- 1Rubber Research Institute, Chinese Academy of Tropical Agricultural Sciences/Danzhou Investigation and Experiment Station of Tropical Crops, Ministry of Agriculture and Rural Affairs, Danzhou, China
- 2Wenchang Rubber Research Institute of Hainan State Farms / Wenchang Experimental Station, China Industry Technological System Construction of Natural Rubber, Wenchang, China
- 3Hainan Meteorological Bureau, Haikou, China
Understanding the process of litterfall production is crucial for sustainable development of plantations. However, the underlying dynamics of litterfall and its nutrient return in plantation agroforestry systems remain unclear. In this study, we investigated litterfall, including leaves, branches, flowers, and fruits, in three patterns: Hevea monoculture system (RM), Hevea–Michelia intercropping system (RAS1), and Hevea–Mytilaria intercropping system (RAS2) in Hainan Island, China. Our findings indicate that total litterfall was significantly higher in RAS1 (27,309 kg ha−1) and RAS2 (34,477 kg ha−1) than in RM (22,364 kg ha−1) and was predominantly composed of leaf litterfall in all three patterns, followed by branches, flowers, and fruits. The seasonal dynamics litterfall production of RM, RAS1, and RAS2 showed characteristic patterns. Litterfall nutrients exhibited peak and sub-peak monthly dynamics, peaking from February to March, during the dry season. Total nitrogen (TN), total phosphorus (TP), and total potassium (TK) content of annual litterfall in RAS1 significantly increased by 120 kg ha−1, 30 kg ha−1, and 139 kg ha−1, respectively, compared to those in RM, with percentage increases of 67.88%, 122.79% and 96.27%, respectively. Similarly, TN, TP, and TK content of annual litterfall in RAS2 significantly increased by 185 kg ha−1, 35 kg ha−1, and 170 kg ha−1, respectively, with percentage increases of 103.70%, 159.15% and 139.46%, respectively, for the abovementioned in RM. Litterfall showed a strong correlation with monthly average temperature, monthly minimum temperature, and monthly average wind speed, contributing 80.5%, 75.5%, 69.8%, and 69.6% to the total litterfall and its components, respectively. Further analysis indicated that monthly average temperature, monthly minimum temperature, and monthly average wind speed contributed 73.9%, 43.0%, and 66.6%, respectively, to TN, TP, and TK content of the annual litterfall, highlighting the significant influence of temperature and wind speed. These findings enhance our understanding of carbon and nutrient cycling and contribute to the sustainable management of tropical plantation ecosystems.
1 Introduction
With the pressing challenges of global change and the imperative for sustainable ecosystems, it becomes essential to ensure a balanced and efficient utilization of materials and energy within each ecosystem. Litter production and decomposition represent pivotal aspects of ecosystem dynamics, playing a crucial ecological role (Liu and Sun, 2013). The biophysical processes involved in litterfall contribute significantly to maintaining soil nutrient levels, enhancing primary productivity, regulating nutrient cycling and energy flow, and even elucidating litter response to global change (Waring and Schlesinger et al., 1985; Liu et al., 2024). Particularly in forest ecosystems, the litterfall process is instrumental in biogeochemical cycling of nutrients (Krishna and Mohan, 2017). Over half of the net primary productivity in forest ecosystems undergoes decomposition as litterfall, replenishing soil nutrients (Schlesinger, 1997). The litterfall process is influenced by various environmental factors (Piscart et al., 2011; Wang et al., 2015). Therefore, studying litterfall process and the influencing factors holds significant practical importance for forest ecosystem management amid global change.
As the world economy globalizes and the material needs of the human population worldwide continue to escalate, the scale and intensity of plantations have been expanding worldwide. However, increasing management intensity in plantations poses challenges in simultaneously practicing sustainability measures and ensuring quality assurance (Liu JQ et al., 2018; Singh et al., 2021). Hence, there arises a need to explore the diverse and crucial roles of plantation systems in material circulation, product supply, ecological environment enhancement, and response to global change. The sustainability of growing plantation systems is fundamental in achieving these goals. The rubber tree [Hevea brasiliensis (Willd. ex A. Juss.) Müll. Arg.] stands out as an economically significant crop in the tropical systems worldwide. Since its introduction in 1904, it has occupied 1,167,300 ha in southern China (Tang et al., 2016). Unfortunately, rubber monocultures have led to severe soil problems, including nutrient leaching, soil erosion, and compaction (Mann, 2009; Liu, 2014; Liu et al., 2015; Liu SR et al., 2018). Notably, soil total nitrogen (TN), available phosphorus, and total phosphorous (TP) degraded more rapidly in rubber monocultures than in rainforests, posing a threat to green development and biodiversity in tropical systems. This trend threatens green development and biodiversity in tropical systems (Sun et al., 2016; Jiang et al., 2017). Agroforestry ecosystems, or intercropping systems, represent sustainable land use patterns wherein agricultural and production systems harmonize to achieve efficient and sustainable utilization of materials and energy, thereby enhancing total productivity and economic benefits (Arisara et al., 2018). By leveraging ecological complementarity between different species, agroforestry systems can conserve water and soil and increase carbon storage (Ehrenbergerová et al., 2016; Weerasekara et al., 2016). Hence, some studies advocate for the establishment of rubber-based agroforestry systems using Hevea and other crops or tree species as an effective method to overcome the aforementioned obstacles (Wu et al., 2016; Qi et al., 2021).
Several studies have explored litterfall dynamics within rubber-based agroforestry ecosystems (Liu et al., 2017; Zhu et al., 2021). However, the challenges presented by rubber plantation ecosystems concerning soil quality degradation and their impact on global climate change remain unclear. Moreover, there is a notable gap in research regarding the influence of rubber-based agroforestry on litterfall composition and nutrient return patterns. We hypothesize that rubber intercropping with native trees can significantly change litterfall and litter main nutrient return. This study speculates that rubber-based agroforestry may alter litterfall composition, leading to increased litterfall and nutrient content in rubber plantations. Through the analysis of litterfall dynamics and its primary nutrient return mechanisms in rubber agroforestry systems, this research aims to provide insights crucial for sustainable development of rubber plantations.
