- 1Department of Biotechnology, IIT Madras, Chennai, Tamil Nadu, India
- 2Department of Science, Vidyadayini Institute of Science, Management, and Technology, Bhopal, India
- 3Department of Agronomy, College of Agriculture, G.B.P.U.A & T, Pantnagar, Uttarakhand, India
- 4ICAR Research Complex for Eastern Region, Patna, Bihar, India
- 5Department of Biotechnology, G. L. A. University, Mathura, Uttar Pradesh, India
This study aimed to document the effects of the long-term organic farming (OF) on soil quality, agronomical parameters, crop productivity, and food grain yield compared to the conventional farming (CF) system. The crop used in this study is chickpea (Cicer arietinum), and the field was located at Pantnagar, India, in the foothills of Himalayas. The organic farming approach involved utilizing a blend of farmyard manure and vermicompost, combined with a biopesticide comprising neem oil and cow urine. Chickpea grain micronutrient analysis was done via atomic absorption spectrophotometry. It was found that the physicochemical properties of soil in the organic plot were improved compared to the conventional counterpart. At the post-harvesting stage, the organically managed field had higher soil organic carbon than the conventional field (OF-0.93± 0.05%, CF-0.75 ± 0.12%), higher available nitrogen (OF-317 ± 11 kg/ha, CF-240 ± 22 kg/ha), and more available phosphorus (OF-37.4 ± 1.3 kg/ha, CF-25.2 ± 2.5 kg/ha). The agronomical parameters of the chickpea crop were better under organic cultivation, with a significantly high nodule number, nodule dry weight, and grains per pod. Hence, the grain yield of the crop was better under organic cultivation, with the yield of 1,048 kg ha−1, whereas it was 896.5 kg ha−1 for conventional cultivation. The Fe and Zn contents of organically produced chickpea grains were almost double of their conventional counterpart. Therefore, organic cultivation led to better soil fertility, chickpea grain yield, and nutrient status of the crop. It will be beneficial for the nutritious and sustainable production of chickpeas in Himalayan regions.
1 Introduction
Currently, agriculture occupies 38% of Earth’s land cover and is expected to feed a growing population worldwide, which is projected to reach 9.7 billion by 2050 (UN DESA, 2022). It places substantial stress on existing natural resources such as soil, water, and biodiversity, a pressure that is destined to escalate with the continual increase in the human population. Another factor that is forcing the conventional agriculture system to change is climate change. Hence, organic farming has emerged as the need of this hour for providing safe as well as healthy food and long-term agricultural sustainability (Christel et al., 2021; Montgomery and Biklé, 2021).
Organic agriculture comprises “management procedures that work with natural processes to conserve all resources, minimize waste and environmental impact and promote agroecosystem resilience” (Rees et al., 2018; Symochko et al., 2024). The Himalayan Foothills, characterized by its diverse topography and fragile ecosystem, presents a compelling setting for investigating the impact of organic farming on agricultural sustainability. A large number of people in such regions live in rural areas with fragmented and small land holdings. These farmers face issues such as water scarcity, soil erosion, and other natural calamities. Therefore, the majority of the rural population in the hills either survives on subsistence agriculture or migrates for better livelihood opportunities (Bisht, 2021). The low-input nature of organic agriculture and use of easily available natural resources such as dung and urine from livestock make it easier for these small-holder farmers to adopt organic farming. However, there are major deterrents like high organic certification charges, initial yield loss, and a low price for the organic produce (Haneef et al., 2019). Hence, the promotion of techniques used in organic agriculture in these regions by agriculture extension services would be beneficial.
Chickpea (Cicer arietinum) is one of the most important rabi pulse crops in the world and is grown mainly in South Asia and Sub-Saharan Africa (Thudi et al., 2017). India is the largest producer of chickpea in the world, with 65.2% share in area and 65.4% share in production (Dixit, 2021). In India, the scenario of legume farming is very different as compared to that of other major crops like wheat and rice. Small and marginal farmers produce legumes and have a poor resource base (Akpo et al., 2021), as 43.6 percent of chickpea farmers in India are smallholder farmers (FAO, 2018). Agrochemicals used in conventional agriculture are expensive, whereas organic fertilizers are innate substances of either animal or plant origin, such as livestock manure, green manure, and crop residues. Being a waste-to-wealth strategy, it reduces the burden of agricultural production from the financial perspective. Furthermore, our previous study has revealed the suppression of plant and animal pathogens in organically managed soils (Suyal et al., 2021). Hence, spreading awareness about organic farming among these farmers can provide them with a low-input and cost-effective alternative. Therefore, a comparative study of conventional and organic agriculture systems was carried out, with chickpea as a candidate crop. The objective of this study was to understand the organic farming systems in the context of soil health, grain yield, and crop agronomical parameters in the unique agro-ecological zone of the Himalayan Foothills.
2 Materials and methods
2.1 Experimental site
The experimental site was situated within the organic farming block at the Breeder Seed Production Center (BSPC) of G.B. Pant University of Agriculture and Technology, Pantnagar, India (29.5° N/79.30° E). This field experiment was conducted on Pant Kabuli Chana-1 variety of chickpea. The soil of the experimental plot taxonomically belongs to the order Mollisol, sub order Udoll, and great group–Hapludoll (USDA classification). The soil is silty clay loam in texture.
