to BPH and blast with a higher yield stability ratio (84.5) (Mohapatra et al., 2021). It has wide adoptability in Odisha. The nursery was prepared using the wet-bed method, where seeds were soaked for 24 h and then incubated in moist gunny bags for 2 days after being treated with Vitavax Power 3 gm/kg−1 of seed. Pre-germinated seeds were broadcast uniformly on nursery-raised beds (4–5 cm high, 1–1.5 m wide). The soil:farmyard manure mixture (1:1) was spread in a thin layer over the seeds with proper space and management. The beds were irrigated daily and thoroughly before lifting the seedlings (Munda et al., 2018).
In the main experimental field, primary tillage was done using a mold board plough, followed by cultivation. After ploughing twice, the experimental field was divided into 30 plots with 3 replications, each containing 10 plots for the imposition of treatments and proper drainage facility. The net plot size was 26.5 m2 (5.9 m × 4.5 m). The main field was puddled just before transplanting.
Nutrients were applied as per the soil test dose 100:50:50 (STD), i.e., 100 kg of N (50:25:25::three splits), 50 kg of P2O5 (basal), and 50 kg K2O (25:25::two splits) per hectare according to the treatment plan using urea, DAP, MOP, and nano DAP, respectively. Foliar-spraying of nano-DAP was done between 25 and 45 days after transplanting (DAT). Nano DAP supplied by Indian Farmer Fertilizer Co-operative Limited (IFFCO) contains 8% N and 16% P with a particle size of <100 nm. The rice seedlings of 27 days were transplanted after a root dip using 5 mL L−1 of nano DAP with the spacing of 10 cm × 20 cm on 27.07.2021 and 12.08.2022. For effective weed control, pretilachlor 50% EC at 625 g ha−1 was applied, followed by first and second hand weeding done after 20 and 40 DAT, respectively. For effective management of leaf-eating caterpillars, 40% of profenophos and streptocycline were sprayed for the management of BLB diseases.
2.3 Agronomic performance and yield data collection
Randomly, five plants were sampled from each treatment in the early morning for the assessment of chlorophyll (Lichtenthaler, 1987) and phosphorous (Jackson, 1973) at 30, 37, 54, and 61 DAT. The growth-attributing parameters such as the root length (cm), plant height (cm), panicle length (cm), number of effective tillers hill−1, total numbers of grain panicle−1, and test weight were documented by averaging the values of randomly tagged five plants from each plot.
Plant height was measured using a graduated straight scale from the base of the shoot at the soil surface to the tip of the tallest leaf, while the number of tillers was counted from each plant. Moreover, the number of effective tillers per plant was also determined by counting the number of tillers with panicle bearings, which are referred to as effective tillers. An average was found for each parameter and recorded (Mboyerwa et al., 2021). The rice panicles with spikelets were collected to measure the panicle length. Then, grains were separated from the panicle and grouped into unfilled and filled grains. A measure of 1,000 filled grains from the sampled panicles were counted, and weight was measured using the weighing balance.
Moreover, at physiological maturity (95 DAT), plants per 1 m2 area were randomly selected from three placed in one treatment and harvested manually, where the grain and straw samples were collected and labeled for further analysis. Grain and straw yields were recorded after harvesting and mechanical threshing from each plot individually, then air dried for 5 days, and converted into Mg ha−1 at 14% (average) moisture content (Jatav et al., 2022). Samples were then ground into a fine powder to estimate total nitrogen (N), phosphorous (P), and potassium (K) contents, and the uptake was calculated. The harvest index of rice was estimated using Equation 1. The nutrient use efficiency, like the agronomic use efficiency (AUE) (Yoshida, 1981), i.e., the additional increasing yield per unit of input as influenced by kg of grain per kg of supplied nutrient, is calculated using Equation 2. Apparent recovery efficiency (RE) specifies the proportion of nutrients absorbed from externally applied nutrient or fertilizer sources. It is expressed in terms of kg of nutrient uptake per kg of nutrient applied. It is calculated as per Equation 3 (Mitra et al., 2023). Relative agronomic efficiency (RAE) was estimated as the ratio of the yield response with the test nutrient to the respective yield responses of the reference (100% N through STD) nutrient (Akinrinde et al., 2005), and it was calculated using Equation 4.
2.4 Economy
The total cost of cultivation, net return, and benefit cost ratio (B:C ratio) of rice cultivation in all fertilizer management practices was calculated using the following equations (Mohapatra et al., 2023):
where Qi is the quantity of the agricultural input used, Pi is the market price of agricultural input, OCi is the agricultural operational cost, YR is the yield of rice grain and straw yield (Mg ha−1), and Pr is the minimum support price (MSP) for rice grain and market price for straw.
2.5 Soil properties
Post-harvest soil was sampled from each treatment using a spade at a depth of 0–15 cm, followed by soil processing using a standard protocol for studying soil’s physical and chemical characteristics (Page et al., 1982). The alkaline KMnO4 method (Subbaiah and Asija, 1956), Bray’s P method (Bray and Kurtz, 1945), and neutral normal ammonium acetate method (Jackson, 1973) were employed to estimate the available N, P2O5, and K2O, respectively, of the post-harvested soil sample (Page et al., 1982). The collected fresh soil samples (during the maturity stage at a depth of 0–15 cm) were store immediately in a refrigerator at 4°C for biological parameter analysis. Soil urease activity was determined using THAM buffer (Tabatabai and Bremnar, 1972), by estimating the difference in the production of NH4-N between control and treated samples. Phosphatase activity involves the colorimetric estimation of p-nitrophenol released by phosphomonoesterase activity (Tabatabai and Bremnar, 1972). Microbial biomass carbon (MBC) and microbial biomass nitrogen (MBN) were determined via the chloroform fumigation extraction technique (Vance et al., 1987), where the amount of carbon and nitrogen generated due to the death of microorganisms was determined. Microbial biomass phosphorus (MBP) was quantified using fumigation–extraction procedures (Brookes et al., 1982), and P concentration was determined via a modified ammonium molybdate blue ascorbic acid technique (Joergensen, 1996).
2.6 Statistical analysis
The data regarding different parameters obtained from this study were analyzed by the method of analysis of variance (ANOVA) given by Gomez and Gomez (1984), and the effects were determined by the value indicated by the “F” test at a 5% level of significance. The data for each parameter were analyzed using R 4.3.3 software and MS-Excel 2016.
