- 1School of Bioengineering and Biosciences, Lovely Professional University, Phagwara, India
- 2Department of Biomedical Sciences, Institute of Health, Jimma University, Jimma, Ethiopia
Engineered nanomaterials have emerged as a promising technology for water treatment, particularly for removing heavy metals. Their unique physicochemical properties enable them to adsorb large quantities of metals even at low concentrations. This review explores the efficacy of various nanomaterials, including zeolites, polymers, chitosan, metal oxides, and metals, in removing heavy metals from water under different conditions. Functionalization of nanomaterials is a strategy to enhance their separation, stability, and adsorption capacity. Experimental parameters such as pH, adsorbent dosage, temperature, contact time, and ionic strength significantly influence the adsorption process. In comparison, engineered nanomaterials show promise for heavy metal remediation, but several challenges exist, including aggregation, stability, mechanical strength, long-term performance, and scalability. Furthermore, the potential environmental and health impacts of nanomaterials require careful consideration. Future research should focus on addressing these challenges and developing sustainable nanomaterial-based remediation strategies. This will involve interdisciplinary collaboration, adherence to green chemistry principles, and comprehensive risk assessments to ensure the safe and effective deployment of nanomaterials in heavy metal remediation at both lab and large-scale levels.
1 Introduction
A clean water source is essential for the long-term survival of life. However, water pollution has become a global issue, threatening the entire ecosystem and life on Earth (Chandran et al., 2023; Rangappa et al., 2024; Rybarczyk et al., 2024). A variety of industrial improvements (Figure 1), including energy plants, mining industries, and environmental disasters caused by climate change, have had a significant impact on the spread of harmful toxins in aquatic ecosystems. Pollution and increased demand due to population development have resulted in a widespread scarcity of safe drinking water in many locations around the world (Sukmana et al., 2021). Heavy metal ions in wastewater are a form of pollution that has caused serious health problems (Sharma et al., 2023; Moukadiri et al., 2024). These ions are of special significance because of their persistence in the environment, long-term accumulation, and potential threat to human health via the food chain. Heavy metals are defined as elements with atomic weights ranging from −63 to −201 and densities above 5 gm/cm³ (Arun Karnwal, 2012; H Karami, 2013). They include toxic elements such as chromium (Cr), nickel (Ni), arsenic (As), cobalt (Co), zinc (Zn), copper (Cu), and mercury (Hg) (Lu and Astruc, 2018). Untreated or badly treated effluents from many enterprises release harmful heavy metals into the environment, hence exacerbating water pollution. The inappropriate treatment of landfill sites has compounded the situation, as the leachates from these sites contain considerable amounts of heavy metals that can infiltrate groundwater (NC). Arun Karnwal (2012); Arun et al. (2018); Sall et al., 2020; Zamora-Ledezma et al. (2021). Scientists and engineers have become more conscious of the gravity of this pollution, but developing effective and economically viable solutions remains a difficult task.
In recent years, several technologies have been utilized to clean wastewater and purify water. These technologies encompass both proven procedures and novel ways for combating water pollution (Qiu et al., 2022; Goyal et al., 2023). Although these technologies have the potential to eradicate a wide range of pollutants, they typically face restrictions such as high costs, complex procedures, and unsatisfactory performance (Abd Elnabi et al., 2023; Fei and Hu, 2023; Solanki et al., 2024). Even when utilizing well-established approaches, the broad dispersion of heavy metal contaminants in water makes their clearance difficult, owing to problems connected to their ionic forms and ion selectivity. The adsorption approach has gained popularity because it is a safe, environmentally acceptable, and highly effective way to treat heavy metal ion-polluted industrial wastewater (K Liu et al., 2023). Adsorbent materials with large surface areas, considerable porosity, and the ability to be reused have proved efficacy, making the adsorption process economically viable (Bahadi et al., 2024). Jain et al. (2022) investigated the use of economical organic biosorbents (SCB, PHB, and OPB) for eliminating Methylene Blue dye from textile wastewater. Through batch adsorption tests employing a central composite design (CCD) approach, optimal conditions for dye removal are determined. Langmuir and Freundlich adsorption isotherms elucidate the equilibrium reached after 90 min. Response surface methodology (RSM) coupled with CCD optimizes experiments, while kinetic modeling assesses pseudo-first- and pseudo-second-order kinetics. Thermodynamic analysis indicates endothermic, natural adsorption. FTIR characterization confirms Methylene Blue adsorption, suggesting SCB, PHB, and OCB as viable substitutes for commercial biosorbents in wastewater treatment.
Certain limitations hinder the usage of typical adsorbents such as activated carbon and metal oxides. As a result, novel nano adsorbents have been created that use nanoparticles to overcome these limitations (Umeh et al., 2023). Nanomaterials have emerged as possible adsorbents for heavy metal removal due to their unique properties, such as a large specific surface area and the ability to be modified (Yu et al., 2021). Because of their specific surface charge, porosity, and high capacity to bind ions, nanomaterials have a wide range of forms and architectures, which improves their effectiveness in removing heavy metals. Nonetheless, several challenges exist in the practical application of these technologies, particularly in the separation of treated water. To solve this difficulty, nanomaterials are chemically modified to increase their ability to segregate and adsorb diverse compounds (Abdelbasir and Shalan, 2019; Abu Shmeis, 2022). Nanomaterials’ recyclability makes them an intriguing and cost-effective option for adsorption-based heavy metal removal from water and wastewater. This review investigates the use of nanomaterials to adsorb and remove heavy metals from water and wastewater. As part of the analysis, a variety of variables influencing this process are examined. These parameters include pH, temperature, contact time, initial heavy metal concentration, and adsorbent quantity.
2 Methods for removing heavy metals from water
The risk of heavy metal contamination looms over our world, endangering ecosystems and human health. These persistent contaminants enter water sources through industrial, mining, and agricultural processes, where they accumulate in living creatures and pose serious health concerns (Abd El-Aziz et al., 2023; Ahmadian et al., 2023; Ali et al. Fortunately, scientists are equipped with a variety of remediation options, and knowing the mechanisms underlying heavy metal removal from aqueous solutions is critical for their successful implementation (Abd Elnabi et al., 2023). Table 1 provides a comparative review of heavy metal removal processes from aqueous solutions, revealing a range of advantages, disadvantages, and applications. Various processes, including as adsorption, precipitation, ion exchange, and membrane filtration, provide distinct advantages, including high efficiency, cost-effectiveness, and adaptability (Lu and Astruc, 2018). However, each technique has limitations, including limited capacity, pH dependence, and regeneration issues (Azimi et al., 2017; Bui et al., 2021). Understanding the merits and disadvantages of each approach is critical for determining the best technique for a given environmental remediation scenario. This detailed investigation helps to advance long-term solutions for reducing heavy metal pollution in water systems.
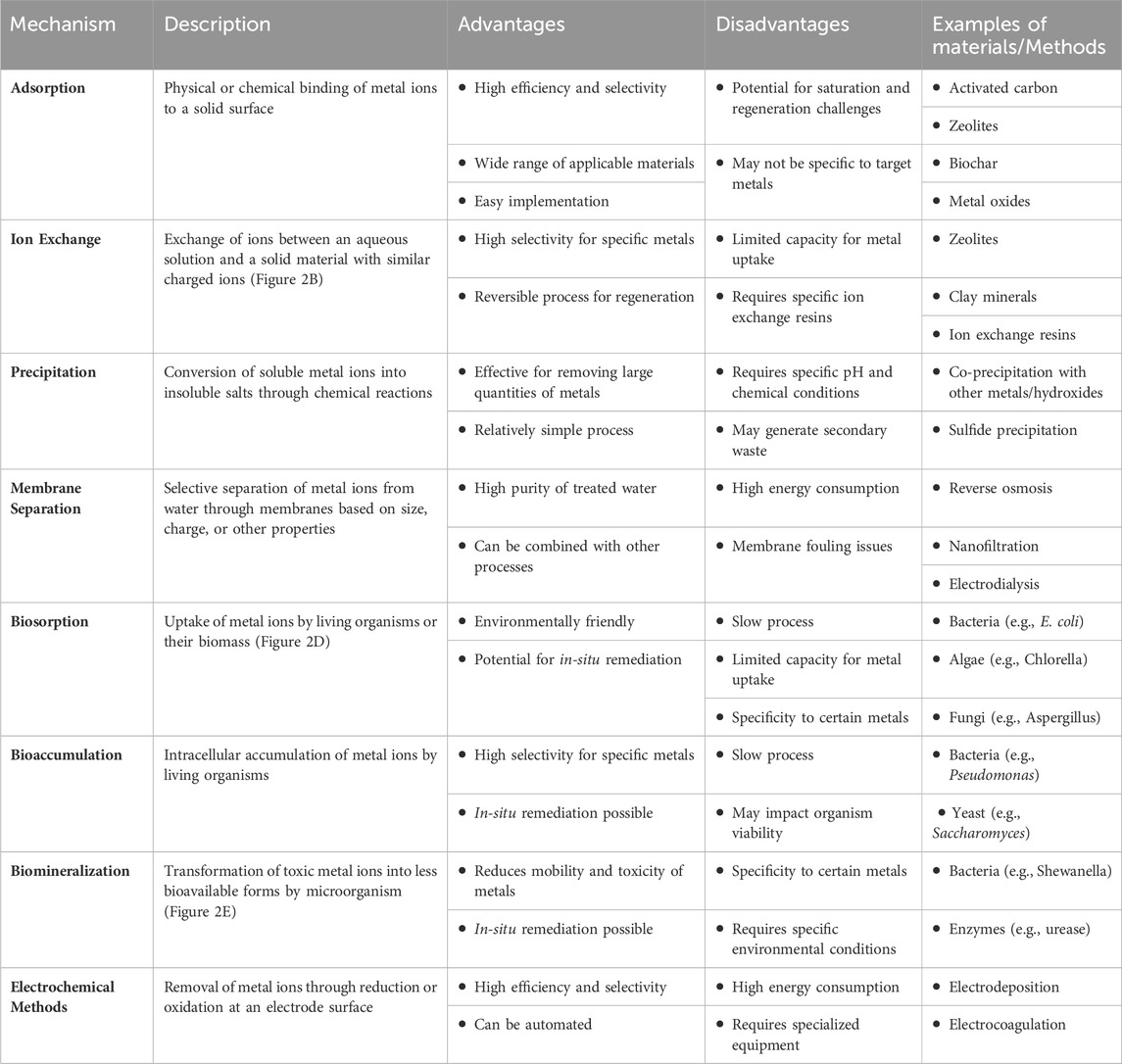
Table 1. Comparative analysis of mechanisms for heavy metal removal from aqueous solutions: Advantages, disadvantages, and applications (Azimi et al., 2017; Almomani et al., 2020; Bhandari et al., 2021; Alzahrani et al., 2022; Aragaw and Ayalew, 2022; Beiranvand et al., 2022; Abdullah et al., 2023).
2.1 Adsorption: a sticky situation for metals
One of the most widely employed techniques for heavy metal removal is adsorption (Figure 2A). In this process, metal ions cling to the surfaces of adsorbent materials, effectively trapping them and preventing their spread (Abobakr and Abdo, 2022; Adisasmito et al., 2023). The key players in this adsorption drama are:
• Adsorbents: These materials, with their high surface areas and abundant functional groups, act as magnets for metal ions. Activated carbon, clays, zeolites, and various nanomaterials are popular choices.
• Metal ions: These positively charged metallic culprits are attracted to the negatively charged sites on the adsorbent surface.
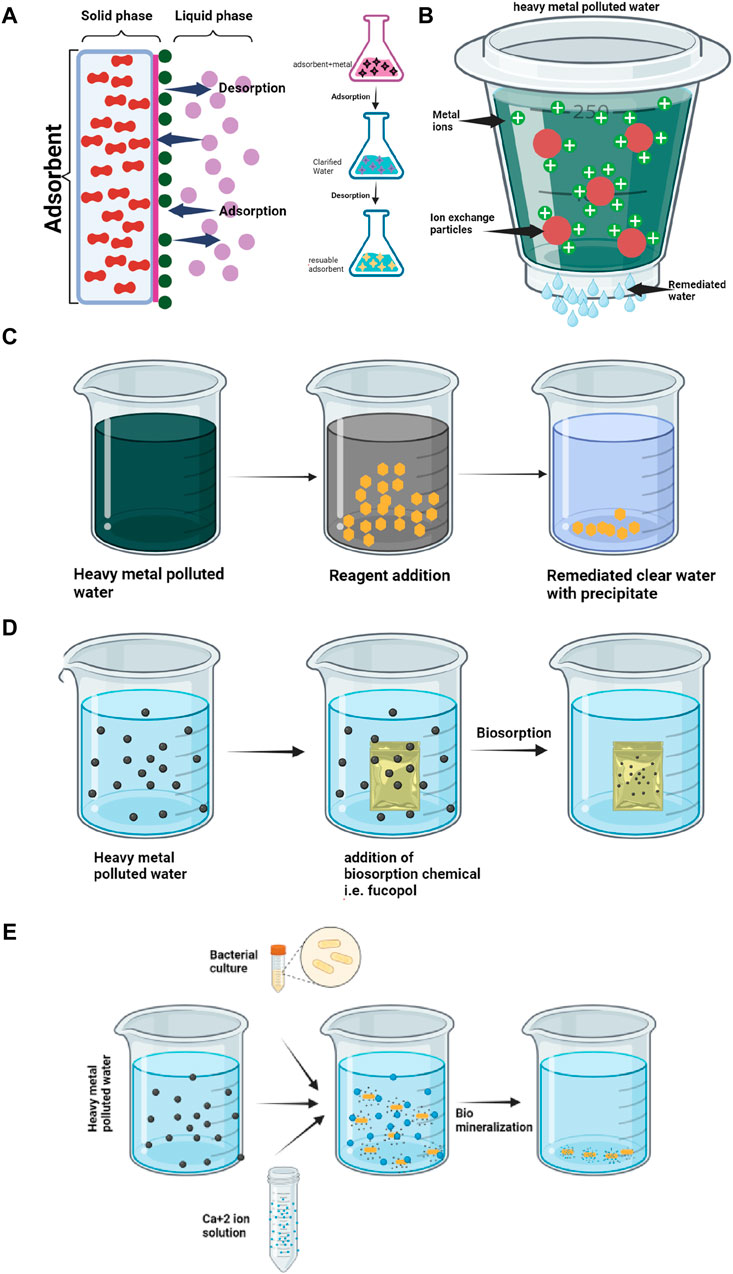
Figure 2. Various mechanisms used for remediation of heavy metals from water (A) Adsorption; (B) Ion Exchange; (C) Precipitation; (D) Biosorption; (E) Biomineralization.
Several mechanisms govern the adsorption dance:
• Physical adsorption (physisorption): This weak, van der Waals force-driven interaction creates a temporary bond between the metal ion and the adsorbent surface.
• Chemical adsorption (chemisorption): This stronger, covalent, or ionic bond formation leads to more permanent metal ion attachment.
• Ion exchange: Metal ions replace other cations bound to the adsorbent surface, achieving selectivity for specific metals (Figure 2B).
Factors like pH, temperature, and initial metal concentration influence the adsorption process. Optimizing these parameters is crucial for maximizing metal removal efficiency.
2.2 Precipitation: turning metals into solid sediments
Another popular approach involves precipitation. By altering the solution’s pH or adding specific chemicals, metal ions are transformed into insoluble precipitates, settling out of the water as solid particles (Figure 2C) (Yu et al., 2021). This method is particularly effective for metals like lead, copper, and cadmium (Godwin et al., 2023; L Liu et al., 2022).
The mechanisms at play here involve:
• Hydrolysis: Metal ions react with water molecules, forming insoluble hydroxide precipitates.
• Precipitation with anions: Adding anions like phosphates or sulfates forms insoluble salts with the metal ions, promoting their removal.
The choice of precipitating agent and optimal pH conditions are critical for efficient precipitation.
2.3 Membrane filtration: straining out the contaminants
Imagine tiny sieves selectively filtering out metal ions while allowing clean water to pass through. That is the essence of membrane filtration (Cevallos-Mendoza et al., 2022; Chadha et al., 2022). Membranes with varying pore sizes can be employed to physically separate metal ions based on their size (Figure 3).
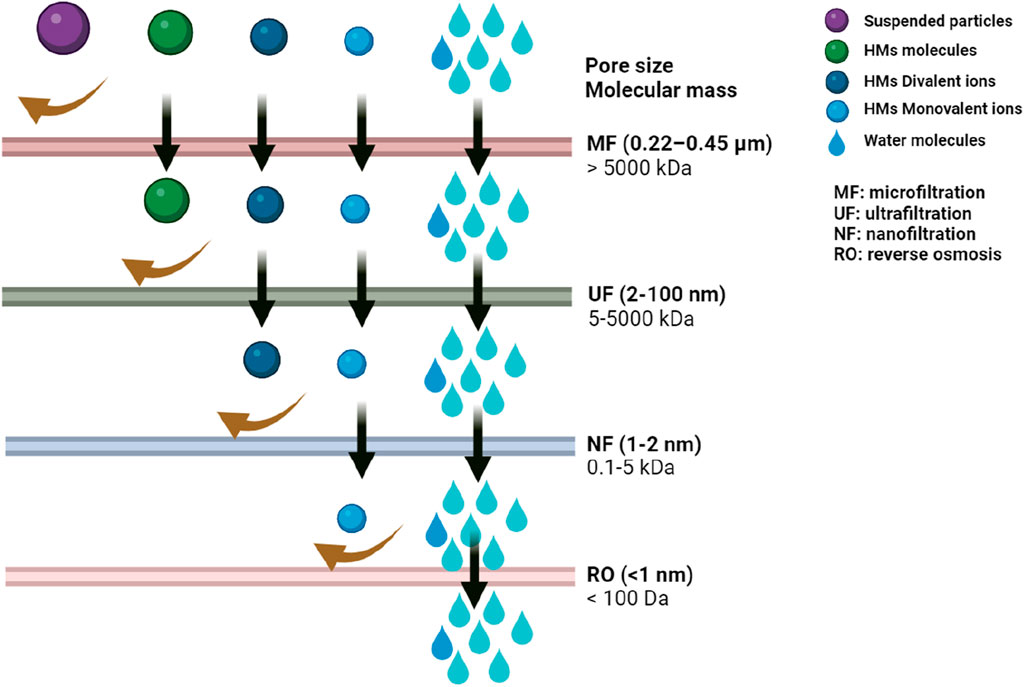
Figure 3. Performance evaluation of four membranes in rejecting various heavy metal particles and ions.
Here is how it works:
• Reverse osmosis: A pressure difference forces water molecules through a semipermeable membrane, leaving behind larger metal ions.
• Nanofiltration: Membranes with smaller pores allow selective passage of water molecules while rejecting larger metal ions and some organic contaminants.
Membrane selection, pressure control, and pretreatment of the solution are crucial aspects of successful metal removal using this technique.
2.4 Bioaccumulation: nature’s detoxification squad
Nature itself holds the key to some promising remediation strategies. Certain microorganisms and plants have the remarkable ability to bioaccumulate heavy metals, taking them up and accumulating them in their tissues (Z Xu et al., 2023). This natural detoxification process offers a sustainable and environmentally friendly approach.
The mechanisms involved are complex and diverse, including:
• Biosorption: Microorganisms bind metal ions to their cell walls or extracellular polymers (Figure 2D).
• Bioaccumulation: Specific proteins within plants or microorganisms actively transport and sequester metal ions.
• Biotransformation: Some microorganisms can convert toxic metal forms into less harmful ones.
While further research is needed to optimize and scale up bioaccumulation techniques, their potential for eco-friendly metal removal is significant.
No single technique holds the silver bullet for heavy metal removal. Often, combining multiple approaches proves most effective (Abbas, 2021). For instance, adsorption followed by membrane filtration can achieve high removal efficiencies. Integrating bioaccumulation with other methods can enhance sustainability and reduce environmental impact. By understanding the diverse mechanisms behind heavy metal removal, scientists can tailor strategies to specific contaminants and environmental conditions. Ongoing research continues to explore novel materials, optimize existing processes, and develop cost-effective solutions. The ultimate goal? A cleaner, healthier planet free from the threat of heavy metal pollution.
3 Nanomaterials for heavy metals removal from aqueous reservoir
Wastewater treatment is undergoing a significant revolution with the application of nanomaterials. Researchers are exploring a diverse range of these materials, including zeolite, various forms of carbon, polymers, chitosan, ferrites, magnetic particles, metal oxides, bimetallic structures, and even pure metals (Aloulou et al., 2022; Amariei et al., 2022; Gao et al., 2022). These nanoparticles boast a remarkable property called adsorption. This essentially means they can act like tiny magnets, attracting and holding onto heavy metals from wastewater, effectively removing them. As a valuable resource for those working in environmental remediation, Table 2 offers a snapshot of the latest nanobiotechnology strategies being used to tackle heavy metal contamination. It details innovative methods and techniques specifically designed to combat this form of environmental pollution.
