- 1Shaanxi Provincial Land Engineering Construction Group Co., Ltd., Xi’an, China
- 2Institute of Land Engineering and Technology, Shaanxi Provincial Land Engineering Construction Group Co., Ltd., Xi’an, China
- 3Technology Innovation Center for Land Engineering and Human Settlements, Shaanxi Land Engineering Construction Group Co., Ltd., Xi’an Jiaotong University, Xi’an, China
- 4Land Engineering Technology Innovation Center, Ministry of Natural Resources, Xi’an, China
In order to accurately understand the relationship between soil structure and climate feedback in the frozen soil area of Mu Us Sandy Land, China, and to explore the key control factors for the structural stability of soft rock and sand compound soil under freeze-thaw environment, the indoor freeze-thaw simulation experiment was applied. The results show that the freeze-thaw period, clay content, organic matter and their interactions have significant effects on the stability of composite soil aggregates. After 10 freeze-thaw cycles, the aggregate content in the 1:0, 1:1, 1:2, and 1:5 composite soil with a diameter greater than 1 mm decreased by 55%, 34%, 44%, and 57%, while the aggregate content with a diameter less than 1 mm increased by 91%, 70%, 66%, and 87%, and the aggregate composition of each particle size is mainly concentrated in the range of 0.25–0.5 mm. Under freeze-thaw conditions, the changes of clay and aggregate content in different proportions of composite soil is the same, all showing 1:1>1:2:>1:5, and 1:1 composite soil with >0.25 mm aggregate content is the highest. Under freeze-thaw alternations, 1:1, 1:2 and 1:5 composite soil aggregates (<0.5 mm) showed a significant positive correlation with soil organic matter, while there is no significant correlation between large aggregates (>1 mm) and soil organic matter. In conclusion, the freeze-thaw cycle reduces the structural stability of composite soil aggregates, and clay are the key controlling factors for the formation and structural stability of composite soil aggregates.
1 Introduction
For a long time, implementing comprehensive management of sandy land has been a burning topic of social concern and a difficult problem that needs to be addressed. The Mu Us Desert is one of the four major sandy lands, located in the interlace zone of agriculture, grazing and grassland in China. It is a typical ecologically fragile area and one of the most serious desertification areas in northern China. In the Mu Us Desert, a large number of soft rock rich in clay minerals are interdistributed with aeolian sand, among which, soft rock with abundant reserves is distributed in 1.67 × 106 ha area in the Mu Us Sandy Land of 3.98 × 106 ha (Wang et al., 2014; Yang et al., 2017). Soft rock has low diagenetic degree and low structural strength. When it encounters wind, it becomes sand and when it encounters water, it becomes mud, and soil erosion is very serious, which is called “earth ecological cancer” by Chinese and foreign scholars (She et al., 2014; Guo et al., 2023a). In order to improve the environment, Han et al. (Han et al., 2012; Han et al., 2015) added soft rock rich in clay minerals to improve the sandy land, which increased the clay content in the sandy soil, provided the requirements of loam particle foundation and cementation mechanics, thus altering the soil structure of the sandy land, realizing the quality improvement of the sandy soil and the virtuous cycle of the ecosystem (Guo Z. et al., 2020), and increasing productivity (Wang et al., 2017; Sun and Han, 2018). However, as a new type of composite soil, its adaptability to regional climate and natural environment during long-term utilization is also a scientific issue that needs further attention.
The freeze-thaw alternation process is a physical-geological process and natural phenomenon that occurs in the middle to high latitudes and high altitudes, and it refers to the freezing and thawing of the soil layer due to the change of temperature around 0°C (Fan et al., 2012). The Yuyang District of Yulin City in northern Shaanxi Province is a typical mid-temperate semi-arid continental monsoon climate area. The temperature enters below 0°C in November every year, and gradually recovers to above 0°C in March of the following year, with a freezing period of 4–5 months. In late autumn and early spring, due to the significant diurnal temperature changes, the soil is in a daily freeze-thaw cycle and thawing state. Freezing-thawing alternation is an abiotic stress acting on the soil, which has direct or indirect effects on the physical, chemical and biological properties of the soil, especially on the instability of the soil, which can directly affect the stability of soil structure and change the composition of soil aggregates (Edwards, 2010; Gao et al., 2016). Micro-aggregates of different particle sizes play different roles in the retention, supply and transformation of nutrient elements (Chang et al., 2014). The Mu Us Sandy Land is affected by the temperate cool climate and the seasonal frozen layer, and the freeze-thaw alternation has a significant effect on the soil structure.
As the basic unit of soil structure, aggregate affects the level of soil organic matter, biological activity and function (Du et al., 2014), which is the material basis of soil fertility and one of the necessary conditions for high crop yield, it is also an important index reflecting soil structure and stability (Zhang et al., 2022). The influence of freeze-thaw alternations on the composition and stability of soil aggregates will directly or indirectly affect the physical structure and chemical environment of soil, as well as the growth, reproduction and activities of large soil animals and soil microorganisms, thus affecting the water and nutrient retention capacity of soil (Wang et al., 2011; Guo Z. Y. et al., 2020). Cemented substances is the basis for the formation of soil aggregates, and its mass fraction, spatial variation and distribution, composition characteristics and mode of action are the material basis and internal driving force for the formation and stability of soil aggregates (Lehmann et al., 2020). Soil clay and organic matter content are important cementing substances for the formation of soil aggregates, which determine the formation of soil water-stable aggregates (>0.25 mm), improve the soil moisture environment, enhance the stability of aggregates, and reduce soil erosion (Xu et al., 2013). There are many studies on the effect of freeze-thaw alternation on the stability of soil aggregates at home and abroad, but the results are different. Some studies have pointed out that freeze-thaw alternation reduces the stability of soil aggregates (Henry, 2007; Sahin et al., 2008; Wen et al., 2013), while others suggest that it increases the stability of soil aggregate structure (Bechmann et al., 2005; Hao et al., 2007). The quantity, particle size distribution and stability of soil aggregates are important indicators for the good development of soil structure. Generally, water-stable aggregates with a particle size of >0.25 mm are regarded as the optimal structures in soil (Wang Y. et al., 2013). Therefore, the higher the aggregate content of >0.25 mm particle size in freeze-thaw environment, the higher the soil stability and the better the soil structure.