3 Results
3.1 Chlorophyll and phosphorus contents
Total chlorophyll and phosphorus (P) contents of rice leaves at 30, 37, 54, and 61 DAT were determined and are mentioned in Figure 1. It was found that the highest mean total chlorophyll contents of 3.39, 4.19, 4.98, and 4.46 mg g−1 of fresh weight at 30, 37, 54, 47, and 61 days after transplanting (DAT) were recorded with T2, which was at par with T10 values of 3.26, 3.68, 4.48, and 4.83 mg g−1, respectively. However, the lowest chlorophyll contents of 2.87, 3.02, and 3.1 mg g−1 of fresh weight were recorded with T1 at respective intervals. Moreover, the significantly highest mean total P contents of 0.26, 0.28, 0.31, and 0.3% at 30, 37, 54, and 61 days after transplanting (DAT) were recorded with T2, which was at par with T10 values of 0.23, 0.25, 0.32, and 0.30%, respectively. However, the lowest P content was recorded with T1 at respective day intervals.
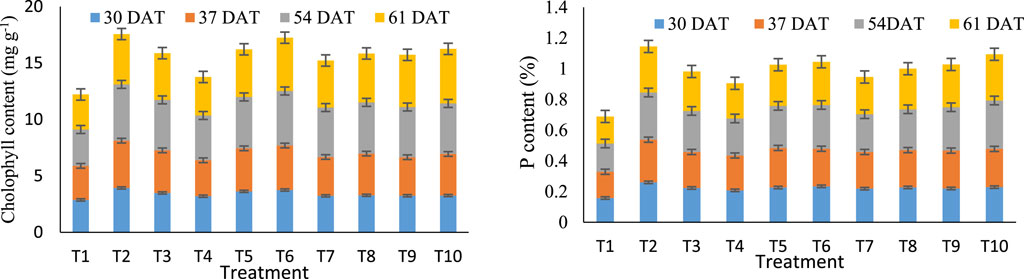
Figure 1. Effects of nano DAP on total chlorophyll contents (mg g−1) and P contents (%) of rice at different growth stages.
3.2 Growth and yield attributes
The growth attributes, viz., root length, plant height, panicle length, and number of effective tiller hill−1 of rice are presented in Table 2. It was observed that the soil application, along with seedling root dipping and foliar sprays of nano DAP, significantly improved the growth parameters in rice. The highest root length of 25.7 cm was recorded with T10, followed by T2 treatment (24.4 cm), which was 5% less than T10, whereas the lowest was perceived in treatment T1 (16.4 cm) i.e., control. It was noticed that the highest plant height of 147 cm was recorded with treatment T10, which was statistically at par with treatment T2 (145 cm), T6 (144 cm), and T9 (143 cm), respectively, and the significantly lowest plant height was recorded with treatment T1 (122 cm) as compared to other treatments, indicating the vital role of N and P in plant growth. Similarly, treatment T10 was found significantly higher than the rest of the treatments, having a panicle length of 29.1 cm, while the minimum was recorded in treatment T1 (24.6 cm). Moreover, in the case of the number of effective tillers, the treatments T2 (12.5) and T10 (12.3) were statistically at par with each other. However, the total number of grain panicle−1 varied from 94 to 145 with the highest value in treatment T2 involving 100% STD, but it was at par with T10, whereas the lowest value was found in treatment T1.
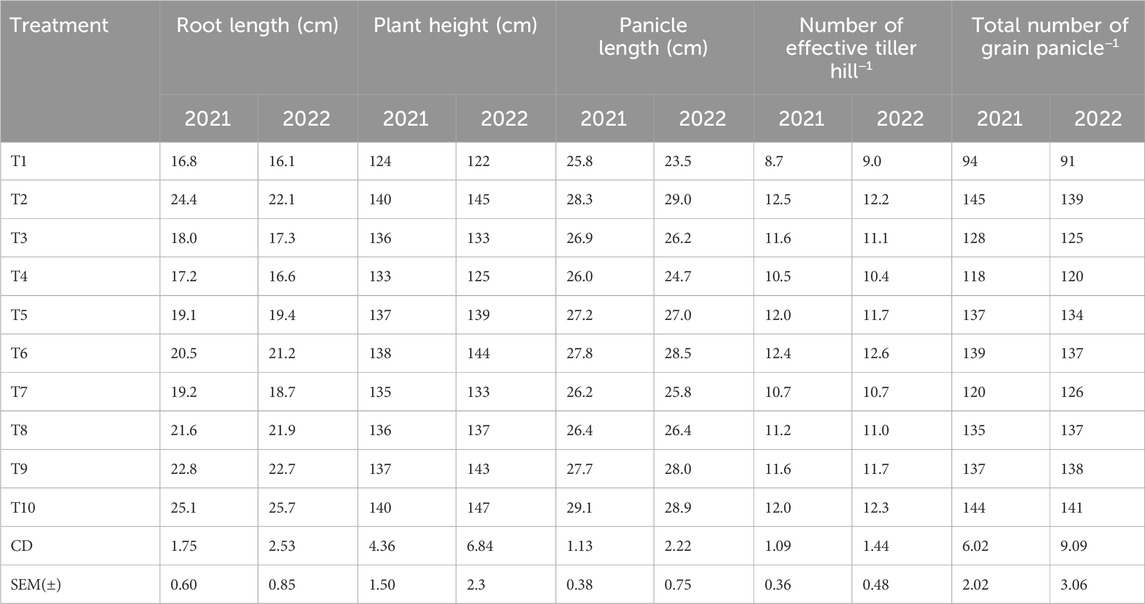
Table 2. Effects of different doses of nano-formulated N and P fertilizers on plant height and yield attributes of rice.
3.3 Grain and straw yield
The data on yield represented that soil application and seedling root dipping followed by foliar spraying of nano DAP caused a significant impact on grain and the straw yield of paddy compared to soil application alone (Table 3). The maximum mean grain and straw yields were recorded at T2 (4.09 and 5.21 Mg ha−1), which were at par with T10 (4.05 and 5.19 Mg ha−1), whereas the minimum mean grain and straw yields were recorded in T1 (2.42 and 3.61 Mg ha−1). However, the treatment T10 with a mean grain yield of (4.05 Mg ha−1) significantly excelled over the rest of the treatments, but it did not show a significant difference between the treatments, viz., T6 (3.8 Mg ha−1), T9 (3.77 Mg ha−1), and T2 (4.09 Mg ha−1).