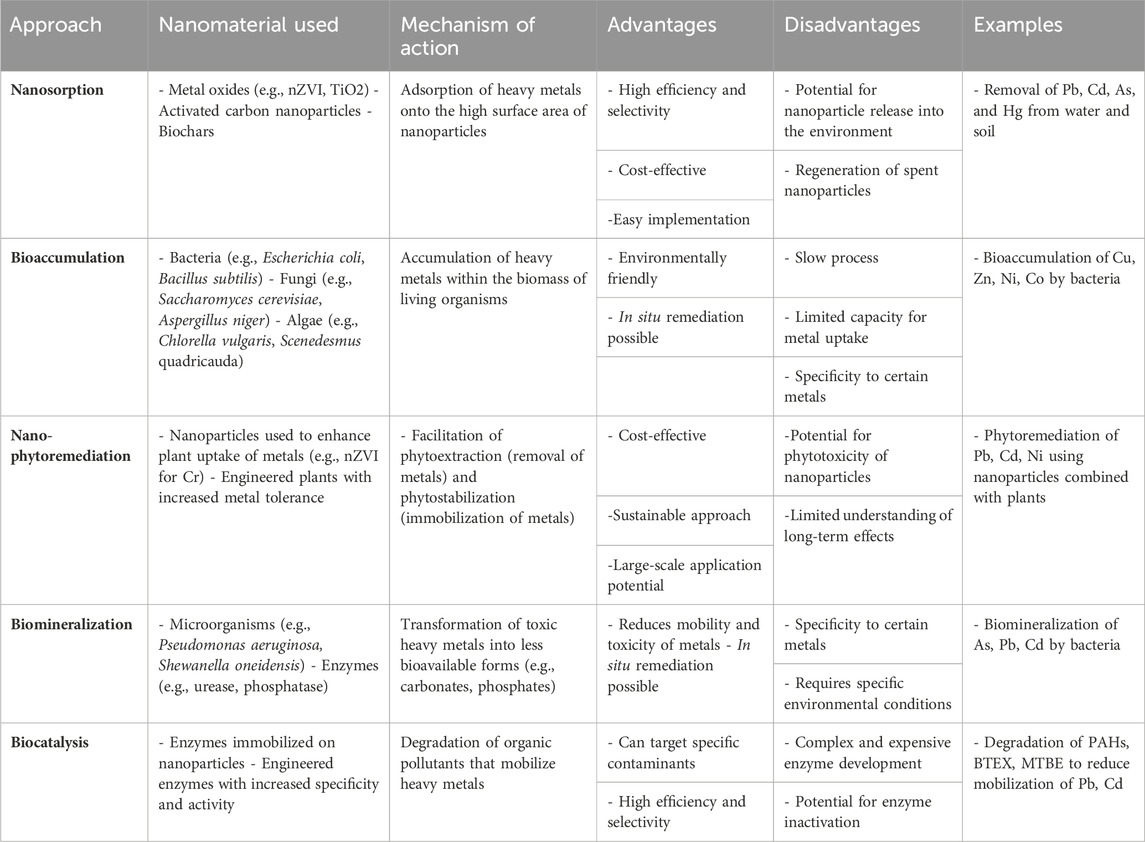
Table 2. Summary of nanobiotechnology approaches for mitigating heavy metal pollution (Biswas et al., 2021; Gong et al., 2021; Kolluru et al., 2021; Baby et al., 2022; Dhara, 2024).
3.1 Functionalized carbon (C) nanomaterials for removing toxic metals from wastewater
Functionalized carbon nanoparticles are currently employed as effective adsorbents for removing hazardous metals from wastewater due to their distinctive chemical and physical characteristics, showing significant potential for application in water purification initiatives (Surti et al., 2023). These materials have gained considerable attention across scientific and technical disciplines for their exceptional thermal, mechanical, optical, chemical, and physical properties. Various carbon-based nanomaterials, such as carbon nanotubes, nanocomposites, and graphene, have been utilized for eliminating contaminants from water sources (Abbasi and Khan, 2021). Graphene, a two-dimensional substance composed of a single layer of carbon atoms, is extremely thin (Abdel Wahab et al., 2023). Carbon nanotubes, on the other hand, are cylindrical nanostructures made of sp2-bonded carbon atoms. The hydrophilic nature of graphene, attributed to the oxygen functional groups present on the surface of graphene oxide (GO), enables exceptional solubility in water (Abdel Wahab et al., 2023). The use of GO in water purification is highly recommended due to its large surface area and various functional groups. Nanoparticles based on graphene have been proven to be exceptionally effective in eliminating various pollutants from water (Ahlawat et al., 2021; H Ahmad and Liu, 2021). Complexes formed between oxygen functional groups on graphene and cationic metals play a crucial role in the adsorption mechanism utilized by graphene for removing hazardous metals. Similarly, in the case of organic dyes (Cano et al., 2023), contact occurs through the expanded configuration of π-electrons within graphene. The incorporation of MnO2 nanotubes into reduced graphene oxide (rGO) hydrogel by Zeng et al. resulted in the creation of a three-dimensional nanomaterial with a size of 20 nm. This material effectively removed various heavy metals from the environment, including zinc (83.9 mg/g), copper (121.5 mg/g), silver (138.2 mg/g), cadmium (177.4 mg/g), and lead (Zeng et al., 2019). These impressive adsorption capacities are attributed to the synergistic interaction between manganese dioxide nanotubes (MnO2) and reduced graphene oxide (rGO) within the material’s 3D porous structure. Notably, GO nanosheets also exhibited good adsorption potential for cobalt, demonstrating a removal quantity of 68.2 mg/g. Similarly, Cd (II) ions were removed using graphene oxide nanosheets, which showed a removal capacity of 106.3 mg/g (Zhou et al., 2023).
Environmental engineers find carbon nanotubes (CNTs) highly appealing due to their unique attributes, such as extensive surface area, small dimensions, cylindrical hollow structure, and electrical conductivity (Suresh and Rajendran, 2022). CNTs primarily exist in two forms: multi-walled carbon nanotubes (MWCNTs) and single-walled carbon nanotubes (SWCNTs). Several investigations have delved into the utilization of CNTs for treating wastewater to eliminate toxic heavy metals via adsorption. Researchers have effectively employed CNTs to remove ions of manganese (II), zinc (II), cobalt (II), lead (II), and copper (II) (Sun et al., 2015; Trojanowicz, 2006; J Zhang et al., 2021). An additional investigation revealed that the effective removal of lead ions from water yielded a remarkable adsorption capacity of 70.2 mg/g (Marwani et al., 2022). The application of MnO2 as a coating on oxidized multi-walled CNTs resulted in an adsorption capacity of 41.7 mg/g for the removal of cadmium ions from water (Y Liu et al., 2021). Recent advancements have led to the development of nanocomposites comprising carbon-layered silicates, offering enhanced properties compared to traditional carbon nanomaterials. One such composite, known as nano adsorbent montmorillonite/carbon (H Zhang et al., 2023), exhibited a remarkable adsorption capacity of 247.86 mg/g for lead ions, making it a promising and eco-friendly solution for water treatment. Table 3 presents a comparative analysis of various nanomaterials used for the removal of heavy metals from aqueous reservoirs, highlighting their effectiveness, mechanisms of action, and specific heavy metals targeted. This comparison aids in understanding the suitability and performance of different nanomaterials in remediation processes.
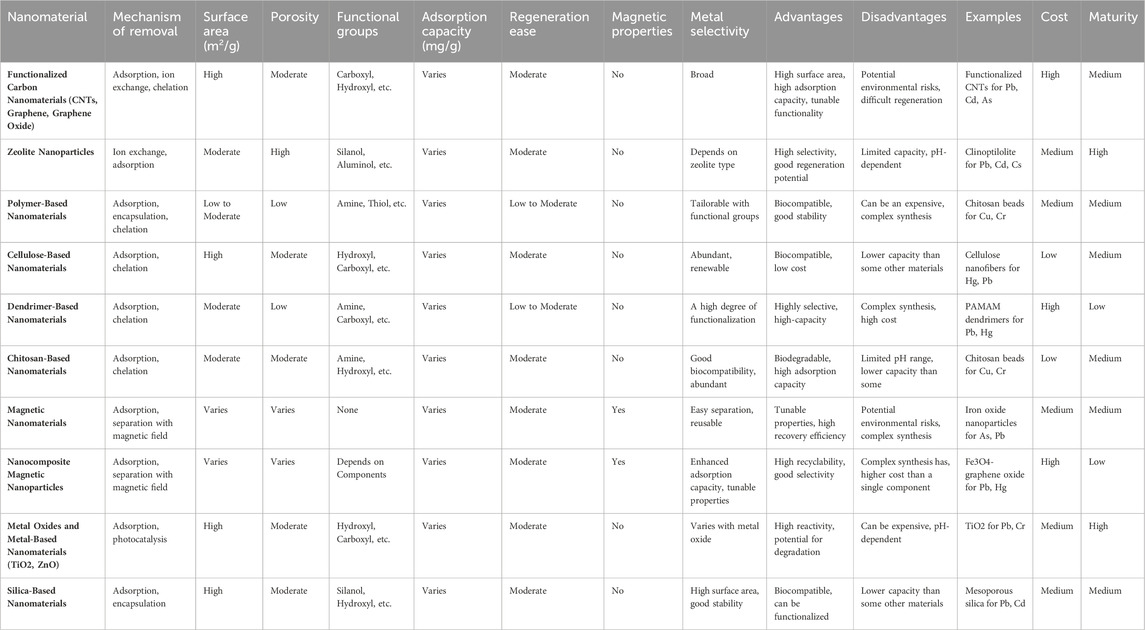
Table 3. A comparative analysis of nanomaterials for heavy metal removal from aqueous reservoirs(Bhagat and Giri, 2021; S Biswas et al., 2021; Dong et al., 2021; Solanki et al., 2024; J Yang et al., 2019).
3.2 Zeolites nanoparticles
Zeolite materials are composed of crystalline hydrated aluminosilicate structures (Jarosz et al., 2022), exhibiting a highly porous skeletal framework with pores and channels spanning from nano to micrometer dimensions. Zeolites consist of tetrahedral SiO4 and AlO4 units (Marotta et al., 2021), these units form a framework of pores and channels capable of accommodating cations, water, and small molecules, resembling a sponge with numerous small or extremely small pores but lacking flexibility. Within the crystal structure, Si and Al atoms occupy central positions, while O atoms are located at the corners, shared between SiO4 and AlO4 units, creating regular gaps and channels. The negatively charged Al3 ions attract positively charged cations such as Na+, Mg2+, K+, and Ca2+, allowing them to occupy vacant positions and neutralize the framework’s negative charge (Mahdavi Far et al., 2022; Mo et al., 2022). Zeolites demonstrate robust three-dimensional framework structures with limited interactions between ions and molecules confined within pores and voids, facilitating the removal of ions without damaging the zeolite structure. Zeolites are categorized into three groups based on their Si/Al molar ratio:
• zeolites A and X, with low silica content and an Al/Si ratio less than 2;
• zeolites Y and L, with intermediate silica content and an Al/Si ratio between2 and 5; and
• zeolites beta and ZSM-5, with high silica content and an Al/Si ratio greater than 5.
These differences result in distinct characteristics in the structure of zeolite frameworks, enabling various industrial applications (Umejuru et al., 2023). For instance, zeolites with low silica content and a Si/Al ratio of 1, possess a larger number of cation exchange sites, leading to higher cation contents and exchange capacities. Zeolites with moderate silica content are essential for enhancing heat and acid resistance, crucial for catalytic purposes. Conversely, high silica zeolites exhibit excellent adsorption capabilities, particularly for organic molecules with lower polarity, and limited affinity for water and other polar substances. They feature a more uniform surface and a preference for organic molecules, along with greater resistance to water due to their porous crystal structure (Mo et al., 2022).
Table 4 presents data on the efficacy of zeolite nanoparticles in removing heavy metals from aqueous solutions, detailing their adsorption capacity and efficiency for various heavy metal contaminants. This table provides valuable insights into the potential of zeolite nanoparticles as efficient adsorbents in water treatment processes.
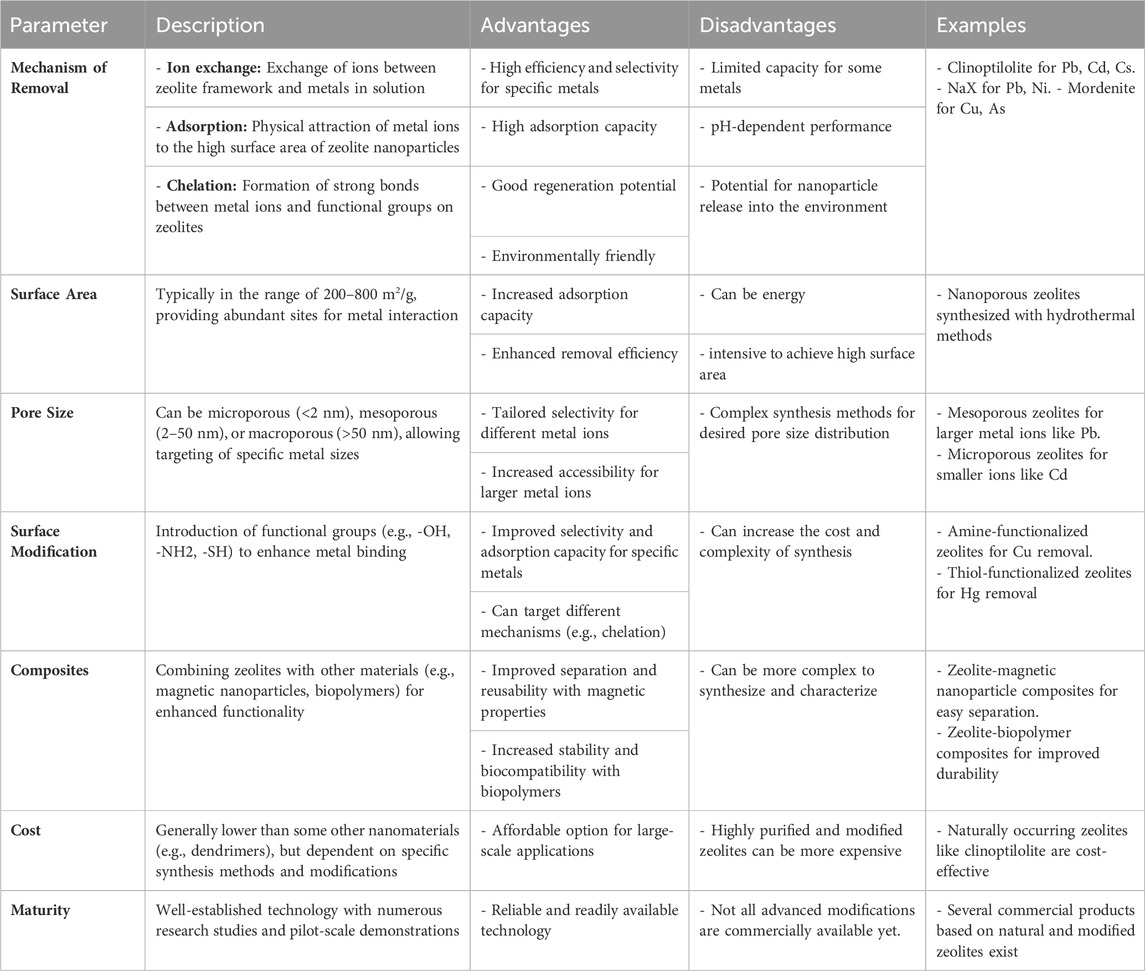
Table 4. Zeolite nanoparticles for heavy metal removal from aqueous solutions (Mo et al., 2022; Umejuru et al., 2023; B Zhao et al., 2024).
Zeolites serve as effective catalysts in chemical processes due to their porous properties, facilitating unimpeded liquid flow through their pores and supporting chemical reactions on their surfaces. Additionally, they can be modified to enhance their adsorption capacity. Zeolite nanoparticles are utilized for eliminating hazardous metals from wastewater owing to their reactive surfaces and expansive area (Deravanesiyan et al., 2015). Both natural zeolites like clinoptilolite and synthetic variants such as zeolite 4A and zeolite X are commonly employed for heavy metal treatment (Gao et al., 2022). These zeolites assist in transmitting alkali and alkaline earth metals to balance the overall negative charge within the framework. Sprynskyy et al. (2006) conducted a sorption experiment using natural zeolites, specifically clinoptilolite, for heavy metal elimination. The process involved three phases: rapid adsorption on the surface, an inversion stage, and diffusion flow from within the microcrystals. Particle size significantly influenced metal ion absorption, with smaller particles enhancing removal. However, clinoptilolite’s adsorption efficacy varied slightly when treating solutions with one metal versus multiple metals due to distinct sorption sites.
Various studies have distinguished natural and synthetic zeolites, highlighting synthetic versions’ superior effectiveness in metal ion removal (Vishnu et al., 2021; Jarosz et al., 2022). Yurekli (2016) demonstrated that incorporating zeolite nanoparticles (NaX) into polysulfone membranes effectively removes lead and nickel from water-based solutions, enhancing metal impurity elimination and reducing membrane pressure. Jain et al. (2021) developed an ultrathin forward osmosis (FO) membrane using cellulose acetate (CA) and titanium dioxide (TiO2) nanoparticles through phase inversion. The membrane exhibited high water permeability and stability, characterized by scanning electron microscopy, elemental mapping, and x-ray diffraction. Evaluating FO performance showed consistent water flux and low reverse salt flux, with an average water flux of 33.63 L/m2/h and reverse salt flux of 10.34 g/m2/h attributed to strong hydrogen bonding between cellulose ester and titania particles. The membrane demonstrated high efficiency for water desalination, promising for practical applications. In another study, Khorram Abadi et al. (2023) employed electrospun nanofibers made of polyvinyl alcohol and nano zeolite (PVA/NaX) to eliminate Ni2+ and Cd2+ ions, finding Cd2+ ions had higher adsorption capacity. Another study evaluated three sorbents (ANIZ, NaX zeolite granules, Al2O3 nanoparticles) for Cr3+ and Co2+ ion removal, emphasizing pH’s crucial role, with pH 5 and 6 most effective for Cr3+ and Co2+ removal, respectively. ANIZ exhibited superior removal efficiency for both ions compared to Al2O3 nanoparticles and NaX.
Furthermore, Al-Jubouri & Holmes (2020) demonstrated the production of carbon composites using 4A and 4A zeolite to extract cobalt ions from water solutions, significantly increasing ion exchange capacity and enhancing cobalt removal efficiency by adjusting pH and temperature.
3.3 Polymer-based nanomaterials
Scientists have shown significant interest in nanofibrous membranes based on polymers such as cellulose and chitosan, widely regarded as environmentally friendly remediation methods (Yu et al., 2021). The presence of a well-designed porous fiber network, along with a large specific surface area and high gas permeability, enhances their ability to absorb pollutants effectively (Muthukumaran et al., 2022). Their biodegradable nature, customizable surface functional groups, and robust structural integrity make them ideal alternatives for adsorption. Polymer-filled nanomembranes exhibit notable selectivity and adsorption prowess due to unique functional moieties such as NH2, COOH, and SO3H (Elakkiya et al., 2021). These nano adsorbents can be categorized based on the substrate utilized, showcasing their versatility in heavy metal removal applications. With their distinct properties and adaptable functionalities, polymer-based nanomaterials have emerged as promising solutions for addressing heavy metal contamination in water.
A significant advantage of these materials lies in their high specific surface area, facilitating increased interaction sites for heavy metal ion adsorption. This feature enhances adsorption efficiency by promoting stronger interactions between the polymer matrix and metal ions. Moreover, the surface chemistry of polymer-based nanomaterials can be tailored to selectively bind to specific heavy metal pollutants, ensuring efficient removal from water sources (Razman Shah et al., 2023; Y Wang and Sun, 2021). Additionally, these materials boast impressive mechanical properties, including high strength and flexibility, ensuring durability and stability during the adsorption process, even under challenging environmental conditions, maintaining consistent performance over time. Table 5 presents an overview of polymer-based nanomaterials used in heavy metal removal processes, showcasing their promising adsorption capacities and tailored characteristics for effective capture of heavy metal ions from aqueous solutions.
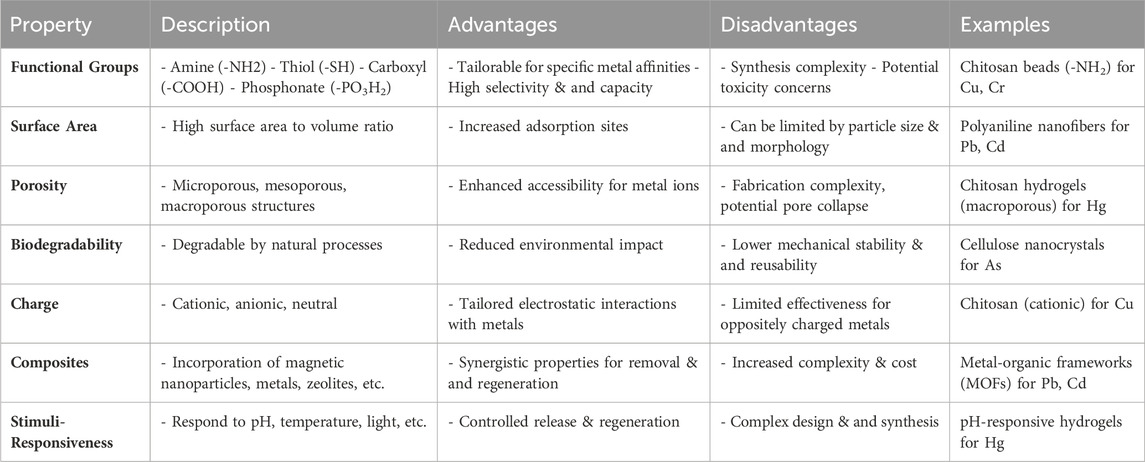
Table 5. Polymer-based nanomaterials for heavy metal removal (Asif et al., 2021; Zambare and Nemade, 2021; Chauhan et al., 2022; Kayanja et al., 2023).
Synthesis methods for polymer-based nanomaterials, such as electrospinning, polymerization, and self-assembly, offer versatility in fabricating diverse structures tailored to specific applications. Moreover, their cost-effectiveness and eco-friendliness make them attractive options for large-scale water treatment endeavors. Polymer-based nanomaterials show great potential for heavy metal removal from water due to their high adsorption capacity, customizable surface chemistry, and robust mechanical properties. Continued research and development efforts are necessary to optimize their performance and overcome challenges for their practical implementation in water treatment processes.
