- Department of Strategic Research and Talent Services, Shenzhen Science and Technology Innovation Strategy Research and Technology Transfer Promotion Center, Shenzhen, China
Blue Carbon is increasingly popular worldwide, and it also belongs to circular economy field which would last a long period in academy. To further understand the development and characteristics of Blue Carbon, this study uses CiteSpace software to perform quantitative analysis on literature published on Web of Science and Chinese CNKI database with the main topic of “Blue Carbon.” The results show that: (1) Since 2009, increasing attention has been paid to Blue Carbon research, among which the United States, Australia and China are the leading countries. (2) Keywords such as “Blue Carbon,” “mangrove,” “carbon sequestration” and “carbon neutrality” are the high-frequency keywords appearing both in China and the World in recent years. (3) Spartina alterniflora, soil organic carbon, livestock, trophic cascade, biodiversity and seagrass ecosystem clustering are hot topics related to international Blue Carbon research, while organic carbon, seagrass-conservation, methodology, sustainable development, climate change, carbon neutralization, coastal zone and carbon storage are hot topics related to Chinese Blue Carbon research. In addition, based on the research trends of Blue Carbon, this paper attempts to sort out the research framework from three levels: core characteristics, theoretical basis and research methods, respectively. Facing a series of challenges brought by climate change, Blue Carbon research should strengthen the accurate estimations of Blue Carbon sink and its market potential, and explore the reasonable market mechanism and protection mechanism, to respond to realistic demands, solve major concerns and promote new progress in Blue Carbon theory and practice.
1 Introduction
In recent years, the global average temperature has risen by 1.2°C. The harm of global warming involves all aspects of human existence, from natural disasters to biological chain breakage. In this context, the “Double Carbon” goal of carbon peak and carbon neutrality, which was first put forward in China, has become the most important economical means for many countries and regions to control the total greenhouse gas emissions. As a component of global climate change adaptation and mitigation strategy, Blue Carbon has become a new field of carbon sink, which is increasingly popular in China.
In 2009, the definition of “Blue Carbon” was first proposed in “Blue Carbon: Carbon Sequestration in a Healthy Ocean——Rapid Response Assessment Report,” that is, carbon could be captured by marine activities and marine organisms (Nellemann et al., 2009). As the planet’s largest active reservoir, the oceans store 93 percent of the planet’s carbon dioxide. Compared with terrestrial carbon sequestration system, marine carbon sequestration system has a higher return on investments in economic and ecological aspects. Coastal ecosystems covered by vegetation, especially carbon sequestered by mangroves, seagrass beds and salt marshes, play an important role in regulating climate and mitigating global warming (Mcleod et al., 2011). Since the United Nations put forward the concept of “Blue Carbon” in 2009 and pointed out its important role in carbon capture, all countries have accelerated the research on Blue Carbon.
In this study, we would set China as the most important sample to conduct analysis and comparison with other countries. China is one of the few countries with three coastal Blue Carbon ecosystems: mangrove forest, salt marsh and seagrass bed. Therefore, Chinese research on Blue Carbon presents more practical values. However, the current research results of Chinese scholars on carbon sinks mainly focus on forest carbon sinks, and the research attention on ocean carbon sinks (also known as Blue Carbon) needs to be improved. On the one hand, compared with the development trend of quantity and quality of Blue Carbon research abroad, the sorting of Blue Carbon theory in China lags behind. On the other hand, at present China faces a series of challenges, such as ecosystem resource degradation, unclear Blue Carbon resource reserves, carbon sequestration capacity and sink increase potential, and imperfect laws and regulations, which require the guidance of Blue Carbon theory. Overall, Chinese research could give practical implications to more areas.
As some countries including China nearly enter the stage of “carbon neutrality,” it is urgent to clarify the domestic and international Blue Carbon research context, grasp the latest research hotspots, and discover the research cooperation network to provide theoretical guidance for the development of Blue Carbon. Therefore, in this paper, CiteSpace 5.8. R3 software was used, and literature in Web of Science and CNKI of China were used as sources. By drawing visual knowledge maps, the Blue Carbon research was summarized by combining econometric reviews (review width) and systematic reviews (review depth). Firstly, the author, institution, country or region distribution of Blue Carbon research was analyzed. Secondly, the keywords co-occurrence map and area map of Blue Carbon research were drawn to summarize the hot spots and trends in this field and identify important topics in Blue Carbon research. Thirdly, the overall theoretical framework of Blue Carbon research was established by sorting and analyzing the core characteristics, the theoretical basis, the protection and restoration, and the research methods of Blue Carbon. Finally, the future research of Blue Carbon is prospected.
2 Research design
2.1 Data sources
This paper focuses on analyzing the evolution process and structure of Blue Carbon research, and the research samples include literature published by Web of Science and Chinese CNKI. Firstly, the Web of Science database was selected and the theme was set as “Blue Carbon.” A total of 962 literature data were retrieved in unlimited time, and the data retrieval time was 2 October 2021. To reflect the integrity, representativeness and academicality of data, irrelevant literature and data such as conference abstracts, letters, data papers, books, news and revisions were removed, and a total of 865 valid literature and data were obtained from 2011 to 2021. Second, the CNKI database of China was selected and the subject was set as “Blue Carbon.” A total of 164 literature were retrieved in unlimited time, and the data retrieval time was 2 October 2021. To reflect the integrity, representativeness and academicality, irrelevant literature was removed. And data of162 valid literature was obtained from 2009 to 2021.
2.2 Methods
This paper adopts the method of bibliometrics and literature review. Firstly, the basic characteristics of Blue Carbon research, such as annual publications, research institutions, authors and countries/regions, were analyzed to discuss the basic trends of Blue Carbon research. Secondly, keyword clustering, keyword co-occurrence and keywords with the strongest citation bursts were carried out to explore the mainstream subdivision fields, research hotspots and frontiers of Blue Carbon. In this paper, CiteSpace software was used for visual analysis of data to show knowledge structure, composition and distribution in Blue Carbon field, and to discuss knowledge development process (Li et al., 2018), research hotspots and frontiers in Blue Carbon field (Chen et al., 2015).
3 Analysis of basic characteristics of Blue Carbon research literature
3.1 Analysis of time characteristics and publications
The annual distribution of Blue Carbon research papers in international and domestic journals is shown in Figure 1. It can be seen from Figure 1 that, from 2009 to 2011, rare Blue Carbon studies were published in international journals. The first paper was published in 2011, and only 3 papers were published in 2011. Since 2015, there have been more than 60 articles every year. Especially in recent years (2018–2021), there are more than one hundred of relevant studies every year, and the number of them reached a record high of 205 in 2020. The rise of Blue Carbon research in international journals is closely related to the policies and scientific research of national organizations such as Conservation International (CI), the International Union of Nature (IUCN), the Intergovernmental Oceanographic Commission (IOC) and the United Nations Educational, Scientific and Cultural Organization (UNESCO) that are committed to promoting Blue Carbon worldwide. Secondly, the first paper of Blue Carbon research in China was published in 2009. No relevant literature was published in 2010 and 2011; The number of papers published from 2012 to 2014 was in single digits, and after 2015, more than 10 papers were published. In 2021, 39 articles were published as of the search date. Therefore, domestic and foreign attentions to Blue Carbon research are both increasing, and the number of papers is expected to increase further. This is closely related to the realistic background of Chinese carbon neutrality strategy.
3.2 Analysis of research institutions
The top 10 research institutions in the number of Blue Carbon research achievements published in international and domestic journals are shown in Table 1. Among them, the top 10 research institutions in international journals are Deakin Univ (59), Edith Cowan Univ (57), Univ Queensland (52), Chinese Acad Sci (40), Southern Cross Univ (35), Griffith Univ (31), CSIC (30), Natl Univ Singapore (29), Univ Technol Sydney (29). The most published institutions are still developed countries in Europe and the United States. However, the number of Chinese Acad Sci (Chinese Academy of Sciences) published in China has risen to the fourth in the world, occupying a place in Blue Carbon research.
The top 10 research institutions in domestic journals are Guangxi Academy of Sciences (40), Ministry of Natural Resources (37), Xiamen University (28), Beijing Normal University (18), Sun Yat-sen University (13), Ocean University of China (16), National Marine Information Center (16), Hainan University (16), State Oceanic Administration of China (13), Shanghai Institute of Geological Survey (13). At present, ocean-related research institutes and government departments are the main body of Blue Carbon research in China. From the geographical location, scientific research and education institutions in coastal areas are the main positions of Blue Carbon research in China. While some comprehensive universities, such as Xiamen University, Beijing Normal University and Sun Yat-sen University have also produced many research results. However, it is worth noting that the Blue Carbon research achievements of non-key scientific research and education institutions need to be further increased, especially the newly-established double first-class universities related to the sea, which should increase the amount of Blue Carbon academic output.
3.3 Analysis of authors
Author co-occurrence analysis can identify the cooperation and cross-citation relationship between the core authors and researchers in a research field (Hu et al., 2013). The cooperation network of Blue Carbon research authors in international and domestic journals is shown in Figures 2, 3. The node size represents the number of articles, the line between nodes represents the existence of cooperative relationship between authors, and the thickness of the line represents the strength of cooperation between authors.
