- 1School of Chemistry and Chemical Engineering, Sichuan University of Arts and Science, Dazhou, China
- 2Soil Fertilizer and Ecological Construction Workstation, Dazhou, China
This study aims to quantitatively evaluate the effectiveness of modified biochar in reducing soil Cd availability and Cd content in plants by performing a meta-analysis using data from 58 papers. Modified biochar enhances the surface function and adsorption efficiency of biochar, making it a cost-effective modifier for immobilizing heavy metals. Various factors influence the cadmium (Cd) passivation effect of modified biochar, including the materials used, modification methods, and specific modified materials, warranting further research and clarification. On average, the application of modified biochar results in a significant reduction in soil Cd availability by 65.01% and Cd content in plants by 70.72%. Notably, biochar made from lignocellulose as a raw material exhibits an impressive reduction effect of 96.34% on soil Cd availability. Furthermore, organic modified biochar and acid-base modified biochar demonstrate reduction effects of 119.53% and 112.03%, respectively, for soil Cd availability. When considering plant uptake, modified biochar composed of herbs and combined modified biochar show better Cd reduction effects, with respective reductions of 100.53% and 88.87%. The optimal application rate of modified biochar falls within the range of 2%–3%, leading to a reduction of effective Cd in soil by 76.94% and Cd content in plants by 88.88%. This meta-analysis underscores the potential of modified biochar in remediating Cd-contaminated soil, offering valuable insights for the preparation and application of this material.
Introduction
The high toxicity, mobility, and bioavailability of cadmium in soil make soil cadmium pollution a significant threat to the environment and human health (Xiao et al., 2018; Liu et al., 2019). Cd is widely present in the environment, with human activities exacerbating its environmental impact, particularly leading to soil pollution (Godt et al., 2006). Recognized as a priority pollutant as early as 1974 by the United Nations Environment Program and the International Labor Health Committee on Heavy Metals, Cd exposure has been linked to various health issues, including renal and hepatic dysfunction, testicular damage, and cancers (Stohs et al., 2001; Chaney, 2015). China’s 2014 National Soil Pollution Status Survey Bulletin showed that 7.0% of monitoring sites exceeded the standard for Cd, and 19.4% of arable soil monitoring sites exceeded the standard. Therefore, it is necessary to remediate soil Cd pollution. To address Cd contamination in soils, different remediation approaches have been developed, such as soil washing and phytoremediation (Cheng et al., 2022; Deng et al., 2022; Xu et al., 2022; Guo et al., 2023). One particular method, immobilization, is considered cost-effective and eco-friendly (Hussain et al., 2021; Yuan et al., 2021). This technique involves the use of soil passivators like biochar, lime, and sepiolite to reduce the bioavailability and activity of Cd in soil (Turan et al., 2018; Xia et al., 2019; Yuan et al., 2021).
Biochar, a solid material resultant from the thermochemical conversion of biomass with restricted oxygen input, possesses a vast specific surface area, micro/mesoporous structure, and an abundance of functional groups, rendering it useful in a wide array of applications including soil enhancement, remediation, water pollution treatment, crop yield improvement, biological carbon fixation, and more. Studies have consistently demonstrated biochar’s capability in diminishing the mobility and bioavailability of Cd in soil. Research conducted by Maharlouei et al. (2021) revealed a 12.63% reduction in soil exchangeable Cd with the application of rice hull biochar, which was enhanced further by modifications involving Fe3O4, NaOH, and H2SO4, resulting in reductions of 38.54%, 56.10%, and 23.12% respectively. Additionally, investigations by Shan et al. (2020) illustrated the efficacy of Mg-modified biochar in diminishing soil Cd bioavailability and content in spinach shoots and roots when compared to peanut shell biochar. Notably, modified biochar has garnered considerable attention in environmental studies owing to its potential in bolstering the availability, exchangeability, and bioavailability of soil Cd. For instance, Yin et al. (2022) reported a remarkable 40.7% and 33.7% decrease in Cd levels in lettuce shoots and roots, respectively, following the application of water hyacinth biochar at a 1% rate. Similarly, Chen et al.'s (2020) research findings show that the available Cd content in soil decreased significantly by 15.86% and 22.72% after treatments with wheat straw biochar and sulfur-modified wheat straw biochar, compared to the control. In a soil-based pot experiment, both MnBC and FeBC were found to significantly lower soil Cd availability by 23%–38% and 36%–45%, respectively, in comparison to the control group (p < 0.05) (Da et al., 2023). The efficacy of modified biochar in reducing soil Cd availability is influenced by a variety of factors, including the materials used for modification, modification techniques, and soil characteristics. Despite the demonstrated effectiveness of modified biochar in reducing the availability, exchangeability, and bioavailability of soil Cd, the specific impact may vary based on these factors.
A meta-analysis is a useful tool to comprehensively evaluate the remediation effect of biochar based on large datasets (Tian et al., 2021). Recently, more and more studies have been conducted on the impact of modified biochar on Cd in soil (He et al., 2022; Yin et al., 2022), and these new studies can provide us with reliable datasets to evaluate the impact of modified biochar on Cd in soil. However, there is limited meta-analysis research on the remediation effect of modified biochar on Cd in soil. By analyzing these studies, we can gain a deeper understanding of how modified biochar influences the concentration of Cd in soil and its potential remediation effects. This lacuna in research highlights the need for further meta-analyses to synthesize findings and draw more comprehensive conclusions regarding the efficacy of modified biochar in remediating Cd-contaminated soil.
In this study, we aimed to systematically investigate the influence of modified biochar on the availability of heavy metals in soil and plant absorption through a meta-analysis of existing research data. Our research objective was to gain insights into the most effective types of modified biochar and assess the impact of different modification methods and soil types on the availability of Cd. Various factors, such as soil physical and chemical properties, biochar raw material type, application rate, preparation temperature, modified materials, and modification methods, were taken into account. The analysis of these factors was conducted to offer valuable insights into the optimal utilization of modified biochar for heavy metal remediation.
Materials and methods
Data sources and compilation
To compile relevant information, searches were conducted on the online databases Web of Science (WOS) (http://apps.web of knowledge.com) and the Chinese National Knowledge Infrastructure (CNKI) (https://www.cnki.net/) on 10 April 2023, using the keywords biochar, modified, soil, and Cd. The literature resulting from this search was thereafter screened based on three criteria: 1) the study must involve original research on the behaviors of Cd in contaminated soils induced by biochar application; 2) treatments and controls must have at least three replicates; and 3) original data should be expressed as the mean ± standard deviation (SD) or mean ± standard error (SE), and should be extractable from tables or figures. After this process (Supplementary Figure S1), 58 articles met the criteria for meta-analysis, as detailed in Supplementary Table S1; of those, 22 articles included data on the availability of soil Cd and plant Cd. The observation results included 239 soil Cd availability observations and 121 plant Cd observations. For studies that included multiple determinations over the course of the experimental period, the results were extracted from the last sampling period, as per the principle of meta-analysis (Hedges et al., 1999). Database information included the properties of biochar, soil, plants, and experimental conditions from each manuscript. Soil Cd availability or plant Cd were employed as response indicators (dependent variables) for this study, with data being extracted directly from tables or figures using the origin Graph Digitizer. The indicators collected included soil pH (<5.5, 5.5–6.5, 6.5–7.5 and ≥7.5), soil organic matter (0–10, 10–20, 20–30 and >30 mg/kg), soil cation exchange capacity (0–10, 10–20, 20–30 and ≥30 mmol/kg), total Cd in soil (<1, 1–5, 5–15, 15–50 and ≥50 mg/kg), feedstock (wood, bio-solid, lignocellulosic, herbaceous), modified materials (metal, Nonmetallic, organic, acid alkali, combination, ultraviolet rays and other), modification methods or temperatures [ultraviolet rays, impregnation, in situ synthesis (400–500°C, 500–600°C, ≥50°C)], application rate (<1%, 1%–2%, 2%–3%, 3%–5%, ≥5%), soil Cd availability, Cd content in plant aboveground or edible parts, and SD.
