- 1College of Geographic Science and Tourism, Xinjiang Normal University, Urumqi, China
- 2Key Laboratory of Lake Environment and Resources in Arid Area of Xinjiang, Urumqi, China
In November 2023, twenty-two sediment samples were collected from the Bosten Lake wetland in Xinjiang to determine the concentrations of eight heavy metals: arsenic (As), cadmium (Cd), chromium (Cr), copper (Cu), mercury (Hg), nickel (Ni), lead (Pb), and zinc (Zn). This data was used to assess heavy metal contamination and potential ecological risks in the sediments using the Pollution Load Index (PLI) and the Potential Ecological Risk Index (RI). Additionally, multivariate statistical analysis and Positive Matrix Factorization (PMF) were employed to elucidate potential sources and their contributions to contamination. The following are the main conclusions: (1) average concentrations of Zn, Cd, Cr, Pb, and Hg in the sediments surpassed Xinjiang soil background levels by factors of 1.01, 3.58, 1.32, 1.94, and 1.53, respectively. (2) Sediments demonstrated severe pollution with Cd, slight pollution with Zn, Cr, Pb, and Hg, while Cu and Ni indicated mild pollution and As showed non-polluted levels. The overall PLI average (1.01) suggested slight contamination. (3) The descending order of average single ecological risk values were Cd, Hg, Pb, Cu, Ni, Cr, As, and Zn, with a comprehensive RI averaging at 184.07, signaling a moderate ecological risk. (4) Source apportionment revealed that Zn, Cu, Pb, and Ni were influenced by transportation and household waste emissions, while Cr and As were dictated by natural background levels. Hg predominately originated from fossil fuel combustion and Cd from agricultural activities. (5) Mixed sources accounted for the following percentage contributions to sediment heavy metal content: transportation and domestic waste (30.41%), natural background (25.88%), fossil fuel combustion (22.40%), and agricultural activities (21.31%). With anthropogenic inputs exceeding those of natural origins, it is imperative to prioritize the management of Cd, Hg, and Pb as primary pollutants within the region.
1 Introduction
Heavy metals are toxic, environmentally persistent, and bioaccumulative, posing significant threats to both ecosystem integrity and human health via food chain trans-fer (Ouyang et al., 2018; Qiao et al., 2019). Recent anthropogenic discharges, particularly into aquatic ecosystems, have significantly increased the environmental burden of heavy metals (Duan and Tan, 2013; Yuan et al., 2014; Zhang et al., 2007). Within aquatic environments, heavy metals may concentrate in sediments through adsorption, complexation, flocculation, and sedimentation, and can be remobilized into the water column under changing thermal conditions (Wang E. et al., 2023).
Recognized as vital components of the planet’s ecosystem, alongside forests and oceans, wetlands are instrumental in preserving biodiversity, sustaining ecological equilibrium, moderating climate, conserving water resources, flood mitigation, drought prevention, soil erosion control, and pollutant filtration (Yong, 2002; Yongze and Xuan., 2001). Lakeshore wet-lands, in particular, embody a crucial intersection between terrestrial and lake ecosystems, distinguished by exceptional productivity and biodiversity. They serve as natural barriers that retain pollutants, stabilize lake shores, and maintain regional biological diversity (Anbumozhi et al., 2005; Chai et al., 2010). The influx of heavy metals from anthropogenic sources into these wetlands poses significant environmental threats and risks (Li F. et al., 2019; Wang et al., 2019). Thus, monitoring heavy metal levels in lakeshore wetland sediments is essential for wetland health assessment, and controlling such pollution is vital for wetland conservation and restoration. Consequently, investigating pollution levels, source attribution, and potential ecological risks in these sediments is crucial for safeguarding and rejuvenating wet-land environments.
Heavy metals are introduced to lakes from natural occurrences and human activities, including hydrological processes, erosion, atmospheric deposition, agricultural runoff, and industrial discharge (Merciai et al., 2014; Zhang et al., 2022; Ros et al., 2014). Most heavy metals settle in sediments, with only a minor fraction remaining dissolved in water (Liu and Dilinuer, 2014). Although extensive research covers the pollution characteristics, ecological risks, and origins of heavy metals in various ecological wetlands (Jiao et al., 2014; Ni et al., 2023; Wang X. M. et al., 2023; Kalani et al., 2021; Engin et al., 2015), arid zone wetlands have received less attention due to climatic challenges and habitat fragmentation. This study examines the western wetlands of Bosten Lake, China’s largest freshwater lake in an arid region, a critical recharge zone for the Tarim River and an essential water resource for the Bayin’guoleng Mongol Autonomous Prefecture. The lake’s ecological health has deteriorated due to industrial and agricultural effluents and overuse, making its wetlands, which lie at the confluence of the lake and human-inhabited areas, paramount for the ecological welfare of the lake, its biodiversity, and the livelihood of the local populace. This research enriches the understanding of heavy metal pollution in the arid wetlands of China by contributing extensive pollution data and environmental analysis, aspiring to offer scientific direction and a theoretical foundation.
2 Materials and methods
2.1 Study area
Bosten Lake (Figure 1), located in Bohu County within the southeast Yanqi Basin of the Xin-jiang Uygur Autonomous Region (441°45′–42°15′N, 86°–87°26′E), is recognized as China’s largest inland freshwater lake with significant throughput. Encompassing an area of 7,710.4 km2, the Bosten Lake basin is composed of the Kaidu River, the Peacock River basin, and extensive wetlands associated with Bosten Lake (Ye et al., 2020). Positioned at the heart of the Eurasian continent, this region experiences a pronounced inland arid cli-mate, characterized by low humidity, infrequent rainfall with an average annual precipitation of 68.2 mm, and high evapotranspiration rates between 1,800 and 2,000 mm annually. As a semi-enclosed lake with limited hydrodynamic activity, Bosten Lake has extended water retention periods and is surrounded by nearly 20,300 ha of reed wetlands, making it one of Xinjiang’s two pivotal fishery hubs (Liu et al., 2017). These wetlands not only serve as critical regulators of the ecological balance in the Bosten Lake Basin but also have a direct impact on the sustainable development of the region’s socio-economic environment. In recent decades, the escalating pace of economic growth within the area has led to a progressive deterioration in the water quality of the Bosten Lake wetlands.
2.2 Sample collection and processing measurements
Sediments (0–10 cm) were collected from 22 locations within Bosten Lake wetlands in November 2023 using a Peterson grab (ETC200, China). The distribution of the sampling sites is depicted in Figure 1. GPS technology was employed to accurately pinpoint all sampling sites. Subsequently, sediments were stored in clean, air-expelled, sealed polyethylene bags and maintained at low temperatures during transit. Upon arrival at the laboratory, samples underwent freeze-drying, pulverization, and sieving through a 100-mesh nylon mesh. The prepared samples were reserved for subsequent analysis.