The cooperative authors network of Blue Carbon research in international journals from 2011 to 2021 is shown in Figure 2. High-yielding authors (in order of publications) form a relatively close cooperative relationship, and the top 10 authors in publications include CARLOS M DUARTE (38), CATHERINE E LOVELOCK (34), OSCAR SERRANO (33), PETER I MACREADIE (27), DANIEL A FRIESS (26), CHRISTIAN J SANDERS (20), PAUL S LAVERY (16), DAMIEN T MAHER (15), ISAAC R SANTOS (14), etc. It can be seen that there is a relatively close cooperative relationship among high-producing authors in international journals.
As for the cooperative authors network of Blue Carbon research in Chinese journals, the top 10 authors in terms of number of publications include Zhao Peng (33), Qiu Guanglong (27), Fan Hangqing (27), Zhong Yunxu (26), Su Zhinan (26), He Qinfei (15), He Feng (15), Liu Xiu (15), Liu Chang (13) and Zhou Jun (13). Compared with the cooperative authors network of Blue Carbon research in international journals, the links in Chinese journals are not very close, whose the overall and relatively centralized cooperative network relationship has not been formed now. The research on Blue Carbon in China is still in the initial stage, which needs to be further expanded.
3.4 Analysis of country or region
The number of publications could reflect the research level and contribution of different countries or regions in Blue Carbon. Figure 3 shows the national or regional network map of Blue Carbon research. The United States, with 294 papers, is the strongest country. Australia (260), China (134), Spain (89), England (85), Germany (49), Saudi Arabia (49), Indonesia (47), Brazil (47), Japan (47), Canada (41), France (35), Denmark (33), Singapore (33), Scotland (33), Malaysia (30) and other countries or regions have also made great contributions to Blue Carbon research. Most countries or regions with more than 30 papers usually have unique natural conditions for developing Blue Carbon, promoting the research on Blue Carbon in these countries or regions. In addition, China ranked third in the number of publications, indicating that China plays an important role in Blue Carbon research. However, in terms of amount, there is still a certain gap——less than half of the amount of the United States and about half of Australia.
3.5 Analysis of highly cited references
Cited references can reflect the overall knowledge base of the field, and highly cited references are regarded as the classic of the field. Cluster analysis reveals the knowledge structure of a certain field and reflects important scholars and classical literature. The co-citation analysis results of Blue Carbon research in international journals are shown in Figure 4. Each node represents a citation, the size of the node is proportional to its co-citation frequency, and the link between nodes means that they are co-cited. Overall, the top 10 highly cited literature explores Blue Carbon conservation and restoration, and measures Blue Carbon and its effect.
4 Results and analysis
4.1 Results and analysis of Blue Carbon research in international journals
4.1.1 Identification of theme
Keywords are a high generalization of the topic of literature. Keywords with high frequency usually indicate that more research has been carried out through this keyword, which is more important and is used to determine the hot spot in a research field. To understand the hot spots of Blue Carbon international research from 2011 to 2021, the sorted 865 literature records were imported into CiteSpace software. The network node was selected as “Keyword,” and appropriate thresholds were set. There were 52 keywords with frequency greater than 20 (as shown in Table 2).
Firstly, in terms of the top 10 keywords, “Blue Carbon” topped the list 273 times. The second most frequent occurrence is “forest,” which can be seen as a specific component of Blue Carbon. In particular, mangrove forests provide a wide range of ecosystem services. Mangrove forests are known for their extraordinary carbon storage capacity, playing an important role in climate change mitigation. Moreover, organic carbon, sediment and other key elements should also belong to this concept. Secondly, storage and sequestration of Blue Carbon are also the focus of scholars. The Blue Carbon ecosystem and its dynamics are also of interest to scholars. In addition, Climate change is also a high-frequency keyword of Blue Carbon in international journals.
To better explain and analyze the academic significance of keywords transmitted in the above international journals, the key points were extracted by LLR algorithm of CiteSpace. Based on this, a cluster map of high-frequency keywords was generated, forming 16 knowledge clusters (Figure 5). They are Spartina Alterniflora, Soil organic carbon, Matter, Livestock, Trophic cascade, Biodiversity, Seagrass ecosystem, Arabian Gulf, Posidonia Oceanica, Sentinel, GIS, Salt marshes, Rehabilitation, Stable isotopes, Brown algae, etc., Modularity = 0.7746 > 0.3, Mean Silhouette = 0.9044 > 0.5, indicating good clustering and a clear silhouette. Seagrass ecosystem, salt marshes and brown algae are the specific components of Blue Carbon. Arabian qulf and Posidonia oceanica are the most frequently studied objects of Blue Carbon. Spartina Alterniflora and Rehabilitation are currently hot spots in Blue Carbon research.
4.1.2 Evolution of theme
After more than 10 years of practice and development, the research content of Blue Carbon in international journals has been enriched and expanded. The time-zone diagram for keyword co-occurrence analysis is shown in Figure 6. To further explore the evolution of topics in international journals, the nodes in the same period were collected in the same time zone and arranged in order from far to near. From the perspective of the main line, high-frequency keywords mainly appeared from 2011 to 2016, during which the newly emerged keywords occupied the majority of all keywords. From the perspective of newly emerged keywords in recent years, globally significant (2017) and global pattern (2018) confirm that international scholars gradually call for strengthening global cooperation and enhancing the attention of Blue Carbon in the global carbon sink system.
4.1.3 Frontier analysis
Citation Burst analysis is one of the most important tools in literature content mining, reflecting active or cutting-edge research nodes. This paper uses “Burst” to predict Blue Carbon research frontier and direction. The top 9 keywords with the strongest citation bursts in Blue Carbon research in international journals are shown in Figure 7.
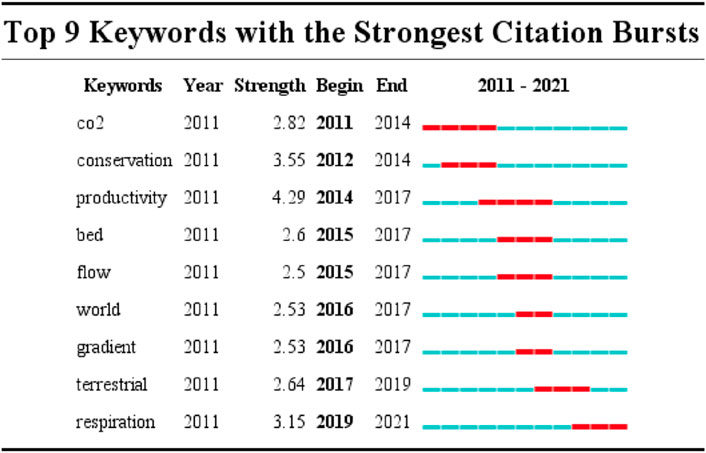
Figure 7. Top 9 keywords with the strongest citation bursts of Blue Carbon in international journals.
In terms of burst intensity, “Productivity” had the highest burst intensity at 4.29. This term reflects that the important development of Blue Carbon research is to explore the productivity of Blue Carbon, which has become a relatively influential research frontier. At the same time, the burst intensity of Conservation, Respiration and CO2 was 3.55, 3.15, and 2.82, respectively, which were important frontiers of Blue Carbon.
In terms of burst time, “CO2” is the theme word with the earliest appearance (2011) and the longest duration (5 years), which has become a frontier issue of continuous research. “Conservation” first appeared in 2012 and lasted for 3 years; “Productivity” first appeared in 2014 and lasts 4 years; “Bed” and “Flow” first appeared in 2015 and lasted for 3 years. They are also frontier studies in the field of Blue Carbon. At the same time, from the keywords with the strongest citation bursts in recent 3 years (2019–2021), “Respiration” is the research frontier in the field of Blue Carbon.
4.2 Results and analysis of Blue Carbon research in domestic journals
4.2.1 Identification of theme
To understand the domestic research hotspots of Blue Carbon from 2009 to 2021, 162 pieces of literature were imported into CiteSpace. The network nodes selected “Keyword,” and appropriate thresholds are set. There were 40 keywords with frequency greater than 3 in total (as shown in Table 3). “Mangrove” is an important ecosystem of marine Blue Carbon sink, and its carbon pool includes vegetation biomass and soil carbon pool. “Blue Carbon” came in second with 45. Blue Carbon sink systems such as seagrass beds, coastal wetlands and coastal zones were also in the top 10.
The centrality represents the status of the keyword in the field, and the higher the value is, the more important it is. The centrality of coastal wetland was 0.40, ranking first among all the keywords. Salt marsh appeared only 11 times, but its centrality ranked second, with 0.38. In addition, seagrass appeared 3 times, with 0.37. Then mangrove forests (centrality = 0.36), organic carbon (centrality = 0.26), seagrass beds (centrality = 0.25), etc.