The field had been under the described conventional and organic management for past 8 years at the time of this study. The detailed weather conditions of the experimental site and field layout are given in Supplementary Material (Supplementary Figures S1, S2). For the present study, the chickpea plots treated with organic and conventional methods were considered. Two plots (165 m2 each) were under organic management and two plots under conventional management. The treatment details are given in Table 1.
2.2 Soil and plant sampling and analysis
Soil samples were collected in triplicate from the depth of 0–15 cm at the pre-sowing and post-harvesting stages. The samples were taken at equal distances along the diagonal of the field. The soil samples were not collected from the same locations at the two stages. The following parameters were calculated using the respective protocols: soil pH, soil organic carbon (Walkley and Black, 1934), available nitrogen (Subbiah and Asija, 1956), available phosphorus (Olsen, 1954), available potassium, and available soil micronutrients such as Fe, Zn, Cu, and Mn (Lindsay and Norvell, 1978).
Five plants per treatment were collected from both organically and conventionally managed fields. The plants were collected at equal distances along the diagonal of the field. The plant sampling was done twice: during the flowering stage (for root and shoot length, plant fresh weight, plant dry weight, nodule numbers, nodule dry weight, and number of branches) and the harvesting stage of chickpea (for no. of pods per plant, no. of grains per pod, weight per 100 grains, and numbers of plants per sq. m.). The yield in kilograms per hectare (kg ha-1) was recorded after the harvesting of the crop.
2.3 Chickpea grain micronutrient content
The analysis was done using the slightly modified wet oxidation method of Zasoski and Burau (1977). Chickpea grains were dried at 70°C for 48 h in an oven. Dried grains were powdered and passed through a 0.5-mm sieve. A dried grain sample (0.2 g) was mixed with 5 mL of a digestion acid mixture (mixture of perchloric acid: nitric acid in the ratio 4:9) and kept overnight at room temperature. The digestion was carried out on a hot plate at 80–90°C for 1 h until vapors stopped coming. Then, the temperature was increased to 180–200°C, and the digestion was carried out until a clear/yellowish solution was obtained (1–2 h). The obtained extract was filtered using Whatman filter paper 1 and then diluted up to 50 mL. The sample filtrates were analyzed via atomic absorption spectrophotometry. For the analysis, the instrument was calibrated using standard solutions of respective micronutrients bought from PerkinElmer. The concentration of micronutrients was calculated as follows: concentration of element in grain (ppm) = reading (in ppm) x dilution factor.
2.4 Statistical analysis
The values of above parameters were expressed as mean ± SEM. Student’s t-test (p ≤ 0.05) was carried out using SPSS software to assess any significant variation between the means of traits under the organic and conventional modes of crop production.
3 Results
A significant difference was found in the soil pH of organic and conventional soils. Before sowing, the pH of organically managed soil was near neutral, i.e., 6.9, while the pH of conventional soil was slightly alkaline, i.e., 7.7 (p < 0.00095) (Figure 1). After crop harvesting, the pH of the organically managed soil decreased to 6.4, while that of conventional soil increased to 7.9 (p < 0.00095).
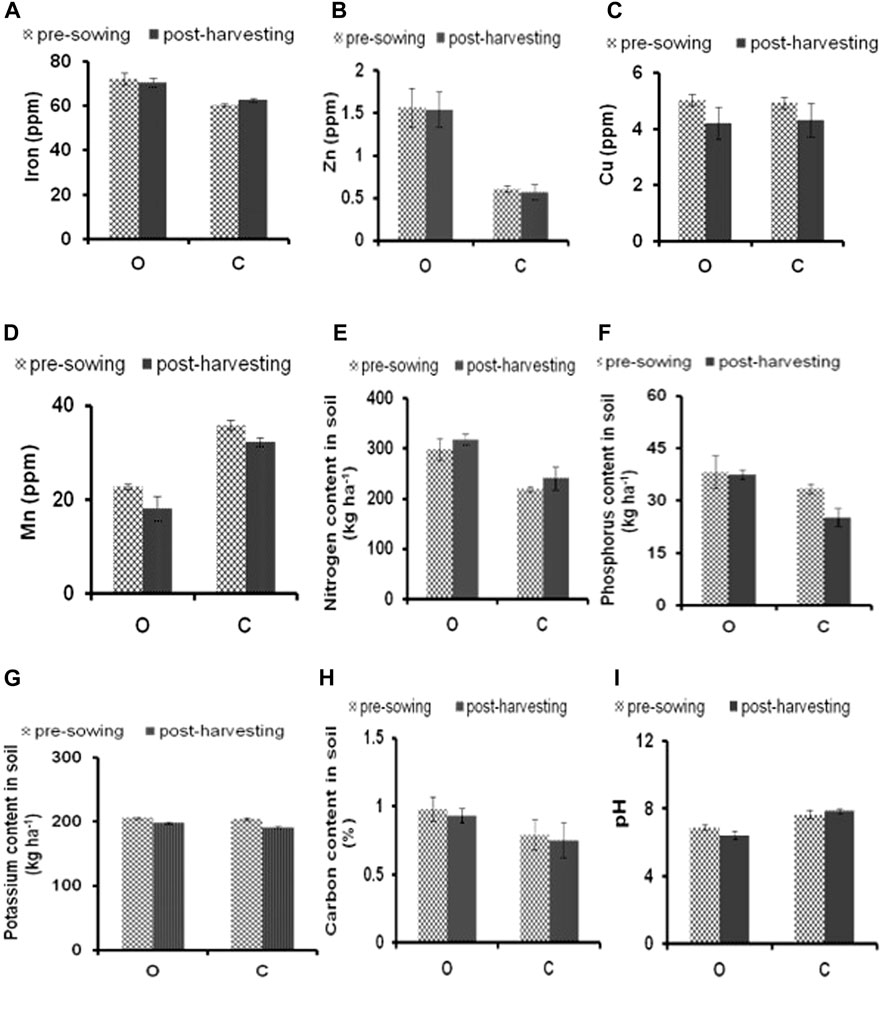
Figure 1. Physicochemical parameters of organic (O) and conventionally (C) managed soils at the pre-sowing and post-harvesting stages. Iron concentration (A); zinc concentration (B); copper concentration (C); manganese concentration (D); available nitrogen concentration (E); available phosphorus concentration (F); available potassium concentration (G); organic carbon concentration (H); and pH (I).
