- Soil Sciences Department, College of Food and Agricultural Sciences, King Saud University, Riyadh, Saudi Arabia
Microplastics (MPs) and heavy metals have recently attracted much attention due to their widespread distribution in the environment. Co-occurrence of MPs and heavy metals in dust poses potential health risks for humans. The objectives of this study were to investigate pollution and health risk factors associated with co-occurring MPs and heavy metals in urban dust. Here, 20 dust samples (both indoor and outdoor environments) were collected from different places in Riyadh, Saudi Arabia using sweeping method. The MPs were separated from dust samples via density separation using ZnCl2 Microscopic techniques were used to investigate MPs while inductively coupled plasma optical emission spectrometry was used heavy metals analyses in the collected samples. The results of the study showed that all the collected dust samples were contaminated with MPs of different shapes, sizes, and colors. Fragment-shaped MPs were the most dominant, followed by granules, fibers, and lines, while the majority of the MP particles were red and black. Furthermore, the size distribution analyses demonstrated that particles above 1,000 µm were abundant. Also, the number of MPs was in the range of 20 particles g-1 to 1,052 particles g-1. Human exposure estimations showed that toddlers could inhale more MPs (0.86 MPs particles kg-1 day-1 and 312.93 particles kg-1 year-1) than children, teenagers, and adults. In addition, the concentrations of heavy metals varied from one site to another, however, the average concentrations were lower than their corresponding values in the guidelines (except for Zn). The integrated potential ecological risk in dust samples was characterized as a low-risk criterion. The enrichment factor for Zn in dust samples at all sites was more than 2, indicating a major contamination concern and anthropogenic sources responsible for the existence of Zn. The estimated hazard quotient indicates that children and adults are saved from the adverse effects of dust inhalation in the studied area. These findings could serve as a preliminary step for efficient pollution management and environmental quality maintenance in Riyadh.
1 Introduction
Plastic products are widely employed in different fields of modern society because of low-cost, light weight, chemical stability, corrosion resistance, and impact resistance (Guo et al., 2020; Wong et al., 2020). Plastics Europe reported that 400.3 million tons of plastic were produced worldwide in 2022. Plastic pollution in the environment is an inevitable result of the widespread usage of plastics. The term “microplastics (MPs)" refer to the plastic particles with size of less than 5 mm, whereas, the plastic particles with size range of 5 mm to 2.5 cm are termed as mesoplastics, and with size of >2.5 cm are termed as macroplastics (Jeyasanta et al., 2020). MPs are smaller and more challenging to recycle and remove from the environment than macro plastics (Thompson et al., 2004). Furthermore, MPs can be quickly released into the air and soil, where they have detrimental effects on the environment (Besseling et al., 2017). As a result, MPs have garnered a lot of interest globally (Sarker et al., 2020).
The prevalence of plastics in the environment is currently a worldwide issue for scientists (Rillig et al., 2021). It is estimated that the plastic emission annually will be increased to 53 million metric tons by 2030 (Borrelle et al., 2020). In developing countries, a minute plastic portion is recycled while a huge portion is dumped in open air, where it comes in contact with microorganisms, sunlight, and photodegradation (Andrady, 2011). MPs are usually produced from two main sources, the primary sources include the micro beads, personal care products, cosmetics, plastic shots, resin pellets and particles from the plastic manufacturing industries, while secondary plastics are produced from the degradation and the breakdown of primary MPs (Li et al., 2016). Further, MPs have low density (<1 g cm-3), thus can be moved with the winds and vehicles movement, consequently contributing to the environmental pollution (Ho et al., 2003; Zhang et al., 2018). Consequently, these MPs are deposited near the roads that could be ingested by the humans. It has been reported that roads wears and tires constitute approximately 42% of the MPs transferred to the sea, that consist of polymer, tires and road makings (Siegfried et al., 2017; Vogelsang et al., 2019). Moreover, the continuous movement of vehicles causes the generation of dust along the road sides and pavements due to the fragmentation of larger debris particles, food containers, construction materials, and soil particles (Monira et al., 2021). Dust is considered as one of the main indicators about the environmental quality of any city as it contains different kinds of pollutants which have been deposited from numerous sources including air, soil and water (Wong et al., 2006). Road dust is considered as one of the main source of MPs introduction to the environment and has been studied in many countries including Japan, India, Iran, Nepal and