- 1Mitra SK Pvt Ltd, Udayan Industrial Estate, Beliaghata, India
- 2School of Science, Engineering and Environment, University of Salford, Salford, United Kingdom
- 3TLabs, Tea Research Association, Kolkata, India
- 4Department of Chemistry, University of Kalyani, Kalyani, India
- 5Department of Agricultural Chemicals, Bidhan Chandra Krishi Viswavidyalaya, Mohanpur, India
- 6Department of Life and Environmental Sciences, Bournemouth University (Talbot Campus), Fern Barrow, United Kingdom
Fluazinam a promising fungicide, is not yet registered in India. Consequently it is important to study the dissipation of its specific formulation in Indian soil and water. This study focuses on the degradation and residue dynamics of Fluazinam (40% SC) in different soil types (alluvial, lateritic, coastal saline and black) and water pH (4.0, 7.0, 9.2). Adsorption kinetic models suggested that the half-life period (days) varies among soils following the order lateritic (Jhargram), 54.07 > alluvial (Mohanpur), 45.10 > coastal saline (Canning), 28.33 > black (Pune) 26.18. These differences are attributed to soil pH and organic carbon (OC) content, where higher pH levels reduce pesticide adsorption, leading to quicker dissipation, while higher organic carbon content provides more binding sites, slowing down the process. The first order kinetics explained the dissipation better compared to second order model across all soil types. The study also found that the half-life of was lowest at pH 9.2, as compared to pH 7.0, and very high stability at pH 4.0. Additionally, the study introduces an interactive R-based tool for analysing dissipation kinetics and half-life of different pesticides offering a valuable resource for researchers and stakeholders.
1 Introduction
Fungal diseases are a common occurrence on plants, often having a significant economic impact on yield and quality, thus managing diseases is an essential component of production for most crops. Fungicides are often a vital part of disease management as they control many diseases satisfactorily, cultural practices often do not provide adequate disease control, and resistant cultivars are not available or not accepted in the market (El-Baky and Amara, 2021; Peng et al., 2021). Fluazinam is a specific type of pesticide that controls fungal disease by specifically inhibiting or killing the fungus causing the disease (Peng et al., 2021).
In this context, Fluazinam plays a crucial role in controlling fungal diseases in crops. Fluazinam is a broad-spectrum fungicide that has been used in agriculture since 1992. It is a diarylamine and more specifically an arylaminopyridine (NCBI, 2023) group of molecules. The mode of action of Fluazinam is preventive contact with a multi-site mode of action that remains primarily on the plant surface and kills any fungal spores that encounter it. It has protectant activity against a range of plant pathogenic fungi including Rhizoctonia spp., Pyricularia spp. and Phytophthora spp. in paddy and potato crops, etc (Roberts and Hutson 1999). Fluazinam is not taken up to any extent by the plant and, unlike systemic fungicides, is not translocated within the plant (Chen et al., 2020). Fluazinam serves as a versatile contact fungicide, with applications possible through foliar spray or soil treatment. Its efficacy extends to combat various pathogenic fungi responsible for specific diseases, including gray mold and downy mildew in grapes, melanose and mites in citrus, scab and alternaria blotch in apples, clubroot in crucifers, sclerotinia blight in peanuts, as well as white root rot and violet root rot in fruit trees (Hu et al., 2020). Notably, it is renowned for its exceptional protection against Foliar blight, tuber blight, and sclerotinia rot in potatoes caused by the Phytophthora infestans fungus, making its impact on potatoes unparalleled (Sedlak et al., 2022). Studies have indicated that unlike in fungi (where it targets ATP synthase), Fluazinam does not have specific target sites in non-target species, but it affects gene expression profiles (Saifullah et al., 2022). Fluazinam persists in soil for a long time, and its degradation is enhanced by an abundance of soil organic matter (SOM) warm temperature, and wetness. Fluazinam is hydrolyzed to 5-Chloro-6-(3-chloro-2,6- dinitro-4- trifluoromethylanilino) nicotinic acid (CAPA), which is then steadily degraded to 6-(4-Carboxy-3-chloro-2,6- dinitroanilino)-5- chloronicotinic acid (DCPA), (FAO and WHO, 2019).
The degradation of pesticides in soil is mainly dependent on various mechanisms like microbial degradation, chemical hydrolysis, photodegradation, volatility, leaching, surface runoff, etc. (Patra et al., 2022). Among the various forces, laboratory studies suggest that degradation in soil mainly occurs due to aerobic microbial activity. It is also observed that dissipation of pesticides in field condition depends on the pH of surface water and soils of different Agroclimatic zones (Roberts and Hutson 1999; Pal et al., 2006). An experiment was undertaken to directly assess the effect of soil organic matter (SOM) on the behavior of Fluazinam. The study found that Fluazinam persisted in soil for a long time, and its degradation was enhanced by an abundance of SOM, warm temperature, and wetness (Hakala et al., 2020).