2 Materials and methods
2.1 Study site
The study area was designated within Wenchang District, Hainan, China, situated at coordinates 19°36 N and 110°38’ E, at an elevation of 15 m above sea level. This region experiences a tropical island monsoon climate characterized by a rainy season spanning from May to October. The average annual temperature is approximately 24°C with an average annual sunshine duration of 1,954 h. During the rainy season, heavy rainfall is common, contributing to an annual precipitation of 1,722 mm on average, accompanied by a mean humidity level consistently exceeding 80%.
2.2 Experiment design
This experiment examined three treatments: the Hevea monoculture system (RM), Hevea–Michelia intercropping system (RAS1), and Hevea–Mytilaria intercropping system (RAS2). Each treatment had three replicates. Both trees tested in this study were 6 years old and consistently cultivated under the same management practices. The rubber trees were planted at a density of 3 m × 7 m, resulting in a stocking density of 495 plants per hectare. In the intercropping system plantations of rubber trees with Michelia macclurei, the planting densities of each rubber tree were kept the same. M. macclurei and Mytilaria laosensis plants were positioned in the middle of every four rubber trees (Figure 1). Throughout the year, each plant received 15 kg (kg) of organic manure fertilizer, 0.5 kg of calcium superphosphate, and 0.5 kg of compound fertilizer.
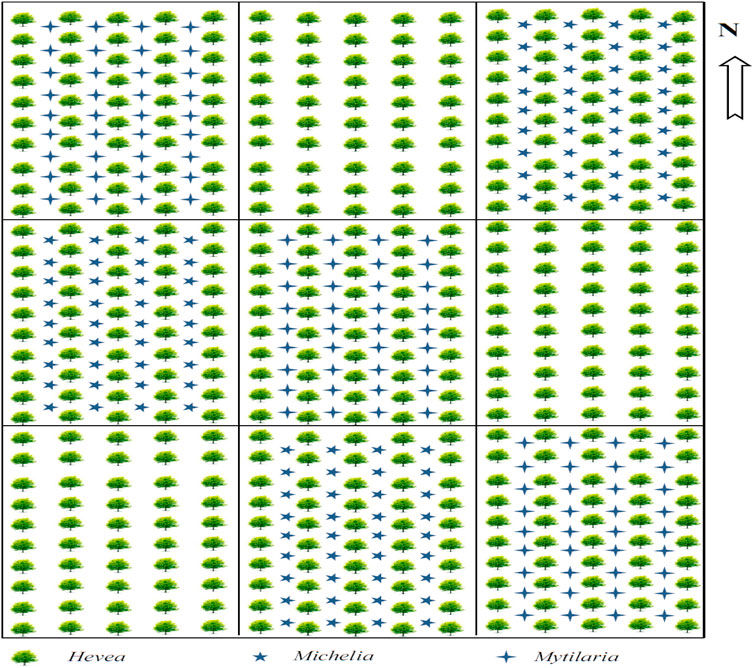
Figure 1. Treatment designs for the Hevea monoculture system, Hevea–Michelia intercropping system, and Hevea–Mytilaria intercropping system.
2.3 Sample collection and pretreatment
Five litter traps measuring 1.0 × 1.0 m were placed on the ground beneath the forest canopy to collect litterfall, with each treatment replicated three times. These traps were strategically positioned in various locations, including the middle of trees, gaps between overlapping crowns, and both the middle and edge of a tree crown. To account for the seasonal variations in litterfall, collections were conducted monthly or bimonthly throughout the year. Stem, leaves, fruits, flowers, and other tissues were collected separately and weighed after drying in an oven (DHG-9620A, Shanghai Yiheng Scientific Instruments Co., Ltd.) at 105°C. The TN content was determined using the Kjeldahl method. The TP content was determined using the molybdenum–antimony anti-colorimetric method, and the total potassium (TK) content was analyzed using a flame spectrophotometer.
2.4 Data processing and analysis
The data in this study were analyzed using Microsoft Excel 2007. We employed one-way analysis of variance (ANOVA) and Duncan least significant difference test to examine the differences between treatments concerning litterfall, nutrient content of litterfall, and meteorological factors. The significance level was set at p < 0.05.
3 Results
3.1 Litterfall
3.1.1 Total amount of litterfall
Intercropping altered the total amount of litterfall. Specifically, the total amount of litterfall for the Hevea monoculture system, Hevea–Michelia intercropping system, and Hevea–Mytilaria intercropping system was 22,364 kg ha−1, 27,309 kg ha−1, and 34,477 kg ha−1, respectively. A significant difference was observed in the total amount of litterfall among the three treatment types (F = 0.082, p = 0.921).
3.1.2 Monthly dynamics in litterfall
The monthly variation in litterfall in the Hevea monoculture system, Hevea–Michelia intercropping system, and Hevea–Mytilaria intercropping system exhibited a peak and sub-peak pattern, with the peak occurring in February to March, followed by June to July. Specifically, the peak and sub-peaks of the monthly change of litterfall in the Hevea monoculture system occurred in February–March and June, respectively, while the peaks and sub-peaks of the monthly variation in litterfall in the Hevea–Michelia intercropping system and Hevea–Mytilaria intercropping system occurred in February–March and July, respectively, and the above results indicate that intercropping delays the occurrence of the second largest peak of litterfall in the rubber intercropping system by 1 month. The monthly variation curves for the three types of litter were consistent (Figure 2). The maximum litterfall for the Hevea monoculture system, Hevea–Michelia intercropping system, and Hevea–Mytilaria intercropping system was observed in February and March, with minimum values occurring in different months: for the Hevea monoculture system and Hevea–Michelia intercropping system in September and for the Hevea–Mytilaria intercropping system in August. From May to November, the litterfall content decreased in the following order: Hevea–Mytilaria intercropping system > Hevea–Michelia intercropping system > and Hevea monoculture system. The litterfall in the Hevea–Mytilaria intercropping system was significantly higher than that in the Hevea–Michelia intercropping system and Hevea monoculture system, with litterfall in RAS1 being significantly higher than that in RM (p < 0.05). Litterfall in both RAS2 and RAS1 was significantly higher than that in RM, occurring in May, June, July, October, and November (p < 0.05). In December and January, there was no significant difference in litterfall between RAS1 and RM, while in February and March, RM litterfall was significantly higher than that of RAS1 and RAS2 (Figure 2).
