- 1Department of Zoology, College of Science, King Saud University, Riyadh, Saudi Arabia
- 2School of Engineering and Built Environment, Griffith University, Nathan Campus, Nathan, QLD, Australia
- 3Department of Fisheries and Marine Science, Noakhali Science and Technology University, Sonapur, Bangladesh
- 4Laboratory of Environmental Health and Ecotoxicology, Department of Environmental Sciences, Jahangirnagar University, Dhaka, Bangladesh
- 5Environmental and Life Sciences Programme, Faculty of Science, Universiti Brunei Darussalam, Gadong, Brunei Darussalam
- 6College of Forestry and Grass, College of Soil and Water Conservation, Nanjing Forestry University, Nanjing, Jiangsu, China
Groundwater (GW) plays a crucial role as the primary source of potable water in numerous developing nations. However, the increasing presence of microplastics (MPs) represents a concealed danger, threatening activities that guarantee safe drinking water in these countries. Despite this, there is limited awareness about the widespread existence of MPs in these regions of the world. In this study, we investigated the contamination levels, distribution, morphological characteristics, chemical composition, and associated public health risk of MPs in groundwater samples from six coastal districts of Bangladesh using stereomicroscopy and Fourier transform infrared spectroscopy (FTIR). MPs were prevalent at all sampling stations, with average concentrations of 24.63 ± 16.13 items/L and a range of 4–75 items/L. The majority of MPs (97.6%) were fibers, indicating a likely origin from fabric disintegration during drying, washing, discarded fishing nets, personal care items, and municipal waste. Smaller-sized MPs (<0.5 mm), transparent and violet-colored, were predominantly observed. Additionally, FTIR confirmed the presence of plastic polymers, including low-density polyethylene (LDPE), high-density polyethylene (HDPE), ethylene vinyl acetate (EVA), and polyethylene terephthalate (PETE). Despite the high visibility observed or focus given for PETE, PE was found to be abundant. These are thought to have come from runoff from agricultural fields, seeping from landfills where plastic trash is disposed of, synthetic fabrics, and inappropriate plastic product disposal. Estimated dietary intake was recorded as 1.85 particles/person/day (i.e., 675 particles/year), suggesting the possible health risk to consumers. Hazard analyses based on the polymeric risk score revealed diverse risks associated with polymer levels, with PETE indicating a medium risk and other polymers, e.g., HDPE, LDPE, and EVA at 39%, 31%, and 17%, respectively, showing a minor risk to human health from drinking water from the studied sources. This is the first study from the regions, and the findings will be valuable for identifying sources, implementing control measures, shaping policies, and safeguarding public health against plastic pollution.
1 Introduction
Groundwater (GW) is critical for ecosystem sustainability, serving as a vital source of drinking water for communities, supporting agriculture, and contributing to a balanced global water cycle (Re, 2019). Over two billion people around the world rely on GW for various purposes such as drinking, household activities, and industrial applications (Re, 2019). Poor people of underdeveloped countries largely depend on GW as a source of potable water. However, the wellbeing of this substantial global population may be at risk due to the drinking water sourced from GW being contaminated. This significant source of freshwater is getting corroded with numerous harmful substances including microplastics (MPs; <5 mm in size) and heavy metals (Panno et al., 2019a; Plastics Europe, 2021; Su et al., 2021; Jiang et al., 2022; Samandra et al., 2022; Hossain M. B. et al., 2023), exposing risks to human health and preventing the efficient use of GW facilities. The prevailing research confirms that MPs in ecosystems is primarily derived from the ubiquitous use of plastic products in our daily life (Jiang et al., 2022). Due to its unique properties, plastics are getting popular, resulting in the increase of plastic production from 1.5 MT in 1950 to 367 MT in 2020 (Plastics Europe, 2021). Human civilization has gone through the Stone, Iron, Bronze, and Copper ages in the ancient times, and the modern period is considered the plastic age (Thompson et al., 2009). Researchers estimated that every year, approximately 71 percent of the plastic manufactured globally is released into the surroundings (Hohn et al., 2020). These plastic particles are disintegrated into tiny fragments and form MPs due to weathering processes such as UV radiation and mechanical abrasion (Andrady, 2011) and then are transported to the groundwater through agricultural runoff, sedimentation process, and recycling of biological solids and sewage (Lapworth et al., 2012; Cox et al., 2019; Xu et al., 2021). As concerns over water quality reach a crescendo, the threat of MPs looms large, revealing a hidden risk in the quest for potable water in developing countries.
MPs are able to enter human tissue through direct consumption of fish, seafood, salt, and drinking water (Bergmann et al., 2017; Alimi et al., 2018; Mattsson et al., 2018; Ajith et al., 2020; Hara et al., 2020; Hossain et al., 2020; Napper et al., 2020; Bajt, 2021; Parvin et al., 2021); inhalation (Zhang et al., 2020); and use of personal care products (Prata et al., 2020). MPs can pose health hazards to the plants, microbiota that live in the soil, and even to humans, if the microplastics can be found in the groundwater sources, which may result in growth inhibition and weight decrease, destructing the gut, and immune response as well as reproduction (Wang et al., 2020; Zhou et al., 2020). In addition, MPs can transport persistent organic pollutants (such as hexachlorobenzene and bisphenol A) as well as metallic substances (such as Cu, Pb, Hg, Cd, and Cr) which may have adverse effects on both terrestrial and aquatic organisms as well as human beings (Camacho et al., 2019; Duan et al., 2020). Moreover, polybrominated diphenyl ether (PBDE), nonylphenol, and bisphenol A are released from MPs which can cause human breast carcinoma, fertility problems, and heart disease (Weidemann et al., 2016). Therefore, it is crucial to estimate the route, dietary intake, and associated health risk of MPs to the human body.