3.1 Soil-derived micronutrients
There was a significant difference in the level of iron present in organic and conventional soils at both stages. The iron content of organically managed soil was 72.25 and 70.58 ppm at the pre-sowing and post-harvesting stages, respectively (Figure 1). The iron content of conventional soil was 60.53 ppm (pre-sowing) and 62.72 ppm (post-harvesting), which was significantly lower than that of organically managed soil (p = 0.0001). However, none of the soils were deficient in iron. Zinc content was sufficient in organically managed soil (1.56 ppm). However, conventional soil was deficient with 0.61 ppm of zinc (Figure 3B). At both stages, zinc was significantly higher in the organically managed soil (p = 0.00014).
The copper content in both the soils was high. At the pre-sowing stage, the copper content of organically managed soil was 5.03 ppm and that of conventional soil was 4.93 ppm. There was no significant difference between both the soils in terms of copper content (p = 0.189) (Figure 1). Furthermore, the manganese content of both the soils was high. It was significantly higher (p < 0.05) for conventional soil at both the stages. At the pre-sowing state, manganese content for conventional soil was 35.83 ppm, and for organically managed soil, it was 22.8 ppm (p = 0.000015). At the post-harvesting stage, it was 32.29 ppm (conventional treatment) and 18.14 ppm (organic treatment) (p = 0.00006) (Figure 1).
3.2 Soil-derived macronutrients
The SOC at the pre-sowing stage was significantly higher (p = 0.002) for the organic plots with 0.98% organic carbon than for the conventional plots with 0.79% of organic carbon. After harvesting, a similar trend was observed in both the treatments, with SOC of the organically managed soil at 0.93% and that of conventional soil at 0.75% (p = 0.0002) (Supplementary Table S1). In the case of available phosphorus, it was 38.6 kg ha−1 during the pre-sowing stage, for organically managed soil and 37.4 kg ha−1 after harvesting. Contrary to that, the available phosphorus was 33.47 kg ha−1 in conventional soil, which decreased to 25.2 kg ha−1 after harvesting. Furthermore, the available phosphorus was significantly lower (p = 0.0245) in conventional soil than in organically managed soil at both pre-sowing and post-harvesting stages (Figure 1).
The available nitrogen in the pre-sowing stage soil was 298 kg ha−1 (medium) for organically managed soil and 219.3 kg ha−1 (low) for conventional soil (Supplementary Table S2). After harvesting, the available nitrogen increased in both the soil systems. It increased to 317.3 kg ha−1 for organically managed soil and 240.3 kg ha−1 for conventional soil. However, at both the stages, conventional soil had significantly lower (p = 0.0002) nitrogen content than the organically managed soil (Figure 1). Similarly, the available potassium content in both the soils was in the medium range. At the pre-sowing stage in organically managed soil, it was 205.96 kg ha−1, and in conventional soil, it was 204.5 kg ha−1 (p = 0.0245). After harvesting, there was a slight decrease in the available potassium content of both soils; it was 197.7 kg ha−1 for organically managed soil and 191.1 kg ha−1 in conventional soil (p = 0.0245) (Supplementary Table S3). There was no significant difference observed between both the soils in terms of “available potassium” content.
3.3 Agronomical parameters of the crop
The root length and shoot length of organic chickpea plants were found to be higher than those of conventional plants (Figures 2A, B). However, the results were not statistically significant (p < 0.05). Furthermore, the dry weight for organic and conventional plants was 3.21 g and 1.63 g, respectively (Figure 2C). There was a significant difference (p < 0.05) in dry weight and fresh weight of the plants. The nodule numbers per plant for organic plants (10.6) were significantly higher than those of conventional plants (8.2) (Figure 2D). Similarly, the nodule dry weight (mg/plant) was also significantly higher for organic plants (52.42 mg) than their counterparts (39.08 mg) (Figure 2E).