Under the background of climate warming, the influence of freeze-thaw action on soil structure and carbon pool stability in permafrost region is currently a burning research topic internationally (Schuur et al., 2015; Haddix et al., 2020). However, previous studies on freeze-thaw processes mainly focused on alpine forest soil (Tan et al., 2011; Guo et al., 2017) and wetland soil (Zhou et al., 2011), but there was no report on cultivated soil, especially the effects of freeze-thaw processes on soil structure of soft rock and sand compound in Mu Us Sandy Land, China. This study takes soft rock and sand compound soil in Mu Us Sandy Land, which are significantly affected by seasonal freeze-thaw action, as the research object. Through laboratory simulated freeze-thaw experiments, the influences of freeze-thaw cycle, clay, organic matter content and interaction between them on aggregate structure of soft rock and sand compound soil with different proportions are studied, and the key controlling factors of structural stability of composite soil under extreme climate environment are revealed. The research results are of great significance to accurately understand the relationship between soil structure and climate feedback in permafrost regions.
2 Materials and methods
2.1 Experimental materials
The research area is located in Xiaojihan Township, Yuyang District, Yulin City, Shaanxi Province, China (E109°28′58″–109°30′10″, N38°27′53″–38°28′23″), with an altitude of 1,206–1,215 m, on the southern edge of the Mu Us Desert. This region is a typical mid-temperate arid and semi-arid continental monsoon climate zone, with an average annual rainfall of 200–600 mm and uneven distribution of precipitation in time and space. The maximum annual extreme rainfall is 695.4 mm and the minimum is 159.6 mm. 60.9% of the rainfall is concentrated in 3 months from July to September, with the same period of rainfall and heat, the planting system of grain crops is one crop per year. The annual sunshine time range from 2,500 to 3,000 h, and the percentage of sunshine is as high as 70%–80%. The average annual temperature difference is 30°C–50°C, the annual active accumulated temperature of ≥10°C ranges from 3,000°C to 5,000°C, the frost-free period is 120–130 days throughout the year, and the average temperature in January is −12 to −9.5°C. Every year, the temperature enters below 0°C in November, and gradually returns to above 0°C in March of the next year, with a freezing period of 4–5 months, and the soil is in freeze-thaw cycle and thawing state during the late autumn and early spring seasons.
The materials used in the experiment are soft rock and aeolian sand, which are interphase distributed in Mu Us Sandy Land. Aeolian sand has poor structure, uniform texture, dispersed particles without agglomeration, and poor water retention. Soft rock is rich in secondary clay minerals, which can promote soil agglomeration and has good water holding capacity. The comparison of basic physical and chemical properties between aeolian sandy soil and composite soil is shown in Table 1.
2.2 Experimental design
The experiment was conducted from October 2022 to March 2023, the experimental soil samples were taken from Xiaojihan Township, Yuyang District, Yulin City, Shaanxi Province, China. The local purplish red soft rock and aeolian sand soil were collected, and the grass roots and other debris were removed. After natural air drying, soft rock and aeolian sand are fully mixed according to four different mass ratios of 1:0 (T0), 1:1 (T1), 1:2 (T2), 1:5 (T3) for reserve use. According to long-term field experiments, the optimal combination scheme of soft rock and sand composite soil is 1:1, 1:2, and 1:5, which are suitable for planting local main crops such as corn and potatoes in the study area (Han et al., 2012; Han et al., 2015; Wang et al., 2017; Sun and Han, 2018; Guo Z. et al., 2020).
Four different proportions of composite soil were used as the culture medium, and five samples of each ratio composite soil were prepared, each 500 g soil sample was put into 20 circular aluminum boxes. In order to make the indoor freeze-thaw conditions closer to the natural environmental state, that is, the temperature fluctuation should start from the surface soil as much as possible, the asbestos mesh should be wrapped outside the aluminum boxes to achieve good insulation effect. Soil samples with 1:0 ratio are recorded as M0, M1, M2, M5, M10; 1:1 ratio are recorded as A0, A1, A2, A5, A10; 1:2 ratio are recorded as S0, S1, S2, S5, S10; and 1:5 ratio are recorded as H0, H1, H2, H5, H10. M0, A0, S0 and H0 were control soil samples, which were not freeze-thaw treated. In order to simulate the actual situation of soil freeze-thaw, the moisture correction was carried out the samples according to the soil moisture content during the spring snowmelt period. According to the investigation and analysis, the moisture content of the 1:0, 1:1, 1:2, and 1:5 composite soil during the spring snowmelt period is 17.32%, 11.72%, 9.92%, and 7.65%. During the experiment, the lost moisture is continuously replenished by weighing method to maintain the corresponding moisture conditions of the experimental soil samples. Each group of soil samples is first placed at room temperature 20°C for 24 h, then completely frozen them in the freezer at −15°C for 72 h, and melt them at room temperature for 24 h, which is a freeze-thaw cycle. The five soil samples of each proportion were set up for 3 repetitions to determine the content of soil aggregates and organic matter in 0, 1, 2, 5 and 10 freeze-thaw cycles.
2.3 Sample measurement methods
The soil aggregate content was determined by dry sieve method, and four particle size groups of > 1 mm, 1–0.5 mm, 0.5–0.25 mm, and <0.25 mm were separated, and the particle aggregate size of each group was weighed, and then the soil aggregate content of different particle sizes was calculated. The organic matter content was determined using the potassium dichromate volumetric-external heating method, and the organic matter was 1.724 times the soil organic carbon content (Jiang et al., 2018). The soil particle composition was determined using a laser particle size analyzer Mastersizer 2000 (Malvern Panalytical, Malvern, United Kingdom), and the soil texture types were determined using the soil texture automatic classification system (STAC) (Haddix et al., 2020).
2.4 Data processing
Using Excel 2010, SigmaPlot 12, and Origin 7.5 tools for multivariate data analysis and validation. The significance of the differences in the experimental results was analyzed using SPSS 13.0 statistical analysis software for t-test, and Duncan’s new multiple range method was used for comparative analysis. Different letters indicated significant differences at the 0.05 level.
3 Results
3.1 Particle composition of soft rock and sand compound soil with different proportions
The particle composition and texture category of soft rock and sand compound soil with different mixing ratios of 0:1, 1:5, 1:2, 1:1, and 1:0 are different (Table 2). When the mixing ratio of soft rock and sand is 0:1, it is the original aeolian sand soil with sand content of 94.07%, which plays a dominant role in the texture category. The silt content in the aeolian sand soil is 3.20%, and the soil texture category is sandy soil, there is no significant difference in sand content of 1:2 and 1:5 compound soil (p > 0.05). When the mixing ratio of soft rock and sand is 1:0, the silt content was reported highest, up to 72.94%, which is 22.8 times of the silt content in the whole sand, and the sand content is 19.57%, which is only 1/5 of the whole sand. There are significant differences in the silt content in the compound soil (p < 0.05). The clay content of aeolian sand soil and soft rock is relatively low, only 2.73% in aeolian sand and 7.49% in soft rock. After mixing the two, the clay content increased from 2.73% to 8.24% with the increase of soft rock content, and the clay content of 1:1 compound soil was the highest, while the clay content of 1:2 and 1:5 compound soil had no significant difference (p > 0.05).