3.4 Nutrient concentration, uptake, and use efficiency of rice
The concentrations of N, P, and K in rice grain and straw were estimated and are provided in Table 4. Soil application, seedling root dipping, and foliar fertilization of nano-formulated DAP resulted in the significantly increased concentrations of N, P, and K in grain and straws compared to the soil application alone and control in 2021–2022. The maximum nitrogen (1.43% and 0.77%) concentrations along with the phosphorous concentrations (0.27% and 0.16%) in both grain and straw, respectively, were observed in T10. The highest mean K concentrations of 0.57% and 1.29% in grain and straw, respectively, were recorded in T2, whereas the lowest concentration was shown in T1.
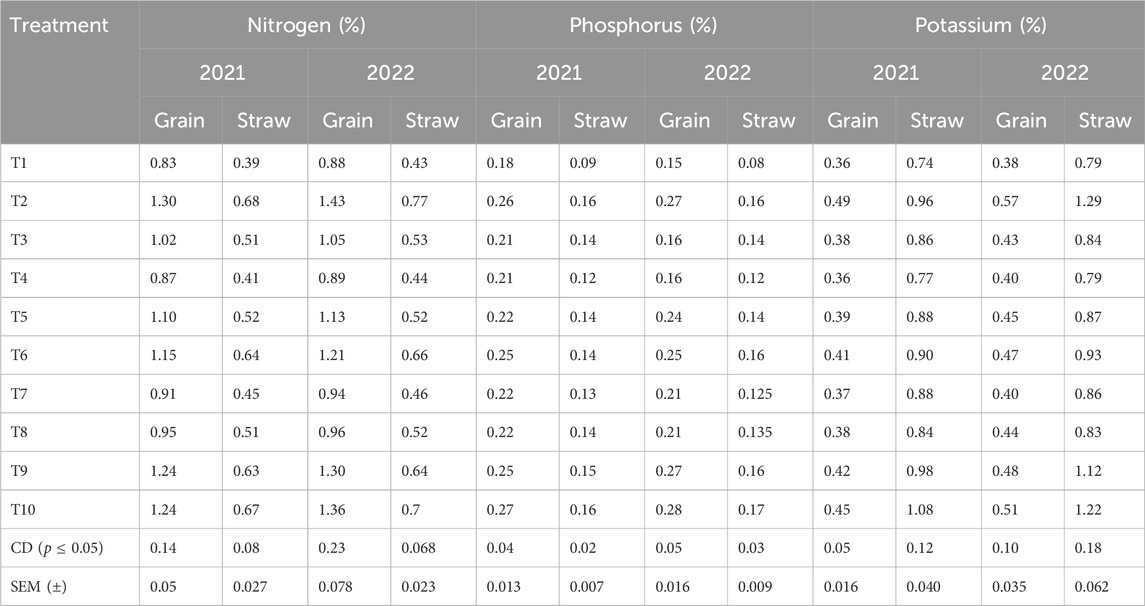
Table 4. Influence of different doses of nano-formulated N and P fertilizers on nutrient concentrations (%) of rice.
Based upon the findings of different levels of nano N and P applications, a beneficial effect on the mean uptake of major macronutrients is elucidated in Tables 5. The total N, P, and K uptakes in rice during 2021–2022 varied from 33.2 to 95.5, 7.9 to 20, and 36.2–84.4 kg ha−1, respectively. The significantly higher total N uptake of 89.3–97.4 kg ha−1 was obtained in T2 than that of control and others but at par with a value of 86.9–95.5 kg ha-1 in T10, followed by T9 of 78.9–81.8 kg ha−1. Similarly, treatment T10 had a higher total P uptake, i.e., 19.3–20 kg ha−1, followed by T2 (18.9–19.4) and T9 (16.9–18.2), respectively, which was at par among each other but significantly higher than other treatments. The higher total K uptake of 89.8 kg ha−1 was obtained in T2, which was at par with T10 (84.4). Moreover, the result specified that nano N and P fertilization treatments were found to be statistically superior in total macronutrient uptake by rice in T9 and T10 compared to other treatments.
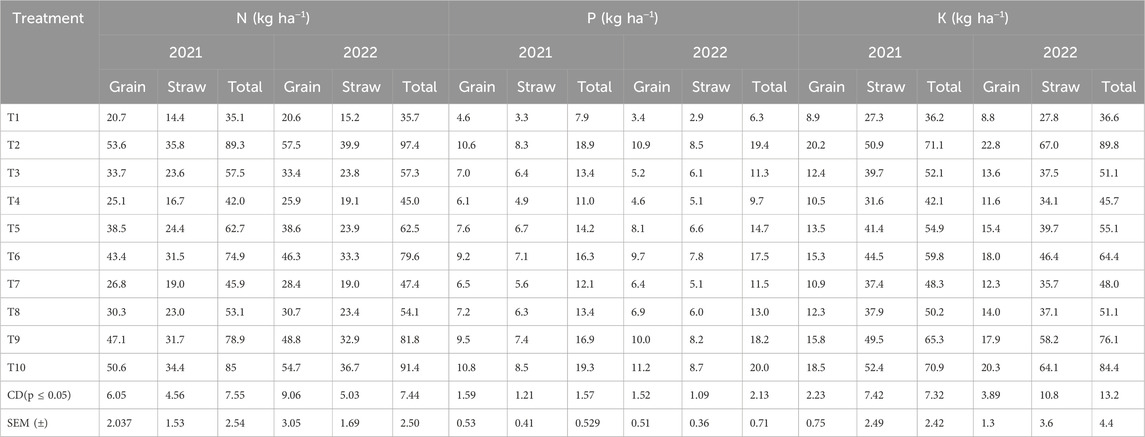
Table 5. Effects of different doses of nano-formulated P and N on nutrient uptake (kg ha−1) of rice.
The relative agronomic efficiency was achieved to be maximum (100%) for T2, which was not significant with T10 (97.7%) (Figure 2). The higher N and P use efficiencies of 33.5 (N) and 67(P)% were obtained in treatment T10, followed by 28 (N) and 56.1(P) % in T9, respectively, which is statistically more over all other treatments. The maximum N and P recovery values of 99.8% and 45.6%, respectively, were recorded with T10, followed by values of 87.26% and 36% of N and P, respectively, with T9.
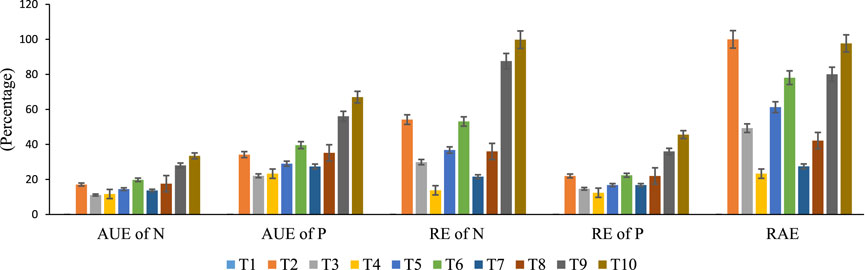
Figure 2. Effects of nano DAP on the mean use efficiency for nutrients of rice crop. *Agronomy use efficiency (AUE), apparent recovery efficiency (ARE), and relative agronomic efficiency (RAE).