To better explain and analyze the academic significance of Blue Carbon research keywords transmitted from Chinese journals, the LLR, algorithm of CiteSpace was used to extract keywords and generate high-frequency keyword clustering map. Nine knowledge clusters were formed (Figure 8), including organic carbon, seagrass conservation, methodology, sustainable development, climate change, carbon neutrality, coastal zone, carbon storage, etc. Modularity = 0.8461 > 0.3, Mean Silhouette = 0.9578 > 0.5, indicating that the keyword clustering is in good condition and has a clear outline.
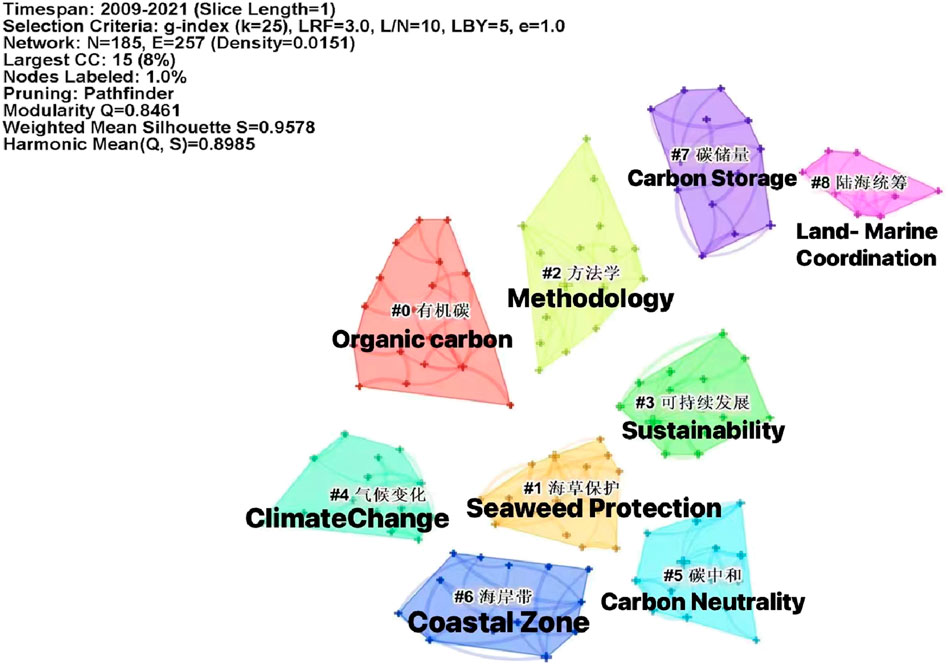
Figure 8. Keywords clustering map of Blue Carbon in domestic journals. Note: the map is automatically generated by importing Citespace from CNKI database. To better reflect the original state of the data, it is displayed both in Chinese and English characters.
4.2.2 Evolution of theme
Co-occurrence analysis of keywords in Blue Carbon research in domestic journals was conducted through CiteSpace to generate time-zone map. The map sets the keywords that appear for the first time at the same time in the same time-zone in order from far to near (Chen, 2017). It can be seen that keywords with high frequency (large node size) are mainly concentrated in the time zone of 2009. As the central theme, the nodes of “mangrove,” “Blue Carbon” and “carbon neutrality” are the largest, and they have intensive links with other keywords, indicating that the research on Blue Carbon in China CNKI database has been inherited since 2009. From the perspective of the evolution of theme in China, although there were many research topics in 2009, the most core themes were Blue Carbon subsystems such as “seagrass bed,” “mangrove,” “coastal wetland,” “salt marsh,” and other realistic backgrounds such as “carbon neutrality.” These mainly focused on the conceptual and policy. In 2012, the focus was on “organic carbon;” In 2013, “Eelgrass,” “Chiu-lung River Estuary” and other specific objects began to appear. In 2014, the emergence of “World First,” “China Blue,” “Land-sea integration” and others showed that the focus of Blue Carbon in China has shifted from simply focusing on marine regions to land-sea integration, and also began to pay attention to China’s position in the world’s Blue Carbon resources and research. In 2015, it began to focus on nitrogen research. From 2017 to 2018, it began to pay attention to the impact of environmental pollution on Blue Carbon. In 2019, the main focus was on the construction of marine pastures.
5 Analysis framework for Blue Carbon research
This paper constructed an overall analysis framework for Blue Carbon research based on the above analysis. As shown in Figure 9, the theoretical framework of Blue Carbon consists of three modules: core characteristics, theoretical basis and research methods.
5.1 Core characteristics of Blue Carbon
5.1.1 Definition, distribution and classification
5.1.1.1 Definition
Blue Carbon is a process, activity and mechanism that uses marine activities and organisms to absorb carbon dioxide from the atmosphere, fix and store it in the ocean. In a broad sense, coastal Blue Carbon refers to the part of carbon that higher plants in the coastal zone, such as salt marsh wetlands, mangroves and seagrass beds, as well as phytoplankton, algae and shellfish, absorb CO2 from the atmosphere and store it in the sediment of the coastal zone for a long time under the joint action of their growth and microorganisms. Furthermore, part of the organic carbon exported from coastal zone to offshore and ocean (Tang et al., 2018). In a narrow sense, Blue Carbon refers to carbon stored in the soils of mangroves, intertidal salt marshes and seagrass beds, aboveground living biomass (roots) and non-living biomass (such as litter and deadwood). This part of Carbon is also called Coastal Blue Carbon (Nellemann et al., 2009; Mcleod et al., 2011).
5.1.1.2 Distribution
In terms of geographical location, Blue Carbon is mainly distributed along the coastlines of countries or regions. The distribution of Blue Carbon in China is mainly from the mouth of the Yalu River in the north to the mouth of the Beilun River in the south, passing through Liaoning, Hebei, Tianjin, Shandong, Jiangsu, Shanghai, Zhejiang, Fujian, Guangdong, Guangxi, Hainan and other 11 provinces (autonomous regions, municipalities directly under the Central Government). According to wetland types, mangroves are mainly distributed in subtropical and tropical coastal areas in the south. Coastal salt marshes are distributed in temperate to subtropical seas; Seagrass beds are mainly distributed in temperate to tropical seas.
5.1.1.3 Type
Coastal Blue Carbon is an important part of marine carbon sink, and the carbon dioxide (CO2) is fixed by vegetation in coastal wetland ecosystem such as mangroves (small but very important), salt marsh (main) and seagrass bed is the main body of coastal Blue Carbon ecosystem. They maintain the highest carbon sequestration per unit area of any natural system (Rogers et al., 2019). In addition, macroalgae (Ortega et al., 2019; Watanabe et al., 2020; Pedersen et al., 2021), shellfish (Lee et al., 2020) and even micro-organisms (Trevathan-Tackett et al., 2018) can also fix and store carbon efficiently, which are components of Blue Carbon.
5.1.2 Functions of Blue Carbon
Blue Carbon has important ecological service functions, including fish and wildlife habitat, coastal erosion and flood prevention, food resources, water purification, carbon sequestration and storage. Blue Carbon has high ecological and economic development value. Among them, mangroves are special wetland forests growing in the intertidal zone of tropical and subtropical regions, which play an important role in windbreak and field retention, promoting silt deposition, resisting natural disasters such as tsunami and typhoon, and protecting coastlines (Zhang et al., 2013).
However, the main function of Blue Carbon is carbon sequestration and carbon sink. Carbon sequestration refers to measures to increase the carbon content of carbon pools other than the atmosphere. Coastal Blue Carbon ecosystem has efficient carbon sequestration capacity, potentially mitigating the increase of atmospheric CO2 concentration (Zhou et al., 2016). Although the area of mangroves is relatively small, their carbon sequestration capacity per unit area is very strong. Mangroves are tropical ecosystems with high carbon sequestration capacity and high light efficiency, and are important “Blue Carbon” carbon pools. Mangrove organic carbon storage and dynamics have an important impact on global carbon balance (Ray et al., 2011; Zhu et al., 2012; Zhao et al., 2013). In addition, macroalgae may also be an important source of carbon sequestration in marine sediments and deep oceans (Krause-Jensen and Duarte, 2016; Ortega et al., 2019). Carbon sink refers to the unstable state of carbon balance in an ecosystem, in which the carbon input (the sum of plant productivity and external input carbon) is greater than the carbon output (carbon consumed by respiration and external output carbon), namely the state of carbon accumulation. Mangrove is an important carbon sink ecosystem because of its high plant productivity and soil carbon sequestration rate. Therefore, the protection and restoration of mangroves has also become an important means of addressing climate change in the marine field (Zhang et al., 2013; Chen et al., 2021).
5.2 Theoretical basis of Blue Carbon research: schools and issues
5.2.1 Calculation of carbon storage
Studies have estimated the carbon storage. For example, Li et al. (2019) conducted methodological analysis on measuring carbon sinks in mangrove, seagrasses bed and salt marsh based on the recommended methods of carbon sink measurement given by IPCC (Intergovernmental Panel on Climate Change) and clean energy mechanism measurement standards. Phang et al. (2015) evaluated the ecosystem carbon storage of mangroves, seagrass meadows, tidal flats and sandbanks in tropical intertidal zone based on field carbon storage.