For heavy metal determination, the samples were digested using a tetra-acid mixture (HCl-HNO3-HF-HCIO4). Post-digestion, 1 mL of the clear supernatant was di-luted to 10 mL. Concentrations of heavy metals—As, Cu, Zn, Pb, Cd, Ni, and Cr—were quantified using an Agilent 7700X inductively coupled plasma mass spectrometer (ICP-MS). Hg analyses were conducted with a Hydra-C fully automated mercury analyzer (Wang et al., 2004). Prior to experimentation, all laboratory containers were acid washed with 2 mol/L HNO3 for 24 h and rinsed thoroughly. All reagents employed were of analytical grade. To ensure the accuracy and precision of the measurement data, a standard solution established the calibration standard curve for the instruments (Hydra-C automatic mercury meter and ICP-MS). The resultant correlation coefficients (R) exceeded 0.9992, and relative standard deviations (RSDs) were below 5%, denoting reliable instrument readings. Quality control of the experimental methods was maintained by employing standard material (GBW07314) alongside sediment samples for simultaneous experiments, with recoveries calculated by comparing standard material measurements to known values. The recoveries of heavy metals ranged from 90% to 110%, signifying that the errors introduced by heavy metals in sediment sample testing and analysis were negligible.
2.3 Pollution load index method
Pollution load index (pollution load index, PLI) is a pollution evaluation method proposed by Tomlinson et al. in the study related to the grading of heavy metal pollution level, which can express the degree of the contribution of each heavy metal to the pollution as well as the trend of the spatial and temporal changes of the heavy metal (Tomlinson et al., 1980), which is calculated by the formula:
formula
2.4 Potential ecological risk index methodology
The Potential Ecological Risk Index method proposed by Hakanson was used to evaluate the potential ecological risk of sediment heavy metals in the study area (Hakanson, 1980). The potential ecological risk index (
formula:
2.5 Potential ecological risk index methodology
Positive definite matrix factor analysis model (PMF) is a quantitative pollution source factor analysis method based on multivariate statistical techniques (Taghvaee et al., 2018).
Where:
2.6 Data analysis
Data processing was performed using Microsoft Excel, while the two-dimensional spatial distribution mapping of features was conducted with ArcGIS 10.8. Origin2021 software facilitated the normal distribution testing and correlation analysis of the data. Moreover, EPA PMF5.0 software assisted in pinpointing potential anthropogenic sources of heavy metals in the sediment samples.
3 Results and discussion
3.1 Descriptive statistics of heavy metals in wetlands
Table 3 presents the descriptive statistics for heavy metal concentrations in sediment samples, including the range, mean, median, standard deviation, coefficient of variation (CV), skewness, and kurtosis. On average, the concentrations for Zn, Cr, Pb, Cu, Ni, As, Cd, and Hg were 69.82, 65.00, 37.63, 25.63, 22.14, 1.70, 0.43, and 0.026 mg/kg, respectively. When compared to the background values of soil elements in Xinjiang, the levels of As were lower in all sampled sites. In contrast, the concentrations of Cd and Pb were higher, peaking at 5.5 and 4.1 times above the background values, respectively. Although the median and mean concentrations of Ni and Cu did not surpass the background levels, their maximum concentrations did, reaching 1.5 and 2.3 times higher, respectively. The median and mean levels of Zn, Cr, and Hg exceeded the background values, while their minimum concentrations did not. The exceedance rates for Pb, Cd, and Cr were 100%, 95.46%, and 68.18%, respectively, suggesting that these may be the primary contaminants in the wetland sediments. The CVs for Zn, Pb, and Ni were classified as medium variability at 33.11%, 31.34%, and 30.07%, respectively (15% < CV < 35%). In comparison, As, Cd, Cr, Cu, and Hg exhibited high variability with CVs of 75.59%, 40.11%, 38.90%, 55.54%, and 57.82%, respectively (CV > 36%). This high variability indicates an uneven spatial distribution and the potential influence of point source pollution. According to skewness, the rank of the eight heavy metals was Pb > Cd > Zn > Hg > As > Ni > Cu > Cr. With all kurtosis values below 3, the data distribution for these metals is broadly platykurtic.
When compared to the average concentrations of heavy metals in sediments from the Zhanjiang Mangrove Wetlands, the Medeca Wetlands, the Setiu Wetland, the Uchalli Wetland, and the East Kolkata Wetlands, the contents of Ni and Cu in the Bosten Lake wetland sediments were found to be lower than those of the Zhanjiang Mangrove Wetlands but markedly higher than those in the remaining wetlands (Table 4). Moreover, levels of As, Cd, Pb, and Cr were significantly elevated compared with those of all five referenced regions. Zn concentrations were only exceeded by those in the Medeca Wetlands, and Hg levels were only outstripped by measurements from the East Kolkata Wetlands. Overall, the heavy metal concentration in the sediments of Bosten Lake’s wetlands is characterized by an intermediate to high level.
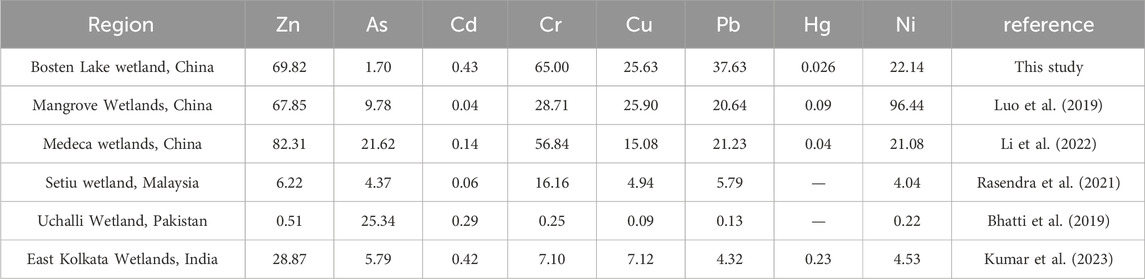
Table 4. Comparison of mean values of heavy metals in sediments of Bosten Lake wetland with sediments of other wetlands (mg/kg).
3.2 Characteristics of spatial distribution of heavy metal content in wetland sediments
This study utilized inverse distance interpolation to visualize the spatial distribution of eight heavy metals in the wetland sediments of Bosten Lake (Figure 2). The distribution patterns for Hg and Ni were similar, showcasing consistently elevated values in the wetland’s southern section and at the Kaidu River inlet, while levels in the north were notably lower. Zn, Cu, and Pb concentrations, with the exception of Cr, were reduced in the northern region; Cd concentration peaks were mainly found in the wet-land’s northern extents, whereas As exhibited high concentrations primarily at the Kaidu River inlet. Field observations revealed that the hotspots for heavy metal accumulation are predominantly situated near traffic routes, river inlets, agricultural lands, and the Bosten Lake tourist area. These areas experience significant human activity, and it is likely that pollutants from vehicular traffic and agricultural runoff are con-tributing to the wetland’s contamination.