The aeolian sandy soil was improved and repaired by soft rock, the content of silt and clay gradually increases, while the content of sand particles gradually decreases. Compared with the original aeolian sand soil, the maximum increase of silt and clay content in the compound soil is 13.04 times and 2.02 times. The soil texture changed from sandy soil to loam and silty loam, and the texture category was significantly improved.
3.2 Effect of freeze-thaw cycles on composite soil aggregates
The freeze-thaw cycle has a certain dispersing effect on compound soil aggregates with different proportions. The freeze-thaw cycle aggravates the damage to the structure of larger aggregates (>1 mm), increases the proportion of other small and medium-sized aggregates (<0.5 mm), and the overall performance of > 0.5 mm aggregate content decreased. Without freeze-thaw treatment (freeze-thaw 0 cycle), four proportions of composite soil aggregate particle size > 1 mm is much higher than that of other particle sizes (Figure 1). As the number of freeze-thaw cycles increases, there is a significant change in the distribution of aggregates with different particle sizes. The proportion of >1 mm aggregates significantly decreases (p < 0.01), and correspondingly, the proportion of <0.5 mm aggregates gradually increases. Meanwhile, the aggregate content of 1:2 and 1:5 composite soil increased significantly at 0.5–0.25 mm particle size, and the aggregate content in this particle size range is greater than 0:1 and 1:1 in the composite soil. After 10 freeze-thaw cycles, the >1 mm aggregate content of 1:0, 1:1, 1:2 and 1:5 composite soils decreased by 55%, 34%, 44% and 57%, while the <0.5 mm aggregate content increased by 91%, 70%, 66% and 87%. The particle size composition of compound soil aggregates is mainly concentrated at 0.25–0.5 mm, the proportion of medium-sized aggregates (0.5–1 mm) and small aggregates (<0.25 mm) is relatively small.
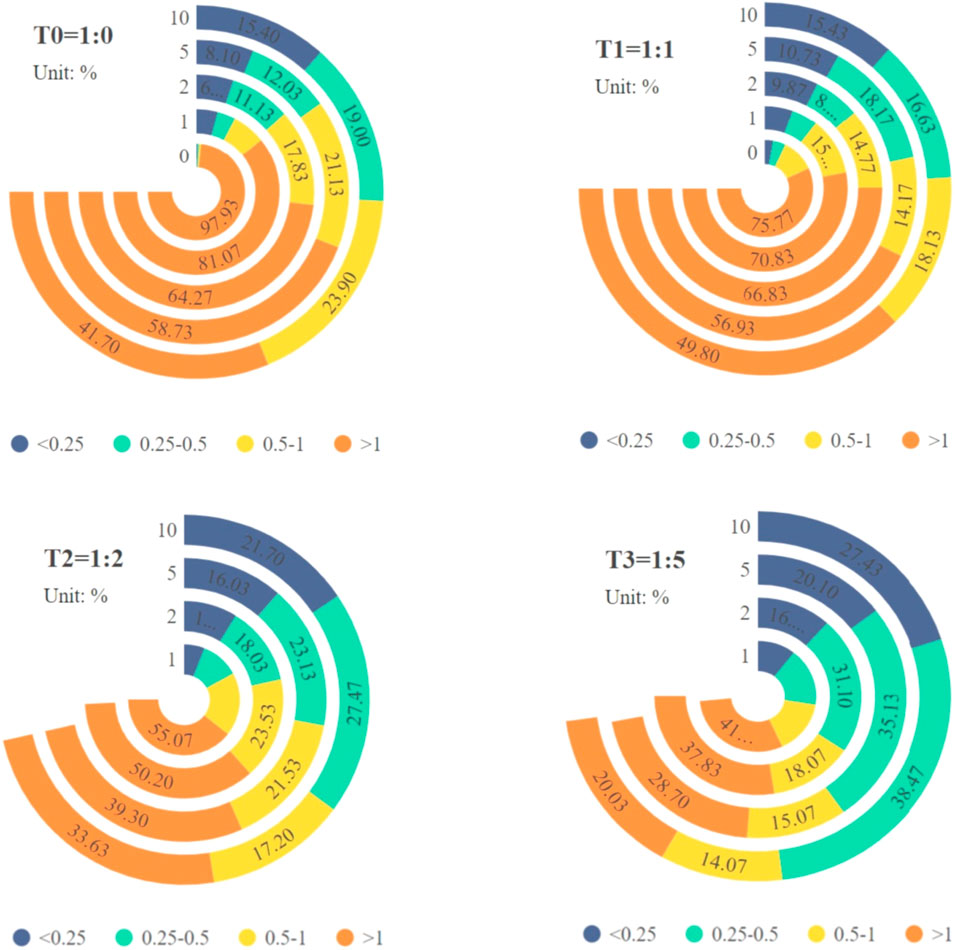
Figure 1. Distribution changes of aggregates in soft rock and sand compound soil with different proportions under different freeze-thaw cycles.
3.3 Influence of the interaction between freeze-thaw cycle and clay content on compound soil aggregates
The influence of clay content on compound soil aggregate content of different particle sizes reached a significant level (p < 0.05). The clay content of the composite soil is 1:1 > 1:2:>1:5. After different freeze-thaw cycles, the >1 mm aggregate content of the composite soil shows a trend of 1:1 > 1:2 > 1:5, which is the same as the change of clay content. With the decrease of soft rock, the proportion of sandy soil increased, the content of clay decreased, the content of >1 mm aggregate decreased, and the content of <1 mm aggregate increased. Among the four ratios, the average content of 1:5 composite soil >1 mm aggregates was the smallest. With the increase of freeze-thaw cycles, the large aggregate content (>1 mm) of 1:0 composite soil significantly decreases, and the >1 mm aggregate content of 1:1 (loam), 1:2 (sandy loam) and 1:5 (sandy loam) composite soil decreased slowly. The decrease rate of large aggregate (>1 mm) content in 1:1 composite soil was the smallest, and the small aggregate (<0.5 mm) content in 1:2,1:5 composite soil was significantly higher than that in 1:0,1:1 composite soil (Figure 2).