Meta-analysis
Effect sizes of biochar application on the bioavailability in soils and the accumulation in plants for As and Cd were expressed as the natural log-transformed response ratio (ln (RR)), and were calculated by Eq. 1 (Hedges et al., 1999):
where Xtreat and Xcontrol are the means of the treatments and controls, respectively.
Variance (ν) of ln (RR) was computed by Eq. 2:
where SDtreat and SDcontrol represent the standard deviations of the treatments and control groups, respectively; and ntreat and ncontrol represent the sample sizes of the treatments and control groups, respectively.
Weighted mean response ratio (ln (RR++)) was calculated by Eq. 3 (Tian et al., 2021):
where the weighting of each response ratio (w) is the reciprocal of its variance (Sha et al., 2019), m is the number of groups, k is the number of observations in The i th group (Tian et al., 2021).
The percentage change was converted by Eq. 4 (Tian et al., 2021):
For each tested variable, ln (RR) = 0 represents no effect; ln (RR) > 0 shows an increased (i.e., negative) effect; ln (RR) < 0 presents a reduced (i.e., positive) effect (Borchard et al., 2019; Tian et al., 2021).
Microsoft Office 2016 (Microsoft, United States) was used to collect, compile, and manage the database. Meta-analysis was performed via MetaWin statistics software (Version 2.1) (Hedges et al., 1999). Origin2018 was utilized to draw figures.
Results
Comparing the effects of modified biochar and raw biochar on Cd availability
Figure 1 displays the percentage changes in Cd availability in soil and Cd content in plant edible parts following the application of raw biochar and modified biochar, relative to raw biochar. Compared with the control group without biochar, the average availability of Cd in soil was reduced by 32.63% [26.41%, 38.84%] after applying the raw biochar (Figure 1A). However, the application of modified biochar reduced the availability of Cd in soil by 65.32%. Furthermore, compared with the raw biochar, the application of modified biochar decreased the availability of Cd in soil by 29.96% (Figure 1A). It also resulted in a decrease in Cd content in the edible parts of plants by 70.72% (Figure 1B). Therefore, modified biochar demonstrates the potential to reduce both the availability of Cd in soil and the Cd content in edible parts of plants (Figure 1).
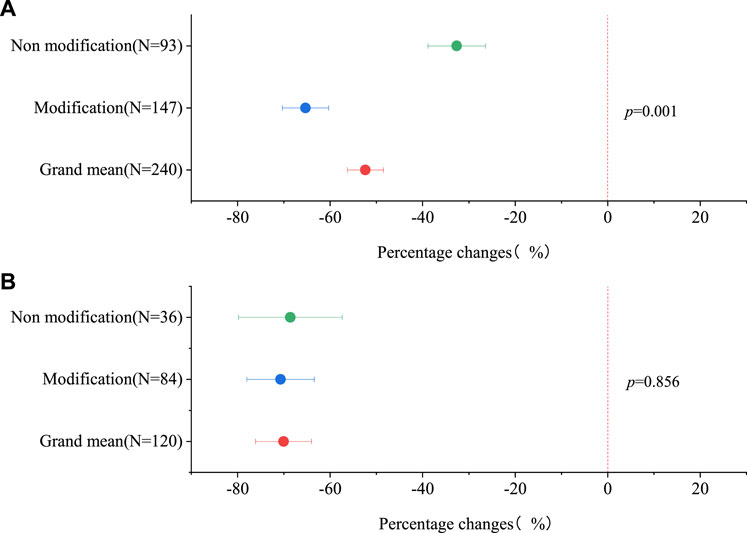
Figure 1. The Percentage changes variation of Cd availability in soil (A) and Cd content in edible parts of plants (B).
Changes in the efficiency of modified biochar with the characteristics of biochar
In Figure 2, the Cd availability demonstrated variation corresponding to different modified biochar characteristics. Among the subgroups of feedstock, biochar derived from lignocellulosic biomass exhibited the most significant reduction in Cd availability (96.34% [85.27%, 107.41%]), followed by wood biochar (62.88% [44.55%, 81.20%]). In the subgroup of modified materials, the reduction in soil Cd availability was most pronounced with organic modification (119.53% [97.77%, 141.30%]), followed by acid alkali modification (112.03% [78.09%, 145.98%]), nonmetallic modification (76.21% [33.23%, 119.20%]), combination modification (73.16% [57.13%, 89.20%]), ultraviolet ray modification (52.19% [9.96%, 94.42%]), other modification (53.99% [22.83%, 85.15%]), and metal load modification (47.44% [37.92%, 56.95%]). The in situ synthesis method achieves the maximum reduction in soil Cd availability when the pyrolysis temperature after in situ synthesis exceeds 500°C–600°C, resulting in a 76.68% [60.09%, 93.26%] reduction. In comparison, the impregnation method of modifying biochar shows a slightly lower effectiveness in reducing soil Cd availability than the in situ synthesis method. The relationship between the application rate and decrease in soil Cd availability is not as simple as higher application rates, resulting in greater decreases. Instead, the relationship can be represented as 1% (48.34% [36.27%, 60.41%]) < 1%–2% (66.46% [51.25%, 81.66%]) < ≥5% (71.57% [51.77%, 91.36%]) < 2%–3% (76.94% [61.48%, 92.40%]) < 3%–5% (77.30% [61.07%, 93.54%]).