Additionally, in over half of soil samples collected from boreal forests, Fluazinam was detected at concentrations above the limit of quantification (Hakala et al., 2020). The laboratory study gives the primary information on the persistence behaviour of a pesticide, which may follow similar trends in field studies. However, there are knowledge gaps regarding the behaviour of Fluazinam in different types of soil (Jain et al., 2019). Being a broad-spectrum fungicide, Fluazinam is effective against a wide range of fungal diseases. This makes it a valuable tool for farmers who are growing multiple crops, as they can use the same fungicide to control diseases on different crops like potato, oilseed, groundnut and hence its high potentiality for use in India. Initially a study was conducted in the United Kingdom, Germany, and the United States to investigate the field dissipation of Fluazinam. However, the United States field trials were considered not relevant for EU conditions and were not used in the risk assessment (EFSA, 2008). The dissipation dynamics of Fluazinam have been investigated in other regions (Feng et al., 2015). A study conducted in China investigated the dissipation and residues of Fluazinam in potatoes, potato plants, and soil. The study found that Fluazinam dissipation fitted first-order kinetics, and the half-lives in potato plants and soil were 3.3–5.4 and 9.4–9.5 days, respectively (Chen et al., 2018). Recently the residue levels of fluazinam in root mustard using a QuEChERS technique with ultra-performance liquid chromatography tandem mass spectrometry was undertaken by Chen et al. (2023). The recoveries of fluazinam were 85.2%–110.8% for leaf mustard and 88.8%–93.3% for root mustard. The risk quotient (RQ) was 72.2%–74.3% for ordinary consumers, indicating negligible risk. Based on the maximum residue limit (MRL) and dietary risk assessment, a pre-harvest interval of 3 days and an MRL of 2 mg kg−1 were suggested for fluazinam in root mustard.
The study of the dissipation of Fluazinam in Indian soil and water is crucial, even though it is not a registered pesticide in India. However, the attempts of registering this pesticide in India by companies, along with the chosen formulation, is the underlying context for this study, emphasizing the importance of conducting the research. Fluazinam is a fungicide widely used in many countries, and understanding its behaviour in different environmental conditions can provide valuable insights for its potential future use or risk assessment in India. Hence, understanding the dissipation of Fluazinam in Indian soil and water should involve similar methodologies, tailored to local conditions. This knowledge could inform decisions about the safe and effective use of this pesticide, should it ever be considered for registration in India. In this context, the present study has been designed to investigate the persistence/fate of Fluazinam formulation (40% SC), in soil at different days intervals. To investigate the persistence nature of Fluazinam 40% SC in different soil types, a residue study in lab condition was conducted. Further persistence nature of Fluazinam 40% SC was investigated after application at different rates in water maintained at different pH viz. acidic (pH 4.0), neutral (pH 7.0) and alkaline (pH 9.2).
Further, our research has led to the development of a novel Shiny application in R, which has significantly enhanced the efficiency and accuracy of dissipation analysis. This application has successfully streamlined data processing, improved the visualization of dissipation patterns, and facilitated more robust statistical analysis, ultimately contributing to more reliable and reproducible results in our study. To the best of our knowledge, there is currently no such application available in the public domain. This application fills a significant gap in the field, as it provides researchers with a much-needed tool for conducting comprehensive and efficient dissipation analysis. The development of this application underscores our commitment to advancing research methodologies and promoting open science.
2 Methods
2.1 Collection of soil samples
For the incubation study four types of soils, namely, new alluvial soil (Inceptisol), red and lateritic soil (Alfisol), saline soil (Inceptisol) and black soil (Vertisol) were used for the purpose. The details of the physio-chemical properties of the experimental soils have been depicted in Supplementary Table S1.
2.2 Fortification of soil samples with fluazinam 40% SC
Two doses of Fluazinam 40% SC, namely, 1, 2 μg g−1 of soil and control was used for the purpose and were designated as T1, T2 and T3 respectively. The 12-treatment combination (4 soil types and 3 doses) was kept at 25°C ± 2°C throughout the incubation period. Soil samples (20 g) were taken in 250 mL conical flasks to form a set for each type of soil. Three replicate flasks for each treatment were taken for analysis on each day of sampling along with untreated control. Samples (three replicates) were processed for analysis of Fluazinam residues at intervals of 0, (2 h) after application, 3, 7, 10, 15, 30, 45, 60 and 90 days after application.