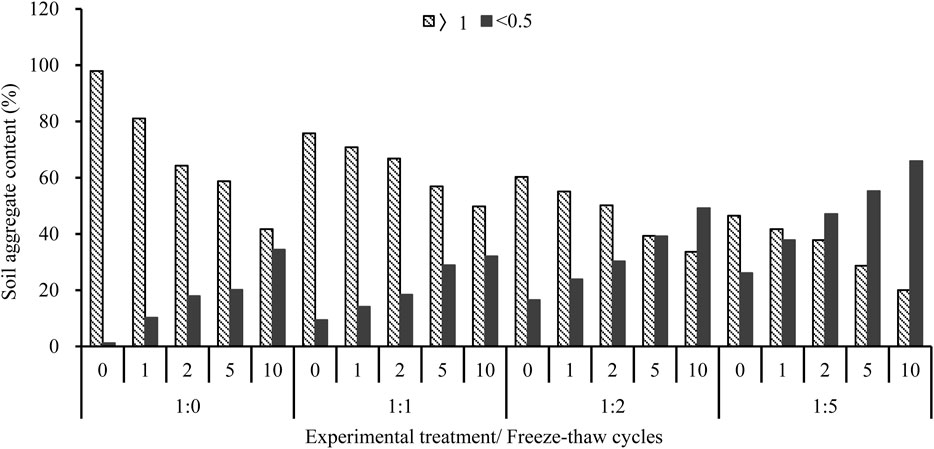
Figure 2. Distribution characteristics of soil aggregates under the interaction between freeze-thaw cycles and clay content.
3.4 Relationship between soil aggregate stability and organic matter under freeze-thaw cycles
As shown in Figure 3, with the increase of freeze-thaw cycles, the large aggregates (>1 mm) in the 1:1, 1:2, and 1:5 composite soil showed a decreasing trend, the decrease rate of large aggregates was slow during 0–2 cycles of freeze-thaw, the decrease rate was obvious after 2 cycles. At the beginning of the freeze-thaw cycle, the content of small aggregates (<0.5 mm) in the compound soil with different proportions showed a gradual increase trend, and the content reached a peak at the second freeze-thaw cycle, and the increase rate was the largest. After two cycles, the content of small aggregates decreased and showed a gentle decreasing trend. The freeze-thaw cycle had a significant impact on the organic matter content of composite soil (p < 0.05). During the 0–2 cycles of freeze-thaw, the organic matter content of composite soil with different proportions increased rapidly, and the organic matter content reached the maximum value at freeze-thaw cycle 2. Compared with the 0 cycles of freeze-thaw, the organic matter content of 1:1, 1:2, and 1:5 composite soil increased by 70%, 55%, and 59%. After 2 cycles, the organic content began to decrease and the change tended to be gradual.
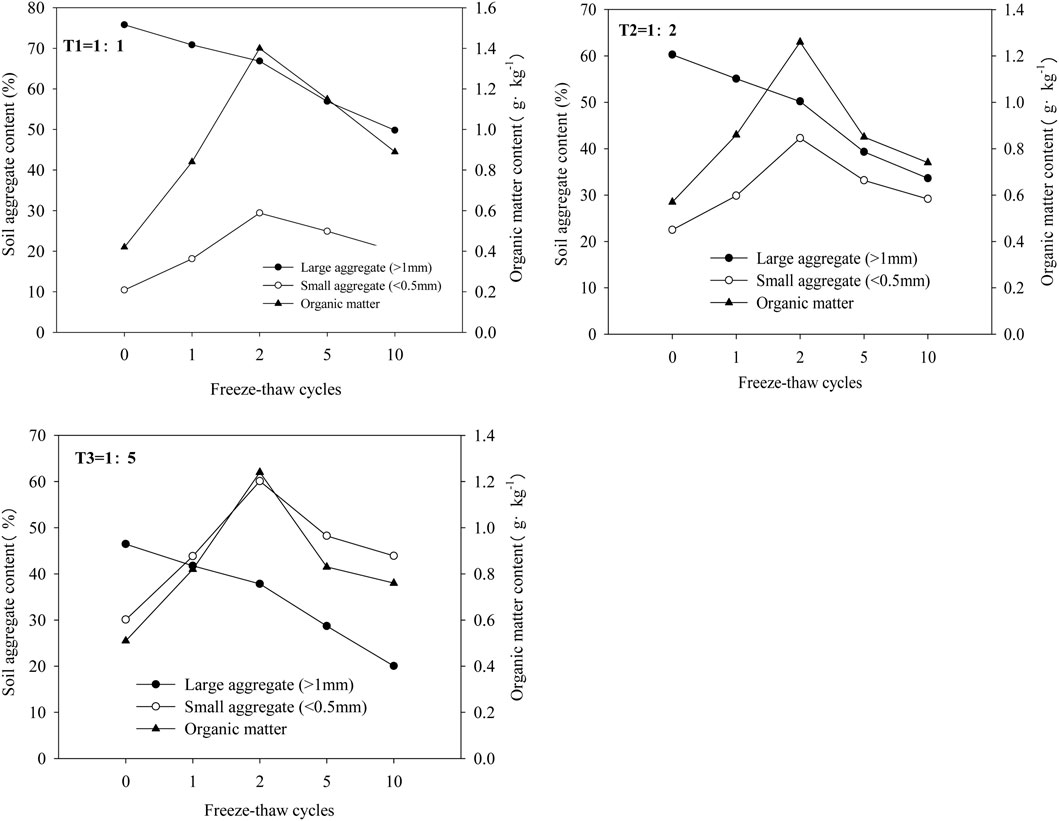
Figure 3. The relationship between aggregate structure and organic matter of compound soil under freeze-thaw cycles.
The interaction between freeze-thaw cycles and organic matter content significantly affected the formation of compound soil aggregates. Under freeze-thaw cycles, 1:1, 1:2 and 1:5 compound soil aggregates (<0.5 mm) stability increases with the increase of organic matter content, and the contents of organic matter and small aggregates (<0.5 mm) reach the peak value in the second cycle of freeze-thaw, and then they gradually decrease, and the decrease rate is slow. Under freeze-thaw cycles, there is a highly significant positive correlation (p < 0.01) between the three proportions of compound soil small aggregates (<0.5 mm) and organic matter content, with a correlation of R > 0.95 or higher. However, with the increase of freeze-thaw cycle, large aggregates (>1 mm) stability slowly decreases with the increase of organic matter content, and there was no significant correlation between the large aggregate structure and organic matter in the compound soil (Table 3).
