- 1Soil Science, Faculty of Regional and Environmental Sciences, Trier University, Trier, Germany
- 2Department of Physical Sciences, School of Science, Kaimosi Friends University, Kaimosi, Kenya
- 3Analytical and Environmental Chemistry, Department of Physical Sciences, Machakos University, Machakos, Kenya
To increase the biodiversity of agricultural systems, aromatic plants appear particularly promising as additional perennial crops in intercropping. They produce essential oils that contain monoterpenes, for example. These compounds have antibiotic properties that make them interesting for commercialisation as medicinal or pesticide products, but also carry the risk of undesirable effects on soil microorganisms and thus on essential soil functions. To investigate this, the monoterpenes thymol and carvacrol and a set of four typical agricultural soils were selected and soil sorption batch tests and soil toxicity tests were carried out to determine dose-response relationships. Sorption followed second order kinetics and was best described at equilibrium by the non-linear BET isotherm that distinguishes between monolayer and multilayer sorption of the non-polar compounds. While the kinetics were very fast with rate constants of 1.66 × 10−4 and 0.70 × 10−4 min−1 for thymol and carvacrol, the strength of sorption remained quite low (Kd 1.93–3.69), indicating a high mobility and bioavailability of the monoterpenes. In addition, the relation to single soil properties remained low, indicating a multivariate impact of several soil properties. Although thymol and carvacrol are isomeric compounds, they differed in the rate and strength of sorption as well as in the effects on five selected soil microbiological enzyme activities. In particular, carvacrol caused inhibition of enzyme activities, whereas thymol did not produce measurable or relevant effects in all cases. The effect concentrations of 10% and 25% percent inhibition (EC10, EC25) ranged from <1 µg to several grams per Gram of soil and hormesis effects were also observed at low concentrations. On the one hand, this indicates only low toxicity; on the other hand, several of the defined effect thresholds can be reached or exceeded by expected environmental concentrations. This may harbour a risk of adverse effects, but may also have a phytosanitary effect, which requires further research.
1 Introduction
Modern, intensified agriculture is often characterised by very reduced crop rotations, if not monocropping, with specialisation in a few crops that are grown in single-variety stands (Di Bene et al., 2022). Such agricultural management is often associated with high use of tillage and agrochemicals, and negative impacts on soil and water quality and biodiversity (Marotti et al., 2023; Zabala et al., 2023). Crop diversification strategies are key to transforming unsustainable farming practices and reducing economic risks. The cultivation of additional main crops or intercrops can promote not only soil quality, biodiversity, crop productivity and resource use efficiency, but also crop resilience, weed and pest/pathogen control (Sharma et al., 2021; Di Bene et al., 2022; Wooliver et al., 2022). These agricultural techniques are not recent inventions, but are based on historical or ancient knowledge, e.g., the Roman “coltura promiscua” in Italy, which disappeared from practice in the last century under the economic constraints of modern agriculture (Ferrario, 2021). Crop diversification with intercropping as an understory to the main crop is especially possible with perennial crops such as olive, fruit and nut trees, and in viticulture, where there is unused, often uncovered soil between rows with large spacing (Dittrich et al., 2021; Mantzanas et al., 2021; Marotti et al., 2023). In this context, aromatic plants such as lavender, thyme, rosemary, oregano, sage, etc. are on one hand classical crop plants and on the other hand promising plants for crop diversification through intercropping (Zhang et al., 2021; Katsoulis et al., 2022; Marotti et al., 2023). Under suitable climatic conditions, they can also be grown on marginal soils and provide high-priced products such as aromatic oils, and the perennial flowering plants foster pollinating insects and contribute to erosion control (Dittrich et al., 2021; Yadav et al., 2023).
Aromatic plants contain essential oils, which consist of volatile isoprenoids such as terpenes and terpenoids that give the plants the characteristic odour. In leaves of aromatic plants, terpenes can account for up to 20% of the dry mass and reach concentrations of several hundred μg g−1 in litter and fresh root material (Langenheim, 1994; Asensio et al., 2008; Adamczyk et al., 2015). For example, the isomeric terpenes thymol and carvacrol are the main components of the essential oil of thyme and oregano. They can account for up to 64% (thymol) or 80% (carvacrol) of all essential oil constituents in oregano, and up to 60% for thymol and 4.2% for carvacrol in thyme (Fournomiti et al., 2015; Sakkas and Papadopoulou, 2017).
Aromatic plants are commonly used in medicine and food preservation because their essential oils have antimicrobial activity against human and animal pathogens as well as an antioxidant effect (Fournomiti et al., 2015; Sakkas and Papadopoulou, 2017). Thyme and oregano are among the widely used aromatic plants that are known for their antimicrobial properties and are used as medicines (Fournomiti et al., 2015; Sakkas and Papadopoulou, 2017). Additionally, insecticidal, fungicidal and herbicidal properties have been identified so that terpenes use as pesticides is proposed (Chudasama and Thaker, 2012; Marei et al., 2012; Li et al., 2022).
Plants release significant amounts of secondary metabolites into the soil through volatilisation and diffusion away from plant tissues, leaching of aboveground plant material, exudation from plant roots and litter decomposition (Lin et al., 2007; Chomel et al., 2016). Consequently, volatile isoprenoids are also present in soil where they may persist for up to a year and some of which are key-drivers of ecological functions, e.g., in soil nutrient cycling (White, 1991; Ehlers, 2011; Sparks et al., 2017). On the other hand, negative impacts may occur such as phytotoxic effects, e.g., on seed germination (Mahdavikia and Saharkhiz, 2015). In addition, the essential oils may influence the enzymatic activities and composition of microbial communities in the soil and especially in the rhizosphere (Asensio et al., 2008; Adamczyk et al., 2015; Barra Caracciolo et al., 2015). This may be of environmental relevance, as the activities of soil biota are crucial for many of the ecosystem services of soil (Ockleford et al., 2017). Several monoterpenes acutely inhibit net nitrogen mineralization and net nitrification in soil by more than 50% depending on concentration and contact time (White, 1991; Heumann et al., 2013) and adversely affect litter decomposition (Chomel et al., 2016; Xie et al., 2017).
Undesirable effects on other crop plants, soil organisms and soil fertility can therefore not be ruled out. Such adverse effects of essential oil components would significantly reduce the utilisation of aromatic plants as an ecologically meaningful solution to the diversification of arable farming systems. However, the few available ecotoxicological studies are limited to the input and effects of aromatic plant material or essential oil in forest soils; only the study by Heumann et al. (2013) investigated the effects of isoprenoids on agricultural soils.
Against this background, the present study comparatively investigated the fate and effects of the two isomeric monoterpenes thymol and carvacrol in agricultural soil. These two components represent monoterpenes as part of the volatile isoprenoids and occur predominantly in numerous aromatic plants and woody plants such as thyme, oregano, marjoram, rosemary, coriander, mint, lemon, bergamot or tagetes (Duke, 2016). In more detail, the sorption as an essential process for the fate of chemicals in soils and effects on selected microbial enzyme activities in the soil were analysed in laboratory batch and microcosm experiments. This was done in short-term experiments using selected representative soil samples, substance concentrations and incubation times to determine the influence of soil and monoterpene properties, substance concentration, and duration of soil contact. In order to obtain data on the potential acute effects of monoterpenes in soil, selected microbial enzyme activities of soil samples exposed to different concentrations of thymol and carvacrol for different short incubation times were investigated.