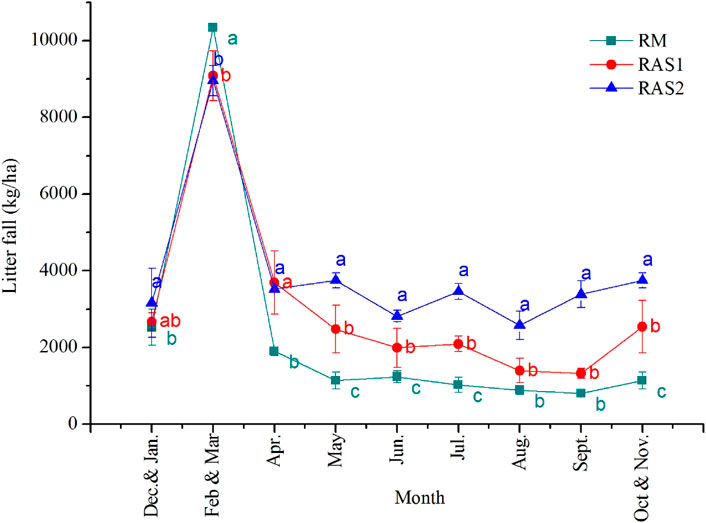
Figure 2. Monthly dynamics of total litterfall in the RM, RAS1, and RAS2. Note: RM, Hevea monoculture system; RAS1, Hevea–Michelia intercropping system; RAS2, Hevea–Mytilaria intercropping system. Note: significant difference at the level of p < 0.05.
3.1.3 Monthly dynamics of litter composition
Intercropping changed the monthly dynamics of litter composition. The leaf litterfall in the Hevea monoculture system, Hevea–Michelia intercropping system, and Hevea–Mytilaria intercropping system exhibited a unimodal distribution, peaking from February to March (Figure 3). From April to November, the leaf litter content in the Hevea–Mytilaria intercropping system surpassed that of the Hevea–Michelia intercropping system and Hevea monoculture system from December to January (Figure 3). Apart from May and February–March, the Hevea–Michelia intercropping system generally exhibited higher leaf litter content than the Hevea monoculture system. The proportion of leaf litter in the Hevea–Mytilaria intercropping system (88.25%) exceeded that of the Hevea–Michelia intercropping system (79.8%) and Hevea monoculture system (77.6%).
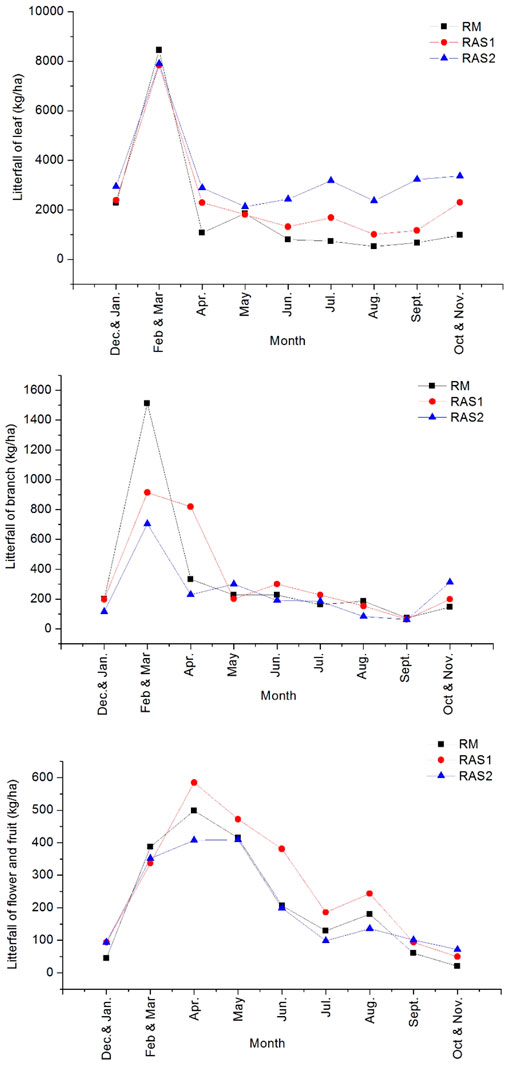
Figure 3. Monthly dynamics of leaf, branch, flower, and fruit litterfall in RM, RAS1, and RAS2. Note: RM, Hevea monoculture system; RAS1, Hevea–Michelia intercropping system; RAS2, Hevea–Mytilaria intercropping system.
The branch components of litterfall in the Hevea monoculture system, Hevea–Michelia intercropping system, and Hevea–Mytilaria intercropping system also followed a unimodal pattern, with the highest peak in February–March and the lowest in September (Figure 3). During June, July, and October–November, the branch litterfall in the Hevea–Michelia intercropping system exceeded that of the Hevea monoculture system, while for the remaining months, it was lower. The branch litterfall in the Hevea–Mytilaria intercropping system was higher than that in the Hevea monoculture system in May, July, and October–November. The proportion of branch litterfall in the Hevea–Mytilaria intercropping system (6.33%) was lower than that of the Hevea–Michelia intercropping system (11.27%) and the Hevea monoculture system (13.72%).
The litter components of flower and fruits in the Hevea monoculture system, Hevea–Michelia intercropping system, and Hevea–Mytilaria intercropping system exhibited a bimodal distribution, with peaks in April and August for the Hevea monoculture system and Hevea–Michelia intercropping system and in May and August for the Hevea–Mytilaria intercropping system, with the lowest values in October–November (Figure 3). From April to January, the Hevea–Michelia intercropping system generally had a higher amount of litterfall of flowers and fruits than the Hevea monoculture system. The litterfall of flowers and fruits in the Hevea–Mytilaria intercropping system exceeded that of the Hevea monoculture system in September, October–November, and December–January. The ratio of litterfall from flowers and fruits in the Hevea–Michelia intercropping system (8.94%) was higher than that in the Hevea monoculture system (8.68%) and Hevea–Mytilaria intercropping system (5.42%).