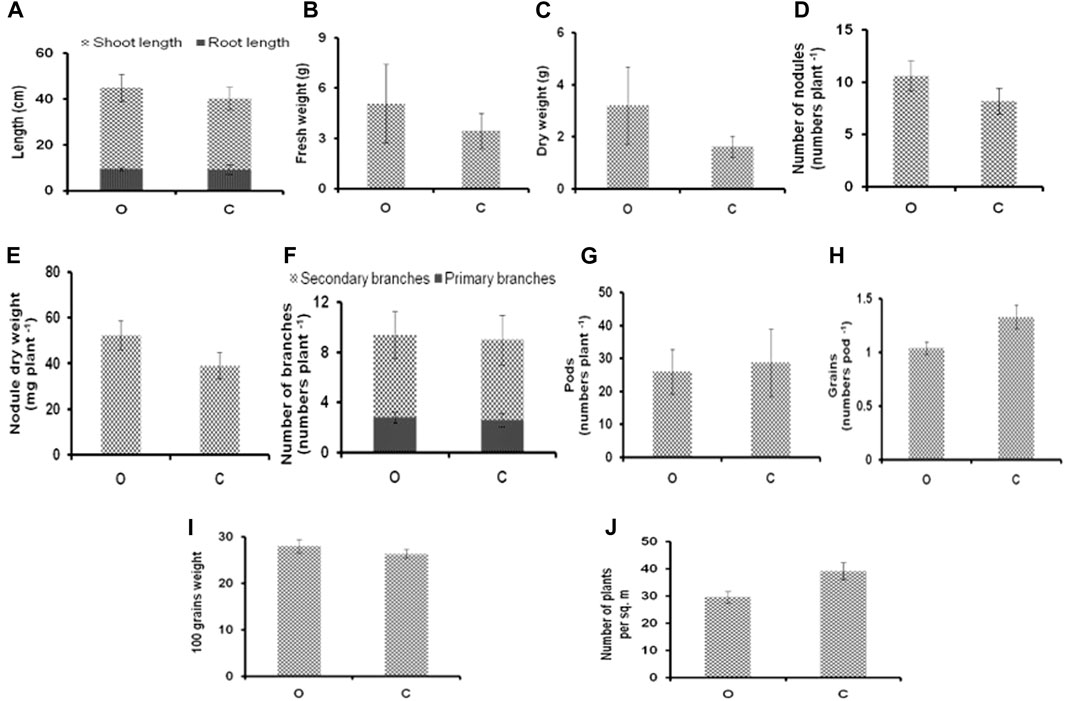
Figure 2. Agronomical parameters of organically (O) and conventionally (C) grown chickpea at flowering and harvesting stages: root and shoot length (A); plant fresh weight (B); plant dry weight (C); nodule numbers (D); nodule dry weight (E); number of branches (F); no. of pods per plant (G) no. of grains per pod (H); weight per 100 grains (I); and numbers of plants per sq. m. (J).
The number of primary and secondary branches counted at the harvesting stage was slightly higher for organic plants (Figure 2F). Nevertheless, these differences were statistically not significant (p < 0.05) (Supplementary Table S4). Furthermore, the yield components like number of pods per plant, number of grains per pod, and 100 grain weight were higher for organically grown plants (Figure 2G). Number of grains per pod was significantly higher for organically grown plants, with 1.33 grains per pod, while the conventional plants had 1.04 grains per pod (Figure 2H). In addition, 100 grain weight of the organically produced chickpea (26.92 g) was also higher than that of the conventionally produced chickpea (26.57 g), thereby indicating the larger seed size of organic grains (Figure 2I). Erdemci et al. (2017) reported that there was a positive correlation between seed weight and germination percentage in chickpeas, as the larger seeds germinated earlier than smaller seeds. Results showed that the number of plants per square meter was higher in the organic plot, with 29.6 plants per sq. m., as compared to the conventional plot, which had 29.4 plants per sq. m (Figure 2J). However, it was not statistically significant (p < 0.05). The seed yield for the organic plot was 1,048 kg ha−1, whereas it was 896.5 kg ha-1 for the conventional plot. This difference was statistically significant (p < 0.05).
3.4 Micronutrient content of chickpea grain
There was a remarkable difference between the organically produced grains with 72 ppm zinc content and the conventionally produced grains with 42.6 ppm zinc content. The zinc content of organic grains was significantly higher (p < 0.05) than that of conventional grains (Figure 3A) (Supplementary Table S4). The iron content of organic chickpea grain was 212.3 ppm, which was significantly higher (p < 0.05) than that of conventional chickpea grain at 106.3 ppm (Figure 3B). The results are in agreement with those of a study by Gopinath et al. (2016), in which they found that organic foods were richer in iron as opposed to inorganic foods.
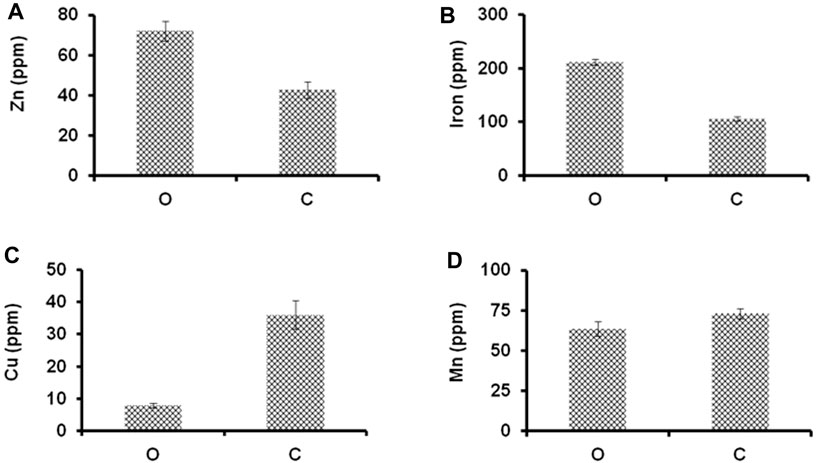
Figure 3. Effects of organic (O) and conventional (C) agricultural systems on zinc (A); iron (B); copper (C); and manganese (D) content of chickpea grains.
