- Department of Anatomy and Comparative Pathology and Toxicology, UIC Zoonosis y Enfermedades Emergentes ENZOEM, Faculty of Veterinary Medicine, Campus de Rabanales, University of Córdoba, Córdoba, Spain
Rare earth elements are a group of chemicals widely utilized in industry, leading to ecosystem contamination. Due to the lack of regulatory measures, there exists a considerable gap in knowledge concerning their potential to induce adverse effects in organisms. Zebrafish serve as a species utilized in evaluating the risk of potentially toxic substances in the aquatic ecosystem. Our objective was to evaluate the acute toxicity in zebrafish embryos induced by two of the most used rare earth elements, neodymium, categorized as a light element, and yttrium, as a heavy element. We followed OECD guideline n°. 236 “Fish Embryo Acute Toxicity Test”, exposing embryos to concentrations of 6.4, 16, 40, 100, and 250 mg/L, and calculated the LC50. Our study revealed comparable LC50 values for both elements (55.58 mg/L for Nd, and 45.61 mg/L for Y), suggesting a probable similarity in toxicity. These concentrations values have previously been identified in various contaminated regions globally, presenting a cause for concern.
1 Introduction
Rare earth elements (REEs) are a group of transition metals that include scandium (Sc) (Z = 21) and yttrium (Y) (Z = 39), as well as the lanthanides family, which comprises from lanthanum (La) (Z = 57) to lutetium (Lu) (Z = 71) (USEPA, 2012; Wu et al., 2022). All the above have similar physical and chemical properties and are found in their natural state on the earth’s crust, except promethium (Pm), which does not have stable isotopes (Elkina and Kurushkin, 2020). Due to their exceptional magnetic, catalytic, optical, and electronic properties, rare earths have acquired a relevant role in innovatory and avant-garde technologies in fields like automotion, electronics, or image diagnosis, among others (Lu et al., 2017; Okeme et al., 2022). The nomenclature “rare earth” originates from the fact that, when discovered, they were found in the form of apparently scanty complex oxides. However, though they are reasonably abundant, they have a disperse distribution in nature, and they need complicated separation processes for their extraction (Dinh et al., 2022).
Currently, there is no universal classification for REEs, although they are usually classified into light and heavy REEs, based on their atomic number and electronic configuration. The light REEs are more numerous in the environment than the heavy ones, and, among the former, La, cerium (Ce), praseodymium (Pr), neodymium (Nd), promethium (Pm), and samarium (Sm) can be found. The heavy REEs include europium (Eu), gadolinium (Gd), terbium (Tb), dysprosium (Dy), holmium (Ho), erbium (Er), thulium (Th), yterbium (Yb) and yttrium (Y) (Huang et al., 2020).
In the last 2 decades, the demand for REEs has considerably increased. Their extensive use in different areas has permitted their release into the environment by different sources, such as mining, extraction and refining, sewage from industrial processes, or electronic residues deposited in landfills (Ayedun et al., 2016). This has enabled the pollution of different ecosystem and their monitoring in different parts of the world (Tepe et al., 2014; Hatje et al., 2016; Liu et al., 2019; Bakhshalizadeh et al., 2023). However, their detection in various environmental matrices has rendered them potential emerging pollutants, possibly triggering alterations in humans and ecosystem fauna (Rogowska et al., 2018; Malhotra et al., 2020), as they are not regulated, or monitored in public health programs. Besides that, the techniques for their detection require a great sensitivity, which is still in a developmental stage (Kulaksiz and Bau, 2013; Gwenzi et al., 2018; Henríquez-Hernández et al., 2023).
From an environmental point of view, it is of interest to learn about the REEs most used, which are practically limited to the following six elements, in order of importance: Ce (39.5%), La (26.4%), Nd (19.9%), Y (7.1%), Pr (4.1%), and Gd (1.1%), whereas the rest only make up 1.9% of the total volume employed (Schreiber et al., 2021; U.S Geological Survey, 2023). Regarding Nd, it is widely used in different areas including wind turbines, hybrid vehicles, in image diagnosis techniques, in electronic devices like mobile telephones, and in military defence. It has also been detected in fertilizers (Ramos et al., 2016). With respect to Y, this has applications in optics, it is used in low energy-cost light bulbs, or in television screens, and it is even employed in radiotherapy treatment for non-Hodgkin lymphoma cases (Gwenzi et al., 2018; Malhotra et al., 2020; Klingelhöfer et al., 2022). Despite being two of the REEs most used, there are not many studies evaluating their toxicity, because most of the few articles assessing the toxicological aspects of REEs have been focussed on Ce, La and Ga (Pagano et al., 2015a; Pagano et al., 2015b).
Regarding the adverse effects that can be caused on the aquatic ecosystem, some current studies have been related to their absorption and accumulation in organic tissues, like, for example, in fish, shellfish or molluscs (Perrat et al., 2017; Trifuoggi et al., 2017; Bakhshalizadeh et al., 2023). However, few are concentrated on evaluating their toxicity (Pagano et al., 2016; Kang et al., 2022a; Kang et al., 2022b; Lachaux et al., 2022). One of the fish species commonly used to evaluate the toxicity of potentially harmful elements is the zebrafish (Danio rerio). The employment of this model has become extended over the last 2 decades in diverse biomedical research fields, using it widely as a model for the assessment of the environmental risk from pollutants, and for estimating chemical substance toxicity (Lora et al., 2016; Katoch and Patial, 2020; Risalde et al., 2021). The embryos of this species are transparent, and they are fully developed in a period of 96 h at 26°C. This makes them ideal individuals for ecotoxicity studies (Fernandes and Lovely, 2021) as their developmental stages are characterized in detail, therefore enabling the detection of any embryonic alteration from their exposure to any toxic substance (Kimmel et al., 1995; OECD, 2013). Also, the use of individuals in the first embryonic stages is a replacement method in relation to the 3Rs concept in animal experimentation (Rosa et al., 2022). In this species, several authors have evaluated the toxicity of different REEs, observing changes both in their growth and locomotion (Zhao et al., 2020; Kang et al., 2022a; Kang et al., 2022b), although the studies assessing the toxicity of Nd and Y in zebrafish are limited.
In this context, the aim of our study was to evaluate and compare the toxicity of different concentrations of two REEs, one categorized as being light, Nd, and the other heavy, Y. This was done through an acute toxicity test in embryos, determining their LC50 and finding out the possible adverse effects from both elements on the embryonic development of the zebrafish.