2.3 Fortification of fluazinam 40% SC in aqueous solution at different pH
Buffer capsules of pH 4.0, 7.0 and 9.2 were used for this pH study. One capsule is required for 100 mL of distilled water (Specific conductivity <1.00 µmhos/cm at 25”C, Grade II water) to maintain the above-mentioned pH. In a series of 250 mL conical flask 200 mL distilled water was taken and two capsules of different pH were added to each of the conical flask separately. The conical flasks were then left at room temperature for overnight for homogeneous mixing. Two (Chelme-Ayala et al., 2005) and four (Chen et al., 2023) mL from diluted 40% SC Fluazinam solution (100 mg L−1) of was added separately to 200 mL water to achieve a final concentration of 1 μg mL−1 (T1) and 2 μg mL−1 (T2). A subsequent pH check was conducted to confirm the pH of the aqueous solution. Each treatment was replicated thrice along with untreated control.
After application of Fluazinam 40% SC solution separately to different water sample maintained at different pH (4.0, 7.0 and 9.2), water samples were collected at 0 (after 2 h of spiking) 3, 7, 10, 15, 30, 45, 60 and 90 days interval. Control water samples were also collected in the same day for each type of water.
2.4 Analysis of fluazinam residues
2.4.1 Standard preparation
An analytical standard with 99.7% purity, supplied by M/s UPL (United Phosphorous Limited), Mumbai and also purchased from Sigma-Aldrich, was used to prepare the standard solution. Ten milligrams of Fluazinam (analytical grade) were placed in a 100 mL volumetric flask. The flask was filled to the mark with HPLC-grade acetonitrile to get a 100 mg L−1 stock standard solution. Necessary dilutions were made from this standard as needed. For the Fluazinam 40% SC formulation, 1 mL was taken and placed in a 1,000 mL volumetric flask. The flask was filled to the mark with HPLC-grade acetonitrile to prepare a 400 ppm stock standard solution. Necessary dilutions were made from this standard as needed.
2.4.2 Extraction and cleanup
2.4.2.1 Water samples
The representative samples (100 mL) were taken in a 500 mL separatory funnel and partitioned with 100 mL hexane. The hexane phase was collected over anhydrous sodium sulphate. Again, the water was re extracted twice with 50 mL of hexane in each time and again the organic phase was collected in 250 mL conical flask. The organic phase was immediately evaporated to dryness in a rotary vacuum evaporator at 45°C. The residue was reconstituted in Acetonitrile and filtered by syringe filter for final HPLC analysis.
2.4.2.2 Soil samples
Soil samples in the respective sampling dates were added with 100 mL mixture of Methanol: acidic water (8:2), kept for overnight and were shaken for a period of 30 min using a mechanical shaker (25°C). The acidic water was 0.2(M) HCL solution. It was then filtered, and extract was collected and re-extracted the sample using 100 mL mixture of Methanol: acidic water (8:2). Combined filtrate was transferred to a 500 mL separatory funnel. This mixture was partitioned thrice (100 + 50+50) mL with Hexane. Hexane fraction was collected over anhydrous Na2SO4. This combined fraction was concentrated in Rotary Vacuum Evaporator at 45°C. Hexane fraction was evaporated to near dryness in a rotary vacuum evaporator and reconstituted in HPLC grade acetonitrile for HPLC analysis.
2.5 HPLC-UV or instrumentation
Fluazinam was detected by Agilent HPLC model HP 1050 (pump) equipped with Agilent 1,100 Series UV detector coupled with HPLC 1100 software. The HPLC operating parameters employed in this study include a column with dimensions of 250 × 4.6 mm, specifically the Thermo Hypersil ODS make with a 5µ (RPC) particle size. The mobile phase consisted of acetonitrile and water in a ratio of 9:1, adjusted to a pH of 3 with phosphoric acid. The flow rate was maintained at 1 mL min−1. The detector wavelength (λmax) was set at 236 nm. The retention time of Fluazinam, a compound under investigation, was determined to be 4.93 ± 0.2 min. The analytical performance was characterized by a limit of quantification of 0.10 μg mL−1 and a limit of detection of 0.05 μg mL−1, providing essential parameters for the accurate analysis of the targeted substance. A linearity check was carried out with the help of the analytical standard. From the stock solution of 100 mg L−1, 0.1, 0.5, 1.0, 2.0 and 5 mg L−1 concentrations were prepared. 20 μL of each sample were injected and the corresponding area were calculated. A calibration curve was prepared with an R2 of 0.99. Considering the lower detection limit (LOD) of the instrument dissipation data below the LOD was represented as below detection limit (BDL). The LOD is determined up to 0.05 ppm Fluazinam and LOQ is 1.0 ppm. The chromatograms for analytical standard of fluazinam, untreated control water sample, water sample (spiked with fluazinam), untreated control soil sample and soil sample spiked with fluazinam can be observed from Supplementary Figure S1, S2 respectively. The Recovery study was done in three different pH solutions (4.0, 7.0.9.2) and the results were varied from 85%–90%
2.6 Kinetics modelling and data analysis
The kinetics data was analysed using R Studio (version: 2023.09.1 Build 494). For linear kinetics modelling and plots the “stats” (version 4.3.2) package was used. The dissipation data represented as BDL was not considered during kinetic analysis. The box and bar plots from the dissipation data was prepared using the “ggpubr” (version 0.6.0) was used. All the codes along with the outputs have been attached as separate Supplementary Material using the “rmarkdown” (version 2.25) and “knitr” (version 1.45) packages.