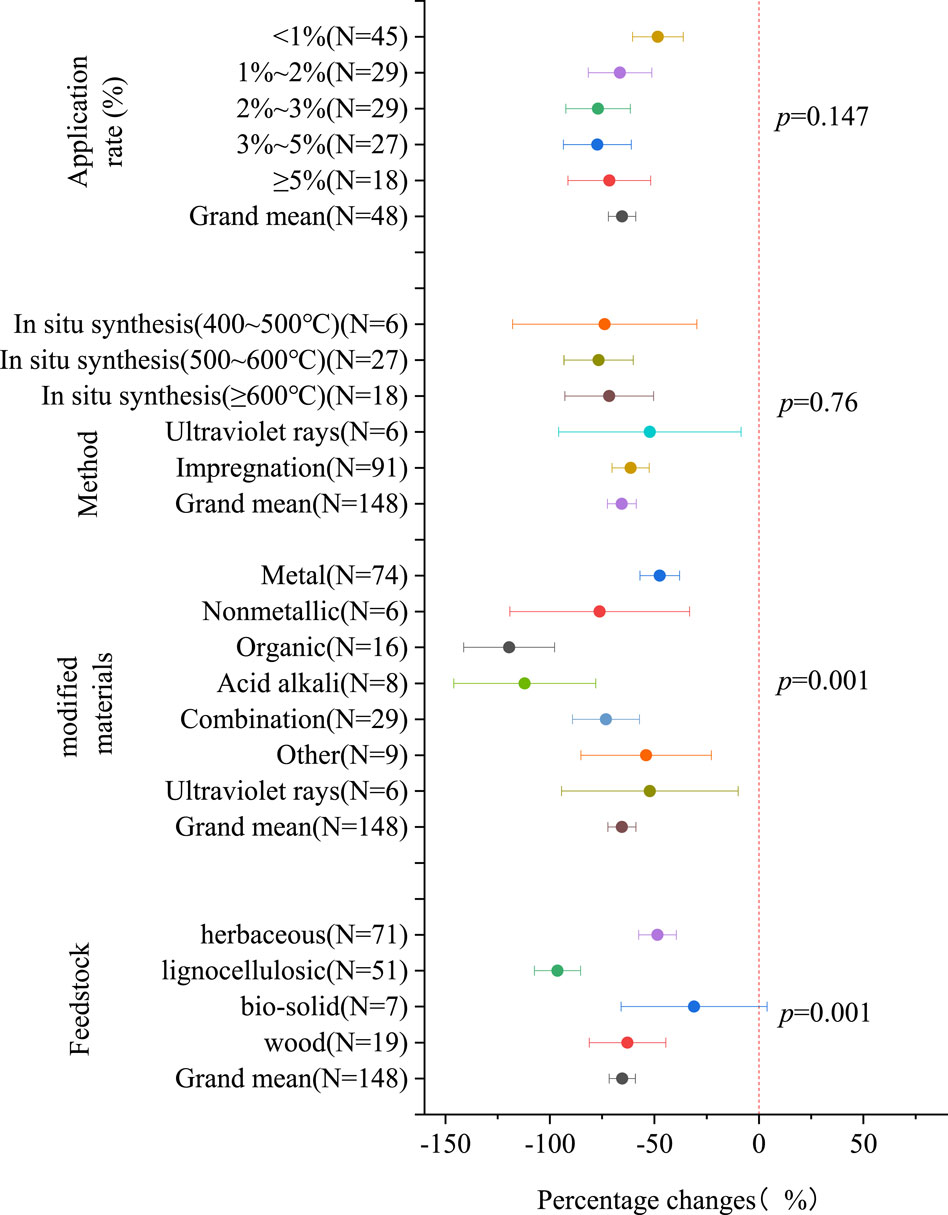
Figure 2. The percentage change in the availability of Cd with the characteristics of modified biochar (compared to raw biochar).
To visually compare the effectiveness of modified biochar and raw biochar in reducing soil effective Cd, we used raw biochar as the control (CK) and represented the results on a forest map (Supplementary Figure S2). Among the subgroup of feedstock, modified biochar derived from lignocellulosic material exhibited the most significant reduction in soil Cd availability (76.17% [57.51%, 94.83%]), followed by wood (41.30% [16.87%, 41.30%]). Among modified materials, the organic modification resulted in the most notable decrease in soil effective Cd (93.72% [71.53%, 115.91%]), followed by nonmetallic modification (61.78% [17.94%, 105.61%]) and then combination modification (42.77% [18.02%, 67.52%]). In situ synthesis has been found to effectively minimize the Cd availability in the soil when the pyrolysis temperature ranges between 500 °C and 600 °C. However, the reduction in soil Cd availability was 56.51% [22.13%, 90.89%] when modified biochar, with a pyrolysis temperature greater than 600 °C in situ synthesis, was applied compared to the raw biochar. Moreover, the application of modified biochar prepared using impregnation method resulted in a 29.44% [18.43%, 40.45%] in reducing soil Cd availability compared to the raw biochar. In the application rate subgroup, the ≥5% application rate had the most significant effect on reducing soil Cd availability (47.41% [17.66%, 77.16%]). Furthermore, even when the application rate is less than 1%, the effect of reducing soil Cd availability after applying modified biochar is superior to that of applying raw biochar.
In Figure 3, the Cd content in plants displays variations corresponding to different characteristics of modified biochar. Among the subgroup of raw materials, modified biochar derived from herbs exhibited the most significant reduction in Cd content in plants (100.53% [92.26%, 108.81%]). In the subgroup of modified materials, UV modification (165.06% [142.66%, 187.45%]) demonstrated the most substantial decrease in Cd content in plants, followed by combination modification (88.87% [74.27%, 103.47%]). Conversely, the impregnation method of modified biochar (74.34% [66.31%, 82.37%]) yields slightly better results in reducing Cd content in plants compared to the in situ synthesis method. The optimal effect on reducing Cd content in plants is achieved when the application rate is between 2% and 3%, reaching 88.88% [58.67%, 119.09%]. To visually compare the effects of modified biochar and raw biochar on reducing Cd content in plants, we employed raw biochar as a control (CK) and presented the results on a forest map (Supplementary Figure S3). Supplementary Figure S3 reveals that some modified biochar may not effectively reduce the Cd content in plants. For instance, in the subgroup of raw materials, the use of wood-modified biochar may even increase the Cd content in plants (17.95% [-53.94%, 89.83%]). Moreover, within the modified materials subgroup, both acid‒base modification (−29.27% [-121.15%, 62.60%]), metal modification (−22.32% [-46.39%, 1.74%]), and nonmetallic modification (−49.83% [-142.00%, 42.34%]) may result in an increase in Cd content in plants. Additionally, compared to the raw biochar, the modified biochar prepared through the impregnation method demonstrated a 32.48% improvement in reducing Cd content in plants [13.09%, 51.88%]. Among the application rate subgroups, an application rate of 1%–2% exhibited the best effect on reducing Cd content in plants (49.15% [0.59%, 97.71%]).