2 Materials and methods
2.1 Chemicals
The two rare earth elements to be evaluated, Nd (neodymium chloride (III) hexahydrate (NdCl3·6H2O), CAS 13477-89-9, Sigma 289183), and Y (yttrium chloride (III) hexahydrate (YCl3·6H2O), CAS 10025-94-2, Sigma 464317) were obtained from Sigma Aldrich Co. (St. Louis, MO, United States). The purity of both compounds was over 99%, and no solvent was required for their dissolution in the embryonic medium. To prepare the dissolutions, for each element a 500 mg/L mother solution, diluted until reaching the desired concentrations (6.4, 16, 40, 100, 250 mg/L) was employed. To select the concentrations, a factor of 2.5 was used and the selection was made following: 1) concentrations proposed by the OECD guideline n° 236 to offer validity and reliability to the results that demonstrated the appearance of adverse effects in zebrafish embryos (OECD, 2013), 2) the development of harmful effects observed in different studies in the aquatic ecosystem, among them in zebrafish (Dubé et al., 2019; Zhao et al., 2020; Kang et al., 2022a; 2022b; Lin et al., 2022); 3) the range of environmentally relevant concentrations detected in water in different parts of the planet (Kulaksiz and Bau, 2013; Ayedun et al., 2016; Liu et al., 2019; Zhao et al., 2020).
2.2 Animals
The embryos used in the study were obtained from adult wild type AB zebrafish, provided by the Córdoba University Animal Experimentation Service (SAEX). These animals were kept in a water recirculation system with filtration, that could measure the water quality, germicidal ultraviolet irradiation, and light and temperature control parameters. The water conditions were as follows: pH 7–8; conductivity of between 300 and 500 μS/cm, temperature of 26°C ± 1°C; hardness of 50–250 mg CaCO3; ammonia (NH3/NH4+) < 0.1 mg/L, nitrite (NO2–) < 0.3 mg/L and nitrate (NO3–) < 25 mg/L. The animals were fed three times a day with a combination of dry food (Tropical Supervit Premium®) and frozen Artemia spp. The photoperiod employed was of 14 h of light and 10 of darkness.
The day before the test, the fish, with a ratio of 2:1 (male:female), were transferred to 5 reproduction aquariums, with a net spread over the bottom and separated as per sex. The following day, when the light was automatically turned on, the separation was withdrawn so that the spawning process could take place. After spawning, the embryos were collected and the reproduction aquarium contents were poured onto a sieve and from there into a 3.5 cm Petri dishes containing a medium of E3 (5.0 mM NaCl, 0.17 mM KCl, 0.33 mM CaCl2 and 0.33 mM MgSO4) and in which the embryos were collected through rinsing them in the same E3 medium, thus eliminating possible organic matter residues. The embryos with a normal cleavage and no apparent modifications, as is shown in Figure 1, were selected by viewing them under a LanOptics® triocular stereomicroscope, at 30x, and exposed to the study concentrations at between the beginning of the blastodisc division and the formation of the first 16 cells, at no longer than 90 min post fertilization. With respect to the fertilization rate, in the five reproduction aquariums used, a percentage of close to 90% was achieved, that was therefore higher than the 70% established in the guideline n° 236 as a minimum fertility value (OECD, 2013). All the experimental procedures employed in this study were approved by the Animal Experimentation Ethics Committee, which is part of the Bioethical and Biosecurity Committee at Córdoba University (Spain).
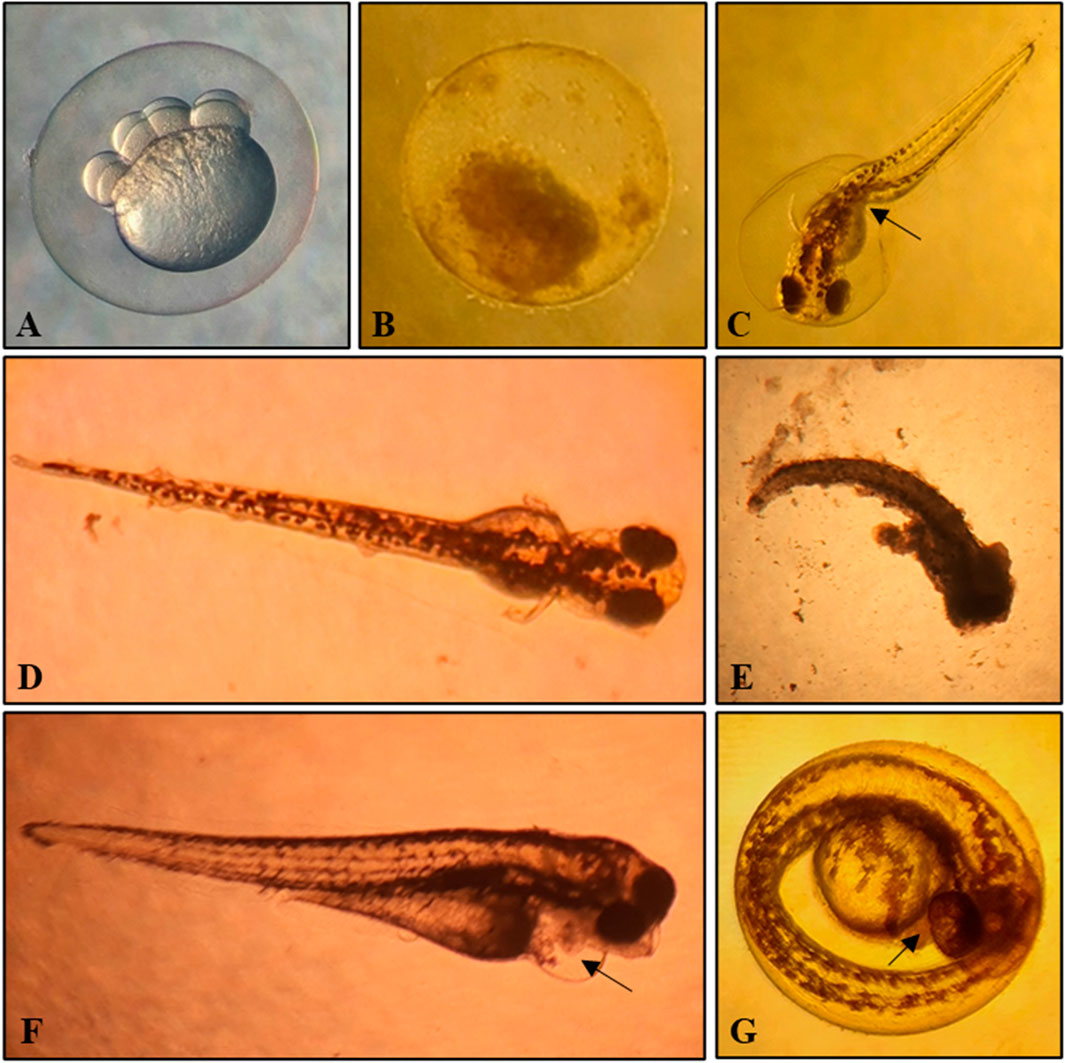
Figure 1. Cleavage, apparently normal embryo, and lethal and non-lethal alterations found in the experimental study. (A)- Embryo selected for the study with normal cleavage and less than 90 min post-fertilization. (B)- Coagulated embryo at 24 h after exposure to Y 250 mg/L. (C)- Spine curvature (arrow) in an embryo exposed to Y 40 mg/L at 72 h. (D)- Embryo apparently normal at 72 h after exposure to Nd 100 mg/L. (E)- Coagulated embryo at 72 h after exposure to Nd 100 mg/L. (F)- Pericardial oedema (arrow) in an embryo exposed to Nd 40 mg/L. (G)- Embryo without a heartbeat (arrow) and un-hatched at 96 h after exposure to Y 100 mg/L.