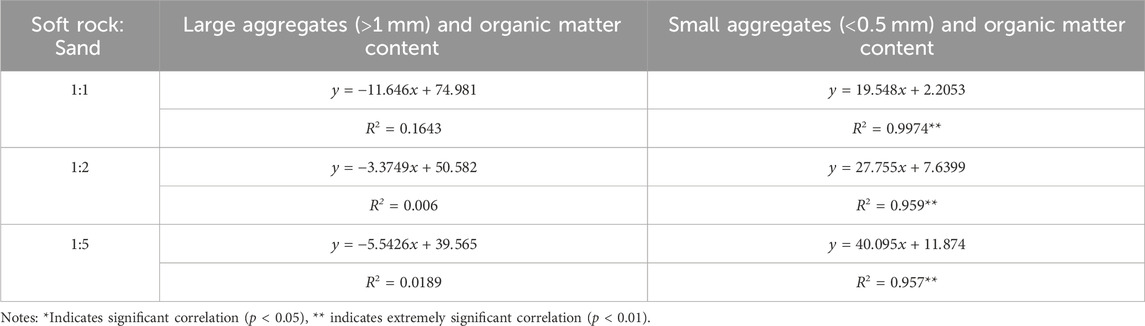
Table 3. Relationship between soil aggregate and organic matter content in compound soil with different proportions.
4 Discussion
4.1 Compound soil particle composition structure
The formation, characteristics and functions of soil aggregates are very complex. In the formation process of soil aggregates, cementing substances plays a very important role, which can be inorganic, organic, or organic-inorganic combined (Zhang et al., 2016). It is generally believed that in soils with low organic matter content, the formation of aggregates mainly depends on the cohesive force of clay particles and the cementation effect of iron and aluminum oxides (Sun et al., 2019). The organic matter content of soft rock and aeolian sandy soil is low. According to the soil nutrient classification standard of cultivated land in China, the organic matter content of the composite soil is grade 6 (<0.6%) (Guo et al., 2023b). Therefore, clay particles play a dominant role in the formation of composite soil aggregates, and the higher the clay content in the composite soil, the higher the stability of the soil aggregate structure (Cai et al., 2013; Wu et al., 2021). The core problem of aeolian sandy soil in Mu Us Sandy Land is the absence of clay particles and mineral colloids, resulting in the lack of cement substance formed by soil aggregates and the low stability of aggregates. The research results found that the content of soft rock had a significant impact on the particle composition of composite soil (p < 0.05), and the content of secondary clay minerals and clay particles in soft rock is much higher than that in sandy soil. With the addition of natural clay mineral material soft rock in aeolian sand soil, the content of silt and clay key particles increases, thereby improving the texture of aeolian sand soil and promotes the formation of aggregates. Therefore, the core technology of soft rock and sand compound soil is to introduce silt and clay particles, transplant colloidal substances from soft rock, and improve the soil structure.
4.2 Freeze-thaw cycles and soil aggregate structure
The freeze-thaw alternation is an important mechanism that affects the formation of soil aggregates, which can change the size and stability of soil aggregates (Wang Y. et al., 2013). It is found that after 10 freeze-thaw cycles, the proportion of large-size aggregates (>1 mm) was significantly reduced, while the proportion of other small and medium-size aggregates (<0.5 mm) was increased in the composite soil. The damage degree of freeze-thaw cycle to the structural stability of large particle size aggregates is greater than that of small particle size aggregates, the research results are consistent with the findings of Yu et al. (2012) and Angst et al. (2021). This is due to the expansion of ice crystals in soil pores during freezing, which breaks the connection between particles, thus reducing the stability of the soil aggregates, this effectively breaks down large size aggregates into small aggregates, while fine particles tend to aggregate towards medium sized particles (Liu et al., 2013; Li and Fan, 2014). The freeze-thaw alternate action can also cause the disintegration of large-sized soft rock structure, forming small particle sized soil structures. In the process of the soft rock and sand compounding into soil, the smaller the diameter of the soft rock, the easier it is to mix with sand, and the better the compounding effect. In addition, due to the bidirectional effect of freeze-thaw action on the stability of soil structure (Wang et al., 2012; Edwards, 2013), freeze-thaw action can not only increase the stability, density and decrease the porosity ratio of loose sand soil, but also reduce the consolidation property, decrease the density and increase the porosity ratio of dense soft rock, resulting in better complementary characteristics between soft rock and sandy soil.
4.3 Soil clay content and aggregate structure
The results showed that the changes of soil aggregate and clay content were the same in different proportions of composite soil after different freeze-thaw cycles, both of which were 1:1>. 1:2 > 1:5. The higher the clay content of the compound soil, the higher the stability of the aggregate, and the smaller the degree of damage to the aggregates after freeze-thaw alternation. Conversely, the lower the clay content of the compound soil, the poorer the stability of the aggregate, and the greater the degree of damage to the aggregate structure after freeze-thaw alternation. Shen et al. (2022) found that when the clay content in the soil exceeded 41%, the stability of the macro-aggregate structure was basically not affected by the frequency of soil freeze-thaw alternations; when the clay content in the soil was lower than 15%, the stability of the macro-aggregate structure decreased with the increase of the frequency of freeze-thaw alternations. The clay content of compound soil with different proportions was lower than 15%, so the number of freeze-thaw cycles had a significant effect on the aggregate content of different particle sizes in compound soil (p < 0.01). With the increase of freeze-thaw cycles, the large aggregate structure of the composite soil was broken into small and medium-size aggregates, which significantly reduced the proportion of large aggregates (>1 mm) and increased the proportion of other small and medium-size aggregates, among which the content of 0.25–0.5 mm aggregates was the largest (Oztas and Fayetorbay, 2003). The content of 0.25–0.5 mm aggregates in 1:2 and 1:5 composite soils was significantly higher than that in 0:1 and 1:1 composite soils, which might be due to the reduced stability of soil aggregate structure caused by freeze-thaw alternation. However, high clay content can increase the stability of soil aggregate structure. The interaction between clay content and freeze-thaw cycles is the result of strengthening or weakening the effect of a single horizontal factor (Chen et al., 2013; Wang et al., 2023).
4.4 Soil organic matter and aggregate structure
The change of soil organic matter under the freeze-thaw alternations is mainly due to the change of soil microorganisms (Kyriakopoulos and Sebos, 2023). During the 0–2 cycles of freeze-thaw, the organic matter content of compound soil with different proportions increases significantly. This is because the intense freezing temperature in the early stages of freeze-thaw kills some microorganisms, and the dead microorganisms release some small molecule organic matter during the decomposition process, which increases the soil organic matter content. On the other hand, the stability of soil aggregates is an important factor determining the content of soil organic matter. Freeze-thaw destroys the stability of soil aggregates, resulting in the early deaggregation of organic matter enclosed and adsorbed by soil, thus increasing the content of organic matter in soil (Wang F. et al., 2013; Liu et al., 2023). After several freeze-thaw cycles, the content of organic matter in the compound soil decreased, which was mainly due to the adaptation of microorganisms to external temperature changes, resulting in the gradual reduction of their absolute death. Correspondingly, the organic matter content released by microorganisms also decreases, and the surviving microorganisms gradually decompose and utilize the original organic matter in the soil, which leads to the decrease of the organic matter content in the soil. Similar to the results of Shi et al. (2023), the changes of soil microorganisms under freeze-thaw cycle were mainly due to the changes in the richness and diversity of bacterial communities and actinomycetes, which affected the changes of soil organic matter content.
