- 1Technical Institute of Bakrajo, Sulaimani Polytechnic University, Sulaymaniyah, Iraq
- 2Institute of Biology, Biotechnology and Environmental Protection, Faculty of Natural Sciences, University of Silesia in Katowice, Katowice, Poland
- 3Institute of Environmental Protection and Engineering, Faculty of Materials, Civil and Environmental Engineering, University of Bielsko-Biala, Bielsko-Biała, Poland
- 4Department of Ecology, Institute of Dendrology, Polish Academy of Sciences, Kórnik, Poland
- 5Department of Game Management and Forest Protection, Faculty of Forestry and Wood Technology, Poznań University of Life Sciences, Poznań, Poland
Coal is the most abundant fossil fuel in Europe, but the excavation of hard coal has covered large areas with disposed rock waste, and turned the natural habitats into disturbed novel ecosystems with harsh conditions differ in time and space. To examine the spontaneous complex successional gradient, we studied a large number of post coalmine heaps in Upper Silesia, which differ in vegetation type and age. Cluster analysis based on plant community composition (367 species in total) separated all surveyed plots on coal mining spoil heaps with herbaceous vegetation from Late Stage (LS) forests aged 14–56 years. Furthermore, the herbaceous vegetation was sub-grouped to three stages: Initial Stage (IS) aged 2–5 years, Early Stage (ES) aged 3–8 years and Mid-Stage (MS) aged 5–12 years. MS vegetation was characterised by the highest species richness and diversity (47 and 2.79) compared to ES (30 and 2.18) and IS (9 and 1.6), but higher species number and a similar diversity index occurred in LS (37 and 2.81). Functional diversity (FD) and community weighted mean (CWM) of nine functional traits showed higher (23.1) functional richness, higher (0.72) functional divergence, higher (4.5) functional dispersion, and higher value (24.4) of Rao’s quadratic entropy in LS compared to those calculated from the first three stages. Species at the initial successional stage (IS) were characterised by lower canopy height, seed mass, higher lateral spread, and specific leaf area (SLA). Additionally, the lowest (0.22 mg CO2 per hour per square metre) soil respiration (Sr) rate was recorded from IS compared to (0.53, 0.82 and 1.00) from ES, LS and MS, respectively. The soil water content (SWC) was the most important factor affecting the soil respiration, while the soil temperature (St) did not follow the well-studied relationship between soil respiration and soil temperature. Our spatial and temporal analyses illustrated changes in plant community assembly processes in the course of spontaneous vegetation succession on post coalmine spoil heaps. The importance of trait mediated abiotic filtration in community assembly in initial-, early-, and mid-stages of succession with an increase in competitive exclusion at the late successional stage was emphasized.
1 Introduction
Succession as an ecological process of changes in the plant community over time following natural or anthropogenic disturbance, has been one of the main areas of scientific interest for a long time (Meiners et al., 2015; Prach and Walker, 2020). In most studies researchers underlined that succession is directed by three main factors: niche availability, species availability to colonise a newly established site and species performance, enabling them to interact with other species or to respond to environmental factors and abiotic stresses (Booth et al., 2003). Theoretical studies suggest that environmental filtering is the crucial assembly rule driving species composition in the early stages of development. It depends on the species pool (geographical, regional) that occurs in the surrounding areas and dispersal modes that enable species to reach a given site. In further stages other mechanisms such as competition between species, facilitation, tolerance and inhibition might become the most important factors (Purschke et al., 2013; Batalha et al., 2015; Chang and HilleRisLambers, 2019).
Newly established coalmine spoil heaps are successfully and quickly colonised by plant species and other organisms (Bierza et al., 2023). The harsh abiotic and biotic conditions force the colonising organisms to assemble according to new rules during the spontaneous succession from pioneer herbaceous vegetation communities to newly developed woodlands. As a result, non-analogous plant species assemblages are formed that differ from natural and semi-natural habitats (Hobbs et al., 2006; Keith et al., 2009). Some research investigated the dynamic changes in floristic composition of patches over time, as well as species selection by functional traits during succession or along environmental gradients (Prach and Bohemia, 1987; Schleicher et al., 2011; Bierza et al., 2023).
The most frequent aspect of studying plant diversity is the taxonomic approach, although species richness as a commonly used diversity measure is unable to relate particular ecosystem processes to each species and determine their contributions to ecosystem functioning and productivity (Lavorel et al., 2007). The formula used for species richness calculation assumes that all species are equivalent and the functional differences among species are not accounted for. Functional diversity is the crucial determinant of ecosystem functioning (Tilman et al., 1996; Grime, 1998; Díaz and Cabido, 2001; Dequéant et al., 2006), with a few highly competitive, dominant species having a more significant influence on ecosystem processes (Grime, 1998; Kirby and Potvin, 2007; Zhang et al., 2012; Isbell et al., 2015; Schuldt et al., 2015). A decrease of functional diversity due to environmental filtering has been suggested (Mouchet et al., 2010). In plant ecology, changes in the vegetation along gradients of habitat stressors are the most studied patterns (Peltzer et al., 2010). Functional diversity indicators can be used to reveal the processes of vegetation community assembly (Botta-Dukát, 2005; Mason et al., 2008). Recommended indicators include functional richness (FRic) to remove any trivial effects of species richness, and two mathematically very close indices (RoaQ and FDis) to measure the functional divergence (Botta-Dukát, 2005; Mason and Pavoine, 2013).
The substrate of coalmine spoil heaps is rock waste mineral material, inert with no buffering capacity, lacking organic matter and seed bank; it has low capacity to hold water and high thermal conductivity (Kompała-Bąba et al., 2020; 2021). Despite all the abiotic and biotic constraints and difficulties, species diaspores enter the area, germinate, and species start to grow on the de novo ecosystem mosaics (Bradshaw, 2000; Prach et al., 2013). The spontaneous succession process offers an opportunity for novel flora and fauna to re-colonise the new disturbed sites (Hobbs et al., 2006; Kowarik, 2011).
In this work, we aimed to show different patterns of initial, early, mid and late vegetation successional stages by characterizing the taxonomic and functional diversity. Additionally, to inspect the hypothesis predicting increasing of functional diversity along with taxonomic diversity during the spontaneous successional stages. Finally, we tested the response of soil respiration to abiotic factors and changes in plant diversity.
2 Material and methods
The studied coalmine heaps are scattered in the urban-industry landscape, providing poor mineral environmental islands. Plot locations on the coalmine spoil heaps were selected randomly and in advance by a computer algorithm, followed by field visits to exclude plots reclaimed and/or affected by human activities to guarantee that all plots exhibit natural spontaneous vegetation succession. The mineral materials gathered on heaps as the by-product of black hard coal excavation are different in terms of time of deposition, and how a given heap was built. In this way, the coalmine heaps that have been established for decades provided the opportunity to investigate a large-scale experiment and created habitats that differed in plant community composition (Anibaba et al., 2023).
2.1 Description of the study site
Upper Silesia is an industrial region in Poland and one of the most affected by human activity, specifically the hard coal mining industry. Hard coal mining has impacted the local ecosystem and directly affected more than 20 square kilometres of urban land (Szczepańska, 1987). Large amounts of rock composed of Carboniferous sediments on Precambrian crystalline rocks, mudstones and sandstones, and small coal particles left are brought to the surface that differ drastically from the soil covered, creating hundreds of spoil heaps piled up on the ground (Cabala et al., 2004).
All the 32 coalmine sites considered in this work are located in the south-western region of Upper Silesia in Poland. The region has a climate that is favourable for forest vegetation, transitory between oceanic and continental, with annual rainfall of 600–700 mm and a mean annual temperature of 8 °C (Kosmala, 2013). To ensure the spontaneity of the succession process, reclaimed sites were excluded and only seres started on bare ground were considered. The origin, nature, properties, and environmental stresses caused by the disposed rock waste were previously described in detail (Rahmonov et al., 2020).
To avoid the subjectivity of preferential sampling, a computer algorithm was used for the random sampling and determining the vegetation type depending on light reflectance and photo imaging, followed by a hierarchical cluster analysis using a set of dissimilarity values of the plant communities based on species composition (Zelený, 2022). Both methods distinguished four successional stages with some mismatches that were corrected by a field visit, resulting in 94 plots, including: Initial Stage (IS) 17 plots aged 2–5 years, Early Stage (ES) 28 plots aged 3–8 years, Mid-Stage (MS) 25 plots aged 5–12 years, and Late Stage (LS) 24 plots of forests aged 14–56 years. In total 94 plots were investigated. The different number of plots among different successional stages is due to the site availability.