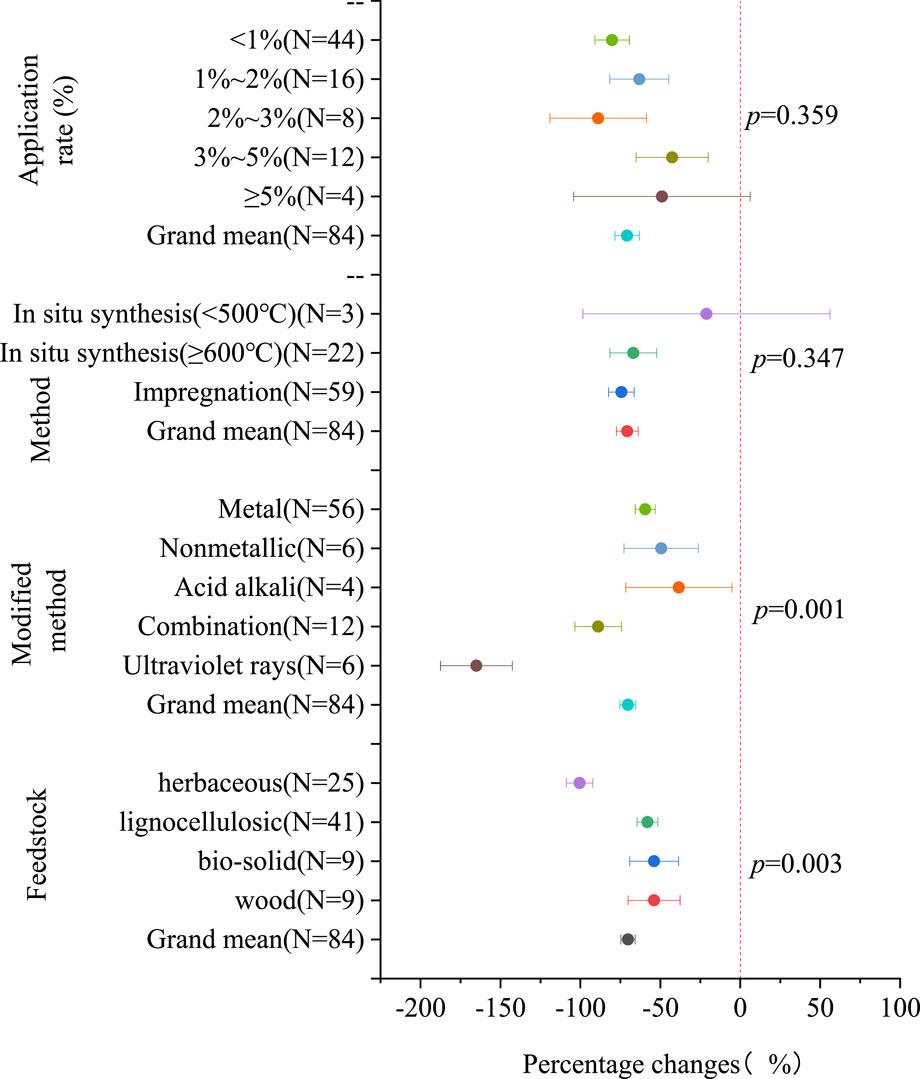
Figure 3. The percentage change in Cd content in plants with the characteristics of modified biochar (compared to raw biochar).
Changes in the efficiency of modified biochar with soil characteristics
Figure 4 demonstrates that modified biochar effectively reduces the bioavailability of Cd in soils, regardless of soil properties. The highest improvement in effectiveness was observed in the subgroup with pH ≥ 7.5, showing a 30.39% enhancement compared to other pH groups. Among the subgroups with SOM concentrations ranging from 0 to 10 mg/kg, the inhibitory effect of modified biochar on Cd bioavailability showed the highest improvement of 44.03% [25.15%, 62.92%]. The impact of various types of SOM on the performance of modified biochar is ranked as follows: 0–10 mg/kg (108.09% [93.37%, 122.81%]) > > 30 mg/kg (83.6% [33.33%, 133.87%]) > 10–20 mg/kg (44.85% [31.97%, 57.73%]) > 20–30 mg/kg (43.28% [-14.8%, 101.36%]). The next subgroup to exhibit significant improvement was CEC. Similarly, in soils with CEC ranging from 10 to 20 mmol/kg, the inhibitory effect of modified biochar on Cd bioavailability demonstrated the greatest improvement, followed by soils with CEC ≥30 mmol/kg. The impact of various types of CEC on the performance of modified biochar is ranked as follows: 10–20 mmol/kg (89.11% [72.71%, 105.51%]) > 20–30 mmol/kg (67.42% [48.27%, 86.57%]) > 0–10 mmol/kg (66.86% [50.82%, 82.90%]) > ≥30 mmol/kg (44.6% [16.68%, 72.52%]).
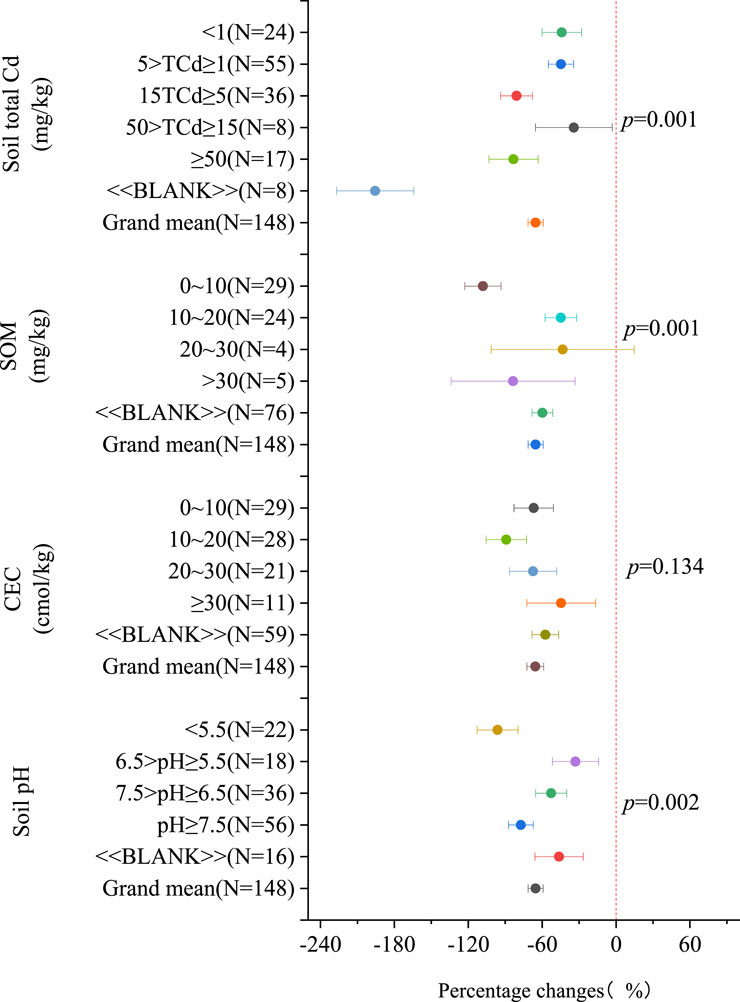
Figure 4. The percentage change in Cd availability with soil characteristics (compared to raw biochar). Note:The “BLANK” in the figure indicates the absence of corresponding indicators, such as CEC data, for this part of the data.
Figure 5 shows that modified biochar can effectively reduce the accumulation of Cd in plants regardless of soil properties. Among the subgroups, the highest efficacy improvement was observed in the pH ≥ 7.5 subgroup, with a decrease of 98.67% [91.89%, 105.45%] compared to other pH groups. The greatest reduction in Cd and Cd content in plants using modified biochar was observed in the subgroup with SOM concentrations ranging from 0 to 10 mg/kg, reaching 165.17% [142.13%, 188.20%]. The next significant improvement was noticed in soils with CEC ranging from 20 to 30 mmol/kg, where modified biochar showed the greatest reduction in the content of Cd in plants. However, it is important to note that the application of modified biochar does not necessarily result in a decrease in Cd content in plants when compared to the application of raw biochar (Supplementary Figure S4). For instance, an increase in Cd content in plants was observed in subgroups with 6.5>pH ≥ 5.5 (effect size = − 3.22%; 95% CI: −36.68%–32.23%).