2 Materials and methods
2.1 Soil and chemicals
The soil samples were collected from a vineyard and three different arable sites in the greater region of the city of Trier, Germany (Table 1). Soil A was sampled from the control plot of a vineyard (49°39′23.976‘‘N, 6°33’27.936E) near Wawern in the Mosel area of Germany. In treated plots of this vineyard, aromatic plants, i.e., thyme and oregano, were tested as understory crops for agricultural crop diversification. For more details see Dittrich et al. (2021). Arable fields are represented by sample B and C from Ferschweiler (49.8631° N, 6.4056° E), and sample D from Ernzen (49.8422° N, 6.4234° E). Composite soil samples were obtained from a sampling depth of 0–10 cm (vineyard) and 0–20 cm (arable) depth. All samples were sieved to grain size <2 mm and either air-dried prior to chemical analyses and sorption experiments or left field moist and stored frozen at −18 °C until toxicity testing (soil A only). The samples representatively cover properties of agricultural soils in temperate regions, e.g., with a pH range from 3.9 to 6.5 and an OC content ranging from 1.16% to 2.60%. These data and information on N content, CEC, clay content and texture class as well as water holding capacity are listed in Table 1. Additionally, the dry mass, water content, and water holding capacity of the field-moist sample A were determined (data not shown).
Analytical grade thymol and carvacrol with purity of 99.9% and 98.8% respectively were purchased from Sigma-Aldrich (Darmstadt, Germany). Selected physicochemical properties of the compounds are presented in Table 2. Stock solutions of thymol and carvacrol were prepared by dissolution in absolute ethanol and serial dilution with absolute ethanol to obtain solutions of different concentrations (10,000–0.1 μg mL−1). This limited the amount of ethanol added when spiking the soil samples to 20 µL ethanol g−1 soil. Preliminary tests have shown that the activity of the tested exoenzymes in the presence of ethanol was between >86% and 100% of the unamended soil and did not affect the soil sorption of other chemicals (data not shown). However, to account for any influence of ethanol, a corresponding volume of ethanol was also added to each control sample.
2.2 Soil sorption experiments
Batch sorption experiments according to OECD guideline 106 (OECD, 2000) were carried out. For this purpose, 4 g of dry soil were dispersed in hermetically closed Falcon tubes in 20 mL of 0.01 M CaCl2. To determine the sorption kinetics, samples were spiked with one of the terpenes, either carvacrol or thymol, at a concentration of 0.6 μg mL−1 or left untreated as a control. To determine the sorption isotherms, four different spiking concentrations were analysed, i.e. 0.1, 0.2, 0.5, and 1.0 μg mL−1, which corresponds to a range of 0.5–5.0 μg g−1 soil. Additional three low spiking concentrations (0.01, 0.02, 0.05 μg mL−1) were exemplarily analysed for soil D. These spiking concentrations were all well below the water solubility of the two monoterpenes (Table 2), which also avoided undesirable effects such as precipitation or phase separation. All samples were prepared in triplicate. To exclude biodegradation, the samples from all sorption experiments were initially sterilized using sodium azide at a final concentration of 0.2 g L−1 as it was done in the study of Hale et al. (2015). The sealed flasks were agitated in an overhead shaker at 15 rpm, thermostated at 22°C. In the kinetics experiments, samples were removed at five predetermined contact times of up to 240 min. Based on the results from these experiments, the equilibrium time for the sorption isotherm experiments was set at 600 min to ensure that sorption equilibrium had been fully achieved. After the respective contact time, the sample tubes were centrifuged at 3,000 g for 15 min. Aliquots of the supernatant were filtered using 0.2-μm PTFE disc syringe filters (Macherey-Nagel, Düren, Germany) and transferred into 1.5 mL brown glass crimp cap bottles for subsequent analysis.
The terpenes were analysed by high performance liquid chromatography (HPLC) with diode array (DAD) detection at 275 nm (LC 20AD, Shimadzu, Duisburg, Germany) according to a modified method by Gámiz et al. (2018). A C-18 chromatographic column (VDSpher© PUR 100 C18-M-SE, VDS optilab, Berlin, Germany) served as stationary phase. The mobile phase was a mixture of acetonitrile and water (50:50 v/v) that was delivered isocratically at a flow rate of 0.5 mL min−1. The injection volume was 50 µL. The limit of detection was <3 ng mL−1 for thymol and carvacrol.
2.3 Dose-response experiments targeting microbial enzyme activities
Possible dose-dependent adverse effects of the two terpenes on soil microbial activities were investigated testing five microbial enzyme activities. Only the vineyard soil A was used for the experiments. To prepare the samples, thymol and carvacrol were separately added to independent soil samples at contents of 0 (control), 1, 10, 100, 1,000 and 10,000 μg g−1 soil. Each sample had a moist soil mass (adjusted to 50% of the water holding capacity) equivalent to 22.5 g dry soil. All samples were prepared in triplicate. After spiking, the soil samples were thoroughly homogenized and incubated for 1, 4 or 9 days in hermetically closed Falcon tubes to avoid gaseous losses of thymol and carvacrol. It was ensured that the gas volume in the headspace was sufficient to maintain aerobic conditions throughout the incubation time.
The enzymes tested are representative of the general microbial activity and are included in the most important pathways of the C, N and P cycles in soil. Four of the enzyme activities were measured using a modified fluorimetric microplate enzyme assay according to Marx et al. (2001) with enzyme substrates based on methylumbelliferone (MUB) and 7-amino-4-methylcoumarin (AMC): MUB-N-acetyl-β-d-glucosamine for N-acetyl-glucosaminidase (EC 3.2.1.14), MUB-β-d-glucopyranoside for ß-glucosidase (EC 3.2.1.3), L-leucine-AMC for leucine-aminopeptidase (EC 3.4.11.1), and MUB-phosphate for acid phosphatase (EC 3.1.3.2). The analytical procedure was described in detail by Ernst et al. (2009). The activity of the dehydrogenase enzyme (EC 1.1.1) was tested using iodonitrotetrazolium chloride (INT) as substrate (von Mersi and Schinner, 1991). Fluorescence was measured using a Victor3 Multi-Label Reader (Perkin Elmer, Rodgau, Germany) at an excitation wavelength of 355 nm and an emission wavelength of 460 nm. The data were recalculated using calibrations and are expressed as MUB or AMC release in nmol per mass of dry soil and time (nmol g−1 h−1). The colorimetric dehydrogenase test was analysed using a Shimadzu UV-1650 PC spectrophotometer (Shimadzu, Duisburg, Germany) at 485 nm. The dehydrogenase activity was expressed as amount of the enzyme reaction product iodonitrotetrazolium formazan (INTF) per mass of dry soil and time (nmol INTF g−1 h−1).
2.4 Data modelling and description
To describe the experimental data with established models, different equations were tested for best-fit using the CurveExpert Professional 2.7.3 software (© by Daniel G. Hyams) for non-linear regression. The best fitting model was selected based on the coefficient of determination (R2), the standard error (SE), and Akaike’s corrected information criterion (AICC).
For sorption kinetics two models were tested, i.e., the pseudo first order with residue model and the second order model (Eqs 1, 2).
In Eqs 1, 2 C is the sorbate concentration (µg mL−1) at time t (min), C0 is the initial spiking concentration (with C0,1 + C0,2 = C0 for the pseudo first order with residue model), and k is the rate constant (min−1).
Five different isotherm models including the Henry, Freundlich, Langmuir and Hill model have been tested (data not shown). However, in all cases, best-fit was obtained using the BET model (Brunauer et al., 1938) according to Eq. 3:
where qe is the amount sorbed per unit weight of adsorbent (µg g−1) at concentration Ce (µg mL−1), Q is the amount sorbed per unit weight of adsorbent in forming a complete monolayer on the surface (µg g−1), Csat is the saturation concentration (µg mL−1) of the solute in the multi-solute system, and K (L kg−1) is the sorption coefficient that indicates the energy of interaction with the surface (Broholm et al., 1999).
The Spearman rank order correlation and the Pearson product moment correlation were used to determine relationships between the parameters of the calculated sorption isotherms and the soil properties. This was done using Sigmaplot 14.0 software (Systat Software Inc., Frankfurt/Main, Germany).