3.2 Litterfall nutrients
Intercropping changed the monthly dynamics and distribution patterns of litterfall nutrients. The monthly dynamics of litterfall nutrients in the Hevea monoculture system, Hevea–Michelia intercropping system, and Hevea–Mytilaria intercropping system followed a unimodal pattern, with a peak from February to March. However, there were differences in the timing of the lowest trough of litterfall nutrient content.
3.2.1 Total nitrogen
Intercropping influenced the TN content of litterfall (Figure 4). Compared with the Hevea monoculture system, the TN content in litterfall of the Hevea–Mytilaria intercropping system and Hevea–Michelia intercropping system increased by 185 kg ha−1 and 120 kg ha−1, respectively, representing a 103.70% and 67.88% increase for the whole year. The differences in TN between the Hevea–Mytilaria intercropping system and Hevea monoculture system, as well as the Hevea–Michelia intercropping system and Hevea monoculture system, reached a significant level from February to March, April, May, June, July, August, September, and October–November.
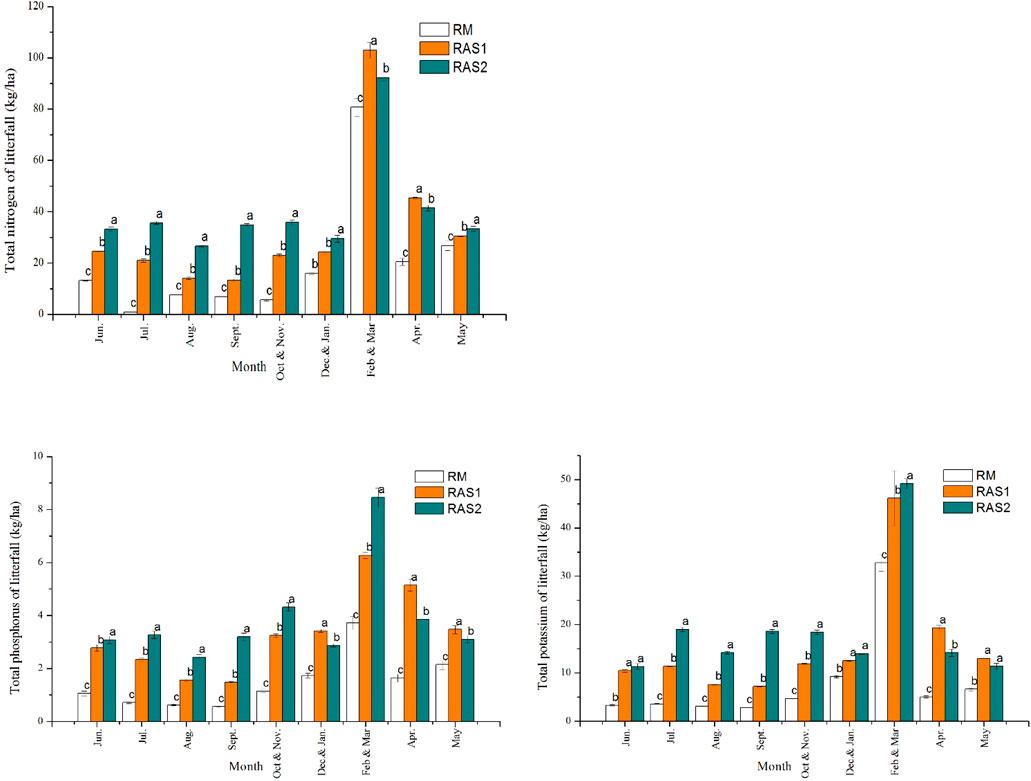
Figure 4. Litterfall nutrient content in RM, RAS1, and RAS2. Note: RM-Hevea monoculture system, RAS1-Hevea-Michelia intercropping system, RAS2-Hevea-Mytilaria intercropping system; Noted: Significant difference at the level of P<0.05.
3.2.2 Total phosphorus
Intercropping significantly altered the TP content of litterfall (Figure 4). The TP contents of the Hevea–Mytilaria intercropping system and Hevea–Michelia intercropping system litterfall were significantly higher than that of the Hevea monoculture system throughout the year. The TP content in litterfall of Hevea–Mytilaria intercropping systems increased by 35 kg ha−1, representing a 159.15% increase compared to the Hevea monoculture system for the whole year. The TP content in Hevea–Michelia intercropping system’s litterfall was significantly higher than that of the Hevea monoculture system, with an annual increase of 30 kg ha−1, representing a 122.79% increase compared to the Hevea monoculture system.
3.2.3 Total potassium
Intercropping impacted the TK content of litterfall (Figure 4). Compared with the Hevea monoculture system, the TK content of the Hevea–Mytilaria intercropping system and Hevea–Michelia intercropping system litterfall increased by 170 kg ha−1 and 139 kg ha−1, respectively, representing a 139.46% and 96.27% increase for the whole year. The differences between the Hevea–Mytilaria intercropping system and Hevea monoculture system, as well as the Hevea–Michelia intercropping system and Hevea monoculture system, reached a significant level in all months of the year. Additionally, the TN content of the Hevea–Mytilaria intercropping system litterfall was significantly higher than that of the Hevea–Michelia intercropping system in February–March, July, August, September, and October–November.