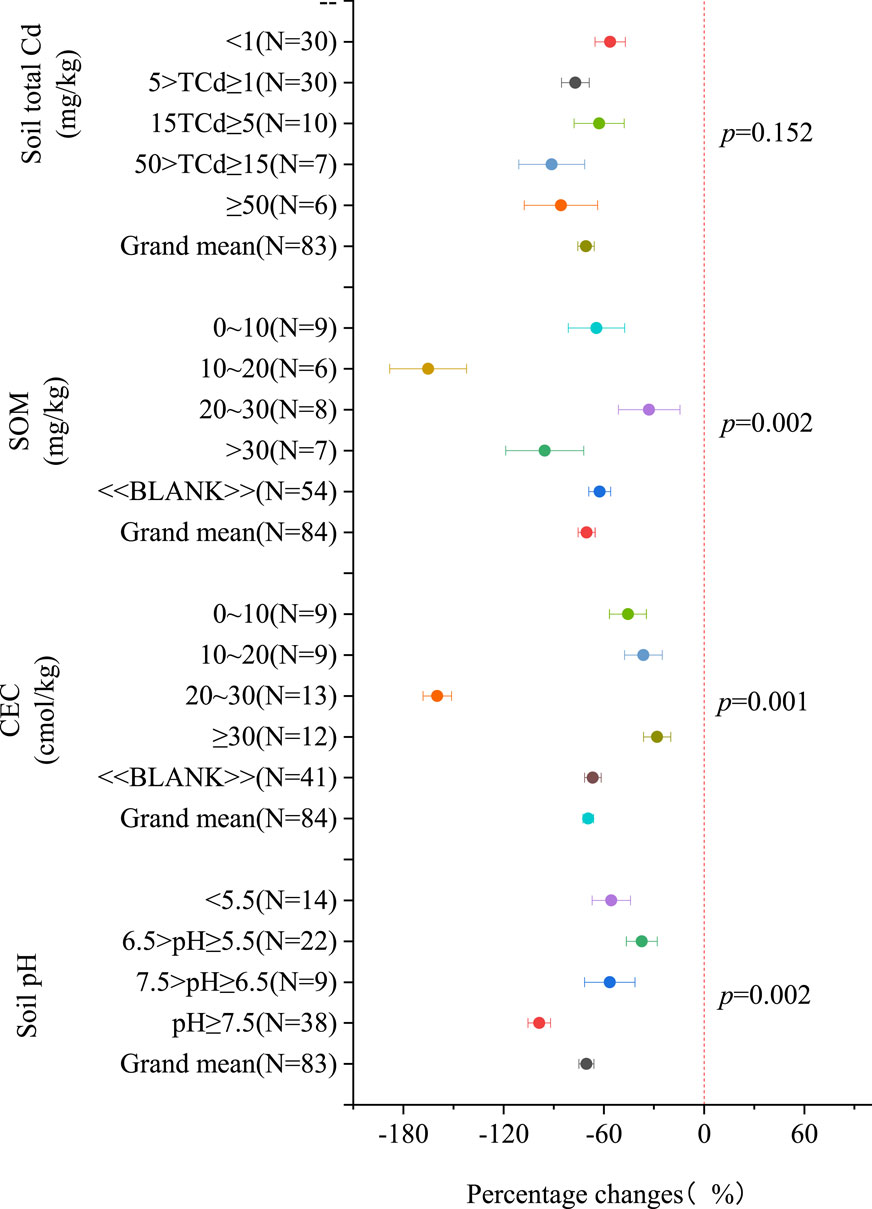
Figure 5. The percentage change of Cd content in plants with soil characteristics (compared to no biochar application), Note:The “BLANK” in the figure indicates the absence of corresponding indicators, such as CEC data, for this part of the data.
Discussion
Modified biochar exhibited a discrepant effect on the availability of Cd
Numerous studies (Fan et al., 2020; Tan et al., 2020; Gholami and Rahimi, 2021; Zhong et al., 2023) have shown that soil cadmium bioavailability is significantly reduced through biochar modification. This is achieved by combining biochar with metal, acids, bases, and organic compounds, which greatly alter the surface function of biochar and enhance its efficiency in reducing soil Cd availability (Wang et al., 2020; Zhu et al., 2020; Wang et al., 2021a; Wang et al., 2021b; Maharlouei et al., 2021; Wen et al., 2021). For example, Wang et al. (2021b) found that biochar modification increased the specific surface area and surface functional groups of biochar. They observed that the application of 3% modified biochar led to an 85.71% reduction in effective Cd in soil, compared to the application of raw biochar. Yang et al. (2021) demonstrated that the effective Cd content in both acidic and alkaline soils was reduced by 57.45% and 52.50%, respectively, when 3% modified biochar was used compared to 3% raw biochar.
There are significant differences in the effectiveness of modified biochar from different raw materials in reducing soil Cd availability and plant Cd content. The specific surface area of biochar is generally an important factor affecting its adsorption effect on Cd. A larger specific surface area results in a stronger adsorption capacity. Among the biochar modifications, lignocellulosic has the best effect on effective Cd in the soil, and herbaceous have the best effect on plant Cd content. Therefore, the larger specific surface area of modified biochar from herbaceous and lignocellulosic raw materials compared to other biochar modifications explains their superior effectiveness in reducing soil Cd availability and plant Cd content. For instance, under the same modification conditions, the specific surface area of modified durian shell (lignin) biochar is greater than that of modified branch (wood) biochar. Consequently, the effect of modified durian shell biochar on reducing soil Cd availability is superior to that of modified branch biochar (Yang et al., 2022).
The effectiveness of modified biochar, including organic modified biochar, in reducing soil Cd availability and plant Cd content is well-documented (Figures 2, 3). Organic modified biochar, in particular, stands out for its remarkable efficiency in this regard, owing to the abundance of functional groups it possesses, namely, carboxyl, thiol, amino, and hydroxyl groups (Fan et al., 2020; Zhu et al., 2020). These functional groups significantly expand the adsorption capacity of organic modified biochar by offering additional sites for metal ion binding and enhancing surface area through the presence of various surface functional groups such as -OH, -NH, and C-O. Several mechanisms, including cation exchange, electrostatic interaction, surface complexation, and precipitation, are instrumental in facilitating the adsorption of Cd by organic modified biochar (Tan et al., 2022). The incorporation of chitosan into biochar further enhances its functional groups and boosts its acid-buffering capacity, a fact substantiated by Fourier transform infrared spectroscopy and Boehm titration analyses (He et al., 2022). Moreover, studies by Zhu et al. (2020) have revealed that thiourea modified tree biochar has the ability to elevate soil pH levels, thereby enhancing the soil’s Cd2+ adsorption capacity; this is due to Cd2+ reacting with OH− at higher pH to form hydrogen oxides and precipitates. Various modifications such as acid-base modification, non-metallic loading, and combined modification significantly contribute to lowering soil Cd availability (Figure 2). However, when it comes to reducing Cd content in plants, the combination modification and metal loading methods prove most effective, with the exception of ultraviolet modification (Figure 3). In particular, the combination modification and metal loading, mainly involving Fe, create an iron film on plant surfaces which effectively blocks the entry of Cd from the soil into the plants, as demonstrated by Irshad et al. (2020). For instance, goethite modified biochar aids in transforming exchangeable Cd components into non-exchangeable Cd forms, thereby restricting the movement and uptake of Cd in the soil (Irshad et al., 2020). Moreover, goethite modified biochar fosters the formation of iron spots on plant surfaces, facilitating Cd absorption by these spots (Irshad et al., 2020).