3.3.1 Cellulose-based nanomaterials
Researchers investigated the efficacy of biopolymer-based adsorbents, particularly cellulose, for the removal of harmful heavy metals from water (Yusuf, 2021). Abou-Zeid et al. (2018) synthesized three bioadsorbents: tetramethyl piperidine oxide-oxidized 2,3,6-tricarboxylic cellulose nanofibers (T-CNFs), TPC-cellulose nanofibers (TPC-CNFs) with and without polyamide-amine-epichlorohydrin crosslinker (PAE). They assessed the effectiveness of these adsorbents in purifying water contaminated with Cu, Pb, and Ca ions. For lead and copper ions, the adsorption capacities of crosslinked TPC-CNFs were determined to be 82.19 mg/g and 97.34 mg/g, respectively. Mautner et al. (2016) showed that CNF/P, formed by adding phosphate groups to cellulose nanofibers using phosphoric acid, efficiently removed copper ions from water. Cellulose nanofibers were developed by Choi and Lee (2022) using electrospun cellulose acetate deacetylation and subsequent esterification to incorporate thiol groups. According to the Langmuir isotherm (Abuhatab et al., 2023), this modified nanomaterial has adsorption capabilities of 22.0 mg/g, 45.9 mg/g, and 49 mg/g for lead (II), cadmium (II), and copper (II) ions, respectively. The functionalized nanomaterial facilitated the removal of metal ions through complexation interactions between surface thiol groups and divalent metals, showcasing its effectiveness in water remediation. Similarly, cellulose nanofibrous mats modified with citric acid were fabricated using the same method, and batch adsorption studies confirmed their efficacy in eliminating chromium ions, particularly Cr (VI), from aqueous solutions (Chen et al., 2022).
3.3.2 Dendrimer-based nanomaterials
A highly efficient method for eliminating toxic metals involves utilizing organic polymers equipped with functional groups capable of strongly binding these harmful substances (Guo et al., 2022). This system comprises a polymeric support with functional appendages, with various ratios of acrylamide and acrylic acid being employed to fabricate a superabsorbent polymer hydrogel (Ahmadian et al., 2023). Designed to remove Cd (II), Ni (II), Cu (II), and Co (II) from water, this hydrogel exhibits remarkable adsorption capacity. However, the effectiveness of the material in adsorbing cadmium and nickel ions is comparatively lower than its ability to adsorb copper and cobalt ions (Naseem et al., 2019). This discrepancy may arise from the smaller size of the cations, allowing them to easily penetrate the polymeric support and undergo rapid chelation by the functional arms.
The adsorption capacities of Zn (II), Pb (II), and Cd (II) ions were found to be 7.2, 8.8, and 6.1 mmol/g, respectively, in nanofibers made of a polyacrylonitrile base polymer, diethylenetriamine, ethylene glycol, and ethylenediamine, according to another study (Morillo Martín et al., 2018). These nanofibers’ remarkable adsorption capacity is due to their large surface area, which allows functional groups to interact with toxic metals more effectively. Additionally, 1,3,5-tris (6-isocyanatohexyl)-1,3,5-triazinane-2,4,6-trione and diethylenetriamine were combined to form two novel polymers (Cegłowski et al., 2018). The ethylene amine groups found in these nanomaterials can undergo complexation processes and bind metallic ions. The effectiveness of the nano adsorbent in absorbing divalent copper, chromium, cobalt, and cadmium ions was greatly enhanced by including pentaethylenehexamine to form longer amine chains, as compared to diethylenetriamine.
Scientists made nanofiber membranes out of polyacrylonitrile and metal-organic frameworks (MOF-808) using a co-electrospinning technique (Efome et al., 2019). The PAN/MOF-808 nano adsorbent that was produced was outstanding in removing heavy metals from water, in the following order: Hg (II) < Pb (II) < Cd (II) < Zn (II). This implies that the size and configuration of the metals influence their interaction with active sites and complex formation. Another study (Ren et al., 2013) showcased the efficient extraction of hexavalent chromium impurities from wastewater using nanofibers composed of poly-(ethylene-co-vinyl alcohol), achieving an impressive adsorption capacity of 90.75 mg/g within a timeframe of less than 100 min. Furthermore, employing amidoxime-modified polyacrylonitrile nanofibers proved highly effective in eliminating divalent lead and copper ions from water.
Poly (styrene-alt-maleic anhydride) resin underwent chemical modification using either 1,2-diaminoethane or 1,3-diaminopropane, alongside 3-aminobenzoic acid, for the removal of Pb (II), Zn (II), Cu (II), and Fe (II) from water (Hasanzadeh et al., 2013; Hasanzadeh et al., 2017). Additionally, Sohail et al. (I Sohail et al., 2020; Sohail et al., 2021) adopted a divergent approach to synthesize poly-amidoamine (PAMAM) dendrimers, yielding zero-generation dendrimers measuring between 200 and 400 nm. These dendrimers, featuring a higher concentration of functional groups on their external surface, effectively eliminated nickel ions from water.
3.4 Chitosan based nanomaterials
Chitosan nanoparticles, derived from shellfish and crustaceans like squid beaks, shrimp, prawns, and crabs, have several uses (Ahmed et al., 2023). This hydrophilic polymer has beneficial properties such as non-toxicity, biodegradability, biocompatibility, renewability, and biorenewability. Chitosan is often obtained by breaking down chitin under acidic conditions such as strong NaOH or enzymatic hydrolysis with chitin deacetylase. Its unique characteristics can be further modified chemically and mechanically to generate new functionalities, expanding its potential applications. The increasing presence of -NH2 and -OH groups in chitosan, coupled with its excellent solubility in water and organic solvents, has attracted attention for its potential use as a chelating agent for pollutants like heavy metals and dyes in water purification processes (Bilal et al., 2022; Agha et al., 2024).
Chemical changes and chelation can add functional groups to chitosan’s structure, increasing its sorption and selectivity properties. For instance, Dubey et al. (2016) produced carbon nanoparticles (CANPs) to remove Hg2+ ions from water, demonstrating an impressive adsorption capacity of 217.4 mg/g at 30°C. According to Saad et al. (2018), a nanocomposite made of ZnO and chitosan successfully removed lead, copper, and cadmium ions from water, exhibiting a strong predilection for Pb2+ ions, while adsorbing Cd2+ and Cu2+ ions at lesser rates. Similarly, a mixture of TiO2 and chitosan nanoparticles efficiently absorbed copper and divalent lead ions (Razzaz et al., 2016).
Esmaeili and Khoshnevisan (2016) developed an alginate-functionalized chitosan nanoparticle composite, achieving a 94.9% removal of Ni2+ ions from water. Moreover, Yuan et al. (2018) engineered porous three-dimensional carbon materials using chitosan, exhibiting strong adsorption capabilities for Pb2+ and Cd2+ ions in aqueous environments. The adsorption mechanism involves coordination and electrostatic interactions with functional groups present in the carbon compounds derived from chitosan. Chitosan-based nanoparticles hold promise for various environmental applications, particularly in heavy metal removal from water, owing to their unique properties and versatile functionalities. Further research and innovation are crucial to enhance their effectiveness and advance their practical implementation in water treatment processes.
3.5 Magnetic nanomaterials
Magnetic nanoparticles, distinguished for their exceptional capacity to enhance toxic metal extraction and facilitate magnetic separation (Zaman et al., 2022), are extensively utilized in environmental remediation due to their excellent recyclability post-separation. As their dimensions decrease, significant alterations occur in their properties. Non-magnetic nanoparticles, possessing smaller surface areas and undergoing challenging separation processes, exhibit lower efficiency in water treatment compared to their magnetic counterparts (Ukhurebor et al., 2023). In contrast, magnetic nanoparticles, with substantial surface areas, lack toxicity, and easily disperse, prove to be reliable, efficient, and cost-effective water purifiers, particularly effective in removing heavy metals (Ukhurebor et al., 2023). Figure 4 illustrates the sequential steps involved in water purification with MNPs, highlighting the efficiency of MNPs in removing heavy metal impurities from water at each stage.
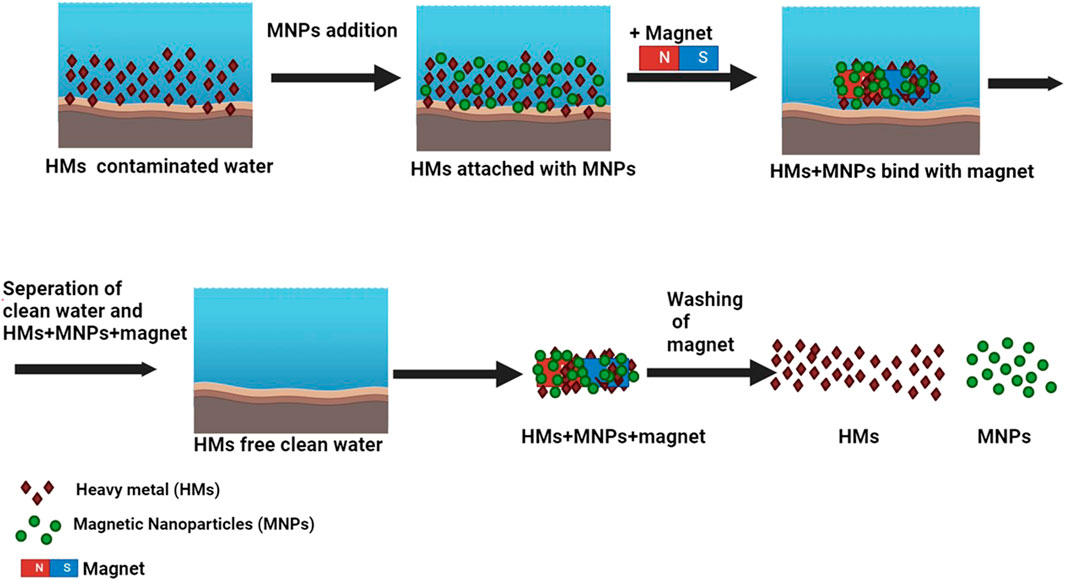
Figure 4. Illustration depicting the procedural steps involved in water purification utilizing MNPs.
Iron oxide nanoparticles, widely acknowledged for their reusability, easy separability, and high adsorption capacity (Tao et al., 2023), play an indispensable role in various applications. Fe3O4 nanoparticles, with an adsorption capability of 36 mg/g, effectively remove divalent lead ions from water-based solutions (Nassar, 2010). Additionally, Fe3O4 nanoparticles have successfully extracted Cu (II), Pb (II), Mn (II), and Zn (II) ions from water in separate research projects. Changes in conditions influence the electrostatic interactions between these nanoparticles and the specific metals, consequently impacting their adsorption capacity (Yamini et al., 2023; K Zhang et al., 2023).
Table 6 provides a comprehensive analysis of magnetic nanomaterials utilized for the removal of heavy metals, offering detailed insights into their efficacy and mechanisms of action. This exploration sheds light on the diverse range of magnetic nanoparticles employed in heavy metal remediation strategies, highlighting their potential for environmental applications. Through a systematic examination, Table 6 elucidates the promising advancements and challenges in harnessing magnetic nanomaterials for efficient heavy metal removal.
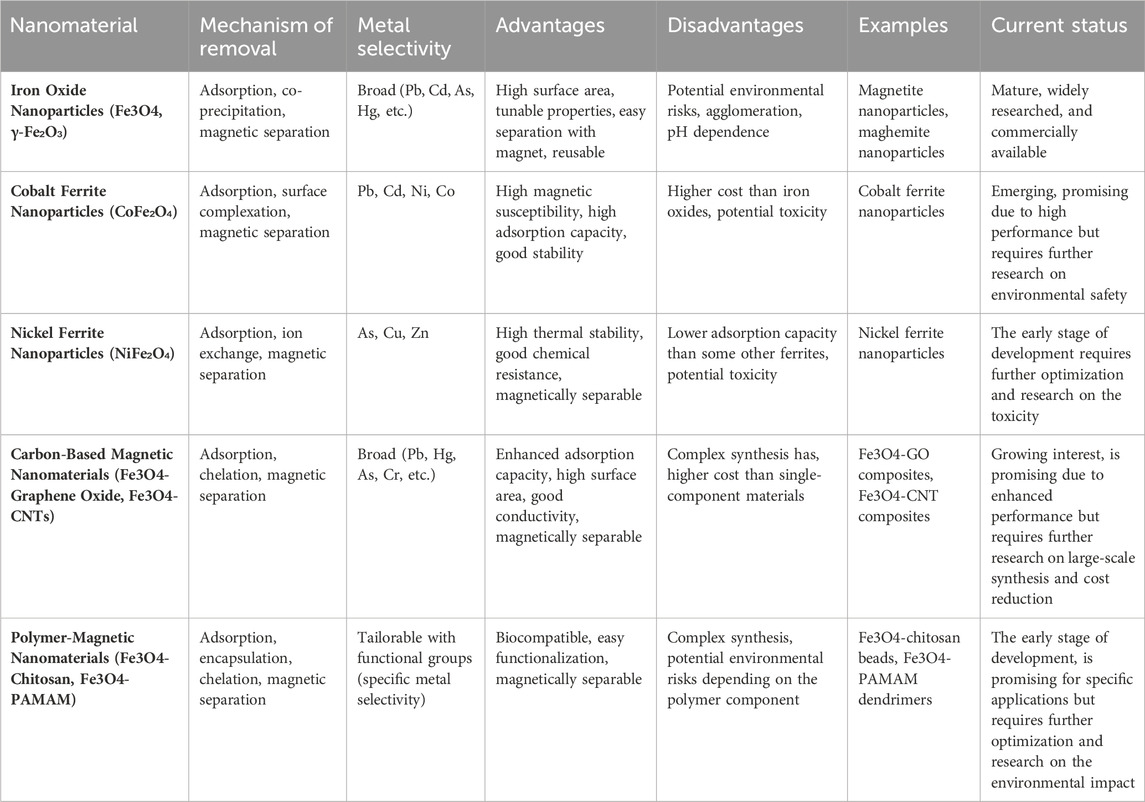
Table 6. Magnetic nanomaterials for heavy metal removal: A detailed exploration (Suhasini & Thiagarajan, 2021; Targuma et al., 2021; Thakur & Kumar, 2023; Yamini and Devi Rajeswari, 2023; Yaseen et al., 2021; K Zhang et al., 2023; Zhu et al., 2016).
Nanorods, nanowires, and nanotubes, owing to their larger surface area, demonstrate remarkable efficiency in removing heavy metals from wastewater and water. For instance, Fe3O4 nanorods exhibited adsorption capacities ranging from 76 to 127 mg/g for Cu+2, Cd+2, Ni+2, Zn+2, Pb+2, and Fe+2 ions in water (H Karami, 2013; N Karami et al., 2024). However, the oxidation and aggregation tendencies of bare magnetic nanoparticles limit their practical value in water. Functionalizing the surface of magnetic nanoparticles enhances stability, increases adsorption capacity, and improves selectivity.
Various surface modifiers, such as carbonaceous, biomolecule, inorganic, organic, and polymer substances, have been utilized to modify magnetic nanoparticles’ surfaces. Functionalization prevents aggregation and enhances interactions between metals and surfaces, including electrostatic, chemical bonding, and complex formation. Tailoring magnetic nanoparticles’ surfaces to selectively bind hazardous metals through chelation significantly influences their selectivity and effectiveness in adsorbing pollutants. Additionally, capping with hydrophilic coatings like polyethylene glycol inhibits aggregation and increases surface area, further enhancing their adsorption capabilities.
3.5.1 Nanocomposite magnetic nanoparticles
Nanocomposite magnetic nanoparticles are recognized as a promising solution for heavy metal contamination due to their unique characteristics and versatile applications (Bui et al., 2021). Comprising a magnetic core enclosed in a composite material, these nanoparticles offer several advantages for efficiently extracting heavy metals from polluted water sources. One significant advantage is their heightened magnetic sensitivity, allowing for easy extraction using external magnetic forces (Hasanzadeh et al., 2017). This simplifies the cleanup process by facilitating the retrieval of nanoparticles along with attached heavy metal pollutants, enabling further treatment or disposal.
Moreover, the composite structure enables the integration of different adsorbent materials onto the magnetic core, allowing for precise customization of performance in removing specific heavy metals. Materials like activated carbon, graphene oxide, or chitosan can be incorporated to enhance adsorption efficiency (Abo Markeb et al., 2023).
Additionally, nanocomposite magnetic nanoparticles exhibit exceptional stability and reusability, making them cost-effective alternatives for prolonged cleanup efforts. The presence of a magnetic core ensures the nanoparticles remain suspended in water during remediation, preventing aggregation and ensuring consistent performance across multiple cycles. Recent research has demonstrated their effectiveness in removing various heavy metals, such as lead, cadmium, mercury, arsenic, and chromium (Hasanzadeh et al., 2017; Shahamati Fard et al., 2021; Joshi et al., 2022; Jjagwe et al., 2023; Mallick et al., 2023; Rani et al., 2023), indicating their potential for practical use in addressing environmental challenges.
Kim et al. (2013) synthesized flower-shaped Fe3O4/MnO2 nanocomposite. The research focuses on the development and application of various metal ferrite nanocomposites for heavy metal removal from aqueous solutions. Firstly, an adsorbent exhibited superior adsorption capacity for Cd2+, Cu2+, Pb2+, and Zn2+ compared to Fe3O4 nanoparticles. Additionally, researchers synthesized metal ferrite magnetic nanoparticles with magnetic separation properties, represented by the general formula M (FexOy), where M denotes the metal atom forming divalent bonds. Several metal ferrites including copper ferrite (CuFe2O4), zinc ferrite (ZnFe2O4) (Tu et al., 2016; Tu et al., 2017), and manganese-zinc ferrite (Mn0.67Zn0.33Fe2O4) (Tu et al., 2013) have been developed for the removal of heavy metals. Specific studies highlighted the efficacy of ZnFe2O4 in eliminating lead ions, CuFe2O4 in removing molybdenum ions, and Mn-Zn ferrites in removing As5+, Cd2+, and Pb2+ ions. Furthermore, cobalt spinel ferrites (CoFe2O4 and MnFe2O4) synthesized via the co-precipitation method were utilized to remove divalent zinc ions with notable adsorption capacities (Asadi et al., 2020). Another study (Vamvakidis et al., 2020) demonstrated the effectiveness of a nanocomposite cobalt ferrite (CoFe2O4) modified with octadecylamine coating in adsorbing Cu2+ ions from aqueous solutions, with efficient separation and recovery processes. Overall, these findings illustrate the potential of metal ferrite nanocomposites as efficient adsorbents for heavy metal removal in water treatment applications.
Various magnetic nanocomposites, including metal oxides, have been developed for extracting heavy metals from water. For example, a Fe3O4/MnO2 nanocomposite with a flower-shaped structure showed improved adsorption compared to iron oxide nanoparticles (Kim et al., 2013). Metal ferrite magnetic nanoparticles, such as ZnFe2O4 and CuFe2O4, have demonstrated a strong affinity for specific heavy metals, effectively removing them from aqueous solutions (Wang et al., 2021; Alshehri et al., 2024). Nanocomposite magnetic nanoparticles offer a promising method for addressing heavy metal contamination by providing effective removal, convenient retrieval, and exceptional stability. Further research and development in this area hold the potential to enhance the performance and scalability of these nanoparticles for broader environmental remediation initiatives.
3.5.2 Inorganic functionalized magnetic nanoparticles
Inorganic magnetic nanoparticles are increasingly being recognized as a viable method for tackling heavy metal pollution in several environmental contexts. The nanoparticles, made of magnetic substances like iron oxides or metal ferrites, have distinct characteristics that make them efficient adsorbents for removing heavy metals in water remediation procedures. An important benefit of inorganic magnetic nanoparticles is their ability to respond to magnetic forces, enabling them to be easily separated from water using external magnetic fields (Gangaraju et al., 2022). This enhances the retrieval of nanoparticles together with absorbed heavy metal contaminants, streamlining the process of cleaning up and allowing for further treatment or disposal.
Furthermore, inorganic magnetic nanoparticles demonstrate elevated surface area-to-volume ratios, which offer abundant active sites for the adsorption of heavy metals. The increased surface area facilitates effective interaction between the nanoparticles and heavy metal ions in water, resulting in fast adsorption rates and high removal efficiencies. Studies have demonstrated that certain kinds of inorganic magnetic nanoparticles, such as iron oxide nanoparticles (Fe3O4) and metal ferrites (e.g., ZnFe2O4, CuFe2O4), have a high attraction to certain heavy metals (El Messaoudi et al., 2024; Y Zhang et al., 2021). Fe3O4 nanoparticles have been employed to effectively eliminate lead (Pb), cadmium (Cd), and arsenic (As) ions from water solutions due to their exceptional adsorption abilities. Conversely, metal ferrite nanoparticles have exhibited distinct adsorption characteristics for various heavy metals, depending on their chemical compositions (Chandrani et al., 2024). For example, ZnFe2O4 nanoparticles have demonstrated a significant attraction to lead ions, whereas CuFe2O4 nanoparticles possess a notable ability to adsorb copper ions (Tu et al., 2017).