3.3 Association between litter components and nutrient content with meteorological factors
The association between monthly litter amount, leaf litter, branch litter, flower and fruit litter, as well as the TN, TP, and TK content of litter, with meteorological factors including monthly mean temperature, monthly maximum temperature, monthly minimum temperature, monthly mean wind speed, and monthly precipitation was analyzed by using linear regression model equations. The results of the analysis are presented in Table 1. Monthly litterfall was significantly affected by monthly average temperature, minimum temperature, and monthly average wind speed. It showed a negative correlation with monthly average temperature and a positive correlation with the minimum temperature and monthly average wind speed, explaining 80.5% of the variation in monthly litterfall (F = 22.5, p = 0.00, Table 1). Leaf litterfall was also significantly affected by these meteorological factors, accounting for 75.5% of the variation (F = 17.067, p = 0.00). Similarly, branch litterfall and flower and fruit litterfall were significantly influenced by these factors, explaining 69.8% and 69.6% of the variation (F = 13.045, p = 0.00; F = 12.906, p = 0.00), respectively. Furthermore, the TN, TP, and TK contents of litterfall were significantly impacted by monthly average temperature, minimum temperature, and monthly average wind speed. They exhibited a negative correlation with monthly average temperature and a positive correlation with the minimum temperature and monthly average wind speed. These variables explained 73.9%, 43%, and 66.66% of the variation in the nutrient content of litterfall, respectively (Table 1).
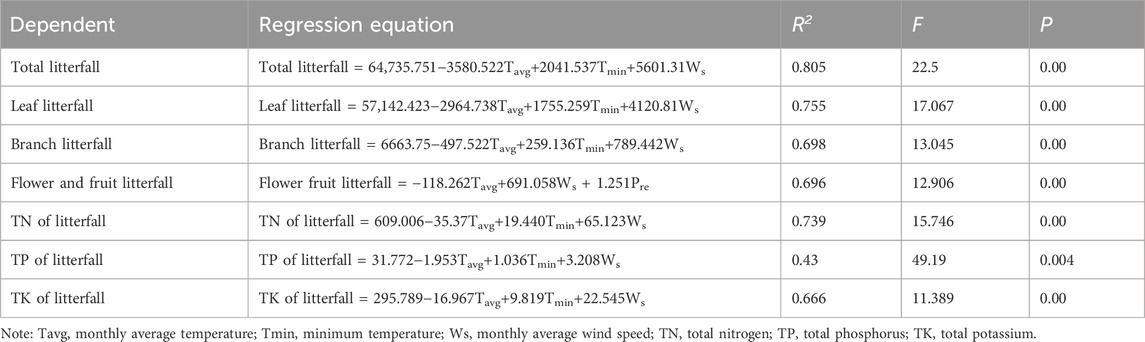
Table 1. Regression analysis between monthly litterfall and its main nutrient and meteorological factors by linear regression model equations.
4 Discussion
The litterfall of rubber plantations has been a topic concern and study since the 1990s (Yang ZX et al., 1997; Chaudhuri et al., 2003). However, few studies have examined litterfall and its main nutrient return dynamics in rubber plantations from the perspective of the rubber-based agroforestry system. In this study, we observed that the litterfall dynamics of the Hevea monoculture system, Hevea–Michelia intercropping system, and Hevea–Mytilaria intercropping system showed seasonal fluctuations, with a peak period of concentrated litterfall occurring from February to March. In the rubber planting areas of Yunnan Province, China, the annual variation in litterfall from rubber trees in the high-altitude terrain areas displayed a unimodal characteristic, peaking around January. Conversely, in middle- and low-altitude terrain areas, the annual volume of litterfall in rubber trees showed a bimodal characteristic, with litter concentrated from January to early February and again in September during the period of concentrated rubber seed production (Ren et al., 1999; Jia, 2006; Zhou et al., 2017). Additionally, in middle-aged and old rubber plantations in the Xishuangbanna area of Yunnan Province, the annual variation in the litterfall volume exhibited bimodal characteristics (Zhou et al., 2017). The presence of two peaks in the annual variation in the litter volume in the middle- and low-altitude areas of Yunnan Province may be attributed to the abundant and efficient light and heat resources in the unique elevation and terrain, the prolific flowering and fruiting of rubber trees, and the abundance of withered fruits during ripening of rubber fruits.
In this study, leaf litter comprised the largest proportion of litterfall, with leaf litter and litter branches primarily determining the overall litterfall pattern across the three systems. Specifically, the litterfall in the Hevea monoculture system, Hevea–Michelia intercropping system, and Hevea–Mytilaria intercropping system accounted for 77.6%, 79.80%, and 88.25% of the total litterfall, respectively. Furthermore, the branch litterfall in these systems represented 13.72%, 11.27%, and 6.33% of the total litterfall, respectively. Some studies conducted in rubber plantations in Guangdong Province, China, also reported the litter composition. These studies revealed that rubber leaves, branches, and flowers and fruits constituted 91.2%, 7.3%, and 1.5% of the litter, respectively, with the total litter pattern being predominantly characterized by leaf and branch litterfall (Yang ZJ et al., 1997). Similarly, research on middle-aged rubber plantations in the Xishuangbanna area of Yunnan Province in China indicated that the proportion of leaves, branches, and flowers and fruits in the litter was 73.8%, 22.06%, and 5.5% of the total litter, respectively, with litter leaves and branches also dominating the total litterfall pattern (Zhou et al., 2017). These findings align with conclusions drawn from other studies on litterfall in tropical forests (Wan et al., 2015). Ren et al. (1999) conducted a study on the litter composition of rubber multilayer plantations comprising old rubber trees, Baccaurea ramiflora and Rauvolfia vomitoria, which had been growing for over 30 years. The total litterfall pattern in this context was dominated by litter leaves and littered flowers and fruits, which accounted for 52.48% and 33.09%, respectively. This outcome may be attributed to the actual plant composition and the age of tree multilayer plantations.