2.3 Acute toxicity test
This was carried out following the OECD guideline n° 236 headed “Fish Embryo Acute Toxicity Test” (OECD, 2013). Plates with twenty-four wells were used, with only one embryo per well. In each plate, 20 embryos were exposed to a concentration and 4 were used as an internal control in E3 medium. They were exposed for 96 h and placed in an incubator under controlled conditions at a temperature of 26°C and a light/dark cycle of 14 h/10 h.
Every 24 h, up to the 96 h that the study lasted, once a day and, for a few seconds, the embryonic development status of every embryo was checked, and the dead embryos were removed. The lethal effects were observed in the embryos at 24, 48, 72, and 96 h. The apical endpoints made in each embryo analysed included: coagulation, lack of somite formation, non-detachment of the tail, and lack of heartbeat. Any positive result from one of these four observations would make the embryo be considered as being dead. In relation to the heartbeat, particular care should be taken, since an irregular heartbeat should not be recorded as being lethal. Moreover, a visible heartbeat without any circulation in the aorta abdominalis can be considered to be non-lethal. To record this endpoint, embryos showing no heartbeat should be observed under a minimum magnification of ×80 for at least 1 minute. Absence of heartbeat is recorded after 48, 72, and 96 h. Regarding the lack of somite formation, a normally developed embryo shows spontaneous movements (side-to-side contractions), which indicate somite formation. Non-formation of somites after 24 h might be due to a general delay in development. After 48 h, at the latest, the somites should have formed; if not, the embryos can be considered as being dead. In addition to the apical endpoints used for calculating the LC50, according to the guideline, the moment at which the embryos are hatched was recorded from 48 h. Any incidence of morphological and physiological abnormalities observed during the study in the results have also been recorded.
The data obtained from the apical endpoints were used to calculate: the survival percentage; the maximum concentration not causing mortality; the minimum concentration triggering 100% mortality; the mortality in the controls; the graph of the concentration-mortality curve at the end of the test; the LC50 at the end of the test, and the regression equation for each of the substances evaluated.
2.4 Analytic measurements
According to OECD guideline, the concentrations of both chemicals were measured in all treatments at the beginning and end of the experiment. These analytic measurements were realized by an inductively coupled plasma mass spectrometer (ICP-MS) (Agilent 8900—Agilent Technologies, Palo Alto, CA, EE. UU.). The recovery of the analytical methods was verified utilizing the certified reference materials REE-1 (Rare Earth Elements, Zirconium and Niobium from Natural Resources Canada) for Nd and Y.
2.5 Statistical analysis
The statistical analysis of the data was made with 4.46 Rstudio software. With the data obtained and due to its nonlinearity, the probit model recommended by the OECD guideline (OECD, 2013) was not used. However, the guideline suggests using the model that best fits the data. Therefore, a non-linear regression equation was employed and adjusted to achieve the highest precision in determining the LC50. The equation used was as follows:
where, Y is the variable, we are trying to predict, i.e., the mortality, X corresponds to the concentration of the element in question, Y0 is the initial value of Y when X is zero, Plateau is the value to which Y approaches as X tends to infinity, and K is a constant that determines the rate of approach of Y towards the plateau. For each element, the parameters Y0, K, and plateau were calculated to achieve a higher R-squared, ensuring greater precision in the calculation. Subsequently, the LC50 was computed as ln2/K.
Other endpoints, such as non-lethal morphological alterations or non-hatching, were only quantified and described.
3 Results
3.1 Nd and Y concentrations in the medium
The results of the analytic measurements of Nd and Y are shown in Table 1. All the concentrations remained within the ± 20% of the nominal values.
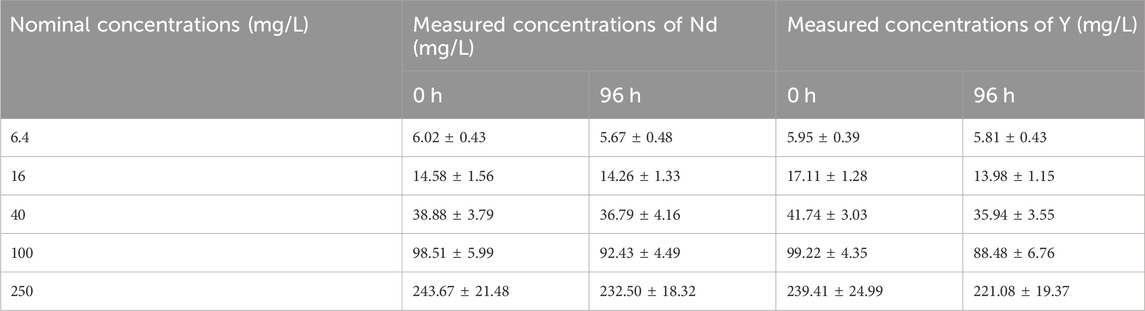
Table 1. Nominal and measured concentrations of Nd and Y in the medium test at the beginning and end of the experiment.
3.2 Acute Nd embryotoxicity
The observations considered as being lethal in the zebrafish embryos exposed to different Nd concentrations at between 24 and 96 h are shown in Table 2. Both the plate used as a control group and the internal controls showed a viability of over 90%. In none of the embryos employed were the lack of somite formation or the non-detachment of the tail detected. As can be observed in Table 2, the alteration detected that caused most of the deaths, independently of the concentration employed, was coagulation, which was fundamentally produced in the first 24 h.