The study primarily involves two methods of modification: in situ synthesis and impregnation. In the in-situ synthesis method, the target modified reagent is directly added to the raw material, followed by pyrolysis, chemical precipitation, and activation to obtain modified biochar. Conversely, the impregnation method involves impregnating biochar into a solution containing a modifier, thus loading commercial or self-made biochar with target elements (Liang et al., 2021). When comparing both methods, it is evident that modified biochar synthesized through in-situ synthesis demonstrates greater effectiveness in reducing Cd compared to the impregnation method (Figure 2). Particularly, the in-situ synthesis method showed the best effect in reducing the Cd availability content in soil at pyrolysis temperatures of 500°C–600°C, followed by 400°C–500°C. On the other hand, for the impregnation method, the optimized pyrolysis temperature range is 400°C–450°C. However, both methods encounter certain challenges. Lower pyrolysis temperatures can result in incomplete carbonization of biochar, whereas higher temperatures may lead to a reduction in acidic functional groups on the biochar surface, a decrease in O and H content, and an increase in C content (Srinivasan and Sarmah, 2015; Muhammad et al., 2020).
The influence of the application amount of modified biochar on the reduction effect of soil Cd availability and plant Cd content is depicted in Figures 2, 3. Effective reduction of Cd in the soil is most apparent with application amounts ranging between 2%–3% and 3%–5% of modified biochar. Likewise, the optimal reduction of Cd content in plants is achieved with an application amount of 2%–3%. Nevertheless, a low application of biochar may lead to a significant amount of exchangeable Cd remaining unconverted to non-exchangeable Cd, resulting in reduced efficacy in lowering soil Cd availability. Conversely, excessive application of modified biochar can induce changes in soil properties that can have adverse effects on both plants and soil health (Wang et al., 2019).
Modified biochar affects the effectiveness of Cd by altering soil properties
The impact of biochar on the bioavailability and bioaccumulation of Cd in the soil-plant system is determined by soil properties such as pH and SOM, similar to the properties of biochar itself(Figures 4, 5). Figure 4 demonstrates that modified biochar has a significant effect on enhancing the availability of Cd in soil with an SOM ranging from 0 to 10 mg/kg, providing the best reduction effect on low SOM Cd availability. Furthermore, the best reduction effect on Cd content in plants is observed in soil with an SOM of 10–20 mg/kg when using modified biochar (Figure 5). It is worth noting that the application of biochar can increase the organic matter content in soil (Cui et al., 2020; Sun et al., 2020), leading to a reduction in Cd availability and plant content within a certain range due to the low organic matter content. However, the complex components (fulminic acid, humic acid and humin) in SOM were reported to have different or even opposite effects on Cd migration and transformation (Pan et al., 2022). Excessive organic matter content can increase Cd availability in the soil and plant content.
The application of modified biochar can significantly impact Cd availability in soil and Cd content in plants, as evidenced in Figures 4, 5. Modified biochar, with its alkaline properties, plays a crucial role in reducing Cd availability in acidic soil by increasing soil pH. Mannn et al. (2022) reported that raw biochar at 3% and 5% concentrations elevated soil pH from 4.45 to 4.79 and 4.93, respectively, while phosphorus-modified biochar increased the pH to a range of 5.11–5.33. Compared to raw biochar, phosphorus-modified biochar reduced the concentration of Cd in soil by 73.0%. Moreover, in alkaline soils with a pH above 7.5, modified biochar has the potential to further elevate pH levels. This elevated pH leads to the precipitation of Cd with anions such as OH− and CO32−, forming CdO, CdCO3, and Cd(OH)2 (Cai et al., 2021; Yang et al., 2021; Li et al., 2022), which reduces Cd availability. The rise in soil pH facilitates the transformation of Cd into carbonate and iron-manganese states through the formation of precipitate complexes, generating increased negative charges that limit the mobility of heavy metals (Chen et al., 2020). Additionally, in alkaline soils, modified biochar may possess various functional groups, including hydroxyl, amino, and carbonyl groups, that interact with available Cd forms. Previous research has shown that these functional groups have strong adsorption and fixation capabilities for Cd (Ng et al., 2022).
A previous meta-analysis (Supplementary Figures S3, S4) observed an increase in Cd content in plants due to modified biochar, particularly compared to raw biochar. The increase is likely due to a decrease in pH after biochar modification, especially when acid and metal modifications were applied. The application of low pH modified biochar can lower soil pH, leading to reduced Cd adsorption on the biochar surface (Wen et al., 2021), resulting in increased Cd desorption and solubility. In a study by Sui et al. (2021), it was found that iron modified biochar reduced Cd migration from buds to leaves but enhanced transport from leaves to grains. This was accompanied by an increase in Fe concentration in rice leaves, suggesting the formation of iron channels that facilitate Cd entry into plant cells, promoting Cd transfer from leaves to rice grains.
Conclusion
This study, based on 58 articles, reviews the effects of modified biochar on Cd effectiveness and bioaccumulation in the soil-plant system. Meta-analysis results show that modified biochar significantly reduces Cd effectiveness compared to raw biochar. The impact of modified biochar on reducing Cd effectiveness and plant Cd accumulation depends on biochar and soil properties such as raw materials, modification methods, and soil pH. Acid-base and organic modifications show ideal effects on reducing effective Cd in soil, while for plants, combination modification (excluding UV modification) is more effective for Cd reduction. Lignocellulose-based modified biochar is ideal for reducing effective Cd in soil, while herb-based modified biochar is effective for plant Cd reduction. The optimal application rate of modified biochar is 2%–3%. Furthermore, modified biochar reduces Cd availability in acidic and alkaline soils. In conclusion, this study provides comprehensive insights into the effects of biochar on soil Cd availability and plant Cd accumulation based on various biochar and soil properties. These results aid in selecting, modifying, and utilizing biochar for effective remediation of Cd-contaminated soil.
Author contributions
HL: Conceptualization, Data curation, Formal Analysis, Funding acquisition, Methodology, Writing–original draft, Writing–review & editing. Chuntan Chen: Writing–review and editing. XL: Data curation, Writing–review and editing. PY: Conceptualization, Supervision, Writing–review & editing.
Funding
The author(s) declare that financial support was received for the research, authorship, and/or publication of this article. This work was supported by High-Level Talent Research Launch Project at Sichuan University of Arts and Sciences (2023RC004Z) and Key Laboratory of Exploitation and Study of Distinctive Plants in Education Department of Sichuan Province (TSZW2011 and TSZW2103).
Conflict of interest
The authors declare that the research was conducted in the absence of any commercial or financial relationships that could be construed as a potential conflict of interest.
Publisher’s note
All claims expressed in this article are solely those of the authors and do not necessarily represent those of their affiliated organizations, or those of the publisher, the editors and the reviewers. Any product that may be evaluated in this article, or claim that may be made by its manufacturer, is not guaranteed or endorsed by the publisher.