2.2 The vegetation sampling
The plant cover representing a spectrum of different vegetation and successional stages was examined in June-September 2021. Each plot was 140 m2 in area, and consisted of five subplots (radius 3 m, area 28 m2) were established in a cross-design pattern, with a central subplot and four subplots at distances of 50 m in north, east, south and west directions (470 subplots in total; Figure 1). In each of the sampled subplots, all the vascular plant species with their abundances (visually estimated as cover of all individuals of recorded species) were recorded along with their percentage cover class (1%, 2%, 5%, 10% and every 10%–100%) (Kompała-Bąba et al., 2021; Woźniak et al., 2021). Scientific names of species were from (Mirek et al., 2020) checklist. The occurrence and abundance of species were recorded in all spatial layers (tree, shrub, and herbaceous) distinguished in vegetation patches.
2.3 Substrate parameters
Substrate samples for the physicochemical analyses were collected from the organic horizons (the top 20 cm) representing the densest rooting layer for all cross-designs in fivefold repetition. Samples were air-dried in the soil laboratory to a constant weight at room temperature. A random 120–150 g (substrate up to 500 g) of dry soil sample was collected and ground in a mortar. Then the sample was sieved through a 2 mm mesh sieve to separate the skeletal from non-skeletal parts and weighed on a scale. The proportion of fine parts <2 mm (PM < 2.0) from each sample was calculated (Kompała-Bąba et al., 2019).
All samples were weighed before drying in oven at 105 °C for 24 hours then they were submerged in water overnight to percolate and weighed again to calculate the percentage of water holding capacity (WHC) using the following equation:
At the same time of measuring the substrate respiration (described below in section 2.4) and in three consecutive seasons, the soil temperature (St) and the volumetric water content (SWC) records were taken from the same depth of the substrate. The thermometer DT1 Z Sonda ST01-1300 was used to measure the temperature, while the soil water content was measured by the moisture metre Delta-T HH2 (Delta-T Devices, England).
The substrate was mixed in a 1:2.5 substrate/distilled water for 24 hours, then the pH was measured by a glass electrode, while the electrical conductivity (EC) was measured in 1:5 substrate/distilled water ratio. The modified Simakov Turin method (Hristov et al., 2016) was used for total carbon determination and the Kjeldahl (Rutherford et al., 2006) method was used for the total nitrogen content. For Na extraction 1 Mol neutral ammonium acetate solution was used and the determination of Na was done by AAS (López-Marcos et al., 2018). Available K, Ca, P, and Mg were extracted by the Mehlich-3 (1:10 soil/solution ratio) solution (Mehlich, 1984; Ziadi and Tran, 2008), while K and Ca were determined by flame photometry method, P was determined by spectrophotometer and Mg was determined by the atomic absorption spectrometry (AAS).
2.4 Soil respiration (Sr)
A soil respiration chamber SRC-2 was linked to a TARGAS-1; PP system (Amesbury, MA, United States) to measure the CO2 efflux from 78 cm2 of the substrate surface attached to the chamber. The closed chamber is made of rugged PVC ending with a stainless-steel ring to seal and prevent gas leakage. The chamber relies on an internal fan to mix and flush the air accumulated to an external infrared gas analyser, where changes in CO2 concentrations are recorded in time. To adapt to the surrounding conditions and sustain the impulse of the carbon dioxide, the PP system uses an auto-zero mechanism to guarantee robust and precise readings.
2.5 Functional traits
The functional approach was based on a set of traits that have significant ecological implications for plant species dispersal, establishment, competitive ability, and stress tolerance. We used only functional traits that were available in the open databases (Supplementary Appendix S1) such as LEDA (Kleyer et al., 2008), BIEN (Maitner et al., 2018), Pladias (Wright et al., 2005; Chytrý et al., 2021), BioFlor (Klotz et al., 2002) and TRY Global Plant Traits Database (Kattge et al., 2011). Specific leaf area (SLA) and leaf dry-matter content (LDMC) serve as proxies of species status on the leaf economic spectrum (Pérez-Harguindeguy et al., 2013). High LDMC and low SLA unveil a conservative approach with resistance to the harsh abiotic stress in the mineral material of post-coalmine heaps, while low LDMC and high SLA infer the increased importance of an acquisitive strategy by plant species (Westoby et al., 2002; Pérez-Harguindeguy et al., 2013) used the canopy height as an approximation of plant competitive ability. Species with lower seed mass has been found on younger post-coalmine spoil heaps and vice versa, therefore the ability of species to colonise a new habitat can be explained through the seed mass (Piekarska-Stachowiak et al., 2014). Dispersal strategies were based on a combination of dispersal modes (e.g., autochory and epizoochory; autochory; anemochory and autochory) (Sádlo et al., 2018). For plants functional traits this study included only species with a high frequency, excluding in the analyses those with a frequency less than 0.25% of all plots.
2.6 Data analysis
To classify the sample plots, a hierarchical cluster analysis was used following the average agglomeration method in the vegan package in R software (Allaire, 2011). The test grouped the 94 cross-design plots sampled into four plant communities based on their overall plant community composition and following the dissimilarity among all plots and along all habitats. The average silhouette method (Rousseeuw, 1987) was used to determine the optimal number of clusters. Clusters (groups) were described in terms of diagnostic, constant as well as dominant species. Diagnostic species were based on fidelity, which is a measure of species concentration in vegetation units (clusters). The phi coefficient of associations was used to calculate fidelity. This measure is useful when comparing fidelity values among differently sized data sets and vegetation units. Its value ranges from −1 to 1 (multiplied by 100). Constant species were distinguished based on their frequency in a given cluster (as a threshold, we chose the occurrence of a species in at least 50% of plots in a given cluster), and dominant species were distinguished by taking into account their cover-abundance.
To find the main factors affecting the studied communities along the gradients, samples were arranged in the DCA biplot using the detrended correspondence analysis (Oksanen and Minchin, 1997), then the direction of plant diversity, functional, and community weighted means (CWM) variables along the gradient was visualised using the veganenvfit () function. Alpha taxonomic diversity indices: H′- Shannon Wiener Index, S - Species richness, E − Evenness obtained using the R Vegan package (Oksanen et al., 2022), and the function dominance () in the {abdiv} package were used to calculate the D-value of the dominance (0 < D ≤ 1), Simpson index. The impact of the studied abiotic habitat parameters on the distribution of the recorded vegetation patches was performed by canonical component analysis CCA. To fit the environmental factors onto the CCA ordination, the envfit function was used and insignificant vectors were eliminated by the (p.max = 0.5) function.
Rao’s quadratic equation index (Botta-Dukát, 2005), community weighted mean (Lavorel et al., 2008), functional dispersion index (Laliberte and Legendre, 2010) and other functional diversity indices based on functional traits have been used, which make up for the deficiency of functional-group approach. Plants functional traits (Supplementary Appendix S1) were used to compute the CWM following (Garnier et al., 2004). Functional diversity (FD) indices: FDis, FDiv, FEve, FRic, and RaoQ entropy were quantified and calculated based on the species function traits using dbFD () function in the FD package (Laliberté and Shipley, 2011).
Shapiro-Wilk multivariate normality test (Royston, 1995) was used to check the normal distribution of the samples, and independent variables were compared using the linear model (Wheeler, 2010) with permutation test in the {lmPerm} package. Variables failed to meet the normality test induced by the non-parametric test (Kruskal–Wallis). To visualise the relationship and its direction between different variables, Pearson correlation (Becker et al., 1998) was used and their significance was calculated with 999 iterations of a permutation test.
3 Results
3.1 Classification of vegetation patches based on their floristic criteria
A total of 376 vascular plant species from 245 genera and 54 families were recorded in 94 vegetation sample plots on coalmine spoil heaps. Based on the floristic criteria, the average silhouette method for the optimal number of clusters suggested two main groups of vegetation patches (Figure 2 “topright”). The first group is represented by spontaneously developed forest on post-coal mining spoil heaps at late stage (LS, framed in red) and the second group by non-forest vegetation. The hierarchical cluster analysis based on the dissimilarities of all plots further divided the non-forest vegetation into three sub stages: initial stage (IS), early stage (ES), and mid-stage (MS) as illustrated in Figure 2. These stages of succession differed in terms of diagnostic, constant as well as dominant species.