2.6.1 Kinetic models
2.6.1.1 First order kinetics model (FO)
where the concentration of a pesticide decreases over time (Fantke and Jursake, 2013). It assumes that the reaction rate is directly proportional to the concentration of the pesticide. In this model (Eq. 1),
2.6.1.2 Second order kinetics model (SO)
Second order kinetics is used when the reaction rate is proportional to the square of the pesticides concentration (Fantke and Jursake, 2013). This model assumes that the reaction occurs when two molecules come into contact and collide. This is dependent on the interaction of the pesticide with another substance in the environment (e.g., a degradant, another chemical, or a catalytic surface in the soil or water).
2.6.2 Coefficient of determination (R2)
R-squared is a statistical measure that represents the proportion of the variance in the dependent variable y that is explained by the independent variables or predictors in a regression model (Eq. 5). It is a value between 0 and 1. An R-squared value of 0 indicates that the model does not explain any of the variance, while a value of 1 means that the model explains all the variance. In simple terms, R2 quantifies how well the model fits the data. A higher R2 suggests a better fit, but it should be used in conjunction with other evaluation metrics such as root mean squared error (RMSE), as a high R2 does not necessarily mean the model is good.
In equation, (Chen et al., 2020), n represents the number of data points,
2.6.3 Application development
The application was developed using R (version: 2023.09.1 Build 494) and the Shiny package (version: 1.7.5.1). Additional R packages used include “readxl” (version:1.4.3) and “ggplot2” (version: 3.4.4).
The application was developed following the structure of a basic Shiny app, which consists of two main components: the user interface (ui) and the server. The ui object controls the layout and appearance of the application. The server function was written to reactively respond to changes in the input elements and update the output elements accordingly. Finally, the shinyApp function was used to create the Shiny app object through an explicit ui/server pair. The application was thoroughly tested to ensure it works as expected. This involved checking that all input and output elements function correctly, and that the app does not produce any errors when given unexpected inputs. The detailed codes and a “readme” have been provided as Supplementary Material. All the codes have been attached as separate Supplementary Material using the “rmarkdown” (version 2.25) and “knitr” (version 1.45) packages.
3 Results
3.1 Dissipation from soil under laboratory simulated condition
A comparison of the residue of Fluazinam across different soil types irrespective of the dose and days of incubation has been depicted in Supplementary Figure S3A. The mean values of residue from alluvial, lateritic, coastal saline and black soil were 0.86, 0.93, 0.76 and 0.71 respectively. From the non-parametric Kruskal–Wallis test it was observed that the soil types have no significant (p > 0.05) impact on it is residue. The mean residue of Fluazinam was significantly affected by the dose as can be observed from Supplementary Figure S3B. The non-parametric Wilcox test revealed a statistically significant (p < 0.05) difference between T1 and T2. The mean residue values of Fluazinam in four different soil types across different doses and days interval is depicted in Supplementary Figure S4A–D. From the Figure it was revealed that Fluazinam dissipates linearly with progress of time. The rate constant, half-life and coefficient of determination (R2) from FO and SO for the four soil types and different pH of water have been depicted in Table 1. The plots of the FO adsorption kinetic models can be observed in Figure 1A,B, for SO Figures 2A,B). From Table 1 from the better fisting FO model the half-life (days) for T1 followed the order lateritic (Jhargram), 54.07 > alluvial (Mohanpur), 45.10 > coastal saline (Canning), 28.33 > black (Pune) 26.18. The same trend was observed for T2 with half-life of 54.42 days for lateritic followed by alluvial (46.16 days), coastal saline (29.49 days) and 27.54 days for black soil. In case of lateritic soil (Jhargram) the initial deposit and half-life value ranges between 0.91–1.83 µg g−1 and 54.07–54.42 days respectively for recommended T1 dose and double the recommended T2. For this soil, more than 50% of initial deposits were dissipated within 60 days. In alluvial soil (Mohanpur), the initial deposit and half-life value of Fluazinam ranges between 0.91–1.80 μg g−1 and 45.10–46.16 days respectively for recommended T1 dose and double the dose T2. More than 50% of initial deposits were dissipated within 50 days in both the cases. For coastal saline soil (Canning) the initial deposit and half-life value ranges between 0.93–1.90 μg g−1 and 28.33–29.49 days respectively for recommended T1 dose and double the recommended T2 dose. In both the cases more than 50% of initial deposits were dissipated within 30 days irrespective of the treatments. In case of black soil (Pune) the initial deposit and half-life value ranges between 0.9–1.80 μg g−1 and 26.18–27.54 days respectively for recommended T1 dose and double the recommended T2 dose. More than 50% of initial deposits were dissipated within 30 days irrespective of the treatments.