3.5 Economy
A detailed breakup of the total cost of cultivation and return per hectare of each treatment is provided in Table 6. The highest mean cost of cultivation amount of 40,209₹ was observed in treatment T2, whereas the lowest amount of 35,086₹ was observed in T1 (Control). However, an amount of 39,017₹ and 38,854₹ was recorded in T6, followed by T10. The Gross return was calculated by adding return from both grain and straw. The MSP price for grain was 19,400 ₹ Mg−1 in 2021, followed by 20,400 ₹ Mg−1 in 2022, and for straw, the local price was 1,200 ₹ Mg−1 in 2021, followed by 1,000 ₹ Mg−1 in 2022, respectively. The highest mean return amount per hectare of 87,016₹ was recorded in T2, followed by the amount of 85,783₹ in T10, whereas the lowest mean return was recorded in T1 (52,195₹). Moreover, the B:C ratio of all the 10 treatments was above 1, as shown in Table 6, which indicates that treatment imposition in rice was on a positive trend. The highest benefit–cost ratio value of 2.26 was observed in treatment T10, which indicated that the average net return is 1.26₹ on an average investment of 1₹, followed by T2 (2.19) and T9 (2.18), whereas the lowest B: C ratio of 1.47 was recorded in T1.
3.6 Post-harvest soil properties
The post-harvest properties of soil under the experiment are enlisted in Table 7. The highest soil pH (5.68) in post-harvest soil was recorded in T10, and the minimum, in T5 (5.13). The soil applications of N and P, along with foliar fertilization of nano N and P treatments, resulted in a forward trend for soil pH, as compared to no foliar spray. For electrical conductivity (EC), a significant reduction was recorded in treatment T10 (0.24 ds m−1), whereas highest EC was observed in T5 (0.36 ds m−1). The available N content ranges from 112 to 186 kg ha−1. The lowest was observed in T1, whereas a significantly highest value of 186 kg ha−1 was recorded with T10 among all treatments. Similarly, soil available P content was influenced by different levels of P fertilization with ranges from 3.7 to 21 kg ha−1. A significantly highest available P content of 21 kg ha−1 was observed in T10, and the lowest value of 3.7 kg ha−1 was obtained in T1. However, the highest available K content of 86.2 kg ha−1 was estimated in treatment T1.
Experimental results pertaining to soil microbial biomass in 2021–22 are depicted in Figure 3. The data demonstrated that the application of nano N and P posed a significant impact on the microbial biomass carbon (MBC), microbial biomass nitrogen (MBN), and microbial biomass phosphorous (MBP). Perusal of results revealed that microbial biomass carbon (MBC) was higher in T2 (117.4–118.4 μg C g−1 soil), followed by T10 (109.2–113.2 μg C g−1 soil), which is significantly at par. Whereas the lowest value of (52.8–53.1 μg C g−1 soil) in unamended control plot T1. The highest MBN content in soil ranged from the highest values of 76.1–78.4 μg N g−1 in treatment T2, whereas the lowest values of 21.6–21.7 μg N g−1 soil in treatment T1 (control). T10 possesses ranges from 60 to 60.7 μg N g−1 soil. It was observed that the MBP content in different treatments was in the range of 2.3–7.3 μg P g−1 soil. Highest MBP was estimated in treatment T2 (9.6–9.8 μg P g−1 soil), whereas the lowest was estimated in control (2.3–2.4 μg P g−1 soil). Soil urease enzyme acts upon urea and organic N to release the plant available end product NH4+ through the process of mineralization. From the analysis of soil urease activity in 2021–2022, the result showed that urease activities were highest in T2 (66.8–67.7 μg NH4+-N g−1 soil hr−1), which was at par with T10 (60.8–61.7 μg NH4+-N g−1 soil hr−1), whereas the lowest activity was observed in control T1 (30.5–34.06 μg NH4+-N g−1 soil hr−1). Soil phosphatase enzyme acts upon the organic form of P and releases the end product of plant-available phosphate through the process of mineralization. Results pertaining on the phosphatase activity showed that it ranged between 127.1 and 156 μg p-nitrophenol g−1 soil hr−1. The highest phosphatase activity in 2021–22 was found in the treatment T2 (153.7 and 156 μg p-nitrophenol g−1 soil hr−1) and the lowest in control T1 (127.1 and 128.7 μg p-nitrophenol g−1 soil hr−1).
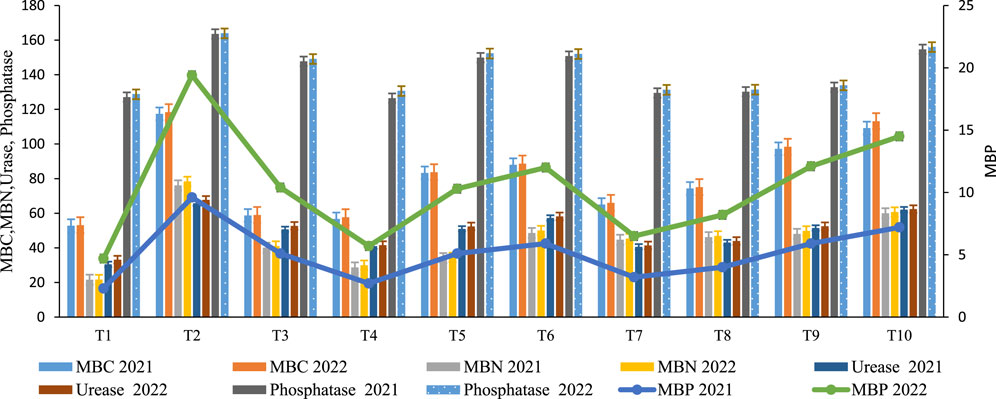
Figure 3. Effects of nano DAP on soil biological properties. * MBC, microbial biomass carbon (μg C g−1 soil); MBN, microbial biomass nitrogen (μg N g−1 soil); MBP, microbial biomass phosphorus (μg P g−1 soil); urease (μg NH4+-N g−1 soil hr−1); and phosphatase (μg p-nitrophenol g−1 soil hr−1).