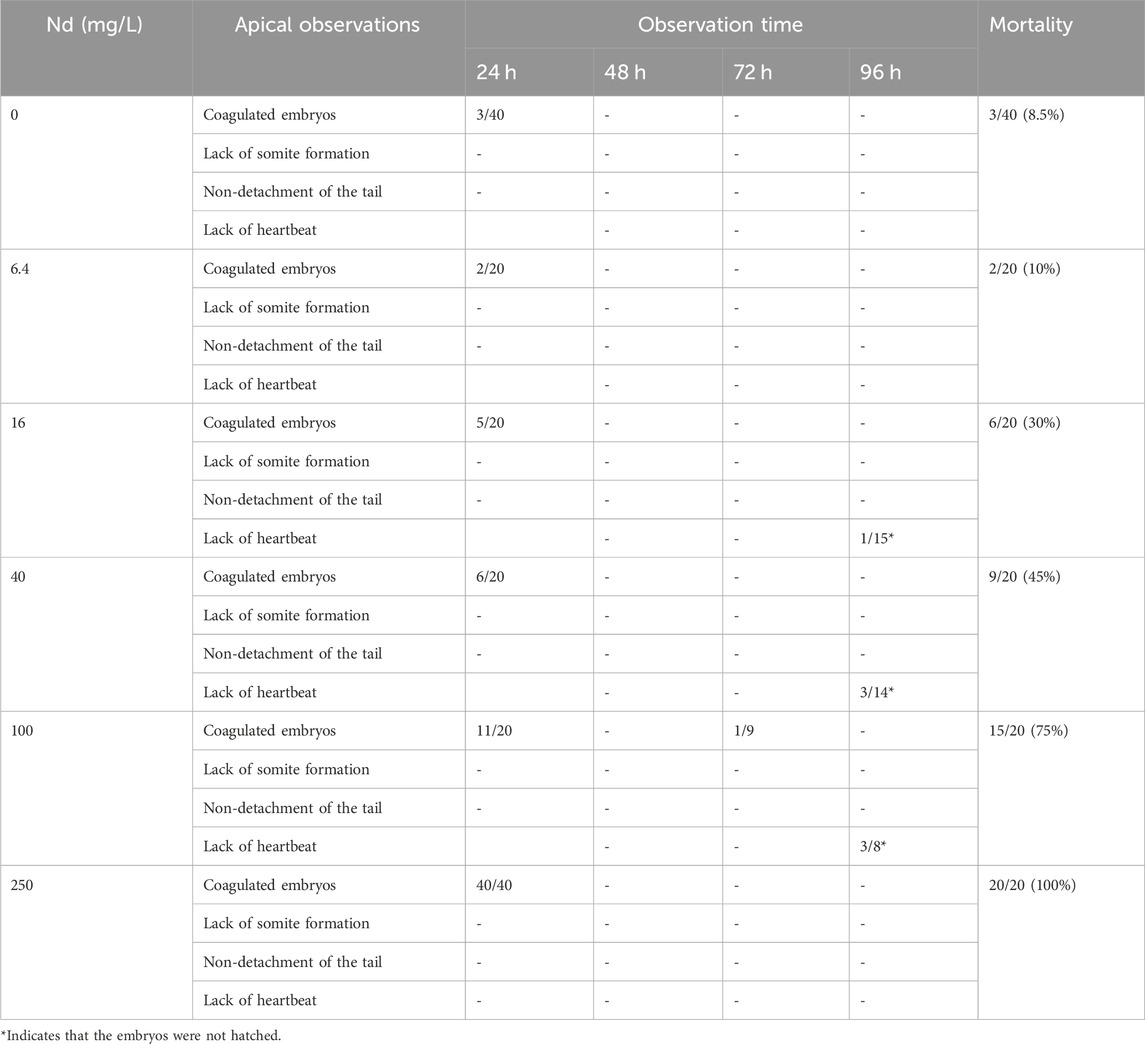
Table 2. Observations of acute toxicity in zebrafish embryos at between 24 and 96 h after fertilization and after exposure to different Nd concentations.
With respect to the concentrations, that of 6.4 mg/L showed a viability of over 90%, the same as the controls, so that it can be established as being a concentration that does not produce alterations in the embryos. Conversely, 250 mg/L concentration induced the coagulation of all the embryos at 24 h after starting the study. In the intermediate concentrations, 16 mg/L, 40 mg/L and 100 mg/L, a mortality at 96 h of 30%, 45%, and 75%, respectively, was determined. In those concentrations, as well as the embryos coagulation, another alteration particularly evidenced were the absence of a heartbeat in 7 embryos at 96 h, one embryo exposed to 16 mg/L, three embryos exposed to 40 mg/L, and three embryos exposed to 100 mg/L of Nd, respectively. These embryos did not hatch.
In relation to other morphological abnormalities evident, these appeared only in some embryos belonging to the exposed groups at 72 h. In this sense, we observed spinal curvature in one embryo at a concentration of 16 mg/L and in two embryos exposed to 40 mg/L. We also observed pericardial oedema in an embryo exposed to 40 mg/L. These alterations can be visualized in Figure 1.
The Y0 values were estimated at 7.545, the plateau at 103.8, and K at 0.01247. With these values, the non-linear regression equation achieved an R-squared of 0.9922, a value very close to unity, indicating high precision in the estimation. Finally, the LC50 for Nd was determined to be 55.58 mg/L. Figure 2 shows the concentration-mortality curve at the end of the test.
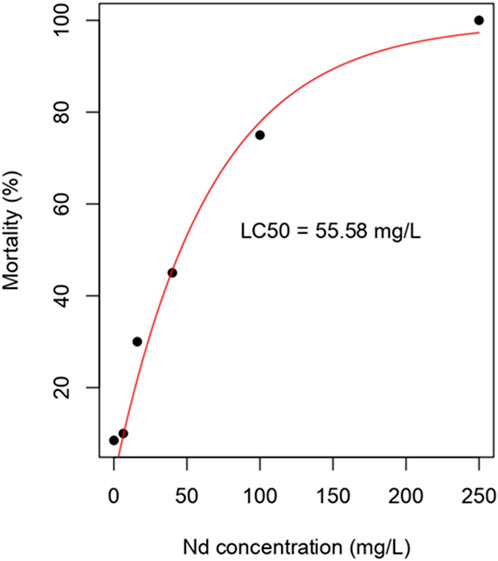
Figure 2. Concentration-mortality curve in zebrafish embryos exposed to different concentrations of Nd at 96 h.
3.3 Acute Y embryotoxicity
The observations considered as being lethal in zebrafish embryos exposed to different Y concentrations at between 24 and 96 h are shown in Table 3. Similarly to Nd, both the plate used as a control group and the internal controls gave a viability of over 90%. Besides, nor were the absence of somite formation or the non-detachment of the tail in any of the embryos detected, regardless of the concentration to which they were exposed. Both their coagulation, which occurred in the first 24 h, and the absence of a heartbeat and non-hatching, were similar to those of Nd, i.e., at 96 h.