Furthermore, most existing scholars are committed to measuring the total amount of carbon stored by mangroves. Currently, the research methods of carbon storage and carbon sink capacity of mangrove wetlands are based on measured methods, including conversion based on biomass (Donato et al., 2011; Peng et al., 2016), soil sampling (Xin et al., 2014), allometric equations (Bulmer et al., 2016), remote sensing inversion (Heumann, 2011), model simulation, field analysis and laboratory determination combined (Li et al., 2014; He et al., 2017), UAV imagery (Jones et al., 2020), LiDAR (Salum et al., 2020), etc.
Seagrass provides many valuable ecosystem services and requires accurate mapping of existing meadows and biomass. For example, Ha et al. (2021) detected and quantified aboveground biomass of seagrass using radar and optical satellite imagery, combined with advanced machine learning and meta-heuristic optimization techniques. Monnier et al. (2021) applied high-resolution seismic reflection technology to estimate the carbon sink of seagrass meadow.
Salt marshes are of unique importance in coastal ecosystems. Van de Broek and Govers (2019) used mid-infrared spectroscopy combined with soil remote sensing to predict the concentration and storage of organic carbon in sediments of tidal marshes. Owers et al. (2020) quantified the change of near-surface carbon storage in salt marshes using stratified sampling method to identify vegetation structure.
Macroalgal communities as donors may contribute ecologically to global Blue Carbon sequestration. However, as the fate of isolated macroalgal biomass remains unclear, some researchers have attempted to quantify the total amount of carbon stored by macroalgae. For example, Hill et al. (2015) used carbon sequestration, macroalgae distribution, abundance and productivity to estimate the total amount of carbon stored by macroalgae.
5.2.2 Influencing factors
5.2.2.1 Natural factors
(1) Climate. Climate warming and precipitation changes will have a dynamic impact on salt marsh plants and nutrients (Charles and Dukes, 2009). Continued warming will reduce the amount of carbon absorbed and transported by kelp forests, with potential consequences for the coastal carbon cycle (Pessarrodona et al., 2018). Marine heatwaves have caused significant losses of seagrass carbon stocks, the largest in the world (Arias-Ortiz et al., 2018). Sea level rise accelerates coastal zone erosion (Zhou et al., 2016). Sanders et al. (2016) pointed out that the increase of rainfall might increase the carbon storage of mangroves.
(2) Geography. Soil texture, dominant species of mangrove and biological invasions significantly affect soil organic carbon content and distribution pattern of mangrove in tropical and subtropical China (Gao et al., 2019). The carbon storage of tropical mangroves is larger than that of subtropical and temperate regions (Sanders et al., 2016).
(3) Time. The carbon storage of mangrove communities tended to increase continuously with the increase of tree age (Gao et al., 2017; Cadiz et al., 2020); There are differences in greenhouse gas emission fluxes in mangrove ecological restoration areas with different forest ages (Li et al., 2020). Tides play an important role in maintaining the carbon sink intensity of salt marsh ecosystems, and their effects on carbon exchange will vary with time scales (Wei et al., 2020a).
(4) Creatures. Chemical bonding of soil organic carbon may be the main protective mechanism of soil organic carbon stability in mangrove wetlands, which is significant to mangrove carbon sink function (Chen et al., 2017). Spartina alterniflora invasion changes the soil organic carbon pool and weakens the stability of soil organic carbon to a certain extent. Invasive species such as Spartina alterniflora threaten the coastal ecosystem of China, resulting in the disappearance of a large number of mangroves. Although some scholars have studied the effects of spartina alterniflora on Blue Carbon (Feng et al., 2015; 2016; Gao et al., 2019; Su et al., 2020; Zhang et al., 2021), the contribution and influencing mechanism of Spartina alterniflora invasion and abnormal growth of algae to soil carbon pool are not clear.
In addition to the complexity of aboveground forms, the productivity of underground biomass is an important factor affecting organic carbon storage of seagrass grasslands (Tanaya et al., 2018). Sedimentary factors are the key factors for predicting carbon storage in salt marshes (Kelleway et al., 2016). Dahl et al. (2016) believed that sediment properties were an important factor in carbon sink management evaluation of high-priority areas. Low carbon storage of meadow may directly result from sediment disturbance (Rozaimi et al., 2017).
5.2.2.2 Human factors
Due to the lack of protection, marine carbon stocks are vulnerable to human interference, leading to carbon remineralization into carbon dioxide, further exacerbating climate change. For example, the compaction of seawalls (Fan and Wang, 2017); Aquaculture pollution (Gao et al., 2019); Eutrophication (Jiang et al., 2018; Herbeck et al., 2021; Mozdzer et al., 2021); Clam fishing not only erodes historical carbon stocks that have accumulated over decades, but also endangers potential carbon stocks (Baranano et al., 2017); Reclamation of land from the sea (Weston et al., 2014); Land reclamation is the most important driving factor of salt marsh loss (Gu et al., 2018). Urbanization (Friess et al., 2016); Submarine Groundwater Discharge (SGD) (Wang et al., 2020); Ocean acidification will affect seagrass carbon sequestration capacity and integration in the future. Garrard and Beaumont (2014) emphasized that sustainable management of seagrass is crucial to avoid continuous degradation and loss of carbon sequestration capacity of seagrass. Human disturbance changes the structure and function of the ecosystem and limits the carbon sequestration function of the ecosystem (Chen et al., 2021).
5.2.3 Protection and restoration of Blue Carbon
Scholars are devoted to the protection and restoration of Blue Carbon. Mangrove conservation should be a high priority component of climate change mitigation strategies (Murdiyarso et al., 2015). In order to reduce losses, rehabilitation is the top priority of protection work (Duncan et al., 2016). Restoration Projects can sequester large amounts of additional carbon and reduce emissions. At present, the conservation and restoration measures discussed mainly include policy and legislation, restoration of abandoned ponds, positive interaction between species and payment mechanism of ecosystem services.
5.2.3.1 Policy and legislation
Rogers et al. (2016) argues that there is an urgent need to properly value ecosystem services and explicitly recognize them in policy and legislation. Friess et al. (2016) argued that many drivers of mangrove loss operate on a large scale and that policy intervention is the most effective way to solve these problems. Sidik et al. (2018) pointed to the need for legal frameworks and the involvement of stakeholders, particularly local communities, to protect mangroves and mitigate climate change. In addition, Thompson (2018) critically assessed mangrove restoration policies and initiatives from the perspective of political ecology, focusing on institutional arrangements and power dynamics. In order to solve the problems of coral degradation and ocean acidification, Mcleod et al. (2011) discussed how to modify the existing design principles of marine protected areas.
5.2.3.2 Mechanisms of payment for ecosystem services
Payment for ecosystem services (PES) schemes offer a viable approach to large-scale wetland restoration, using economic means and avoiding artificial overdevelopment. Jakovac et al. (2020) believes that the valuation of common benefits and the combination of carbon-based mechanisms and sustainable management may be feasible. Muenzel and Martino (2018) assessed the feasibility of carbon payments and ecosystem services payments to reduce livestock grazing pressure in salt marsh areas. Canning et al. (2021) recommends that wetland-based PES programs use common asset trusts to construct wetland landscape portfolios to restore wetland values and global services.
5.2.3.3 Positive interactions between species
Although positive interspecies interactions play a crucial role in coastal wetland regeneration, only a small number of wetland and mangrove restoration studies have examined their role. For example, Renzi et al. (2019) utilized species interactions, such as trophic facilitation, stress reduction and associational defenses, to facilitate coastal wetland biological reconstruction and promote coastal wetland restoration.
5.2.3.4 Abandoned pond reversion
Fan et al. (2021) explored the ecological co-cultivation of tidal water in the restoration of mangroves in abandoned shrimp ponds. Duncan et al. (2016) points out that due to the large area and suitable site conditions, the targeted restoration of abandoned fish ponds into former mangroves will strengthen ecological environment protection in coastal areas.
5.2.3.5 Community engagement
Gu et al. (2018) pointed out that the restoration, protection and management of salt marshes can be further enhanced by strengthening the participation of local communities. Ranjan (2019) believes that community participation can restore mangrove forests, gain multiple environmental benefits, and help local communities generate income through ecosystem services to increase livelihoods.
5.2.4 Development and trading of Blue Carbon projects
In recent years, Blue Carbon has been involved in carbon trading, finance and other fields, driving the protection and development of Blue Carbon ecosystem through economic leverage. The topic of Blue Carbon sink has been expanded from scientific research to the global economy.