In this study, the total annual litterfall of the Hevea–Michelia intercropping system (27,309 kg ha−1) and Hevea–Mytilaria intercropping system (34,477 kg ha−1) was significantly higher than that of the Hevea monoculture system (22,364 kg ha−1). Specifically, the annual litter content of the Hevea–Michelia intercropping system and Hevea–Mytilaria intercropping system was 1.22 times and 1.54 times that of the Hevea monoculture system, respectively. Furthermore, the annual litter content of the rubber mixed cropping model exceeded that of the southern subtropical broad-leaved forest in China (ranging from 7,000 kg ha-1 to 11000 kg ha−1) and the mountainous tropical rainforest of Hainan (ranging from 7,700 kg ha-1 to 9,700 kg ha−1), as well as that of the tropical rainforest in Malaysia in Southeast Asia (ranging from 7,500 kg ha−1 to 10,200 kg ha−1) (Lim, 1978; Lu and Liu, 1988; Tu et al., 1993; Yang ZX et al., 1997). Comparatively, the annual litter content of rubber monocropping (7,510 kg ha−1) was higher than that of rubber cocoa hybrid (6,810 kg ha−1) and rubber large-leaf jack pulling, although no significant difference was observed between the above monocropping and mixed species (Lu et al., 2021). This comparative analysis of annual litterfall highlights that the annual litterfall volume in the rubber Michelia/Mytilaria intercropping system not only surpasses that of the rubber Amomum villosum intercropping pattern but also exceeds the annual litterfall volume of the southern subtropical broad-leaved forest in China, the mountainous tropical rainforest in Hainan, and the tropical rainforest of Malaysia in Southeast Asia (Lim, 1978; Lu and Liu, 1988; Tu et al., 1993; Yang ZJ et al., 1997).
Rubber intercropping with native trees significantly changed the return of main litter nutrients. Specifically, compared to the Hevea monoculture system, the TN content of litterfall increased by 185 kg ha−1 and 120 kg ha−1 in the Hevea–Mytilaria and Hevea–Michelia intercropping systems, respectively, representing a 103.70% and 67.88% increase for the whole year. Similarly, the TP content in litterfall in the Hevea–Mytilaria intercropping systems and Hevea–Michelia intercropping system increased by 35 kg ha−1 and 30 kg ha−1, respectively, indicating a 159.15% and 122.79% increase for the whole year. Additionally, the TK content litterfall in the Hevea–Mytilaria intercropping system and Hevea–Michelia intercropping system litterfall increased by 170 kg ha−1 and 139 kg ha−1, respectively, representing a 139.46% and 96.27% increase for the whole year. In terms of the nutrient content of litter, TN was the highest, followed by TK, and the lowest was TP. Regarding the nutrient content of litter, TN exhibited the highest levels, followed by TK, with TP being the lowest. Concerning the annual growth rate of main litter nutrient return, the TP content of litter showed the most significant increase in the intercropping system, followed by TK and TN, respectively. Additionally, the nutrient content of litter in the three systems displayed a seasonal trend, particularly in February and March during spring. Some scholars have also observed dynamic monthly trends in the N, P, and K contents of leaf litter in young and old rubber plantations in Hainan, noting a decrease in these elements during autumn and winter, with enrichment occurring in winter and spring (Miao et al., 2012; Xue et al., 2022). The above-mentioned research shows that the intercropping production practice of rubber plantations has significantly increased the amount of litter on the rubber plantation surface. We believe that these abundant litters are expected to significantly improve the soil and water conservation capacity of the surface soil, thereby optimizing the surface ecological environment. More importantly, these returned rubber plantations have enriched litter nutrients, which can offset the input of artificial fertilizer to the rubber plantation to a certain extent, which is equivalent to reducing labor input and fertilizer input.
Some scholars tend to focus on the effects of rubber tree age on litter and nutrient return, while paying little attention to the influence of meteorological factors on litter and nutrient return in rubber plantations. The litterfall yield in rubber plantations in Hainan showed a “double-peak” dynamic change (Miao et al., 2012; Xue et al., 2022). In this study, we selected the monthly mean temperature, monthly maximum temperature, monthly minimum temperature, monthly average wind speed, monthly rainfall, and other meteorological and environmental factors to analyze their association with the litter and nutrient content of rubber plantations. We found that litterfall was significantly correlated with monthly average temperature, monthly minimum temperature, and monthly average wind speed, contributing 80.5%, 75.5%, 69.8%, and 69.6% to the total litterfall and its leaf, branch, and flower and fruit components, respectively. Moreover, the contribution rates of monthly average temperature, monthly minimum temperature, and monthly average wind speed factors to TN and TK content of the annual litterfall were 73.9% and 66.6%, respectively, indicating the predominant role of temperature and wind speed. Interestingly, the changes in litter composition and nutrient content in the three patterns, including the Hevea monoculture system, Hevea–Michelia intercropping system, and Hevea–Mytilaria intercropping system, were not significantly affected by monthly maximum temperature and precipitation. Previous studies have also indicated that average monthly temperature is the primary climatic factor influencing the leaf litter pattern in rubber plantations (Cao et al., 2011). Similar conclusions have been drawn in other forest types, where temperature and extreme wind speed profoundly affected litter yield and different components in monsoon evergreen broad-leaved forests and mixed coniferous and broad-leaved forests (Liu et al., 2024).
5 Conclusion
Based on the findings regarding the effect of intercropping rubber with native tree species on litterfall, our conclusions are summarized as follows:
(1) Rubber intercropping with native tree species significantly enhances litter production in rubber plantations. The increase is expected to effectively cover the rubber plantation surface, supplement the deficit in soil organic matter, and mitigate or reverse the soil degradation trend resulting from long-term rubber plantation operations.
(2) Litterfall, peaking from February to March during the dry season, is predominantly composed of leaf litter in the three intercropping systems: the Hevea monoculture system, Hevea–Michelia intercropping system, and Hevea–Mytilaria intercropping system. Strengthening the management and utilization of leaf litterfall in intercropping systems, particularly during the dry season, is crucial for maximizing the role of litterfall.
(3) Rubber intercropping with native tree species leads to a significant increase in the TN, TP, and TK contents of annual litterfall. This suggests that the intercropping pattern effectively enhances the nutrient content of rubber plantation litter in the field.
(4) Meteorological factors, including temperature and wind speed, exhibit strong correlations with total litterfall, its components, and the main nutrients of litter. This highlights the importance of considering these meteorological factors in the utilization and management of litter in rubber intercropping patterns. Managing these factors accordingly is essential for optimizing litter utilization and management in rubber intercropping systems.
Data availability statement
The original contributions presented in the study are included in the article/Supplementary Material; further inquiries can be directed to the corresponding authors.