The coastal zone of Bangladesh covers 30% of the agricultural land, large forestry, fisheries, and abundant flora and fauna, which supports many provisionary ecological services (such as food, feed, fiber, and fuel) and economic growth (Hossain et al., 2014). Therefore, the area is overpopulated with mostly farmers and fishermen. Due to the upstream river discharge and tidal activities, floods occur annually in the coastal regions facing the Bay of Bengal traversed by numerous rivers. As a result, pollutants including plastic waste from both terrestrial and marine environments are accumulated in flood plains and agricultural lands (Anik et al., 2022; Hossain M. B. et al., 2023). Over time, these plastic particles can seep through sediment layers to ground aquifers (Zhang et al., 2020; Golwala et al., 2021). For drinking water and other everyday needs, coastal residents rely primarily on GW sources in Bangladesh. So it is expected that they are consuming a huge number of MPs through drinking GW and daily use without their knowledge. Khant and Kim (2022) reported that studies on MPs in GW have largely been ignored around the world. In Bangladesh, several primary investigations were conducted on the presence and characterization of MPs in surface water of rivers and estuaries (Hossain M. B. et al., 2023; Al Nahian et al., 2023; Fatema et al., 2023; Haque et al., 2023). However, no scientific research has been performed to reveal MP contamination and its health risk in GW-sourced drinking water in Bangladesh. Therefore, comprehending the prevalence and characteristics of MPs in GW-sourced drinking water from Bangladesh is vital for safeguarding human health, protecting the environment, shaping policies, promoting sustainability, and sharing knowledge worldwide. The objectives of this study were to unravel the presence, morpho-chemical characteristics, and polymeric hazard analysis of MPs from the GW for the first time. The study also estimated the dietary intake and exposure levels of MPs to the coastal population through drinking GW. The findings have both regional and international importance as the data will aid in uncovering the hidden contamination of MPs beneath the ground, raising awareness among local residents and assisting policymakers in implementing necessary actions. In addition, lessons learned from this research can contribute to a broader understanding of the MP issue and its management on a global scale as plastic pollution has no national boundaries.
2 Materials and methods
2.1 Study area
The coastal geographic area of Bangladesh is located in a subtropical climate between 21°–23° N and 89°–93° E with a 710-km-long coastline along the Bay of Bengal and is divided into three distinct regions: the steady deltaic western part (Atlantic type), the deltaic eastern section (Pacific type), and the deltaic middle zone. The coastal zone is covered with an extensive river infrastructure (24,000 km in length), a significant number of islands across channels, and an underwater canyon (Swatch of No Ground), in addition to the funnel-like part of the northern Bay of Bengal. Cox’s Bazar, Chattogram, Feni, Noakhali, Laxmipur, Chandpur, Patuakhali, Khulna, Satkhira, and Bagerhat are some coastal districts of Bangladesh. Among them, Cox’s Bazar (21°25′38″N 92°00′18″E), Chattogram (22°20′06″N 91°49′57″E), Feni (23°1.1′N 91°24.6′E), Noakhali (22.70°N 91.10°E), Chandpur (23.2139°N 90.6361°E), and Patuakhali (22.3542°N 90.3181°E) were selected for conducting the present investigation in this study. The study areas are situated in the catchment area of the Meghna River (Noakhali and Chandpur), the Galachipa River and the Tetulia River (Patuakhali), the Feni River (Feni), the Karnaphuli and the Sangu rivers (Chattogram), and the Bara Matamuhuri River, the Naf River, and the Bakkhali River (Cox’s Bazar) (Figure 1). These rivers carried huge amount of wastes that are mainly of different synthetic origins (plastic and other debris) (Hossain et al., 2021). The people of these areas depend on GW for drinking and other household purposes.
2.2 Sample collection and preparation
A total of 54 GW samples were collected, with three stations from each district. Each sample with a volume of 500 mL was collected in triplicate, resulting in a total volume of 27 L. The samples were collected in 500-mL glass bottles and promptly sealed to prevent airborne contamination. Subsequently, the sealed bottles were preserved in an icebox at −20°C and transferred to the Laboratory for Ecology, Ecosystem, and Biodiversity (LEEB) for further investigation. Upon arrival at the laboratory, the conserved bottles were defrosted at room temperature. The groundwater was then filtered through a 5-micron cellulose nitrate membrane filter. The filter papers containing the filtered material were placed in transparent Petri dishes for microscopic assessment in the subsequent stage.
2.3 Visual identification of microplastics and polymer analysis
MP samples were analyzed and identified using established procedures (Barrows et al., 2018; McNeish et al., 2018) at the LEEB, Noakhali Science and Technology University. The protocol is outlined in Supplementary Figure S1. The filter paper was thoroughly examined under a stereomicroscope (Leica EZ4E, Germany) with 8 to 35 x magnification to obtain visual detection and quantification of MPs. We applied laboratory control samples (about two microplastic particles per filter) to adjust the levels of microplastic pollution. An exceptionally high-resolution webcam was used to capture photographs of MPs which was attached to a microscope, and the sizes of MPs were measured by ImageJ software (ver. 2.0.0). We classified particles as fiber, fragment, bead, or film as well as recorded the coloration. Colors are significant because yellow to brown, e.g., is generally associated with fibers from natural sources. Thirty microplastic specimens obtained from water samples were studied using a Fourier transform infrared spectroscope (FTIR, Nicolet 6700, Thermo Fisher Scientific, United States) to detect the chemical structure of the plastic particles.
2.4 Contamination control
The following precautions were employed to avoid cross-contamination during the processing and analysis of samples originating via external influences (i.e., atmospheric microplastics). All glass Petri dishes and other necessary equipment were washed with filtered distilled water before and after use. Any types of plastic equipment were skipped over to the greatest extent possible during the measurement of MPs, except during sample collection. When performing laboratory operations, all technicians were obliged to wear button-front cotton lab suits and powder-free nitrile-coated gloves. For control, a blank experiment was conducted following the same procedure, and no synthetic components were found in the blank sample.