Modifying inorganic magnetic nanoparticles offers a versatile approach to increase their affinity for specific heavy metals. Applying organic ligands or polymers to these nanoparticles can improve their specificity and durability, thereby boosting their effectiveness in heavy metal removal. The magnetic nanoparticles can be altered using various inorganic elements such as silica, metals, nonmetals, and metal oxides. The coatings stabilize nanoparticles in water-based solutions and increase their chemical bond to particular ligands. The magnetic nanoparticles were modified by adding amorphous oxide shells made of Mn-Co. As a result, surfaces with substantial negative charges were formed at different pH values. These nanoparticles were modified to enhance their ability to bind to Cu (II), Cd (II), and Pb (II) ions (Ma et al., 2013). The reference provided the adsorption capacities for these ions as 481.2, 386.2, and 345.5 (Ma et al., 2013). According to the electrical properties of the atoms participating in the complexation reaction, the technique efficiently removed lead ions but zinc ions to a lesser extent. To influence the creation of stable complexes by highly ionic-potential metals, the adsorption sequence tracked the hydrated ionic radius of the metals.
Calcium carbonate, an inexpensive, non-toxic, and easily soluble chemical, belongs to the carbonate group of inorganic chemicals utilized to modify magnetic nanoparticles for recovering heavy metals from water. Despite its numerous advantages, constraints such sludge formation, restricted efficacy, and separation challenges hinder its widespread application in water and wastewater treatment (Fadia et al., 2021). These challenges can be reduced through the use of magnetic nanoparticles, which increase their adsorption capacity and facilitate their separation from the experimental media. Nanocomposites composed of magnetic mesoporous calcium carbonate were synthesized by Wang et al., 2020 via annealing after solvothermal treatment. The nanocomposites, measuring 50 nm in size and having an irregular spherical shape, efficiently eliminated Pb (II) and Cd (II) from water, with maximum adsorption capacities of 821 mg/g and 1179 mg/g, respectively. Inorganic magnetic nanoparticles have the potential to remove heavy metals due to their extensive surface area, specific affinity for heavy metals, and the capability to be controlled using magnets (A Islam et al., 2021; Islam et al., 2017). Further research and development are required to enhance the design and application of these nanoparticles for efficient environmental remediation.
3.5.3 Carbon materials magnetic nanoparticles
There have been significant breakthroughs made in the removal of heavy metals from aqueous solutions by the utilization of nano adsorbents, like graphene oxide and activated carbon. In order to improve the adsorption capacity of surface nano adsorbents, Danesh et al., 2016 carried out a study in which they investigated the efficacy of graphene oxide, iron oxide, and EDTA for the adsorption of lead ions. This improvement is attributed to the coordinating properties of ethylenediaminetetraacetic acid (EDTA) and the hydroxyl and carboxyl groups that are thought to be present in graphene oxide. Increased electrostatic interactions between the surface functional groups and hazardous metals are made possible as a result of these properties. By producing extremely effective Fe3O4 nano adsorbents that were treated with EDTA, Ghasemi et al., 2017 were able to attain adsorptive capacities that ranged between 71 and 169 mg/g for ions such as Hg2+, Ag+, Cd2+, Pb2+, Mn2+, and Zn2+. A nanocomposite with a peak adsorption capability of 28.8 mg/g for the removal of lead2+ was created by Li et al., 2020 by the synthesis of Fe3O4@C-SH nanoparticles. This was accomplished by functionalizing the nanoparticles with thiol (SH) groups.
Functional groups derived from organic sources have been explored to enhance the ability of magnetic nanoparticles to adsorb heavy metals. Ge and co-authors (Ge et al., 2012) synthesized Fe3O4 magnetic nanoparticles and functionalized them with 3-amino propyl triethoxysilane (APTES), acrylic acid (AA), and crotonic acid (CA) for the removal of metal ions. The use of the modified adsorbent demonstrated a preferential elimination sequence of metal ions, with decreasing reactivity observed in the order of Pb > Cu > Zn > Cd. Another study utilized EDTA-modified silica-coated magnetic nanoparticles to remove divalent mercury, with the addition of dithiocarbamate groups leading to increased hazardous metal removal and enhanced adsorption capability. The modification of silica-coated magnetic nanoparticles was done using glutathione to create the GSH/SiO2/Fe3O4 nano adsorbent, specifically designed for removing lead ions from water (J Wang et al., 2024; P Xu et al., 2017). Lead ion adsorption was enhanced at higher temperatures and the magnetic properties of the adsorbent aided in its removal from the reaction mixture. The study used iron oxide nanoparticles treated with metformin and amine to remove copper ions from water. The addition of silica and 0.1 wt% metformin led to a significant reduction of 92% in copper ions (Ghaemi et al., 2015; Ghaemi, 2016). Meanwhile, Shen et al. (Shen et al., 2012; Shen et al., 2013) investigated the adsorption process of Cr6+ using tetraethylene pentaamine-modified magnetic nanoparticles. Their findings revealed that a decrease in electrostatic interaction and charge transfer on the resulting adsorbents facilitated the adsorption of Cr6+. Zhang et al., 2019 effectively extracted Cr6+ from water using nitrogen-doped Fe3O4 magnetic porous carbon modified with humic acid, achieving a mass of 130.5 mg/g due to the presence of surface functional groups. A nanocomposite comprising Fe3O4/SiO2/chitosan and triethylenetetramine was capable of extracting Cr6+ from water, adsorbing 254.6 mg/g Cr6+ within 15 min (X Wang et al., 2020). Additionally, Shen et al., 2013 synthesized core-shell Fe3O4 nanoparticles with NH2 magnetic nanoparticles, demonstrating the adsorption of Cr6+ and Cu2+ ions in both simultaneous and individual metal-ion systems (MISs). Changes in pH significantly impacted how well copper (Cu2+) and chromium (Cr6+) ions stuck (adsorbed) to the material. At low pH (2–4), electrostatic forces were the main driver of adsorption. However, as the solution became more acidic, the -NH2 groups changed their charge (protonated), reducing their ability to bind metal ions. Interestingly, Cu2+ adsorption still improved despite this change. Above pH 4, Cu2+ likely formed precipitates due to the attraction between negatively charged chromate (HCrO4) and positively charged -NH3+ groups. Furthermore, competition between chromate and hydroxide (OH-) ions for binding sites weakened the adsorption as the pH rose. While other components present in low amounts or at higher pH levels didn't affect the process, this method proved effective for removing metal ions at low pH and high metal concentrations.
3.6 Metal-based nanomaterials for efficient heavy metal remediation
Metal oxides and metal-based nanomaterials play a significant role in heavy metal remediation due to their unique properties and high surface area-to-volume ratios, which make them efficient adsorbents for removing heavy metals from contaminated environments (Gupta et al., 2021; Ani and Egbosiuba, 2023; Bichave et al., 2023). These nanomaterials can be tailored to target specific heavy metals, offering a versatile and effective approach to remediation. Iron oxide (Fe3O4), titanium dioxide (TiO2), and manganese dioxide (MnO2) nanoparticles have been extensively studied for their ability to adsorb heavy metals through mechanisms like ion exchange, surface complexation, and precipitation (Bichave et al., 2023). For example, Fe3O4 nanoparticles have shown promising results in removing heavy metals like lead, cadmium, and arsenic from water systems.
These materials can be functionalized or modified with specific ligands or coatings to enhance their adsorption capacity and selectivity for target heavy metals. For instance, silver nanoparticles have demonstrated excellent antibacterial properties and can be utilized in conjunction with other nanomaterials for the removal of heavy metals and microbial contaminants from water sources (Alamier et al., 2023; Dutta et al., 2023). Table 7 presents an overview of nanoparticles and metal oxides utilized in the removal of heavy metals from water. It details their properties, efficiency, and mechanisms of action, providing valuable insights for environmental remediation strategies. This comprehensive compilation aids in understanding the diverse applications of nanomaterials in tackling heavy metal pollution in aqueous environments.
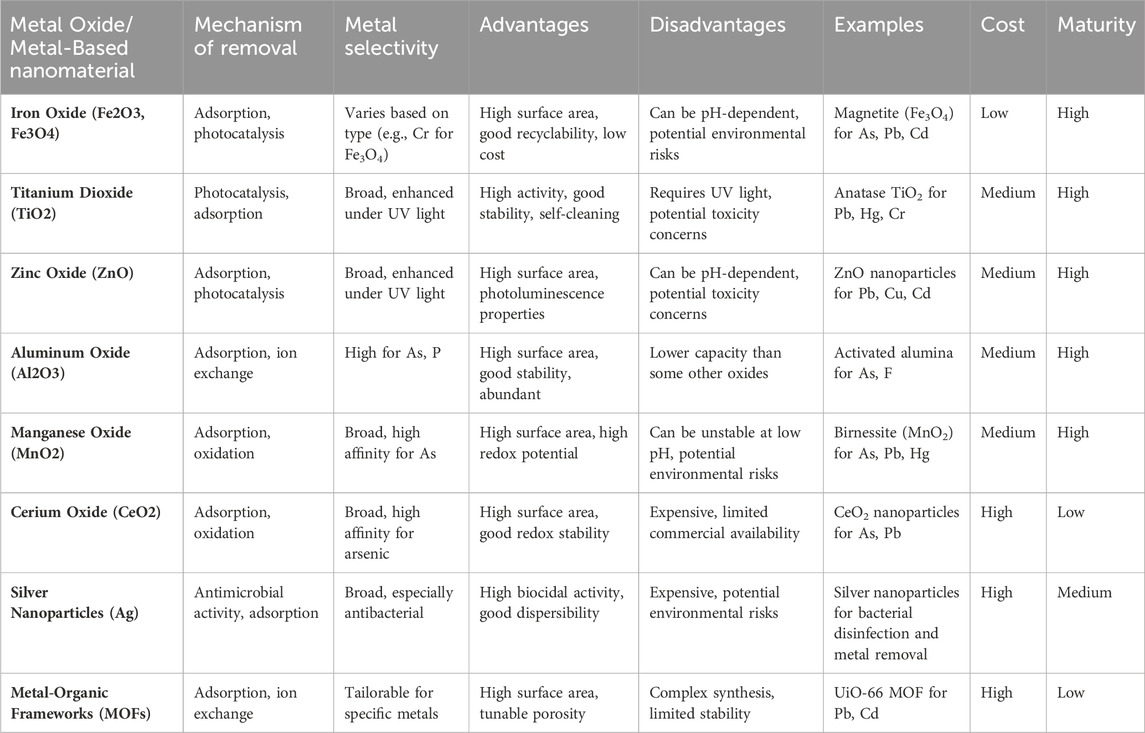
Table 7. Nanoparticles and metal oxides for the removal of heavy metals from water (Balakumar and Manivannan, 2021; Iqbal et al., 2022; Alhalili, 2023; Chakraborty et al., 2023; El-Sawy et al., 2023).
Regeneration: Additionally, the utilization of hybrid nanomaterials, which amalgamate metal oxides with organic compounds or polymers, engenders synergistic effects enhancing adsorption efficiency and stability (Babar et al., 2022). These hybrid materials offer tailored properties that mitigate challenges related to heavy metal contamination, such as pH sensitivity, competition from other ions, and environmental stability. Recent investigations (Raza et al., 2023; Vinoth and Wang, 2023) suggest that metallic and metal oxide nanoparticles hold promise in removing heavy metals from water. However, due to their inherent instability and difficulties in separation, pure metal nanoparticles are not ideal for use as adsorbents. Encapsulating or modifying the surface of nanostructured adsorbents improves their stability and facilitates their separation (Kanel et al., 2006). Zero-valent nanoiron Fe0 is biologically inert, exhibits stability in water treatment applications, boasts a substantial surface area, and demonstrates excellent adsorption capacity (Kanel et al., 2006). Consequently, some researchers have utilized Fe0 as a means to eliminate harmful metals from water (Kanel et al., 2006; Xiao et al., 2017; Xia et al., 2023). The oxygen present in metallic oxides reacts with aqueous pollutants, leading to their removal. Numerous studies worldwide have investigated various nano metal oxides, including ferric oxides such as hydrous ferric oxide, hematite (α-Fe2O3), goethite (α-FeOOH), maghemite (γ-Fe2O3), magnetite (Fe3O4), zinc oxides, cerium oxides, titanium oxides, aluminum oxides, and magnesium oxides, as well as hydrous manganese oxide and mixed-valence manganese oxide (Recillas et al., 2010; Wang et al., 2010; Wang et al., 2011; El Mouden et al., 2023). Hierarchically structured metal oxides are renowned for their high reactivity towards heavy metal ions, favorable surface-to-volume ratio, mechanical strength, and ease of regeneration. Fe⁰ exhibits a higher absorption capacity than metallic nanoparticles. When combined with water, Fe⁰ nanoscale zero-valent iron effectively eliminates 99% of pentavalent arsenic.
The plant Syzygium jambos exhibits a high adsorption capacity for Cr+6, reaching 983.3 mg/g. In a relevant study (Xiao et al., 2017), zero-valent iron (Fe⁰) was synthesized from ferric chloride (FeCl₃) using this plant material. To ensure the stability of nano-iron particles, some studies incorporate stabilizing agents in conjunction with Fe⁰. The chitosan carboxymethyl β-cyclodextrin containing Fe⁰ acts as a biodegradable stabilizer that reduces Cu2+ and Cr+6 to Cu0 and Cr3+, while also oxidizing Fe⁰ to Fe3+(Sikder et al., 2014). In addition, bimetallic nanoparticles can eliminate heavy metals. The efficiency of removing divalent copper ions by kaolinite-embedded Fe/Ni nanoparticles is 99.8% (C Li et al., 2022; Zhan et al., 2021). Metal oxides possess the ability to effectively remove heavy metals from water. The metal oxide nanoparticles were categorized into magnetic and non-magnetic types. Copper, Manganese, Iron, Cerium, Aluminum, and Zinc oxides are frequently used to purify water contaminated with heavy metals. Sounthararajah et al., 2015 demonstrated that the process of single-system adsorption effectively eliminated a greater number of metals as a result of the competition for adsorption sites among many adsorbates. A sodium titanate-based nanofibrous adsorbent effectively eliminated Pb+2, Cu+2, Cd+2, Ni+2, and Zn+2 (Zhou et al., 2021). The CuO nanoparticles, produced via magnetron sputtering, exhibited a high absorption capacity of 37.02 mg/g for Pb2+ and 15.62 mg/g for Cr6+ in aqueous solutions (Sounthararajah et al., 2015).
3.7 Silica based nanomaterials
Silica-based nanomaterials are essential for cleansing water contaminated with heavy metals due to their carefully designed pore size, regulated surface properties, and large surface area. Modifying these nano adsorbents with thiol and amino groups improves their ability to selectively adsorb metals and increases their adsorption capacity (Sankareswaran et al., 2022; Trofymchuk et al., 2023). Due to their eco-friendly and non-toxic characteristics, they have become more widely used in water treatment applications. Studies have compared the effectiveness of functionalized and non-functionalized silica nano adsorbents in binding divalent ions such as lead, nickel, and cadmium (Y Li et al., 2019; Peralta et al., 2021). The materials evaluated included NH2-functionalized silica gel, non-functionalized silica nanoparticles, and hollow silica spheres (Najafi et al., 2012). The modified versions showed adsorption capabilities of 96.80 mg/g for lead, 31.40 mg/g for nickel, and 40.74 mg/g for cadmium. Chemical changes using phenyl groups and 3-aminopropyl were utilized on silica nanospheres to improve the adsorption and elimination of divalent copper ions.
Interestingly, adding amino groups increased copper ion adsorption. In a separate investigation, nitrilotriacetic acid altered silica gel to treat wastewater with two-valence lead, cadmium, and copper ions. The improved nano adsorbent adsorbs 76.23, 53.15, and 63.6 mg/g of Pb, Cd, and Cu in 2–20 min. The authors used silicon waste with kerf loss to create nanoporous silicon (NPSi) functionalized with 3-amino-propyl-ethoxysilane (APTES) via chemical etching and nanosilver. The functionalized nano adsorbent was investigated for hexavalent chromium Cr (VI) adsorption from water (Z Yang et al., 2020). The greatest adsorption capacity was 103.75 mg/g in 1 hour. Protonated amino groups reduced Cr (VI) to Cr (III) for adsorption. This improved nano adsorbent works after 5 adsorption cycles.
4 Environmental factors affecting the performance of nanomaterials
Nanomaterials have gained significant attention in environmental remediation due to their unique properties and potential applications in removing heavy metals from aqueous environments. However, the performance of these nanomaterials can be influenced by various environmental factors (Kolluru et al., 2021; Kumar & Kumar, 2023; Madkour et al., 2021; Solanki et al., 2024; J Yang et al., 2019). Understanding these factors is crucial for optimizing the efficiency and effectiveness of nanomaterial-based remediation strategies.
4.1 pH impact
The solution pH plays a crucial role in the interactions between nanomaterials and heavy metal ions. pH levels influence the surface charge of nanomaterials, consequently affecting their adsorption capacity. At lower pH values, increased proton reactions occur with nanomaterials like Nanoscale Zero-Valent Iron (NZVI), leading to a higher rate of conversion from H+ to H2, resulting in more reactive H+ and faster reduction rates (Suazo-Hernández et al., 2023; Y Zhang et al., 2022). Under neutral pH conditions, surface coordination, electrostatic sorption, and precipitation become more robust, leading to enhanced removal rates. Several studies have investigated the influence of pH on the adsorption capacity of nanoscale zero-valent iron (NZVI). Zhang et al. (2023) and Zhao et al. (2021) observed a significant decrease in NZVI’s adsorption ability under extreme alkaline or acidic conditions. This phenomenon can be attributed to the impact of pH on NZVI corrosion and its consequent reactive lifespan. Liu et al. (2014) explored the effect of pH on the removal efficiency of Hg(II) and Cr(VI) using pumice-supported NZVI in aqueous solutions. Their findings revealed that higher pH levels led to increased removal rates for Hg(II) but decreased removal rates for Cr(VI). Similarly, Wu et al. (2019) reported enhanced removal rates of Cr(VI) with FeS nanoparticles stabilized by sodium alginate at increasing pH levels (from 4.0 to 6.0). However, they also observed a decline in removal efficiency at a pH of 10.0. Xu and Zhao (2007) investigated the impact of pH on the immobilization of Cr(VI) in contaminated soil using carboxymethyl cellulose-stabilized NZVI. Lowering soil pH from 9.0 to 5.0 decreased Cr(VI) leaching from around 30%–20%. pH levels in aqueous solutions significantly influence the performance of nanomaterials, affecting their surface charge, reactivity, and adsorption capacity for heavy metals (Alli et al., 2023; Asmat-Campos et al., 2023; Assad et al., 2022; R Biswas et al., 2023).
4.2 Impact of contact duration
The length of time nanosorbents interact with metal ions plays a crucial role in the cost-efficient treatment of water or wastewater polluted with harmful heavy metals. Extended contact durations between pollutants and adsorbents enhance adsorption efficiency by prolonging the interaction between active chelation sites and the metals (Aghababai Beni and Jabbari, 2022; Ahmad et al., 2023; Areche et al., 2023). Usually, in the early stages of adsorption, the removal efficiency shows quick advancement, then progresses gradually. A study on the adsorption of divalent mercury ions onto carbon-based nanoparticles (CANPs) showed a significant increase in adsorption as the contact duration increased from 0 to 90 min (Dubey et al., 2016). The adsorption rate initially increased rapidly, then slowed down gradually, and finally stabilized at 90 min.
Another study examined how divalent cadmium and lead ions bind to the surfaces of composite materials made from magnetic nanoparticles (Hasanzadeh et al., 2017). Adsorption efficiency reached 91% within the initial 20 min of contact, achieving the maximum adsorption capacity of 48.54 mg/g for Cd(II) ions and 100% efficiency with a capacity of 53.35 mg/g for Pb(II) ions (Hasanzadeh et al., 2017).
4.3 Adsorbent dosage impact
The amount of pollutants eliminated is directly proportional to the dosage of adsorbent used in the adsorption process. The increase in dosage results in a greater number of active sites that can bind to heavy metals, hence improving the ability to absorb these metals (T Ahmed et al., 2023; Baby et al., 2022; Chandran et al., 2023; Li et al., 2021). Nevertheless, even though there is a beneficial impact, the effectiveness of active sites declines when the surface area becomes smaller, worsened by the clumping together of nanoparticles, finally limiting the ability to adsorb. Several research studies have examined how varying amounts of adsorbents affect the removal of heavy metals from water solutions (Kaur and Roy, 2021; Guo et al., 2022; Kumar & Kumar, 2023). An instance of using TiO2-coated chitosan was to eliminate divalent copper and lead ions from water (Razzaz et al., 2016; Kashi et al., 2024). The concentration utilized for this procedure was 2000 mg/L. It was noted that exceeding the ideal dosage of nanosorbent resulted in the buildup of nanoparticles and reduction of surface area, which in turn diminished the ability to adsorb harmful metals.
Similarly, Huang et al. (Huang et al., 2017; Huang et al., 2019) showed that increasing the amount of adsorbent from 0.5 to 2 g/L enhanced the efficacy of Fe3O4@C nano adsorbent in eliminating lead ions from water, resulting in an increase in removal efficiency from 41% to 92%. Nevertheless, a higher dosage of the adsorbent led to a decrease in the efficiency of removal, decreasing from 41% to 22%. This fall can be due to the clustering of the active sites responsible for binding lead ions. In a separate investigation, Nithya et al. (K Nithya et al., 2018; R Nithya et al., 2021) conducted an experiment using superparamagnetic iron oxide nanoparticles at varying doses between 0.1 and 1.8 g. The objective was to eliminate Ni2+ from aqueous solutions within a 90-min timeframe. (Rad et al., 2014). The researchers discovered that the highest level of removal effectiveness, reaching 99%, was attained when using an adsorbent dosage of 0.2 g (K Nithya et al., 2018). The quantity of nanomaterials employed is a critical determinant affecting the efficacy of heavy metal ion removal. Multiple studies have identified the optimal quantities of adsorbents needed to achieve specific degrees of pollutant removal, aiding in the efficient and cost-effective utilization of nanomaterials. Arshadi et al., 2014 found that increasing the quantity of immobilized NZVI on sineguelas waste biomaterial from 0.05 to 0.15 g resulted in a rise in the clearance rate of Pb(II) from 15.6% to 89%. However, escalating the dosage further did not notably enhance the elimination effectiveness.