Vietnam (Abbasi et al., 2017; Yukioka et al., 2020). Approximately 90%–95% of the MPs found on the road dust are flushed to the rivers, soils and oceans as a result of rainfall, and when they have reached there it is very difficult to treat them as more than 90% of the MPs accumulate there (Kang et al., 2022). Street and road dust consist of mostly organic and inorganic pollutants, among them MPs contribute almost 0.06% of the dry mass (Prabhakar et al., 2014; O’Brien et al., 2021). Plastic particles with the size of 5 mm are considered as MPs, however, due to the increasing concerns of the MPs toxicity numerous studies are focusing to consider the upper limit size up to 1 mm as MPs (Thiele et al., 2021). The toxicity of the MPs increases with the decreasing size, when they are inhaled or ingested by organisms, because persistent organic pollutants are also reported on the surface of the MPs (Lehner et al., 2019). Human can intake the MPs via three ways, i.e., ingestion, inhalation and dermal intake (Cox et al., 2019). MPs intake can create the obstruction, inflammation and accumulation in the different organs which cause serious health threats including oxidative stress, fluctuating the metabolism and disturbing the energy balance, cytotoxicity, immune system disruption, neurotoxicity, reproductive system inefficiency, and cancer in the humans (Rahman et al., 2021; Ahmad et al., 2023). Moreover, MPs also contain very harmful chemicals including additives of plastics, unreacted monomers, persistent organic pollutants, poly aromatic hydrocarbons, heavy metals and micro-organisms (Gasperi et al., 2018).
Along with the MPs, street dust has been reported to be loaded with inorganic and organic pollutants, to be specific, the high concentration of trace metals (Saeedi et al., 2012; Prabhakar et al., 2014). The characterization of dust is challenging as it is a complex mixture of inorganic and organic compounds (Cheng et al., 2018). Vehicles, deterioration of the road surface, brake pad wear and tear, brake fluid leakage, deterioration of the road paint, and air deposition are the primary sources of heavy metals pollution (Opher and Friedler, 2010). The weathering of building, road sides and natural geochemical processes also contribute heavy metals to the environment (Manasreh, 2010). Higher concentrations of the heavy metals cause serious health damages including nervous system disorders, renal system diseases, cardiovascular disorders, reproductive system disorders, attention deficit, intelligence weakness, and other behavioral disorders (Alloway et al., 2005). As a result, it is crucial to look into potential hazards and conduct investigations such as risk assessment for human health.
The Kingdom of Saudi Arabia is located in arid region with minimum precipitation where summers are extremely hot and sometimes the temperature reaches to 50°C. The humidity level in the air is very low and sunlight is blazing. Further, the Riyadh city, capital of Saudi Arabia, is highly populated and road traffic is huge. When the vehicles run on the roads, the tires abrasion increases which results in the production of MPs in the environment. Moreover, these MPs accumulate along the road sides, pavements and on the trees planted in between and along the road sides. With the wind speed and vehicles, these MPs particles, move from one place to another and there are higher chances of the MPs to be taken in by the humans.
Due to hot climate, sand storms are common in Riyadh, which favor the transportation of dust from one place to other, and even inside the houses. Therefore, the chances of MPs and heavy metals to be inhaled via dust are more. However, very limited studies have investigated the prevalence of MPs and heavy metals in urban dust deposits. Specifically, in Riyadh (Saudi Arabia), there is substantial lack of research about the presence of MPs and heavy metals in urban dust. This study investigates the presence of MPs and heavy metals in urban dust samples simultaneously. Indoor and outdoor dust samples were collected from different locations within the Riyadh city and were analyzed for occurrence of MPs and heavy metals. Furthermore, potential ecological risks and human exposure risks associated with heavy metals and MPs contamination were estimated in this study.
2 Materials and methods
2.1 Samples collection
The dust samples were collected from various urban areas of Riyadh city, the capital of Saudi Arabia. Riyadh is characterized by the desert climate with extreme hot weather conditions. The average summer and winter temperature ranges from 45°C−50°C and 5°C−20°C, respectively (Alsabhan et al., 2022). Due to surrounding desert and hot climate, dust storms are common in Riyadh. Twenty sites were selected for dust sample collection during summer 2023, including both indoor and outdoor environments (Figure 1). A wooden brush was used to collect dust samples from the surfaces through the sweeping method. The collected samples were kept in Ziploc and transported to the laboratory for further analyses. Visible trash and stones were removed from the samples, and then the dust samples were air-dried at room temperature (23°C–25°C).