2.5 Dietary intake (DI) of MPs
The following calculation was used to compute the dietary intake of MPs in water (Almaiman et al., 2021):
where C is the recommended consumption of water/person/day, M is the average MP particles/L, and BW is the average body weight for an adult. The World Health Organization’s recommended daily water intake (4.5 L/day) for an adult was used to calculate the per capita exposure estimation (Grandjean and Leparoux, 2004) because reliable information on consuming water in Bangladesh was lacking. The average weight of adult men/women in Bangladesh is 60 kg (Ullah et al., 2017).
2.6 Potential risk assessment of plastic particles
The polymer hazard index (PHI) is a tool designed to evaluate the environmental and health-related hazards posed by different types of polymers, likely based on their chemical constituents (Hossain M. B. et al., 2023; Al Nahian et al., 2023). Using the equation provided below, the polymer risk index can be estimated:
where Sn is the hazard score of the plastic polymers and Pn is the percentage of each plastic polymer type detected (Al Nahian et al., 2023). Applying the PHI score, referred to as the methodology of Al Nahian et al. (2023), the risk grades and hazard classes (Supplementary Table S1) of plastic components in all samples were estimated.
2.7 Statistical analysis
Each station of studied areas contained three replicates in our experiments; therefore, the concentration of MPs was expressed as mean ± standard deviation (SD). The mean abundance of MPs from different stations of each site was compared using the statistical analyses (ANOVA) through PAST (PAleontological STatistics, Version 4.03) statistical software, where a probability level less than 0.05 was considered as significant. Before conducting ANOVA, the pairwise differences were tested by Tukey’s HSD test. The homogeneity of variances was thoroughly checked by Levene’s test during the analysis. If necessary, square root or logarithm was used to transform the data. On the other hand, data heteroscedasticity was tested, even after data transformations. Levene’s test was allowed to reject the hypotheses at levels lower than the p values. Arc-GIS (version 10.7) software was used to show the sampling point in the study area map.
3 Results and discussion
3.1 Occurrence of MPs in groundwater (GW)
MPs were extracted and identified from all 18 sampling points from six coastal districts of Bangladesh (Figure 2). The abundance of MPs in the GW ranged from 4 to 75 items/L water with an average of 24.63 ± 16.13 items/L water (Figure 3). One-way ANOVA results demonstrated that there were significant variations (F = 2.96, p = 0.003) among the sampling points. Samples from the districts of Chandpur, Noakhali, and Cox’s Bazar had more MPs than those from other districts. Due to the dense population, coexistence of industrial and agricultural areas, as well as commercial and tourism activities, it is possible that these three major towns use a significant number of plastic items. According to several studies, MP pollution has caused the municipal water system to degenerate into a catastrophe (Hossain et al., 2021; Nur et al., 2022). Tukey’s pairwise comparisons revealed that the number of MPs in the GW of Chandpur and Chittagong differed significantly.
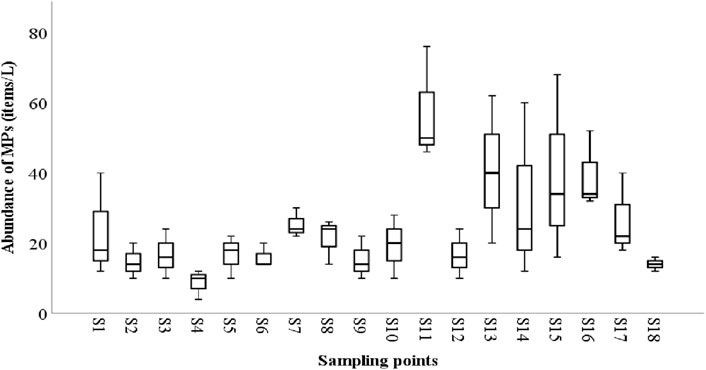
Figure 3. Abundance of MPs in the groundwater (GW) of coastal areas of Bangladesh. ANOVA analysis revealed significant variations (p < 0.05) among the sampling points.
The results of this study were contrasted with those of other studies carried out globally (Table 1) and found that the GW of Bangladesh is severely polluted compared to the public drinking water collected and supplied from a variety of sources in United States, Germany, India, Denmark, Mexico, and Sweden (Strand et al., 2018; Panno et al., 2019b; Mintenig et al., 2019; Kirstein et al., 2021; Selvam et al., 2021; Weber et al., 2021). As previously mentioned, GW is predominantly used for drinking and crop cultivation in rural areas of Bangladesh. If contaminated with microplastic particles, it could pose a hazard to human health compared to ingesting seafood contaminated with pollutants (Re, 2019). The occurrence of MPs in GW demonstrates the susceptible nature of fractured reservoirs to sewer lines, sinkholes, and urban wastewater systems (Ganesan et al., 2019). A microplastic debris phase mechanism can be established in agricultural operations if GW polluted by upward microplastic transportation is used for farming and productive land. Excessive contamination by microplastics in GW can be harmful to GW microbes. GW has a diverse range of organisms, such as troglofauna (White, 1988; Breitenbach, 2008; Momot et al., 2008) and stygofauna (Huang et al., 2021), which could be infected with plastic particles. Nevertheless, the precise mechanism by which GW microplastics harm such fauna species is unexplained and requires further investigation.
3.2 Morphological characteristics of MPs
The MPs detected in the GW samples were grouped into four categories, e.g., fibers, films, fragments, and microbeads (Figure 4A). Fibers were the most abundant (97.6%), followed by films (1.8%), fragments (0.3%), and microbeads (0.3%). Fibers were abundant at every sampling location (Figure 4A) and were filamentous in shape. The maximum number of fibers was recorded from S11 (average 57.33 items/L) and minimum in S4 (average 8 items/L). Microbeads were observed only in S13 (average 1.33 items/L). Although it is still difficult to specifically identify the source of MPs, the morphological characteristic generally identifies the sources of MPs. Fibers might have generated from the disintegration of regular plastic products, clothing materials, and sewage (Hossain et al., 2021). Additionally, fibers from fishing nets and ropes may have accumulated in the GW as fishing is one of the main industries in the studied area. All films and fragments were irregular in shape, and microbeads were round. Previous research studies have hypothesized that fibers may originate from clothing or rope; films may be related to single-use plastic bags, wrappers, and so forth. Microbeads are likely to be found in personal care products sold to consumers (Wang et al., 2020; Nur et al., 2022). When compared, this result is in line with observations from Selvam et al. (2021) and Strand et al. (2018), where fibers predominated over other types of MPs.