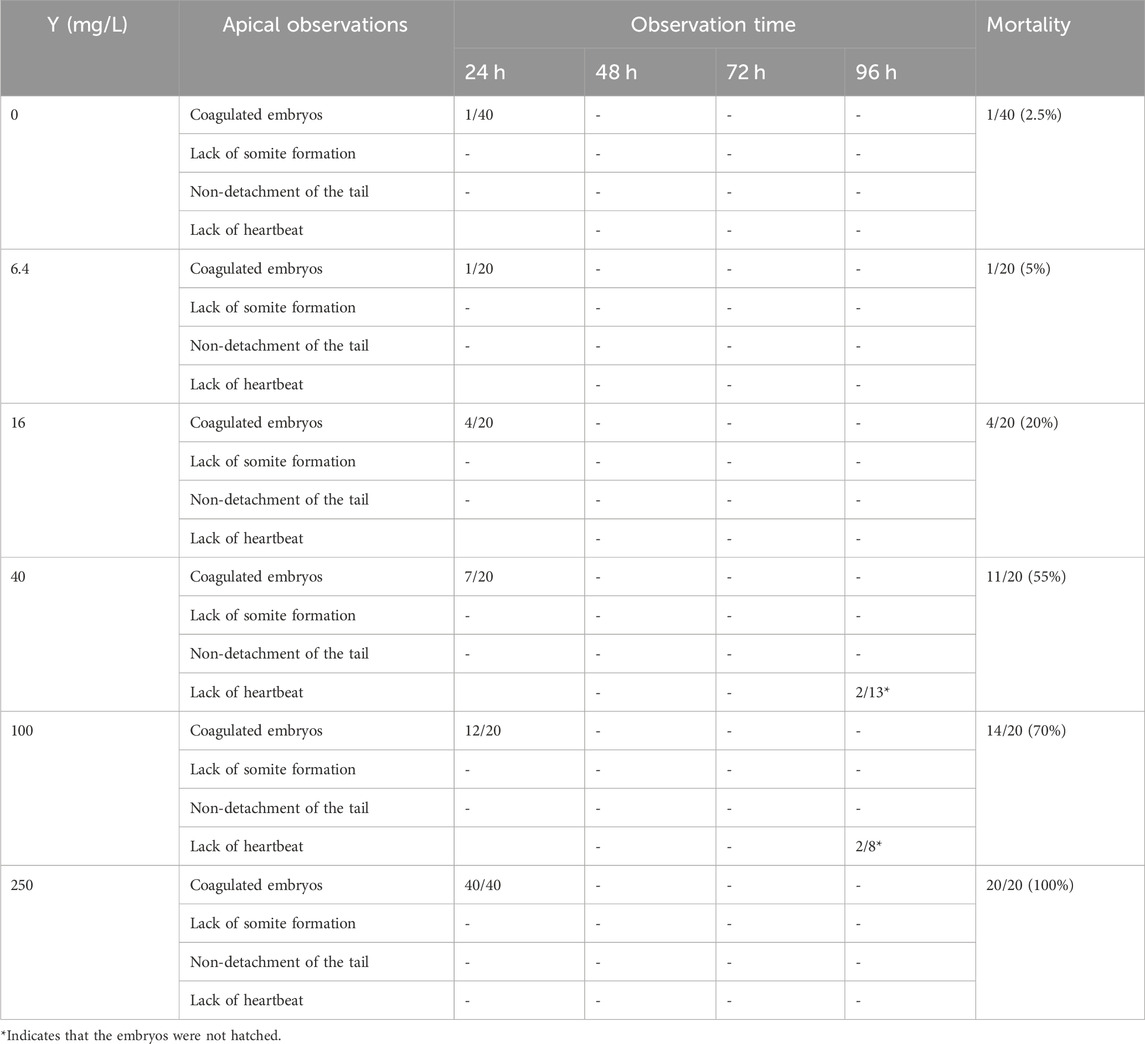
Table 3. Observations of acute toxicity in zebrafish at between 24 and 96 h after fertilization and being exposed to different concentrations of Y.
The test demonstrated that nor was the concentration of 6.4 mg/L sufficient to cause a higher mortality than over 90%. With respect to the rest of the concentrations, that of 16 mg/L triggered a mortality of 20%, that of 40 mg/L a 55% mortality, and that of 100 mg/L, 70%. In the 250 mg/L concentration, all the embryos were coagulated in the first 24 h, the same as in the case of Nd.
The Y0 values were estimated at 0.4066, the plateau at 99.52, and K at 0.01520. With these values, the non-linear regression equation achieved an R-squared of 0.9759. Finally, the LC50 for Y was determined to be 45.61 mg/L. Figure 3 shows the concentration-mortality curve at 96 h.
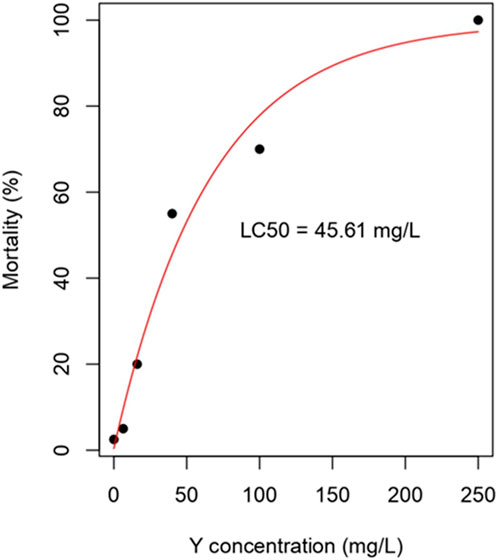
Figure 3. Concentration-mortality curve in zebrafish embryos exposed to different concentrations of Y at 96 h.
Regarding other morphological and physiological abnormalities observed, only spinal curvature was observed in two embryos exposed to the concentration of 40 mg/L at 48 h. These alterations can be visualized in Figure 1.
4 Discussion
Considering the limited toxicological information available about REEs, focused mainly on La and Ce for their extensive use, and on Gd for its exposure in humans (Pagano et al., 2015a; Rogowska et al., 2018; Egler et al., 2022). Our study provides relevant information about the acute toxicity of Nd, a light REEs, and Y, a heavy one. This is especially important when considering that the use of some of these elements is increasing in a variety of industrial applications, indicating a possible potential impact on human and environmental health (Trifuoggi et al., 2017). Moreover, it should be noted that REEs take a critical and irreplaceable role in various fields, especially the high-tech electronics industry, which is usually comparable to the vitamin of industry (Qiao et al., 2024). Therefore, it is possible to expect that the concentrations of these contaminants will increase in the next years, and, at the same time, to consider that the treatment and removal of these elements are limited currently (Al Momani et al., 2023), which increases the damage that they can cause in the ecosystems.