Fu et al., 2015 investigated how sepiolite-supported NZVI performed in removing Cr(VI) and Pb(II) from groundwater. Increasing the adsorbent quantity from 0.05 to 3.2 g/L improved the removal rates of Cr(VI) and Pb(II). The most effective dosage was determined to be 1.6 g/L, although clearance rates only showed a small improvement above this concentration. Zand et al., 2020 did a study using different quantities of TiO2 nanoparticles for phytoremediation of soil contaminated with Cd. Higher dosages of TiO2 NPs were found to enhance the absorption of Cd by Trifolium repens. Excessive doses led to a reduction in plant biomass as a result of toxicity.
4.4 The effect of temperature
The kinetics of adsorption processes incorporating nanoparticles are greatly affected by temperature. The temperature variations can influence the speed at which heavy metal ions are adsorbed, diffused, and desorbed on the surface of nanomaterials (J Liu et al., 2020). Elevated temperatures can promote the movement of metal ions and intensify their interaction with nanomaterials, leading to an augmentation in adsorption capacity (Shi et al., 2023). Nevertheless, high or low temperatures might induce alterations or clustering of nanomaterials, which can have a detrimental impact on their functionality (Behnam and Firouzi, 2023; Khorram Abadi et al., 2023). The temperature-dependent energy of reaction activity is a critical factor in the adsorption process. Temperature variations significantly affect the equilibrium adsorption capacity of nanomaterials. Elevated temperatures lead to a reduction in the interparticle spacing and expedite the redox reaction process (Yu et al., 2023). Dubey et al., 2016 investigated to evaluate the efficacy of chitosan-alginate nanoparticles in the removal of Hg(II) across a temperature range of 10°C–40°C. The results revealed an enhancement in removal efficiency with increasing temperatures, peaking at 30°C and subsequently declining gradually. Similar trends were consistently observed across various studies (Roostaee et al., 2022; Khorram Abadi et al., 2023; Morales et al., 2023). Nassar (Nassar, 2010) noted an increase in the adsorption of Pb(II) using Fe3O4 nanoparticles at elevated temperatures, particularly within the range of 298–328 K, indicative of an endothermic adsorption process. Furthermore, Liu et al. (1996) investigated the immobilization of Re(VII) in soil and groundwater employing starch-stabilized NZVI. They observed a positive correlation between temperature and immobilization efficiency, with higher temperatures ranging from 15°C to 45°C resulting in increased efficiency, consistent with the principles outlined in the Arrhenius equation.
4.5 Effect of ionic strength
A solution’s ionic strength, or ion concentration, is an important determinant of nanomaterials’ efficacy in heavy metal removal. According to Yang et al. (F Yang and Yang, 2022; G Yang et al., 2022), nanomaterials may have their adsorption effectiveness reduced in solutions with high ionic strength because metal ions compete more strongly with other ions in the solution. An increase in ionic strength may also affect nanomaterial performance by changing their stability and aggregation behaviour (L Kong et al., 2023; Q Kong et al., 2021). Adsorption processes rely on ionic strength, which quantifies the effect of extra ions on molecule adsorption onto the adsorbent surface (Y Liu et al., 2022; Musso et al., 2019), as it estimates the concentration of ions dissolved in a solution. To explore how experimental ionic strength affects chelation efficacy, ions like Cl− and Na + are commonly added to the solution. The concentrations and affinities of these extra ions can greatly impact the adsorption effectiveness.
The role of ionic strength in particle aggregation cannot be overstated when considering electrostatic interactions. Particles aggregate when ionic strength increases, which lowers electrostatic repulsion and, consequently, the number of available binding sites and the number of ions that can be adsorbed (Y Liu et al., 2022). Sodium chloride, at a concentration of 0.025 mM, enhanced the surface functional group dispersion of Fe3O4/SiO2/GSH nanoparticles, which in turn promoted the adsorption of Pb2+ ions (P Xu et al., 2017). Increasing the sodium chloride concentration to 0.2 mM reduced lead ion adsorption, perhaps because it decreased the number of available chelation sites (P Xu et al., 2017). Hasanzadeh et al., 2017 showed that the nanocomposite surface’s affinity for Cd2+ and Pb2+ ions over Na + ions remained constant, as heavy metal ion adsorption was unaffected even in the presence of 3 mol/L NaCl. These data highlight the sensitivity of the adsorption process to sodium chloride content, as well as other parameters that influence the adsorbent’s affinity for the target adsorbate.
5 Navigating the nano-revolution: environmental impacts and challenges of nanomaterial-based heavy metal remediation
Although nanoparticles present promising opportunities for wastewater treatment due to their versatile features and ability to selectively remove various contaminants through mechanisms such as adsorption and photocatalysis, there are still some obstacles that need to be overcome (Elsaid et al., 2023). The exponential advancement of nanomaterials surpasses our comprehension of their potential environmental and health hazards (Table 8). Significant areas of knowledge that need to be addressed include the mechanisms by which nanomaterials are released, transported, and exhibit behavior in water systems. Furthermore, it is necessary to conduct thorough toxicological studies to investigate any negative health consequences at appropriate dosages. Membrane filtration, a current treatment technique, is hindered by issues such as pore obstruction and reduced effectiveness over time caused by fouling (Morales et al., 2023; Roostaee et al., 2022; Yaqoob et al., 2020). Likewise, the ability to be used again is a significant obstacle for nanosorbents. Optimally, these materials should effectively eliminate contaminants while facilitating straightforward post-treatment retrieval. The US Environmental Protection Agency (USEPA) has recognized crucial inquiries regarding the elimination of nanoparticles throughout the process of wastewater treatment. These factors encompass comprehending the processes involved in eliminating nanoparticles, their interactions with other pollutants, and the efficacy of conventional techniques such as coagulation and carbon adsorption. Moreover, it is necessary to develop tests in order to detect probable degradation products that are produced during the treatment process using these approaches. Continued research is essential to create economical and reliable methods for assessing the intricate characteristics of nanoparticles in wastewater treatment (Babu et al., 2021; Elsaid et al., 2023; Ahmed et al., 2024).
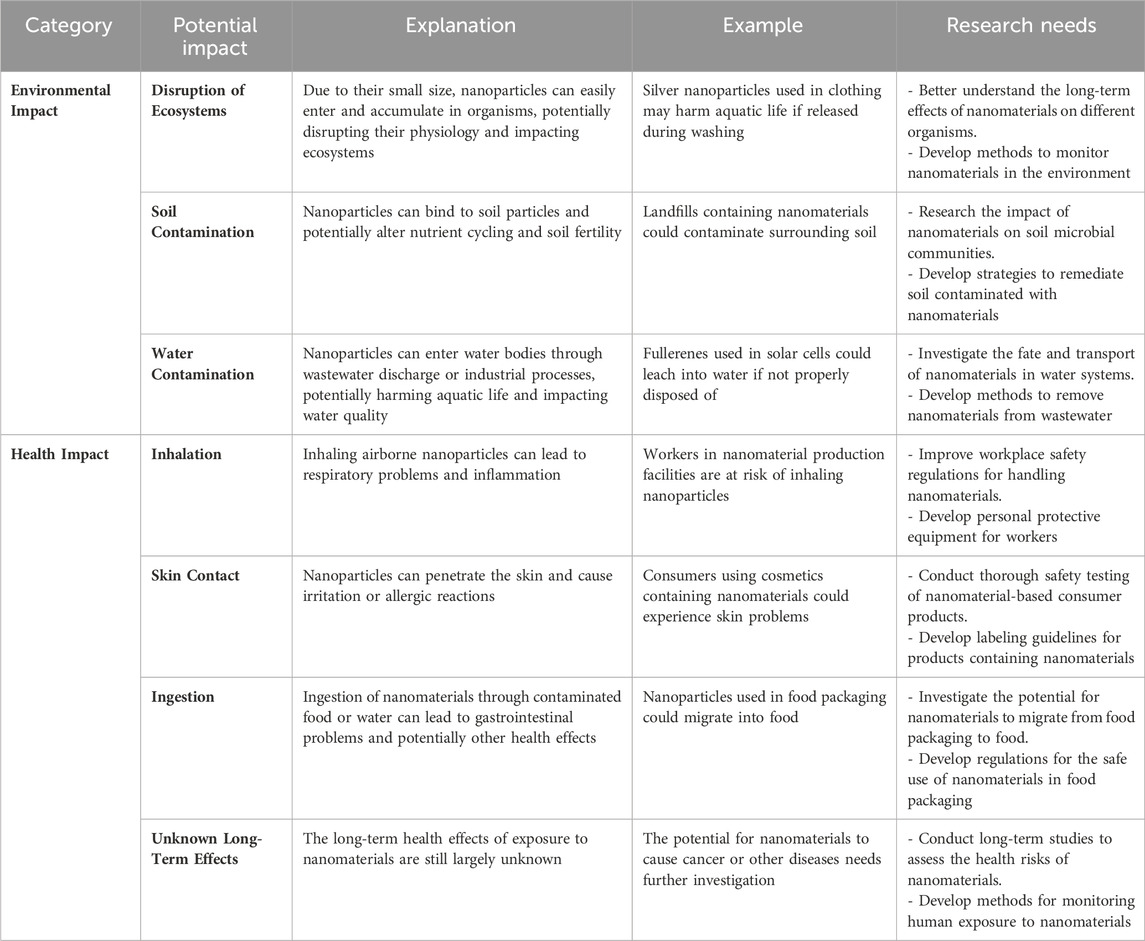
Table 8. Potential Environmental and Health Impacts of Nanomaterials (Ahmed et al., 2024; Babu et al., 2021; Elsaid et al., 2023; Roostaee et al., 2022; Yaqoob et al., 2020).
5.1 Advantages of nanomaterials
• High Efficiency and Selectivity: Due to their large surface area and unique functionalities, nanomaterials can effectively capture and remove even very low concentrations of specific heavy metals from aqueous solutions and soil.
• Versatility and Tunability: Different types of nanomaterials can be tailored to target various metals through surface modifications and functionalization, offering a highly adaptable approach.
• Potential In-situ Remediation: Certain nanomaterials can be directly injected into contaminated sites, enabling targeted and efficient decontamination without extensive excavation.
5.2 Challenges and environmental concerns
• Limited Understanding of Long-Term Impacts: The long-term fate and behavior of nanomaterials in the environment are still not fully understood, raising concerns about potential unintended consequences.
• Unforeseen Toxicity and Ecotoxicity: Some nanomaterials can exhibit toxicity towards organisms, potentially harming beneficial bacteria and disrupting ecosystems.
• Release and Persistence in the Environment: Accidental release or leaching of nanomaterials from treatment sites can lead to their accumulation in various environmental compartments, posing risks to water resources and food chains.
• Difficulties in Recovery and Reuse: Efficient and cost-effective methods for recovering and reusing spent nanomaterials after remediation are still under development, raising concerns about waste management.
• Regulatory Uncertainty: As nanotechnology is a rapidly evolving field, regulatory frameworks often struggle to keep pace, creating challenges for responsible development and application.
5.3 Mitigating the risks
Several strategies can be adopted to minimize the environmental impact of nanomaterial-based remediation:
• Environmental Design and Risk Assessment: Developing nanomaterials with inherent biodegradability or biocompatibility and conducting thorough risk assessments before large-scale application.
• Surface Modification and Coating: Coating nanomaterials with biocompatible and environmentally friendly materials can reduce their toxicity and enhance their stability.
• Development of Recovery Technologies: Investing in efficient and cost-effective methods for collecting and reusing spent nanomaterials is crucial for sustainable application.
• Improved Regulation and Collaboration: Strengthening regulatory frameworks and promoting collaboration between scientists, engineers, and policymakers is essential for responsible development and deployment.
5.4 The future of nanoremediation
Despite the challenges, nanotechnology holds immense potential for revolutionizing heavy metal remediation. Through ongoing research, development, and responsible implementation, the risks can be mitigated while harnessing the benefits of these transformative technologies. Public awareness, engagement, and transparent communication are all vital aspects of building trust and ensuring the responsible development and application of nanomaterial-based solutions for a cleaner and healthier environment.
6 Conclusion
In conclusion, the advancements in nanotechnology and nanoscience have heralded a new era in environmental engineering, offering many eco-friendly, cost-effective, and efficient materials for addressing pressing challenges such as heavy metal pollution in water. The unique physicochemical properties of nanomaterials make them highly promising for water treatment, particularly in removing heavy metals, owing to their high adsorption capacity and selectivity, even at low concentrations. Throughout this review, we have explored the efficacy of various nanomaterials, ranging from zeolites, polymers, chitosan, and metal oxides to metals, in extracting heavy metals from water under diverse environmental conditions. Moreover, the functionalization of nanomaterials has emerged as a strategic approach to enhance separation efficiency, stability, and adsorption capacity, achieved through the incorporation of molecules such as biomolecules, polymers, and inorganic materials.
However, despite the remarkable potential of engineered nanomaterials, several challenges and limitations persist, including issues related to aggregation, stability, mechanical strength, long-term performance, and scalability. Furthermore, nanomaterials’ potential environmental and health impacts necessitate thorough investigation and consideration. In order to overcome these issues and create sustainable nanomaterial-based remediation techniques, focused efforts must be made in the future. This will require interdisciplinary collaboration, adherence to green chemistry principles, and comprehensive risk assessments to ensure nanomaterials’ safe and effective deployment in heavy metal remediation at both laboratory and large-scale levels.
Further research endeavours are essential to optimize synthesis processes, enhance reusability and separation techniques, and minimize environmental impacts. By doing so, we can contribute to developing robust solutions for mitigating heavy metal pollution in water and safeguarding human health and the environment for future generations. In essence, while the journey towards harnessing the full potential of nanomaterials for heavy metal remediation may be challenging, it is a journey worth undertaking to improve society and preserve our planet’s precious water resources.
Author contributions
AK: Conceptualization, Methodology, Supervision, Validation, Writing–original draft, Writing–review and editing. TM: Data curation, Formal Analysis, Investigation, Resources, Writing–original draft.
Funding
The author(s) declare that no financial support was received for the research, authorship, and/or publication of this article.
Acknowledgments
I am obliged to Lovely Professional University, Phagwara, and Jimma University, Ethiopia to conduct my research.
Conflict of interest
The authors declare that the research was conducted in the absence of any commercial or financial relationships that could be construed as a potential conflict of interest.
Publisher’s note
All claims expressed in this article are solely those of the authors and do not necessarily represent those of their affiliated organizations, or those of the publisher, the editors and the reviewers. Any product that may be evaluated in this article, or claim that may be made by its manufacturer, is not guaranteed or endorsed by the publisher.
References
Abbas, W. T. (2021). Advantages and prospective challenges of nanotechnology applications in fish cultures: a comparative review. Environ. Sci. Pollut. Res. 28 (7), 7669–7690. doi:10.1007/s11356-020-12166-0
Abbasi, N., and Khan, T. A. (2021). “Progress in carbon nanotubes for water treatment,” in Contamination of water: health risk assessment and treatment strategies, 509–518.
Abd El-Aziz, S. M., Sleem, A. A., and Abdel Maksoud, M. I. A. (2023). Comparative study of the antioxidant, toxicity, anti-inflammatory, and wound healing activities of both Digenea simplex polysaccharides and their corresponding (ZnO–Au) bimetallic nanoparticles. Cellulose 30 (1), 303–321. doi:10.1007/s10570-022-04878-y
Abdelbasir, S. M., and Shalan, A. E. (2019). An overview of nanomaterials for industrial wastewater treatment. Korean J. Chem. Eng. 36 (8), 1209–1225. doi:10.1007/s11814-019-0306-y
Abd Elnabi, M. K., Elkaliny, N. E., Elyazied, M. M., Azab, S. H., Elkhalifa, S. A., Elmasry, S., et al. (2023). Toxicity of heavy metals and recent advances in their removal: a review. Toxics 11 (7), 580. doi:10.3390/toxics11070580
Abdel Wahab, M. M., Sayed, G. H., Ramadan, R. M., Mady, A. H., Rabie, A. M., Farag, A. A., et al. (2023). Synergistic effects of graphene oxide grafted with barbituric acid nanocomposite for removal of heavy metals from aqueous solution. Nanotechnol. Environ. Eng. 8 (2), 347–359. doi:10.1007/s41204-022-00274-w
Abdullah, T. A., Rasheed, R. T., Adnan, M., Qasim, B., Sajet, F., Mansoor, H. S., et al. (2023). A comprehensive review of the application of modified carbon nanotubes (CNTs) for the removal of metals from wastewater. Mater. Res. Express 10 (12), 122005. doi:10.1088/2053-1591/ad1770
Abobakr, S. M., and Abdo, N. I. (2022). Adsorption studies on chromium ion removal from aqueous solution using magnetite nanoparticles. Egypt. J. Chem. 65 (8), 0–29. doi:10.21608/ejchem.2022.95319.4475
Abo Markeb, A., Moral-Vico, J., Sánchez, A., and Font, X. (2023). Optimization of lead (II) removal from water and wastewater using a novel magnetic nanocomposite of aminopropyl triethoxysilane coated with carboxymethyl cellulose cross-linked with chitosan nanoparticles. Arabian J. Chem. 16 (8), 105022. doi:10.1016/j.arabjc.2023.105022
Abou-Zeid, R. E., Dacrory, S., Ali, K. A., and Kamel, S. (2018). Novel method of preparation of tricarboxylic cellulose nanofiber for efficient removal of heavy metal ions from aqueous solution. Int. J. Biol. Macromol. 119, 207–214. doi:10.1016/j.ijbiomac.2018.07.127
Abu, S. R. M. (2022). Nanotechnology in wastewater treatment. Compr. Anal. Chem. 99, 105–134. doi:10.1016/bs.coac.2021.11.002
Abuhatab, S., Pal, S., Roberts, E. P. L., and Trifkovic, M. (2023). Electrochemical regeneration of highly stable and sustainable cellulose/graphene adsorbent saturated with dissolved organic dye. Langmuir. doi:10.1021/acs.langmuir.3c03265
Adisasmito, S., Pramudita, D., Sumampouw, G. A., Mohtar, WHMW, and Indarto, A. (2023). “Sustainable applications and prospects of nanoadsorbents for wastewater treatment,” in Adsorption through advanced Nanoscale materials: applications in environmental remediation, 533–584.
Agha, H. M., Abdulhameed, A. S., Jawad, A. H., Aazmi, S., Sidik, N. J., De Luna, Y., et al. (2024). Enhancing cationic dye removal via biocomposite formation between chitosan and food grade algae: optimization of algae loading and adsorption parameters. Int. J. Biol. Macromol. 258, 128792. doi:10.1016/j.ijbiomac.2023.128792
Aghababai Beni, A., and Jabbari, H. (2022). Nanomaterials for environmental applications. Results Eng. 15, 100467. doi:10.1016/j.rineng.2022.100467
Ahlawat, W., Dilbaghi, N., and Kumar, S. (2021). Evaluation of graphene oxide and its composite as potential sorbent for removal of cationic and anionic dyes. in Paper presented at the materials today: proceedings.