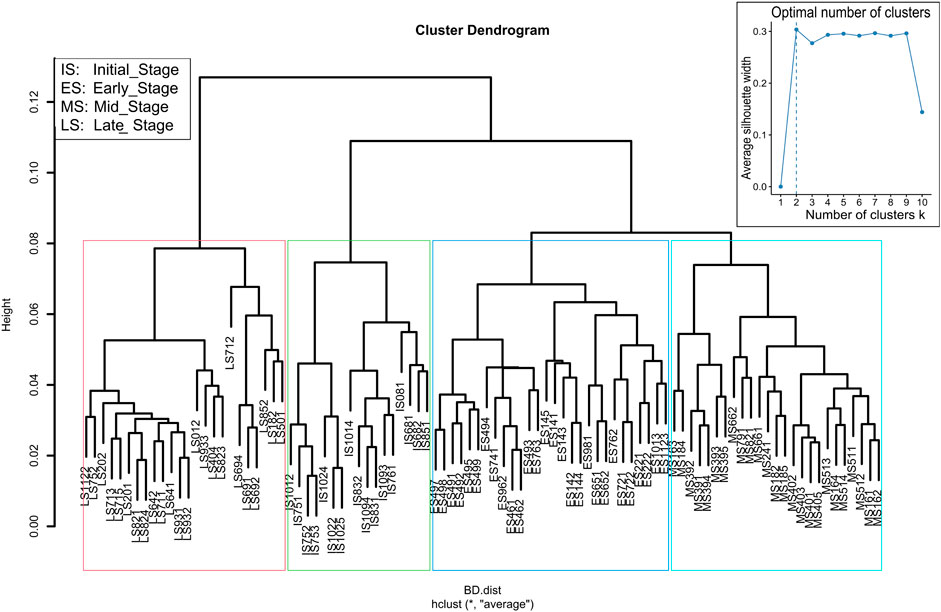
Figure 2. Hierarchical clustering of different vegetation patches developed on coalmine spoil heaps based on their floristic composition. The optimal number of clusters is indicated by the Average Silhouette method “top right”.
There were no diagnostic species in the initial stage (IS), while constant species were Calamagrostis epigejos, Polygonum aviculare, Atriplex prostrata, Puccinellia distans and Chenopodium rubrum. Only 60 species were recorded in initial stage patches as a whole.
Tussilago farfara was the only diagnostic species in early stage (ES). The species composition included ruderal species (Conyza canadensis, Oenothera biennis, Tussilago farfara), species of necrophilous fringe communities (Eupatorium cannabinum, Solidago gigantea), meadow species (Daucus carota, T. sec. Taraxacum). C. epigejos and Phragmites australis were the dominants among 168 species found in patches of the ES group (Supplementary Appendix S2).
The mid-stage (MS) had 16 diagnostic species including meadow species (Achillea millefolium, Dactylis glomerata, Galium mollugo, Lotus corniculatus, Plantago lanceolata, Trifolium pratense), and ruderal species (Melandrium album, Picris hieracioides, Tanacetum vulgare). C. epigejos, P. australis, P. lanceolata, S. gigantea reached up to 20% in some patches. MS was the richest successional stage with 238 species that occurred in all patches (Supplementary Appendix S3).
Forests in late stage (LS) had 12 diagnostic species including shrubs and trees that constitute tree and shrub layers, e.g., Acer pseudoplatanus, Betula pendula, Populus tremula, Robinia pseudoacacia, Tilia cordata, Padus serotina, Sambucus nigra, Crataegus monogyna and some forbs Geum urbanum, Impatiens parviflora. In total 135 vascular species were recorded in all forests in the LS that developed on coalmine spoil heaps (Supplementary Appendix S3).
3.2 Distribution of samples in environmental and diversity space
Among all fifteen habitat environmental factors, substrate parameters, fine particles (PM < 2.0 mm), water holding capacity (WHC), soil water content (SWC), soil temperature (St), and pH constrained the plant species distribution in the ordination space in the CCA ordination the most (10.80%). Substrate parameters: SWC, WHC, St, and pH were significant at (p ≤ 0.0001) alpha level, while the PM < 2.0 was significant at (p = 0.019) alpha level (Table 2).
A higher share of fine particles affected the water holding capacity and soil water content and decreased the substrate temperature on coalmine spoil heaps with MS and heap forest LS. On the contrary, patches in IS were characterised by very low SWC, WHC, and higher substrate temperature. As one of the most important soil parameter, the pH was higher in heap forests LS, which in turn positively correlated with the soil calcium content, total nitrogen, and total carbon (Figure 3).
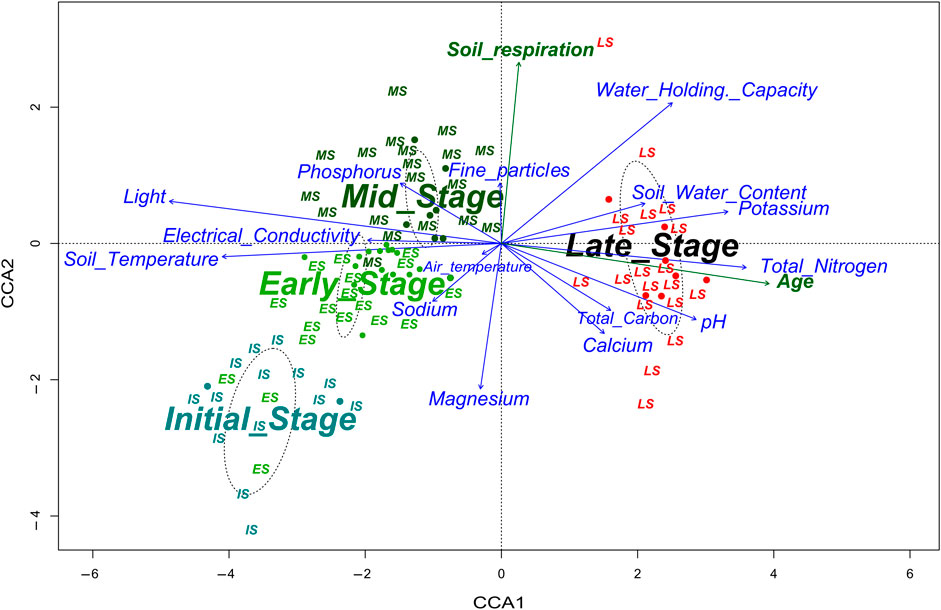
Figure 3. The CCA ordination biplot illustrates significant differences in the substrate parameters on species composition. Soil respiration and age are projected not constrained (green arrow).
Patches in the MS group were species-rich, and had the highest values of Shannon-Wiener diversity index and the vegetation mainly consisted of native species, mostly grasses (e.g., Arrhenatherum elatius, Briza media, D. glomerata, Festuca rubra, Phleum pratense).
The IS stage was species-poor and dominated by some single species (e.g., T. farfara, C. epigejos, A. prostrata, Chamaenerion palustre, and P. distans). Additionally, the IS was characterised by higher functional evenness, while other functional indices such as FRic and RaoQ entropy are more pronounced in forests developed on coalmine spoil heaps in the LS stage (Supplementary Appendix S2). Woodlands in the LS group were species rich with a well-developed tree, shrub, and herb layer, higher canopy height, and specific leaf area (Figure 4).
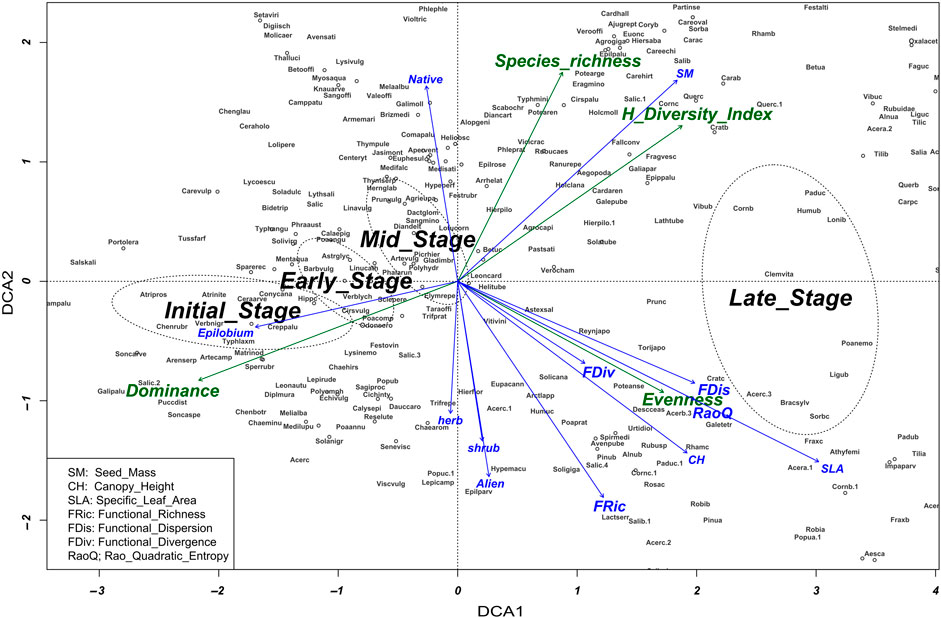
Figure 4. Detrended Correspondence Analysis (DCA) displays the correspondence of succession stages in the ordination space. Only significant variables are shown.