Organic matter plays a very important role in maintaining the stability of soil aggregates, it is an important base nucleus for the formation of aggregates, and soil aggregates are also the main storage place for organic matter (Chen et al., 2016). Under freeze-thaw cycles, the stability of soil aggregates is closely related to the organic matter content in different proportions of compound soil. 1:1 compound soil has high content of organic matter and high stability of soil aggregates. The content of organic matter in 1:2 and 1:5 compound soil is low, thus the soil aggregates are easy to disaggregate, and the soil organic matter is released quickly after freeze-thaw cycles. Under freeze-thaw cycles, there is a significant positive correlation between small aggregates (<0.5 mm) and organic matter content, while there is no significant correlation between large aggregates (>1 mm) and organic matter content in 1:1, 1:2, and 1:5 compound soil. This is similar to the research results of relevant scholars (Jin et al., 2019), which shows a relatively obvious positive correlation between soil organic matter and aggregates content within a certain particle size range.
5 Conclusion
Clay is the key controlling factor for the restoration of sandy soil structure and the stability of compound soil aggregates, the higher the clay content of the compound soil, the better the stability of the soil aggregate structure after freeze-thaw cycle. The freeze-thaw cycle had a significant effect on the destruction of large particle size aggregates (>1 mm) in different proportions of compound soil, and increased the proportion of other small and medium-sized particle aggregates (0.25–0.5 mm), especially the contents of 0.25–0.5 mm aggregates in 1:2 and 1:5 compound. The freeze-thaw cycle improves the surface compound soil structure, rather than being dominated by destructive effects, resulting in a better mixing effect of soft rock and sand. There was a significant positive correlation between the compound soil organic matter and the aggregates content in the range of small particle size (0.25–0.5 mm). The results can provide practical guidance and scientific basis for exploring soil degradation, managing vegetation in early spring, improving soil fertility, and promoting sustainable agricultural development in seasonal frozen areas.
The formation and quality evolution of soil is a complex process. In the future, A more accurate understanding of the effect of freeze-thaw alternation on the composite soil structure needs to be supported by experimental data combined with field experiment observation and laboratory simulation, and a wider range of influencing factors such as freezing temperature, soil moisture content and freeze-thaw period should be considered. It is of great significance to accurately understand the relationship between soil structure and climate feedback in Mu Us Sandy Land.
Data availability statement
The original contributions presented in the study are included in the article/Supplementary material, further inquiries can be directed to the corresponding author.
Author contributions
HZ: Conceptualization, Data curation, Formal Analysis, Software, Validation, Writing–original draft. TC: Conceptualization, Funding acquisition, Project administration, Supervision, Writing–original draft. ZG: Conceptualization, Funding acquisition, Project administration, Supervision, Writing–original draft. YW: Conceptualization, Formal Analysis, Software, Validation, Writing–original draft. XH: Conceptualization, Formal Analysis, Software, Validation, Writing–original draft.
Funding
The author(s) declare that financial support was received for the research, authorship, and/or publication of this article. This study was financially supported by the Key Research and Development Program of Shaanxi Province (2023-ZDLNY-48, 2022NY-082), Shaanxi Provincial Natural Science Basic Research Program (2021JZ-57), funded by Shaanxi Provincial Land Engineering Construction Group internal research project (DJNY-YB-2023-32, DJNY2024-20). The funder was not involved in the study design, collection, analysis, interpretation of data, the writing of this article or the decision to submit it for publication.
Conflict of interest
Authors HZ, TC, ZG, YW, and XH were employed by Shaanxi Provincial Land Engineering Construction Group Co., Ltd.
Publisher’s note
All claims expressed in this article are solely those of the authors and do not necessarily represent those of their affiliated organizations, or those of the publisher, the editors and the reviewers. Any product that may be evaluated in this article, or claim that may be made by its manufacturer, is not guaranteed or endorsed by the publisher.
References
Angst, G., Mueller, K. E., Nierop, K. G. J., and Simpson, M. J. (2021). Plant-or microbial-derived? A review on the molecular composition of stabilized soil organic matter. Soil Biol. biochem. 156, 108189. doi:10.1016/j.soilbio.2021.108189
Bechmann, M. E., Kleinman, P. J. A., Sharpley, A. N., and Saporito, L. S. (2005). Freeze-thaw effects on phosphorus loss in run off from manured and catch-cropped soils. J. Environ. Qual. 34, 2301–2309. doi:10.2134/jeq2004.0415
Cai, Y. J., Wang, X. D., Ding, W. X., Yan, Y., Lu, X. Y., and Du, Z. Y. (2013). Effects of freeze-thaw on soil nitrogen transformation and N2O Emission: a review. Acta Pedol. Sin. 50, 1032–1042.
Chang, Z. Q., Ma, Y. L., Liu, W., Feng, Q., Su, Y. H., and Xi, H. Y. (2014). Effect of soil freezing and thawing on the carbon and nitrogen in forest soil in the Qilian Mountains. J. Glaciol. Geocryol. 36, 200–206.
Chen, S., Ouyang, W., Hao, F., and Zhao, X. (2013). Combined impacts of freeze-thaw processes on paddy land and dry land in Northeast China. Sci. Total Environ. 456-457, 24–33. doi:10.1016/j.scitotenv.2013.03.059
Chen, Z., Yang, S. Q., Zhang, Q. W., Zhou, H. K., Jing, X., Zhang, A. P., et al. (2016). Effect of freeze-thaw cycles on soil nitrogen loss and availability. Acta Ecol. Sin. 36, 1083–1094.