2.2 Microplastic extraction
To extract MPs from the collected dust samples, density separation method was employed using a ZnCl2 solution with a density of 1.60 kg L-1 (Liu et al., 2019). Briefly, ZnCl2 solution was filtered using 0.22 µm membrane filters and 15 mg of the dust sample was placed in 50 mL of ZnCl2 solution in a glass beaker. The suspension was stirred for 5 min, and thereafter covered and left static for 2 h. The upper suspended fraction was separated and placed in 20 mL of ZnCl2 solution. After stirring, the MPs particles were separated with the help of 0.45 μm glass microfiber filters. The filters were placed in clean Petri dishes, dried in an oven at 35°C and subjected to further analyses.
2.3 Occurrence of microplastics in dust
The occurrence of MPs in dust samples was estimated through microscopic techniques. For the identification and quantification of MPs visual inspection methods were followed. Abundance, shape, and color of MPs were observed using a phase contrast microscope (DM2500, Leica®, Germany). Thereafter, the morphology of MPs was inspected under a scanning electron microscopy (SEM; EFI S50 Inspect, Netherlands). Briefly, carbon conductive tabs were placed on aluminum stubs, and then dried filters were gently tapped on the stubs to spread MPs particles. The images of the MPs were taken using different magnifications. The captured images were processed further to measure the size of the MPs particles using ImageJ software (version 1.54d, National Institutes of Health, United States).
2.4 Human exposure of microplastics
The exposure of human beings to MPs was estimated through calculating the estimated daily intake (EDI) of MPs via inhalation of dust particles with the help of Eq 1, whereas, the estimated annual intake (EAI) was calculated by using Eq. 2.
Where, m is the average dust ingestion rate (g day-1), C represents the number of MPs in dust, and BW represents body weight in kgs. The calculations were done based on different age groups, i.e., 1–5 year old (toddlers), 6–11 year old (children), 12–19 year old (teenagers), and ≥20 year old (adult). The average dust ingestion rate was used as 0.06 g day-1 for toddlers, children, and teenagers, while 0.03 g day-1 for adults (US EPA, 2011). The average body weights used in this study were 19, 29, 53, and 63 kg for toddlers, children, teenagers, and adults, respectively (Liao et al., 2012).
2.5 Heavy metals contents in dust
For heavy metal analysis, the samples passed through 100 µm sieve, and digested according to Hossner (Hossner, 1996). Approximately 0.5 g of dry, homogenized sample was digested using a mixture of 10 mL of concentrated HNO3 and 70% of concentrated HClO4 on a hot plate. The digestion process was conducted for approximately 30–40 min, and concentrated H2O2 was added to the sample to aid complete digestion. After digestion, the samples were filtered. The total heavy metals contents including of cadmium (Cd), copper (Cu), lead (Pb), iron (Fe), nickel (Ni), Zinc (Zn), Mercury (Hg), Selenium (Se), Cobalt (Co), Manganese (Mn), Molybdenum (Mn) and arsenic (As), were measured using inductively coupled plasma optical emission spectrometry (ICP-OES; PerkinElmer Optima 4300 DV, United States). All samples analyses were repeated three times for precision. (Maqsoom et al., 2020).
2.6 Level of heavy metals pollution and health risk assessment
Enrichment factor (EF), pollution index (PI), integrated pollution index (IPI), and potential ecological risk index (RI) were used to assess the levels of heavy metal contamination (Chen et al., 2005). According to Sutherland (Sutherland et al., 2000), the EF value is a useful metric for determining the extent of metal pollution and predicting the likelihood of natural or man-made sources. Because Fe is naturally occurring, a reference concentration is token for normalization. The following equation was used to estimate the EF value:
where Cm (dust sample) is the examined metal content in the dust sample, CFe(dust sample) is the content of the reference metal (Fe) in the dust sample, Cm(earth crust) is examined metal content in the earth’s crust, and CFe(earth crust) is the content of the reference metal (Fe) in the earth’s crust. Generally, EF values higher than two are mainly considered to have anthropogenic sources, while EF values less than two predominantly originate form background soil material. Moreover, the EF value also assists in estimating the metal contamination degree. Five categories of contamination are standard based on EF factor: EF ˂2 indicates deficiency to minimal enrichment; EF = two to five, indicates moderate enrichment; EF = 5–20, indicates significant enrichment; EF = 20–40, indicates very high enrichment; and EF ˃40, indicates extremely high enrichment.