In the instance of MPs in GW from coastal regions of Bangladesh, colored MPs were predominantly recorded (57.3%), whereas the rest (42.7%) were transparent (Figure 4B). The colors recorded were violet (28.6%), pink (15.3%), blue (5.7%), green (3.9%), and red (3.8%). Transparent and violet-colored MPs were observed in all the sampling points, whereas the others differed from station to station. Since the color of MPs depend on the local climate, they can differ from one location to another due to their quick disintegration after being exposed to heat and sunlight for a long time (Shruti et al., 2020). The sizes of observed MPs were categorized into three groups, and it was interestingly found that smaller-sized MPs (<0.5 mm in size) were predominant (91.7% of the total MPs found), followed by 0.5–1 mm (6.9%) and 1–5 mm (1.4%) (Figure 4C). MPs less than 0.5 mm in size were abundant in all the sampling points. In contrast, MPs with size between 1 and 5 mm were observed occasionally. Our results correspond with those of Połeć et al. (2018) and Strand et al. (2018). The local climate, including temperature degradation, the longevity of the plastics, wind action, UV light intensity, transportation, residency time, and the distance from the plastics’ point of origin in the environment, largely determine the size of MPs (Nur et al., 2022; Hossain M. B. et al., 2023).
3.3 Chemical composition of MPs
The chemical composition of MPs may also partially indicate their origin. In this study, representative plastic particle samples taken from filter paper were analyzed by FTIR, and the resulting spectrum was contrasted with polymer types and additional referent collections. The characteristic of FTIR spectra confirmed that the studied particles were composed of four types of polymers, e.g., low-density polyethylene (LDPE), high-density polyethylene (HDPE), ethylene vinyl acetate (EVA), and polyethylene terephthalate (PETE) in the GW samples of Bangladesh (Supplementary Figure S2). The highest frequencies in the LDPE and HDPE spectra were indistinguishable at 2,916 cm−1, 2,846 cm−1, 2,914 cm−1, and 2,848 cm−1, respectively, indicating C-H stretching. Peaks at 1,466 cm−1 and 1,462 cm−1 in LDPE and 1,470 cm−1 and 1,462 cm−1 in HDPE, on the other hand, showed CH2 bending. The highest point in LDPE at 1,382 cm−1 wavelength symbolizes CH3 bending. Additionally, the spectral lines of 730 cm−1, 719 cm−1, and 717 cm−1 in LPDE and HDPE specify CH2 rock. Conversely, the electromagnetic spectrum of EVA revealed a strong peak at 2,920 cm−1 and 2,850 cm−1 showing C-H bond bending, accordingly, whereas 1,747 cm−1 and 1,769 cm−1 reflect C=O and CH2/CH3 stretch, respectively. PETE also revealed strong peaks at wavelengths of 713 cm−1, which represent aromatic C-H bond stretching at 1,241 cm−1 and C-O stretching at 1,035 cm−1. The -CH2 fingerprint in lipid structures can resemble that of polyethylene (PE), posing a challenge for differentiation. However, while both lipids and PE exhibit -CH2 stretching vibrations, their exact absorption bands may differ slightly due to variations in the molecular structure and environment. To distinguish between them, we used spectral databases to reference distinctive absorption peaks associated with each compound. Differentiating and quantification of polyesters like PETE from lipids can be difficult due to the presence of ester groups in both. Through careful examination of variations in peak locations, intensities, and shapes within the FTIR spectra of lipids and PETE, we have successfully differentiated between the two substances and evaluated the respective amounts in a given sample. For instance, lipids frequently exhibit wide, distinct peaks as a result of the existence of numerous functional groups and intricate molecular structures. On the other hand, PETE may display more distinct and concentrated peaks that correspond to certain vibrational modes of the ester groups in its polyester backbone. Lipids generally display absorption peaks at specific wavenumbers that correspond to functional groups, such as hydrocarbons (approximately 2,850–2,950 cm−1 for the stretching vibrations of CH bonds), and alkenes (approximately 3,010–3,070 cm−1 for the stretching vibrations of C-H bonds). On the other hand, PETE has absorption peaks that correspond to ester groups in its backbone structure. In addition, lipids generally display very high absorption bands for functional groups such as esters and carbonyls, but PETE may demonstrate somewhat less intense absorption in these locations. The quantification of these polymers was accomplished by establishing a correlation between the absorbance of certain bands and the content of lipids or PETE in the sample, utilizing calibration curves.
The results were supported by those of Mintenig et al. (2019) and Selvam et al. (2021), who also claimed that PE was more common. Since PE dominated among the various polymer categories, it has been hypothesized that the main sources of MPs in the area include agricultural wastes, synthetic fabrics, landfill leaching, and municipal effluents (Shruti et al., 2020; Selvam et al., 2021). According to Ganesan et al. (2019), polyethylene (PE), polystyrene (PS), and polypropylene (PP), which are frequently used for packaging, were found to be the most prevalent polymers in the environment. Fishing ropes and nets are also constructed from low-density polymers, which might be a reasonable explanation for the high abundance of the polymer LDPE in this study (Hossain M. B. et al., 2023). In addition, stretchy PE (LDPE and LLDPE) films with a short life cycle may be released into the environment as a result of the breakdown of agricultural films, plastic bags, wrapping materials, or industrial usage (Hossain M. B. et al., 2023).