Supplementary material
The Supplementary Material for this article can be found online at: https://www.frontiersin.org/articles/10.3389/fenvs.2024.1413047/full#supplementary-material
References
Borchard, N., Schirrmann, M., Luz Cayuela, M., Kammann, C., Wrage-Moennig, N., Estavillo, J. M., et al. (2019). biochar, soil and land-use interactions that reduce nitrate leaching and N2O emissions: a meta-analysis. Sci. Total Environ. 651, 2354–2364. doi:10.1016/j.scitotenv.2018.10.060
Cai, T., Liu, X., Zhang, J., Tie, B., Lei, M., Wei, X., et al. (2021). Silicate-modified oiltea camellia shell-derived biochar: a novel and cost-effective sorbent for cadmium removal. J. Clean. Prod. 281, 125390. doi:10.1016/j.jclepro.2020.125390
Chaney, R. L. (2015). How does contamination of rice soils with Cd and Zn cause high incidence of human Cd disease in subsistence rice farmers. Curr. Pollut. Rep. 1 (1), 13–22. doi:10.1007/s40726-015-0002-4
Chen, D., Wang, X., Wang, X., Feng, K., Su, J., and Dong, J. (2020). The mechanism of cadmium sorption by sulphur-modified wheat straw biochar and its application cadmium-contaminated soil. Sci. Total Environ. 714, 136550. doi:10.1016/j.scitotenv.2020.136550
Cheng, X., Cao, X., Tan, C., Liu, L., Bai, J., Liang, Y., et al. (2022). Effects of four endophytic bacteria on cadmium speciation and remediation efficiency of Sedum plumbizincicola in farmland soil. Environ. Sci. Pollut. Res. 29 (59), 89557–89569. doi:10.1007/s11356-022-21711-y
Cui, L., Li, L., Bian, R., Yan, J., Quan, G., Liu, Y., et al. (2020). Short- and long-term biochar cadmium and lead immobilization mechanisms. Environments 7 (7), 53. doi:10.3390/environments7070053
Da, Y., Xu, M., Ma, J., Gao, P., Zhang, X., Yang, G., et al. (2023). Remediation of cadmium contaminated soil using K2FeO4 modified vinasse biochar. Ecotoxicol. Environ. Saf. 262, 115171. doi:10.1016/j.ecoenv.2023.115171
Deng, X., Liu, R., and Hou, L. (2022). Promotion effect of graphene on phytoremediation of Cd-contaminated soil. Environ. Sci. Pollut. Res. 29 (49), 74319–74334. doi:10.1007/s11356-022-20765-2
Fan, J., Cai, C., Chi, H., Reid, B. J., Coulon, F., Zhang, Y., et al. (2020). Remediation of cadmium and lead polluted soil using thiol-modified biochar. J. Hazard. Mater. 388, 122037. doi:10.1016/j.jhazmat.2020.122037
Gholami, L., and Rahimi, G. (2021). Chemical fractionation of copper and zinc after addition of carrot pulp biochar and thiourea-modified biochar to a contaminated soil. Environ. Technol. 42 (22), 3523–3532. doi:10.1080/09593330.2020.1733101
Godt, J., Scheidig, F., Grosse-Siestrup, C., Esche, V., Brandenburg, P., Reich, A., et al. (2006). The toxicity of cadmium and resulting hazards for human health. J. Occup. Med. Toxicol. 1, 22. doi:10.1186/1745-6673-1-22
Guo, X., Gao, Y., Han, W., Zhao, G., Zhang, G., and Liu, D. (2023). Effect of multiple washing operations on the removal of potentially toxic metals from an alkaline farmland soil and the strategy for agricultural reuse. Environ. Sci. Pollut. Res. 30 (1), 739–751. doi:10.1007/s11356-022-22126-5
He, X., Nkoh, J. N., Shi, R.-y., and Xu, R.-k. (2022). Application of chitosan- and alginate-modified biochars in promoting the resistance to paddy soil acidification and immobilization of soil cadmium. Environ. Pollut. 313, 120175. doi:10.1016/j.envpol.2022.120175
Hedges, L. V., Gurevitch, J., and Curtis, P. S. (1999). The meta-analysis of response ratios in experimental ecology. Ecology 80 (4), 1150–1156. doi:10.2307/177062
Hussain, B., Ashraf, M. N., Shafeeq ur, R., Abbas, A., Li, J., and Farooq, M. (2021). Cadmium stress in paddy fi elds: effects of soil conditions and remediation strategies. Sci. Total Environ. 754, 142188. doi:10.1016/j.scitotenv.2020.142188
Irshad, M. K., Chen, C., Noman, A., Ibrahim, M., Adeel, M., and Shang, J. (2020). Goethite-modified biochar restricts the mobility and transfer of cadmium in soil-rice system. Chemosphere 242, 125152. doi:10.1016/j.chemosphere.2019.125152
Li, Y., Su, J., Ali, A., Hao, Z., Li, M., Yang, W., et al. (2022). Simultaneous removal of nitrate and heavy metals in a biofilm reactor filled with modified biochar. Sci. Total Environ. 851, 158175. doi:10.1016/j.scitotenv.2022.158175
Liang, M., Lu, L., He, H., Li, J., Zhu, Z., and Zhu, Y. (2021). Applications of biochar and modified biochar in heavy metal contaminated soil: a descriptive review. Sustainability 13 (24), 14041. doi:10.3390/su132414041
Liu, B., Wu, C., Pan, P., Fu, Y., He, Z., Wu, L., et al. (2019). Remediation effectiveness of vermicompost for a potentially toxic metal-contaminated tropical acidic soil in China. Ecotoxicol. Environ. Saf. 182, 109394. doi:10.1016/j.ecoenv.2019.109394
Maharlouei, Z. D., Fekri, M., Saljooqi, A., Mahmoodabadi, M., and Hejazi, M. (2021). Effect of modified biochar on the availability of some heavy metals speciation and investigation of contaminated calcareous soil. Environ. Earth Sci. 80 (3), 119. doi:10.1007/s12665-021-09418-8
Muhammad, N., Mohammad, N., Khan, M. H., Ge, L., and Lisak, G. (2020). Effect of biochars on bioaccumulation and human health risks of potentially toxic elements in wheat (Triticum aestivum L.) cultivated on industrially contaminated soil. Environ. Poll. 260, 113887. doi:10.1016/j.envpol.2019.113887
Ng, C. W. W., Wang, Y. C., Ni, J. J., and So, P. S. (2022). Effects of phosphorus-modified biochar as a soil amendment on the growth and quality of Pseudostellaria heterophylla. Sci. Rep. 12 (1), 7268. doi:10.1038/s41598-022-11170-3
Pan, S.-F., Ji, X.-H., Xie, Y.-H., Liu, S.-H., Tian, F.-X., and Liu, X.-L. (2022). Influence of soil properties on cadmium accumulation in vegetables: thresholds, prediction and pathway models based on big data. Environ. Pollut. (Barking, Essex 1987) 304, 119225. doi:10.1016/j.envpol.2022.119225
Sha, Z., Li, Q., Lv, T., Misselbrook, T., and Liu, X. (2019). Response of ammonia volatilization to biochar addition: a meta-analysis. Sci. Total Environ. 655, 1387–1396. doi:10.1016/j.scitotenv.2018.11.316
Shan, R., Li, W., Chen, Y., and Sun, X. (2020). Effects of Mg-modified biochar on the bioavailability of cadmium in soil. Bioresources 15 (4), 8008–8025. doi:10.15376/biores.15.4.8008-8025
Srinivasan, P., and Sarmah, A. K. (2015). Characterisation of agricultural waste-derived biochars and their sorption potential for sulfamethoxazole in pasture soil: a spectroscopic investigation. Sci. Total Environ. 502, 471–480. doi:10.1016/j.scitotenv.2014.09.048