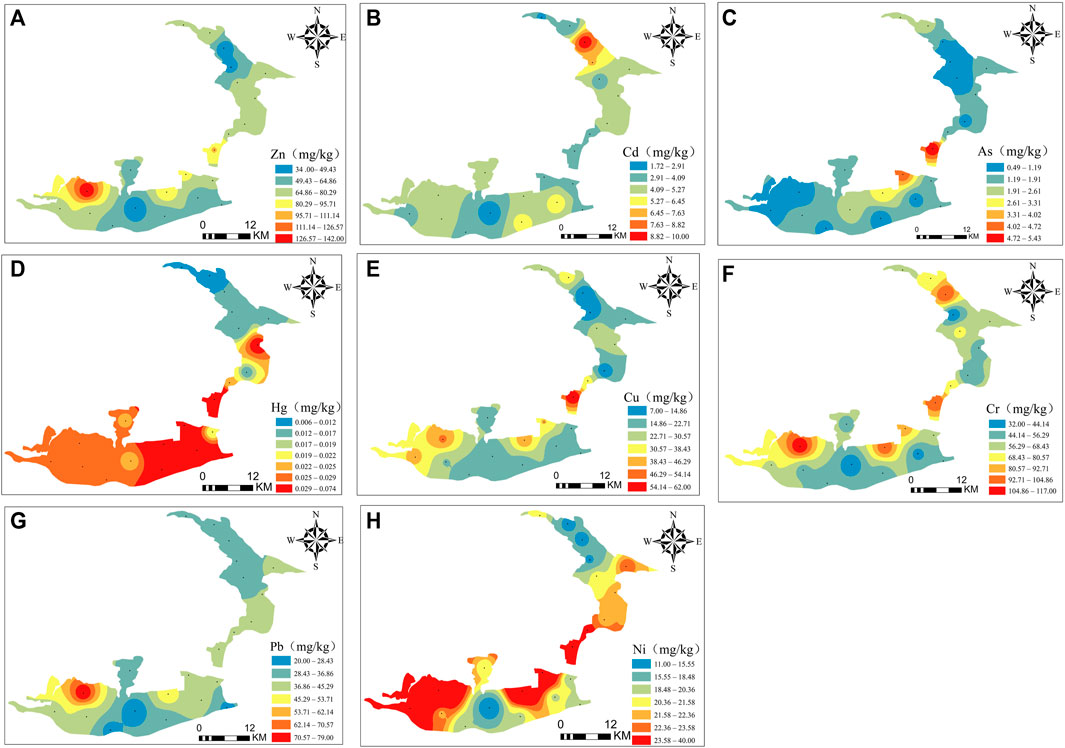
Figure 2. Spatial distribution map of heavy metals in wetlands. (A–H) represent (Zn, Cd, As, Hg, Cu, Cr, Pb, Ni), respectively.
3.3 Spatial variation in heavy metal contamination of sediments
Sediments exhibit natural spatial variability, and thus the heavy metal content from a specific sampling point reflects the environmental conditions of that site alone. The Kriging technique was employed to map the spatial distribution patterns of heavy metal contamination across the sediment. Figure 3 demonstrates these patterns using both the contamination factor (CF) (Figures 3A–H) and pollution load index (PLI) (Figure 3I) for Bosten Lake wetlands. Notably, Figure 3 illustrates that the spatial distribution patterns of the environmental risks, indicated by individual pollution indices for the eight studied heavy metals, vary considerably. Cd is characterized by the highest degree of pollution and the most extensive contaminated area, generally classified as moderate to severe pollution. Pb ranks second in terms of pollution extent and intensity, predominantly displaying regional pollution symptomatic of moderate contamination, except for the southern wetland fringe where a small segment falls within the heavily polluted bracket. Cr and Hg both primarily reflect low levels of pollution, yet their spatial distribution is markedly distinct. Cr decreases in pollution degree from north to south, with light pollution sporadically positioned along the southern border. Conversely, Hg pollution intensifies in the same direction. The pollutants Cu, Ni, and Zn exemplify a parallel arrangement of light pollution, minor pollution, and unpolluted zones–with the majority of the study area experiencing minor to no pollution and light pollution centering around the Kaidu River mouth and the southern periphery of the wetland. As, in contrast, shows no detectable pollution throughout the study area.
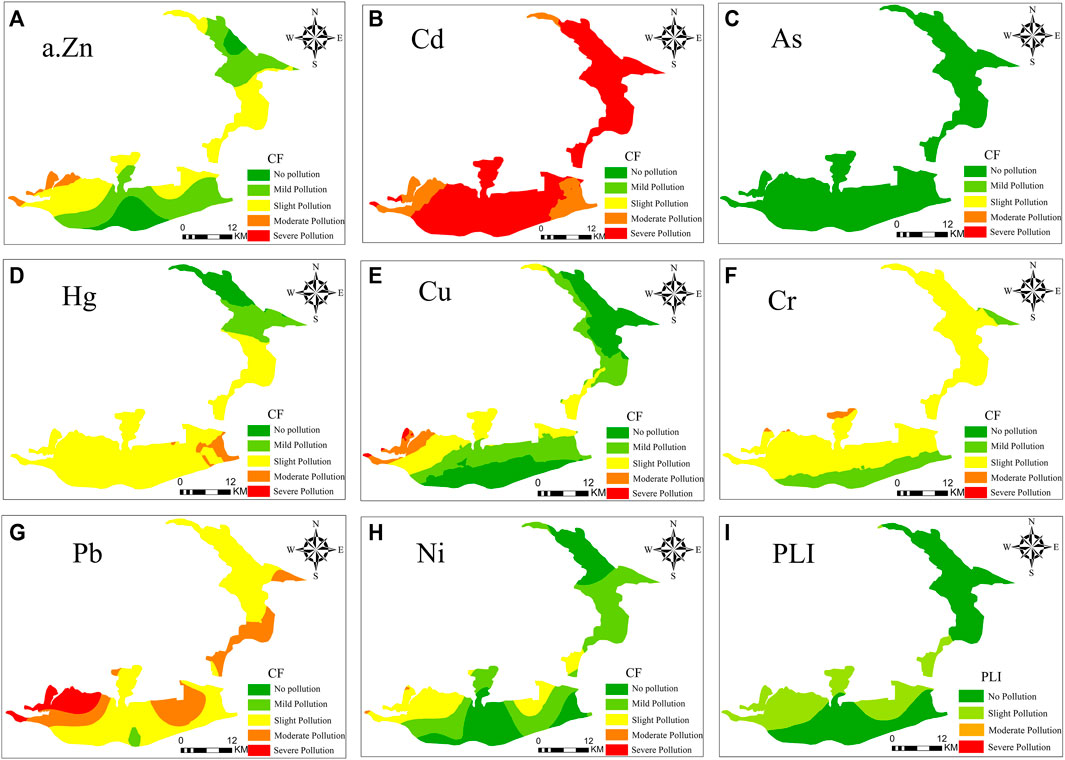
Figure 3. Spatial distribution of CF (A–H) for (Zn, Cd, As, Hg, Cu, Cr, Pb, and Ni, respectively) and PLI (I) in soil heavy metals from wetland sediments in Bosten Lake.