Ahmad, H., and Liu, C. (2021). Ultra-thin graphene oxide membrane deposited on highly porous anodized aluminum oxide surface for heavy metal ions preconcentration. J. Hazard. Mater. 415, 125661. doi:10.1016/j.jhazmat.2021.125661
Ahmad, M. A., Adeel, M., Shakoor, N., Ali, I., Ishfaq, M., Haider, F. U., et al. (2023). Unraveling the roles of modified nanomaterials in nano enabled agriculture. Plant Physiology Biochem. 202, 107944. doi:10.1016/j.plaphy.2023.107944
Ahmadian, Z., Kazeminava, F., Afrouz, M., Abbaszadeh, M., Mehr, N. T., Shiran, J. A., et al. (2023). A review on the impacts of metal/metal nanoparticles on characteristics of hydrogels: special focus on carbohydrate polymers. Int. J. Biol. Macromol. 253, 126535. doi:10.1016/j.ijbiomac.2023.126535
Ahmed, A. M., Mekonnen, M. L., and Mekonnen, K. N. (2023). Review on nanocomposite materials from cellulose, chitosan, alginate, and lignin for removal and recovery of nutrients from wastewater. Carbohydr. Polym. Technol. Appl. 6, 100386. doi:10.1016/j.carpta.2023.100386
Ahmed, S. F., Kumar, P. S., Ahmed, B., Mehnaz, T., Shafiullah, G. M., Nguyen, V. N., et al. (2024). Carbon-based nanomaterials: characteristics, dimensions, advances and challenges in enhancing photocatalytic hydrogen production. Int. J. Hydrogen Energy 52, 424–442. doi:10.1016/j.ijhydene.2023.03.185
Ahmed, T., Noman, M., Rizwan, M., Ali, S., Shahid, M. S., and Li, B. (2023). Recent progress on the heavy metals ameliorating potential of engineered nanomaterials in rice paddy: a comprehensive outlook on global food safety with nanotoxicitiy issues. Crit. Rev. Food Sci. Nutr. 63 (16), 2672–2686. doi:10.1080/10408398.2021.1979931
Alamier, W. M., Hasan, N., Syed, I. S., Bakry, A. M., Ismail, K. S., Gedda, G., et al. (2023). Silver nanoparticles’ biogenic synthesis using caralluma subulata aqueous extract and application for dye degradation and antimicrobials activities. Catalysts 13 (9), 1290. doi:10.3390/catal13091290
Alhalili, Z. (2023). Metal oxides nanoparticles: general structural description, chemical, physical, and biological synthesis methods, role in pesticides and heavy metal removal through wastewater treatment. Molecules 28 (7), 3086. doi:10.3390/molecules28073086
Ali, A., Javed, R., Farhangi, S., Shah, T., Ullah, S., ul Ain, N., et al. (2023). Metal phenolic networks (MPNs)-based pH-sensitive stimulus responsive nanosystems for drug delivery in tumor microenvironment. J. Drug Deliv. Sci. Technol. 84, 104536. doi:10.1016/j.jddst.2023.104536
Al-Jubouri, S. M., and Holmes, S. M. (2020). Immobilization of cobalt ions using hierarchically porous 4A zeolite-based carbon composites: ion-exchange and solidification. J. Water Process Eng. 33, 101059. doi:10.1016/j.jwpe.2019.101059
Alli, Y. A., Oladoye, P. O., Ejeromedoghene, O., Bankole, O. M., Alimi, O. A., Omotola, E. O., et al. (2023). Nanomaterials as catalysts for CO2 transformation into value-added products: a review. Sci. Total Environ. 868, 161547. doi:10.1016/j.scitotenv.2023.161547
Almomani, F., Bhosale, R., Khraisheh, M., kumar, A., and Almomani, T. (2020). Heavy metal ions removal from industrial wastewater using magnetic nanoparticles (MNP). Appl. Surf. Sci. 506, 144924. doi:10.1016/j.apsusc.2019.144924
Aloulou, W., Aloulou, H., and Amar, R. B. (2022). Low-cost composite ultrafiltration membrane made from TiO2 and nanocomposite clay materials over zeolite support for oily wastewater purification and heavy metals removal. Desalination Water Treat. 246, 166–173. doi:10.5004/dwt.2022.28027
Alshehri, A., Alharbi, L., Wani, A. A., and Malik, M. A. (2024). Biogenic punica granatum flower extract assisted ZnFe2O4 and ZnFe2O4-Cu composites for excellent photocatalytic degradation of RhB dye. Toxics 12 (1), 77. doi:10.3390/toxics12010077
Alzahrani, F. M., Alsaiari, N. S., Katubi, K. M., Amari, A., and Tahoon, M. A. (2022). Synthesis, characterization, and application of magnetized lanthanum (III)-Based metal-organic framework for the organic dye removal from water. Adsorpt. Sci. Technol. 2022. doi:10.1155/2022/3513829
Amariei, G., Valenzuela, L., Iglesias-Juez, A., Rosal, R., and Visa, M. (2022). ZnO-functionalized fly-ash based zeolite for ciprofloxacin antibiotic degradation and pathogen inactivation. J. Environ. Chem. Eng. 10 (3), 107603. doi:10.1016/j.jece.2022.107603
Ani, I. J., and Egbosiuba, T. C. (2023). “Metal oxides and their nanocomposites in wastewater treatment,” in Adsorption through advanced Nanoscale materials: applications in environmental remediation, 185–203.
Aragaw, T. A., and Ayalew, A. A. (2022). “Application of metal-based nanoparticles for metal removal for treatments of wastewater -- a review,” in Emerging techniques for treatment of toxic metals from wastewater (Elsevier), 183–231.
Areche, F. O., Mamani, C. M. C., Cárdenas, J. A. L., Sumarriva-Bustinza, L. A., Pastrana, P. A. P., Porras-Roque, M. S., et al. (2023). A comprehensive review on monitoring and purification of water through tunable 2D nanomaterials. Braz. J. Biol. 83, e273843. doi:10.1590/1519-6984.273843
Arshadi, M., Soleymanzadeh, M., Salvacion, J. W. L., and SalimiVahid, F. (2014). Nanoscale Zero-Valent Iron (NZVI) supported on sineguelas waste for Pb(II) removal from aqueous solution: kinetics, thermodynamic and mechanism. J. Colloid Interface Sci. 426, 241–251. doi:10.1016/j.jcis.2014.04.014
Arun, K. V. B., Dohroo, A., and Kumar Upadhyay, A.Ritu Bala %J Pollution Research (2018). Effect of microbial surfactants on heavy metal polluted wastewater, 37, 39–46.
Arun Karnwal, N. C. (2012). Bioremediation of heavy metals. LAP Lambert Academic Publishing GmbH & Co. KG.
Asadi, R., Abdollahi, H., Gharabaghi, M., and Boroumand, Z. (2020). Effective removal of Zn (II) ions from aqueous solution by the magnetic MnFe2O4 and CoFe2O4 spinel ferrite nanoparticles with focuses on synthesis, characterization, adsorption, and desorption. Adv. Powder Technol. 31 (4), 1480–1489. doi:10.1016/j.apt.2020.01.028
Asif, M. B., Zhang, Z., Iftekhar, S., and Lehto, V. P. (2021). “Polysaccharide-derived biopolymeric nanomaterials for wastewater treatment,” in Biopolymeric nanomaterials: fundamentals and applications, 447–469.
Asmat-Campos, D., Lindsay Rojas, M., and Carreño-Ortega, A. (2023). Toward sustainable nanomaterials: an innovative ecological approach for biogenic synthesis of TiO2 nanoparticles with potential photocatalytic activity. Clean. Eng. Technol. 17, 100702. doi:10.1016/j.clet.2023.100702
Assad, H., Fatma, I., Kumar, A., Kaya, S., Vo, D. V. N., Al-Gheethi, A., et al. (2022). An overview of MXene-Based nanomaterials and their potential applications towards hazardous pollutant adsorption. Chemosphere 298, 134221. doi:10.1016/j.chemosphere.2022.134221
Azimi, A., Azari, A., Rezakazemi, M., and Ansarpour, M. (2017). Removal of heavy metals from industrial wastewaters: a review. ChemBioEng Rev. 4 (1), 37–59. doi:10.1002/cben.201600010
Babar, Z. B., Shahi, A., Rauf, A., Sattar, H., and Rizwan, K. (2022). “Organic–inorganic nanohybrids for the removal of environmental pollutants,” in Materials horizons: from nature to nanomaterials, 277–309.
Babu, P. J., Ningthoujam, R., Singh, Y. D., Sarma, M. K., and Panda, M. K. (2021). “NANOMATERIALS MEDIATED WASTEWATER TREATMENT,” in Nano-biotechnological advancements in environmental issues: applications and challenges, 65–99.
Baby, R., Hussein, M. Z., Abdullah, A. H., and Zainal, Z. (2022). Nanomaterials for the treatment of heavy metal contaminated water. Polymers 14 (3), 583. doi:10.3390/polym14030583
Bahadi, S. A., Drmosh, Q. A., and Onaizi, S. A. (2024). Adsorption of anionic and cationic azo dyes from wastewater using novel and effective multicomponent adsorbent. Sep. Purif. Technol. 337, 126402. doi:10.1016/j.seppur.2024.126402
Balakumar, V., and Manivannan, R. (2021). “Metal oxide-graphitic carbon nitride nanocomposite-modified electrochemical sensors for toxic chemicals,” in Metal oxides in nanocomposite-based electrochemical sensors for toxic chemicals, 263–292.
Behnam, H., and Firouzi, A. F. (2023). Effects of synthesis method, feedstock type, and pyrolysis temperature on physicochemical properties of biochar nanoparticles. Biomass Convers. Biorefinery 13 (15), 13859–13869. doi:10.1007/s13399-021-02108-2
Beiranvand, M., Farhadi, S., and Mohammadi-Gholami, A. (2022). Adsorptive removal of tetracycline and ciprofloxacin drugs from water by using a magnetic rod-like hydroxyapatite and MIL-101(Fe) metal-organic framework nanocomposite. RSC Adv. 12 (53), 34438–34453. doi:10.1039/d2ra06213e
Bhagat, N. R., and Giri, A. (2021). “Nanotechnology for detection and removal of heavy metals from contaminated water,” in Functionalized nanomaterials for catalytic application, 185–226.
Bhandari, H., Garg, S., and Gaba, R. (2021). Advanced nanocomposites for removal of heavy metals from wastewater. Macromol. Symp. 397 (1). doi:10.1002/masy.202000337
Bichave, M. S., Kature, A. Y., Koranne, S. V., Shinde, R. S., Gongle, A. S., Choudhari, V. P., et al. (2023). Nano-metal oxides-activated carbons for dyes removal: a review. Mater. Today Proc. 77, 19–30. doi:10.1016/j.matpr.2022.08.451
Bilal, M., Ikram, M., Shujah, T., Haider, A., Naz, S., Ul-Hamid, A., et al. (2022). Chitosan-Grafted polyacrylic acid-doped copper oxide nanoflakes used as a potential dye degrader and antibacterial agent: in silico molecular docking analysis. ACS Omega 7 (45), 41614–41626. doi:10.1021/acsomega.2c05625
Biswas, R., Anshuman, A., and Samal, B. N. (2023). A review on arsenic removal from wastewater using carbon nanotube and graphene-based nanomaterials as adsorbents. Nanotechnol. Environ. Eng. 8 (4), 1033–1046. doi:10.1007/s41204-023-00332-x
Biswas, S., Danish, M., and Pal, A. (2021). “Nanomaterials for the removal of heavy metals from water,” in Nanomaterials for water treatment and remediation, 181–215.
Bui, N. T., Duong, L. T. B., Nguyen, T. T. C., and Tran, N. T. T. (2021). Heavy metal removal from polluted water by Nopal cactus biopolymer-based magnetic nanocomposites. Int. J. Nanoparticles 13 (1), 42–61. doi:10.1504/IJNP.2021.114901
Cano, F. J., Reyes-Vallejo, O., Ashok, A., Olvera, MDLL, Velumani, S., and Kassiba, A. (2023). Mechanisms of dyes adsorption on titanium oxide– graphene oxide nanocomposites. Ceram. Int. 49 (13), 21185–21205. doi:10.1016/j.ceramint.2023.03.249
Cegłowski, M., Gierczyk, B., Frankowski, M., and Popenda, Ł. (2018). A new low-cost polymeric adsorbents with polyamine chelating groups for efficient removal of heavy metal ions from water solutions. React. Funct. Polym. 131, 64–74. doi:10.1016/j.reactfunctpolym.2018.07.006
Cevallos-Mendoza, J., Amorim, C. G., Rodríguez-Díaz, J. M., and Montenegro, MDCBSM (2022). Removal of contaminants from water by membrane filtration: a review. Membranes 12 (6), 570. doi:10.3390/membranes12060570
Chadha, U., Selvaraj, S. K., Vishak Thanu, S., Cholapadath, V., Abraham, A. M., Zaiyan, M. M., et al. (2022). A review of the function of using carbon nanomaterials in membrane filtration for contaminant removal from wastewater. Mater. Res. Express 9 (1). doi:10.1088/2053-1591/ac48b8
Chakraborty, U., Kaur, G., Rubahn, H. G., Kaushik, A., Chaudhary, G. R., and Mishra, Y. K. (2023). Advanced metal oxides nanostructures to recognize and eradicate water pollutants. Prog. Mater. Sci. 139, 101169. doi:10.1016/j.pmatsci.2023.101169
Chandran, D. G., Muruganandam, L., and Biswas, R. (2023). A review on adsorption of heavy metals from wastewater using carbon nanotube and graphene-based nanomaterials. Environ. Sci. Pollut. Res. 30 (51), 110010–110046. doi:10.1007/s11356-023-30192-6
Chandrani, D. N., Ghosh, S., and Tanna, A. R. (2024). Green synthesis for fabrication of cobalt ferrite nanoparticles with photocatalytic dye degrading potential as a sustainable effluent treatment strategy. J. Inorg. Organomet. Polym. Mater. doi:10.1007/s10904-023-02981-6
Chauhan, P., Nehra, S., Sharma, R., and Kumar, D. (2022). Biopolymers supported nanomaterials for water treatment. in Applications of advanced nanomaterials in water treatment (Boca Raton: CRC Press), 63–80.
Chen, Y., Yang, G., Liu, B., Kong, H., Xiong, Z., Guo, L., et al. (2022). Biomineralization of ZrO2 nanoparticles on graphene oxide-supported peptide/cellulose binary nanofibrous membranes for high-performance removal of fluoride ions. Chem. Eng. J. 430, 132721. doi:10.1016/j.cej.2021.132721
Choi, W. S., and Lee, H. J. (2022). Nanostructured materials for water purification: adsorption of heavy metal ions and organic dyes. Polymers 14 (11), 2183. doi:10.3390/polym14112183
Danesh, N., Hosseini, M., Ghorbani, M., and Marjani, A. (2016). Fabrication, characterization and physical properties of a novel magnetite graphene oxide/Lauric acid nanoparticles modified by ethylenediaminetetraacetic acid and its applications as an adsorbent for the removal of Pb(II) ions. Synth. Met. 220, 508–523. doi:10.1016/j.synthmet.2016.07.025
Deravanesiyan, M., Beheshti, M., and Malekpour, A. (2015). The removal of Cr (III) and Co (II) ions from aqueous solution by two mechanisms using a new sorbent (alumina nanoparticles immobilized zeolite) - equilibrium, kinetic and thermodynamic studies. J. Mol. Liq. 209, 246–257. doi:10.1016/j.molliq.2015.05.038
Dhara, P., and Grattan, K. T. (2024). “Nanomaterials for recovery of lead heavy metal from wastewater,” in Application of nanotechnology for resource recovery from wastewater, 178–215.
Dong, X., Bhattacharjee, S., Zhang, C., and Chai, F. (2021). Titanium carbide-based adsorbents for removal of heavy metal ions and radionuclides: from nanomaterials to 3D architectures. Adv. Mater. Interfaces 8 (21). doi:10.1002/admi.202100703
Dubey, R., Bajpai, J., and Bajpai, A. K. (2016). Chitosan-alginate nanoparticles (CANPs) as potential nanosorbent for removal of Hg (II) ions. Environ. Nanotechnol. Monit. Manag. 6, 32–44. doi:10.1016/j.enmm.2016.06.008
Dutta, T., Barman, A., Bhattacherjee, S., Chakraborty, J., and Dutta, T. (2023). Antimicrobial silver nanoparticles for water disinfection: a short review on recent advances. Nanotechnol. Environ. Eng. 9, 111–131. doi:10.1007/s41204-023-00354-5
Efome, J. E., Rana, D., Matsuura, T., and Lan, C. Q. (2019). Effects of operating parameters and coexisting ions on the efficiency of heavy metal ions removal by nano-fibrous metal-organic framework membrane filtration process. Sci. Total Environ. 674, 355–362. doi:10.1016/j.scitotenv.2019.04.187
Elakkiya, S., Arthanareeswaran, G., Ismail, A. F., Goh, P. S., and Lukka Thuyavan, Y. (2021). Review on characteristics of biomaterial and nanomaterials based polymeric nanocomposite membranes for seawater treatment application. Environ. Res. 197, 111177. doi:10.1016/j.envres.2021.111177
El Messaoudi, N., Ciğeroğlu, Z., Şenol, Z. M., Kazan-Kaya, E. S., Fernine, Y., Gubernat, S., et al. (2024). Green synthesis of CuFe2O4 nanoparticles from bioresource extracts and their applications in different areas: a review. Biomass Convers. Biorefinery. doi:10.1007/s13399-023-05264-9
El Mouden, A., El Messaoudi, N., El Guerraf, A., Bouich, A., Mehmeti, V., Lacherai, A., et al. (2023). Multifunctional cobalt oxide nanocomposites for efficient removal of heavy metals from aqueous solutions. Chemosphere 317, 137922. doi:10.1016/j.chemosphere.2023.137922
Elsaid, K., Olabi, A. G., Abdel-Wahab, A., Elkamel, A., Alami, A. H., Inayat, A., et al. (2023). Membrane processes for environmental remediation of nanomaterials: potentials and challenges. Sci. Total Environ. 879, 162569. doi:10.1016/j.scitotenv.2023.162569
El-Sawy, A. M., Salem, M. A., Salem, I. A., Hydara, M. M., and Zaki, A. B. (2023). Sonophotocatalytic degradation of malachite green in aqueous solution using six competitive metal oxides as a benchmark. Photochem. Photobiological Sci. 22 (3), 579–594. doi:10.1007/s43630-022-00336-7
Esmaeili, A., and Khoshnevisan, N. (2016). Optimization of process parameters for removal of heavy metals by biomass of Cu and Co-doped alginate-coated chitosan nanoparticles. Bioresour. Technol. 218, 650–658. doi:10.1016/j.biortech.2016.07.005
Fadia, P., Tyagi, S., Bhagat, S., Nair, A., Panchal, P., Dave, H., et al. (2021). Calcium carbonate nano- and microparticles: synthesis methods and biological applications. 3 Biotech. 11 (11), 457. doi:10.1007/s13205-021-02995-2
Fei, Y., and Hu, Y. H. (2023). Recent progress in removal of heavy metals from wastewater: a comprehensive review. Chemosphere 335, 139077. doi:10.1016/j.chemosphere.2023.139077
Fu, R., Yang, Y., Xu, Z., Zhang, X., Guo, X., and Bi, D. (2015). The removal of chromium (VI) and lead (II) from groundwater using sepiolite-supported nanoscale zero-valent iron (S-NZVI). Chemosphere 138, 726–734. doi:10.1016/j.chemosphere.2015.07.051
Gangaraju, G., Ganesh, G., Uma Devi, R., and Balakrishna, K. (2022). “Plant material assisted magnetic nanoparticles (MNPs) for the separation of inorganic pollutants,” in Phytonanotechnology (Springer Nature), 181–197.