To describe the toxicity test data, the data were first converted to enable a non-linear curve fit. This included the expression of the enzyme activities as relative values in percent of the activity in the untreated control (100%) and the addition of a constant value (0.01 μg g−1) to all spiking concentrations in order to be able to calculate the logarithm also for the 0-spiking concentration added to the control (Thiele-Bruhn, 2005). In the case of enzyme activation rather than inhibition at low concentrations, modelling was limited to the data points starting from the highest activity. Of 24 dose-response models tested, the following three (Eqs 4–6) gave the best fit to the experimental data:
Equations 4–6 describe the effect y (activity/activity in control %) as a function of the dose x (µg g−1) and the coefficients a, b, c (Eq. 6) and α, β and γ (Eqs 4, 5) as fitting parameters with γ = (xβ–1)/β. The term erf refers to the error function and e is the Euler number. Effect concentrations (EC; μg g−1) of 10, 25% and 50% inhibition of the tested activity were calculated using the fitted data (Eqs 4–6).
3 Results and discussion
3.1 Soil sorption kinetics and isotherms
The adsorption of thymol and carvacrol on soil A was clearly time-dependent, with the concentration remaining in the solution decreasing consistently with time (Figure 1A). The residual concentration of thymol reached a state of equilibrium within 120 min, represented by a plateau of rather constant concentrations in the following period from 120 to 240 min. The time-dependent process was less clear for carvacrol, where the analytical data indicate an intermediate plateau (60–180 min) before the residual concentration continued to approach that of thymol at 240 min (Figure 1A). However, this apparent intermediate plateau was characterised by increasing standard deviations of the data over time, which is why a continuous decrease is more likely to be assumed. Consequently, both data series were best fitted using the same second order model (Eq. 2; Figure 1B). Fitting and statistical parameters are given in Table 3. In comparison, the pseudo first order model with residue (Eq. 1) failed to fit the sorption kinetics of carvacrol. Consistently, the description of the sorption kinetics of thymol on clay minerals by the second order model was superior to the first order model (Ziyat et al., 2020).
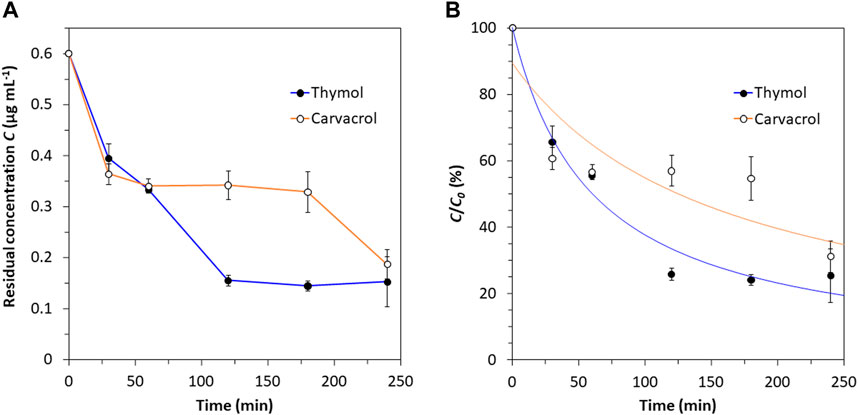
Figure 1. Sorption kinetics of the two monoterpenes thymol and carvacrol on soil A shown (A) as experimentally analysed residual concentrations (µg mL−1) and (B) as a percentage of the initial concentration (C/C0); lines in (B) were modelled using the pseudo second order model (Eq. 2).

Table 3. Parameters of the pseudo second order kinetic model (Eq. 2) of the soil sorption of thymol and carvacrol to a selected topsoil (D).
Overall, the residual concentrations in the aqueous phase (Figure 1B) of about 25% for thymol and 31% for carvacrol show that soil sorption of both monoterpenes was largely similar, but was slightly higher and faster (as indicated by the rate constant k, Table 3) for thymol. This is remarkable as both compounds differ structurally only in the position of the phenol group (Table 1). In line with this, Gámiz et al. (2018) found approximately similar dissipation rates for carvone in autoclaved sandy loam soils with even significant differences for the two enantiomers R-carvone and S-carvone (rate constant k [min−1] of 0.32 × 10−4 and 0.035 × 10−4). While the general physicochemical characteristics show no differences between thymol and carvacrol, there are differences in the quantum-chemical parameters dipole moment and energy of the highest occupied molecular orbital (EHOMO; Table 1). The latter two parameters have been repeatedly reported to explain chemical sorption well in poly-parameter linear free energy relationships (e.g., Cai et al., 2019; Pérez-Guzmán et al., 2021) and in this case consistently indicate stronger sorption of thymol. Furthermore, the sorption of thymol to a natural clayey material consisting mainly of stevensite reached equilibrium within a roughly similar but slightly shorter period of about 60 min (Ziyat et al., 2020). This may indicate that the more complex composition of soil, e.g., with organic matter and quartz, delays the sorption of monoterpenes. It should be noted, though, that the experimental conditions in the study of Ziyat et al. (2020) were significantly different in some regards (e.g., pH value of 9 instead of 6.5 for soil A). The best fit by the pseudo-second-order model may indicate that soil sorption of monoterpenes is not only driven by the residual aqueous concentration of the compounds at a given time, but also depends on the different sorption sites provided by a heterogeneous sorbent such as soil (Xing and Pignatello, 1997).
Heterogeneous sorption was further indicated by the non-linear sorption isotherms of both thymol and carvacrol for all four tested soils (Figures 2A, B). Sorption was best fit using the BET model (Eq. 3; R2 ≥ 0.997), which shows s-shaped sorption isotherms. Particularly in the low solution concentration range, the sorbed fractions increased disproportionately with the concentrations in the equilibrium solutions. This indicates increasingly unfavourable sorption, in which increasingly low-energy exchanger sites have to be occupied by the sorbates (Pignatello, 2011). The strong increase in sorption (qe) at high solution concentrations is probably due to the transition from monolayer to multilayer sorption (Broholm et al., 1999). This is reflected in the calculated equation parameters by Ce approaching Csat (Table 4). Multilayer sorption of phenolic compounds with structural similarity to terpenes has been repeatedly and consistently reported (e.g., Kamgaing et al., 2017; Qasemi et al., 2018; Lawal et al., 2021). In addition, quantum chemical modelling by Dhaundiyal et al. (2019) revealed that donor-acceptor interactions between the π-electrons of the aromatic ring and the corresponding positions of the sorbent contribute significantly to the sorption of thymol and carvacrol. Such π-π stacking interactions result in multilayer sorption (Tang et al., 2007; Mrozik et al., 2021). It should be noted that in other studies, the sorption of the monoterpenes carvone, α-pinene and limonene to soils or carbonaceous geosorbents was modelled using the Freundlich isotherm instead (Hale et al., 2015; Gámiz et al., 2018). However, the figures in these publications show deviations from this model for several monoterpene-sorbent combinations.
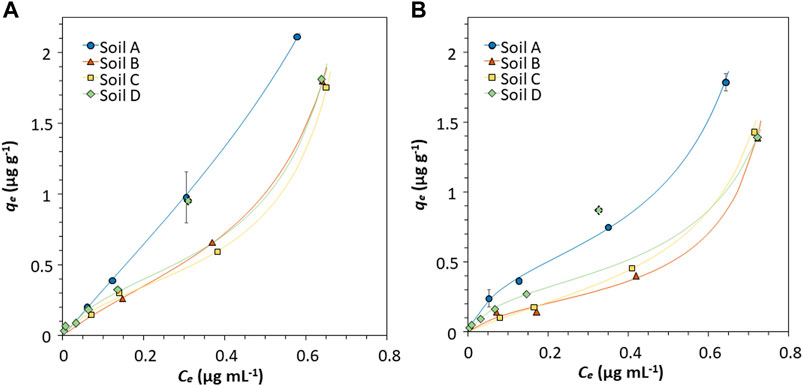
Figure 2. Sorption isotherms of the two monoterpenes (A) thymol and (B) carvacrol on four selected topsoil samples (A–D); shown are the experimentally analysed data points and non-linear isotherms modelled using the BET model (Eq. 3). Error bars not shown are smaller than symbols. One data point in (A,B) marked by a dashed line frame was not used for modelling.