Author contributions
DQ: funding acquisition, investigation, writing–original draft, and writing–review and editing. ZW: conceptualization, funding acquisition, resources, and writing–review and editing. RS: resources and writing–review and editing. CY: investigation and writing–review and editing. XZ: investigation and writing–review and editing. SL: resources and writing–review and editing. XC: resources and writing–review and editing.
Funding
The author(s) declare that financial support was received for the research, authorship, and/or publication of this article. The authors gratefully acknowledge financial support from Hainan Province Key R and D Program of China (ZDYF2024XDNY173), Hainan Provincial Natural Science Foundation of China (319MS090), China Agriculture Research System (CARS-33-ZP3).
Acknowledgments
We thank the reviewers and editors for their work in analyzing our study.
Conflict of interest
The authors declare that the research was conducted in the absence of any commercial or financial relationships that could be construed as a potential conflict of interest.
Publisher’s note
All claims expressed in this article are solely those of the authors and do not necessarily represent those of their affiliated organizations, or those of the publisher, the editors, and the reviewers. Any product that may be evaluated in this article, or claim that may be made by its manufacturer, is not guaranteed or endorsed by the publisher.
References
Arisara, R., Palakorn, S., and Somboon, C. (2018). Investigation of rubber-based intercropping system in Southern Thailand. Kasetsart J. Soc. Sci. 39, 135–142. doi:10.1016/j.kjss.2017.12.002
Cao, J. H., Tao, Z. L., Zhao, C. M., Jiang, J. S., and Xie, G. S. (2011). Nutrient return in fallitter of Hevea brasisiliensis at different age. Chin. J. Trop. Crops 32 (1), 1–6. doi:10.3969/j.issn.1000-2561.2011.01.001
Chaudhuri, P. S., Pal, T. K., Bhattacharjee, G., and Dey, S. K. (2003). Rubber leaf litters (Hevea brasiliensis, var RRIM 600) as vermiculture substrate for epigeic earthworms, -Perionyx excavatus, Eudrilus eugeniae and Eisenia fetida: the 7th international symposium on earthworm ecology Cardiff Wales 2002. Pedobiologia 47 (5–6), 796–800. doi:10.1016/s0031-4056(04)70270-x
Ehrenbergerová, L., Cienciala, E., Kučera, A., Guy, L., and Habrová, H. (2016). Carbon stock in agroforestry coffee plantations with different shade trees in Villa Rica, Peru. Agrofor. Syst. 90 (3), 433–445. doi:10.1007/s10457-015-9865-z
Jia, K. X. (2006) “Study on growth of clover rubber forest with altitude in Xishuangbanna[D],” in Xishuangbanna tropical botanical garden. China: Chinese Academy of Sciences.
Jiang, X. J., Liu, W. J., Wu, J. E., Wang, P. Y., Liu, C. G., and Yuan, Z. Q. (2017). Land degradation controlled and mitigated by rubber-based agroforestry systems through optimizing soil physical conditions and water supply mechanisms: a Case Study in Xishuangbanna, China. Land Degrad. Dev. 28 (7), 2277–2289. doi:10.1002/ldr.2757
Krishna, M. P., and Mohan, M. (2017). Litter decomposition in forest ecosystems: a review. Energy, Ecol. Environ. 2 (4), 236–249. doi:10.1007/s40974-017-0064-9
Lim, M. T. (1978). Litterfall and mineral content of litter in Pasoh forest. Malay. Nat. J. 30, 376–378.
Liu, C., and Sun, X. (2013). A review of ecological stoichiometry: basic knowledge and advances. Reference Module Earth Syst. Environ. Sci. doi:10.1016/b978-0-12-409548-9.05519-6
Liu, J. Q., Liu, W. J., Li, W. X., Jiang, X. J., and Wu, J. E. (2018). Effects of rainfall on the spatial distribution of the throughfall kinetic energy on a small scale in a rubber plantation. Hydrol. Sci. J. 63 (7), 1078–1090. doi:10.1080/02626667.2018.1473580
Liu, S. R., Yang, Y. J., and Wan, H. (2018). Development strategy and management countermeasures of planted forests in China: transforming from timber-centered single objective management towards multi-purpose management for enhancing quality and benefits of ecosystem services. Acta Ecol. Sin. 38 (1), 1–10. doi:10.5846/stxb201712072201
Liu, W. J., Luo, Q. P., Li, J. T., Wang, P. Y., Lu, H. J., Liu, W. Y., et al. (2015). The effects of conversion of tropical rainforest to rubber plantation on splash erosion in Xishuangbanna, SW China. Hydrol. Res. 46, 168–174. doi:10.2166/nh.2013.109
Liu, W. J., Luo, Q. P., Lu, H. J., Wu, J. N., and Duan, W. P. (2017). The effect of litter layer on controlling surface runoff and erosion in rubber plantations on tropical mountain slopes, SW China. CATENA 149 (1), 167–175. doi:10.1016/j.catena.2016.09.013
Liu, X. D., Feng, Y. J., Zhao, X. Y., Cui, Z. J., Liu, P. L., Chen, X. Z., et al. (2024). Climatic drivers of litterfall production and its components in two subtropical forests in South China: a 14-year observation. Agric. For. Meteorology 344, 109798. doi:10.1016/j.agrformet.2023.109798
Liu, Z. W. (2014). Soil nutrient status and rational fertilization in Hainan reclamation area. Hainan Agr Reclam. Technol. (5), 12–18.
Lu, E. F., Zhu, X. A., Zeng, H. H., and Liu, W. J. (2021). Litter and its hydrological characteristics of typical forest types in Xishuangbanna. Chin. J. Ecol. 40 (7), 2104–2112. doi:10.13292/j.1000-4890.202107.017
Lu, J. P., and Liu, Q. H. (1988). Litterfall in tropical forest at jianfengling mountains,hainan island. Acta Phytoecol. Geobotanica Sinica 12 (2), 104–111.