In the review carried out by Pereto et al. (2024), it was observed that the concentrations of REEs in marine organisms were between the concentrations in sediments and water. However, they also indicated that there is currently a lot of uncertainty regarding these elements concerning their distribution in aquatic organisms. This uncertainty is transferred to its toxic effects, Gonzalez et al. (2014) collected information on different articles in aquatic organisms and their conclusion was there is no consensus as to lanthanides showing a coherent and predictable pattern of (eco)toxicity in the same way as their atomic properties. They evaluated data from different articles about different species, such as Hyalella azteca, Chlorella vulgaris, Daphnia magna, Skeletonema costatum, Arcantia tonsa, or Poecilia reticulata, and, their results were contradictory, showing similar toxicity among elements, toxicity increasing based on atomic number, or toxicity following different patterns for each evaluated element. This suggests that the inherent toxicity of these elements should be evaluated individually for each element.
In our study, if we compare both REEs based on the value of their LC50 (55.58 mg/L for Nd, and 45.61 mg/L for Y), it can be said that the toxicity is higher for Y, whose LC50 is lower. However, their LC50 values are relatively close, differing by just 10 mg/L, so it could be said that their toxicity is alike for both REEs. In zebrafish embryos, Zhao et al. (2020) reported that, in general, there was a greater embryonic toxicity in heavy REEs compared to light ones. They obtained a very similar range of LC50 values within the light REEs group (7.49–15.4 mg/L), although, in their study, Nd toxicity was an exception and resembled that of the heavy REEs. On the contrary, Lin et al. (2022) determined that the REEs classified as being light were more toxic than the heavy ones since they presented lower values of LC50. Specifically, they established LC50 of 11.27 mg/L for Nd and 16.37 mg/L for Y. Independently, both articles mentioned established LC50 values lower than those determined in this study, attributing greater toxicity to Nd and Y. This suggests that these differences in the LC50 could be due to factors in the experimental design, namely, at what moment of the embryonic development the exposure begins, whether it has been done in wells or in Petri dishes, and the breeding and health conditions of the reproducers, among other factors. In our study, all the validity criteria stipulated by guideline were ensured. The latter comprised: fertilization of over 70%, 90% survival in the controls, hatching of over 80% at the end of the study, and the beginning of exposure in fewer than 90 min after fertilization. It is precisely there where the value of the implementation of a standardized methodology for the determination of toxicity stands out.
As for the studies carried out in zebrafish embryos on other REEs, Cui et al. (2012), at a concentration of 0.694 mg/L. They also perceived that exposure to La and Yb caused a delay in embryonic development, malformations in the tail, and diminution of the hatching rate. It should be noted that Cui et al. (2012) followed the methodology proposed by Schulte and Nagel (1994) and that they exposed the fish at the 4-cell stage (−35 min post fertilization). In our study, we have observed that some exposed embryos exhibited morphological and physiological alterations such as pericardial edema (only in Nd) or spinal curvature (Nd and Y). These alterations, although not used for calculating the LC50, could have an impact on the future survival of the adult individual. In the study by Cui et al. (2012), other morphological abnormalities were also observed after exposure to La and Yb, such as spinal curvature, caudal curving, or yolk sac edema. Zhao et al. (2020) also observed pericardial edema in embryos exposed to both Pr (at concentrations of 2.07 and 7.52 mg/L) and La (at concentrations of 0.65 mg/L, 1.90 mg/L, and 7.33 mg/L).
The results of this study are relevant in the context of the growing demand for REEs in diverse areas. The 96 h LC50 values obtained in zebrafish embryos, raise concern about their possible environmental pollution and their effects on aquatic biodiversity. These LC50 are usually above environmentally relevant concentrations, nevertheless, even higher values have been reported in surface waters (87.7 mg/L), soils, or sediments in areas near mining activities (Zhao et al., 2020). Moreover, it should be mentioned that those results are based on fish embryo exposure without considering their long-term accumulative impact on aquatic ecosystems, especially in areas contaminated with these types of elements. All the studies made also reveal the need to make appropriate regulations for these emerging pollutants, both industrially and environmentally, that would also be essential for the preservation of public health and of the ecosystems. Future research could be focussed on long term exposure, using different adult individuals from various trophic levels, and include the evaluation of toxicity between generations and of joint exposure to several REEs.
5 Conclusion
The main lethal effects were the coagulation and in minor measure the absence of heartbeat in the zebrafish embryos. These elements do not affect somite formation or tail detachment. On the other hand, the LC50 for both REEs in zebrafish embryos at 96 h could be said that it's similar, albeit slightly higher in Y. The fact that they share lethal effects and have similar LC50 values suggests that the toxicity between light and heavy REE is likely to be similar, at least between Nd and Y. These LC50 values have already been determined in contaminated areas, and the demand for these elements is expected to continue increasing in the coming years. Therefore, governments and institutions should focus on regulating them appropriately with the support of the scientific community. Studies like this could contribute to this effort.
Data availability statement
The raw data supporting the conclusion of this article will be made available by the authors, without undue reservation.
Ethics statement
The manuscript presents research on animals that do not require ethical approval for their study.
Author contributions
AJL-R: Conceptualization, Investigation, Writing–original draft, Writing–review and editing. AMM-L: Writing–review and editing, Conceptualization. RM-M: Formal Analysis, Visualization, Writing–original draft, Writing–review and editing. JA-H: Investigation, Methodology, Writing–original draft. NA-S: Supervision, Validation, Writing–original draft. RM-S: Supervision, Validation, Writing–review and editing.
Funding
The author(s) declare that no financial support was received for the research, authorship, and/or publication of this article.
Conflict of interest
The authors declare that the research was conducted in the absence of any commercial or financial relationships that could be construed as a potential conflict of interest.
Publisher’s note
All claims expressed in this article are solely those of the authors and do not necessarily represent those of their affiliated organizations, or those of the publisher, the editors and the reviewers. Any product that may be evaluated in this article, or claim that may be made by its manufacturer, is not guaranteed or endorsed by the publisher.