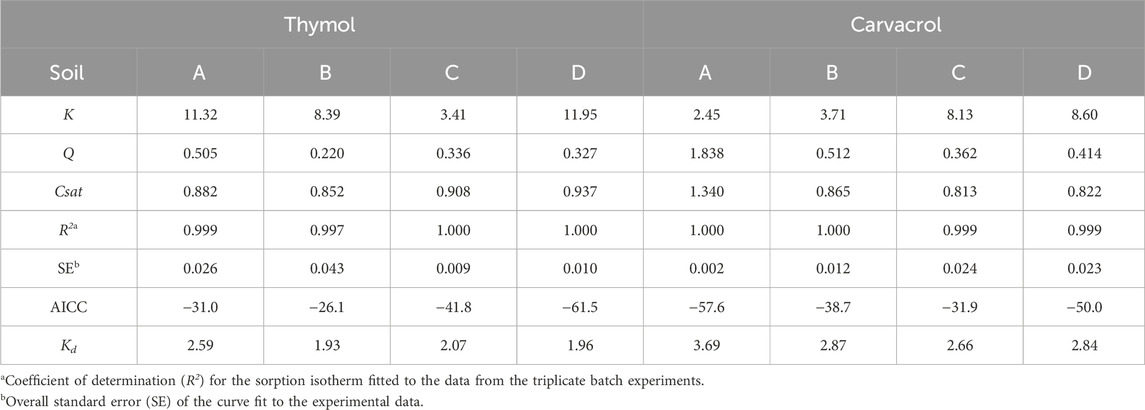
Table 4. Parameters of the isotherm models of the soil sorption of thymol and carvacrol to four selected topsoils (A-D) using the BET model (Eq. 3), and the distribution coefficient Kd using the linear Henry model.
When evaluating BET isotherms, both parameters K and Q must be taken into account with regard to the sorption strength. The parameter Q describes the steepness of the isotherm in the low concentration range; the smaller K is, the flatter the further course of the isotherm. In a comparison of the two monoterpenes, the differences in the isotherm parameters were small but evident. The value for K was higher for thymol than for carvacrol in three (A, B, D) of the four soils investigated, whereas the value for Q was for all four soils highest for carvacrol (Table 4). Overall, the position of the isotherms in Figures 2A, B indicates a stronger sorption of thymol compared to carvacrol. Apparently, the position of the methyl or isopropyl group in relation to the hydroxyl group of the phenol is of significant importance for the sorption of the two monoterpenes. The closer vicinity of the hydroxyl group to the isopropyl group results in a higher polarity (see molecular structure and dipole moment in Table 2) which favours the overall sorption of thymol (Table 2). This stronger sorption will be due in particular to the stronger interaction of the hydroxyl group with corresponding functional groups on the soil matrix, as shown by Dhaundiyal et al. (2019) in quantum chemical modelling for thymol and carvacrol. In contrast, formation of a complete monolayer on the surface (see values for Q in Table 4) and multilayer sorption at higher concentrations (see steeper sloped isotherms at higher concentrations, Figure 2) is apparently preferred for carvacrol. This will result from a stronger contribution of π-π stacking interactions of the less polar carvacrol as it was modelled by Dhaundiyal et al. (2019).
The significantly stronger sorption of thymol and carvacrol on soil A, which has both the highest pH value and the highest OC content of the four soils analysed (Table 1), is striking (Figure 2). This could indicate the relevance of soil organic matter and pH for the sorption of the two monoterpenes. Overall, however, the values of the BET isotherm parameters were poorly correlated with the soil properties (data not shown). This indicated that the sorption was not univariate, depending on individual soil properties, but on the interaction of several properties. However, the data set was too small to test this by multivariate analysis. The sorption of thymol showed only a weak, non-significant correlation with the OC content of the soils. The sorption of carvacrol, on the other hand, decreased with the pH value of the soils, which was also the only significant correlation (Spearman r = −0.956, p = 0.04). The sorption of monoterpenes to soil organic matter clearly outweighs sorption to mineral colloids such as clay minerals and pedogenic oxides (Van Roon et al., 2005; Gámiz et al., 2018). It can be assumed that the sorption of the comparatively non-polar monoterpenes to soil organic matter increased with decreasing pH in this study because the dissociation and charge of the functional groups of soil organic matter decrease with decreasing pH, making it more non-polar as well (Blume et al., 2015). Accordingly, the sorption of the monoterpenes α-pinene and limonene to uncharged graphite was stronger than to peat rich in functional groups, as indicated by a Kd that was about 1.2 times higher (Hale et al., 2015).
Although the linear Henry model was not suitable to describe the non-linear course of the sorption data of the monoterpenes, distribution coefficients were calculated with this equation to be able to compare them with the results of other studies. The Kd values ranged between 1.93 and 3.69 L kg−1 (Table 4). Consistently, Kd values of carvone sorption to six agricultural soils with largely lower OC contents of <1.4% ranged from 0.24 to 1.26 L kg−1 (Gámiz et al., 2018). Based on these results, it can be assumed that monoterpenes are relatively mobile substances in soils in the low concentration range below multilayer sorption despite their low water solubility. In addition to this mobility, there is also the possibility of volatilization of the monoterpenes from the soil, that are also known as volatile organic compounds (Leff and Fierer, 2008). However, using the Henry air-water partition coefficients (Table 2), it was calculated that despite the intense odour in the gas phase, the proportion volatilised into the headspace of the Falcon tubes was negligibly small (data not shown). It was consistently shown that the total emissions of various monoterpenes from forest soils under spruce and oak, as monoterpene-producing plants, are relatively insignificant in relation to the total emissions from forest ecosystems (Hayward et al., 2001; Asensio et al., 2007).
3.2 Effects on soil microbial enzyme activities
Both thymol and carvacrol exhibited dose- and time-dependent effects on the tested soil microbial enzyme activities. This is illustrated in Figure 3 on the example of N-acetylglucosaminidase and dehydrogenase. In all samples studied, there was a concentration-dependent reduction in enzyme activity, although in most treatments this was only evident from a concentration of >1 μg g−1. In a few cases, almost complete inhibition was achieved over the concentration range investigated (see, e.g., Figure 3B).
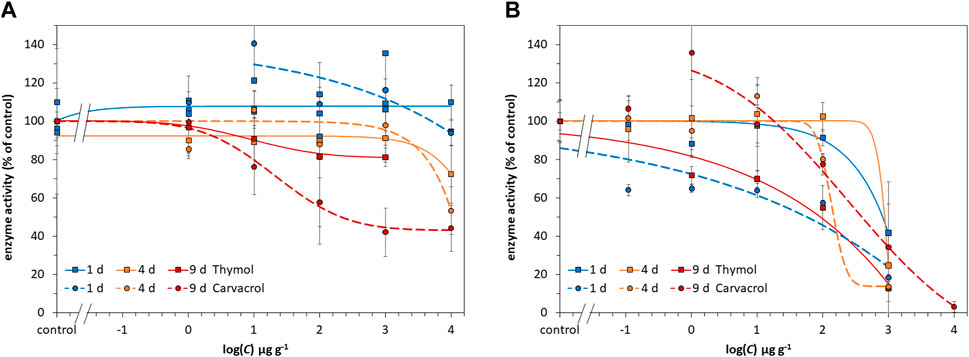
Figure 3. Dose related effects of the two monoterpenes thymol and carvacrol on (A) the N-acetyl-glucosaminidase activity and (B) dehydrogenase activity in soil A after an incubation time of 1, 4 and 9 days; shown are the experimentally analysed data as symbols and the modelled dose-response curves as lines. Error bars not shown are smaller than symbols.