The mean pollution index values for each heavy metal in the wetland sediments of Lake Bosten, presented in descending order, are as follows: Cd (3.62), Pb (1.94), Hg (1.55), Cr (1.32), Zn (1.01), Cu (0.96), Ni (0.83), and As (0.15). According to these indices, Cd is classified as severely polluted; Pb, Hg, Cr, and Zn as moderately polluted; Cu and Ni as mildly polluted; and As unpolluted. The analysis of sample point distributions across varying pollution levels (Table 5) reveals that 100% of sampling points for As fall under non-pollution. In contrast, the percentages of sampling points with slight, moderate, and severe pollution levels for Cd are 4.55%, 31.82%, and 63.64%, respectively. Most sampling points for Cr, Pb, and Hg show slight pollution at 50.00%, 63.64%, and 87.37%, respectively, with a smaller proportion at 40.91% for Cr. Zn contamination is largely characterized by mild or slight pollution, each accounting for 40.91%. Ni predominantly exhibits mild pollution, representing 54.55% of sampling points, whereas Cu displays regional pollution variation, with non-polluted, slight, mild, and moderate contamination levels constituting 36.36%, 27.27%, 31.82%, and 4.55% of points, respectively.
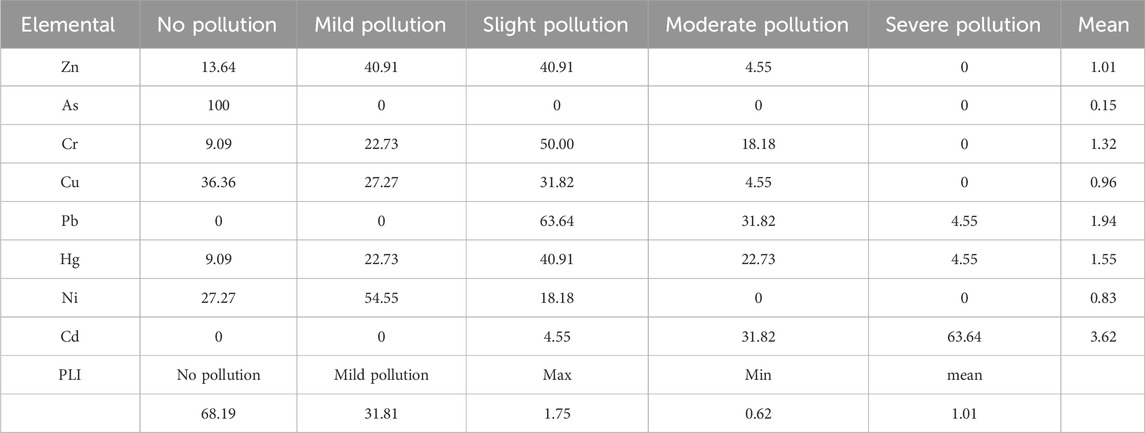
Table 5. Proportion (%) and statistical analysis of the number of sample points with different pollution levels of CF and PLI in the wetlands of Lake Bosten.
From the Bosten Lake wetland sediment heavy metal pollution load index (PLI) varied between 0.62 and 1.75, with an average value of 1.01, showing mild pollution. The number of sample points with PLI belonging to no pollution and mild pollution accounted for 68.19% and 31.81% of the total number of sample points, respectively. In terms of spatial distribution (Figure 3I), light pollution was mainly distributed in the river inlet and the southern edge area of the wetland, while the northern part of the wetland was less polluted and dominated by no pollution.
The sediments of Bosten Lake, along with those from various other regions, display the most pronounced heavy metal contamination for Cd, Pb, and Hg. Research conducted by Jane Yuefeng and colleagues (Jian et al., 2023) on the wetlands surrounding the Bohai Sea identified exceptionally high levels of single-factor pollution of Cd and Hg. Similarly, studies by Yang Pan et al. (Yang et al., 2021) on Chaohu Lake revealed that the mean concentrations of Cd and Hg significantly exceeded the soil background levels in Anhui Province, with the risk index for Cd reaching a level indicative of serious pollution, thereby constituting the primary pollutant. Further studies by Zhou Feng et al. (Zhou et al., 2023) on Longyang Lake and Moshui Lake in Wuhan City indicated that Cd was the predominant contributor to heavy metal pollution and ecological risk, with the sediment cumulative index method suggesting a state of moderate pollution in these lakes. These high levels of contamination are attributable to China’s status as a major agricultural nation where crop cultivation often occurs near water bodies and the widespread use of conventional fertilizers, which can lead to Cd pollution in the sediments of lakes, rivers, and wetlands.
3.4 Potential ecological risks and regional differences in wetland sediment contamination
The single and comprehensive potential ecological risk indices (E and RI, respectively) for heavy metals in the wetland sediments of Bosten Lake were assessed at each sampling location. In the Xinjiang region, the background values for river sediments are not yet well-established; therefore, this study has opted to employ the available soil background values as a reference. The assessment was conducted according to Hakanson’s criteria for potential ecological risk. The average values of the individual potential ecological risk indices for heavy metals in the sediments, arranged in descending order of risk, were as follows (Table 6): Cd (108.53), Hg (61.93), Pb (9.70), Cu (4.80), Ni (4.16), Cr (2.64), As (1.52), and Zn (1.01). Ecological risk levels for Zn, As, Cr, Pb, Ni, and Cu were negligible across all sampling sites. However, Cd exhibited a predominantly significant risks level, with 68.18% of the sampling points falling into this category. The risk index for Hg varied regionally, with negligible, moderate, significant, and high risk levels represented by 36.36%, 40.91%, 18.18%, and 4.55% of the total sampling points, respectively. Consequently, Cd and Hg represent the primary ecological risk concerns for heavy metals in the wetland sediments of Bosten Lake.
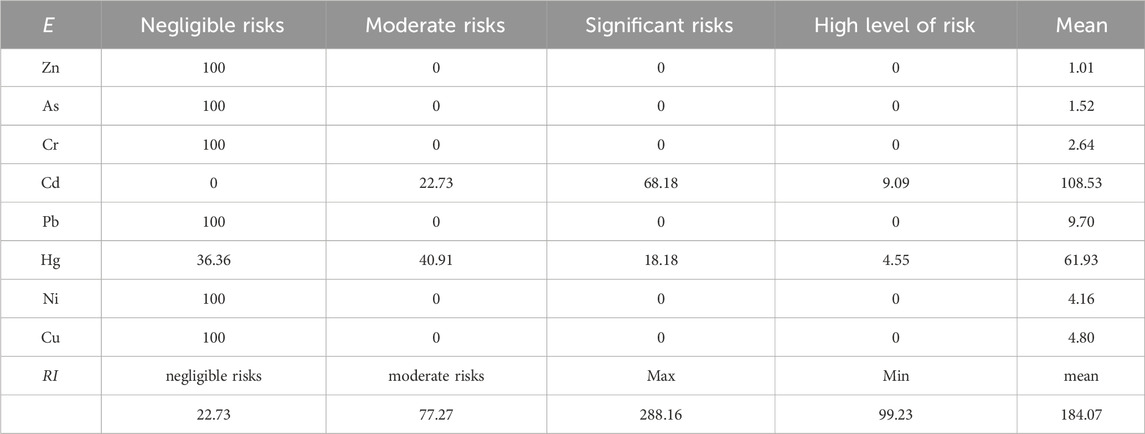
Table 6. Proportion (%) and statistical analysis of the number of sample points of different risk levels in the wetland sediments of Lake Bosten in relation to the total number of sam-ple points.