Although Blue Carbon is beginning to be incorporated into the carbon market, it is still a relatively new concept. Blue Carbon research is not enough to understand the research methodology and development process, resulting in the development of Blue Carbon sink project constrained (Chen S. Y. et al., 2021). In order to make Blue Carbon mechanism play a reasonable role, the international mainstream approach is to moderately include Blue Carbon into the international carbon trading market. In 2011, the Blueprint for Sustainable Development of Oceans and Coastal Zones set out the goal of protecting marine ecosystems and establishing a global Blue Carbon market. The report aims to increase carbon sinks by protecting and restoring coastal wetlands for use in trade. At present, there are two types of carbon trading markets: compliance markets and voluntary markets. Compliance markets deal with mandatory emission reductions under regulations. It is driven by the need of regulated greenhouse gas emitters for quotas and offsets (Sapkota and White, 2020). In voluntary markets, compensation prices are mainly determined by supply and demand. In some cases, prices also depend on whether buyers are willing to pay more to promote environmentally sustainable carbon markets (Xia et al., 2020). Mangrove forests, for example, provide many benefits for human welfare, but they are rapidly disappearing. One reason for this disruption is that policymakers and markets have not understandably evaluated the benefits of mangroves (Tanner et al., 2019). Using the value of carbon stored in mangroves on the voluntary carbon market can generate revenue. This helps support and motivate sustainable mangrove management, improve livelihoods and reduce anthropogenic stress (Benson et al., 2017).
Optimizing Blue Carbon sequestration products and developing trading mechanisms are important ways for China and the world to deal with global climate change and achieve carbon neutrality. So far, China has successfully developed and traded its first Blue Carbon sequestration project, the Zhanjiang Mangrove Forest Project. In the future, it will continue to promote the development of Blue Carbon sink projects and the development of trading market (Chen et al., 2021), to promote ecological protection and restoration through market mechanism (Tang et al., 2018).
5.3 Research methods of Blue Carbon
Blue Carbon research mainly adopts quantitative research method. Referring to Tang et al. (2018), quantitative research methods used in Blue Carbon research can be divided into monitoring carbon budget (including carbon flux and carbon pool), simulation experiment and modeling research, etc.
5.3.1 Monitoring
Monitoring can be divided into flux monitoring and carbon pool monitoring. First, the monitoring of carbon flux. It is to observe all kinds of fluxes and calculate the total amount. For example, Dahl et al. (2020) measured total organic carbon (TOC) deposited in seagrass meadows at two Z-dock sites to assess seasonal variations in sediment carbon storage of seagrass meadows. Potouroglou et al. (2017) conducted a multi-site study on sediment surface elevation of seagrass in different environments and species through long-term monitoring. Macklin et al. (2019) explored the driving factors of CO2 along the mangrove seagrass transect through 8 seasonal surveys and 55 h fixed location time series. This method——the monitoring of carbon flux——can understand the mechanism and process of carbon pool changes and provide data basis for modeling. For example, using more than 900 soil carbon measurements data, Jardine and Siikamaeki (2014) established a global prediction model for mangrove soil carbon.
Second, the monitoring of carbon pools. It involves directly observing changes in the carbon pool. This method is relatively simple and can obtain the changes of 1 year or several years, but fails to give seasonal changes and the contribution of each carbon flux. For example, Peng et al. (2016) calculated the vegetation carbon storage of each community by investigating the components of each vegetation carbon pool in four representative mangrove communities.
5.3.2 Experiment
Experiment is to change one or more environmental factors artificially through the comparison with the control group, explore the response of the ecosystem to this kind of factors, and serve the construction of the model. In the existing studies, the simulated factors include temperature, precipitation, nitrogen and phosphorus content, soil oxygen content, etc. For example, in order to further understand how salt marshes respond to warming and precipitation changes, Charles and Dukes (2009) conducted a manipulation experiment in tidal salt marshes through passive open indoor warming, double precipitation and warming, etc. Deegan et al. (2012) conducted a 9-year experiment on nutrient enrichment across the ecosystem in coastal wetlands, which showed that nutrient enrichment is a global problem in coastal ecosystems, and it may be also a driving factor of salt marsh loss. Using nutrient enrichment (N & P) experiments, Armitage and Fourqurean (2016) assessed the effects of long-term and near-term nutrient inputs on aboveground and underground carbon accumulation in seagrass beds. Wei et al. (2020b) explored the effects of tidal flood on CO2 and CH4 fluxes of salt marsh ecosystem through field experiments. Chapman et al. (2019) points out that changes in soil oxygen content may be a vital driving factor for Blue Carbon storage in coastal wetlands in the future by manipulating soil oxygen state in real soil.
5.3.3 Modeling
The purpose of the long-term observation and simulation study of the ecosystem in Blue Carbon research is to build a process-based mathematical model to understand the system process and predict and analyze the future. Most models use input parameters that are easy to measure, such as meteorological factors and vegetation structure, and other data obtained with remote sensing data. For example, Morris et al. (2016) used marsh equilibrium model to predict the carbon sequestration capacity of salt marsh wetlands and their response to sea-level rise. Owers et al. (2018) collects high-resolution three-dimensional point cloud through terrestrial laser scanning and proposes the point cloud elevation histogram model. So, the aboveground biomass of coastal wetland vegetation with complex structures can be quantified. Sohma et al. (2018) established a coastal ecosystem model (Ecosystem Model for Aquatic Integrated Network for Blue Carbon) to estimate climate change mitigation. In addition, Jardine and Siikamaeki (2014) explored several predictive modeling methods using climate and location data for prediction. Based on these models, an estimated global soil carbon concentration and storage dataset was constructed.
6 Conclusion and prospects
The potential impact of Blue Carbon destruction on greenhouse gas emissions is becoming more significant, which puts forward a new proposition and research space. Based on previous research, this paper tries to put forward some research perspectives, in order to provide some references for scholars to carry out Blue Carbon research.
(1) Analyze the concept and content of Blue Carbon and clarify the type of Blue Carbon. Although scholars at home and abroad have tentatively proposed and summarized the concept, connotation and types of Blue Carbon, the research on Blue Carbon is still in its infancy, and human cognition of Blue Carbon is still not comprehensive. As natural science advances and theories change, some new ecosystems may be incorporated into the concept of Blue Carbon. For example, the discovery of shale gas has shattered our understanding of the composition of fossil energy. Therefore, the related concepts, connotations and main types of Blue Carbon will be constantly updated with the progress of natural science and the innovation of social science theories.
(2) Strengthen accurate estimation and prediction of Blue Carbon sink and its potential. Although relevant measurement methods and standards have been preliminarily proposed, they are still incomplete due to the many subsystems involved in Blue Carbon. Therefore, in the next step, we should constantly develop measurement methods and formulate corresponding industry standards to improve the accuracy of Blue Carbon storage measurement and simulation model. Although the carbon storage of Blue Carbon in China has been estimated successively, there is a lack of systematic observation and unified standard for the carbon storage and carbon sink research of coastal wetlands in China. Therefore, there is still a large data gap in existing estimates, and the storage of Blue Carbon sink needs to be further explored.
(3) Explore the protective mechanism of Blue Carbon system. With the continuous advancement of urbanization and industrialization in China, offshore pollution is becoming more serious. Moreover, maricultural pollution and domestic sewage pollution in the primary industry also aggravate marine pollution in China, which will cause serious damage to the Blue Carbon ecosystem in the long run. Therefore, strengthening the research on the protection mechanism of the Blue Carbon system should also become one of the significant directions.
(4) Explore the Blue Carbon sink trading market mechanism. For instance, in China Guangdong, Shandong and Shanghai have taken the lead in carrying out multi-agent Blue Carbon trading projects, but the overall benefits of the projects have yet to be tested over time. In the future, the government can effectively integrate the supply and demand of Blue Carbon by developing Blue Carbon fund, exploring Blue Carbon credit certification and constructing Blue Carbon free trading market, to serve China’s carbon neutrality strategy in 2060.
(5) Explore new research methods and focus on the dynamic change of Blue Carbon. As for the existing quantitative research methods, although specific quantitative process can be understood to a certain extent in terms of monitoring, there will be certain errors. As far as the experiment is concerned, the cost of simulation experiment is relatively high and the effect is not ideal. As far as modeling is concerned, the prediction ability of existing models needs to be further studied. Future research can consider using big data, artificial intelligence, cloud computing and other high-end core technologies to capture marine activities, paying attention to the complex development process of Blue Carbon, and using big data to conduct in-depth research and dig financial markets to explore Blue Carbon and its economic effects more effectively.
Author contributions
LZ: Conceptualization, Formal Analysis, Methodology, Project administration, Resources, Software, Writing–original draft, Writing–review and editing.
Funding
The author(s) declare that no financial support was received for the research, authorship, and/or publication of this article.
Acknowledgments
During the creative stage, Dr. Yang Chen and Dr. Wang Xilong have provided operation method, Dr. Wang Xilong has given me instructions on Citespace software, and Prof. Wu Yigen has made several academic discussions with me to help improve my manuscript.
Conflict of interest
The author declares that the research was conducted in the absence of any commercial or financial relationships that could be construed as a potential conflict of interest.
Publisher’s note
All claims expressed in this article are solely those of the authors and do not necessarily represent those of their affiliated organizations, or those of the publisher, the editors and the reviewers. Any product that may be evaluated in this article, or claim that may be made by its manufacturer, is not guaranteed or endorsed by the publisher.