The copper content of conventionally produced grains was five times more than that of organically produced grains. The copper content of conventional grain was 36 ppm, and that of organic grain was 7.9 ppm (Figure 3C). Furthermore, there was a significant difference between the manganese content in the grains produced by organic agricultural practices and grains produced by conventional agricultural practices. The trend was similar to that of soil manganese content, i.e., organically produced grains had lower manganese content (63.4 ppm) than the conventionally produced grains (73.1 ppm) (Figure 3D).
4 Discussion
The intensification of agriculture, while considered a vital requirement for sustaining the nourishment of the expanding global population, is an intricate matter. It encompasses various complexities and challenges, including environmental degradation from increased chemical inputs and land-use changes (Gomiero et al., 2011), pesticide resistance (Bras et al., 2022), pesticide residues in food products (Geissen et al., 2021), and increased greenhouse gas emissions (Venkat, 2012; Li et al., 2022). Given the indispensable role of agriculture in human survival, continuous enhancements are necessary to align it with environmental sustainability. Organic farming, distinguished by its reliance on minimal external inputs and reduced environmental footprint, offers a promising alternative. India, boasting four biodiversity hotspots including the Himalayas, faces a unique responsibility (Walter and Gillett, 1998). Implementing sustainable farming practices in these ecologically rich regions, viz., the Himalayan Foothills, becomes inevitable to protect and preserve the diverse and fragile ecosystems harbored by them. It has been well-established that native communities of soil microorganisms are adversely affected by conventional agricultural practices like manure application and conventional tillage (Hartman et al., 2018; Khan et al., 2023).
Overall, in this study, the chemical properties of organically managed soil were better in comparison with those of their conventional counterpart. Soil pH of organically managed soil was slightly acidic. This slight acidification of the organically managed soil can be attributed to organic amendments like farmyard manure and vermicompost. It is because soil organic matter upon mineralization releases acids that lower the soil pH (Dhaliwal et al., 2023). Contrary to that, nitrate-based chemical fertilizers may be responsible for increasing the pH of conventional soil, which is slightly alkaline (Zilio et al., 2020). Previously, it has been observed that the pH range of 6–7 is ideal for autotrophic nitrifying and ammonia-oxidizing bacteria (Liang et al., 2017). Therefore, organically managed soil is better suited for agriculturally important microbial groups.
Furthermore, soil organic carbon (SOC) serves as a crucial indicator in assessing soil wellbeing, playing a role in both the physical structure and chemical characteristics of the soil. Additionally, it enhances the soil’s capacity to retain water. The increase in soil organic matter has a positive impact on soil microbiota, attributed to the increased availability of energy sources, particularly organic carbon (Liang et al., 2017). The present study suggests that employing organically managed soil management methods is more effective in enhancing soil organic carbon levels. Earlier studies showed that soil phosphorus availability increases with the increase in organic matter in the soil because such soils have higher biological activity (Bhat et al., 2017). The results are consistent with this observation, as in the organically managed soil, both SOC and available phosphorus were significantly higher than those in the conventionally managed soil. Zinc deficiency in soils is the most common micronutrient deficiency worldwide (Suhr et al., 2018). Higher zinc content of organically managed soil can be attributed to the cow dung used for making farmyard manure. It is because micronutrients provided to animals in their feed which are not assimilated by their body make way into their excreta. Hence, overuse of manures consisting of animal slurry can even cause zinc toxicity in soils (Xu et al., 2013). Moreover, higher micronutrient content in organically managed soil can be attributed to its higher soil organic matter content because organic matter acts as a chelating agent for these elements and forms stable bonds with them (Sheoran et al., 2018).
Plant agronomical parameters are greatly influenced by the nutrient status of the soil. The better agronomical parameters of organic chickpea plant can be attributed to better soil structure and chemical properties of organically managed soil. Higher plant biomass (plant dry weight and nodule dry weight) of the organically produced chickpea plants could be due to better synchrony between nutrient release by the microbes and nutrient uptake by the plant. Lim et al. (2015) reported that the application of vermicompost led to increase in plant biomass because of the slow and sustained release of minerals from the compost. The reason for increased nodulation can be attributed to the greater amount of available phosphorus in organically managed soil. It has been reported that phosphorus affects the overall nitrogen-fixing capability of root nodules because there is a high requirement for mineral phosphorus for carrying out biological nitrogen fixation (Valentine et al., 2017). The yield of grain legumes depends upon various yield components, such as the number of pods per plant, number of grains per pod, 100 grain weight, and plants per square meter. If all the yield components increase, the yield will be maximized. Thus, the better seed yield of the organic plot is due to the higher yield components of the organic plants.