The diversity of spontaneous vegetation assemblages established during succession reflected the variety of each successional stage condition (Graphical abstract). As it is illustrated in the Graphical abstract, a steady increase in species number occurred from the start until the mid-stage of succession with a decline at the late stage. On the other hand, the opposite trend suggested an increase of functional diversity at late stage due to the replacement of redundant individuals by species that are more different.
Apart from the numerical analysis and the impact of the factors tested by using the permutation tests, the quantitative test of the subsequent successional stages showed significant differences. The comparison of the species richness between the four studied successional stages revealed that the highest number of species (47) was recorded in the mid-stage, followed by late stage (37), early stage (30), and finally the least number of species (9) was found in initial stage patches. The highest values of the Shannon-Wiener diversity index were recorded in MS and LS groups compared to other ones. Values of Shannon-Wiener diversity index were much higher than in IS, but did not reach a statistically significant level (Table 1).
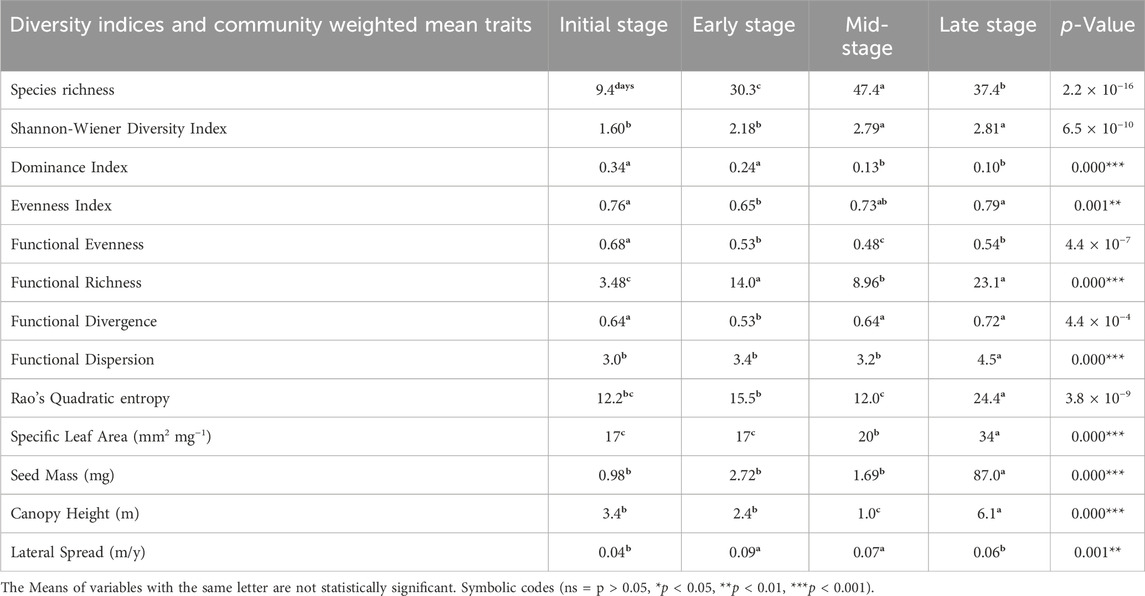
Table 1. The comparison of successional stages in terms of taxonomic diversity, functional diversity, and community weighted mean (CWM). Normally distributed variables subjected to ANOVA, Kruskal–Wallis test (with asterisks) for variables failed to meet the ANOVA assumption test; Df is 3.
Forests developed at the late stage had higher functional richness (23.1) and Rao’s quadratic entropy (24.4), followed by mid stage (FRic 14.0; RaoQ 15.5), early-stage (FRic 8.96; RaoQ 12.0), and initial stage (FRic 3.48; RaoQ 12.2). The functional divergence index value of the ES group was low and varied in others, while the functional dispersion was the highest (4.5) in LS forests and followed the same trend as RaoQ entropy (Table 1).
Soil water content and water holding capacity followed a gradual increase along the successional stages with time, while the substrate temperature took the opposite path. The gradual increase of soil respiration (Sr) form (0.22 mg-CO2 per hour per square metre) in IS to (0.53, 0.82 and 1.00) in ES, LS and MS respectively, was mainly derived from soil water content (SWC) and soil temperature (St), and vegetation species composition (Table 2). Moreover, the highest values of pH (6.25), total Nitrogen (0.46%), available potassium (210 mg kg-1), and available Calcium (1770 mg kg-1) were recorded from the forest soils in LS.
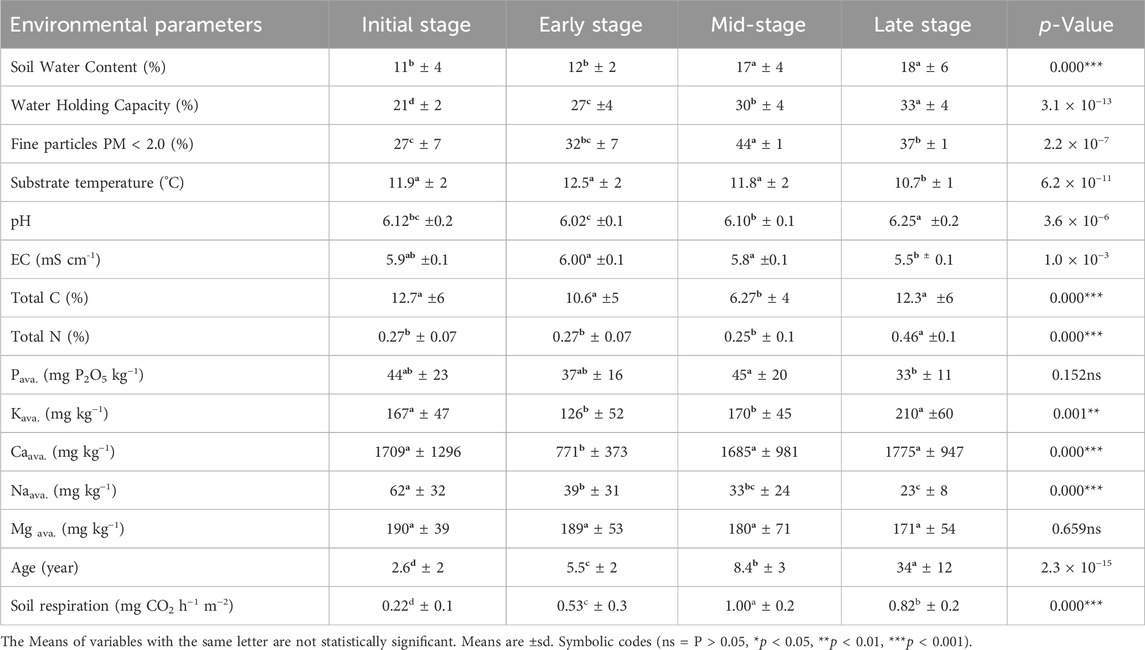
Table 2. The comparison of successional stages in terms of environmental parameters. Normally distributed variables subjected to ANOVA, Kruskal–Wallis test (with asterisks) for variables failed to meet the ANOVA assumption test; Df is 3.
Soil respiration was positively affected by substrate water holding capacity, water content, fine particles portion, plant diversity, functional richness, and to a lesser extent functional diversity (WHC; r = 0.62***, SWC; r = 0.59***, PM < 2.0; r = 0.57***, H; r = 0.50***, and RaoQ; r = 0.33**) respectively (Figure 5).