Stohs, S. J., Bagchi, D., Hassoun, E., and Bagchi, M. (2001). Oxidative mechanisms in the toxicity of chromium and cadmium ions. J. Environ. pathology, Toxicol. Oncol. 20 (2), 12–88. doi:10.1615/jenvironpatholtoxicoloncol.v20.i2.10
Sui, F., Kang, Y., Wu, H., Li, H., Wang, J., Joseph, S., et al. (2021). Effects of iron-modified biochar with S-rich and Si-rich feedstocks on Cd immobilization in the soil-rice system. Ecotoxicol. Environ. Saf. 225, 112764. doi:10.1016/j.ecoenv.2021.112764
Sun, J., Cui, L., Quan, G., Yan, J., Wang, H., and Wu, L. (2020). Effects of biochar on heavy metals migration and fractions changes with different soil types in column experiments. Bioresources 15 (2), 4388–4406. doi:10.15376/biores.15.2.4388-4406
Tan, X., Wei, W., Xu, C., Meng, Y., Bai, W., Yang, W., et al. (2020). Manganese-modified biochar for highly efficient sorption of cadmium. Environ. Sci. Pollut. Res. 27 (9), 9126–9134. doi:10.1007/s11356-019-07059-w
Tan, Y., Wan, X., Ni, X., Wang, L., Zhou, T., Sun, H., et al. (2022). Efficient removal of Cd (II) from aqueous solution by chitosan modified kiwi branch biochar. Chemosphere 289, 133251. doi:10.1016/j.chemosphere.2021.133251
Tian, X., Wang, D., Chai, G., Zhang, J., and Zhao, X. (2021). Does biochar inhibit the bioavailability and bioaccumulation of As and Cd in co-contaminated soils? A meta-analysis. Sci. Total Environ. 762, 143117. doi:10.1016/j.scitotenv.2020.143117
Turan, V., Khan, S. A., Mahmood ur, R., Iqbal, M., Ramzani, P. M. A., and Fatima, M. (2018). Promoting the productivity and quality of brinjal aligned with heavy metals immobilization in a wastewater irrigated heavy metal polluted soil with biochar and chitosan. Ecotoxicol. Environ. Saf. 161, 409–419. doi:10.1016/j.ecoenv.2018.05.082
Wang, J., Odinga, E. S., Zhang, W., Zhou, X., Yang, B., Waigi, M. G., et al. (2019). Polyaromatic hydrocarbons in biochars and human health risks of food crops grown in biochar-amended soils: a synthesis study. Environ. Int. 130, 104899. doi:10.1016/j.envint.2019.06.009
Wang, Y., Ren, Q., Li, T., Zhan, W., Zheng, K., Liu, Y., et al. (2021a). Influences of modified biochar on metal bioavailability, metal uptake by wheat seedlings (Triticum aestivum L.) and the soil bacterial community. Ecotoxicol. Environ. Saf. 220, 112370. doi:10.1016/j.ecoenv.2021.112370
Wang, Y., Zheng, K., Zhan, W., Huang, L., Liu, Y., Li, T., et al. (2021b). Highly effective stabilization of Cd and Cu in two different soils and improvement of soil properties by multiple-modified biochar. Ecotoxicol. Environ. Saf. 207, 111294. doi:10.1016/j.ecoenv.2020.111294
Wang, Y.-M., Wang, S.-W., Wang, C.-Q., Zhang, Z.-Y., Zhang, J.-Q., Meng, M., et al. (2020). Simultaneous immobilization of soil Cd(II) and as(V) by Fe-modified biochar. Int. J. Environ. Res. Public Health 17 (3), 827. doi:10.3390/ijerph17030827
Wen, E., Yang, X., Chen, H., Shaheen, S. M., Sarkar, B., Xu, S., et al. (2021). Iron-modified biochar and water management regime-induced changes in plant growth, enzyme activities, and phytoavailability of arsenic, cadmium and lead in a paddy soil. J. Hazard. Mater. 407, 124344. doi:10.1016/j.jhazmat.2020.124344
Xia, Y., Liu, H., Guo, Y., Liu, Z., and Jiao, W. (2019). Immobilization of heavy metals in contaminated soils by modified hydrochar: efficiency, risk assessment and potential mechanisms. Sci. Total Environ. 685, 1201–1208. doi:10.1016/j.scitotenv.2019.06.288
Xiao, W., Ye, X., Zhang, Q., Chen, D., Hu, J., and Gao, N. (2018). Evaluation of cadmium transfer from soil to leafy vegetables: influencing factors, transfer models, and indication of soil threshold contents. Ecotoxicol. Environ. Saf. 164, 355–362. doi:10.1016/j.ecoenv.2018.08.041
Xu, L., Dai, H., Skuza, L., Xu, J., Shi, J., and Wei, S. (2022). Co-high-efficiency washing agents for simultaneous removal of Cd, Pb and as from smelting soil with risk assessment. Chemosphere 300, 134581. doi:10.1016/j.chemosphere.2022.134581
Yang, T., Xu, Y., Huang, Q., Sun, Y., Liang, X., and Wang, L. (2022). Removal mechanisms of Cd from water and soil using Fe-Mn oxides modified biochar. Environ. Res. 212, 113406. doi:10.1016/j.envres.2022.113406
Yang, T., Xu, Y., Huang, Q., Sun, Y., Liang, X., Wang, L., et al. (2021). An efficient biochar synthesized by iron-zinc modified corn straw for simultaneously immobilization Cd in acidic and alkaline soils. Environ. Pollut. 291, 118129. doi:10.1016/j.envpol.2021.118129
Yin, X., Wang, Y., Wang, L. e., Huang, H., Zhou, C., and Ni, G. (2022). Reduced cadmium(Cd) accumulation in lettuce plants by applying KMnO4 modified water hyacinth biochar. Heliyon 8 (11), e11304. doi:10.1016/j.heliyon.2022.e11304
Yuan, C., Gao, B., Peng, Y., Gao, X., Fan, B., and Chen, Q. (2021). A meta-analysis of heavy metal bioavailability response to biochar aging: importance of soil and biochar properties. Sci. Total Environ. 756, 144058. doi:10.1016/j.scitotenv.2020.144058
Zhong, M., Li, W., Jiang, M., Wang, J., Shi, X., Song, J., et al. (2023). Improving the ability of straw biochar to remediate Cd contaminated soil: KOH enhanced the modification of K3PO4 and urea on biochar. Ecotoxicol. Environ. Saf. 262, 115317. doi:10.1016/j.ecoenv.2023.115317
Keywords: modified biochar, Cd, Cd availability, plant, meta-analysis
Citation: Liu H, Chen C, Li X and Yang P (2024) Meta-analysis compares the effectiveness of modified biochar on cadmium availability. Front. Environ. Sci. 12:1413047. doi: 10.3389/fenvs.2024.1413047
Received: 06 April 2024; Accepted: 21 May 2024;
Published: 07 June 2024.
Edited by:
Rubén Forján, University of Oviedo, SpainReviewed by:
Tian Xiaosong, Chongqing Vocational Institute of Engineering, ChinaCopyright © 2024 Liu, Chen, Li and Yang. This is an open-access article distributed under the terms of the Creative Commons Attribution License (CC BY). The use, distribution or reproduction in other forums is permitted, provided the original author(s) and the copyright owner(s) are credited and that the original publication in this journal is cited, in accordance with accepted academic practice. No use, distribution or reproduction is permitted which does not comply with these terms.
*Correspondence: Hanyi Liu, liuhy4214@sasu.edu.cn