3.4 Dietary intake of MPs
We assessed the daily water consumption of MP per person in order to assess human health exposure to MP pollutants and generate dietary guidelines for public health strategies. Using the mean recommended daily consumption of water (4.5 L per day as suggested by the WHO) for an adult (60 kg), the mean value of MPs (24.63 particles/L) in water and the per capita daily dietary intake of MPs have been determined to be 1.85 particles per person per day. These figures represent an average yearly MP intake of 675 particles per individual. According to Cox et al. (2019), the estimated average yearly intake of MPs in the US was 127,000 for male adults and 93,000 for female adults who only drank bottled water. The variation in the detection rate, and therefore consumption may be related to the size of the sample used.
3.5 Human health hazard risk assessment of identified polymers
The hazard scoring model using PHI based on their chemical constituents has been applied in the present study in order to identify and categorize the corresponding risk level (Figure 5). The results indicated varying levels of risks to human health with the polymer levels. PETE contributed around 13% of the identified microplastics, commonly originated from fabrics and packaging wastes showed a medium risk (Type-II) to human health (Figure 5). Additionally, the other three polymers, HDPE (39%), LDPE (31%), and EVA (17%), showed minor risk (Type-I) (Figure 5), which would cause less harm due to drinking water from studied sources.
The variation in risk levels among these polymers highlights the significance of not just considering the prevalence of microplastics but also recognizing their potential risks to human health. While PETE poses a moderate risk, the other polymers present a more favorable risk profile. This nuanced comprehension can steer future endeavors in handling and alleviating the impact of microplastics on water quality, placing specific attention on the types of polymers predominantly identified in the studied sources. The findings stress the necessity for targeted interventions to tackle the unique challenges posed by various microplastics, especially those originating from packaging waste. Furthermore, it emphasizes the ongoing importance of research to improve our understanding of the potential health hazards linked to microplastics and to guide effective strategies for water resource management and pollution prevention.
3.6 Limitations of the study
To obtain a thorough understanding of the health hazards associated with MPs, it is imperative to include the mass of MPs and increasing sample sizes. The precision of risk assessments is improved by these measurements, which allow for a more accurate evaluation of toxicity levels and bioaccumulation potential. Nevertheless, a number of reasons, including the goals of the study, available resources, technological difficulties, and methodological limitations, have made it difficult for this study to include mass measurements of MPs. It is possible to underestimate the actual health effects of microplastic exposure if the mass of MPs is ignored. Because of their higher surface area-to-volume ratios, smaller particles could be more dangerous per unit mass. Therefore, the inclusion of mass of MPs into MP research enhances the accuracy of risk evaluations and streamlines the creation of focused mitigation solutions.
4 Conclusion
The widespread occurrence of microplastics (MPs) in GW remains largely unnoticed despite its escalating global impact. This study provides a preliminary overview of MP presence, along with their morphological and chemical attributes, in Bangladesh coastal GW. MPs were detected at all 18 sampling sites, averaging 24.63 ± 16.13 items/L of water, suggesting a complex distribution influenced by changes in land use, coastal hydrology, and geology across the area. The majority of the identified MPs (97.6%) were fibers of less than 0.5 mm in size, which might be generated from the disintegration of regular plastic products, fishing nets, and ropes. Common polymers identified included polyethylene, ethylene vinyl acetate, and PETE. Among these, PE was found to be the predominant polymer type among the MPs. The disintegration of bigger plastic waste, landfill leaching, agricultural wastes, synthetic fabrics, and municipal effluents was most likely what gave rise to this polymer. Expected daily MP consumption was calculated to be 1.85 particles/person/day, which is concerning for human health. Hazard analysis findings revealed diverse risks associated with polymer levels, with PETE indicating a medium risk and other polymers (HDPE, LDPE, and EVA, showing a minor risk to human health in drinking water from the studied sources. The relatively smaller sample size and omission of MP mass measurement in this study may have led to an underestimation of the health or environmental risks associated with MPs. Disregarding the mass of MPs may lead to an underestimation of the actual health impacts of MP exposure. Although MPs are tiny in size, they have the potential to cause enormous harm to human health and the environment. This is because they may attract and carry pollutants, they remain in the environment for a long time, and they can build up in organisms at various levels of the food chain. Therefore, disregarding the mass of MPs may result in an underestimation of their total impact on environmental contamination and related health hazards. Hence, it is imperative to take into account both the quantity and mass of MPs when evaluating their possible consequences. In addition, the primary possible entry points for MPs into GW are via sediment and water in the surface layer; consequently, further in-depth research on the destiny of MPs and their presence in various soil strata is needed. Moreover, understanding GW issues will be aided by research on MPs found in the hyporheic region, the areas where surface water and groundwater interact.
Data availability statement
The original contributions presented in the study are included in the article/Supplementary Material; further inquiries can be directed to the corresponding authors.
Author contributions
BP: funding acquisition, writing–review and editing, and validation. JY: writing–review and editing. SS: investigation, methodology, and writing–original draft. PB: investigation, methodology, writing–original draft, and software. A-AUN: investigation, methodology, and writing–original draft. MRH: investigation, writing–original draft, and data curation. MR: writing–original draft. TA: investigation and writing–review and editing. LY: investigation and writing–review and editing. MBH: conceptualization, funding acquisition, and writing–review and editing.
Funding
The authors declare that financial support was received for the research, authorship, and/or publication of this article. This study was supported by Researchers Supporting Project Number (RSP2024R144), King Saud University, Riyadh, Saudi Arabia.
Acknowledgments
Assistance from all volunteers is highly appreciated. The authors would like to extend their sincere appreciation to the Researchers Supporting Project Number (RSP2024R144), King Saud University, Riyadh, Saudi Arabia.
Conflict of interest
The authors declare that the research was conducted in the absence of any commercial or financial relationships that could be construed as a potential conflict of interest.