Gao, M., Yang, L., Yang, S., Jiang, T., Wu, F., and Nagasaka, T. (2022). Simple aminated modified zeolite 4A synthesized using fly ash and its remediation of mercury contamination: characteristics and mechanism. Sustain. Switz. 14 (23), 15924. doi:10.3390/su142315924
Ge, F., Li, M. M., Ye, H., and Zhao, B. X. (2012). Effective removal of heavy metal ions Cd 2+, Zn 2+, Pb 2+, Cu 2+ from aqueous solution by polymer-modified magnetic nanoparticles. J. Hazard. Mater. 212, 366–372. doi:10.1016/j.jhazmat.2011.12.013
Ghaemi, N. (2016). A new approach to copper ion removal from water by polymeric nanocomposite membrane embedded with γ-alumina nanoparticles. Appl. Surf. Sci. 364, 221–228. doi:10.1016/j.apsusc.2015.12.109
Ghaemi, N., Madaeni, S. S., Daraei, P., Rajabi, H., Zinadini, S., Alizadeh, A., et al. (2015). Polyethersulfone membrane enhanced with iron oxide nanoparticles for copper removal from water: application of new functionalized Fe3O4 nanoparticles. Chem. Eng. J. 263, 101–112. doi:10.1016/j.cej.2014.10.103
Ghasemi, E., Heydari, A., and Sillanpää, M. (2017). Superparamagnetic Fe3O4@EDTA nanoparticles as an efficient adsorbent for simultaneous removal of Ag(I), Hg(II), Mn(II), Zn(II), Pb(II) and Cd(II) from water and soil environmental samples. Microchem. J. 131, 51–56. doi:10.1016/j.microc.2016.11.011
Godwin, J., Njimou, J. R., Abdus-Salam, N., Adegoke, H. I., Panda, P. K., Tripathy, B. C., et al. (2023). Nanosorbent based on coprecipitation of ZnO in goethite for competitive sorption of Cd(II)-Pb(II) and Cd(II)-Pb(II)-Ni(II) systems. J. Environ. Health Sci. Eng. doi:10.1007/s40201-023-00882-x
Gong, Z., Chan, H. T., Chen, Q., and Chen, H. (2021). Application of nanotechnology in analysis and removal of heavy metals in food and water resources. Nanomaterials 11 (7), 1792. doi:10.3390/nano11071792
Goyal, S., Dhanker, R., Hussain, T., Ferreira, A., Gouveia, L., Kumar, K., et al. (2023). Modern advancement in biotechnological applications for wastewater treatment through microalgae: a review. Water, Air, Soil Pollut. 234 (7), 417. doi:10.1007/s11270-023-06409-2
Guo, D., Huang, S., and Zhu, Y. (2022). The adsorption of heavy metal ions by poly (amidoamine) dendrimer-functionalized nanomaterials: a review. Nanomaterials 12 (11), 1831. doi:10.3390/nano12111831
Gupta, A. D., Rene, E. R., Giri, B. S., Pandey, A., and Singh, H. (2021). Adsorptive and photocatalytic properties of metal oxides towards arsenic remediation from water: a review. J. Environ. Chem. Eng. 9 (6), 106376. doi:10.1016/j.jece.2021.106376
Hasanzadeh, R., Moghadam, P. N., Bahri-Laleh, N., and Sillanpää, M. (2017). Effective removal of toxic metal ions from aqueous solutions: 2-Bifunctional magnetic nanocomposite base on novel reactive PGMA-MAn copolymer@Fe3O4 nanoparticles. J. Colloid Interface Sci. 490, 727–746. doi:10.1016/j.jcis.2016.11.098
Hasanzadeh, R., Najafi Moghadam, P., and Samadi, N. (2013). Synthesis and application of modified poly (styrene-alt-maleic anhydride) networks as a nano chelating resin for uptake of heavy metal ions. Polym. Adv. Technol. 24 (1), 34–41. doi:10.1002/pat.3046
Huang, Q., Liu, M., Chen, J., Wan, Q., Tian, J., Huang, L., et al. (2017). Facile preparation of MoS 2 based polymer composites via mussel inspired chemistry and their high efficiency for removal of organic dyes. Appl. Surf. Sci. 419, 35–44. doi:10.1016/j.apsusc.2017.05.006
Huang, Q., Liu, Y., Cai, T., and Xia, X. (2019). Simultaneous removal of heavy metal ions and organic pollutant by BiOBr/Ti 3 C 2 nanocomposite. J. Photochem. Photobiol. A Chem. 375, 201–208. doi:10.1016/j.jphotochem.2019.02.026
Iqbal, Z., Tanweer, M. S., and Alam, M. (2022). Recent advances in adsorptive removal of wastewater pollutants by chemically modified metal oxides: a review. J. Water Process Eng. 46, 102641. doi:10.1016/j.jwpe.2022.102641
Islam, A., Teo, S. H., Taufiq-Yap, Y. H., Ng, C. H., Vo, D. V. N., Ibrahim, M. L., et al. (2021). Step towards the sustainable toxic dyes removal and recycling from aqueous solution- A comprehensive review. Resour. Conservation Recycl. 175, 105849. doi:10.1016/j.resconrec.2021.105849
Islam, M. S., Choi, W. S., Nam, B., Yoon, C., and Lee, H. J. (2017). Needle-like iron oxide@CaCO3 adsorbents for ultrafast removal of anionic and cationic heavy metal ions. Chem. Eng. J. 307, 208–219. doi:10.1016/j.cej.2016.08.079
Jain, H., Dhupper, R., Verma, A. K., and Garg, M. C. (2021). Development of titanium dioxide incorporated ultrathin cellulose acetate membrane for enhanced forward osmosis performance. Nanotechnol. Environ. Eng. 6, 67–68. doi:10.1007/s41204-021-00161-w
Jain, H., Yadav, V., Rajput, V. D., Minkina, T., Agarwal, S., and Garg, M. C. (2022). An eco-sustainable green approach for biosorption of methylene blue dye from textile industry wastewater by sugarcane bagasse, peanut hull, and orange peel: a comparative study through response surface methodology, isotherms, kinetic, and thermodynamics. Water, Air, & Soil Pollut. 233 (6), 187. doi:10.1007/s11270-022-05655-0
Jarosz, R., Szerement, J., Gondek, K., and Mierzwa-Hersztek, M. (2022). The use of zeolites as an addition to fertilisers – a review. Catena 213, 106125. doi:10.1016/j.catena.2022.106125
Jjagwe, J., Olupot, P. W., and Carrara, S. (2023). Iron oxide nanoparticles/nanocomposites derived from steel and iron wastes for water treatment: a review. J. Environ. Manag. 343, 118236. doi:10.1016/j.jenvman.2023.118236
Joshi, N. C., Gururani, P., and Gairola, S. P. (2022). Metal oxide nanoparticles and their nanocomposite-based materials as photocatalysts in the degradation of dyes. Biointerface Res. Appl. Chem. 12 (5), 6557–6579. doi:10.33263/BRIAC125.65576579
Kanel, S. R., Greneche, J. M., and Choi, H. (2006). Arsenic(V) removal from groundwater using nano scale zero-valent iron as a colloidal reactive barrier material. Environ. Sci. Technol. 40 (6), 2045–2050. doi:10.1021/es0520924
Karami, H. (2013). Heavy metal removal from water by magnetite nanorods. Chem. Eng. J. 219, 209–216. doi:10.1016/j.cej.2013.01.022
Karami, N., Mohammadpour, A., Samaei, M. R., Amani, A. M., Dehghani, M., Varma, R. S., et al. (2024). Green synthesis of sustainable magnetic nanoparticles Fe3O4 and Fe3O4-chitosan derived from Prosopis farcta biomass extract and their performance in the sorption of lead(II). Int. J. Biol. Macromol. 254, 127663. doi:10.1016/j.ijbiomac.2023.127663
Karnwal, A., Dohroo, A., and Malik, TJBRI (2023). Unveiling the potential of bioinoculants and nanoparticles in sustainable agriculture for enhanced plant growth and food security. 2023(Article ID 6911851). doi:10.1155/2023/6911851
Karnwal, A., Kumar, G., Pant, G., Hossain, K., Ahmad, A., and Alshammari, M. B. (2023). Perspectives on usage of functional nanomaterials in antimicrobial therapy for antibiotic-resistant bacterial infections. ACS Omega 8 (15), 13492–13508. doi:10.1021/acsomega.3c00110
Kashi, E., Surip, S. N., Khadiran, T., Nawawi, W. I., De Luna, Y., Yaseen, Z. M., et al. (2024). High adsorptive performance of chitosan-microalgae-carbon-doped TiO2 (kronos)/salicylaldehyde for brilliant green dye adsorption: optimization and mechanistic approach. Int. J. Biol. Macromol. 259, 129147. doi:10.1016/j.ijbiomac.2023.129147
Kaur, S., and Roy, A. (2021). Bioremediation of heavy metals from wastewater using nanomaterials. Environ. Dev. Sustain. 23 (7), 9617–9640. doi:10.1007/s10668-020-01078-1
Kayanja, O., Abdel-Aty, A. A. R., Hassan, M. A., Hassanin, A., Ohashi, H., and Khalil, A. S. G. (2023). A review on TMDCs nanomaterials and their surface engineered polymeric membrane nanocomposites for water remediation and wastewater treatment. Surfaces Interfaces 43, 103578. doi:10.1016/j.surfin.2023.103578
Khorram Abadi, V., Habibi, D., Heydari, S., and Ariannezhad, M. (2023). The effective removal of Ni2+, Cd2+, and Pb2+ from aqueous solution by adenine-based nano-adsorbent. RSC Adv. 13 (9), 5970–5982. doi:10.1039/D2RA07230K
Kim, E. J., Lee, C. S., Chang, Y. Y., and Chang, Y. S. (2013). Hierarchically structured manganese oxide-coated magnetic nanocomposites for the efficient removal of heavy metal ions from aqueous systems. ACS Appl. Mater. Interfaces 5 (19), 9628–9634. doi:10.1021/am402615m
Kolluru, S. S., Agarwal, S., Sireesha, S., Sreedhar, I., and Kale, S. R. (2021). Heavy metal removal from wastewater using nanomaterials-process and engineering aspects. Process Saf. Environ. Prot. 150, 323–355. doi:10.1016/j.psep.2021.04.025
Kong, L., Liang, X., Zhan, Y., Jiao, S., Zhen, Y., Liu, M., et al. (2023). Efficient adsorption of selenium (Se(IV) and Se(VI)) from water using Acacia Senegal polysaccharide with multiple amine groups: synthesis and application. Int. J. Biol. Macromol. 253, 127458. doi:10.1016/j.ijbiomac.2023.127458
Kong, Q., Shi, X., Ma, W., Zhang, F., Yu, T., Zhao, F., et al. (2021). Strategies to improve the adsorption properties of graphene-based adsorbent towards heavy metal ions and their compound pollutants: a review. J. Hazard. Mater. 415, 125690. doi:10.1016/j.jhazmat.2021.125690
Kumar, A., and Kumar, V. (2023). A comprehensive review on application of lignocellulose derived nanomaterial in heavy metals removal from wastewater. Chem. Afr. 6 (1), 39–78. doi:10.1007/s42250-022-00367-8
Li, C., Yang, S., Bian, R., Tan, Y., Dong, X., Zhu, N., et al. (2021). Clinoptilolite mediated activation of peroxymonosulfate through spherical dispersion and oriented array of NiFe2O4: upgrading synergy and performance. J. Hazard. Mater. 407, 124736. doi:10.1016/j.jhazmat.2020.124736
Li, C., Yang, S., Bian, R., Tan, Y., Zhang, X., Zheng, S., et al. (2022). Efficient catalytic degradation of bisphenol A coordinated with peroxymonosulfate via anchoring monodispersed zero-valent iron on natural kaolinite. Chem. Eng. J. 448, 137746. doi:10.1016/j.cej.2022.137746
Li, N., Li, Z., Zhang, L., Shi, H., Li, J., Zhang, J., et al. (2020). One-step fabrication of bifunctional self-assembled oligopeptides anchored magnetic carbon nanoparticles and their application in copper (II) ions removal from aqueous solutions. J. Hazard. Mater. 382, 121113. doi:10.1016/j.jhazmat.2019.121113
Li, Y., He, J., Zhang, K., Liu, T., Hu, Y., Chen, X., et al. (2019). Super rapid removal of copper, cadmium and lead ions from water by NTA-silica gel. RSC Adv. 9 (1), 397–407. doi:10.1039/C8RA08638A
Li, Y. K., Yang, T., Chen, M. L., and Wang, J. H. (2021). Recent advances in nanomaterials for analysis of trace heavy metals. Crit. Rev. Anal. Chem. 51 (4), 353–372. doi:10.1080/10408347.2020.1736505
Liu, J., Yu, G., Jiang, P., Zhang, X., Meng, D., Chen, Z., et al. (2020). Interaction of Mn and Cd during their uptake in Celosia argentea differs between hydroponic and soil systems. Plant Soil 450 (1-2), 323–336. doi:10.1007/s11104-020-04514-3
Liu, K., Yao, Y., Xue, S., Zhang, M., Li, D., Xu, T., et al. (2023). Recent advances of tumor microenvironment-responsive nanomedicines-energized combined phototherapy of cancers. Pharmaceutics 15 (10), 2480. doi:10.3390/pharmaceutics15102480
Liu, L., Li, A., Cao, M., Ma, J., Tan, W., Suib, S. L., et al. (2022). Photoinduced self-organized precipitation in leachate for remediation of heavy metal contaminated soils. ACS ES T Eng. 2 (8), 1376–1385. doi:10.1021/acsestengg.1c00483
Liu, T., Wang, Z. L., Yan, X., and Zhang, B. (2014). Removal of mercury (II) and chromium (VI) from wastewater using a new and effective composite: pumice-supported nanoscale zero-valent iron. Chem. Eng. J. 245, 34–40. doi:10.1016/j.cej.2014.02.011
Liu, Y., Deng, H., Lu, Z., Zhong, X., and Zhu, Y. (2021). The study of MnO2 with different crystalline structures for U(VI) elimination from aqueous solution. J. Mol. Liq. 335, 116296. doi:10.1016/j.molliq.2021.116296
Liu, Y., Ma, J., Gao, J., Chen, X., Ouyang, X., Weng, L., et al. (2022). Stability and interaction of biochar and iron mineral nanoparticles: effect of pH, ionic strength, and dissolved organic matter. Biochar 4 (1), 47. doi:10.1007/s42773-022-00172-z
Lu, F., and Astruc, D. (2018). Nanomaterials for removal of toxic elements from water. Coord. Chem. Rev. 356, 147–164. doi:10.1016/j.ccr.2017.11.003
Ma, Z., Zhao, D., Chang, Y., Xing, S., Wu, Y., and Gao, Y. (2013). Synthesis of MnFe2O4@Mn-Co oxide core-shell nanoparticles and their excellent performance for heavy metal removal. Dalton Trans. 42 (39), 14261–14267. doi:10.1039/c3dt51310f
Madkour, M., Nazer, H. A. E., and Abdel-Monem, Y. K. (2021). “Use of chalcogenides-based nanomaterials for photocatalytic heavy metal reduction and ions removal,” in Chalcogenide-based nanomaterials as photocatalysts, 261–283.
Mahdavi Far, R., Van der Bruggen, B., Verliefde, A., and Cornelissen, E. (2022). A review of zeolite materials used in membranes for water purification: history, applications, challenges and future trends. J. Chem. Technol. Biotechnol. 97 (3), 575–596. doi:10.1002/jctb.6963
Mallick, P., Gadtya, A. S., Tripathy, D., Satpathy, S. K., and Moharana, S. (2023). “Carbon–metal hybrid nanomaterials for high technologies,” in Nanoparticles reinforced metal nanocomposites: mechanical performance and durability, 273–295.
Marotta, A., Luzzi, E., De Luna, M. S., Aprea, P., Ambrogi, V., and Filippone, G. (2021). Chitosan/zeolite composite aerogels for a fast and effective removal of both anionic and cationic dyes from water. Polymers 13 (11), 1691. doi:10.3390/polym13111691
Marwani, H. M., Ahmed, J., and Rahman, M. M. (2022). Development of a toxic lead ionic sensor using carboxyl-functionalized MWCNTs in real water sample analyses. Sensors 22 (22), 8976. doi:10.3390/s22228976
Mautner, A., Maples, H. A., Kobkeatthawin, T., Kokol, V., Karim, Z., Li, K., et al. (2016). Phosphorylated nanocellulose papers for copper adsorption from aqueous solutions. Int. J. Environ. Sci. Technol. 13 (8), 1861–1872. doi:10.1007/s13762-016-1026-z
Mo, Z., Tai, D., Zhang, H., and Shahab, A. (2022). A comprehensive review on the adsorption of heavy metals by zeolite imidazole framework (ZIF-8) based nanocomposite in water. Chem. Eng. J. 443, 136320. doi:10.1016/j.cej.2022.136320
Morales, H. M., Torreblanca, G., Mar, A., Alcoutlabi, M., Eubanks, T. M., Plata, E., et al. (2023). Investigation of the thermodynamics for the removal of as(III) and as(V) from water using synthesized ZnO nanoparticles and the effects of pH, temperature, and time. Appl. Sci. Switz. 13 (18), 10525. doi:10.3390/app131810525
Morillo Martín, D., Faccini, M., García, M. A., and Amantia, D. (2018). Highly efficient removal of heavy metal ions from polluted water using ion-selective polyacrylonitrile nanofibers. J. Environ. Chem. Eng. 6 (1), 236–245. doi:10.1016/j.jece.2017.11.073
Moukadiri, H., Noukrati, H., Ben Youcef, H., Iraola, I., Trabadelo, V., Oukarroum, A., et al. (2024). Impact and toxicity of heavy metals on human health and latest trends in removal process from aquatic media. Int. J. Environ. Sci. Technol. 21 (3), 3407–3444. doi:10.1007/s13762-023-05275-z
Musso, T. B., Parolo, M. E., and Pettinari, G. (2019). pH, ionic strength, and ion competition effect on Cu(II) and Ni(II) sorption by a Na-bentonite used as liner material. Pol. J. Environ. Stud. 28 (4), 2299–2309. doi:10.15244/pjoes/84922
Muthukumaran, P., Suresh Babu, P., Shyamalagowri, S., Aravind, J., Kamaraj, M., and Govarthanan, M. (2022). Polymeric biomolecules based nanomaterials: production strategies and pollutant mitigation as an emerging tool for environmental application. Chemosphere 307, 136008. doi:10.1016/j.chemosphere.2022.136008
Najafi, M., Yousefi, Y., and Rafati, A. A. (2012). Synthesis, characterization and adsorption studies of several heavy metal ions on amino-functionalized silica nano hollow sphere and silica gel. Sep. Purif. Technol. 85, 193–205. doi:10.1016/j.seppur.2011.10.011
Naseem, K., Begum, R., Wu, W., Usman, M., Irfan, A., Al-Sehemi, A. G., et al. (2019). Adsorptive removal of heavy metal ions using polystyrene-poly(N-isopropylmethacrylamide-acrylic acid) core/shell gel particles: adsorption isotherms and kinetic study. J. Mol. Liq. 277, 522–531. doi:10.1016/j.molliq.2018.12.054
Nassar, N. N. (2010). Rapid removal and recovery of Pb(II) from wastewater by magnetic nanoadsorbents. J. Hazard. Mater. 184 (1-3), 538–546. doi:10.1016/j.jhazmat.2010.08.069
Nithya, K., Sathish, A., Senthil Kumar, P., and Ramachandran, T. (2018). Fast kinetics and high adsorption capacity of green extract capped superparamagnetic iron oxide nanoparticles for the adsorption of Ni(II) ions. J. Industrial Eng. Chem. 59, 230–241. doi:10.1016/j.jiec.2017.10.028
Nithya, R., Thirunavukkarasu, A., Sathya, A. B., and Sivashankar, R. (2021). Magnetic materials and magnetic separation of dyes from aqueous solutions: a review. Environ. Chem. Lett. 19 (2), 1275–1294. doi:10.1007/s10311-020-01149-9
Peralta, M. E., Mártire, D. O., Moreno, M. S., Parolo, M. E., and Carlos, L. (2021). Versatile nanoadsorbents based on magnetic mesostructured silica nanoparticles with tailored surface properties for organic pollutants removal. J. Environ. Chem. Eng. 9 (1), 104841. doi:10.1016/j.jece.2020.104841
Qiu, Z., Chen, J., Dai, R., and Wang, Z. (2022). Modification of ultrafiltration membrane with antibacterial agent intercalated layered nanosheets: toward superior antibiofouling performance for water treatment. Water Res. 219, 118539. doi:10.1016/j.watres.2022.118539
Rad, L. R., Momeni, A., Ghazani, B. F., Irani, M., Mahmoudi, M., and Noghreh, B. (2014). Removal of Ni2+ and Cd2+ ions from aqueous solutions using electrospun PVA/zeolite nanofibrous adsorbent. Chem. Eng. J. 256, 119–127. doi:10.1016/j.cej.2014.06.066
Rangappa, H. S., Herath, I., Lin, C., and Ch, S. (2024). Industrial waste-based adsorbents as a new trend for removal of water-borne emerging contaminants. Environ. Pollut. 343, 123140. doi:10.1016/j.envpol.2023.123140
Rani, A., Kumar, N., and Kumar, M. (2023). Environmentally friendly green approaches and applications of nanoparticles. in Metal oxide-based carbon nanocomposites for environmental remediation and safety (Boca Raton: CRC Press), 21–63.
Raza, A., Rehman, R., Batool, M., Jahangir, M. M., Ghfar, A. A., Pradhan, S., et al. (2023). Adsorptive elimination of rhodamine B dye by synthetic clay-based hetero-metallic oxide nanocomposite KAB-ben for rapid wastewater treatment. Water, Air, Soil Pollut. 234 (10), 654. doi:10.1007/s11270-023-06589-x
Razman Shah, N. B. B., Sazali, R. A. B., Sorbie, K. S., and Azizi, A. (2023). Nanomaterials for scaling prevention in alkaline–surfactant–polymer flooding: a review. Appl. Nanosci. Switz. 13 (6), 3945–3974. doi:10.1007/s13204-022-02652-x
Razzaz, A., Ghorban, S., Hosayni, L., Irani, M., and Aliabadi, M. (2016). Chitosan nanofibers functionalized by TiO2 nanoparticles for the removal of heavy metal ions. J. Taiwan Inst. Chem. Eng. 58, 333–343. doi:10.1016/j.jtice.2015.06.003
Recillas, S., Colón, J., Casals, E., González, E., Puntes, V., Sánchez, A., et al. (2010). Chromium VI adsorption on cerium oxide nanoparticles and morphology changes during the process. J. Hazard. Mater. 184 (1-3), 425–431. doi:10.1016/j.jhazmat.2010.08.052
Ren, T., He, P., Niu, W., Wu, Y., Ai, L., and Gou, X. (2013). Synthesis of α-Fe2O3 nanofibers for applications in removal and recovery of Cr(VI) from wastewater. Environ. Sci. Pollut. Res. 20 (1), 155–162. doi:10.1007/s11356-012-0842-z
Roostaee, M., Sheikhshoaie, I., and karimi maleh, H. (2022). Low-temperature synthesis of hetero-structures of magnetically separable iron oxide@Au-rGO nanocomposite for efficient degradation of organic dye under visible light irradiation. Environ. Res. 205, 112510. doi:10.1016/j.envres.2021.112510
Rybarczyk, A., Szada, D., Badzińska, W., Degórska, O., Jesionowski, T., and Zdarta, J. (2024). Nanobiocatalysts as efficient tools for wastewater pollutants remediation. Adv. Chem. Pollut. Environ. Manag. Prot. 10, 57–82. doi:10.1016/bs.apmp.2023.06.014
Saad, A. H. A., Azzam, A. M., El-Wakeel, S. T., Mostafa, B. B., and Abd El-latif, M. B. (2018). Removal of toxic metal ions from wastewater using ZnO@Chitosan core-shell nanocomposite. Environ. Nanotechnol. Monit. Manag. 9, 67–75. doi:10.1016/j.enmm.2017.12.004
Sall, M. L., Diaw, A. K. D., Gningue-Sall, D., Efremova Aaron, S., and Aaron, J. J. (2020). Toxic heavy metals: impact on the environment and human health, and treatment with conducting organic polymers, a review. Environ. Sci. Pollut. Res. 27 (24), 29927–29942. doi:10.1007/s11356-020-09354-3
Sankareswaran, M., Periakaruppan, R., Sasivarnam, M., Danaraj, J., Dhanasekaran, S., and Abomughaid, M. M. (2022). Bio-fabrication of bio-inspired silica nanomaterials from bryophyllum pinnatum leaf for agricultural applications. Appl. Biochem. Biotechnol. 194 (9), 4266–4277. doi:10.1007/s12010-022-03996-4
Shahamati Fard, F., Kazemi, H., Mighri, F., and Rodrigue, D. (2021). “Hybrid nanocomposites based on cellulose nanocrystals/nanofibrils and carbon nanotubes: from preparation to applications,” in Cellulose nanocrystal/nanoparticles hybrid nanocomposites: from preparation to applications, 65–98.
Sharma, S. N., Kadri, U., and Naha, N. (2023). Impact of heavy metal-based nanomaterials on environment and health: small things having big impacts. in Implications of nanoecotoxicology on environmental sustainability (Pennsylvania: IGI Global), 224–277.