The average value of the integrated potential ecological risk index (RI) for heavy metal pollution in the wetland sediments of the Bosten Lake Basin was 184.07, signifying a moderate ecological risk. The variation ranged from 99.23 to 288.16. Respectively, 22.73% of the sample points faced a slight risk, while 77.27% faced a moderate risk. According to Hakanson’s evaluation criteria, the predominant potential ecological risk within the study area was moderate, with no incidents of high or severe ecological risk detected. The spatial distribution pattern of RI, illustrated in Figure 4, reveals that RI is unevenly dispersed, with negligible risks identified along the edge of the northern wetland and moderate risks prevalent in all other regions. A comparison of the spatial distribution patterns of Cd and Hg contents shows a correlation between the areas at moderate RI risk and the areas with elevated levels of Cd and Hg. This indicates that Cd and Hg are the principal contributors to the moderate risk level. Due to its higher toxicity coefficient, Cd poses a greater ecological hazard and has a larger impact on ecological risks.
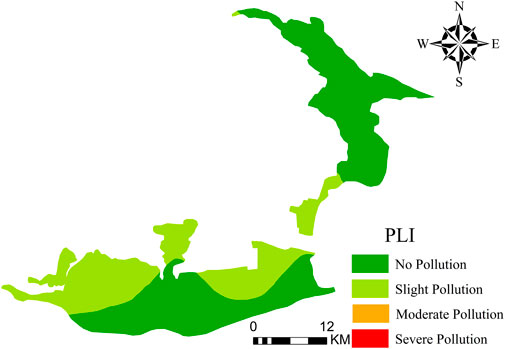
Figure 4. Potential ecological risk distribution of sediment heavy metal synthesis in Lake Bosten wetland.
3.5 Analysis of the sources of heavy metal pollution in wetland sediments of Bosten Lake
3.5.1 Correlation analysis of heavy metal elements in sediments
Correlation analysis and principal component analysis can be used to identify the sources of heavy metals in sediments. The results of correlation analysis among heavy metals showed that (Figure 5), sediment Ni in the study area showed highly significant positive correlation with Cr, Cu, Pb (p < 0.01), and significant positive correlation with Hg (p < 0.05); Hg showed highly significant positive correlation with As, Cu; Cu showed highly significant positive correlation with Zn, As, and Cr; Pb showed highly significant positive correlation with Cr, Cu, and Zn, and significant positive correlation with Zn; Cd showed significant negative correlation with As; and Cd showed significant negative correlation with As. Pb was positively correlated with Cr, Cu and Zn, Cr was positively correlated with Zn, Cr was positively correlated with Zn, and As was positively correlated with As; Cd was negatively correlated with As. The strong correlation between the heavy metal elements suggests that these elements may have com-mon anthropogenic and natural sources of pollution.
3.5.2 Principal component analysis (PCA) of heavy metal elements in sediments
Principal component analysis (PCA) was conducted to elucidate the sources of heavy metal pollution in the study area, thereby extending the insights gained from correlation analysis (Han et al., 2022). The Kaiser-Meyer-Olkin (KMO) statistic and Bartlett’s test of sphericity were employed to verify the suitability of PCA for the heavy metal distribution data across eight sediment samples. With a KMO value of 0.706 and a sphericity test yielding a significance level of zero, the results confirmed that the heavy metal elements were interrelated rather than independent, meeting the necessary prerequisites for PCA. Subsequent PCA with varimax rotation on the dataset identified four principal components with eigenvalues of 3.475, 1.525, 1.335, and 1.331, cumulatively accounting for 95.828% of the variance in data; these findings substantively under-score the sources of heavy metal pollution in the region, detailed in Table 7.
The contribution of the first component (PC1) was 43.433%, in which four elements, Zn, Cu, Pb and Ni, had high loading values, indicating that the five heavy metal elements in the wetland sediments for Lake Bosten had similar sources. Against the results of descriptive statistics (Table 1), the mean values of Zn, Cu, and Ni were close to or slightly larger than the background values for Xinjiang soils, and the correlation between these elements was also strong (Figure 5), with a consistent pattern of contamination distribution (Figure 3). It has been shown that the main sources of Cu, Ni, and Zn are industrial activities, agriculture, and domestic garbage discharge, etc. (Wang et al., 2022), and the settlements around the wetland were distributed in the edge of the wetland in a point-like manner, and at the same time, with the development of the tourism industry, the tourists have little awareness of environmental protection, and the frequent dis-charge of domestic sewage affects the concentration of the heavy metals to a certain degree, and at the same time, Pb was identified as the transportation source, and the wetland sampling process found that transportation routes surrounded the edge of the wetland. During the wetland sampling process, it was found that the major transportation routes surrounded the edge of the wetland. Since the 1970s, the use of leaded gasoline has been restricted globally, but it is still an important source of lead pollution (Del Rio-Salas et al., 2012; Zhu et al., 2001), while some local villagers still keep the leaded gasoline vehicles as a means of agricultural transportation, which suggests that PC1 is a mixed source of transportation and domestic waste emissions.
The contribution of the second component (PC2) was 19.062%, in which As and Cr had high positive loadings, and the loadings of As were significantly higher than those of Cr. The study showed that Cr and As were significantly correlated with soil-forming matrices, soil-forming processes and geological activities (Zhang et al., 2022; Li W. D. et al., 2019), and the correlation between Cr and As was significant, and the contents of As in all the sample sites were much smaller than the background values of Xinjiang soils. Some studies have shown that As is significantly correlated with natural soil-forming matrices or soil-forming processes, but natural leaching causes the whole As content in the surface soil to be continuously exported, which results in the topsoil As content being lower than the background value (Zhao et al., 2022; Chen et al., 2022), suggesting that PC2 is a natural background source, in addition to which the mean value of Cr is slightly greater than the background value of Xinjiang soils, and it also occupies a larger proportion in PC1, suggesting that in addition to the No. 1 matrices source, Cr is also affected by the transportation and domestic waste emission sources, which is also similar to the results of Maimaiti Tursun’s study in the cultivated area of Bosten Lake (Maimaitituerxun et al., 2017).
With a 16.345% contribution to variance, PC3 includes solely Cd with notably high positive loading, indicative of a distinctive and singular pollution profile. Cd’s significant negative correlation with As and a concentration notably exceeding the regional background for soils point towards its isolated behavior. Research denotes Cd as a signature element of agricultural activities, where the prolonged misuse of agricultural inputs, such as fertilizers, culminates in Cd accumulation, harmonized with mechanical wear from agricultural machinery contributing to Cd-laden soil deposition (Zhang et al., 2018; Hu et al., 2022). The prevalence of farmland cultivating chili peppers around the study zone, alongside the rising scale and intensity of agricultural practices, affirms agricultural input as a principal factor for Cd enrichment in sediment, categorizing PC3 predominantly as an agricultural activities source.
Finally, the fourth principal component (PC4), also contributing 16.345% to the variance, revealed a significant positive loading exclusively for Hg. Hg enrichment correlates with coal combustion and non-ferrous metal smelting as primary pollution sources (Wang et al., 2021) Considering the Xinjiang locale, characterized by a temperate continental climate and coal-dependent heating practices among residents, atmospheric deposition and surface runoff are the main pathways for Hg-laden particles and gases to reach and accumulate in sediments, identifying PC4 as indicative of fossil fuel combustion.