Based on the modelled dose-response curves, effect concentrations (EC) of 10%, 25% and 50% inhibition were derived for all five enzyme activities investigated (Table 5). These data varied considerably between the enzymes and monoterpenes tested and ranged for EC10 from <1 µg to several grams per Gram of soil. In most cases, the EC50 was not reached at the dose range tested or data had to be calculated by extrapolation from the model (Table 5). (Detailed information on the fitted model parameters can be found in Supplementary Table S1 in the Supplementary Material). In addition, in many cases no EC25 values could be derived for thymol. The EC values were mostly smaller for carvacrol than for thymol (EC10 on average 90 times smaller; EC50 on average 5 times smaller), pointing to the fact that carvacrol had a stronger inhibitory effect than thymol, and this was also true for the duration of the adverse effect at the different incubation times tested. The EC values for thymol were smaller than for carvacrol only in individual cases, particularly in the case of β-glucosidase. As a result, thymol is not generally less effective than carvacrol. Rather, the small differences in the chemical structure and properties of the substances, which also affected sorption, apparently caused also an enzyme-specific difference in toxicity. In the environment, monoterpenes have been measured in soil grown with pine trees producing aromatic oils, with the highest concentrations up to 13.6 μg g−1 in the vicinity of roots for a single compound (α-pinene) and up to 15.9 μg g−1 for the sum of five monoterpenes analysed (Asensio et al., 2008). Monoterpenes can migrate up to 20 m laterally through soil; however, concentrations considerably decline with increasing distance (Lin et al., 2007; Asensio et al., 2008). Such field data are not available for thymol and carvacrol, but in comparison with the effect concentrations obtained in this study it can be assumed that EC10 and in some cases even EC25 can be reached in rhizosphere soil, which will then cause significant inhibition of individual enzyme activities. However, no stronger effects can be assumed; the soil concentrations reported by Asensio et al. (2008) do not reach the EC50 determined in this study.
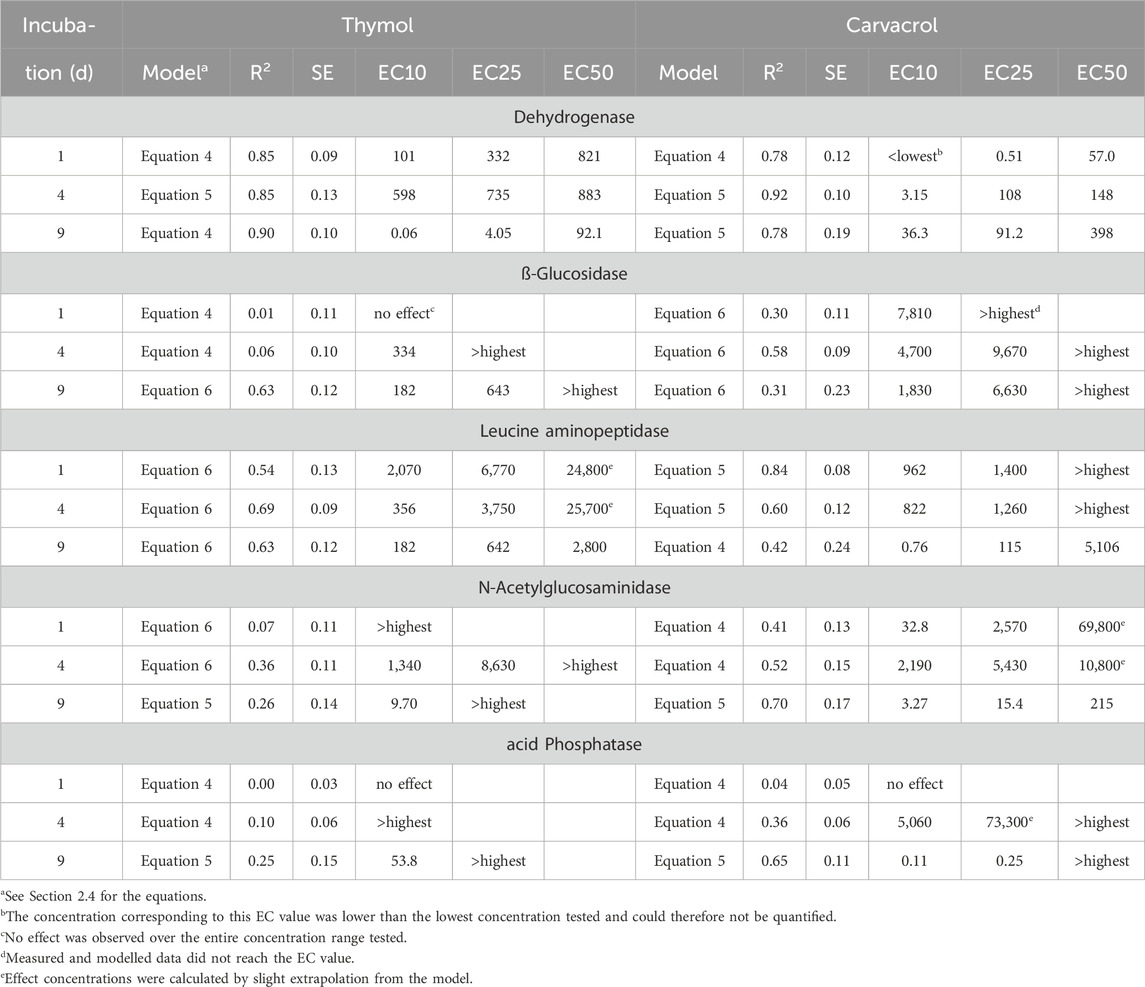
Table 5. Models used to fit the measured dose-response data, statistical parameters of the goodness of the curve fit (coefficient of determination R2, standard error SE), and derived effect concentrations (µg g−1) of 10% (EC10), 25% (EC25) and 50% (EC50) inhibition of five soil microbial enzyme activities.
The effect of the two monoterpenes always changed as the incubation period progressed. In seven out of ten cases investigated (Table 5), the effect increased with the extension of the incubation time from 1 to 4 and 9 days. This is illustrated by the example of N-acetylglucosaminidase (Figure 3A). While thymol had no significant effect over the entire concentration range after 1 day, carvacrol at a concentration of 10 μg g−1 even led to an increase in enzyme activity to approximately 130%. Only after 4 days did a significant reduction occur due to both monoterpenes at the highest concentration tested, whereas after 9 days significant reductions already occurred at concentrations of thymol and carvacrol ≥10 μg g−1 (Figure 3A). Such a time-dependent increasing inhibition of microbial activity is observed with antibiotics that are bacteriostatic and not bacteriotoxic (Thiele-Bruhn and Beck, 2005; Zhang et al., 2019). This is consistent with the reported antibacterial effect of monoterpenes (Fournomiti et al., 2015; Sakkas and Papadopoulou, 2017).
The result can also be explained by the fact that the substances bioaccumulate and therefore the concentrations in the soil microorganisms increase over time, a process that takes several days before the effective concentrations are exceeded. Arguments in favour of this interpretation are that this study has shown that soil sorption is relatively low and therefore the bioavailability of the monoterpenes might be quite high. In addition, the molecular sizes are small enough and the non-polarity high enough that bioaccumulation would be possible (Benz and Bauer, 1988; Nikaido, 1992; Pandey and Roy, 2021). However, this somewhat speculative interpretation requires further research.
In contrast, stimulatory effects at low soil concentrations were determined for the effect of carvacrol on both the N-acetylglucosaminidase and the dehydrogenase activity (Figure 3). At a spiking concentration of 10 and 1 μg g−1, respectively, the enzyme activity after 1 and 9 days, was increased to 130% of the activity in the control. Such an increase is typical of hormesis (Henschler, 2006; Calabrese, 2008), which is explained by excessive activity of microorganisms that compensate for an adverse effect. Hormesis has previously been reported for the effects of pharmaceutical antibiotics in soil (Thiele-Bruhn, 2005). It is also possible that the stimulation resulted from the utilisation of monoterpenes as a food substrate. Essential oils from aromatic plants were substantially decomposed in soil by 50% and up to 90% of the initial concentration within 30 days, demonstrating their degradability in principle (Karamanoli et al., 2018). However, the remaining fraction largely persisted during an extended incubation period of 1 year (Karamanoli et al., 2018). Furthermore, in the case of biodegradation, no increasing toxic effect over time would be expected, contrary to the results of this study. The range between stimulating and inhibiting effects shows the complexity of the possible effects of essential oils on the soil microbiome. This needs to be investigated further. On the other hand, although the effect of the aromatic plant on microbial activity in the soil was demonstrated in field trials using rosemary planting, the higher plant diversity in the arable system also led to a higher biodiversity of the soil microbiome (Barra Caracciolo et al., 2015).