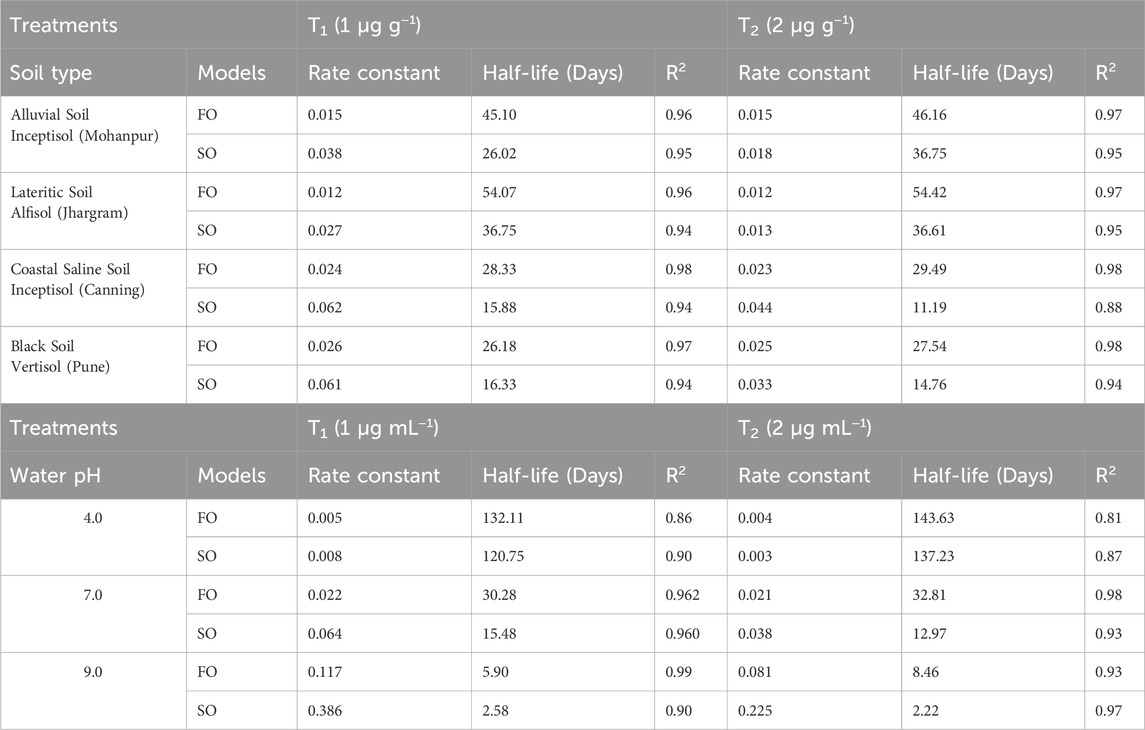
Table 1. Rate constant, half-life period and coefficient of determination (R2) from First Order (FO) and Second Order (SO) kinetics model from laboratory incubation studies of Fluazinam in soil and water.
3.2 Dissipation in water under laboratory simulated condition
Supplementary Figure S5A represents a comparison of the recoveries of Fluazinam from water at different pH irrespective of treatment and days of incubation. The mean values of recoveries at pH 4, 7 and 9.2 were 1.03, 0.69 and 0.36 μg mL−1 of water respectively. From non-parametric Kruskal–Wallis test it was observed that the effect of water pH on recovery of Fluazinam was statistically significant (p < 0.05). From Supplementary Figure S5B it was observed that the recovery was significantly (p < 0.05) affected by the dose of Fluazinam. Effect of pH on the dissipation of Fluazinam at different treatment levels and days intervals is depicted in Supplementary Figure S6A–C. From the figure it was revealed that Fluazinam dissipates linearly with progress of time. No residue was obtained in the untreated control throughout the study.
For different pH of water, the plots (representing the actual and predicted values) of FO and SO are showed in Figure 3A,B and Figure 4A,B respectively. These results showed that the dissipation of fluazinam varied across different soil types and treatments. The rate constant, half-life, and R2 values provide insights into the rate of dissipation, the persistence of the substance in the soil, and the fit of the model to the data, respectively. The results in Table 1 showed that the FO kinetics better explained the dissipation of fluazinam compared to SO kinetics, having higher R2 values of the former for all the soil types and dose. From Table 1 it was observed that the SO better explained the dissipation kinetics of fluazinam at pH 4.0 compared to FO, the former having higher R2 values at both the doses. The half-life at pH 4.0 were 120.75 and 137.23 days for T1 and T2 respectively. For pH 7.0 the dissipation kinetics was better explained by the FO (having higher R2) compared to SO for both the does resulting the half-life of 30.28 and 32.81 for T1 and T2 respectively. In case of pH 9.0 at T1 the dissipation of fluazinam followed the FO (R2 = 0.99) kinetics compared to SO (R2 = 0.90) resulting a half-life of 5.90 days. For T2 the SO (R2 = 0.97) explained a better kinetics compared to FO (R2 = 0.93) resulting a half-life of 2.22 days. The half-life value shows that stability of Fluazinam was lowest at pH 9.2, as compared to pH 7.0, and very high stability at pH 4.0.