The PI is estimated as the ratio of the metal concentration in the dust sample to the background content of the corresponding metal in the earth’s crust. The PI value can be classified as: PI ≤ 1, low level; 1 < PI ≤ 3, middle level; and PI > 3, high level. The IPI values of all measured metals in dust samples were identified as the mean value of the PI metals. The classified IPI is as follows: IPI ≤1, low level; 1 < IPI ≤2, middle level; and IPI >2, high level.
The potential ecological risks (RI) originally reported by Hakanson (1980) was also estimated to evaluate the degree of metal pollution in dust samples by using Eqs 4–6.
where RI is the sum of all six risk factors for HMs, Ei is the monomial potential ecological risk factor, Ti is the metal toxic factor (with the values for each metal in the order of Zn = 1 < Cr = 2 < Cu = Ni = Pb = 5 < Cd = 30), fi is the metal pollution factor, Ci is the metals concentration of in dust, and Bi is a reference value for metals (Hakanson, 1980). Different RI classifications of metal pollution are low ecological risk (RI ≤ 150), moderated ecological risk (150 ≤ RI < 300), considerable ecological risk (300 ≤ RI < 600), and high ecological risk (RI ≥ 600).
Risk assessment is a measure to estimate the nature and probability of heavy metals in an environment and their adverse effects on human health (Wang et al., 2014). Health risk assessment considers human exposure to dust via three various pathways, including inhalation pathway. The average daily dosages (ADDs) through inhalation pathway for dust was calculated according to the following Eq 7:
Where,
The non-carcinogenic quotients of exposure to heavy metals in dust was estimated. Non-cancer risks are expressed as a hazard quotient (HQ). The HQ is the quotient of the average daily dosages (ADDs) divided by the reference dosage of a specific heavy metals and for the exposure through inhalation pathway. The HQ of each metal was estimated by the following Eq 8:
Where,
3 Results and discussion
3.1 Occurrence of MPs in dust
The indoor and outdoor dust samples collected from different locations in Riyadh city were analyzed for the presence of MPs. The microscopic analyses of the samples showed that all of the collected samples contained different levels of MPs. Figure 2 shows different shapes of MPs as detected through a phase contract microscope, while Figure 3 represents different MPs as observed under an SEM. The detected MPs were of different shapes and colors. However, red and black were the dominant colors, followed by green, brown, and transparent. The variation in the colors suggested multiple sources of MPs in dust samples. The black colored particles could be of microrubbers tire abrasions, black textile products, and building materials (Narmadha et al., 2020; Zhu et al., 2022). The transparent MPs could be due to the transparent polythene bags, disposable plastics as well as prolonged photoleaching of colored MPs (Nematollahi et al., 2022). The colorful MPs such as red, green, and brown could be due to consumable and highly durable plastic products (Nematollahi et al., 2022). Though color of MPs does not directly indicate the source; however, the identification of MPs based on their color is considered an important aspect as it may suggest a possible source.