References
Arias-Ortiz, A., Serrano, O., Masque, P., Lavery, P. S., Mueller, U., Kendrick, G. A., et al. (2018). A marine heatwave drives massive losses from the world's largest seagrass carbon stocks. Nat. Clim. Chang. 8 (4), 338–344. doi:10.1038/s41558-018-0096-y
Armitage, A. R., and Fourqurean, J. W. (2016). Carbon storage in seagrass soils: long-term nutrient history exceeds the effects of near-term nutrient enrichment. Biogeosciences 13 (1), 313–321. doi:10.5194/bg-13-313-2016
Baranano, C., Fernandez, E., and Mendez, G. (2017). Clam harvesting decreases the sedimentary carbon stock of a Zostera marina meadow. Aquat. Bot. 146, 48–57. doi:10.1016/j.aquabot.2017.12.002
Benson, L., Glass, L., Jones, T. G., Ravaoarinorotsihoarana, L., and Rakotomahazo, C. (2017). Mangrove carbon stocks and ecosystem cover dynamics in Southwest Madagascar and the implications for local management. Forests 8 (6), 190. doi:10.3390/f8060190
Bulmer, R. H., Schwendenmann, L., and Lundquist, C. J. (2016). Allometric models for estimating aboveground biomass, carbon and nitrogen stocks in temperate avicennia marina forests. Wetlands 36 (5), 841–848. doi:10.1007/s13157-016-0793-0
Cadiz, P. L., Calumpong, H. P., Sinutok, S., and Chotikarn, P. (2020). Carbon storage potential of natural and planted mangals in Trang, Thailand. Appl. Ecol. Environ. Res. 18 (3), 4383–4403. doi:10.15666/aeer/1803_43834403
Canning, A. D., Jarvis, D., Costanza, R., Hasan, S., Smart, J. C. R., Finisdore, J., et al. (2021). Financial incentives for large-scale wetland restoration: beyond markets to common asset trusts. One Earth 4 (7), 937–950. doi:10.1016/j.oneear.2021.06.006
Chapman, S. K., Hayes, M. A., Kelly, B., and Langley, J. A. (2019). Exploring the oxygen sensitivity of wetland soil carbon mineralization. Biol. Lett. 15 (1), 20180407. doi:10.1098/rsbl.2018.0407
Charles, H., and Dukes, J. S. (2009). Effects of warming and altered precipitation on plant and nutrient dynamics of a New England salt marsh. Ecol. Appl. 19 (7), 1758–1773. doi:10.1890/08-0172.1
Chen, C. (2017). Science mapping: a systematic review of the literature. J. Data Inf. Sci. 2 (2), 1–40. doi:10.1515/jdis-2017-0006
Chen, G. C., Wang, J., Xu, F. H., Yang, Y. X. Y., Fan, M., Huang, S. F., et al. (2021a). Progress of coastal wetland blue carbon projects in carbon market and advice on facilitating the development of blue carbon projects in China. J. App. Oceanogr., 1–8. doi:10.3969/J.ISSN.2095-4972.2022.02.001
Chen, G. X., Gao, D. Z., Chen, G., Zeng, C. S., and Wang, W. Q. (2017). Effects of spartina alterniflora invasion on soil carbon fractions in mangrove wetlands of China. J. Soil Water Conserv. 31 (6), 249–256. doi:10.13870/j.cnki.stbcxb.2017.06.039
Chen, L. Z., Pan, L. H., and Qiu, G. L. (2021b). Coastal blue carbon sink in China under the influence of human activity. J. Guangxi Acad. Sci. 37 (3), 186–194. doi:10.13657/j.cnki.gxkxyxb.20210927.006
Chen, S. Y., An, W. S., Chen, B., and Chen, G. C. (2021c). Decisive factors impacting the carbon sequestration in mangrove ecological restoration. J. App. Oceanogr. 40 (01), 34–42. doi:10.3969/J.ISSN.2095-4972.2021.01.004
Chen, Y., Chen, C. M., and Liu, Z. Y. (2015). The methodology function of Cite Space mapping Knowledge domains. Stud. Sci. Sci. 33 (2), 242–253. doi:10.16192/j.cnki.1003-2053.2015.02.009
Dahl, M., Asplund, M. E., Deyanova, D., Franco, J. N., Koliji, A., Infantes, E., et al. (2020). High seasonal variability in sediment carbon stocks of cold-temperate seagrass meadows. J. Geophys. Res. Biogeosci. 125 (1). doi:10.1029/2019JG005430
Dahl, M., Deyanova, D., Gütschow, S., Asplund, M. E., Lyimo, L. D., Karamfilov, V., et al. (2016). Sediment properties as important predictors of carbon storage in Zostera marina meadows: a comparison of four European areas. Plos One 11 (12), e0167493. doi:10.1371/journal.pone.0167493
Deegan, L. A., Johnson, D. S., Warren, R. S., Peterson, B. J., Fleeger, J. W., Fagherazzi, S., et al. (2012). Coastal eutrophication as a driver of salt marsh loss. Nature 490 (7420), 388–392. doi:10.1038/nature11533
Donato, D. C., Kauffman, J. B., Murdiyarso, D., Kurnianto, S., Stidham, M., and Kanninen, M. (2011). Mangroves among the most carbon-rich forests in the tropics. Nat. Geosci. 4 (5), 293–297. doi:10.1038/ngeo1123
Duncan, C., Primavera, J. H., Pettorelli, N., Thompson, J. R., Loma, R. J. A., and Koldewey, H. J. (2016). Rehabilitating mangrove ecosystem services: a case study on the relative benefits of abandoned pond reversion from Panay Island, Philippines. Mar. Pollut. Bull. 109 (2), 772–782. doi:10.1016/j.marpolbul.2016.05.049
Fan, H. Q., and Wang, W. Q. (2017). Some thematic issues in mangrove conservation in China. J. Xiamen Univ. Nat. Sci. 56 (3), 323–330. doi:10.6043/j.issn.0438-0479.201612003
Fan, H. Q., Zhong, Y. X., Sue, Z. N., Luo, Y., Ni, K. P., and Wang, X. (2021). An exploratory study on the tide driving eco-polyculture in an abandoned shrimp pond with mangrove restoration in Guangxi, China. J. Guangxi Acad. Sci. 37 (3), 195–203. doi:10.13657/j.cnki.gxkxyxb.20210927.004
Feng, Z. X., Gao, J. H., Chen, L., Wang, Y. P., Gao, J. H., and Bai, F. L. (2015). The response of organic carbon content to biomass dynamics in spartina alterniflora marsh. Acta Ecol. Sin. 35 (07), 2038–2047. doi:10.5846/stxb201404090685
Feng, Z. X., Gao, J. H., Chen, L., Wang, Y. P., Gao, J. H., and Bai, F. L. (2016). Impact of spartina alterniflora biomass variation on content and sources of organic carbon fractions in salt marsh: a case study of tidal flat in Wanggang Estuary, Jiangsu Province. Geochimica 45 (1), 87–97. doi:10.19700/j.0379-1726.2016.01.007
Friess, D. A., Richards, D. R., and Phang, V. X. H. (2016). Mangrove forests store high densities of carbon across the tropical urban landscape of Singapore. Urban Ecosyst. 19 (2), 795–810. doi:10.1007/s11252-015-0511-3
Gao, T. L., Guan, W., Mao, J., Jiang, Z. M., and Liao, B. W. (2017). Carbon storage and influence factors of major mangrove communities Fucheng, Leizhou Peninsula, Guangdong Province. Ecol. Environ. Sci. 26 (06), 985–990. doi:10.16258/j.cnki.1674-5906.2017.06.011
Gao, Y., Zhou, J., Wang, L., Guo, J., Feng, J., Wu, H., et al. (2019). Distribution patterns and controlling factors for the soil organic carbon in four mangrove forests of China. Glob. Ecol. Conserv. 17, e00575. doi:10.1016/j.gecco.2019.e00575
Garrard, S. L., and Beaumont, N. J. (2014). The effect of ocean acidification on carbon storage and sequestration in seagrass beds; a global and UK context. Mar. Pollut. Bull. 86 (1-2), 138–146. doi:10.1016/j.marpolbul.2014.07.032
Gu, J. L., Luo, M., Zhang, X. J., Christakos, G., Agusti, S., Duarte, C. M., et al. (2018). Losses of salt marsh in China: trends, threats and management. Estuar. Coast. Shelf Sci. 214, 98–109. doi:10.1016/j.ecss.2018.09.015
Ha, N. T., Manley-Harris, M., Pham, T. D., and Hawes, I. (2021). The use of radar and optical satellite imagery combined with advanced machine learning and metaheuristic optimization techniques to detect and quantify above ground biomass of intertidal seagrass in a New Zealand estuary. Int. J. Remote Sens. 42 (12), 4712–4738. doi:10.1080/01431161.2021.1899335
He, Q. F., Zheng, W., Huang, X. R., Liu, X., Shen, W. H., and He, F. (2017). Carbon storage and distribution of mangroves at Qinzhou Bay. Guangxi. J. Cent. South Univ. For. Technol. 37 (11), 121–126. doi:10.14067/j.cnki.1673-923x.2017.11.020