4 Conclusion
In this study, the sorption and dose-dependent effects of selected monoterpenes in agricultural soils were investigated together for the first time. Parameters of sorption kinetics and strength as well as effect concentrations were determined. The results of the sorption experiments showed that although the two monoterpenes are sorbed to the soil very rapidly, a considerable fraction remains in solution, which is reflected in low sorption coefficients. Both thymol and carvacrol showed essentially the same second order sorption kinetics and the sorption isotherms followed the BET model in the same way. It can therefore be assumed that the results obtained in this study are also indicative of other monoterpenes. In detail, however, there were differences between thymol and carvacrol, which, although they are isomeric molecules, differ somewhat in sorption rate and strength. Based on the sorption experiments, a rather high mobility of the substances can be assumed. Considering the reported persistence of the essential oils and their constituents in soils of about 1 year, a considerable bioavailability can be assumed. This is reflected in the clear, dose- and time-dependent toxic effects of the two monoterpenes on various enzyme activities of the soil microbiome. The five soil microbial enzyme activities analysed in this study were not all inhibited by thymol and carvacrol and not to the same extent. This shows that the compounds, which are known to have antibiotic activity, do not have a general baseline toxicity but have specific effects. Effect concentrations of 10% and 25% inhibition of enzyme activity were in part in ranges that may occur in the environment, particularly in the rhizosphere of essential oil-producing plants. This may pose a risk of adverse effects, but may also have a phytosanitary effect. However, further research is needed to clarify this. It should also be noted that this study focussed on short-term experiments to determine the acute fate and effects of the selected monoterpenes. In agricultural practice, however, cultivation of aromatic plants over several years and thus additional, long-term effects of more complex terpene mixtures must be expected, which may be different from the reported short-term effects of single compounds. In any case, it should be noted that the ingredients of essential oils are active substances that exert effects on soil biota.
Data availability statement
The raw data supporting the conclusions of this article will be made available by the authors, without undue reservation.
Author contributions
ST-B: Conceptualization, Formal Analysis, Supervision, Writing–original draft, Writing–review and editing. VS: Formal Analysis, Investigation, Writing–original draft, Writing–review and editing. FD: Conceptualization, Validation, Writing–review and editing. DT: Data curation, Investigation, Writing–review and editing. MS: Data curation, Investigation, Writing–review and editing. ZG: Conceptualization, Funding acquisition, Supervision, Writing–review and editing.
Funding
The author(s) declare that financial support was received for the research, authorship, and/or publication of this article. Funding of VS was obtained through the Alexander von Humboldt foundation. Funding for FD was provided by the EU Horizon 2020 research programme as part of the DiverFarming project (grant agreement 728003).
Acknowledgments
We thank Petra Ziegler and Elvira Sieberger for their reliable laboratory work and Katharina Frey-Treseler and Cord-Henrich Treseler for supporting the field soil sampling. We acknowledge the collaboration with colleagues from the Department of Physical Geography, Trier University, on the DiverFarming project. We also thank two reviewers whose constructive comments helped to further improve the manuscript.
Conflict of interest
The authors declare that the research was conducted in the absence of any commercial or financial relationships that could be construed as a potential conflict of interest.
The author(s) declared that they were an editorial board member of Frontiers, at the time of submission. This had no impact on the peer review process and the final decision.
Publisher’s note
All claims expressed in this article are solely those of the authors and do not necessarily represent those of their affiliated organizations, or those of the publisher, the editors and the reviewers. Any product that may be evaluated in this article, or claim that may be made by its manufacturer, is not guaranteed or endorsed by the publisher.
Supplementary material
The Supplementary Material for this article can be found online at: https://www.frontiersin.org/articles/10.3389/fenvs.2024.1379018/full#supplementary-material
References
Adamczyk, S., Adamczyk, B., Kitunen, V., and Smolander, A. (2015). Monoterpenes and higher terpenes may inhibit enzyme activities in boreal forest soil. Soil Biol. Biochem. 87, 59–66. doi:10.1016/j.soilbio.2015.04.006
Asensio, D., Owen, S. M., Llusià, J., and Peñuelas, J. (2008). The distribution of volatile isoprenoids in the soil horizons around Pinus halepensis trees. Soil Biol. Biochem. 40 (12), 2937–2947. doi:10.1016/j.soilbio.2008.08.008
Asensio, D., Peñuelas, J., Llusià, J., Ogaya, R., and Filella, I. (2007). Interannual and interseasonal soil CO2 efflux and VOC exchange rates in a Mediterranean holm oak forest in response to experimental drought. Soil Biol. Biochem. 39 (10), 2471–2484. doi:10.1016/j.soilbio.2007.04.019
Barra Caracciolo, A., Bustamante, M. A., Nogues, I., Di Lenola, M., Luprano, M. L., and Grenni, P. (2015). Changes in microbial community structure and functioning of a semiarid soil due to the use of anaerobic digestate derived composts and rosemary plants. Geoderma 245-246, 89–97. doi:10.1016/j.geoderma.2015.01.021
Benz, R., and Bauer, K. (1988). Permeation of hydrophilic molecules through the outer membrane of gram-negativ bacteria: review of becterial porins. Eur. J. Biochem. 176 (1), 1–19. doi:10.1111/j.1432-1033.1988.tb14245.x
Blume, H.-P., Brümmer, G. W., Fleige, H., Horn, R., Kandeler, E., Kögel-Knabner, I., et al. (2015). Scheffer/Schachtschabel: soil science. Berlin, Heidelberg, Germany: Springer.