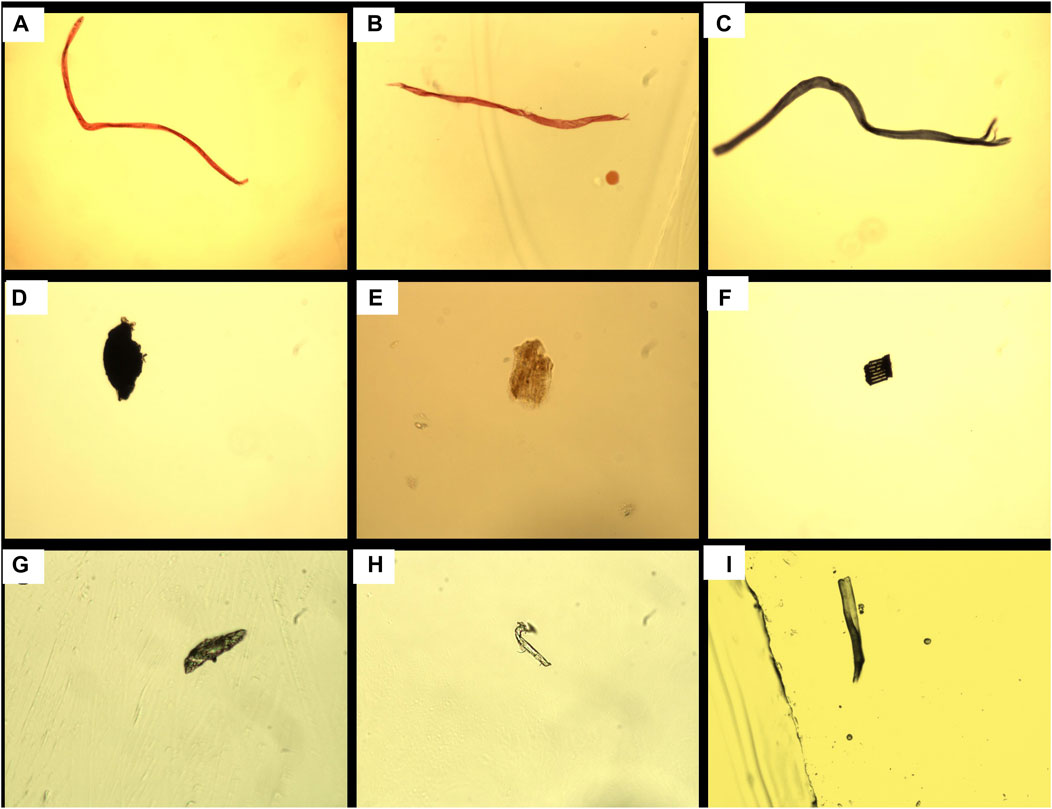
Figure 2. Phase contrast microscope analyses of the microplastics found in dust samples collected from different areas in Riyadh city, Saudi Arabia. (Alphabets (A–I) represent different shapes of microplastics).
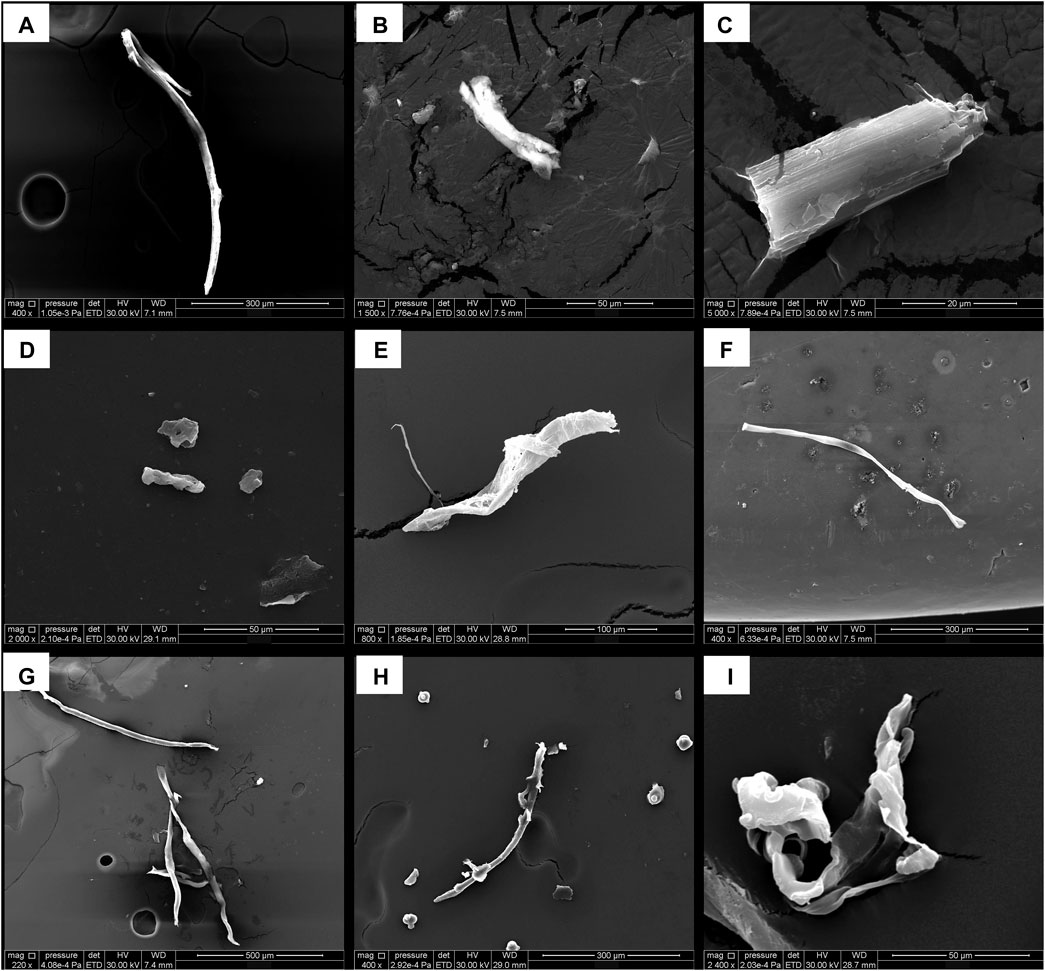
Figure 3. Scanning electron microscope (SEM) analyses of the microplastics in dust samples collected from different areas in Riyadh city, Saudi Arabia. (Alphabets (A–I) represent different shapes of microplastics).
The relative shape distribution of MPs particles in dust samples was also studied and presented in Figure 4. As of right now, several forms of air MPs have been identified, including films, spherules, granules, fibers, foam, and pellets (Abbasi et al., 2017; Abbasi et al., 2019; Stanton et al., 2019; Vianello et al., 2019). The different morphotypes’ existence is dependent upon the atmospheric compartment in which they are found as well as