4 Discussion
It was observed that the total chlorophyll content of rice leaves was found to be increased from 30 DAT to 54 DAT, irrespective of the nutrient doses. After 54 DAT, the chlorophyll content was decreased in all treatments except in T9 and T10, and this might be due to the comparative addition of more N and P for a longer period through a second foliar spray of nano DAP, which delayed the reproductive process, whereas the vegetative phase stretched beyond 54 DAT. Thereafter, the chlorophyll content was reduced where N and P was utilized for grain production during reproductive stages. The reason may be due to the utilization of nutrients for the production of total chlorophyll which were diverted toward the production of grains at the time of reproductive stages (Monica et al., 2020; Tari et al., 2013; Pramanik and Bera, 2013). A higher photosynthetic rate was acquired by applying 50% of the STD for N and P with nano DAP foliar spray than that of 100% of N and P from conventional fertilizer sources because of the higher amount of N and P obtained from foliar sprays of nano DAP (Villagomez et al., 2019). Higher growth and yield-attributing characteristics were recorded in T10 than that in T2 treatment. This might be due to improved nutrient absorption by plant cells, resulting in the optimal development of plant parts (Upadhyay et al., 2023) and metabolic processes such as photosynthesis, which result in increased photosynthates accumulation and translocation to plant’s economic portions (Benzon et al., 2015; Chavan, 2019; Monica et al., 2020). Two foliar sprays of nano DAP facilitated more nutrient absorption through the opening of stomata and nutrient translocation inside the plant, which enhance the yield attributing characteristics of rice. Nano fertilizers enhanced the activity of chloroplast, and the antioxidant enzyme system affects the yield attributing characteristics (Prusty et al., 2022; Hong et al., 2005; Nekrasova et al., 2011; Upadhyay et al., 2023).
The highest mean grain yield of T2 was due to the application of more amounts of N and P through soil application. The grain yield was increased in T10 due to the combined application of nitrogen and phosphorous through a foliar application of nano DAP and soil application. This might be due to improved absorption and translocation of nano forms of N and P, which creates a conducive environment for the higher production of rice grains (Deo et al., 2022; Poudel et al., 2023). The availability of N and P was enhanced due to the foliar application of nano DAP and soil application of N and P at 50% of the STD along with the seedling root dip, which facilitates the availability, translocation, and production of biomolecules such as proteins and carbohydrates and also helped in efficient root establishment (Chavan, 2019). Moreover, the highest straw yield was recorded with only soil application of inorganic fertilizers at 100% of STD for N and P due to the higher availability of nutrients for plant uptake. On the other hand, the straw yield was increased in T9, followed by T10. This might be due to the accessibility of nutrients by rice at various growth stages for a longer period through twice foliar applications of nano DAP, from which N and P can be easily taken up by plants (Poudel et al., 2023). In addition, the growth hormone, photosynthetic activities, and metabolic process enhance due to nano forms of fertilizers, resulting in increased biomass (Sivaleela et al., 2018; Kunta and Thatikunta, 2011; Valojai et al., 2021; Upadhyay et al., 2023).
The significantly highest nitrogen uptake in T2 and T10 was because of the higher and effective availability of N along with effective usage due to the soil application of nutrients in T2. However, in T10, availability of N and P was increased for a longer period due to the foliar application of nano DAP (Poudel et al., 2023). Similarly, phosphorus and potassium uptake was enhanced because of more availability and efficient utilization of nutrients through the application of nutrients as per 100% of STD or the addition of nano DAP. A positive interaction between phosphorus and nitrogen enhances the availability of other nutrients by creating a conducive environment for the better uptake of nutrients (Soliman et al., 2016). The increase in nutrient uptake might be due to the synchronization of the release of nutrients with the active crop growth stage for a longer release period, which leads to the effective utilization of nutrients through nano fertilizers (De Rosa et al., 2010; Chavan, 2019).
Different efficiencies of nitrogen and phosphorous concerning rice crops indicated that higher relative agronomic efficiency (RAE) based on grain yield, higher agronomic use efficiency (AUE) for nitrogen and phosphorus, and higher recovery efficiency of nitrogen and phosphorous were recorded in T10 (Deo et al., 2022; Hagab et al., 2018; Mejias et al., 2021). This might be due to the productive and coherent utilization of nutrients by plants due to the application of nano DAP. The nutrients smaller than the stomatal pore with a higher surface area are available to the plant for a longer period of time, for which its productivity was increased (Patil et al., 2020; Talboys et al., 2020).
The highest B:C ratio is obtained in T10, followed by T2. This might be due to the higher yield recorded with the soil application of nitrogen and phosphorous at 50% STD along with twice foliar sprays of nano DAP, whereas in T2, nitrogen and phosphorous were applied at 100% of STD to the soil, which leads to greater cost of cultivation, as compared to gross income.
The enrichment in available nitrogen and phosphorous levels in post-harvest soil could be attributed to decreased nutrient uptake from the soil as a result of the utilization of nano-formulated N and P fertilizer by crops, resulting in an increased amount of nutrients in soil after harvest (Soliman et al., 2016; Hasaneen et al., 2016). The nano fertilizers uplifted nutrient concentrations in the soil solution, developing higher osmotic potential of soil and reduction in nutrient absorption and resulting in leftover nutrients in the soil after harvesting (El-Azizy et al., 2021; Patil et al., 2020). The gradual increase in soil microbial biomass and enzyme activity is due to seedling root dipping with 5 mL L−1 nano N and P during transplanting, combined with 50% soil application of granular fertilizers at active growth stages, which improved root development and rhizosphere activity while also favoring the soil enzymatic activity (Maloth et al., 2024). A significant increase in MBC, MBN, and MBP was seen with the combined application of nano N and P compared to soil application. Furthermore, phosphatase activity was closely associated with the favorable properties of nanomaterials (Sahu et al., 2023). The gradual decomposition of nano fertilizers enhances the diversity and quantity of microbes, consequently enhancing enzymatic activity and minimizing loss.
5 Conclusion
Based on the abovementioned findings, it may be concluded that the application of nano-formulated N and P fertilizers combined with seedling root dipping and soil application has immense impact on yield, nutrient uptake, soil microbial biomass, and enzyme activity, as well as post-harvest soil properties of rice. The application of 50% STD + SRD with 5 mL L−1 nano DAP + twice foliar sprays of nano N and P at 25 and 45 DAT (T10) validated superior efficacy for achieving higher growth, yield, and yield-attributing properties and simultaneously conserved 50% soil application of conventional fertilizer. The findings suggested that nano DAP formulation of N and P as a novel liquid fertilizer could serve as a promising substitute for traditional DAP in precision agriculture due to its improved fertilizer use efficiency. Additionally, it is worth noting that the unit cost of nano fertilizers is comparatively low. Further research trials need to be conducted to gain a better standardization of nano DAP doses and its application, as well as effectiveness of foliar fertilization.