Broholm, M. M., Broholm, K., and Arvin, E. (1999). Sorption of heterocyclic compounds from a complex mixture of coal-tar compounds on natural clayey till. J. Contam. Hydrology 39 (3-4), 201–226. doi:10.1016/s0169-7722(99)00037-6
Brunauer, S., Emmett, P. H., and Teller, E. (1938). Adsorption of gases in multimolecular layers. J. Am. Chem. Soc. 60 (2), 309–319. doi:10.1021/ja01269a023
Cai, J., Gu, C., Ti, Q., Liu, C., Bian, Y., Sun, C., et al. (2019). Mechanistic studies of congener-specific adsorption and bioaccumulation of polycyclic aromatic hydrocarbons and phthalates in soil by novel QSARs. Environ. Res. 179, 108838. doi:10.1016/j.envres.2019.108838
Calabrese, E. J. (2008). Hormesis: why it is important to toxicology and toxicologists. Environ. Toxicol. Chem. 27 (7), 1451–1474. doi:10.1897/07-541
Chomel, M., Guittonny-Larchevêque, M., Fernandez, C., Gallet, C., DesRochers, A., Paré, D., et al. (2016). Plant secondary metabolites: a key driver of litter decomposition and soil nutrient cycling. J. Ecol. 104 (6), 1527–1541. doi:10.1111/1365-2745.12644
Chudasama, K. S., and Thaker, V. S. (2012). Screening of potential antimicrobial compounds against Xanthomonas campestris from 100 essential oils of aromatic plants used in India: an ecofriendly approach. Archives Phytopathology Plant Prot. 45 (7), 783–795. doi:10.1080/03235408.2011.595967
Colón, D., Weber, E. J., and Baughman, G. L. (2002). Sediment-associated reactions of aromatic amines. 2. QSAR development. Environ. Sci. Technol. 36 (11), 2443–2450. doi:10.1021/es0113551
Dhaundiyal, P., Bashir, S., Sharma, V., and Kumar, A. (2019). An investigation on mitigation of corrosion of mildsteel by Origanum vulgare in acidic medium. Bull. Chem. Soc. Ethiop. 33 (1), 159–168. doi:10.4314/bcse.v33i1.16
Di Bene, C., Francaviglia, R., Farina, R., Álvaro-Fuentes, J., and Zornoza, R. (2022). Agricultural diversification. Agriculture 12 (3), 369. doi:10.3390/agriculture12030369
Dittrich, F., Iserloh, T., Treseler, C. H., Hüppi, R., Ogan, S., Seeger, M., et al. (2021). Crop diversification in viticulture with aromatic plants: effects of intercropping on grapevine productivity in a steep-slope vineyard in the mosel area, Germany. Agric. Switz. 11 (2), 1–15. doi:10.3390/agriculture11020095
Duke, J. A. (2016). Dr. Duke's phytochemical and ethnobotanical databases. Ag data commons. Dataset. U.S. Department of Agriculture. doi:10.15482/USDA.ADC/1239279
Ehlers, B. K. (2011). Soil microorganisms alleviate the allelochemical effects of a thyme monoterpene on the performance of an associated grass species. PLoS ONE 6 (11), e26321. doi:10.1371/journal.pone.0026321
Ernst, G., Henseler, I., Felten, D., and Emmerling, C. (2009). Decomposition and mineralization of energy crop residues governed by earthworms. Soil Biol. Biochem. 41 (7), 1548–1554. doi:10.1016/j.soilbio.2009.04.015
Ferrario, V. (2021). Learning from agricultural heritage? Lessons of sustainability from Italian “coltura promiscua”. Sustain. Switz. 13 (16), 8879. doi:10.3390/su13168879
Fournomiti, M., Kimbaris, A., Mantzourani, I., Plessas, S., Theodoridou, I., Papaemmanouil, V., et al. (2015). Antimicrobial activity of essential oils of cultivated oregano (Origanum vulgare), sage (Salvia officinalis), and thyme (Thymus vulgaris) against clinical isolates of Escherichia coli, Klebsiella oxytoca, and Klebsiella pneumoniae. Microb. Ecol. health Dis. 26, 23289. doi:10.3402/mehd.v26.23289
Gámiz, B., Hermosín, M. C., and Celis, R. (2018). Appraising factors governing sorption and dissipation of the monoterpene carvone in agricultural soils. Geoderma 321, 61–68. doi:10.1016/j.geoderma.2018.02.005
Hale, S. E., Endo, S., Arp, H. P. H., Zimmerman, A. R., and Cornelissen, G. (2015). Sorption of the monoterpenes α-pinene and limonene to carbonaceous geosorbents including biochar. Chemosphere 119, 881–888. doi:10.1016/j.chemosphere.2014.08.052
Hayward, S., Muncey, R. J., James, A. E., Halsall, C. J., and Hewitt, C. N. (2001). Monoterpene emissions from soil in a Sitka spruce forest. Atmos. Environ. 35 (24), 4081–4087. doi:10.1016/s1352-2310(01)00213-8
Henschler, D. (2006). The origin of hormesis: historical background and driving forces. Hum. Exp. Toxicol. 25 (7), 347–351. doi:10.1191/0960327106ht642oa
Heumann, S., Rimmer, D. L., Schlichting, A., Abbott, G. D., Leinweber, P., and Böttcher, J. (2013). Effects of potentially inhibiting substances on C and net N mineralization of a sandy soil-a case study. J. Plant Nutr. Soil Sci. 176 (1), 35–39. doi:10.1002/jpln.201200353
IUSS Working Group WRB (2022). World Reference Base for Soil Resources. International soil classification system for naming soils and creating legends for soil maps. 4th ed. Vienna, Austria: International Union of Soil Sciences IUSS.
Kamgaing, T., Doungmo, G., Melataguia Tchieno, F. M., Gouoko Kouonang, J. J., and Mbadcam, K. J. (2017). Kinetic and isotherm studies of bisphenol A adsorption onto orange albedo(Citrus sinensis): sorption mechanisms based on the main albedo components vitamin C, flavones glycosides and carotenoids. J. Environ. Sci. Health–Part A Toxic/Hazardous Subst. Environ. Eng. 52 (8), 757–769. doi:10.1080/10934529.2017.1303315
Karamanoli, K., Ainalidou, A., Bouzoukla, F., and Vokou, D. (2018). Decomposition profiles of leaf essential oils in the soil environment. Industrial Crops Prod. 124, 397–401. doi:10.1016/j.indcrop.2018.07.082
Katsoulis, G. I., Kimbaris, A. C., Anastasaki, E., Damalas, C. A., and Kyriazopoulos, A. P. (2022). Chamomile and anise cultivation in olive agroforestry systems. Forests 13 (1), 128. doi:10.3390/f13010128
Langenheim, J. H. (1994). Higher plant terpenoids: a phytocentric overview of their ecological roles. J. Chem. Ecol. 20 (6), 1223–1280. doi:10.1007/bf02059809
Lawal, A. A., Hassan, M. A., Ahmad Farid, M. A., Tengku Yasim-Anuar, T. A., Samsudin, M. H., Mohd Yusoff, M. Z., et al. (2021). Adsorption mechanism and effectiveness of phenol and tannic acid removal by biochar produced from oil palm frond using steam pyrolysis. Environ. Pollut. 269, 116197. doi:10.1016/j.envpol.2020.116197
Leff, J. W., and Fierer, N. (2008). Volatile organic compound (VOC) emissions from soil and litter samples. Soil Biol. Biochem. 40 (7), 1629–1636. doi:10.1016/j.soilbio.2008.01.018
Li, S., Li, H., Zhou, Q., Zhang, F., Desneux, N., Wang, S., et al. (2022). Essential oils from two aromatic plants repel the tobacco whitefly Bemisia tabaci. J. Pest Sci. 95 (2), 971–982. doi:10.1007/s10340-021-01412-0
Lin, C., Owen, S., and Penuelas, J. (2007). Volatile organic compounds in the roots and rhizosphere of Pinus spp. Soil Biol. Biochem. 39, 951–960. doi:10.1016/j.soilbio.2006.11.007
Mahdavikia, F., and Saharkhiz, M. J. (2015). Phytotoxic activity of essential oil and water extract of peppermint (Mentha × piperita L. CV. Mitcham). J. Appl. Res. Med. Aromatic Plants 2 (4), 146–153. doi:10.1016/j.jarmap.2015.09.003
Mantzanas, K., Pantera, A., Koutsoulis, D., Papadopoulos, A., Kapsalis, D., Ispikoudis, S., et al. (2021). Intercrop of olive trees with cereals and legumes in Chalkidiki, Northern Greece. Agrofor. Syst. 95 (5), 895–905. doi:10.1007/s10457-021-00618-6
Marei, G. I., Abdel Rasoul, M. A., and Abdelgaleil, S. A. M. (2012). Comparative antifungal activities and biochemical effects of monoterpenes on plant pathogenic fungi. Pesticide Biochem. Physiology 103 (1), 56–61. doi:10.1016/j.pestbp.2012.03.004
Marotti, I., Whittaker, A., Bağdat, R. B., Akin, P. A., Ergün, N., and Dinelli, G. (2023). Intercropping perennial fruit trees and annual field crops with aromatic and medicinal plants (MAPs) in the mediterranean basin. Sustain. Switz. 15 (15), 12054. doi:10.3390/su151512054
Marx, M. C., Wood, M., and Jarvis, S. C. (2001). A microplate fluorimetric assay for the study of enzyme diversity in soils. Soil Biol. Biochem. 33 (12-13), 1633–1640. doi:10.1016/s0038-0717(01)00079-7
Mrozik, W., Minofar, B., Thongsamer, T., Wiriyaphong, N., Khawkomol, S., Plaimart, J., et al. (2021). Valorisation of agricultural waste derived biochars in aquaculture to remove organic micropollutants from water – experimental study and molecular dynamics simulations. J. Environ. Manag. 300, 113717. doi:10.1016/j.jenvman.2021.113717
Nikaido, H. (1992). Porins and specific channels of bacterial outer membranes. Mol. Microbiol. 6 (4), 435–442. doi:10.1111/j.1365-2958.1992.tb01487.x
Ockleford, C., Adriaanse, P., Berny, P., Brock, T., Duquesne, S., Grilli, S., et al. (2017). EFSA PPR Panel (EFSA Panel on Plant Protection Products and their Residues) Scientific Opinion addressing the state of the science on risk assessment of plant protection products for in-soil organisms. EFSA J. 15 (2), e04690. doi:10.2903/j.efsa.2017.4690
OECD (2000). Test No. 106: Adsorption—desorption using a batch equilibrium method, OECD guidelines for the testing of chemicals, section 1. Paris: OECD Publishing. doi:10.1787/9789264069602
Pandey, S. K., and Roy, K. (2021). QSPR modeling of octanol-water partition coefficient and organic carbon normalized sorption coefficient of diverse organic chemicals using Extended Topochemical Atom (ETA) indices. Ecotoxicol. Environ. Saf. 208, 111411. doi:10.1016/j.ecoenv.2020.111411
Pérez-Guzmán, L., Phillips, L. A., Acevedo, M. A., and Acosta-Martínez, V. (2021). Comparing biological methods for soil health assessments: EL-FAME, enzyme activities, and qPCR. Soil Sci. Soc. Am. J. 85 (3), 636–653. doi:10.1002/saj2.20211
Pignatello, J. J. (2011). “Interactions of anthropogenic organic chemicals with natural organic matter and black carbon in environmental particles,” in Biophysico-chemical processes of anthropogenic organic compounds in environmental systems, 1–50.