Shen, H., Chen, J., Dai, H., Wang, L., Hu, M., and Xia, Q. (2013). New insights into the sorption and detoxification of chromium(VI) by tetraethylenepentamine functionalized nanosized magnetic polymer adsorbents: mechanism and pH effect. Industrial Eng. Chem. Res. 52 (36), 12723–12732. doi:10.1021/ie4010805
Shen, H., Pan, S., Zhang, Y., Huang, X., and Gong, H. (2012). A new insight on the adsorption mechanism of amino-functionalized nano-Fe 3O 4 magnetic polymers in Cu(II), Cr(VI) co-existing water system. Chem. Eng. J. 183, 180–191. doi:10.1016/j.cej.2011.12.055
Shi, T., Xie, Z., Mo, X., Shi, W., Qiu, H., Lan, G., et al. (2023). Adsorption behaviors of heavy metal ions by different hydrazone-modified sodium alginate in aqueous medium: experimental and DFT studies. Colloids Surfaces A Physicochem. Eng. Aspects 659, 130754. doi:10.1016/j.colsurfa.2022.130754
Sikder, M. T., Mihara, Y., Islam, M. S., Saito, T., Tanaka, S., and Kurasaki, M. (2014). Preparation and characterization of chitosan–caboxymethyl-β-cyclodextrin entrapped nanozero-valent iron composite for Cu (II) and Cr (IV) removal from wastewater. Chem. Eng. J. 236, 378–387. doi:10.1016/j.cej.2013.09.093
Sohail, I., Bhatti, I. A., Ashar, A., Sarim, F. M., Mohsin, M., Naveed, R., et al. (2020). Polyamidoamine (PAMAM) dendrimers synthesis, characterization and adsorptive removal of nickel ions from aqueous solution. J. Mater. Res. Technol. 9 (1), 498–506. doi:10.1016/j.jmrt.2019.10.079
Sohail, M. I., Ayub, M. A., Zia Ur Rehman, M., Azhar, M., Farooqi, Z. U. R., Siddiqui, A., et al. (2021). “Sufficiency and toxicity limits of metallic oxide nanoparticles in the biosphere,” in Nanomaterials: synthesis, characterization, hazards and safety, 145–221.
Solanki, S., Sinha, S., Tyagi, S., and Singh, R. (2024). Nanomaterials for efficient removal of heavy metals. Adv. Chem. Pollut. Environ. Manag. Prot. 10, 117–136. doi:10.1016/bs.apmp.2023.08.008
Sounthararajah, D. P., Loganathan, P., Kandasamy, J., and Vigneswaran, S. (2015). Adsorptive removal of heavy metals from water using sodium titanate nanofibres loaded onto GAC in fixed-bed columns. J. Hazard. Mater. 287, 306–316. doi:10.1016/j.jhazmat.2015.01.067
Sprynskyy, M., Buszewski, B., Terzyk, A. P., and Namieśnik, J. (2006). Study of the selection mechanism of heavy metal (Pb2+, Cu2+, Ni2+, and Cd2+) adsorption on clinoptilolite. J. Colloid Interface Sci. 304 (1), 21–28. doi:10.1016/j.jcis.2006.07.068
Suazo-Hernández, J., Sepúlveda, P., Cáceres-Jensen, L., Castro-Rojas, J., Poblete-Grant, P., Bolan, N., et al. (2023). nZVI-based nanomaterials used for phosphate removal from aquatic systems. Nanomaterials 13 (3), 399. doi:10.3390/nano13030399
Suhasini, R., and Thiagarajan, V. (2021). “Magnetic nanomaterials for wastewater remediation,” in Nanomaterials for water treatment and remediation, 279–308.
Sukmana, H., Bellahsen, N., Pantoja, F., and Hodur, C. (2021). Adsorption and coagulation in wastewater treatment - review. Prog. Agric. Eng. Sci. 17 (1), 49–68. doi:10.1556/446.2021.00029
Sun, W., Jiang, B., Wang, F., and Xu, N. (2015). Effect of carbon nanotubes on Cd(II) adsorption by sediments. Chem. Eng. J. 264, 645–653. doi:10.1016/j.cej.2014.11.137
Suresh, R., and Rajendran, S. (2022). Surface modified carbon nanotubes for organic pollutants' removal. ACS Symp. Ser. 1425, 249–273. doi:10.1021/bk-2022-1425.ch011
Surti, P., Kailasa, S. K., and Mungray, A. K. (2023). Enhancement of electrode properties using carbon dots functionalized magnetite nanoparticles for azo dye decolorization in microbial fuel cell. Chemosphere 313, 137601. doi:10.1016/j.chemosphere.2022.137601
Tao, Z., Zhou, Q., Zheng, T., Mo, F., and Ouyang, S. (2023). Iron oxide nanoparticles in the soil environment: adsorption, transformation, and environmental risk. J. Hazard. Mater. 459, 132107. doi:10.1016/j.jhazmat.2023.132107
Targuma, S., Njobeh, P. B., and Ndungu, P. G. (2021). Current applications of magnetic nanomaterials for extraction of mycotoxins, pesticides, and pharmaceuticals in food commodities. Molecules 26 (14), 4284. doi:10.3390/molecules26144284
Thakur, A., and Kumar, A. (2023). Magnetic composites of biochar and its applications. In materials horizons: from Nature to nanomaterials. Part F1442, 185–209. doi:10.1007/978-981-99-5239-7_9
Trofymchuk, I. M., Roik, N. V., and Belyakova, L. O. (2023). Cyclodextin-silica nanomaterials for aromatics removal from aqueous media: kinetic and equilibrium studies. Appl. Nanosci. Switz. 13 (12), 7425–7443. doi:10.1007/s13204-023-02914-2
Trojanowicz, M. (2006). Analytical applications of carbon nanotubes: a review. TrAC - Trends Anal. Chem. 25 (5), 480–489. doi:10.1016/j.trac.2005.11.008
Tu, Y. J., Chan, T. S., Tu, H. W., Wang, S. L., You, C. F., and Chang, C. K. (2016). Rapid and efficient removal/recovery of molybdenum onto ZnFe2O4 nanoparticles. Chemosphere 148, 452–458. doi:10.1016/j.chemosphere.2016.01.054
Tu, Y. J., You, C. F., and Chang, C. K. (2013). Conversion of waste Mn–Zn dry battery as efficient nano-adsorbents for hazardous metals removal. J. Hazard. Mater. 258, 102–108. doi:10.1016/j.jhazmat.2013.04.029
Tu, Y. J., You, C. F., Chen, M. H., and Duan, Y. P. (2017). Efficient removal/recovery of Pb onto environmentally friendly fabricated copper ferrite nanoparticles. J. Taiwan Inst. Chem. Eng. 71, 197–205. doi:10.1016/j.jtice.2016.12.006
Ukhurebor, K. E., Aigbe, U. O., Onyancha, R. B., Balogun, V. A., Anani, O. A., Adama, K. K., et al. (2023). “An overview of magnetic nanomaterials,” in Engineering materials (Springer Science and Business Media Deutschland GmbH), 1–20.
Umeh, C. T., Akinyele, A. B., Okoye, N. H., Emmanuel, S. S., Iwuozor, K. O., Oyekunle, I. P., et al. (2023). Recent approach in the application of nanoadsorbents for malachite green (MG) dye uptake from contaminated water: a critical review. Environ. Nanotechnol. Monit. Manag. 20, 100891. doi:10.1016/j.enmm.2023.100891
Umejuru, E. C., Mashifana, T., Kandjou, V., Amani-Beni, M., Sadeghifar, H., Fayazi, M., et al. (2023). Application of zeolite based nanocomposites for wastewater remediation: evaluating newer and environmentally benign approaches. Environ. Res. 231, 116073. doi:10.1016/j.envres.2023.116073
Vamvakidis, K., Kostitsi, T. M., Makridis, A., and Dendrinou-Samara, C. (2020). Diverse surface chemistry of cobalt ferrite nanoparticles to optimize copper (II) removal from aqueous media. Materials 13 (7), 1537. doi:10.3390/ma13071537
Vinoth, S., and Wang, S. F. (2023). Construction of functionalized carbon nanotube@metal oxide nanocomposite for high-performance electrochemical measurement of antipyretic drug in water samples. Environ. Sci. Pollut. Res. doi:10.1007/s11356-023-26043-z
Vishnu, D., Dhandapani, B., Kannappan Panchamoorthy, G., Vo, D. V. N., and Ramakrishnan, S. R. (2021). Comparison of surface-engineered superparamagnetic nanosorbents with low-cost adsorbents of cellulose, zeolites and biochar for the removal of organic and inorganic pollutants: a review. Environ. Chem. Lett. 19 (4), 3181–3208. doi:10.1007/s10311-021-01201-2
Wang, H. Q., Yang, G. F., Li, Q. Y., Zhong, X. X., Wang, F. P., Li, Z. S., et al. (2011). Porous nano-MnO2: large scale synthesis via a facile quick-redox procedure and application in a supercapacitor. New J. Chem. 35 (2), 469–475. doi:10.1039/c0nj00712a
Wang, J., Niu, K., Hou, J., Zhuang, Z., Zhu, J., Jing, X., et al. (2024). Advanced integration of glutathione-functionalized optical fiber SPR sensor for ultra-sensitive detection of lead ions. Materials 17 (1), 98. doi:10.3390/ma17010098
Wang, P., Shen, T., Li, X., Tang, Y., and Li, Y. (2020). Magnetic mesoporous calcium carbonate-based nanocomposites for the removal of toxic Pb(II) and Cd(II) ions from water. ACS Appl. Nano Mater. 3 (2), 1272–1281. doi:10.1021/acsanm.9b02036
Wang, W. D., Cui, Y. X., Zhang, L. K., Li, Y. M., Sun, P., and Han, J. H. (2021). Synthesis of a novel ZnFe2O4/porous biochar magnetic composite for Th(IV) adsorption in aqueous solutions. Int. J. Environ. Sci. Technol. 18 (9), 2733–2746. doi:10.1007/s13762-020-03023-1
Wang, X., Cai, W., Lin, Y., Wang, G., and Liang, C. (2010). Mass production of micro/nanostructured porous ZnO plates and their strong structurally enhanced and selective adsorption performance for environmental remediation. J. Mater. Chem. 20 (39), 8582–8590. doi:10.1039/c0jm01024c
Wang, X., Liu, X., Xiao, C., Zhao, H., Zhang, M., Zheng, N., et al. (2020). Triethylenetetramine-modified hollow Fe3O4/SiO2/chitosan magnetic nanocomposites for removal of Cr(VI) ions with high adsorption capacity and rapid rate. Microporous Mesoporous Mater. 297, 110041. doi:10.1016/j.micromeso.2020.110041
Wang, Y., and Sun, H. (2021). Polymeric nanomaterials for efficient delivery of antimicrobial agents. Pharmaceutics 13 (12), 2108. doi:10.3390/pharmaceutics13122108
Wu, Y., Pang, H., Liu, Y., Wang, X., Yu, S., Fu, D., et al. (2019). Environmental remediation of heavy metal ions by novel-nanomaterials: a review. Environ. Pollut. 246, 608–620. doi:10.1016/j.envpol.2018.12.076
Xia, J., Twinney, J., Marthi, R., and Ghahreman, A. (2023). Ultra-efficient and selective recovery of Au(III) using magnetic Fe3S4/Fe7S8. Sep. Purif. Technol. 306, 122611. doi:10.1016/j.seppur.2022.122611
Xiao, Z., Zhang, H., Xu, Y., Yuan, M., Jing, X., Huang, J., et al. (2017). Ultra-efficient removal of chromium from aqueous medium by biogenic iron based nanoparticles. Sep. Purif. Technol. 174, 466–473. doi:10.1016/j.seppur.2016.10.047
Xu, P., Zeng, G. M., Huang, D. L., Yan, M., Chen, M., Lai, C., et al. (2017). Fabrication of reduced glutathione functionalized iron oxide nanoparticles for magnetic removal of Pb(II) from wastewater. J. Taiwan Inst. Chem. Eng. 71, 165–173. doi:10.1016/j.jtice.2016.11.031
Xu, Y., and Zhao, D. (2007). Reductive immobilization of chromate in water and soil using stabilized iron nanoparticles. Water Res. 41 (10), 2101–2108. doi:10.1016/j.watres.2007.02.037
Xu, Z., Zhang, Z., and Wang, X. (2023). Ecotoxicological effects of soil lithium on earthworm Eisenia fetida: lethality, bioaccumulation, biomarker responses, and histopathological changes. Environ. Pollut. 330, 121748. doi:10.1016/j.envpol.2023.121748
Yamini, V., and Devi Rajeswari, V. (2023). Effective bio-mediated nanoparticles for bioremediation of toxic metal ions from wastewater – a review. J. Environ. Nanotechnol. 12 (2), 12–33. doi:10.13074/jent.2023.06.232467
Yamini, V., Shanmugam, V., Rameshpathy, M., Venkatraman, G., Ramanathan, G., Al Garalleh, H., et al. (2023). Environmental effects and interaction of nanoparticles on beneficial soil and aquatic microorganisms. Environ. Res. 236, 116776. doi:10.1016/j.envres.2023.116776
Yang, F., and Yang, P. (2022). Biopolymer-based membrane adsorber for removing contaminants from aqueous solution: progress and prospects. Macromol. Rapid Commun. 43 (3), e2100669. doi:10.1002/marc.202100669
Yang, G., Kong, H., Chen, Y., Liu, B., Zhu, D., Guo, L., et al. (2022). Recent advances in the hybridization of cellulose and carbon nanomaterials: interactions, structural design, functional tailoring, and applications. Carbohydr. Polym. 279, 118947. doi:10.1016/j.carbpol.2021.118947
Yang, J., Hou, B., Wang, J., Tian, B., Bi, J., Wang, N., et al. (2019). Nanomaterials for the removal of heavy metals from wastewater. Nanomaterials 9 (3), 424. doi:10.3390/nano9030424
Yang, Z., Chen, X., Li, S., Ma, W., Li, Y., He, Z., et al. (2020). Effective removal of Cr(VI) from aqueous solution based on APTES modified nanoporous silicon prepared from kerf loss silicon waste. Environ. Sci. Pollut. Res. 27 (10), 10899–10909. doi:10.1007/s11356-019-07427-6
Yaseen, M., Farooq, M. U., Ahmad, W., and Subhan, F. (2021). Fabrication of rGO-CuO and/or Ag2O nanoparticles incorporated polyvinyl acetate based mixed matrix membranes for the removal of Cr6+from anti-corrosive paint industrial wastewater. J. Environ. Chem. Eng. 9 (2), 105151. doi:10.1016/j.jece.2021.105151
Yu, G., Wang, X., Liu, J., Jiang, P., You, S., Ding, N., et al. (2021). Applications of nanomaterials for heavy metal removal from water and soil: a review. Sustainability 13 (2), 713. doi:10.3390/su13020713
Yu, L., Wang, Z., and Wang, D. G. (2023). Factors affecting the toxicity and oxidative stress of layered double hydroxide-based nanomaterials in freshwater algae. Environ. Sci. Pollut. Res. 30 (22), 63109–63120. doi:10.1007/s11356-023-26522-3
Yuan, X., An, N., Zhu, Z., Sun, H., Zheng, J., Jia, M., et al. (2018). Hierarchically porous nitrogen-doped carbon materials as efficient adsorbents for removal of heavy metal ions. Process Saf. Environ. Prot. 119, 320–329. doi:10.1016/j.psep.2018.08.012
Yurekli, Y. (2016). Removal of heavy metals in wastewater by using zeolite nano-particles impregnated polysulfone membranes. J. Hazard. Mater. 309, 53–64. doi:10.1016/j.jhazmat.2016.01.064
Yusuf, M. (2021). Cellulose-based nanomaterials for water pollutant remediation: review. Handb. Nanomater. Nanocomposites Energy Environ. Appl. 1, 213–228. doi:10.1007/978-3-030-36268-3_17
Zaman, H., Shah, AUHA, Ali, N., Zhou, C., Khan, A., Ali, F., et al. (2022). Magnetically recoverable poly (methyl methacrylate-acrylic acid)/iron oxide magnetic composites nanomaterials with hydrophilic wettability for efficient oil-water separation. J. Environ. Manag. 319, 115690. doi:10.1016/j.jenvman.2022.115690
Zambare, R. S., and Nemade, P. R. (2021). “Polymer nanocomposite membranes for wastewater treatment,” in Handbook of nanomaterials for wastewater treatment: fundamentals and scale up issues, 605–672.
Zamora-Ledezma, C., Negrete-Bolagay, D., Figueroa, F., Zamora-Ledezma, E., Ni, M., Alexis, F., et al. (2021). Heavy metal water pollution: a fresh look about hazards, novel and conventional remediation methods. Environ. Technol. Innovation 22, 101504. doi:10.1016/j.eti.2021.101504
Zand, A. D., Mikaeili Tabrizi, A., and Vaezi Heir, A. (2020). Application of titanium dioxide nanoparticles to promote phytoremediation of Cd-polluted soil: contribution of PGPR inoculation. Bioremediation J. 24 (2-3), 171–189. doi:10.1080/10889868.2020.1799929
Zeng, T., Yu, Y., Li, Z., Zuo, J., Kuai, Z., Jin, Y., et al. (2019). 3D MnO2 nanotubes@reduced graphene oxide hydrogel as reusable adsorbent for the removal of heavy metal ions. Mater. Chem. Phys. 231, 105–108. doi:10.1016/j.matchemphys.2019.04.019
Zhan, W. Y., Liu, G. S., and Zhong, H. (2021). Stability of nC60 nanoparticles in the presence of kaolinite and montmorillonite. Zhongguo Huanjing Kexue/China Environ. Sci. 41 (3), 1366–1377. doi:10.0000/j.zghjkx.1000-6923.20214117452
Zhang, D., Tang, H., Zhao, B., Liu, L., Pang, H., Wang, X., et al. (2023). Immobilization of uranium by S-NZVI and UiO-66-NO2 composite through combined adsorption and reduction. J. Clean. Prod. 390, 136149. doi:10.1016/j.jclepro.2023.136149
Zhang, H., Dong, X., and Yang, H. (2023). Montmorillonite-mediated electron distribution of zirconium phosphate for accelerating remediation of cadmium-contaminated water and soil. Appl. Clay Sci. 236, 106883. doi:10.1016/j.clay.2023.106883
Zhang, H., Tan, X., Qiu, T., Zhou, L., Li, R., and Deng, Z. (2019). A novel and biocompatible Fe3O4 loaded chitosan polyelectrolyte nanoparticles for the removal of Cd2+ ion. Int. J. Biol. Macromol. 141, 1165–1174. doi:10.1016/j.ijbiomac.2019.09.040
Zhang, J., Li, T., Li, X., Liu, Y., Li, N., Wang, Y., et al. (2021). A key role of inner-cation-π interaction in adsorption of Pb(II) on carbon nanotubes: experimental and DFT studies. J. Hazard. Mater. 412, 125187. doi:10.1016/j.jhazmat.2021.125187
Zhang, K., Song, X., Liu, M., Chen, M., Li, J., and Han, J. (2023). Review on the use of magnetic nanoparticles in the detection of environmental pollutants. WaterSwitzerl. 15 (17), 3077. doi:10.3390/w15173077
Zhang, Y., Chen, Y., Kang, Z. W., Gao, X., Zeng, X., Liu, M., et al. (2021). Waste eggshell membrane-assisted synthesis of magnetic CuFe2O4 nanomaterials with multifunctional properties (adsorptive, catalytic, antibacterial) for water remediation. Colloids Surfaces A Physicochem. Eng. Aspects 612, 125874. doi:10.1016/j.colsurfa.2020.125874
Zhang, Y., Xu, H., Ma, S., Chen, M., Tian, L., Gou, G., et al. (2022). A research on the removal characteristics of naphthol Green B from wastewater using nanomaterials nZVI/CS/APT. Phys. Chem. Liq. 60 (2), 219–232. doi:10.1080/00319104.2021.1939344
Zhao, B., Wu, D., Hou, H., Liu, L., Wang, X., Yao, W., et al. (2024). Functionalized zeolite imidazolium framework materials for uranium removal performance and mechanism research. J. Taiwan Inst. Chem. Eng. 156, 105387. doi:10.1016/j.jtice.2024.105387
Zhao, C., Zhou, J., Yan, Y., Yang, L., Xing, G., Li, H., et al. (2021). Application of coagulation/flocculation in oily wastewater treatment: a review. Sci. Total Environ. 765, 142795. doi:10.1016/j.scitotenv.2020.142795
Zhou, Y., Li, Y., Liu, D., Liu, D., Xu, L., and Liu, C. (2021). Adsorption optimization of uranium(VI) onto polydopamine and sodium titanate co-functionalized MWCNTs using response surface methodology and a modeling approach. Colloids Surfaces A Physicochem. Eng. Aspects 627, 127145. doi:10.1016/j.colsurfa.2021.127145
Zhou, Y., Xue, C., Gan, L., Owens, G., and Chen, Z. (2023). Simultaneous removal of triclosan and Cd(Ⅱ) by bio-reduced graphene oxide and its mechanism. Chemosphere 311, 137021. doi:10.1016/j.chemosphere.2022.137021
Keywords: adsorption, engineered nanomaterials, functionalization, heavy metal removal, sustainability, water treatment
Citation: Karnwal A and Malik T (2024) Nano-revolution in heavy metal removal: engineered nanomaterials for cleaner water. Front. Environ. Sci. 12:1393694. doi: 10.3389/fenvs.2024.1393694
Received: 29 February 2024; Accepted: 15 April 2024;
Published: 06 May 2024.
Edited by:
Ahmed El Nemr, National Institute of Oceanography and Fisheries (NIOF), EgyptReviewed by:
Manoj Chandra Garg, Amity University, IndiaHeidi Lynn Richards, University of the Witwatersrand, South Africa
Copyright © 2024 Karnwal and Malik. This is an open-access article distributed under the terms of the Creative Commons Attribution License (CC BY). The use, distribution or reproduction in other forums is permitted, provided the original author(s) and the copyright owner(s) are credited and that the original publication in this journal is cited, in accordance with accepted academic practice. No use, distribution or reproduction is permitted which does not comply with these terms.
*Correspondence: Arun Karnwal, YXJ1bmthcm53YWxAZ21haWwuY29t; Tabarak Malik, bWFsaWtpdHJjQGdtYWlsLmNvbQ==