Most of the cereals like rice, wheat, and legumes are deficient in Zn (Cakmak and Kutman, 2018). However, the results of this study are contrasting, as in organically grown chickpea grains, there is a high zinc content. Therefore, organically produced grains could be a better choice for food and feed purposes. The micronutrient analysis of grains revealed that conventionally produced chickpea grains had a lesser amount of Zn and Fe. This could be due to the higher copper concentration in the conventional chickpea grain, as it has been reported in previous studies that a high copper concentration can cause decreased absorption of other micronutrients from the soil (Adrees et al., 2015; Colautti et al., 2023). Conventional chickpea grains also had a higher manganese content, which can be attributed to the higher manganese content of the conventional soil. Moreover, Gunes et al. (2007) also revealed that the manganese content of chickpea plant was higher for the plants treated with inorganic phosphorus fertilizers (used in conventional agriculture) as compared to those treated with organic manures.
In our previous study, the comparative microbiomes of the organic and conventional farms used in the present study have been analyzed (Suyal et al., 2021). It has revealed a higher population of nitrogen-fixing and phosphate-solubilizing bacteria in organically managed soils. Moreover, several clinically important bacterial genera, i.e., Staphylococcus, Ruminococcus, Actinobacillus, Treponema, Corynebacterium, Mycobacterium, Prevotella, Coxiella, Enterococcus, Neisseria, Hemophilus, and Mycoplasma, were found completely absent in organic farms. The absence of these human pathogens in organic farms suggests potential benefits for food safety and reduced risk of spread of diseases. However, further research is needed to fully understand the implications of these findings. Therefore, in addition to encouraging PGPR and suppressing pathogens, organic farming is able to enhance crop productivity and soil fertility. In future studies, the co-application of cold-adapted bioinoculants and organic amendments for chickpea will enhance the productivity and soil health of the Himalayan agroecosystem. Marginal and resource-poor farmers of Himalayan regions whose livelihood relies on hill agriculture in the targeted low-nutrient soils will be the direct beneficiaries in this case.
5 Conclusion
It is evident from this work that chickpea (variety-Pant Kabuli Chana 1) production in the organic system was nutritious, economical, and environment-friendly. An added advantage of growing leguminous crops in organically managed soil, contrary to other cereal crops, is that they can meet their nitrogen demands by biological nitrogen fixation. In addition to these, support to the farmers in the initial phase of the transition from conventional to organic farming will be beneficial for the farmer and soil ecosystem in general.
Data availability statement
The datasets presented in this study can be found in online repositories. The names of the repository/repositories and accession number(s) can be found in the article/Supplementary Material.
Author contributions
PS: writing–original draft. DSu: writing–review and editing. SK: data curation and writing–review and editing. DSi: methodology, resources, and writing–review and editing. RG: conceptualization, resources, supervision, validation, and writing–review and editing.
Funding
The author(s) declare that no financial support was received for the research, authorship, and/or publication of this article.
Acknowledgments
The authors are extremely grateful to G.B. Pant University of Agriculture and Technology, Pantnagar (Uttarakhand), for providing financial assistance and space to conduct experiments. The author (DSu) acknowledges the Science and Engineering Research Board (SERB) young scientist scheme, Grant No. YSS/2015/001214 during the course of this study. In addition, Senior Research Fellowship (CSIR Award No: 09/171(0126)/2015-EMR-I) to SK is duly acknowledged.
Conflict of interest
The authors declare that the research was conducted in the absence of any commercial or financial relationships that could be construed as a potential conflict of interest.
The reviewer GM declared a past co-authorship with the author DSu to the handling editor.
Publisher’s note
All claims expressed in this article are solely those of the authors and do not necessarily represent those of their affiliated organizations, or those of the publisher, the editors, and the reviewers. Any product that may be evaluated in this article, or claim that may be made by its manufacturer, is not guaranteed or endorsed by the publisher.