human activity in the immediate vicinity. According to the results of the study, the composition of MPs particles by shape varies. However, considering the overall results, it could be deduced that the shapes of the MPs particles are more abundant in the order fragments > granules > fibers > lines. The variation in the composition of MPs particles by shape was also affirmed by previous studies. An example of such is the research conducted by Abbasi et al., where fiber was reported to be the most abundant shape with a record 75.87% of observed morphotypes. On the contrary, granule (65.9%) and spherule (65.9%) were reported to be the dominant shapes among all the shapes present in the studies by Dehghani et al. (2017) and Abbasi et al. (2017), respectively.
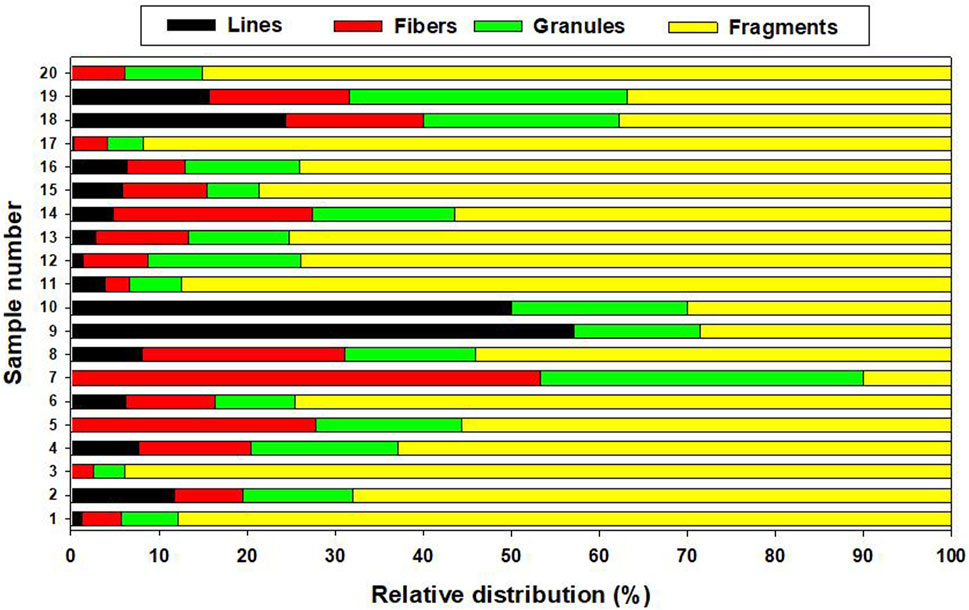
Figure 4. Relative distribution of different shaped microplastics found in dust samples collected from different areas in Riyadh city, Saudi Arabia.