Herbeck, L. S., Krumme, U., Nordhaus, I., and Jennerjahn, T. C. (2021). Pond aquaculture effluents feed an anthropogenic nitrogen loop in a SE Asian estuary. Sci. Total Environ. 756, 144083. doi:10.1016/j.scitotenv.2020.144083
Hill, R., Bellgrove, A., Macreadie, P. I., Petrou, K., John, B., Steven, A., et al. (2015). Can macroalgae contribute to blue carbon? An Australian perspective. Limnol. Oceanogr. 60 (5), 1689–1706. doi:10.1002/lno.10128
Jakovac, C. C., Latawiec, A. E., Lacerda, E., Lucas, I. L., Korys, K. A., Iriba Rr Em, A., et al. (2020). Costs and carbon benefits of mangrove conservation and restoration: a global analysis. Ecol. Econ. 176, 106758. doi:10.1016/j.ecolecon.2020.106758
Jardine, S. L., and Siikamaeki, J. V. (2014). A global predictive model of carbon in mangrove soils. Environ. Res. Lett. 9 (10), 104013. doi:10.1088/1748-9326/9/10/104013
Jiang, Z., Liu, S., Zhang, J., Wu, Y., Zhao, C., Lian, Z., et al. (2018). Eutrophication indirectly reduced carbon sequestration in a tropical seagrass bed. Plant Soil 426 (1-2), 135–152. doi:10.1007/s11104-018-3604-y
Jones, A. R., Segaran, R. R., Clarke, K. D., Waycott, M., Goh, W. S. H., and Gillanders, B. M. (2020). Estimating mangrove tree biomass and carbon content: a comparison of forest inventory techniques and drone imagery. Front. Mar. Sci. 6, 6. doi:10.3389/fmars.2019.00784
Kelleway, J. J., Saintilan, N., Macreadie, P. I., and Ralph, P. J. (2016). Sedimentary factors are key predictors of carbon storage in SE Australian saltmarshes. Ecosystems 19 (5), 865–880. doi:10.1007/s10021-016-9972-3
Krause-Jensen, D., and Duarte, C. M. (2016). Substantial role of macroalgae in marine carbon sequestration. Nat. Geosci. 9 (10), 737–742. doi:10.1038/NGEO2790
Lee, H. Z. L., Davies, I. M., Baxter, J. M., Diele, K., and Sanderson, W. G. (2020). Missing the full story: first estimates of carbon deposition rates for the European flat oyster, <i>Ostrea edulis</i>. Ostrea Edulis. Aquat. Conserv. 30 (11), 2076–2086. doi:10.1002/aqc.3402
Li, J., Liu, Y. M., Sun, F., Huang, J. T., and Lu, J. F. (2019). Analysis of blue carbon in China's coastal zone. Environ. Sci. Technol. 42 (10), 207–216. doi:10.19672/j.cnki.1003-6504.2019.10.031
Li, M. F., Wang, M. T., Qi, H. H., and Qi, Y. Y. (2018). Evolution of international research on science and technology policy. Stud. Sci. Sci. 36 (09), 1565–1574. doi:10.16192/j.cnki.1003-2053.2018.09.005
Li, N., Chen, P. M., Qiao, P. P., and Qin, C. X. (2014). Density, storage and distribution in mangrove ecosystem. Guangdong Agric. Sci. 41 (16), 161–164. doi:10.16768/j.issn.1004-874x.2014.16.036
Li, S., Cai, H. C., Chen, W. D., Lin, L., Ni, X. P., Wu, E. W., et al. (2020). Analysis on CH4 and CO2 of mangroves with different ages in the coastal ecological restoration zone. Ecol. Environ. Sci. 29 (12), 2414–2422. doi:10.16258/j.cnki.1674-5906.2020.12.012
Macklin, P. A., Suryaputra, I. G. N. A., Maher, D. T., Murdiyarso, D., and Santos, I. R. (2019). Drivers of CO2 along a mangrove-seagrass transect in a tropical bay: delayed groundwater seepage and seagrass uptake. Cont. Shelf Res. 172, 57–67. doi:10.1016/j.csr.2018.10.008
Mcleod, E., Chmura, G. L., Bouillon, S., Salm, R., Bjork, M., Duarte, C. M., et al. (2011). A blueprint for blue carbon: toward an improved understanding of the role of vegetated coastal habitats in sequestering CO2. Front. Ecol. Environ. 9 (10), 552–560. doi:10.1890/110004
Monnier, B., Pergent, G., Mateo, M. N., Carbonell, R., Clabaut, P., and Pergent-Martini, C. (2021). Sizing the carbon sink associated with Posidonia oceanica seagrass meadows using very high-resolution seismic reflection imaging. Mar. Environ. Res. 170, 105415. doi:10.1016/j.marenvres.2021.105415
Morris, J. T., Barber, D. C., Callaway, J. C., Chambers, R., Hagen, S. C., Hopkinson, C. S., et al. (2016). Contributions of organic and inorganic matter to sediment volume and accretion in tidal wetlands at steady state. Earths Future 4 (4), 110–121. doi:10.1002/2015EF000334
Mozdzer, T. J., Drew, S. E., Caplan, J. S., Weber, P. E., and Deegan, L. A. (2021). Rapid recovery of carbon cycle processes after the cessation of chronic nutrient enrichment. Sci. Total Environ. 750, 140927. doi:10.1016/j.scitotenv.2020.140927
Muenzel, D., and Martino, S. (2018). Assessing the feasibility of carbon payments and payments for ecosystem services to reduce livestock grazing pressure on saltmarshes. J. Environ. Manag. 225, 46–61. doi:10.1016/j.jenvman.2018.07.060
Murdiyarso, D., Purbopuspito, J., Kauffman, J. B., Warren, M. W., Sasmito, S. D., Donato, D. C., et al. (2015). The potential of Indonesian mangrove forests for global climate change mitigation. Nat. Clim. Chang. 12 (5), 1089–1092. doi:10.1038/nclimate2734
Nellemann, C., Corcoran, E., Duarte, C., Valdes, L., and Grimsditch, G. (2009). Blue carbon: the role of healthy oceans in binding carbon. United Nations Environment Programme. Grid-Arendal.
Ortega, A., Geraldi, N. R., Alam, I., Kamau, A. A., Acinas, S. G., Logares, R., et al. (2019). Important contribution of macroalgae to oceanic carbon sequestration. Nat. Geosci. 12 (9), 748–754. doi:10.1038/s41561-019-0421-8
Owers, C. J., Rogers, K., Mazumder, D., and Woodroffe, C. D. (2020). Temperate coastal wetland near-surface carbon storage: spatial patterns and variability. Estuar. Coast. Shelf Sci. 235, 106584. doi:10.1016/j.ecss.2020.106584
Owers, C. J., Rogers, K., and Woodroffe, C. D. (2018). Terrestrial laser scanning to quantify above-ground biomass of structurally complex coastal wetland vegetation. Estuar. Coast. Shelf Sci. 204, 164–176. doi:10.1016/j.ecss.2018.02.027
Pedersen, M. F., Filbee-Dexter, K., Frisk, N. L., Sarossy, Z., and Wernberg, T. (2021). Carbon sequestration potential increased by incomplete anaerobic decomposition of kelp detritus. Mar. Ecol. Prog. Ser. 660, 53–67. doi:10.3354/meps13613
Peng, C. J., Qian, J. H., Guo, X. D., Zhao, H. W., Hu, N. X., Yang, Q., et al. (2016). Vegetation carbon stocks and net primary productivity of the mangrove forests in Shenzhen, China. Chin. J. App. Ecol. 27 (07), 2059–2065. doi:10.13287/j.1001-9332.201607.029
Pessarrodona, A., Moore, P. J., Sayer, M. D. J., and Smale, D. A. (2018). Carbon assimilation and transfer through kelp forests in the NE Atlantic is diminished under a warmer ocean climate. Glob. Chang. Biol. 24 (9), 4386–4398. doi:10.1111/gcb.14303
Phang, V., Chou, L. M., and Friess, D. A. (2015). Ecosystem carbon stocks across a tropical intertidal habitat mosaic of mangrove forest, seagrass meadow, mudflat and sandbar. Earth Surf. Process Landf. 40 (10), 1387–1400. doi:10.1002/esp.3745
Potouroglou, M., Bull, J. C., Krauss, K. W., Kennedy, H. A., Fusi, M., Daffonchio, D., et al. (2017). Measuring the role of seagrasses in regulating sediment surface elevation. Sci. Rep. 7 (1), 11917. doi:10.1038/s41598-017-12354-y
Ranjan, R. (2019). Optimal mangrove restoration through community engagement on coastal lands facing climatic risks: the case of Sundarbans region in India. Land use policy 81, 736–749. doi:10.1016/j.landusepol.2018.11.047
Ray, R., Ganguly, D., Chowdhury, C., Dey, M., Das, S., Dutta, M. K., et al. (2011). Carbon sequestration and annual increase of carbon stock in a mangrove forest. Atmos. Environ. 45 (28), 5016–5024. doi:10.1016/j.atmosenv.2011.04.074
Renzi, J. J., He, Q., and Silliman, B. R. (2019). Harnessing positive species interactions to enhance coastal wetland restoration. Front. Ecol. Evol. 7. doi:10.3389/fevo.2019.00131