Supplementary material
The Supplementary Material for this article can be found online at: https://www.frontiersin.org/articles/10.3389/fenvs.2024.1378926/full#supplementary-material
References
Adrees, M., Ali, S., Rizwan, M., Ibrahim, M., Abbas, F., Farid, M., et al. (2015). The effect of excess copper on growth and physiology of important food crops: a review. Environ. Sci. Pollut. Res. 22 (11), 8148–8162. doi:10.1007/s11356-015-4496-5
Akpo, E., Ojiewo, C. O., Kapran, I., Omoigui, L. O., Diama, A., and Varshney, R. K. (2021). Enhancing smallholder farmers’ access to seed of improved legume varieties through multi-stakeholder platforms: learning from the TLIII project experiences in sub-saharan Africa and South asia. Springer Nat. doi:10.1007/978-981-15-8014-7
Bhat, N. A., Riar, A., Ramesh, A., Iqbal, S., Sharma, M. P., Sharma, S. K., et al. (2017). Soil biological activity contributing to phosphorus availability in vertisols under long-term organic and conventional agricultural management. Front. Plant Sci. 8, 1523. doi:10.3389/fpls.2017.01523
Bisht, I. S. (2021). Agri-food system dynamics of small-holder hill farming communities of Uttarakhand in north-western India: socio-economic and policy considerations for sustainable development. Agroecol. Sustain. Food Syst. 45 (3), 417–449. doi:10.1080/21683565.2020.1825585
Bras, A., Roy, A., Heckel, D. G., Anderson, P., and Karlsson Green, K. (2022). Pesticide resistance in arthropods: ecology matters too. Ecol. Lett. 25 (8), 1746–1759. doi:10.1111/ele.14030
Cakmak, I., and Kutman, U. B. (2018). Agronomic biofortification of cereals with zinc: a review. Eur. J. Soil Sci. 69 (1), 172–180. doi:10.1111/ejss.12437
Christel, A., Maron, P.-A., and Ranjard, L. (2021). Impact of farming systems on soil ecological quality: a meta-analysis. Environ. Chem. Lett. 19 (6), 4603–4625. doi:10.1007/s10311-021-01302-y
Colautti, A., Civilini, M., Contin, M., Celotti, E., and Iacumin, L. (2023). Organic vs. conventional: impact of cultivation treatments on the soil microbiota in the vineyard. Front. Microbiol. 14, 1242267. doi:10.3389/fmicb.2023.1242267
Dhaliwal, S. S., Sharma, V., Verma, V., Kaur, M., Singh, P., Gaber, A., et al. (2023). Impact of manures and fertilizers on yield and soil properties in a rice-wheat cropping system. PLoS ONE 18 (11), e0292602. doi:10.1371/journal.pone.0292602
Dixit, G. (2021). Sustaining chickpea growth in India: breeders perspective. J. Food Legumes 34 (2), 73–75.
Erdemci, I., Aktaş, H., and Nadeem, M. A. (2017). Effect of fertilization and seed size on nodulation, yield and yield components of chickpea (Cicer arietinum l.). Appl. Ecol. Env. Res. 15 (1), 563–571. doi:10.15666/aeer/1501_563571
Food and Agriculture Organization of the United Nations (FAO) (2018). Food loss analysis: causes and solutions. Chickpea supply chain India. ISBN 978-92-5-130669-7.
Geissen, V., Silva, V., Lwanga, E. H., Beriot, N., Oostindie, K., Bin, Z., et al. (2021). Cocktails of pesticide residues in conventional and organic farming systems in Europe–Legacy of the past and turning point for the future. Environ. Pollut. 278, 116827. doi:10.1016/j.envpol.2021.116827
Gomiero, T., Pimentel, D., and Paoletti, M. G. (2011). Environmental impact of different agricultural management practices: conventional vs. organic agriculture. Crit. Rev. plant Sci. 30 (1-2), 95–124. doi:10.1080/07352689.2011.554355
Gopinath, K. A., Kumari, V. V., Chary, G. R., Jayalakshmi, M., and Venkatesh, G. (2016). 27 nutritional quality of organically grown food crops. Reshaping Agric. Nutr. Linkages Food Nutr. Secur. 326, 248.
Gunes, A., Inal, A., Adak, M. S., Alpaslan, M., Bagci, E. G., Erol, T., et al. (2007). Mineral nutrition of wheat, chickpea and lentil as affected by mixed cropping and soil moisture. Nutr. Cycl. Agroecosyst. 78 (1), 83–96. doi:10.1007/s10705-006-9075-1
Haneef, R., Sharma, G., and Ahmad, T. (2019). Constraints faced by farmers practicing organic farming in hill region of Uttarakhand, India. Int. J. Curr. Microbiol. Appl. Sci. 8 (5), 1149–1157. doi:10.20546/ijcmas.2019.805.130
Hartman, K., van der Heijden, M. G., Wittwer, R. A., Banerjee, S., Walser, J. C., and Schlaeppi, K. (2018). Cropping practices manipulate abundance patterns of root and soil microbiome members paving the way to smart farming. Microbiome 6 (1), 14. doi:10.1186/s40168-017-0389-9
Khan, M. H., Liu, H., Zhu, A., Khan, M. H., Hussain, S., and Cao, H. (2023). Conservation tillage practices affect soil microbial diversity and composition in experimental fields. Front. Microbiol. 14, 1227297. doi:10.3389/fmicb.2023.1227297
Li, Y., Moinet, G. Y. K., Clough, T. J., and Whitehead, D. (2022). Organic matter contributions to nitrous oxide emissions following nitrate addition are not proportional to substrate-induced soil carbon priming. Sci. Total Environ. 851 (2), 158274. doi:10.1016/j.scitotenv.2022.158274
Liang, C., Schimel, J. P., and Jastrow, J. D. (2017). The importance of anabolism in microbial control over soil carbon storage. Nat. Microbiol. 2 (8), 17105. doi:10.1038/nmicrobiol.2017.105
Lim, S. L., Wu, T. Y., Lim, P. N., and Shak, K. P. Y. (2015). The use of vermicompost in organic farming: overview, effects on soil and economics. J. SciFood Agric. 95 (6), 1143–1156. doi:10.1002/jsfa.6849
Lindsay, W. L., and Norvell, W. A. (1978). Development of a DTPA soil test for zinc, iron, manganese, and copper. Soil Sci. Soc. Am. J. 42 (3), 421–428. doi:10.2136/sssaj1978.03615995004200030009x
Montgomery, D. R., and Biklé, A. (2021). Soil health and nutrient density: beyond organic vs. Conventional farming. Frontiers in Sustainable Food Systems, 5, 417. multielement tissue analysis. Commun. Soil Sci. Plant Anal. 3, 425–436. doi:10.1080/00103627209366397
Olsen, S. R. (1954) Estimation of available phosphorus in soils by extraction with sodium bicarbonate. Washington: United States Department Of Agriculture.