3.3 Application for dissipation kinetics
In this study, we developed a user-friendly application using Shiny in R for the analysis of dissipation kinetics. Link to the application is as follows: https://jajatimandal.shinyapps.io/Half_Life_Hero/
This interactive tool will allow users to input their own data and select from kinetic models, including First Order and Second Order models, to best fit their data.
4 Discussion
Both the FO and SO models were used to describe the kinetics of various processes, including the dissipation of pesticides in soil. In a FO reaction, the rate of reaction is proportional to the concentration of only one reactant. In a SO reaction, the rate of reaction is proportional to the square of the concentration of one reactant or to the product of the concentrations of two reactants. Further the FO is a simpler compared to SO which made it easier to fit the data. The better fit to FO is probably due the involvement of diffusion-based mechanisms. Further, Zhao et al., in (2019) reported a better fit of FO dissipation kinetics for fluazinam across six locations in China. The maximum dissipation was observed in the soils having a higher pH and OC content like alluvial (pH = 7.02, OC = 1.00%), costal saline (pH = 7.60, OC = 1.03%) and black (pH = 8.14, OC = 0.67%) compared to lateritic soil (pH = 5.45, OC = 0.64%). This resulted in a higher half-life of fluazinam in lateritic soil compared to black soil. This could be since both pH and OC content can affect the adsorption and desorption of pesticides in the soil. Higher pH can increase the negative charge of soil particles, which might reduce the adsorption of certain pesticides, leading to faster dissipation. Similarly, soils with higher OC content can have more binding sites for pesticides, which can also affect their behaviour in the soil. As a result, the half-life of fluazinam was found to be higher in lateritic soil compared to black soil. Half-life is an important parameter in understanding the persistence of a pesticide in the environment. Previously soil organic matter enhancing the degradation of Fluazinam in soil and additionally, the persistence of fluazinam in soil is influenced by temperature and wetness was reported by Hakala et al. (2020) in soils under boreal conditions. Degradation of Fluazinam in soil might be due aerobic soil degradation, soil photolysis, aqueous photolysis as reported by FAO and WHO (2019). The half-life of Fluazinam ranged from 17–56 days for sandy loam soil (FAO, 2018). Results from photo-degradation study in loamy sand soil using [C14]-fluazinam revealed that the half-life for net photo degradation of Fluazinam were 32 and 21 days for the phenyl and pyridyl labels respectively (FAO and WHO, 2019).
The results of dissipation of Fluazinam in water revealed the stability in acidic compared to alkaline pH. Previously in a study, the hydrolytic stability of Fluazinam, a fungicide, was examined under varying pH and temperature conditions. Fluazinam remained stable at pH 4 for 5 days at 50°C but proved to be hydrolytically unstable at pH 7 and 9 when stored for extended periods. Under these conditions, Fluazinam underwent hydrolysis to form degradation products, predominantly 5-chloro-6-(3-chloro- α,α,α-trifluoro-2,6-dinitro-p-toluidine) –nicotinic acid (CAPA), with concentrations exceeding 90% of the initial amount at pH 7°C and 25°C. At pH 7°C and 50°C, both CAPA and 6 -(4- carboxy-3-chloro-2,6-dinitroaniline)-5-chloronicotinic acid (DCPA) were generated, with DCPA accounting for up to 71% and CAPA for up to 29% of the initial amount at the end of the incubation period. The study also found comparable hydrolysis at pH 9 to that observed at pH 7. Additionally, the calculated half-life values at pH 7°C and 25°C ranged from 2.7 to 4.5 days, indicating relatively quick degradation, while at pH 9°C and 25°C, DT50 values ranged from 3.5 to 3.9 days, suggesting a similar degradation rate (Chelme-Ayala et al., 2005; FAO and WHO, 2019).
Additionally, the whole probable mechanism and the dissipation of Fluazinam in basic medium can be justified from Figure 5. Fluazinam in presence of OH- ion is outlined in Figure 5. The most abundant metabolite that is produced due to the hydrolysis of Fluazinam is CAPA where the triflouromethyl group is converted to COOH group. Although a trifluoromethyl group on an aromatic ring had been regarded as a very stable substituent, the experimental results described indicate that a trifluoromethyl group on a heterocyclic ring undergoes interesting reactions with nucleophiles owing to electronic interaction of the heterocyclic system with the trifluoromethyl group. The first attack of the nucleophile could be at the 2-position as the attack in two positions as it produces more no of resonance stabilized structure. The second and third replacements of fluorine atoms by hydroxy groups may be favoured by the lone-pair electrons of the oxygen atom of the first OH group. The pH values of these soils reveal the percent base saturation in the surface soil horizon is in the order of black soil > coastal saline soil > new alluvial soil > red and lateritic soil. The abundance of hydroxyl ion follows the same trend while concentration of proton follows the reverse. Fluazinam hydrolyzed more rapidly in presence of OH- than H+ therefore, the half-life values in the order of black soil < coastal saline soil < new alluvial soil < red and lateritic soil. Similar type of result was also observed in persistence study of lab water of different pH. In case of acidic water (pH 4) the compound was very much stable and for alkaline pH (9.2) it dissipates more rapidly.