References
Al Momani, D. E., Al Ansari, Z., Ouda, M., Abujayyab, M., Kareem, M., Agbaje, T., et al. (2023). Occurrence, treatment, and potential recovery of rare earth elements from wastewater in the context of a circular economy. J. Water Proc.engineering 55, 104223. doi:10.1016/j.jwpe.2023.104223
Ayedun, H., Arowolo, T. A., Gbadebo, A. M., and Idowu, O. A. (2016). Evaluation of rare earth elements in groundwater of lagos and ogun states, southwest Nigeria. Environ. Geochem. Health 39, 649–664. doi:10.1007/s10653-016-9839-8
Bakhshalizadeh, S., Liyafoyi, A. R., Mora-Medina, R., and Ayala-Soldado, N. (2023). Bioaccumulation of rare earth elements and trace elements in different tissues of the golden grey mullet (Chelon auratus) in the southern Caspian Sea. Environ. Geochem. Health 45, 6533–6542. doi:10.1007/s10653-023-01593-w
Cui, J., Zhang, Z., Bai, W., Zhang, L., He, X., Ma, Y., et al. (2012). Effects of rare earth elements La and Yb on the morphological and functional development of zebrafish embryos. J. Environ. Sci. 24, 209–213. doi:10.1016/s1001-0742(11)60755-9
Dinh, T., Dobo, Z., and Kovacs, H. (2022). Phytomining of rare earth elements – a review. Chemosphere 297, 134259. doi:10.1016/j.chemosphere.2022.134259
Dubé, M., Auclair, J., Hanana, H., Turcotte, P., Gagnon, C., Gagné, F., et al. (2019). Gene expression changes and toxicity of selected rare earth elements in rainbow trout juveniles. Comp. Biochem. Physiol. C-Toxicol. Pharmacol. 223, 88–95. doi:10.1016/j.cbpc.2019.05.009
Egler, S. G., Niemeyer, J. C., Correia, F. V., and Saggioro, E. M. (2022). Effects of rare earth elements (REE) on terrestrial organisms: current status and future directions. Ecotoxicology 31, 689–699. doi:10.1007/s10646-022-02542-6
Elkina, V., and Kurushkin, M. (2020). Promethium: to strive, to seek, to find and not to yield. Front. Chem. 8, 588. doi:10.3389/fchem.2020.00588
Fernandes, Y., and Lovely, C. B. (2021). Zebrafish models of fetal alcohol spectrum disorders. Genesis 59, e23460. doi:10.1002/dvg.23460
Gonzalez, V., Vignati, D. A. L., Leyval, C., and Giamberini, L. (2014). Environmental fate and ecotoxicity of lanthanides: are they a uniform group beyond chemistry? Environ. Int. 71, 148–157. doi:10.1016/j.envint.2014.06.019
Gwenzi, W., Mangori, L., Danha, C., Chaukura, N., Dunjana, N., and Sanganyado, E. (2018). Sources, behaviour, and environmental and human health risks of high-technology rare earth elements as emerging contaminants. Sci. Total Environ. 636, 299–313. doi:10.1016/j.scitotenv.2018.04.235
Hatje, V., Bruland, K. W., and Flegal, A. R. (2016). Increases in anthropogenic gadolinium anomalies and rare earth element concentrations in San Francisco bay over a 20 Year record. Environ. Sci. Technol. 50, 4159–4168. doi:10.1021/acs.est.5b04322
Henríquez-Hernández, L. A., Acosta-Dacal, A. C., Boada, L. D., Zumbado, M., Serra-Majem, L., and Luzardo, O. P. (2023). Concentration of essential, toxic, and rare earth elements in ready-to-eat baby purees from the Spanish market. Nutrients 15, 3251. doi:10.3390/nu15143251
Huang, X., He, E., Qiu, H., Zhang, L., Tang, Y., Zhao, C., et al. (2020). Do toxicokinetic and toxicodynamic processes hold the same for light and heavy rare earth elements in terrestrial organism Enchytraeus crypticus? Environ. Pollut. 262, 114234. doi:10.1016/j.envpol.2020.114234
Kang, S., Guo, C., Ma, C., Mu, H., Liu, Z., and Sun, L. (2022a). Assessment of the biotoxicity of lanthanides (La, Ce, Gd, and Ho) on zebrafish (Danio rerio) in different water environments. Ecotoxicol. Environ. Saf. 246, 114169. doi:10.1016/j.ecoenv.2022.114169
Kang, S., Guo, C., Xue, C., Ma, C., Mu, H., and Sun, L. (2022b). Toxic effects of two representative rare earth elements (La and Gd) on Danio rerio based on transcriptome analysis. Toxics 10, 519. doi:10.3390/toxics10090519
Katoch, S., and Patial, V. (2020). Zebrafish: an emerging model system to study liver diseases and related drug discovery. J. Appl. Toxicol. 41, 33–51. doi:10.1002/jat.4031
Kimmel, C. B., Ballard, W. W., Kimmel, S. R., Ullmann, B., and Schilling, T. F. (1995). Stages of embryonic development of the zebrafish. Dev. Dyn. 203, 253–310. doi:10.1002/aja.1002030302
Klingelhöfer, D., Braun, M., Dröge, J., Fischer, A., Brüggmann, D., and Groneberg, D. A. (2022). Environmental and health-related research on application and production of rare earth elements under scrutiny. Glob. Health 18, 86. doi:10.1186/s12992-022-00879-5
Kulaksız, S., and Bau, M. (2013). Anthropogenic dissolved and colloid/nanoparticle-bound samarium, lanthanum and gadolinium in the Rhine River and the impending destruction of the natural rare earth element distribution in rivers. Earth Planet. Sci. Lett. 362, 43–50. doi:10.1016/j.epsl.2012.11.033
Lachaux, N., Cossu-Leguille, C., Poirier, L., Gross, E. M., and Giamberini, L. (2022). Integrated environmental risk assessment of rare earth elements mixture on aquatic ecosystems. Front. Environ. Sci. 10. doi:10.3389/fenvs.2022.974191
Lin, Y.-T., Liu, R.-X., Audira, G., Suryanto, M. E., Roldan, M. J. M., Lee, J.-S., et al. (2022). Lanthanides toxicity in zebrafish embryos are correlated to their atomic number. Toxics 10, 336. doi:10.3390/toxics10060336
Liu, W.-S., Guo, M.-N., Liu, C., Yuan, M., Chen, X.-T., Huot, H., et al. (2019). Water, sediment and agricultural soil contamination from an ion-adsorption rare earth mining area. Chemosphere 216, 75–83. doi:10.1016/j.chemosphere.2018.10.109
Lora, A. J., Molina, A. M., Bellido, C., Blanco, A., Monterde, J. G., and Moyano, M. R. (2016). Adverse effects of bisphenol A on the testicular parenchyma of zebrafish revealed using histomorphological methods. Vet. Med. - Czech 61, 577–589. doi:10.17221/212/2015-vetmed
Lu, V. M., McDonald, K. L., and Townley, H. E. (2017). Realizing the therapeutic potential of rare earth elements in designing nanoparticles to target and treat glioblastoma. Nanomedicine 12, 2389–2401. doi:10.2217/nnm-2017-0193