Du, Z. Y., Cai, Y. J., Wang, X. D., Yan, Y., Lu, X. Y., and Liu, S. Z. (2014). Research progress on the effects of soil freeze-thaw on plant physiology and ecology. Chin. J. Eco-Agr. 22, 1–9. doi:10.3724/sp.j.1011.2014.30941
Edwards, L. M. (2010). The effect of alternate freezing and thawing on aggregate stability and aggregate size distribution of some Prince Edward Island soils. Eur. J. Soil Sci. 42, 193–204. doi:10.1111/j.1365-2389.1991.tb00401.x
Edwards, L. M. (2013). The effects of soil freeze–thaw on soil aggregate breakdown and concomitant sediment flow in Prince Edward Island: a review. Can. J. Soil Sci. 93, 459–472. doi:10.4141/cjss2012-059
Fan, J. H., Cao, Y. Z., Yan, Y., Lu, X. Y., and Wang, X. D. (2012). Freezing-thawing cycles effect on the water soluble organic carbon, nitrogen and microbial biomass of alpine grassland soil in Northern Tibet. Afr. J. Microbiol. Res. 6, 562–567.
Gao, M., Li, Y. X., Zhang, X. L., Zhang, F. S., Liu, B., and Gao, S. Y. (2016). Influence of freeze-thaw process on soil physical, chemical and biological properties: a review. J. Agro-Environ. Sci. 35, 2269–2274.
Guo, J. T., Han, F. L., Hu, Y. M., Nie, Z. W., Ren, B. H., and Bu, R. C. (2017). Ecological characteristics of vegetation and their responses to permafrost degradation in the north slope of Great Khingan Mountain valley of northeast China. Acta Ecol. Sin. 37, 6552–6561. doi:10.5846/stxb201607021363
Guo, Z., Han, J. C., and Li, J. (2020a). Response of organic carbon mineralization and bacterial communities to soft rock additions in sandy soils. PeerJ 8, 8948. doi:10.7717/peerj.8948
Guo, Z., Han, J. C., Zhang, Y., and Wang, H. Y. (2023b). Mineralization mechanism of organic carbon in maize rhizosphere soil of soft rock and sand mixed soil under different fertilization modes. Front. Plant Sci. 14, 1278122. doi:10.3389/fpls.2023.1278122
Guo, Z., Li, J., Zhang, Y., Wang, H. Y., and Li, W. Y. (2023a). The soft rock can promote the improvement of aeolian sandy soil in Mu Us Sandy Land, China. Sci. Rep-UK 13, 11813. doi:10.1038/s41598-023-38928-7
Guo, Z. Y., Wang, Y. H., Wan, Z. M., Zuo, Y. J., He, L. Y., Li, D., et al. (2020b). Soil dissolved organic carbon in terrestrial ecosystems: global budget, spatial distribution and controls. Glob. Ecol. Biogeogr. 29, 2159–2175. doi:10.1111/geb.13186
Haddix, M. L., Gregorich, E. G., Helgason, B. L., Janzen, H., Cotrufo, M. F., and Francesca Cotrufo, M. (2020). Climate, carbon content, and soil texture control the independent formation and persistence of particulate and mineral-associated organic matter in soil. Geoderma 363, 114160. doi:10.1016/j.geoderma.2019.114160
Han, J. C., Liu, Y. S., and Zhang, Y. (2015). Sand stabilization effect of feldspathic sandstone during the fallow period in Mu Us Sandy Land. J. Geogr. Sci. 4, 428–436. doi:10.1007/s11442-015-1178-7
Han, J. C., Xie, J. C., and Zhang, Y. (2012). Potential role of feldspathic sandstone as a natural water retaining agent in Mu Us Sandy Land, Northwest China. Chin. Geogr. Sci. 22, 550–555. doi:10.1007/s11769-012-0562-9
Hao, R. J., Li, Z. P., and Che, Y. P. (2007). Effects of freezing and thawing cycles on the contents of WSOC and the organic carbon mineralization in Paddy soil. Chin. J. Soil Sci. 38, 1052–1057.
Henry, H. A. L. (2007). Soil freeze–thaw cycle experiments: trends, methodological weaknesses and suggested improvements. Soil Biol. biochem. 39, 977–986. doi:10.1016/j.soilbio.2006.11.017
Jiang, H., Han, X. Z., Zou, W. X., Hao, X. X., and Zhang, B. (2018). Seasonal and long-term changes in soil physical properties and organic carbon fractions as affected by manure application rates in the Mollisol region of Northeast China. Agr. Ecosyst. Environ. 268, 133–143. doi:10.1016/j.agee.2018.09.007
Jin, W. P., Fan, H. M., Liu, B., Jiang, Y. Z., Jiang, Y., and Ma, R. M. (2019). Effects of freeze-thaw cycles on aggregate stability of black soil. J. Appl. Ecol. 30, 4195–4201. doi:10.13287/j.1001-9332.201912.025
Kyriakopoulos, G. L., and Sebos, I. (2023). Enhancing climate neutrality and resilience through coordinated climate action: review of the synergies between mitigation and adaptation actions. Climate 11, 105. doi:10.3390/cli11050105
Lehmann, J., Hansel, C. M., Kaiser, C., Kleber, M., Maher, K., Manzoni, S., et al. (2020). Persistence of soil organic carbon caused by functional complexity. Nat. Geosci. 13, 529–534. doi:10.1038/s41561-020-0612-3
Li, G. Y., and Fan, H. M. (2014). Effect of freeze-thaw on water stability of aggregates in a black soil of Northeast China. Pedosphere 24, 285–290. doi:10.1016/s1002-0160(14)60015-1
Liu, H. M., Li, X. L., Yu, Z. Y., Tan, Y., Ding, Y. S., Chen, D. Y., et al. (2023). Influence of hole diameter on mechanical properties and stability of granite rock surrounding tunnels. Phys. Fluids 35, 064121.
Liu, J., Khalaf, R., Ulén, B., and Bergkvist, G. (2013). Potential phosphorus release from catch crop shoots and roots after freezing-thawing. Plant Soil 371, 543–557. doi:10.1007/s11104-013-1716-y
Oztas, T., and Fayetorbay, F. (2003). Effect of freezing and thawing processes on soil aggregate stability. Cetena 52, 1–8. doi:10.1016/s0341-8162(02)00177-7
Sahin, U., Angin, I., and Kiziloglu, F. M. (2008). Effect of freezing and thawing processes on some physical properties of saline-sodic soils mixed with sewage sludge or fly ash. Soil Till Res. 99, 254–260. doi:10.1016/j.still.2008.03.001
Schuur, E. A. G., McGuire, A. D., Schädel, C., Grosse, G., Harden, J. W., Hayes, D. J., et al. (2015). Climate change and the permafrost carbon feedback. Nature 520, 171–179. doi:10.1038/nature14338
She, X. Y., Zhang, X. C., and Wei, X. R. (2014). Improvement of water absorbing and holding capacities of sandy soil by appropriate amount of soft rock. Trans. CSAE. 30, 115–123.