The application provides valuable outputs such as rate constants and half-lives, which are crucial parameters in understanding the behaviour of substances in the environment. Furthermore, the application includes data visualization features, enabling users to generate plots of their data and model fits. This tool serves as a valuable resource for researchers and practitioners in the field, facilitating the analysis and interpretation of dissipation data.
5 Conclusion
Several key findings have emerged in our study of Fluazinam residue across different soil types, doses, and incubation periods. These findings shed light on the behaviour of Fluazinam in various environmental conditions and its adsorption kinetics. The results from an overall comparison (irrespective of dose and time) between soil types suggested soil properties not significantly influencing the impact Fluazinam residue, but the dose of application have a significant effect. However, from dissipation kinetic study in each soil revealed a wide variation in half-life period which highlighted the role of soil properties influencing the dissipation. Additionally, the first order was found to be the best model for describing Fluazinam’s dissipation process with exceptions in case of dissipation in water where a better model fit was observed in the second order. The effect of pH in water was found to significantly influence the recovery of Fluazinam. Further the application tool which provides crucial data such as half-life and rate constant of pesticide is a testament towards enhancing our understanding of pesticide behaviour in the environment. We believe that this application will serve as a valuable tool in the scientific community. Overall, these findings contribute to a better understanding of the environmental fate of Fluazinam, which can be valuable for pesticide management and environmental protection efforts. The results highlight the importance of considering both soil properties and water characteristics when assessing the behaviour of pesticides like Fluazinam. While these findings provide valuable insights for the specific conditions studied, further research may be needed to explore the behaviour of Fluazinam in field studies including a wider range of crops, soil types, and environmental conditions.
Data availability statement
The datasets presented in this study can be found in online repositories. The names of the repository/repositories and accession number(s) can be found in the article/Supplementary Material.
Author contributions
SM: Formal Analysis, Methodology, Project administration, Validation, Writing–original draft, Writing–review and editing. JM: Data curation, Investigation, Software, Writing–original draft, Writing–review and editing. BK: Methodology, Writing–review and editing. DC: Conceptualization, Funding acquisition, Project administration, Supervision, Writing–review and editing. AB: Conceptualization, Funding acquisition, Methodology, Project administration, Resources, Supervision, Writing–original draft. SM: Conceptualization, Funding acquisition, Investigation, Project administration, Resources, Supervision, Writing–review and editing.
Funding
The author(s) declare that no financial support was received for the research, authorship, and/or publication of this article.
Conflict of interest
Author SM was employed by Mitra SK Pvt Ltd.
The remaining authors declare that the research was conducted in the absence of any commercial or financial relationships that could be construed as a potential conflict of interest.
The reviewer PG declared a past collaboration with the authors JM, AB to the handling editor.
Publisher’s note
All claims expressed in this article are solely those of the authors and do not necessarily represent those of their affiliated organizations, or those of the publisher, the editors and the reviewers. Any product that may be evaluated in this article, or claim that may be made by its manufacturer, is not guaranteed or endorsed by the publisher.
Supplementary material
The Supplementary Material for this article can be found online at: https://www.frontiersin.org/articles/10.3389/fenvs.2024.1394847/full#supplementary-material
References
Chen, L., Jia, C., Li, F., Jing, J., Yu, P., He, M., et al. (2018). Dissipation and residues of fluazinam and dimethomorph in potatoes, potato plants, and soil, determined by QuEChERS ultra-performance liquid chromatography tandem mass spectrometry. Environ. Sci. Pollut. Res. 25, 32783–32790. doi:10.1007/s11356-018-3281-7
Chen, L., Li, F., Zhang, Z., Jing, J., Zhao, E., Yu, P., et al. (2023). Residues and dietary risk assessment of fluazinam in root mustard after field experiments. Environ. Sci. Pollut. Res. 30, 56836–56843. doi:10.1007/s11356-023-26336-3
Chen, Y., Lu, Y., Nie, E., Akhtar, K., Zhang, S., Ye, Q., et al. (2020). Uptake, translocation and accumulation of the fungicide benzene kresoxim-methyl in Chinese flowering cabbage (Brassica campastris var. parachinensis) and water spinach (Ipomoea aquatica). Environ. Pollut. 264, 114815. doi:10.1016/j.envpol.2020.114815
EFSA (2008). Conclusion regarding the peer review of the pesticide risk assessment of the active substance fluazinam. EFSA J. 6, 137r. doi:10.2903/j.efsa.2008.137r
El-Baky, N. A., and Amara, A. A. A. F. (2021). Recent approaches towards control of fungal diseases in plants: an updated review. J. Fungi 7, 900. doi:10.3390/jof7110900
Fantke, P., and Juraske, R. (2013). Variability of pesticide dissipation half-lives in plants. Environ. Sci. Technol. 47, 3548–3562. doi:10.1021/es303525x
FAO and WHO (2019). “Pesticide residues in food 2018 - report 2018 - joint FAO/WHO meeting on pesticide residues,” in FAO plant production and protection paper no. 234. Rome, 668.