Malhotra, N., Hsu, H.-S., Liang, S.-T., Roldan, M. J. M., Lee, J.-S., Ger, T.-R., et al. (2020). An updated review of toxicity effect of the rare earth elements (REEs) on aquatic organisms. Animals 10, 1663. doi:10.3390/ani10091663
OECD (2013). Test No. 236: fish embryo acute toxicity (FET) test. OECD Guidel. Test. Chem. Section 2. doi:10.1787/9789264203709-en
Okeme, I. C., Crane, R. A., Nash, W. M., Ojonimi, T. I., and Scott, T. B. (2022). Characterisation of rare earth elements and toxic heavy metals in coal and coal fly ash. RSC Adv. 12, 19284–19296. doi:10.1039/d2ra02788g
Pagano, G., Aliberti, F., Guida, M., Oral, R., Siciliano, A., Trifuoggi, M., et al. (2015b). Rare earth elements in human and animal health: state of art and research priorities. Environ. Res. 142, 215–220. doi:10.1016/j.envres.2015.06.039
Pagano, G., Guida, M., Siciliano, A., Oral, R., Koçbaş, F., Palumbo, A., et al. (2016). Comparative toxicities of selected rare earth elements: sea urchin embryogenesis and fertilization damage with redox and cytogenetic effects. Environ. Res. 147, 453–460. doi:10.1016/j.envres.2016.02.031
Pagano, G., Guida, M., Tommasi, F., and Oral, R. (2015a). Health effects and toxicity mechanisms of rare earth elements—knowledge gaps and research prospects. Ecotoxicol. Environ. Saf. 115, 40–48. doi:10.1016/j.ecoenv.2015.01.030
Pereto, C., Baudrimont, M., and Coynel, A. (2024). Global natural concentrations of Rare Earth Elements in aquatic organisms: progress and lessons from fifty years of studies. Sci. Total Environ. 922, 171241. doi:10.1016/j.scitotenv.2024.171241
Perrat, E., Parant, M., Py, J.-S., Rosin, C., and Cossu-Leguille, C. (2017). Bioaccumulation of gadolinium in freshwater bivalves. Environ. Sci. Pollut. Res. 24, 12405–12415. doi:10.1007/s11356-017-8869-9
Qiao, J., Li, L., Liu, J., Wu, N., Liu, W., Wu, F., et al. (2024). The vital application of rare earth for future high-performance electromagnetic wave absorption materials: a review. J. Mater. Sci. 176, 188–203. doi:10.1016/j.jmst.2023.09.003
Ramos, S. J., Dinali, G. S., Oliveira, C., Martins, G. C., Moreira, C. G., Siqueira, J. O., et al. (2016). Rare earth elements in the soil environment. Curr. Pollut. Rep. 2, 28–50. doi:10.1007/s40726-016-0026-4
Risalde, M. A., Molina, A. M., Lora, A. J., Ayala, N., Gómez-Villamandos, J. C., and Moyano, M. R. (2021). Immunohistochemical expression of aromatase cyp19a1a and cyp19a1b in the ovary and brain of zebrafish (Danio rerio) exposed to different concentrations of bisphenol A. Aquat. Toxicol. 237, 105876. doi:10.1016/j.aquatox.2021.105876
Rogowska, J., Olkowska, E., Ratajczyk, W., and Wolska, L. (2018). Gadolinium as a new emerging contaminant of aquatic environments. Enviro. Toxic. Chem. 37, 1523–1534. doi:10.1002/etc.4116
Rosa, J. G. S., Lima, C., and Lopes-Ferreira, M. (2022). Zebrafish larvae behavior models as a tool for drug screenings and pre-clinical trials: a review. IJMS 23, 6647. doi:10.3390/ijms23126647
Schreiber, A., Marx, J., and Zapp, P. (2021). Life Cycle Assessment studies of rare earths production - findings from a systematic review. Sci. Total Environ. 791, 148257. doi:10.1016/j.scitotenv.2021.148257
Schulte, C., and Nagel, R. (1994). Testing Acute Toxicity in the Embryo of Zebrafish, Brachydanio rerio, as an Alternative to the Acute Fish Test: Preliminary Results. Altern. Lab. Anim. 22, 12–19. doi:10.1177/026119299402200104
Tepe, N., Romero, M., and Bau, M. (2014). High-technology metals as emerging contaminants: strong increase of anthropogenic gadolinium levels in tap water of Berlin, Germany, from 2009 to 2012. App. Geochem. 45, 191–197. doi:10.1016/j.apgeochem.2014.04.006
Trifuoggi, M., Pagano, G., Guida, M., Palumbo, A., Siciliano, A., Gravina, M., et al. (2017). Comparative toxicity of seven rare earth elements in sea urchin early life stages. Environ. Sci. Pollut. Res. 24, 20803–20810. doi:10.1007/s11356-017-9658-1
USEPA (2012). Rare earth elements: a review of production, processing, recycling, and associated environmental issues. Washington D.C.: Office of Research and Development Cincinnati, OH. EPA/600/R-12/572.
Wu, S., Bie, C., Su, H., Gao, Y., and Sun, X. (2022). The effective separation of yttrium and other heavy rare earth elements with salicylic acid derivatives. Min. Eng. 178, 107396. doi:10.1016/j.mineng.2022.107396
Keywords: aquatic organism, embryonic mortality, emerging pollutant, rare earth elements, water pollution
Citation: Lora-Benítez AJ, Molina-López AM, Mora-Medina R, Aguilar-Herrera JE, Ayala-Soldado N and Moyano-Salvago R (2024) Evaluation of acute toxicity of neodymium and yttrium in zebrafish (Danio rerio) embryos. Front. Environ. Sci. 12:1390948. doi: 10.3389/fenvs.2024.1390948
Received: 24 February 2024; Accepted: 15 April 2024;
Published: 17 May 2024.
Edited by:
Yalçın Tepe, Giresun University, TürkiyeReviewed by:
Nüket Sivri, Istanbul University-Cerrahpasa, TürkiyeVerónica Nogueira, University of Porto, Portugal
Carlos Tolussi, Universidade Federal do Ceará, Brazil
Copyright © 2024 Lora-Benítez, Molina-López, Mora-Medina, Aguilar-Herrera, Ayala-Soldado and Moyano-Salvago. This is an open-access article distributed under the terms of the Creative Commons Attribution License (CC BY). The use, distribution or reproduction in other forums is permitted, provided the original author(s) and the copyright owner(s) are credited and that the original publication in this journal is cited, in accordance with accepted academic practice. No use, distribution or reproduction is permitted which does not comply with these terms.
*Correspondence: Antonio Jesús Lora-Benítez, v12lobea@uco.es; Rafael Mora-Medina, v02momer@uco.es
†These authors have contributed equally to this work
‡These authors have contributed equally to this work and share senior authorship