Publisher’s note
All claims expressed in this article are solely those of the authors and do not necessarily represent those of their affiliated organizations, or those of the publisher, the editors, and the reviewers. Any product that may be evaluated in this article, or claim that may be made by its manufacturer, is not guaranteed or endorsed by the publisher.
Supplementary material
The Supplementary Material for this article can be found online at: https://www.frontiersin.org/articles/10.3389/fenvs.2024.1379311/full#supplementary-material
References
Ajith, N., Arumugam, S., Parthasarathy, S., Manupoori, S., and Janakiraman, S. (2020). Global distribution of microplastics and its impact on marine environment—a review. Environ. Sci. Pollut. Res. 27, 25970–25986. doi:10.1007/s11356-020-09015-5
Alimi, O. S., Farner Budarz, J., Hernandez, L. M., and Tufenkji, N. (2018). Microplastics and nanoplastics in aquatic environments: aggregation, deposition, and enhanced contaminant transport. Environ. Sci. Technol. 52, 1704–1724. doi:10.1021/acs.est.7b05559
Almaiman, L., Aljomah, A., Bineid, M., Aljeldah, F. M., Aldawsari, F., Liebmann, B., et al. (2021). The occurrence and dietary intake related to the presence of microplastics in drinking water in Saudi Arabia. Environ. Monit. Assess. 193 (7), 390. doi:10.1007/s10661-021-09132-9
Al Nahian, S., Rakib, M. R. J., Kumar, R., Haider, S. M. B., Sharma, P., and Idris, A. M. (2023). Distribution, characteristics, and risk assessments analysis of microplastics in shore sediments and surface water of Moheshkhali channel of Bay of Bengal, Bangladesh. Sci. Total Environ. 855, 158892. doi:10.1016/j.scitotenv.2022.158892
Andrady, A. L. (2011). Microplastics in the marine environment. Mar. Pollut. Bul. 62 (8), 1596–1605. doi:10.1016/j.marpolbul.2011.05.030
Anik, A. H., Khan, R., Hossain, S., Siddique, M. A. B., Tamim, U., Islam, A. T., et al. (2022). Reconciling the geogenic and non-crustal origins of elements in an Indo-Bangla transboundary river, Atrai: pollution status, sediment quality, and preliminary risk assessment. Environ. Res. 214, 114134. doi:10.1016/j.envres.2022.114134
Bajt, O. (2021). From plastics to microplastics and organisms. FEBS Ope. Bio. 11 (4), 954–966. doi:10.1002/2211-5463.13120
Barrows, A. P., Christiansen, K. S., Bode, E. T., and Hoellein, T. J. (2018). A watershed-scale, citizen science approach to quantifying microplastic concentration in a mixed land-use river. Wat. Res. 147, 382–392. doi:10.1016/j.watres.2018.10.013
Bergmann, M., Wirzberger, V., Krumpen, T., Lorenz, C., Primpke, S., Tekman, M. B., et al. (2017). High quantities of microplastic in Arctic deep-sea sediments from the HAUSGARTEN observatory. Environ. Sci. Tech. 51 (19), 11000–11010. doi:10.1021/acs.est.7b03331
Breitenbach, A. (2008). Two views on nature: a solution to Kant's antinomy of mechanism and teleology. Brit. Jour. Hist. Philos. 16 (2), 351–369. doi:10.1080/09608780801969167
Camacho, M., Herrera, A., Gómez, M., Acosta-Dacal, A., Martínez, I., Henríquez-Hernández, L. A., et al. (2019). Organic pollutants in marine plastic debris from Canary Islands beaches. Sci. Total Environ. 662, 22–31. doi:10.1016/j.scitotenv.2018.12.422
Cox, K. D., Covernton, G. A., Davies, H. L., Dower, J. F., Juanes, F., and Dudas, S. E. (2019). Human consumption of microplastics. Environ. Sci. 53 (12), 7068–7074. doi:10.1021/acs.est.9b01517
Duan, Z., Duan, X., Zhao, S., Wang, X., Wang, J., Liu, Y., et al. (2020). Barrier function of zebrafish embryonic chorions against microplastics and nanoplastics and its impact on embryo development. J. Haz. Mater. 395, 122621. doi:10.1016/j.jhazmat.2020.122621
Fatema, K., Rahman, T., Islam, M. J., Sumon, K. A., Uddin, M. H., Hasan, S. J., et al. (2023). Microplastics pollution in the river Karnaphuli: a preliminary study on a tidal confluence river in the southeast coast of Bangladesh. Environ. Sci. Pollut. Res. 30 (13), 38853–38868. doi:10.1007/s11356-022-24998-z
Ganesan, M., Nallathambi, G., and Srinivasalu, S. (2019). Fate and transport of microplastics from water sources. Cur. Sci. 117 (11), 1879–1885. doi:10.18520/cs/v117/i11/1879-1885
Golwala, H., Zhang, X., Iskander, S. M., and Smith, A. L. (2021). Solid waste: an overlooked source of microplastics to the environment. Sci. Total Environ. 769, 144581. doi:10.1016/j.scitotenv.2020.144581
Grandjean, G., and Leparoux, D. (2004). The potential of seismic methods for detecting cavities and buried objects: experimentation at a test site. Jour. App. Geophy. 56 (2), 93–106. doi:10.1016/j.jappgeo.2004.04.004
Haque, M. R., Ali, M. M., Ahmed, W., Siddique, M. A. B., Akbor, M. A., Islam, M. S., et al. (2023). Assessment of microplastics pollution in aquatic species (Fish, Crab, and Snail), water, and sediment from the Buriganga River, Bangladesh: an ecological risk appraisals. Sci. Total Environ. 857, 159344. doi:10.1016/j.scitotenv.2022.159344