Shen, J. J., Wang, Q., Chen, Y. T., Han, Y., Zhang, X. D., and Liu, Y. W. (2022). Evolution process of the microstructure of saline soil with different compaction degrees during freeze-thaw cycles. Eng. Geol. 304, 106699. doi:10.1016/j.enggeo.2022.106699
Shi, G. X., Hou, R. J., Li, T. X., Fu, Q., Wang, J. W., Zhou, W. Q., et al. (2023). Effects of biochar and freeze‒thaw cycles on the bacterial community and multifunctionality in a cold black soil area. J. Environ. Manage. 342, 118302. doi:10.1016/j.jenvman.2023.118302
Sun, B. Y., Li, Z. B., Xiao, J. B., Zhang, L. T., Ma, B., Li, J. M., et al. (2019). Research progress on the effects of freeze-thaw on soil physical and chemical properties and wind and water erosion. Chin. J. Appl. Ecol. 30, 337–347. doi:10.13287/j.1001-9332.201901.019
Sun, Z. H., and Han, J. C. (2018). Effect of soft rock amendment on soil hydraulic parameters and crop performance in Mu Us Sandy Land, China. Field Crop Res. 222, 85–93. doi:10.1016/j.fcr.2018.03.016
Tan, B., Wu, F. Z., Yang, W. Q., Yang, Y. L., Wang, A., and Kang, L. N. (2011). Effects of snow pack removal on the dynamics of winter-time soil temperature, carbon, nitrogen, and phosphorus in alpine forests of west Sichuan. Chin. J. Appl. Ecol. 22, 2553–2559.
Wang, E. H., Cruse, R. M., Chen, X. W., and Daigh, A. (2012). Effects of moisture condition and freeze/thaw cycles on surface soil aggregate size distribution and stability. Can. J. Soil Sci. 92, 529–536. doi:10.4141/cjss2010-044
Wang, E. Q., Chen, J. F., Liu, L., Cui, L. H., Xue, J., Ren, J. M., et al. (2023). Effect of soil texture on water and salt transport in freeze-thaw soil in the shallow groundwater area. Water 15, 2587. doi:10.3390/w15142587
Wang, F., Zhu, Y., Chen, S., Zhang, K. Q., Bai, H. L., and Yao, H. (2013b). Effect of freeze-thaw cycles on available nitrogen and phosphorus, enzymatic activities of typical cultivated soil. Trans. CSAE. 29, 118–123.
Wang, H. Y., Han, J. C., Tong, W., Cheng, J., and Zhang, H. O. (2017). Analysis of water and nitrogen use efficiency for maize (Zea mays L.) grown on soft rock and sand compound soil. J. Sci. Food Agr. 97, 2553–2560. doi:10.1002/jsfa.8075
Wang, J. Y., Song, C. C., Wang, X. W., and Wang, L. L. (2011). Progress in the study of effect of freeze-thaw processes on the organic carbon pool and microorganisms in soils. J. Glaciol. Geocryol. 33, 442–452.
Wang, N., Xie, J. C., Han, J. C., and Luo, L. T. (2014). A comprehensive framework on land-water resources development in Mu Us Sandy Land. Land Use Policy 40, 69–73. doi:10.1016/j.landusepol.2013.09.022
Wang, Y., Liu, J. S., and Wang, Q. Y. (2013a). The Effects of freeze-thaw processes on soil aggregates and organic carbon. Ecol. Env. Sci. 22, 1269–1274.
Wen, J., Wang, Y. B., Gao, Z. Y., and Liu, G. H. (2013). Soil hydrological characteristics of the degrading meadow in permafrost regions in the Beiluhe River basin. J. Glaciol. Geocryol. 35, 929–937.
Wu, M. H., Qu, D. Y., Li, T., Liu, F., Gao, Y. Y., Chen, S. Y., et al. (2021). Effects of permafrost degradation on soil microbial biomass carbon and nitrogen in the Shule River headwaters, the Qilian Mountains. Sci. Geogr. Sin. 41, 177–186.
Xu, G. C., Li, Z. B., and Li, P. (2013). Fractal features of soil particle-size distribution and total soil nitrogen distribution in a typical watershed in the source area of the middle Dan River, China. Catena 101, 17–23. doi:10.1016/j.catena.2012.09.013
Yang, M. H., Cao, M. M., and Zhu, Z. M. (2017). Vegetation degradation and its stability in desertification at southeastern edge of Mu Us Sandy Land. Bull. Soil Water Conserv. 37, 10–15.
Yu, B., Zhao, L. P., and Wang, H. B. (2012). Responses of soil structure characteristics of high-yield farmland to freeze and its mechanism in corn belt of the Songliao plain. J. Soil Water Conserv. 26, 172–177.
Zhang, B., Chen, Q., Wen, J. H., Ding, X. L., and Agathokleous, E. (2022). Straw addition decreased the resistance of bacterial community composition to freeze-thaw disturbances in a clay loam soil due to changes in physiological and functional traits. Geoderma 424, 116007. doi:10.1016/j.geoderma.2022.116007
Zhang, Z., Ma, W., Feng, W. J., Xiao, D. H., and Hou, X. (2016). Reconstruction of soil particle composition during freeze-thaw cycling: a review. Pedosphere 26, 167–179. doi:10.1016/s1002-0160(15)60033-9
Keywords: freeze-thaw cycle, soft rock, aeolian sand soil, composite soil, aggregates, clay, soil organic matter
Citation: Zhang H, Cao T, Guo Z, Wang Y and Hou X (2024) Response of soft rock and sand compound soil structure to freeze-thaw cycles in Mu Us Sandy Land, China. Front. Environ. Sci. 12:1405203. doi: 10.3389/fenvs.2024.1405203
Received: 22 March 2024; Accepted: 07 May 2024;
Published: 24 May 2024.
Edited by:
Anil K. Choudhary, ICAR–Central Potato Research Institute, IndiaReviewed by:
Ingudam Bhupenchandra, Central Agricultural University, IndiaM. N. Harish, Indian Agricultural Research Institute (ICAR), India
Anil Kumar, Guru Angad Dev Veterinary and Animal Sciences University, India
Vishal Tyagi, Indian Agricultural Research Institute (ICAR), India
Copyright © 2024 Zhang, Cao, Guo, Wang and Hou. This is an open-access article distributed under the terms of the Creative Commons Attribution License (CC BY). The use, distribution or reproduction in other forums is permitted, provided the original author(s) and the copyright owner(s) are credited and that the original publication in this journal is cited, in accordance with accepted academic practice. No use, distribution or reproduction is permitted which does not comply with these terms.
*Correspondence: Haiou Zhang, academic_our@163.com