Data availability statement
The original contributions presented in the study are included in the article/Supplementary Material; further inquiries can be directed to the corresponding author.
Author contributions
BS: formal analysis, methodology, and writing–original draft. AD: conceptualization, funding acquisition, investigation, methodology, project administration, validation, and writing–review and editing. KM: data curation, software, visualization, and writing–review and editing. SM: formal analysis, methodology, software, and writing–review and editing. SS: formal analysis, funding acquisition, project administration, resources, supervision, and writing–review and editing. BS: data curation, funding acquisition, software, validation, visualization, and Writing–review and editing. MP: data curation, formal analysis, supervision, and writing–review and editing. EP: data curation, formal analysis, software, supervision, and writing–review and editing.
Funding
The author(s) declare that no financial support was received for the research, authorship, and/or publication of this article.
Acknowledgments
The authors are thankful to Dr. Yogendra Kumar, Dr. Tarunendu Singh, S. K. Chatterjee, Prof. (Dr) Manojranjan Nayak, Adarsha Divyadarshan for providing encouragement and Indian Farmers Fertiliser Cooperative Limited (IFFCO) for providing nano materials and funding for conduct of this experiment.
Conflict of interest
The authors declare that the research was conducted in the absence of any commercial or financial relationships that could be construed as a potential conflict of interest.
Publisher’s note
All claims expressed in this article are solely those of the authors and do not necessarily represent those of their affiliated organizations, or those of the publisher, the editors, and the reviewers. Any product that may be evaluated in this article, or claim that may be made by its manufacturer, is not guaranteed or endorsed by the publisher.
References
Akinrinde, E. A., Bello, O. S., Ayegboyin, K. O., and Iroh, L. (2005). Added benefits of combined organic and mineral phosphate fertilizers applied to maize and melon. J. Agric. Food Environ. 3 (3/4), 75.
Alam, M. S., Khanam, M., and Rahman, M. M. (2023). Environment-friendly nitrogen management practices in wetland paddy cultivation. Front. Sustain. food Syst. 7, 1020570. doi:10.3389/fsufs.2023.1020570
Astaneh, S. H., Faverani, L. P., Sukotjo, C., and Takoudis, C. G. (2021). Atomic layer deposition on dental materials: processing conditions and surface functionalization to improve physical, chemical, and clinical properties-A review. Acta Biomater. 121, 103–118. doi:10.1016/j.actbio.2020.11.024
Bal, S. K., Sattar, A., Chandran, M. A. S., Rao, A. V. M. S., Manikandan, N., Banerjee, S., et al. (2023). Critical weather limits for paddy rice under diverse ecosystems of India. Front. Plant Sci. 14, 1226064. doi:10.3389/fpls.2023.1226064
Benzon, H. R. L., Rubenecia, M. R. U., Ultra, V. U., and Lee, S. (2015). Chemical and biological properties of paddy soil treated with herbicides and pyroligneous acid. Int. J. Agric. Res. 7 (1), 105–117. doi:10.5539/jas.v7n4p20
Bray, R. H., and Kurtz, L. T. (1945). Determination of total organic and available forms of phosphorus in soils. Soil Sci. 59, 39–46. doi:10.1097/00010694-194501000-00006
Brookes, P. C., Powlson, D. S., and Jenkinson, D. S. (1982). Measurement of microbial biomass phosphorus in soil. Soil Biol. biochem. 14, 319–329. doi:10.1016/00380717(82)90001-3
Burhan, M. G., and Al-Hassan, S. A. (2019). Impact of Nano NPK fertilizers to correlation between productivity, quality and flag leaf of some bread wheat varieties. Iraqi J. Agric. Sci. 50. doi:10.36103/ijas.v50iSpecial.171
Chavan, Y. S. (2019). Response of rice to age of seedlings, crop geometry and nano-fertilizers in terms of yield, economics, nutrient content and uptake pattern in konkan region. Int. J. Agric. Sci. 11 (18), 9106–9109.
Deo, H. R., Chandrakar, T., Srivastava, L. K., Nag, N. K., Singh, D. P., and Thakur, A. (2022). Effect of Nano-DAP on yield, nutrient uptake and nutrient use efficiency by rice under Bastar plateau. J. Pharm. Innov. 11 (9), 1463–1465.
De Rosa, M. C., Monreal, C., Schnitzer, M., Walsh, R., and Sultan, Y. (2010). Nanotechnology in fertilizers. Nat. Nanotechnol. 5 (2), 91. doi:10.1038/nnano.2010.2
El-Azizy, F. A., Habib, A. A. M., and Am, A. E. B. (2021). Effect of nano phosphorus and potassium fertilizers on productivity and mineral content of broad bean in North Sinai. J. Agric. Eng. 12 (4), 239–246. doi:10.21608/jssae.2021.161844
FAI (2022). Fertiliser stat book. The fertiliser association of India. Available at: https://fertiliserindia.com/wp-content/uploads/2023/05/Fertiliser-Stat-Book-2021-22.pdf.
FAOSTAT (2016). Food and agriculture organization of the united nations (FAO). FAOSTAT database. Available at: http://faostat.fao.org/site/291/default.aspx.
Gomez, K. A., and Gomez, A. A. (1984). Statistical procedures for agricultural research. John Wiley & Sons.
Hagab, R. H., Yousra, H. K., and Eissa, D. (2018). Using nanotechnology for enhancing phosphorus fertilizer use efficiency of peanut bean grown in sandy soils. J. Adv. Pharm. Res. 8 (3), 59–67.
Hanyabui, E., ObengApori, S., AgyeiFrimpong, K., Atiah, K., Abindaw, T., Ali, M., et al. (2020). Phosphorus sorption in tropical soils. AIMS Agric. Food 5 (4), 599–616. doi:10.3934/agrfood.2020.4.599
Hasaneen, M. N. A. G., Abdel-aziz, H. M. M., and Omer, A. M. (2016). Effect of foliar application of engineered nanomaterials: carbon nanotubes NPK and chitosan nanoparticles NPK fertilizer on the growth of French bean plant. Biochem. Biotechnol. Res. 4 (4), 68–76.
Hong, F., Zhou, J., Liu, C., Yang, F., Wu, C., Zheng, L., et al. (2005). Effect of nano-TiO 2 on photochemical reaction of chloroplasts of spinach. Biol. Trace Elem. Res. 105, 269–280. doi:10.1385/BTER:105:1-3:269
Jackson, M. L. (1973). Soil chemical analysis. New Delhi, India: Pentice hall of India Pvt. Ltd., 498, 151–154.