3.5.3 Positive Matrix Factorization (PMF) model
The origins of Zn, As, Cd, Cr, Cu, Pb, Hg, and Ni pollution were investigated by employing the Positive Matrix Factorization (PMF) model. After numerous iterations, the optimal number of factors was determined to be four, as shown in Figure 6A. PMF revealed four pollution sources for the heavy metals under scrutiny. Zn, Cu, Pb, and Ni predominantly originated from mixed sources of transportation and domestic waste emissions, with contribution rates to Factor 1 of 51.50%, 50.70%, 41.5%, and 40.30%, respectively. Hg was primarily attributed to fossil fuel combustion, contributing 71.4% to Factor 3. Cd was linked to agricultural activities, with a 71.8% contribution to Factor 4. As and Cr largely emanated from natural sources, with As contributing 80.60% to Factor 2 and Cr showing significant contributions of 40.20% and 31.70% to Factors 1 and 2, respectively. This aligns with PCA findings, corroborating the presence of mixed traffic and domestic waste emissions in addition to natural sources for Cr. Consequently, Factor 2 is likely a natural source.
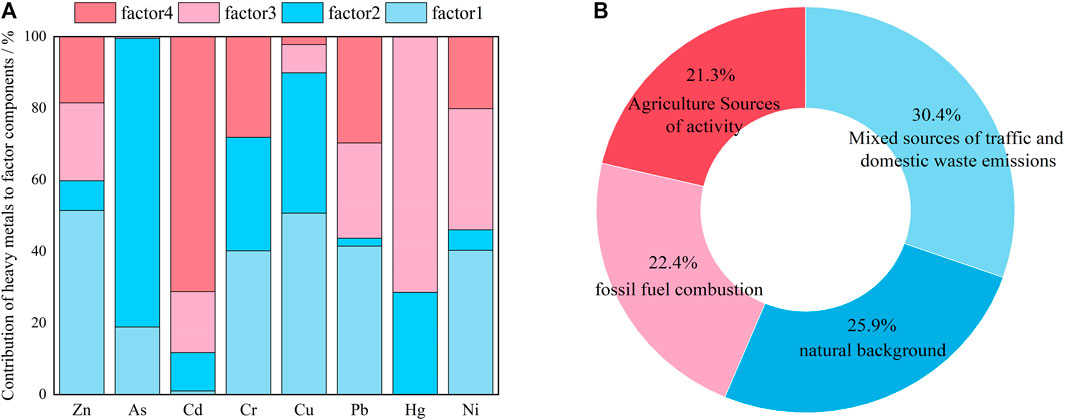
Figure 6. Sediment heavy metal PMF analysis pollution source composition spectrum (A) and its component contribution (B).
Considering the overall contribution to heavy metal pollution in sediments across the study area (Figure 6B), mixed transportation and domestic waste discharges, natural sources, fossil fuel combustion, and agricultural activities accounted for 30.41%, 25.88%, 22.40%, and 21.31% of the pollution, respectively. This implies that anthropo-genic sources in the Lake Bosten Wetland sediments have a more substantial impact than natural sources.
4 Conclusion
The average values of Zn, Cd, Cr, Pb and Hg in the sediments of Lake Bosten are higher than the background values of soil elements in Xinjiang, and As, Cu and Ni are lower than the background values of soil.
The spatial distribution pattern of environmental risks reflected by the CF values of eight heavy metals in the wetland sediments of Bosten Lake is different, and the order of the average CF values of each heavy metal element is as follows: Cd > Pb > Hg > Cr > Zn > Cu > Ni > As, Cd belongs to the heavy pollution and contamination, Pb, Hg, Cr, and Zn are mildly contaminated, Cu and Ni are mildly contaminated, and As is not contaminated. Cd, Pb, Hg are polluted areas and high degree of pollution and Hg are polluted area and high degree of pollution, which to some extent increased the degree and scope of heavy metal pollution in wet-land sediments in the study area. The mean value of heavy metal PLI in farmland soil in the study area showed mild pollution.
The mean values of the potential ecological risk indices of each heavy metal in the wetland sediments of Lake Bosten were in the following order: Cd > Hg > Pb > Cu > Ni > Cr > As > Zn. The mean value of the RI in the study area showed moderate ecological risk.
The homology of Zn, Cu, Pb and Ni in the wetland sediments of Bosten Lake is significant, mainly originating from the mixed sources of transportation and domestic garbage discharge; As and Cr are mainly influenced by the natural parent material; Hg mainly originates from fossil combustion; and Cd mainly originates from agricultural activities.
The sources of heavy metal pollution in the sediments of Bosten Lake wetland can be categorized into four types, i.e., mixed sources of traffic and domestic waste discharge, natural parent sources, fossil combustion, and sources of agricultural activities, which contributed 30.41%, 25.88%, 22.40%, and 21.31% to the enrichment of soil heavy metals, with anthropogenic sources contributing more than the natural sources, respectively.
Agricultural activities should be carried out scientifically, the management of discharges from recreational spots should be strengthened, and coal combustion and traffic emissions should be rationalized, so as to ensure the safe use of the wetlands of Lake Bosten and consolidate the ecological environment barrier of the Lake Bosten wetlands.
Data availability statement
The original contributions presented in the study are included in the article/Supplementary material, further inquiries can be directed to the corresponding author.
Author contributions
KW: Writing–original draft, Conceptualization, Data curation, Investigation, Methodology, Project administration, Resources, Software, Validation, Visualization, Writing–review and editing. DA: Funding acquisition, Writing–review and editing. PL: Investigation, Writing–review and editing. CH: Supervision, Writing–review and editing.
Funding
The author(s) declare that financial support was received for the research, authorship, and/or publication of this article. This project was funded by the National Natural Science Foundation Program (42361006).
Acknowledgments
We thank the reviewers for their constructive comments and suggestions that have helped to improve the original manuscript. Thanks are also due to the editorial staff.
Conflict of interest
The authors declare that the research was conducted in the absence of any commercial or financial relationships that could be construed as a potential conflict of interest.
Publisher’s note
All claims expressed in this article are solely those of the authors and do not necessarily represent those of their affiliated organizations, or those of the publisher, the editors and the reviewers. Any product that may be evaluated in this article, or claim that may be made by its manufacturer, is not guaranteed or endorsed by the publisher.
References
Anbumozhi, V., Radhakrishnan, J., and Yamaji, E. (2005). Impact of riparian buffer zones on water quality and associated management considerations. Ecol. Eng. 24 (5), 517–523. doi:10.1016/j.ecoleng.2004.01.007
Bhatti, S. G., Tabinda, A. B., Yasin, F., Mehmood, A., Salman, M., Yasar, A., et al. (2019). Ecological risk assessment of metals in sediments and selective plants of Uchalli Wetland Complex (UWC)-a Ramsar site. Environ. Sci. Pollut. Res. Int. 26 (19), 19136–19152. doi:10.1007/s11356-019-04711-3
Chai, P. H., Dai, Y. R., Liang, W., Chen, S. P., and Wu, Z. B. (2010). Progress of ecological restoration research in the lakeshore zone. Chin. Acad. Eng. Sci. 12 (06), 32–35.