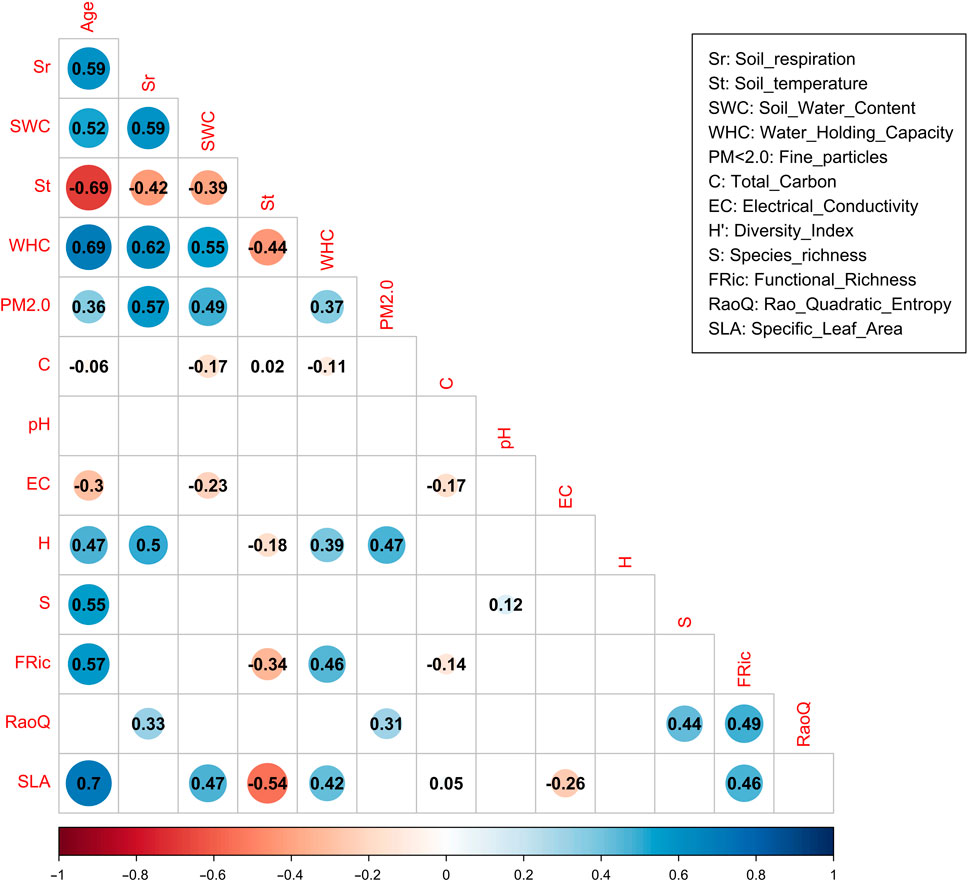
Figure 5. The correlation matrix presents the correlation coefficients combined and correlation tests for several pairs of environmental, taxonomic, and functional diversity variables.
Except for the correlation between the total carbon (r = −06 ns), pH, and RaoQ, which did not reach significant levels, all other parameters were correlated with age, either positively or negatively. Substrate temperature declined with age (St; r = −0.69***), the electrical conductivity decreased (EC; r = −0.30***), and other positive effects of aging were reflected positively and significantly the ability of the substrate to hold water (WHC; r = 0.69***), increased the soil water content (SWC; r = 0.52***), higher fine particle fraction (PM < 2.0 mm; r = 0.36***), higher number of species (S; r = 0.55***), higher plant diversity (H: r = 0.47***), higher functional richness (FRic: r = 0.57***) and higher specific leaf area (SLA: r = 0.70***). The specific leaf area correlated positively with the soil water content (SWC; r = 0.47***), water holding capacity (WHC; r = 0.42***), and functional richness (FRic; r = 0.46***).
4 Discussion
4.1 Changes in taxonomic and functional diversity along the successional stages
The four distinguished stages of succession differed in species richness, species diversity, and cover of a dominant species. Woody species rarely occurred at the beginning of the succession while seedlings of woody species started to appear just from the second stage. Pioneers such as A. prostrata, C. rubrum, P. distans, P. aviculare and T. farfara on steep slopes prone to erosion, were the first to colonise the newly disposed of mineral material on moist coal mine spoil heaps. In other studies on coal mine sites, initial plant communities in sedimentation pools were also dominated by one or two forbs (A. prostrata, P. distans) or more species in their floristic composition occurred with varied cover/abundance and that were distributed more evenly (C. rubrum, C. glaucum) (Kompała-Bąba et al., 2023). Along with the succession, both the number of species and their abundance increased, which resulted in higher values of Shannon-Wiener diversity index. In all vegetation classes studied and out of 376 species surveyed, 238 species were found in herbaceous vegetation patches that developed within a decade of heap formation and increased in abundance before reaching a more stable level in the mid-successional stage and started to decrease with development of woody species at the late successional stage. C. epigejos grows on sites with a wide range of nutrient availability, and can overcome extreme surplus and scarcity of water. The fact that C. epigejos cannot tolerate shade (Rebele and Lehmann, 2001), was probably the cause of its decline when forests developed on the coal mine sites. In further stages of succession on coal mine sedimentation pools, some perennial, tall forbs started to dominate, among them both native (T. vulgare, C. epigejos) or alien species (S. canadensis) (Błońska et al., 2019). The main direction of the successional stages considered in this study was towards the establishment of a woodland, which is typical potential vegetation in the study area (Řehounková et al., 2018).
In our study, the non-analogous species composition of the novel ecosystems established on mineral habitats of the coalmine heap environmental sites revealed that the taxonomic diversity indices were significantly different between the spontaneous vegetation successional stages distinguished in the classification analysis. The analysis of variance revealed significant differences in values of Shannon-Wiener diversity index between initial stage and early stage as compared to higher values in mid-stage and late stage vegetation. In the opposite direction, the dominance index followed an opposite trend, higher in both initial and early stages than in mid and late stages.
Species richness and diversity showed a fast gradual growth with time from initial stage to early stage reaching their peaks in mid-stage then changes were more related to plant species rather than growth in numbers (Table 1). On coalmine heaps, the absence of a light barrier is in the favour of plant diversity until canopy closure by dominant trees during the late successional stage, which accounts for the strongest environmental filter in forests (Sheil and Burslem, 2003). We observed that the stages representing the dynamic successional processes are significantly different, increasing the plant taxonomic diversity with time until reaching a certain level of herbaceous development and when forests are established. In late stage where forests developed on coal mine heaps, the canopy closure played a critical role in decreasing the species richness and the plant diversity.
In comparison to species diversity, functional diversity reflects a different approach that estimates the distribution and the range of organisms in communities and ecosystems and thus assesses the complementarity and redundancy of the co-occurring species (Tilman et al., 1996; Hulot et al., 2000; Díaz and Cabido, 2001; Heemsbergen et al., 2004; Petchey and Gaston, 2006) Functional richness and functional divergence detected changes in the plant assembly processes along our proposed stress gradients; higher calculated values of functional richness and functional divergence occurred when forests developed on coalmine spoil heaps at the late stage. This indicates higher ecological resilience of woodlands developed on coalmine spoil heaps to the environmental stressors, and better maintenance of ecosystem functioning (Hooper et al., 2005).
4.2 Environmental conditions, species diversity changes and vegetation development during the succession process
Environmental filtering is the most recognized abiotic mechanism that acts as a sieve to select species that can establish and persist in a given habitat condition from those that fail due to species-specific environmental needs (Keddy, 1992). Underlying this approach is the assumption that species, often unrelated, share similar adaptations to harsh habitat conditions that lead to a higher similarity concerning functional traits (traits convergence). Fine particles, water holding capacity, water content, temperature, and the pH of the substrate were the most crucial environmental factors that decide which species pass through environmental filters and cope with harsh abiotic conditions. This is the logical result of the niche differentiation idea that assumes that plant species in a particular location must display differences in resource acquisition to co-exist (Macarthur and Levins, 1967; Angert et al., 2009). Apart from the above concepts, the novel ecosystem conditions bring new dimensions into habitat-diversity relationships.
The current results regarding shifts in diversity patterns during succession are inconclusive (Helsen et al., 2012), while other results were ambiguous (Schleicher et al., 2011; Chang and HilleRisLambers, 2019), concluding that there are no consistent changes in community assembly processes along successional gradients.
The process of plant colonization on newly open mineral habitats depends primarily on the type of the material and its properties (Tang et al., 2003; Alday et al., 2011). In our study, the soil water content turned out to be the most important variable that influenced the colonisation pattern on post-coal mine spoil heaps. Moreover, fine particles (smaller than 2 mm) of the substrate played an important role in vegetation development and increased substrate water holding capacity and soil water content. The mineral rock with a significant amount of nutrient elements and soil small or medium particle fractions associated with high water holding capacity and aeration promotes the colonisation of vegetation (Rahmonov et al., 2020).
The total carbon measured from the rock substrates was higher in initial and late stages. Higher values of parameters such as total nitrogen, available potassium, and calcium were mainly due to the decomposition of dead roots and above-ground dead biomass in forests developed on heaps (Shannon et al., 2022). The release of different elements from the organic matter, especially the calcium to the organic layer affected the pH, which was higher in substrates of forests developed on coalmine spoil heaps. The Na concentration declined along the successional gradient, reaching its lowest levels in heap forests; with time soluble salts washed out and the electrical conductivity decreased.
4.3 Soil respiration as the functional aspect parallel to vegetation diversity
The carbon dioxide released from the substrate differs remarkably in different vegetation and ecosystem types due to specific assembly rules of the plant community depending on the habitat filtering concept (Kraft et al., 2014). Our study confirmed that carbon release differs on coalmine spoil heaps and higher soil respiration in the mid-stage of succession due to differences in vegetation composition and habitats. In woodlands at late succession, the carbon is stored in below and above-ground living biomass, deadwood, and leaf litter (Shannon et al., 2022), following the sequestration through the products of photosynthesis.