Jatav, S. S., Singh, S. K., Parihar, M., Alsuhaibani, A. M., Gaber, A., and Hossain, A. (2022). Application of sewage sludge in a rice (Oryza sativa L.) wheat (Triticum aestivum L.) system influences the growth, yield, quality and heavy metals accumulation of rice and wheat in the Northern Gangetic Alluvial Plain. Life 12 (4), 484–84. doi:10.3390/life12040484
Joergensen, R. G. (1996). The fumigation-extraction method to estimate soil microbial biomass: calibration of the kEC value. Soil Biol. biochem. 28 (1), 25–31. doi:10.1016/0038-0717(95)00102-6
Joudeh, N., and Linke, D. (2022). Nanoparticle classification, physicochemical properties, characterization, and applications: a comprehensive review for biologists. J. Nanobiotechnology. 20 (1), 262. doi:10.1186/s12951-022-01477-8
Kaufman, R. C., Wilson, J. D., Bean, S. R., Presley, D. R., Blanco-Canqui, H., and Mikha, M. (2013). Effect of nitrogen fertilization and cover cropping systems on sorghum grain characteristics. J. Agr. Food Chem. 61, 5715–5719. doi:10.1021/jf401179n
Kunta, R., and Thatikunta, R. (2011). Influence of nitrogen levels on physiological response, nitrogen use efficiency and yield of rice (Oryza sativa L.) genotypes. Curr. Appl. Sci. Technol. 39 (48), 145–152. doi:10.9734/cjast/2020/v39i4831211
Lee, Y. C., and Moon, J. Y. (2020). “Introduction to nanotechnology and bio nanotechnology.” in Introduction to bio nanotechnology, 1–14. doi:10.1007/978-981-15-1293-3_1
Lichtenthaler, H. K. (1987). [34] Chlorophylls and carotenoids: pigments of photosynthetic biomembranes. Methods Enzym. 148, 350–382. doi:10.1016/0076-6879(87)48036-1
Maloth, A., Thatikunta, R., Parida, B. K., Naik, D. S., and Varma, N. (2024). Evaluation of nano-DAP on plant growth, enzymatic activity and yield in paddy (Oryza sativa L.). Int. J. Environ. Clim. 14 (1), 890–897. doi:10.9734/ijecc/2024/v14i13907
Maqbool, A., Ali, S., Rizwan, M., Arif, M. S., Yasmeen, T., Riaz, M., et al. (2020). N-fertilizer (urea) enhances the phytoextraction of cadmium through solanumnigrum L. Int. J. Environ. Res. Public Health. 17 (11), 3850. doi:10.3390/ijerph17113850
Mboyerwa, P. A., Kibret, K., Mtakwa, P., and Aschalew, A. (2022). Greenhouse gas emissions in irrigated paddy rice as influenced by crop management practices and nitrogen fertilization rates in eastern Tanzania. Front. Sustain. food Syst. 6, 868479. doi:10.3389/fsufs.2022.868479
Mboyerwa, P. A., Kibret, K., Mtakwa, P. W., and Aschalew, A. (2021). Evaluation of growth, yield, and water productivity of paddy rice with water-saving irrigation and optimization of nitrogen fertilization. Agronomy 11 (8), 1629. doi:10.3390/agronomy11081629
Mejias, J. H., Salazar, F., Pérez Amaro, L., Hube, S., Rodriguez, M., and Alfaro, M. (2021). Nano fertilizers: a cutting-edge approach to increase nitrogen use efficiency in grasslands. Front. Environ. Sci. 9, 52. doi:10.3389/fenvs.2021.635114
Mitra, B., Singha, P., Roy Chowdhury, A., Sinha, A. K., Skalicky, M., Brestic, M., et al. (2023). Normalized difference vegetation index sensor-based nitrogen management in bread wheat (Triticum aestivum L.): nutrient uptake, use efficiency, and partial nutrient balance. Front. Plant Sci. 14, 1153500. doi:10.3389/fpls.2023.1153500
Mohapatra, K. K., Nayak, A. K., Patra, R. K., Tripathi, R., Swain, C. K., Moharana, K. C., et al. (2023). Multi-criteria assessment to screen climate smart rice establishment techniques in coastal rice production system of India. Front. Plant Sci. 14, 1130545. doi:10.3389/fpls.2023.1130545
Mohapatra, S., Tripathy, S. K., Mohanty, A. K., and Tripathy, S. (2021). Effect of heat stress on yield and economics of rice (Oryza sativa L.) cultivars under different sowing dates. J. Agric. Meteorol. 23 (1), 38–45. doi:10.54386/jam.v23i1.86
Monica, M., Dash, A. K., Panda, N., and Prusty, M. (2020). Effect of urea phosphate foliar supplementation on yield, nutrient uptake and grain quality of rice (Oryza sativa L.) in acid Inceptisols. ORYZA-An Int. J. Rice 57 (2), 132–138. doi:10.35709/ory.2020.57.2.7
Munda, S., Saha, S., and Adak, T. (2018). Rice nursery bed preparation and management. Indian Farming. Delhi, India 68 (5). Available at: https://epubs.icar.org.in/index.php/IndFarm/article/view/81906.
Nekrasova, G. F., Ushakova, O. S., Ermakov, A. E., Uimin, M. A., and Byzov, I. V. (2011). Effects of copper (II) ions and copper oxide nanoparticles on Elodeadensa planch. Russ. J. Ecol. 42 (6), 458–463. doi:10.1134/S1067413611060117
Page, A. L., Millar, R. H., and Keeney, D. R. (1982). Phosphorus, methods of soil analysis, 9, part-II. Madison, WI: American Society of Agronomy, Inc. Publisher, 416–417.
Patil, S. S., Balpande, S. S., Mairan, N. R., Mohammad, S., and Ghodpage, R. M. (2020). Influence of integrated nutrient management using nano phosphatic fertilizer on nutrient use efficiency and yield of wheat (Triticum aestivum L.) in Vertisols. Int. J. Chem. Stud. 8 (6), 757–762. doi:10.22271/chemi.2020.v8.i6k.10860
Poudel, A., Singh, S. K., Jiménez-Ballesta, R., Jatav, S. S., Patra, A., and Pandey, A. (2023). Effect of nano-phosphorus formulation on growth, yield and nutritional quality of wheat under semi-arid climate. Agronomy 13 (3), 768. doi:10.3390/agronomy13030768
Pramanik, K., and Bera, A. K. (2013). Effect of seedling age and nitrogen fertilizer on growth, chlorophyll content, yield and economics of hybrid rice. Intl J. Agron. Plant Prod. 4, 3489–3499.