Qasemi, M., Afsharnia, M., Zarei, A., Najafpoor, A. A., Salari, S., and Shams, M. (2018). Phenol removal from aqueous solution using Citrullus colocynthis waste ash. Data Brief 18, 620–628. doi:10.1016/j.dib.2018.03.049
Sakkas, H., and Papadopoulou, C. (2017). Antimicrobial activity of basil, oregano, and thyme essential oils. J. Microbiol. Biotechnol. 27 (3), 429–438. doi:10.4014/jmb.1608.08024
Sharma, G., Shrestha, S., Kunwar, S., and Tseng, T. M. (2021). Crop diversification for improved weed management: a review. Agric. Switz. 11 (5), 461. doi:10.3390/agriculture11050461
Sparks, T. C., Hahn, D. R., and Garizi, N. V. (2017). Natural products, their derivatives, mimics and synthetic equivalents: role in agrochemical discovery. Pest Manag. Sci. 73 (4), 700–715. doi:10.1002/ps.4458
Tang, Z., Johal, M. S., Scudder, P., Caculitan, N., Magyar, R. J., Tretiak, S., et al. (2007). Study of the non-covalent interactions in Langmuir-Blodgett films: an interplay between π-π and dipole-dipole interactions. Thin Solid Films 516 (1), 58–66. doi:10.1016/j.tsf.2007.04.149
Thiele-Bruhn, S. (2005). Microbial inhibition by pharmaceutical antibiotics in different soils–dose-response relations determined with the iron(III) reduction test. Environ. Toxicol. Chem. 24 (7), 869–876. doi:10.1897/04-166r.1
Thiele-Bruhn, S., and Beck, I. C. (2005). Effects of sulfonamide and tetracycline antibiotics on soil microbial activity and microbial biomass. Chemosphere 59, 457–465. doi:10.1016/j.chemosphere.2005.01.023
Van Roon, A., Parsons, J. R., Krap, L., and Govers, H. A. J. (2005). Fate and transport of monoterpenes through soils. Part II: calculation of the effect of soil temperature, water saturation and organic carbon content. Chemosphere 61 (1), 129–138. doi:10.1016/j.chemosphere.2005.02.082
von Mersi, W., and Schinner, F. (1991). An improved and accurate method for determining the dehydrogenase activity of soils with iodonitrotetrazolium chloride. Biol. Fertil. Soils 11 (3), 216–220. doi:10.1007/bf00335770
White, C. S. (1991). The role of monoterpenes in soil nitrogen cycling processes in ponderosa pine–results from laboratory bioassays and field studies. Biogeochemistry 12 (1), 43–68. doi:10.1007/bf00002625
Wooliver, R., Kivlin, S. N., and Jagadamma, S. (2022). Links among crop diversification, microbial diversity, and soil organic carbon: mini review and case studies. Front. Microbiol. 13, 854247. doi:10.3389/fmicb.2022.854247
Xie, Y. J., Xie, Y. H., Xiao, H. Y., Deng, Z. M., Pan, Y., Pan, B. H., et al. (2017). Inhibition of litter decomposition of two emergent macrophytes by addition of aromatic plant powder. Sci. Rep. 7 (1), 16685. doi:10.1038/s41598-017-16615-8
Xing, B., and Pignatello, J. J. (1997). Dual-mode sorption of low-polarity compounds in glassy poly(vinyl chloride) and soil organic matter. Environ. Sci. Technol. 31, 792–799. doi:10.1021/es960481f
Yadav, D., Yadav, A., Singh, M., and Khare, P. (2023). Cultivation of aromatic plant for nature-based sustainable solutions for the management of degraded/marginal lands: techno-economics and carbon dynamic. Carbon Res. 2 (1), 27. doi:10.1007/s44246-023-00055-3
Yalkowsky, S. H., and Dannenfelser, R. M. (1992). Aquasol database of aqueous solubility. – version 5, college of pharmacy. Tucson, AZ: University of Arizona.
Zabala, J. A., Martínez-García, V., Martínez-Paz, J. M., López-Becerra, E. I., Nasso, M., Díaz-Pereira, E., et al. (2023). Crop diversification practices in Europe: an economic cross-case study comparison. Sustain. Sci. 18 (6), 2691–2706. doi:10.1007/s11625-023-01413-1
Zhang, W., Wang, J., Wang, J., Zhu, L., Lv, N., Wang, R., et al. (2019). New insights into dose- and time-dependent response of five typical PPCPs on soil microbial respiration. Bull. Environ. Contam. Toxicol. 103 (1), 193–198. doi:10.1007/s00128-019-02655-5
Zhang, Y., Han, M., Song, M., Tian, J., Song, B., Hu, Y., et al. (2021). Intercropping with aromatic plants increased the soil organic matter content and changed the microbial community in a pear orchard. Front. Microbiol. 12, 616932. doi:10.3389/fmicb.2021.616932
Zhu, P., Chen, Y., Fang, J., Wang, Z., Xie, C., Hou, B., et al. (2016). Solubility and solution thermodynamics of thymol in six pure organic solvents. J. Chem. Thermodyn. 92, 198–206. doi:10.1016/j.jct.2015.09.010
Keywords: volatile isoprenoids, antibiotic, dose-response relationships, effect concentrations, toxicity, sorption kinetics, sorption isotherms
Citation: Thiele-Bruhn S, Shikuku V, Dittrich F, Torjir DN, Saini M and Getenga Z (2024) Soil sorption and effects on soil microorganisms of thymol and carvacrol monoterpenes from essential oils of aromatic plants. Front. Environ. Sci. 12:1379018. doi: 10.3389/fenvs.2024.1379018
Received: 30 January 2024; Accepted: 10 July 2024;
Published: 26 July 2024.
Edited by:
Martin Siegert, University of Exeter, United KingdomReviewed by:
Robert A. Root, University of Arizona, United StatesKaterina Dontsova, University of Arizona, United States
Copyright © 2024 Thiele-Bruhn, Shikuku, Dittrich, Torjir, Saini and Getenga. This is an open-access article distributed under the terms of the Creative Commons Attribution License (CC BY). The use, distribution or reproduction in other forums is permitted, provided the original author(s) and the copyright owner(s) are credited and that the original publication in this journal is cited, in accordance with accepted academic practice. No use, distribution or reproduction is permitted which does not comply with these terms.
*Correspondence: Sören Thiele-Bruhn, thiele@uni-trier.de