Studies lacking the seasonal variability and inter-annual records for soil respiration rely on how the diversity of plant communities stabilise carbon dynamic (Korrensalo et al., 2017) to explain the role of plant richness in the carbon cycle especially if different species reach their optimal performance at different times. We measured the soil respiration in spring, summer and autumn to avoid seasonal variation of respiration. Our results showed an increase of the respiration rate by two folds shifting from the initial to the early stage and another two folds more in mid-stage which counts as an evident proof that the species richness played the main role. This is in line with results suggesting significant differences between vegetation types and the role of environmental filtering in plant community assembly (Kraft et al., 2014).
5 Conclusion
Considering that the harsh conditions of post-coalmine spoil heaps persist for decades after their abandonment, we detected four development stages during the spontaneous succession depending on the plant community composition and the age of the heap. The taxonomic diversity showed a different pattern than the functional diversity during succession; heap forest had the highest functional diversity, whereas the herbaceous vegetation, especially in the mid-stage, was richer in species number and species diversity. Native plant species that can cope with hostile habitats such as heaps and mounds of technological wastes, and saline habitats, were the first and the most abundant species recolonizing such de novo habitats. Their rapid development is clear evidence that spontaneous succession is the most suitable process to restore the altered site due to coal excavation in the temperate zone. More studies are needed to investigate the carbon dynamics on post-coalmine spoil heaps and whether such novel ecosystems act as a carbon storage pool.
Data availability statement
The original contributions presented in the study are included in the article/Supplementary Material, further inquiries can be directed to the corresponding author.
Author contributions
JB: Conceptualization, Formal Analysis, Investigation, Methodology, Resources, Software, Validation, Writing–original draft, Writing–review and editing. AK-B: Conceptualization, Formal Analysis, Investigation, Methodology, Resources, Validation, Writing–original draft, Writing–review and editing. WB: Conceptualization, Investigation, Methodology, Resources, Validation, Writing–original draft, Writing–review and editing. DC: Formal Analysis, Software, Validation, Writing–review and editing. AH: Data curation, Investigation, Project administration, Resources, Validation, Visualization, Writing–review and editing. AB: Investigation, Resources, Validation, Writing–review and editing. TN: Validation, Writing–review and editing. FM: Validation, Writing–review and editing. AMJ: Funding acquisition, Writing–review and editing, Validation. GW: Conceptualization, Data curation, Funding acquisition, Investigation, Methodology, Project administration, Resources, Supervision, Validation, Writing–original draft.
Funding
The author(s) declare that financial support was received for the research, authorship, and/or publication of this article. This research funded by the Poland National Science Centre (Grant Number OPUS 2019/35/B/ST10/04141).
Conflict of interest
The authors declare that the research was conducted in the absence of any commercial or financial relationships that could be construed as a potential conflict of interest.
Publisher’s note
All claims expressed in this article are solely those of the authors and do not necessarily represent those of their affiliated organizations, or those of the publisher, the editors and the reviewers. Any product that may be evaluated in this article, or claim that may be made by its manufacturer, is not guaranteed or endorsed by the publisher.
Supplementary material
The Supplementary Material for this article can be found online at: https://www.frontiersin.org/articles/10.3389/fenvs.2024.1412631/full#supplementary-material
References
Alday, J. G., Pallavicini, Y., Marrs, R. H., and Martínez-Ruiz, C. (2011). Functional groups and dispersal strategies as guides for predicting vegetation dynamics on reclaimed mines. Plant Ecol. 212, 1759–1775. doi:10.1007/s11258-011-9947-6
Allaire, J. (2011). RStudio: integrated development environment for R. J. Wildl. Manage. 75, 1753–1766.
Angert, A. L., Huxman, T. E., Chesson, P., and Venable, D. L. (2009). Functional tradeoffs determine species coexistence via the storage effect. Proc. Natl. Acad. Sci. U. S. A. 106, 11641–11645. doi:10.1073/pnas.0904512106
Anibaba, Q. A., Dyderski, M. K., Woźniak, G., and Jagodziński, A. M. (2023). Native plant community characteristics explain alien species success in post-industrial vegetation. NeoBiota 85, 1–22. doi:10.3897/NEOBIOTA.85.97269
Batalha, M. A., Pipenbaher, N., Bakan, B., Kaligarič, M., and Škornik, S. (2015). Assessing community assembly along a successional gradient in the North Adriatic Karst with functional and phylogenetic distances. Oecologia 178, 1205–1214. doi:10.1007/s00442-015-3295-5
Becker, R. A., Chambers, J. M., and Wilks, A. R. (1998). “Wadsworth and brooks/cole,” in The new S language.
Bierza, W., Czarnecka, J., Błońska, A., Kompała-Bąba, A., Hutniczak, A., Jendrzejek, B., et al. (2023). Plant diversity and species composition in relation to soil enzymatic activity in the novel ecosystems of urban–industrial landscapes. Sustain 15, 7284. doi:10.3390/su15097284
Błońska, A., Kompała-Bąba, A., Sierka, E., Bierza, W., Magurno, F., Besenyei, L., et al. (2019). Diversity of vegetation dominated by selected grass species on coal-mine spoil heaps in terms of reclamation of post-industrial areas. J. Ecol. Eng. 20, 209–217. doi:10.12911/22998993/93870
Booth, B. D., Murphy, S. D. D., and Clarence, J. J. S. (2003). Weed ecology in natural and agricultural systems, 1st Edn. Oxford United States Oxford University Press.
Botta-Dukát, Z. (2005). Rao’s quadratic entropy as a measure of functional diversity based on multiple traits. J. Veg. Sci. 16, 533. doi:10.1658/1100-9233(2005)16[533:rqeaam]2.0.co;2
Bradshaw, A. (2000). The use of natural processes in reclamation - advantages and difficulties. Landsc. Urban Plan. 51, 89–100. doi:10.1016/S0169-2046(00)00099-2
Cabala, J. M., Cmiel, S. R., and Idziak, A. F. (2004). Environmental impact of mining activity in the upper Silesian coal basin (Poland). Geol. Belg. 7, 225–229.