Another metric for classifying MPs is size distribution. One goal of size reporting in atmospheric investigations is to ascertain their tendencies for ingestion and inhalation among the human population (Gasperi et al., 2018). Microplastic characteristics include size, color, and type, depending on the characteristics of the textiles used. For instance, in a study, the discovery of polypropylene particles was consistent with the polypropylene carpet in the living room (Abbasi et al., 2019). Figure 5 describes the relative size distribution of MPs particles in different dust samples collected from the city of Riyadh, Saudi Arabia. The classification of MPs by size is mostly reported in terms of particle length or diameter due to their irregular shapes. Across all the samples collected, the results of the study indicate that most of the MPs particles have sizes greater than 1,000 μm, followed by 501–1,000 µm sizes, then 251–500 µm sizes, followed by 101–250 µm sizes, and 1–100 µm sizes. Similarly, Dris et al. (2017) reported the predominance of microfiber distribution in the large particle size range (200–600 μm) with fewer fibers less than 200 μm. Dris et al. (2017) and Dehghani et al. (2017) reported a lower proportion of MPs in the smallest size range. It could be educed that larger sizes of MPs particles are more abundant as compared with smaller sizes.
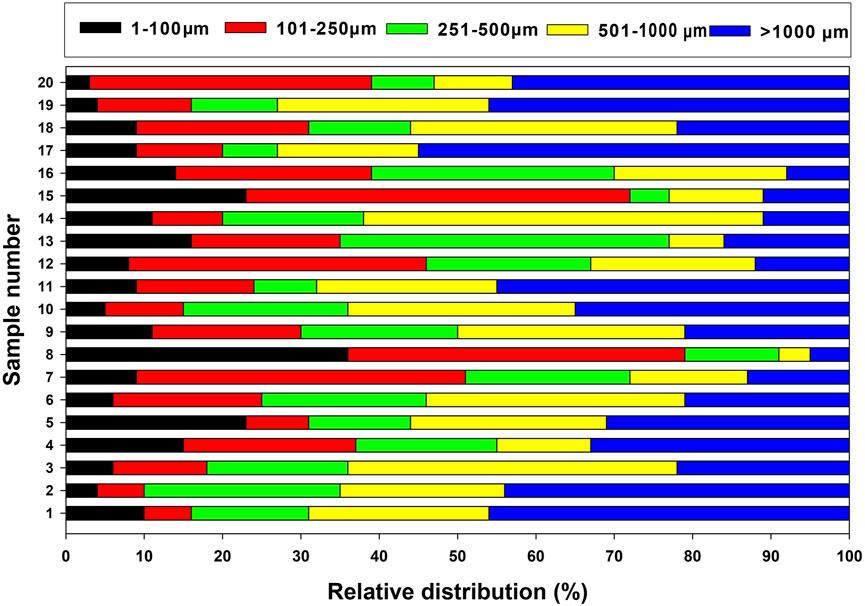
Figure 5. Particle size distribution of microplastics detected in dust samples collected from different areas in Riyadh city, Saudi Arabia.
The number of MPs (particles g-1) in the dust samples collected from different locations in Riyadh, Saudi Arabia is presented in Figure 6. From the results of the study, sample collected from site-17 had the highest number of MPs (1,052 particles g-1) while the least number of MPs (20 particles g-1) was found in the dust sample collected from site-10. A significant number of MPs were also found in dust samples collected at site 3, site 11, site 15, and site 20. Overall, the results indicated the presence of a higher number of MPs particles in the outdoor samples as compared to indoor samples. The abundance of MPs particles in the outdoor environment has been associated to wind erosion paying a major role (Rezaei et al., 2019; La Porta et al., 2023).