Rees, R. M., Griffiths, B. S., and McVittie, A. (2018). “Sustainable intensification of agriculture: impacts on sustainable soil management,” in International yearbook of soil law and policy 2017 (Cham: Springer), 7–16. doi:10.1007/978-3-319-68885-5_2
Sheoran, H. S., Phogat, V. K., Dahiya, R., Dhull, S., and Kakar, R. (2018). Comparative effect of organic and conventional farming practices on micronutrient content in different textured soils of Haryana. Int. J. Curr. Microbiol. App. Sci. 7 (4), 3399–3407. doi:10.20546/ijcmas.2018.704.384
Subbiah, B., and Asija, G. L. (1956). Alkaline permanganate method of available nitrogen determination. Curr. Sci. 25, 259.
Suhr, N., Schoenberg, R., Chew, D., Rosca, C., Widdowson, M., and Kamber, B. S. (2018). Elemental and isotopic behaviour of Zn in Deccan basalt weathering profiles: chemical weathering from bedrock to laterite and links to Zn deficiency in tropical soils. Sci. Total Environ. 619, 1451–1463. doi:10.1016/j.scitotenv.2017.11.112
Suyal, D. C., Soni, R., Singh, D. K., and Goel, R. (2021). Microbiome change of agricultural soil under organic farming practices. Biologia 76 (4), 1315–1325. doi:10.2478/s11756-021-00680-6
Symochko, L., Demyanyuk, O., Crisan, V., and Dinca, L. (2024). Microbial transformation of soil organic matter under varying agricultural management systems in Ukraine. Front. Microbiol. 14, 1287701. doi:10.3389/fmicb.2023.1287701
Thudi, M., Roorkiwal, M., Kudapa, H., Chaturvedi, S. K., Singh, N. P., and Varshney, R. K. (2017). An overview of chickpea research: from discovery to delivery. Pulse India 2 (5), 22–25.
United Nations, Department of Economic and Social Affairs, Population Division (UNDESA) (2022). World population prospects 2022: summary of results. U. N. DESA/POP/2022/TR/NO 3. Available at: https://www.un.org/development/desa/pd/sites/www.un.org.development.desa.pd/files/wpp2022_summary_of_results.pdf.
Valentine, A. J., Kleinert, A., and Benedito, V. A. (2017). Adaptive strategies for nitrogen metabolism in phosphate deficient legume nodules. Plant Sci. 256, 46–52. doi:10.1016/j.plantsci.2016.12.010
Venkat, K. (2012). Comparison of twelve organic and conventional farming systems: a life cycle greenhouse gas emissions perspective. J. Sustain. Agric. 36 (6), 620–649. doi:10.1080/10440046.2012.672378
Walkley, A., and Black, I. A. (1934). An examination of the Degtjareff method for determining soil organic matter, and a proposed modification of the chromic acid titration method. Soil Sci. 37 (1), 29–38. doi:10.1097/00010694-193401000-00003
Xu, Y., Yu, W., Ma, Q., and Zhou, H. (2013). Accumulation of copper and zinc in soil and plant within ten-year application of different pig manure rates. Plant Soil Environ. 59 (11), 492–499. doi:10.17221/121/2013-pse
Zasoski, R. J., and Burau, R. G. (1977). A rapid nitric-perchloric acid digestion method for multi-element tissue analysis. Commun. Soil Sci. Plant Analysis 8 (5), 425–436. doi:10.1080/00103627709366735
Zilio, M., Motta, S., Tambone, F., Scaglia, B., Boccasile, G., Squartini, A., et al. (2020). The distribution of functional N-cycle related genes and ammonia and nitrate nitrogen in soil profiles fertilized with mineral and organic N fertilizer. PLoS One 15 (6), e0228364. doi:10.1371/journal.pone.0228364
Keywords: organic farming, soil-derived nutrient, chickpea, micronutrient, available nitrogen
Citation: Singh P, Suyal DC, Kumar S, Singh DK and Goel R (2024) Long-term organic farming impact on soil nutrient status and grain yield at the foothill of Himalayas. Front. Environ. Sci. 12:1378926. doi: 10.3389/fenvs.2024.1378926
Received: 30 January 2024; Accepted: 15 May 2024;
Published: 06 June 2024.
Edited by:
Gilles Colinet, University of Liège, BelgiumReviewed by:
Gaurav Mishra, Indian Council of Forestry Research and Education (ICFRE), IndiaYuan Li, Lanzhou University, China
Copyright © 2024 Singh, Suyal, Kumar, Singh and Goel. This is an open-access article distributed under the terms of the Creative Commons Attribution License (CC BY). The use, distribution or reproduction in other forums is permitted, provided the original author(s) and the copyright owner(s) are credited and that the original publication in this journal is cited, in accordance with accepted academic practice. No use, distribution or reproduction is permitted which does not comply with these terms.
*Correspondence: Reeta Goel, reeta.goel@gla.ac.in