Rogers, K., Boon, P. I., Branigan, S., Duke, N. C., Field, C. D., Fitzsimons, J. A., et al. (2016). The state of legislation and policy protecting Australia's mangrove and salt marsh and their ecosystem services. Mar. Policy. 72, 139–155. doi:10.1016/j.marpol.2016.06.025
Rogers, K., Kelleway, J. J., Saintilan, N., Megonigal, J. P., Adams, J. B., Holmquist, J. R., et al. (2019). Wetland carbon storage controlled by millennial-scale variation in relative sea-level rise. Nature 567 (7746), 91–95. doi:10.1038/s41586-019-0951-7
Rozaimi, M., Fairoz, M., Hakimi, T. M., Hamdan, N. H., Omar, R., Ali, M. M., et al. (2017). Carbon stores from a tropical seagrass meadow in the midst of anthropogenic disturbance. Mar. Pollut. Bull. 119 (2), 253–260. doi:10.1016/j.marpolbul.2017.03.073
Salum, R. B., Souza-Filho, P. W. M., Simard, M., Silva, C. A., Fernandes, M. E. B., Cougo, M. F., et al. (2020). Improving mangrove above-ground biomass estimates using LiDAR. Estuar. Coast. Shelf Sci. 236, 106585. doi:10.1016/j.ecss.2020.106585
Sanders, C. J., Maher, D. T., Tait, D. R., Williams, D., Holloway, C., Sippo, J. Z., et al. (2016). Are global mangrove carbon stocks driven by rainfall? J. Geophys. Res. Biogeosci. 121 (10), 2600–2609. doi:10.1002/2016JG003510
Sapkota, Y., and White, J. R. (2020). Carbon offset market methodologies applicable for coastal wetland restoration and conservation in the United States: a review. Sci. Total Environ. 701, 134497. doi:10.1016/j.scitotenv.2019.134497
Sidik, F., Supriyanto, B., Krisnawati, H., and Muttaqin, M. Z. (2018). Mangrove conservation for climate change mitigation in Indonesia. Wiley Interdiscip. Rev. Clim. Chang. 9 (5). doi:10.1002/wcc.529
Sohma, A., Shibuki, H., Nakajima, F., Kubo, A., and Kuwae, T. (2018). Modeling a coastal ecosystem to estimate climate change mitigation and a model demonstration in Tokyo Bay. Ecol. Modell. 384, 261–289. doi:10.1016/j.ecolmodel.2018.04.019
Su, Z. N., Qiu, G. L., Fan, H. Q., Li, M., and Fang, C. (2020). Changes in carbon storage and macrobenthic communities in a mangrove-seagrass ecosystem after the invasion of smooth cordgrass in southern China. Mar. Pollut. Bull. 152, 110887. doi:10.1016/j.marpolbul.2020.110887
Tanaya, T., Watanabe, K., Yamamoto, S., Hongo, C., Kayanne, H., and Kuwae, T. (2018). Contributions of the direct supply of belowground seagrass detritus and trapping of suspended organic matter to the sedimentary organic carbon stock in seagrass meadows. Biogeosciences 15 (13), 4033–4045. doi:10.5194/bg-15-4033-2018
Tang, J. W., Ye, S. F., Chen, X. C., Yang, H. L., Sun, X. H., Wang, F. M., et al. (2018). Coastal blue carbon: concept, study method, and the application to ecological restoration. Sci. Sin. Terr. 48 (06), 661–670. doi:10.1360/N072017-00341
Tanner, M. K., Moity, N., Costa, M. T., Marin Jarrin, J. R., Aburto-Oropeza, O., and Salinas-De-Leon, P. (2019). Mangroves in the Galapagos: ecosystem services and their valuation. Ecol. Econ. 160 (JUN), 12–24. doi:10.1016/j.ecolecon.2019.01.024
Thompson, B. S. (2018). The political ecology of mangrove forest restoration in Thailand: institutional arrangements and power dynamics. Land use policy 78, 503–514. doi:10.1016/j.landusepol.2018.07.016
Trevathan-Tackett, S. M., Thomson, A., Ralph, P. J., and Macreadie, P. I. (2018). Fresh carbon inputs to seagrass sediments induce variable microbial priming responses. Sci. Total Environ. 621, 663–669. doi:10.1016/j.scitotenv.2017.11.193
Van de Broek, M., and Govers, G. (2019). Quantification of organic carbon concentrations and stocks of tidal marsh sediments via mid-infrared spectroscopy. Geoderam 337, 555–564. doi:10.1016/j.geoderma.2018.09.051
Wang, Y. L., Zhang, F. F., Chen, X. G., Li, L. W., Wang, X. L., Lao, Y. L., et al. (2020). Influence of submarine groundwater discharge in the blue carbon budget of typical mangrove: a case study from the Zhenzhu Bay, Guangxi. Acta Ecol. Sin. 42 (10), 37–46. doi:10.3969/j.issn.0253-4139.2020.10.004
Watanabe, K., Yoshida, G., Hori, M., Umezawa, Y., Moki, H., and Kuwae, T. (2020). Macroalgal metabolism and lateral carbon flows can create significant carbon sinks. Biogeosciences 17 (9), 2425–2440. doi:10.5194/bg-17-2425-2020
Wei, S. Y., Han, G. X., Chu, X. J., Song, W. M., He, W. J., Xia, J. Y., et al. (2020a). Effect of tidal flooding on ecosystem CO2 and CH4 fluxes in a salt marsh in the Yellow River Delta. Estuar. Coast. Shelf Sci. 232, 106512. doi:10.1016/j.ecss.2019.106512
Wei, S. Y., Han, G. X., Jia, X., Song, W. M., Xia, J. Y., Wu, H. T., et al. (2020b). Tidal effects on ecosystem CO2 exchange at multiple timescales in a salt marsh in the Yellow River Delta. Estuar. Coast. Shelf Sci. 238, 106727. doi:10.1016/j.ecss.2020.106727
Weston, N. B., Neubauer, S. C., Velinsky, D. J., and Vile, M. A. (2014). Net ecosystem carbon exchange and the greenhouse gas balance of tidal marshes along an estuarine salinity gradient. Biogeochemistry 120 (1-3), 163–189. doi:10.1007/s10533-014-9989-7
Xia, X. Q., Li, C. Y., and Zhu, Q. H. (2020). Game analysis for the impact of carbon trading on low-carbon supply chain. J. Clean. Prod. 276, 123220. doi:10.1016/j.jclepro.2020.123220
Xin, K., Yan, K., Li, Z., Hu, J. L., and Qiu, M. H. (2014). Distribution of soil organic carbon in mangrove wetland of Hainan Island and its influencing factors. Acta Pedol. Sin. 51 (05), 1078–1086. CNKI:SUN:TRXB.0.2014-05-017.
Zhang, G., Bai, J., Zhao, Q., Jia, J., Wang, X., Wang, W., et al. (2021). Soil carbon storage and carbon sources under different Spartina alterniflora invasion periods in a salt marsh ecosystem. Catena 196, 104831. doi:10.1016/j.catena.2020.104831
Zhang, L., Guo, Z. H., and Li, Z. Y. (2013). Carbon storage and carbon sink of mangrove wetland: research progress. Chin. J. App. Ecol. 24 (4), 1153–1159. doi:10.13287/j.1001-9332.2013.0272
Zhao, M. Q., Chen, K. L., and Shi, Y. F. (2013). Analysis of the carbon storage and fixation of mangrove forests in Sanya. J. Hainan Trop. Ocean. Univ. 20 (05), 85–88. doi:10.13307/j.issn.1008-6722.2013.05.007
Zhou, C. H., Mao, Q. Y., Xu, X., Fang, C. M., Luo, Y. M., and Li, B. (2016). Preliminary analysis of C sequestration potential of blue carbon ecosystems on Chinese coastal zone. Sci. Chin. Life Sci. 46 (4), 475–486. doi:10.1360/n052016-00105
Keywords: Blue Carbon, frontier evolution, analysis framework, knowledge map, double carbon goal, scientific measurement
Citation: Zhang L (2024) Environmental hotspots, frontiers and analytical framework of Blue Carbon research: a quantitative analysis of knowledge map based on CiteSpace. Front. Environ. Sci. 12:1387655. doi: 10.3389/fenvs.2024.1387655
Received: 18 February 2024; Accepted: 07 June 2024;
Published: 25 June 2024.
Edited by:
Martin Siegert, University of Exeter, United KingdomReviewed by:
Xiaoyang Zhao, Huaqiao University, ChinaAsmaa Hassan Mohammed, National Authority for Remote Sensing and Space Sciences, Egypt
Copyright © 2024 Zhang. This is an open-access article distributed under the terms of the Creative Commons Attribution License (CC BY). The use, distribution or reproduction in other forums is permitted, provided the original author(s) and the copyright owner(s) are credited and that the original publication in this journal is cited, in accordance with accepted academic practice. No use, distribution or reproduction is permitted which does not comply with these terms.
*Correspondence: Lipai Zhang, cHp5ejA5MDdAMTI2LmNvbQ==