Chang, C. C., and HilleRisLambers, J. (2019). Trait and phylogenetic patterns reveal deterministic community assembly mechanisms on Mount St. Helens. Plant Ecol. 220, 675–698. doi:10.1007/s11258-019-00944-x
Chytrý, M., Danihelka, J., Kaplan, Z., Wild, J., Holubová, D., Novotný, P., et al. (2021). Pladias database of the Czech flora and vegetation. Preslia 93, 1–87. doi:10.23855/PRESLIA.2021.001
Dequéant, M. L., Glynn, E., Gaudenz, K., Wahl, M., Chen, J., Mushegian, A., et al. (2006). A complex oscillating network of signaling genes underlies the mouse segmentation clock. Sci. (80-. ) 314, 1595–1598. doi:10.1126/science.1133141
Díaz, S., and Cabido, M. (2001). Vive la différence: Plant functional diversity matters to ecosystem processes. Trends Ecol. Evol. 16, 646–655. doi:10.1016/S0169-5347(01)02283-2
Garnier, E., Cortez, J., Billès, G., Navas, M. L., Roumet, C., Debussche, M., et al. (2004). Plant functional markers capture ecosystem properties during secondary succession. Ecology 85, 2630–2637. doi:10.1890/03-0799
Grime, J. P. (1998). Benefits of plant diversity to ecosystems: immediate, filter and founder effects. J. Ecol. 86, 902–910. doi:10.1046/j.1365-2745.1998.00306.x
Heemsbergen, D. A., Berg, M. P., Loreau, M., Van Hal, J. R., Faber, J. H., and Verhoef, H. A. (2004). Biodiversity effects on soil processes explained by interspecific functional dissimilarity. Sci. (80-. ) 306, 1019–1020. doi:10.1126/science.1101865
Helsen, K., Hermy, M., and Honnay, O. (2012). Trait but not species convergence during plant community assembly in restored semi-natural grasslands. Oikos 121, 2121–2130. doi:10.1111/j.1600-0706.2012.20499.x
Hobbs, R. J., Arico, S., Aronson, J., Baron, J. S., Bridgewater, P., Cramer, V. A., et al. (2006). Novel ecosystems: theoretical and management aspects of the new ecological world order. Glob. Ecol. Biogeogr. 15, 1–7. doi:10.1111/j.1466-822X.2006.00212.x
Hooper, D. U., Chapin, F. S., Ewel, J. J., Hector, A., Inchausti, P., Lavorel, S., et al. (2005). Effects of biodiversity on ecosystem functioning: a consensus of current knowledge. Ecol. Monogr. 75, 3–35. doi:10.1890/04-0922
Hristov, B., Filcheva, E., and Ivanov, P. (2016). Organic matter content and composition of soils with stagnic properties from Bulgaria. Bulg. J. Soil Sci., 25–32. doi:10.5281/zenodo.2579058
Hulot, F. D., Lacroix, G., Lescher-Moutoué, F., and Loreau, M. (2000). Functional diversity governs ecosystem response to nutrient enrichment. Nature 405, 340–344. doi:10.1038/35012591
Isbell, F., Craven, D., Connolly, J., Loreau, M., Schmid, B., Beierkuhnlein, C., et al. (2015). Biodiversity increases the resistance of ecosystem productivity to climate extremes. Nature 526, 574–577. doi:10.1038/nature15374
Kattge, J., Díaz, S., Lavorel, S., Prentice, I. C., Leadley, P., Bönisch, G., et al. (2011). TRY - a global database of plant traits. Glob. Chang. Biol. 17, 2905–2935. doi:10.1111/j.1365-2486.2011.02451.x
Keddy, P. A. (1992). Assembly and response rules: two goals for predictive community ecology. J. Veg. Sci. 3, 157–164. doi:10.2307/3235676
Keith, H., Mackey, B. G., and Lindenmayer, D. B. (2009). Re-evaluation of forest biomass carbon stocks and lessons from the world’s most carbon-dense forests. Proc. Natl. Acad. Sci. U. S. A. 106, 11635–11640. doi:10.1073/pnas.0901970106
Kirby, K. R., and Potvin, C. (2007). Variation in carbon storage among tree species: implications for the management of a small-scale carbon sink project. For. Ecol. Manage. 246, 208–221. doi:10.1016/j.foreco.2007.03.072
Kleyer, M., Bekker, R. M., Knevel, I. C., Bakker, J. P., Thompson, K., Sonnenschein, M., et al. (2008). The LEDA Traitbase: a database of life-history traits of the Northwest European flora. J. Ecol. 96, 1266–1274. doi:10.1111/j.1365-2745.2008.01430.x
Klotz, S., Kühn, I., and Durka, W. (2002). BIOLFLOR - Eine Datenbank mit biologisch-ökologischen Merkmalen zur Flora von Deutschland. Schriftenr. für Veg. 38, 1–334.
Kompała-Bąba, A., Bąba, W., Ryś, K., Hanczaruk, R., Radosz, Ł., Prostański, D., et al. (2023). Taxonomic diversity and selection of functional traits in novel ecosystems developing on coal-mine sedimentation pools. Sustain 15, 2094–2119. doi:10.3390/su15032094
Kompała-Bąba, A., Bierza, W., Błońska, A., Sierka, E., Magurno, F., Chmura, D., et al. (2019). Vegetation diversity on coal mine spoil heaps – how important is the texture of the soil substrate? Biol. Bratisl. 74, 419–436. doi:10.2478/s11756-019-00218-x
Kompała-Bąba, A., Bierza, W., Sierka, E., Błońska, A., Besenyei, L., and Woźniak, G. (2021). The role of plants and soil properties in the enzyme activities of substrates on hard coal mine spoil heaps. Sci. Rep. 11, 5155–5213. doi:10.1038/s41598-021-84673-0
Kompała-Bąba, A., Sierka, E., Dyderski, M. K., Bierza, W., Magurno, F., Besenyei, L., et al. (2020). Do the dominant plant species impact the substrate and vegetation composition of post-coal mining spoil heaps? Ecol. Eng. 143, 105685. doi:10.1016/j.ecoleng.2019.105685
Korrensalo, A., Alekseychik, P., Hájek, T., Rinne, J., Vesala, T., Mehtätalo, L., et al. (2017). Species-specific temporal variation in photosynthesis as a moderator of peatland carbon sequestration. Biogeosciences 14, 257–269. doi:10.5194/bg-14-257-2017
Kowarik, I. (2011). Novel urban ecosystems, biodiversity, and conservation. Environ. Pollut. 159, 1974–1983. doi:10.1016/j.envpol.2011.02.022
Kraft, B., Tegetmeyer, H. E., Sharma, R., Klotz, M. G., Ferdelman, T. G., Hettich, R. L., et al. (2014). The environmental controls that govern the end product of bacterial nitrate respiration. Sci. (80-. ) 345, 676–679. doi:10.1126/science.1254070
Laliberte, E., and Legendre, P. (2010). A distance-based framework for measuring functional diversity from multiple traits. Ecology 91, 299–305. doi:10.1890/08-2244.1
Laliberté, E., and Shipley, B. (2011). FD: measuring functional diversity from multiple traits, and other tools for functional ecology. R. package version 1.
Lavorel, S., Díaz, S., Cornelissen, J. H. C., Garnier, E., Harrison, S. P., McIntyre, S., et al. (2007). “Plant functional types: are we getting any closer to the holy grail?,” in Terrestrial Ecosystems in a Changing World (Berlin, Heidelberg: Springer Berlin Heidelberg), 149–160. doi:10.1007/978-3-540-32730-1_13
Lavorel, S., Grigulis, K., McIntyre, S., Williams, N. S. G., Garden, D., Dorrough, J., et al. (2008). Assessing functional diversity in the field - Methodology matters. Funct. Ecol. 22, 134–147. doi:10.1111/j.1365-2435.2007.01339.x
López-Marcos, D., Martínez-Ruiz, C., Turrión, M. B., Jonard, M., Titeux, H., Ponette, Q., et al. (2018). Soil carbon stocks and exchangeable cations in monospecific and mixed pine forests. Eur. J. For. Res. 137, 831–847. doi:10.1007/s10342-018-1143-y
Macarthur, R., and Levins, R. (1967). The limiting similarity, convergence, and divergence of coexisting species. Am. Nat. 101, 377–385. doi:10.1086/282505
Maitner, B. S., Boyle, B., Casler, N., Condit, R., Donoghue, J., Durán, S. M., et al. (2018). The bien r package: a tool to access the Botanical Information and Ecology Network (BIEN) database. Methods Ecol. Evol. 9, 373–379. doi:10.1111/2041-210X.12861
Mason, N. W. H., Irz, P., Lanoiselée, C., Mouillot, D., and Argillier, C. (2008). Evidence that niche specialization explains species-energy relationships in lake fish communities. J. Anim. Ecol. 77, 285–296. doi:10.1111/j.1365-2656.2007.01350.x
Mason, N. W. H., and Pavoine, S. (2013). Does trait conservatism guarantee that indicators of phylogenetic community structure will reveal niche-based assembly processes along stress gradients? J. Veg. Sci. 24, 820–833. doi:10.1111/jvs.12033
Mehlich, A. (1984). Mehlich 3 soil test extractant: a modification of Mehlich 2 extractant. Commun. Soil Sci. Plant Anal. 15, 1409–1416. doi:10.1080/00103628409367568
Meiners, S. J., Cadotte, M. W., Fridley, J. D., Pickett, S. T. A., and Walker, L. R. (2015). Is successional research nearing its climax? New approaches for understanding dynamic communities. Funct. Ecol. 29, 154–164. doi:10.1111/1365-2435.12391
Mirek, Z., Piękoś-Mirkowa, H., Zając, A., and Zając, M. (2020). Vascular Plants of Poland. An annotated checklist [Rośliny naczyniowe Polski. Adnotowany wykaz gatunków]. 3rd Edn. Krakow: Institute of Botany, Polish Academy of Sciences.
Mouchet, M. A., Villéger, S., Mason, N. W. H., and Mouillot, D. (2010). Functional diversity measures: an overview of their redundancy and their ability to discriminate community assembly rules. Funct. Ecol. 24, 867–876. doi:10.1111/j.1365-2435.2010.01695.x
Oksanen, J., Blanchet, F. G., Simpson, G. L., Blanchet, F. G., Solymos, P., Stevens, M. H. H., et al. (2022). Title community ecology package. Vegan Community Ecol. Packag.