Chen, M., Peng, Y. X., Huang, Y. X., and Zhang, R. D. (2022). Spatial distribution characteristics and source analysis of soil heavy metals in watersheds of typical lead-zinc mining areas in Yangshuo, China. Environ. Sci. 43 (10), 4545–4555. doi:10.13227/j.hjkx.202201127
Del Rio-Salas, R., Ruiz, J., De la O-Villanueva, M., Valencia-Moreno, M., Moreno-Rodríguez, V., Gómez-Alvarez, A., et al. (2012). Tracing geogenic and anthropogenic sources in urban dusts: insights from lead isotopes. Atmos. Environ. 60, 202–210. doi:10.1016/j.atmosenv.2012.06.061
Duan, J., and Tan, J. (2013). Atmospheric heavy metals and Arsenic in China: situation, sources and control policies. Atmos. Environ. 74, 93–101. doi:10.1016/j.atmosenv.2013.03.031
Engin, M. S., Uyanik, A., and Kutbay, H. G. (2015). Accumulation of heavy metals in water, sediments and wetland plants of kizilirmak delta (samsun, Turkey). Int. J. Phytoremediation 17, 66–75. doi:10.1080/15226514.2013.828019
Hakanson, L. (1980). An ecological risk index for aquatic pollution control. A sedimentological approach. Water Res. 14 (8), 975–1001. doi:10.1016/0043-1354(80)90143-8
Han, C. L., Luo, B. S., Chang, C. Y., Deng, Y. R. ., Xiong, J., and Wang, J. (2022). Analysis of the causes of heavy metal pollution in regional agricultural soils based on multiple methods. J. Ecol. Rural Environ. 38 (02), 176–183. doi:10.19741/j.issn.1673-4831.2021.0178
Hu, J., Zhao, X. Y., Wang, T. T., Gou, K. X., and Wang, C. L. (2022). Characterization, evaluation and source analysis of heavy metal distribution in soil of fen river riparian zone in taiyuan city, China. Environ. Sci. 43 (05), 2500–2509. doi:10.13227/j.hjkx.202107258
Jian, Y. F., Yue, F. J., Zhu, Z. Z., Liu, X. L., and Zhang, Y. L. (2023). Analysis of spatial and temporal changes and sources of heavy metals in coastal wetlands around the Bohai Sea. China Environ. Sci. 43 (11), 6025–6038. doi:10.19674/j.cnki.issn1000-6923.20230808.002
Jiao, W., Wei, O., Hao, F., Wang, F., and Liu, B. (2014). Long-term cultivation impact on the heavy metal behavior in a reclaimed wetland, Northeast China. J. Soils Sediments 14, 567–576. doi:10.1007/s11368-013-0812-1
Kalani, N., Riazi, B., Karbassi, A., and Moattar, F. (2021). Measurement and ecological risk assessment of heavy metals accumulated in sediment and water collected from Gomishan international wetland, Iran. Water Sci. Technol. a J. Int. Assoc. Water Pollut. Res. 84 (6), 1498–1508. doi:10.2166/wst.2021.317
Kumar, N., Chandan, N. K., Bhushan, S., Singh, D. K., and Kumar, S. (2023). Health risk assessment and metal contamination in fish, water and soil sediments in the East Kolkata Wetlands, India, Ramsar site. Sci. Rep. 13 (1), 1546. doi:10.1038/s41598-023-28801-y
Li, F., Liu, Y. N., Guo, D. F., Qi, X. T., Zang, S. Y., and Ni, H. W. (2019a). Spatial distribution of heavy metals and potential ecological risks in songjiang wetland, harbin, China. Environ. Sci. Res. 32 (11). doi:10.13198/j.issn.1001-6929.2019.01.10
Li, W., Zhang, N., Xiong, J., Gao, H. T., Sun, J., Zhang, Q. Y., et al. (2022). Characterization of soil heavy metal distribution and evaluation of pollution in Medica wetlands. Appl. Chem. 51 (12), 3514–3518+3523. doi:10.16581/j.cnki.issn1671-3206.20221031.012
Li, W. D., Cui, Y. X., Zeng, C. C., Zhu, Y. Q., Peng, Y., Wang, K., et al. (2019b). Pollution characteristics and source analysis of heavy metals in farmland soils in the taige canal valley. Environ. Sci. 40 (11), 5073–5081. doi:10.13227/j.hjkx.201903252
Li, Y. M., Ma, J. H., Liu, D. X., Sun, Y. L., and Chen, Y. F. (2015). Evaluation of heavy metal pollution and potential ecological risks in kaifeng urban soil. Environ. Sci. 36 (03), 1037–1044. doi:10.13227/j.hjkx.2015.03.037
Liu, H. F., Liu, W., Liu, Y. Y., Liu, C., and Shen, Y. X. (2017). Distribution characteristics of Cu, Ni and Pb in the wetland of Bosten Lake and its ecological risk. Arid. Zone Stud. 34 (02), 390–394. doi:10.13866/j.azr.2017.02.21
Liu, X., and Dilinuer, A. J. (2021). Removal effects of surface flow artificial wetland on heavy metal ions in water body on the west bank of Bosten Lake. Wetl. Sci. 2014 (91), 220–231. doi:10.13248/j.cnki.wetlandsci.01.014
Luo, S. Y., Xing, W. L., Liang, W. L., Deng, Z. Y., Liu, X. L., and Quan, X. W. (2019). Speciation, ecological risk assessment and source analysis of heavy metals in the surface sediments of mangrove wetland in Zhanjiang Bay. Ecol. Environ. Sci. 28 (2), 348–358. doi:10.16258/j.cnki.1674-5906.2019.02.017
Maimaitituerxun, A., Ajiguli, M., Ainiwaer, M., and Ma, G. F. (2017). Evaluation of heavy metal pollution and potential ecological risk of oasis farmland soil in Bosten Lake Basin. Geogr. J. 72 (09), 1680–1694. doi:10.11821/dlxb201709012
Merciai, R., Guasch, H., Kumar, A., Sabater, S., and García-Berthou, E. (2014). Trace metal concentration and fish size: variation among fish species in a Mediterranean river. Ecotoxicol. Environ. Saf. 107, 154–161. doi:10.1016/j.ecoenv.2014.05.006
Ni, X., Zhao, G., Ye, S., Li, G., Yuan, H., He, L., et al. (2023). Spatial distribution and sources of heavy metals in the sediment and soils of the Yancheng coastal ecosystem and associated ecological risks. Environ. Sci. Pollut. Res. 30 (7), 18843–18860. doi:10.1007/s11356-022-23295-z
Ouyang, W., Wang, Y., Lin, C., He, M., Hao, F., Liu, H., et al. (2018). Heavy metal loss from agricultural watershed to aquatic system: a scientometrics review. Sci. Total Environ. 637–638, 208–220. doi:10.1016/j.scitotenv.2018.04.434
Qiao, P., Lei, M., Yang, S., Yang, J., Zhou, X., Dong, N., et al. (2019). Development of a model to simulate soil heavy metals lateral migration quantity based on SWAT in Huanjiang watershed, China. J. Environ. Sci. 77, 115–129. doi:10.1016/j.jes.2018.06.020