Prusty, M., Swain, D., Alim, M. A., Ray, M., and &Sethi, D. (2022). Effect of integrated nutrient management on yield, economics and post-harvest soil properties of sweet corn grown under mid-central table land zone of odisha. Int. J. Plant Sci. 34 (14), 55–61. doi:10.9734/ijpss/2022/v34i1430990
Qiao, J., Wang, J., Zhao, D., Zhou, W., Schwenke, G., Yan, T., et al. (2022). Optimizing N fertilizer rates sustained rice yields, improved N use efficiency, and decreased N losses via runoff from rice-wheat cropping systems. Agric. Ecosyst. Environ. 324, 107724. doi:10.1016/j.agee.2021.107724
Sahu, D., Banwasi, R., Shrivastava, L. K., Chowdhury, T., Jatav, G., and Kumar, H. (2023). Performance of nano-DAP under rice-wheat cropping system in vertisol of Chhattisgarh,India. Int. J. Environ. Clim. 13 (9), 2864–2875. doi:10.9734/ijecc/2023/v13i92521
Samreen, T., Rasool, S., Kanwal, S., Riaz, S., and Nazir, M. Z. (2022). Role of nanotechnology in precision agriculture. Environ. Sci. Proc. 23 (1), 17. doi:10.3390/environsciproc2022023017
Sivaleela, S., Srinivas, M., Pullarao, C., and Sireesha, A. (2018). Influence of Nitrogen on economics of medium duration rice varieties. Int. J. Curr. Microbiol. App. Sci. 7 (12), 915–920. doi:10.20546/ijcmas.2018.712.114
Soliman, A. S., Hassan, M., Abou-Elella, F., Ahmed, A. H., and El-Feky, S. A. (2016). Effect of nano and molecular phosphorus fertilizers on growth and chemical composition of Baobab (Adansonia digitata L.). J. Plant Sci. 11 (4), 52–60. doi:10.3923/jps.2016.52.60
Subbaiah, B. V., and Asija, G. L. (1956). A rapid procedure for estimation of available nitrogen in soils. Curr. Sci. 25, 259–260.
Tabatabai, M. A., and Bremner, J. M. (1972). Michaelis constants of soil enzymes. Soil Biol. biochem. 3 (4), 317–323. doi:10.1016/0038-0717(71)90041-1
Talboys, P. J., Healey, J. R., Withers, P. J. A., Roose, T., Edwards, A. C., Pavinato, P. S., et al. (2020). Combining seed dressing and foliar applications of phosphorus fertilizer can give similar crop growth and yield benefits to soil applications together with greater recovery rates. Front. Agron. 2, 605655. doi:10.3389/fagro.2020.605655
Tarafdar, J. C. (2020). Novel bioformulations for nano-phosphorus synthesis and its use efficiency. Indian J. Fert. 16 (12), 1278–1282.
Tari, D. B., Daneshian, J., Amiri, E., Rad, A. H. S., and Moumeni, A. (2013). Investigation chlorophyll condition at different nitrogen fertilization methods in rice by applied mathematics relations. J. Sci. Res. 14, 1056–1058. doi:10.5829/idosi.mejsr.2013.14.8.586
Upadhyay, P. K., Singh, V. K., Rajanna, G. A., Dwivedi, B. S., Dey, A., Singh, R. K., et al. (2023). Unveiling the combined effect of nano fertilizers and conventional fertilizers on crop productivity, profitability, and soil well-being. Front. Sustain. Food Syst. 7, 1260178. doi:10.3389/fsufs.2023.1260178
Valojai, S. T., Niknejad, Y., Fallah, H., and Tari, D. B. (2021). Effect of nitrogen, phosphorus and potassium nano-fertilizers on growth and seed of two rice (Oryza sativa L.) cultivars. J. Crop Ecophysiol. 15 (1). doi:10.30495/jcep.2021.681005
Vance, E. D., Brookes, P. C., and Jenkinson, D. S. (1987). An extraction method for measuring soil microbial biomass carbon. Soil Biol. Biochem. 19, 703–706. doi:10.1016/0038-0717(87)90052-6
Villagomez, M., Tellez, E. T., Merino, L. G. I., Sandoval-Villa, F. C., García, P. S., and Aguilar-Mendez, M. A. (2019). Nano phosphors fertilizer stimulates growth and photosynthetic activity and improves P status in rice. J. Nanomater., 5368027. doi:10.1155/2019/5368027
Xia, Y., Zhang, M., Tsang, D. C., Geng, N., Lu, D., Zhu, L., et al. (2020). Recent advances in control technologies for non-point source pollution with nitrogen and phosphorous from agricultural runoff: current practices and future prospects. Appl. Biol. Chem. 63 (1), 8–13. doi:10.1186/s13765-020-0493-6
Zhang, L., Zhang, X., Gao, Q., and Yan, L. (2023). Nitrogen application effect on maize yield, NH3, and N2O emissions in northeast China by meta-analysis. Agronomy 13 (6), 1479. doi:10.3390/agronomy13061479
Keywords: rice, nano N, nano P, foliar application, nutrient uptake
Citation: Sahoo BR, Dash AK, Mohapatra KK, Mohanty S, Sahu SG, Sahoo BR, Prusty M and Priyadarshini E (2024) Strategic management of nano-fertilizers for sustainable rice yield, grain quality, and soil health. Front. Environ. Sci. 12:1420505. doi: 10.3389/fenvs.2024.1420505
Received: 20 April 2024; Accepted: 12 August 2024;
Published: 16 September 2024.
Edited by:
Krishnaswamy Jayachandran, Florida International University, United StatesReviewed by:
Sangeeta Lenka, Indian Institute of Soil Science (ICAR), IndiaPankaj Kumar Mishra, ICAR-Vivekananda Parvatiya Krishi Anusandhan Sansthan, India
Copyright © 2024 Sahoo, Dash, Mohapatra, Mohanty, Sahu, Sahoo, Prusty and Priyadarshini. This is an open-access article distributed under the terms of the Creative Commons Attribution License (CC BY). The use, distribution or reproduction in other forums is permitted, provided the original author(s) and the copyright owner(s) are credited and that the original publication in this journal is cited, in accordance with accepted academic practice. No use, distribution or reproduction is permitted which does not comply with these terms.
*Correspondence: Bidwan Ranjan Sahoo, Ymlkd2Fuc2Fob29Ac29hLmFjLmlu