Hara, J., Frias, J., and Nash, R. (2020). Quantification of microplastic ingestion by the decapod crustacean Nephrops norvegicus from Irish waters. Mar. Pollut. Bul. 152, 110905. doi:10.1016/j.marpolbul.2020.110905
Hohn, S., Acevedo-Trejos, E., Abrams, J. F., Fulgencio de Moura, J., Spranz, R., and Merico, A. (2020). The long-term legacy of plastic mass production. Sci. Total Environ. 746, 141115. doi:10.1016/j.scitotenv.2020.141115
Hossain, M. B., Banik, P., Nur, A. A. U., and Rahman, T. (2021). Abundance and characteristics of microplastics in sediments from the world's longest natural beach, Cox's Bazar, Bangladesh. Mar. Pollut. Bul. 163, 111956. doi:10.1016/j.marpolbul.2020.111956
Hossain, M. B., Sultana, J., Jolly, Y. N., Nur, A. A. U., Sultana, S., Miazee, R., et al. (2023b). Seasonal variation, contamination and ecological risk assessment of heavy metals in sediments of coastal wetlands along the Bay of Bengal. Mar. Pollut. Bul. 194, 115337. doi:10.1016/j.marpolbul.2023.115337
Hossain, M. B., Yu, J., Nur, A. A. U., Banik, P., Jolly, Y. N., Al-Mamun, M., et al. (2023a). Microplastics in surface water from a mighty subtropical estuary: first observations on occurrence, characterization, and contamination assessment. Environ. Res. 226, 115594. doi:10.1016/j.envres.2023.115594
Hossain, M. S., Rahman, M. S., Uddin, M. N., Sharifuzzaman, S. M., Chowdhury, S. R., Sarker, S., et al. (2020). Microplastic contamination in penaeid shrimp from the northern Bay of bengal. Chemos 238, 124688. doi:10.1016/j.chemosphere.2019.124688
Hossain, M. S., Roy, K., and Datta, D. K. (2014). Spatial and temporal variability of rainfall over the south-west coast of Bangladesh. Clim 2 (2), 28–46. doi:10.3390/cli2020028
Huang, J., Chen, H., Zheng, Y., Yang, Y., Zhang, Y., and Gao, B. (2021). Microplastic pollution in soils and groundwater: characteristics, analytical methods and impacts. Chem. Engin. Jour. 425, 131870. doi:10.1016/j.cej.2021.131870
Jiang, X., Yang, Y., Wang, Q., Liu, N., and Li, M. (2022). Seasonal variations and feedback from microplastics and cadmium on soil organisms in agricultural fields. Environ. Int. 161, 107096. doi:10.1016/j.envint.2022.107096
Khant, N. A., and Kim, H. (2022). Review of current issues and management strategies of microplastics in groundwater environments. Water 14 (7), 1020. doi:10.3390/w14071020
Kirstein, I. V., Hensel, F., Gomiero, A., Iordachescu, L., Vianello, A., Wittgren, H. B., et al. (2021). Drinking plastics? Quantification and qualification of microplastics in drinking water distribution systems by µFTIR and Py-GCMS. Wat. Res. 188, 116519. doi:10.1016/j.watres.2020.116519
Lapworth, D. J., Baran, N., Stuart, M. E., and Ward, R. S. (2012). Emerging organic contaminants in groundwater: a review of sources, fate and occurrence. Environ. Pollut. 163, 287–303. doi:10.1016/j.envpol.2011.12.034
Mattsson, K., Jocic, S., Doverbratt, I., and Hansson, L. A. (2018). Nanoplastics in the aquatic environment. Micropl. conta. Aqua. Environ. 379–399. doi:10.1016/B978-0-12-813747-5.00013-8
McNeish, R. E., Kim, L. H., Barrett, H. A., Mason, S. A., Kelly, J. J., and Hoellein, T. J. (2018). Microplastic in riverine fish is connected to species traits. Sci. Rep. 8 (1), 11639–11712. doi:10.1038/s41598-018-29980-9
Mintenig, S. M., Löder, M. G. J., Primpke, S., and Gerdts, G. (2019). Low numbers of microplastics detected in drinking water from ground water sources. Sci. Total Environ. 648, 631–635. doi:10.1016/j.scitotenv.2018.08.178
Momot, O., Synzynys, B., Kozmin, G., and Silin, I. (2008). “Pollution of ground sources of drinking water with technogenic tritium,” in Dang. Pollut. (Xenobiot.) urb (Berlin, Germany: Wat. Cyc. Sprin. Nether.), 331–340.
Napper, I. E., Davies, B. F. R., Clifford, H., Elvin, S., Koldewey, H. J., Mayewski, P. A., et al. (2020). Reaching new heights in plastic pollution—preliminary findings of microplastics on Mount Everest. Eart. 3, 621–630. doi:10.1016/j.oneear.2020.10.020
Nur, A. A. U., Hossain, M. B., Banik, P., Choudhury, T. R., Liba, S. I., Umamaheswari, S., et al. (2022). Microplastic contamination in processed and unprocessed sea salts from a developing country and potential risk assessment. Chemos 308, 136395. doi:10.1016/j.chemosphere.2022.136395
Panno, S. V., Kelly, W. R., Scott, J., Zheng, W., McNeish, R. E., Holm, N., et al. (2019a). Microplastic contamination in karst groundwater systems. Groundwat 57 (2), 189–196. doi:10.1111/gwat.12862
Panno, S. V., Kelly, W. R., Scott, J., Zheng, W., McNeish, R. E., Holm, N., et al. (2019b). Microplastic contamination in karst groundwater systems. Groundwat 57 (2), 189–196. doi:10.1111/gwat.12862
Parvin, F., Jannat, S., and Tareq, S. M. (2021). Abundance, characteristics and variation of microplastics in different freshwater fish species from Bangladesh. Sci. Total Environ. 784, 147137. doi:10.1016/j.scitotenv.2021.147137
Plastics Europe (2021). Plastics - the facts 2021. An analysis of European plastics production, demand and waste data. Available at: https://plasticseurope.org/knowledge-hub/plastics-the-facts-2021/ (Accessed July 07, 2022).