Rasendra, T., Md, H. R., Baharim, N. B., and Carnicelli, S. (2021). Source identification and ecological risk assessment of heavy metal pollution in sediments of Setiu wetland, Malaysia. Environ. Forensics 23, 241–254. doi:10.1080/15275922.2021.1892871
Ros, À., Colomer, J., Serra, T., Pujol, D., Soler, M., and Casamitjana, X. (2014). Experimental observations on sediment resuspension within submerged model canopies under oscillatory flow. Cont. Shelf Res. 91, 220–231. doi:10.1016/J.CSR.2014.10.004
Taghvaee, S., Sowlat, M. H., Mousavi, A., Hassanvand, M. S., Yunesian, M., Naddafi, K., et al. (2018). Source apportionment of ambient PM2.5 in two locations in central Tehran using the Positive Matrix Factorization (PMF) model. Sci. total Environ. 628-629, 672–686. doi:10.1016/j.scitotenv.2018.02.096
Tomlinson, D. L., Wilson, J. G., Harris, C., and Jeffrey, D. W. (1980). Problems in the assessment of heavy-metal levels in estuaries and the formation of a pollution index. Helgoländer Meeresunters. 33, 566–575. doi:10.1007/bf02414780
Wang, C. Y., Li, Y. C., Yu, C. G., and Wang, D. P. (2021) Characterization of soil heavy metal distribution and source analysis in northeastern Huludao, China. China Environ. Sci., 41(11): 5227–5236. doi:10.19674/j.cnki.issn1000-6923.20210608.007
Wang, E., Zhang, K., Chang, S., Zhang, M., and Fu, Q. (2023a). Spatiotemporal distribution and pollution risk assessment of heavy metals in sediments of main water supply reservoirs in central zhuhai city. Huan jing ke xue= Huanjing kexue 44 (1), 189–197. doi:10.13227/j.hjkx.202204006
Wang, H., Wang, C. X., Wang, Z. J., and Cao, Z. (2004). Fractionation of heavy metals in surface sediments of taihu lake, East China. Environ. Geochem. Health 26, 303–309. doi:10.1023/b:egah.0000039594.19432.80
Wang, J., Ge, J., Yang, X., Cheng, D., Yuan, C., Liu, Z., et al. (2022). Distribution and ecological risk assessment of heavy metals in sediments of Dajiuhu Lake Wetland in Shennongjia, China. Environ. Sci. Pollut. Res. 30, 25999–26011. doi:10.1007/s11356-022-23952-3
Wang, X. M., Shen, J., Fan, T. Y., Chu, Z. X., Dong, Z. B., Dong, P., et al. (2023b). Characteristics of heavy metal distribution and health risk evaluation of Wuhu inland river water environment. J. Yangtze River Acad. Sci. 12-291-9. doi:10.11988/ckyyb.20230602
Wang, Z. J., Liu, S. J., Zheng, J., and Li, Y. F. (2019). Soil heavy metal pollution and its ecological risk evaluation in Caohai Basin. J. Ecol. Environ. 28 (12). doi:10.16258/j.cnki.1674-5906.2019.12.017
Yang, P., Yang, C. H., Ma, X. Y., and Yin, H. Bl. (2021). Characteristics of sediment pollution and dredging decision in the estuary of Nanfang River, Chaohu Lake. Environ. Sci. 42 (02), 712–722. doi:10.13227/j.hjkx.202005320
Ye, Z., Yang, Y., Zhou, H., and Guo, B. (2020). Ecological water rights of the Bosten Lake wetlands in Xinjiang, China. Wetlands 40, 2597–2607. doi:10.1007/s13157-020-01379-1
Yong, Y. (2002). Main characteristics, progress and prospect of international wetland science research. Prog. Geogr. (2), 111–120.
Yongze, Z., and Xuan, W. (2001). A review of ecological restoration studies on natural wetland. Acta Ecol. Sin. 21 (2), 309–314.
Yuan, H., Liu, E., Shen, J., Zhou, H., Geng, Q. F., and An, S. (2014). Characteristics and origins of heavy metals in sediments from Ximen Co Lake during summer monsoon season, a deep lake on the eastern Tibetan Plateau. J. Geochem. Explor. 136, 76–83. doi:10.1016/j.gexplo.2013.10.008
Zhang, M. Y., Cui, L. J., and Sheng, L. X. (2007). Pollution and ecological risk assessment of heavy metals in the Hengshuihu Wetland. Wetl. Sci. 5 (4), 362–369. doi:10.13248/j.cnki.wetlandsci.2007.04.012
Zhang, S., Ye, H., Zhang, A., Ma, Y., Liu, Q., Shu, Q., et al. (2022). Pollution characteristics, sources, and health risk assessment of heavy metals in the surface soil of lushan scenic area, jiangxi Province, China. Front. Environ. Sci. 10. doi:10.3389/fenvs.2022.891092
Zhang, Z., Lu, Y., Li, H., Tu, Y., Liu, B., and Yang, Z. (2018). Assessment of heavy metal contamination, distribution and source identification in the sediments from the Zijiang River, China. Sci. total Environ. 645, 235–243. doi:10.1016/j.scitotenv.2018.07.026
Zhao, X. L., Li, X., Lu, X. B., Wang, T. ., Zhang, S. L., Guo, X. C., et al. (2022). Characterization of heavy metal contamination in surface sediments of Dongjiang Lake and evaluation of potential ecological risk. Environ. Sci. 43 (06), 3048–3057. doi:10.13227/j.hjkx.202109123
Zheng, G. G. (2017) Theory and practice of research on heavy metal pollution of agricultural soil. Beijing: China Environmental Science Publishing House.
Zhou, F., Li, P., Wen, M. Z., Xin, X. L., Liu, L., Zhang, Y., et al. (2023). Ecological risk assessment of heavy metals in sediments of Longyang Lake and Moshui Lake, wuhan, China. China Environ. Sci. 43 (10), 5433–5443. doi:10.19674/j.cnki.issn1000-6923.2023.0179
Keywords: wetlands, sediment heavy metals, Xinjiang, pollution assessment, source analysis, ecological risk
Citation: Wang K, Aji D, Li P and Hu C (2024) Characterization of heavy metal contamination in wetland sediments of Bosten lake and evaluation of potential ecological risk, China. Front. Environ. Sci. 12:1398849. doi: 10.3389/fenvs.2024.1398849
Received: 10 March 2024; Accepted: 19 April 2024;
Published: 07 May 2024.
Edited by:
Qi Liao, Central South University, ChinaCopyright © 2024 Wang, Aji, Li and Hu. This is an open-access article distributed under the terms of the Creative Commons Attribution License (CC BY). The use, distribution or reproduction in other forums is permitted, provided the original author(s) and the copyright owner(s) are credited and that the original publication in this journal is cited, in accordance with accepted academic practice. No use, distribution or reproduction is permitted which does not comply with these terms.
*Correspondence: Dilinuer Aji, ZGlsbnVyQHhqbnUuZWR1LmNu