Oksanen, J., and Minchin, P. R. (1997). Instability of ordination results under changes in input data order: explanations and remedies. J. Veg. Sci. 8, 447–454. doi:10.2307/3237336
Peltzer, D. A., Wardle, D. A., Allison, V. J., Baisden, W. T., Bardgett, R. D., Chadwick, O. A., et al. (2010). Understanding ecosystem retrogression. Ecol. Monogr. 80, 509–529. doi:10.1890/09-1552.1
Pérez-Harguindeguy, N., Díaz, S., Garnier, E., Lavorel, S., Poorter, H., Jaureguiberry, P., et al. (2013). New handbook for standardised measurement of plant functional traits worldwide. Aust. J. Bot. 61, 167–234. doi:10.1071/BT12225
Petchey, O. L., and Gaston, K. J. (2006). Functional diversity: back to basics and looking forward. Ecol. Lett. 9, 741–758. doi:10.1111/j.1461-0248.2006.00924.x
Piekarska-Stachowiak, A., Szary, M., Ziemer, B., Besenyei, L., and Woźniak, G. (2014). An application of the plant functional group concept to restoration practice on coal mine spoil heaps. Ecol. Res. 29, 843–853. doi:10.1007/s11284-014-1172-z
Prach, K. (1987). Succession of vegetation on dumps from strip coal mining, N. W. Bohemia, Czechoslovakia. Czechoslov. Folia Geobot. Phytotaxon. 22, 339–354. doi:10.1007/BF02853231
Prach, K., Lencová, K., Řehounková, K., Dvořáková, H., Jírová, A., Konvalinková, P., et al. (2013). Spontaneous vegetation succession at different central European mining sites: a comparison across seres. Environ. Sci. Pollut. Res. 20, 7680–7685. doi:10.1007/s11356-013-1563-7
Prach, K., and Walker, L. R. (2020). Comparative plant succession among terrestrial biomes of the world. Cambridge United Kingdom: Cambridge University Press. doi:10.1017/9781108561167
Purschke, O., Schmid, B. C., Sykes, M. T., Poschlod, P., Michalski, S. G., Durka, W., et al. (2013). Contrasting changes in taxonomic, phylogenetic and functional diversity during a long-term succession: insights into assembly processes. J. Ecol. 101, 857–866. doi:10.1111/1365-2745.12098
Rahmonov, O., Krzysztofik, R., Środek, D., and Smolarek-Lach, J. (2020). Vegetation-and environmental changes on non-reclaimed spoil heaps in Southern Poland. Biol. (Basel) 9, 164–222. doi:10.3390/biology9070164
Rebele, F., and Lehmann, C. (2001). Biological flora of central europe: Calamagrostis epigejos (L.) Roth. Flora 196, 325–344. doi:10.1016/S0367-2530(17)30069-5
Řehounková, K., Lencová, K., and Prach, K. (2018). Spontaneous establishment of woodland during succession in a variety of central European disturbed sites. Ecol. Eng. 111, 94–99. doi:10.1016/j.ecoleng.2017.11.016
Rousseeuw, P. J. (1987). Silhouettes: a graphical aid to the interpretation and validation of cluster analysis. J. Comput. Appl. Math. 20, 53–65. doi:10.1016/0377-0427(87)90125-7
Royston, P. (1995). Remark as R94: a remark on algorithm as 181: the W-test for normality. Appl. Stat. 44, 547. doi:10.2307/2986146
Rutherford, P. M., McGill, W., Arocena, J. M., and Figueiredo, C. T. (2006). “Total nitrogen,” in Soil sampling and methods of analysis. Editors M. R. Carter, and E. G. Gregoric (Boca Raton: CRC Press), 267–278.
Sádlo, J., Chytrý, M., Pergl, J., and Pyšek, P. (2018). Plant dispersal strategies: a new classification based on the multiple dispersal modes of individual species. Preslia 90, 1–22. doi:10.23855/preslia.2018.001
Schleicher, A., Biedermann, R., and Kleyer, M. (2011). Dispersal traits determine plant response to habitat connectivity in an urban landscape. Landsc. Ecol. 26, 529–540. doi:10.1007/s10980-011-9579-1
Schuldt, A., Wubet, T., Buscot, F., Staab, M., Assmann, T., Böhnke-Kammerlander, M., et al. (2015). Multitrophic diversity in a biodiverse forest is highly nonlinear across spatial scales. Nat. Commun. 6, 10169. doi:10.1038/ncomms10169
Shannon, V. L., Vanguelova, E. I., Morison, J. I. L., Shaw, L. J., and Clark, J. M. (2022). The contribution of deadwood to soil carbon dynamics in contrasting temperate forest ecosystems. Eur. J. For. Res. 141, 241–252. doi:10.1007/s10342-021-01435-3
Sheil, D., and Burslem, D. F. R. P. (2003). Disturbing hypotheses in tropical forests. Trends Ecol. Evol. 18, 18–26. doi:10.1016/S0169-5347(02)00005-8
Szczepańska, J. (1987). Zwałowiska odpadów górnictwa węgla kamiennego jako ogniska zanieczyszczeń środowiska wodnego. Kraków: Akademia Górniczo-Hutnicza im. S. Staszica w Krakowie.
Tang, J., Baldocchi, D. D., Qi, Y., and Xu, L. (2003). Assessing soil CO2 efflux using continuous measurements of CO2 profiles in soils with small solid-state sensors. Agric. For. Meteorol. 118, 207–220. doi:10.1016/S0168-1923(03)00112-6
Tilman, D., Wedin, D., and Knops, J. (1996). Productivity and sustainability influenced by biodiversity in grassland ecosystems. Nature 379, 718–720. doi:10.1038/379718a0
Westoby, M., Falster, D. S., Moles, A. T., Vesk, P. A., and Wright, I. J. (2002). Plant ecological strategies: some leading dimensions of variation between species. Annu. Rev. Ecol. Syst. 33, 125–159. doi:10.1146/annurev.ecolsys.33.010802.150452
Wheeler, R. E. (2010). Permutation tests for linear models in R. R doc. Available at: http://www.papers3://publication/uuid/6B7EDCFC-D1A6-4EED-9923-443041BD089E.
Woźniak, G., Dyderski, M. K., Kompała-Bąba, A., Jagodziński, A. M., Pasierbiński, A., Błońska, A., et al. (2021). Use of remote sensing to track postindustrial vegetation development. L. Degrad. Dev. 32, 1426–1439. doi:10.1002/ldr.3789
Wright, I. J., Reich, P. B., Cornelissen, J. H. C., Falster, D. S., Garnier, E., Hikosaka, K., et al. (2005). Assessing the generality of global leaf trait relationships. New Phytol. 166, 485–496. doi:10.1111/j.1469-8137.2005.01349.x
Zelený, D. (2022). Analysis of community ecology data in R. Available at: https://www.davidzeleny.net/anadat-r/doku.php/en:sampling_design.
Zhang, Y., Chen, H. Y. H., and Reich, P. B. (2012). Forest productivity increases with evenness, species richness and trait variation: a global meta-analysis. J. Ecol. 100, 742–749. doi:10.1111/j.1365-2745.2011.01944.x
Keywords: abiotic factors, plant functional traits, community weighted mean, novel ecosystems, soil respiration
Citation: Bakr J, Kompała-Bąba A, Bierza W, Chmura D, Hutniczak A, Błońska A, Nowak T, Magurno F, Jagodziński AM and Woźniak G (2024) Taxonomic and functional diversity along successional stages on post-coalmine spoil heaps. Front. Environ. Sci. 12:1412631. doi: 10.3389/fenvs.2024.1412631
Received: 05 April 2024; Accepted: 04 June 2024;
Published: 08 July 2024.
Edited by:
Vinicius Londe, University of Georgia, United StatesReviewed by:
Pavla Vachová, Czech University of Life Sciences Prague, CzechiaLaura Riba-Hernández, National University of Costa Rica, Costa Rica
Copyright © 2024 Bakr, Kompała-Bąba, Bierza, Chmura, Hutniczak, Błońska, Nowak, Magurno, Jagodziński and Woźniak. This is an open-access article distributed under the terms of the Creative Commons Attribution License (CC BY). The use, distribution or reproduction in other forums is permitted, provided the original author(s) and the copyright owner(s) are credited and that the original publication in this journal is cited, in accordance with accepted academic practice. No use, distribution or reproduction is permitted which does not comply with these terms.
*Correspondence: Agnieszka Hutniczak, agnieszka.hutniczak@us.edu.pl
†ORCID: Jawdat Bakr, orcid.org/0000-0002-2539-3889; Agnieszka Kompała-Bąba, orcid.org/0000-0002-4736-4704; Wojciech Bierza, orcid.org/0000-0002-1789-5512; Damian Chmura, orcid.org/0000-0002-0242-8962; Agnieszka Hutniczak, orcid.org/0000-0002-6235-6139; Agnieszka Błońska, orcid.org/0000-0002-1696-0001; Teresa Nowak, orcid.org/0000-0001-7519-6309; Franco Magurno, orcid.org/0000-0002-3117-8149; Andrzej M. Jagodziński, orcid.org/0000-0001-6899-0985; Gabriela Woźniak: orcid.org/0000-0003-1936-2880