Połeć, M., Aleksander-Kwaterczak, U., Wątor, K., and Kmiecik, E. (2018). The occurrence of microplastics in freshwater systems–preliminary results from Krakow (Poland). Geol. Geophy. Environ. 44 (4), 391–400. doi:10.7494/geol.2018.44.4.391
Prata, J. C., da Costa, J. P., Lopes, I., Duarte, A. C., and Rocha-Santos, T. (2020). Environmental exposure to microplastics: an overview on possible human health effects. Sci. Total Environ. 702, 134455. doi:10.1016/j.scitotenv.2019.134455
Re, V. (2019). Shedding light on the invisible: addressing the potential for groundwater contamination by plastic microfibers. Hydrogeo 27, 2719–2727. doi:10.1007/s10040-019-01998-x
Samandra, S., Johnston, J. M., Jaeger, J. E., Symons, B., Xie, S., Currell, M., et al. (2022). Microplastic contamination of an unconfined groundwater aquifer in Victoria, Australia. Sci. Total Environ. 802, 149727. doi:10.1016/j.scitotenv.2021.149727
Selvam, S., Jesuraja, K., Venkatramanan, S., Roy, P. D., and Kumari, V. J. (2021). Hazardous microplastic characteristics and its role as a vector of heavy metal in groundwater and surface water of coastal south India. Jour. Hazar. Mat. 402, 123786. doi:10.1016/j.jhazmat.2020.123786
Shruti, V. C., Pérez-Guevara, F., Elizalde-Martínez, I., and Kutralam-Muniasamy, G. (2020). First study of its kind on the microplastic contamination of soft drinks, cold tea and energy drinks-Future research and environmental considerations. Sci. Tot. Environ. 726, 138580. doi:10.1016/j.scitotenv.2020.138580
Strand, J., Feld, L., Murphy, F., Mackevica, A., and Hartmann, N. B. (2018). Analysis of microplastic particles in Danish drinking water. DCE-Dan. Cent. Environ. Ener, 1–34.
Su, S., Zhou, S., and Lin, G. (2021). Existence of microplastics in soil and groundwater in Jiaodong Peninsula. E3S Web Conf. 251, 02045. doi:10.1051/e3sconf/202125102045
Thompson, R. C., Moore, C. J., Vom Saal, F. S., and Swan, S. H. (2009). Plastics, the environment and human health: current consensus and future trends. Philos. Trans. R. Soc. B Biol. Sci. 364, 2153–2166. doi:10.1098/rstb.2009.0053
Ullah, A. A., Maksud, M. A., Khan, S. R., Lutfa, L. N., and Quraishi, S. B. (2017). Dietary intake of heavy metals from eight highly consumed species of cultured fish and possible human health risk implications in Bangladesh. Toxico. Rep. 4, 574–579. doi:10.1016/j.toxrep.2017.10.002
Wang, W., Ge, J., Yu, X., and Li, H. (2020). Environmental fate and impacts of microplastics in soil ecosystems: progress and perspective. Sci. Total Environ. 708, 134841. doi:10.1016/j.scitotenv.2019.134841
Weber, F., Kerpen, J., Wolff, S., Langer, R., and Eschweiler, V. (2021). Investigation of microplastics contamination in drinking water of a German city. Sci. Total Environ. 755, 143421. doi:10.1016/j.scitotenv.2020.143421
Weidemann, E., Andersson, P. L., Bidleman, T., Boman, C., Carlin, D. J., Collina, E., et al. (2016). 14th congress of combustion by-products and their health effects—origin, fate, and health effects of combustion-related air pollutants in the coming era of bio-based energy sources. Environ. Sci. Pollut. Res. 23, 8141–8159. doi:10.1007/s11356-016-6308-y
White, W. B. (1988) Geomorphology and hydrology of karst terrains. Oxford, England: Oxford University Press.
Xu, B., Liu, S., Zhou, J. L., Zheng, C., Weifeng, J., Chen, B., et al. (2021). PFAS and their substitutes in groundwater: occurrence, transformation and remediation. Jour. Hazar. Mater. 412, 125159. doi:10.1016/j.jhazmat.2021.125159
Zhang, Y., Kang, S., Allen, S., Allen, D., Gao, T., and Sillanpää, M. (2020). Atmospheric microplastics: a review on current status and perspectives. Earth Sci. Rev. 203, 103118. doi:10.1016/j.earscirev.2020.103118
Keywords: microplastics, characteristics, groundwater contamination, polymers, health risk, groundwater
Citation: Paray BA, Yu J, Sultana S, Banik P, Nur A-AU, Haque MR, Rahman MM, Arai T, Yan L and Hossain MB (2024) Contamination, morphological and chemical characterization, and hazard risk analyses of microplastics in drinking water sourced from groundwater in a developing nation. Front. Environ. Sci. 12:1379311. doi: 10.3389/fenvs.2024.1379311
Received: 31 January 2024; Accepted: 29 April 2024;
Published: 24 May 2024.
Edited by:
Yu Xia, Southern University of Science and Technology, ChinaReviewed by:
Ramani Narayan, Michigan State University, United StatesLuka Traven, University of Rijeka, Croatia
Copyright © 2024 Paray, Yu, Sultana, Banik, Nur, Haque, Rahman, Arai, Yan and Hossain. This is an open-access article distributed under the terms of the Creative Commons Attribution License (CC BY). The use, distribution or reproduction in other forums is permitted, provided the original author(s) and the copyright owner(s) are credited and that the original publication in this journal is cited, in accordance with accepted academic practice. No use, distribution or reproduction is permitted which does not comply with these terms.
*Correspondence: M. Belal Hossain, YmVsYWwuaG9zc2FpbkBuc3R1LmVkdS5iZA==; Li Yan, THlsZUBuamZ1LmVkdS5jbg==