Feng, X., Wang, K., Mu, Z., Zhao, Y., and Zhang, H. (2015). Fluazinam residue and dissipation in potato tubers and vines, and in field soil. Am. J. Potato Res. 92, 567–572. doi:10.1007/s12230-015-9469-1
Hakala, K. P., Yli-Halla, M. J., Tuomainen, P. M., and Hartikainen, H. (2020). Persistence of fluazinam in soil under boreal conditions. J. Environ. Sci. Health, Part B 55, 539–549. doi:10.1080/03601234.2020.1729631
Hu, J., Wu, J., Gu, M., Geng, J., Guo, C., Yang, Z., et al. (2020). Baseline sensitivity and control efficacy of Fluazinam against Clarireedia homoeocarpa. Crop Prot. 137, 105290. doi:10.1016/j.cropro.2020.105290
Jain, A., Sarsaiya, S., Wu, Q., Lu, Y., and Shi, J. (2019). A review of plant leaf fungal diseases and its environment speciation. Bioengineered 10, 409–424. doi:10.1080/21655979.2019.1649520
National Center for Biotechnology Information. (2023). PubChem compound summary for CID 91731, fluazinam. Avaliable at: https://pubchem.ncbi.nlm.nih.gov/compound/Fluazinam.
Peng, Y., Li, S. J., Yan, J., Tang, Y., Cheng, J. P., Gao, A. J., et al. (2021). Research progress on phytopathogenic fungi and their role as biocontrol agents. Front. Microbiol. 12, 670135. doi:10.3389/fmicb.2021.670135
Pal, R., Chakrabarti, K., Chakraborty, A., and Chowdhury, A. (2006). Degradation and effects of pesticides on soil microbiological parameters-a review. Internat. J. Agricult. Res. 1, 240–258. doi:10.3923/ijar.2006.240.258
Patra, S., Das, A., Rakshit, R., Choudhury, S. R., Roy, S., Mondal, T., et al. (2022). Persistence and exposure assessment of insecticide indoxacarb residues in vegetables. Front. Nutr. 9, 863519. doi:10.3389/fnut.2022.863519
Roberts, T. R., and Hutson, D. H. (1999). Metabolic pathways of agrochemicals—part 2: insecticides and fungicides. Roy. Soc. Chem., 235–242. doi:10.1039/9781847551375
Saifullah, S., Margus, A., Kankare, M., and Lindström, L. (2022). Repeated exposure of Fluazinam fungicides affects gene expression profiles yet carries no costs on a nontarget pest. Insect Sci. 29, 1373–1386. doi:10.1111/1744-7917.13013
Keywords: fluazinam, pesticides, dissipation kinetics, soil, water
Citation: Mukhopadhyay S, Mandal J, Kanrar B, Chatterjee D, Bhattacharyya A and Majumder S (2024) Degradation and residue dynamics of fluazinam in diverse indian soil types and water pH conditions: a comprehensive study using kinetic models. Front. Environ. Sci. 12:1394847. doi: 10.3389/fenvs.2024.1394847
Received: 02 March 2024; Accepted: 11 June 2024;
Published: 27 June 2024.
Edited by:
Oladele Ogunseitan, University of California, Irvine, United StatesReviewed by:
Osama I. Abdallah, Agricultural Research Center, EgyptPritam Ganguly, Bihar Agricultural University, India
Copyright © 2024 Mukhopadhyay, Mandal, Kanrar, Chatterjee, Bhattacharyya and Majumder. This is an open-access article distributed under the terms of the Creative Commons Attribution License (CC BY). The use, distribution or reproduction in other forums is permitted, provided the original author(s) and the copyright owner(s) are credited and that the original publication in this journal is cited, in accordance with accepted academic practice. No use, distribution or reproduction is permitted which does not comply with these terms.
*Correspondence: Jajati Mandal, J.Mandal2@salford.ac.uk; Debasish Chatterjee, debashis.chatterjee.ku@googlemail.com; Santanu Majumder, smajumder@bournemouth.ac.uk