Miao, X. L., Jiang, J. S., Wang, C. Y., Peng, Z. B., Li, J. H., and Cui, Z. F. (2012). Comparison of annual returned carbon content of litters and annual carbon emission of the rubber trees of different ages. Ecol. Sci. 31 (6), 625–630. doi:10.3969/j.issn.1008-8873.2012.06.005
Piscart, C., Navel, S., Maazouzi, C., Montuelle, B., Cornut, J., Mermillod-Blondin, F., et al. (2011). Leaf litter recycling in benthic and hyporheic layers in agricultural streams with different types of land use. Sci. Total Environ. 409 (20), 4373–4380. doi:10.1016/j.scitotenv.2011.06.060
Qi, D. L., Wu, Z. X., Yang, C., Xie, G. S., Li, Z. M., Yang, X. B., Li, D. H., et al. (2021). Can intercropping with native trees enhance structural stability in young rubber (Hevea brasiliensis) agroforestry system? Eur. J. Agron. 130, 126353. doi:10.1016/j.eja.2021.126353
Ren, Y. H., Cao, M., Tang, J. W., Tang, Y., and Zhang, J. H. (1999). A comparative study on litterfall dynamics in a seasonal rain forest and a rubber plantation in Xishuangbanna, SW China. Chin. J. Plant Ecol. 23 (5), 418–425. doi:10.3321/j.issn:1005-264X.1999.05.005
Schlesinger, W. H. (1997). Biogeochemistry: an analysis of global change. Q. Rev. Biol. 54 (4), 353–423. doi:10.1016/C2012-0-01654-7
Singh, A. K., Liu, W., Zakari, S., Wu, J., Nath, J., Jiang, X. J., et al. (2021). A global review of rubber plantations: impacts on ecosystem functions, mitigations, future directions, and policies for sustainable cultivation. Sci. Total Environ. 796, 148948. doi:10.1016/j.scitotenv.2021.148948
Sun, H. D., Liu, B., Wu, B. S., Wei, J. S., He, P., Gao, L., et al. (2016). Characteristics and causes of soil acidification in the rubber plantation. J. Northwest For. Univ. 31 (2), 49–54. doi:10.3969/j.issn.1001-7461.2016.02.09
Tang, C., Yang, M., Luo, Y., Gao, S., Xiao, X., An, Z., et al. (2016). The rubber tree genome reveals new insights into rubber production and species adaptation. Nat. Plants 2, 16073. doi:10.1038/nplants.2016.73
Tu, M. Z., Yao, W. H., Weng, H., and Li, Z. A. (1993). Characteristics of litter in evergreen broadleaved forest of the dinghu mountain. Acta Pedol. Sin. 30 (1), 34–42.
Wan, C. H., Tao, C., Yang, X. B., Long, W. X., Feng, D. D., Zhou, W. S., et al. (2015). Impact of forest community species composition on litter species composition. Acta Ecol. Sin. 35 (22), 7435–7443. doi:10.5846/stxb201404160742
Wang, J., Liu, L. L., Wang, X., and Chen, Y. W. (2015). The interaction between abiotic photodegradation and microbial decomposition under ultraviolet radiation. Glob. Change Biol. 21 (5), 2095–2104. doi:10.1111/gcb.12812
Waring, R. H., and Schlesinger, W. H. (1985). Forest ecosystems, concepts and management. Clin. Exp. Allergy 75 (75), 284.
Weerasekara, C., Udawatta, R. P., Jose, S., and Kremer, R. J. (2016). Soil quality differences in a row-crop watershed with agroforestry and grass buffers. Agrofor. Syst. 90 (5), 829–838. doi:10.1007/s10457-016-9903-5
Wu, J., Liu, W., and Chen, C. (2016). Can intercropping with the world’s three major beverage plants help improve the water use of rubber trees? J. Appl. Ecol. 53 (6), 1787–1799. doi:10.1111/1365-2664.12730
Xue, X. X., Ren, C. Q., Xu, Z. W., Wang, W. B., Zhang, Y. F., Luo, X. H., et al. (2022). Characteristic of defoliation of rubber plantations (Hevea brasiliensis) in Hainan, China. Chin. J. Trop. Crops 43 (2), 377–384. doi:10.3969/j.issn.1000-2561.2022.02.019
Yang, Z. J., Zheng, H. S., Zhou, Z. Z., and Yin, G. T. (1997). Litter characteristic of Hevea brasiliensis plantation intercropped with Amomum villosum. Guangdong For. Sci. Technol. 13 (4), 19–24.
Yang, Z. X., Zheng, H. H., Zhou, Z. Z., and Yin, G. T. (1997). Characteristics of litter in rubber interplanting mode. Guangdong For. Sci. Technol. (4), 19–24.
Zhou, H. P., Wei, L. P., Xie, J., Chen, G. Y., and Xu, R. (2017). Litter dynamics of rubber plantation and macadamia plantation in Xishuangbanna. J. Anhui Agric. Univ. 44 (3), 422–428. doi:10.13610/j.cnki.1672-352x.20170524.029
Keywords: Hevea brasiliensis, rubber-based agroforestry system, litterfall, climatic factor, intercrop
Citation: Qi D, Wu Z, Sun R, Yang C, Zhang X, Li S and Che X (2024) Effects of rubber intercropping with native trees on litterfall and litter main nutrient return in Hainan Island, China. Front. Environ. Sci. 12:1407821. doi: 10.3389/fenvs.2024.1407821
Received: 27 March 2024; Accepted: 05 June 2024;
Published: 02 July 2024.
Edited by:
Mukesh K. Gautam, The City University of New York, United StatesReviewed by:
Monika Rawat, Auburn, United StatesAbhishek Kumar, Forest Research Institute (FRI), India
Copyright © 2024 Qi, Wu, Sun, Yang, Zhang, Li and Che. This is an open-access article distributed under the terms of the Creative Commons Attribution License (CC BY). The use, distribution or reproduction in other forums is permitted, provided the original author(s) and the copyright owner(s) are credited and that the original publication in this journal is cited, in accordance with accepted academic practice. No use, distribution or reproduction is permitted which does not comply with these terms.
*Correspondence: Zhixiang Wu, emhpeGlhbmd3dUBjYXRhcy5jbg==; Dongling Qi, ZG9uZ2xpbmdxaUAxNjMuY29t