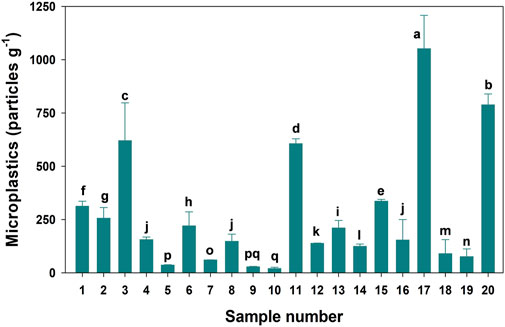
Figure 6. Number of microplastic particles found dust samples collected from different areas in Riyadh city, Saudi Arabia. (Different alphabets on the bars show the level of significance (p < 0.05) between the samples).
3.2 Human exposure to microplastics
Dust can be a source for the exposure of various environmental pollutants to humans. The occurrence of MPs in dust has come up as a widespread health concern for human beings in recent times. The results showed that toddlers were relatively more prone to the MPs ingestion through dust due to higher inhalation rate. Our calculations show that toddlers can potentially inhale 0.86 MPs particles kg-1 day-1 and 312.93 particles kg-1 year-1, suggesting a higher risk of MPs toxicity (Table 1). Followed by toddlers, children can inhale 0.65 particles kg-1 year-1, and teenagers can inhale 7.32 × 10−4 particles kg-1 year-1. Adults could inhale relatively lower MPs (6.98 × 10−7 particles kg-1 year-1). Similar results have previously been reported by Zhang et al. (2020) where it was suggested that toddlers can inhale 10-time higher MPs than adults through dust. Therefore, indoor and outdoor dust could be one to the important pathways for human exposure to MPs.
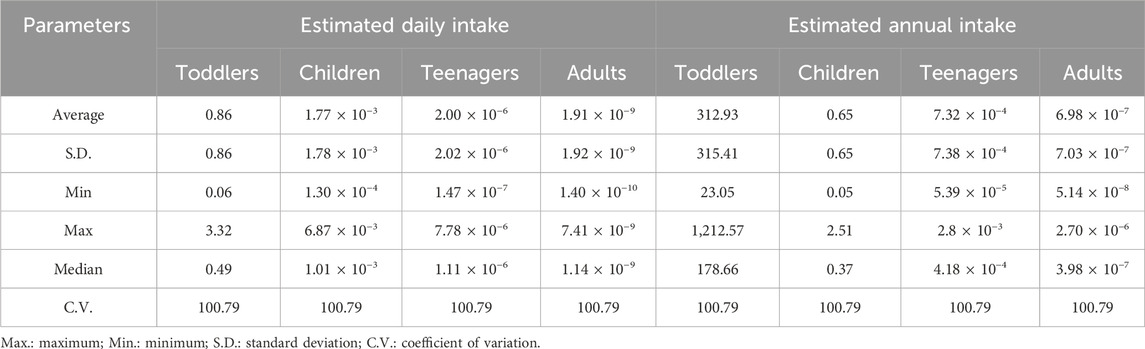
Table 1. Estimated daily intake (particles kg-1 day-1) and estimated annual intake (particles kg-1 year-1) of microplastics through the inhalation of dust.
3.3 Assessment of heavy metals concentration in dust samples
Table 2 reveals the concentrations of heavy metals in dust samples collected from the indoor and outdoor environments from Riyadh city. The results showed that the concentration of heavy metals varied from one site to other. The average Co, Cr, Cu, Fe, Mn, Ni, Pb, and Zn concentrations in the dust samples were 5.84, 28.20, 10.88, 11125.25, 175.68, 8.75, 3.37, and 162.94 mg kg-1, respectively. On the other hand, Hg, Se, Cd, and Mo concentration was not detected in all dust road samples. Overall, the highest heavy metals concentrations varied from site to other. For instance, Co was highest at site 17, Cr was highest at site 1, Fe and Ni were highest at site 13, and Pb and Zn were highest at site 6. The heavy metals concentrations in dust samples were compared with guidelines, such as the common range in the earth’s crust, the average shale values, and the average concentrations in world soil. In our finding, the average concentrations heavy metals in dust samples were lower than their corresponding values of the guidelines except Zn, which was higher than their corresponding values of the guidelines (Turekian and Wedepohl, 1961; Lindsay, 1979; Huang et al., 2009; Al-Swadi et al., 2022; Alotaibi et al., 2022).
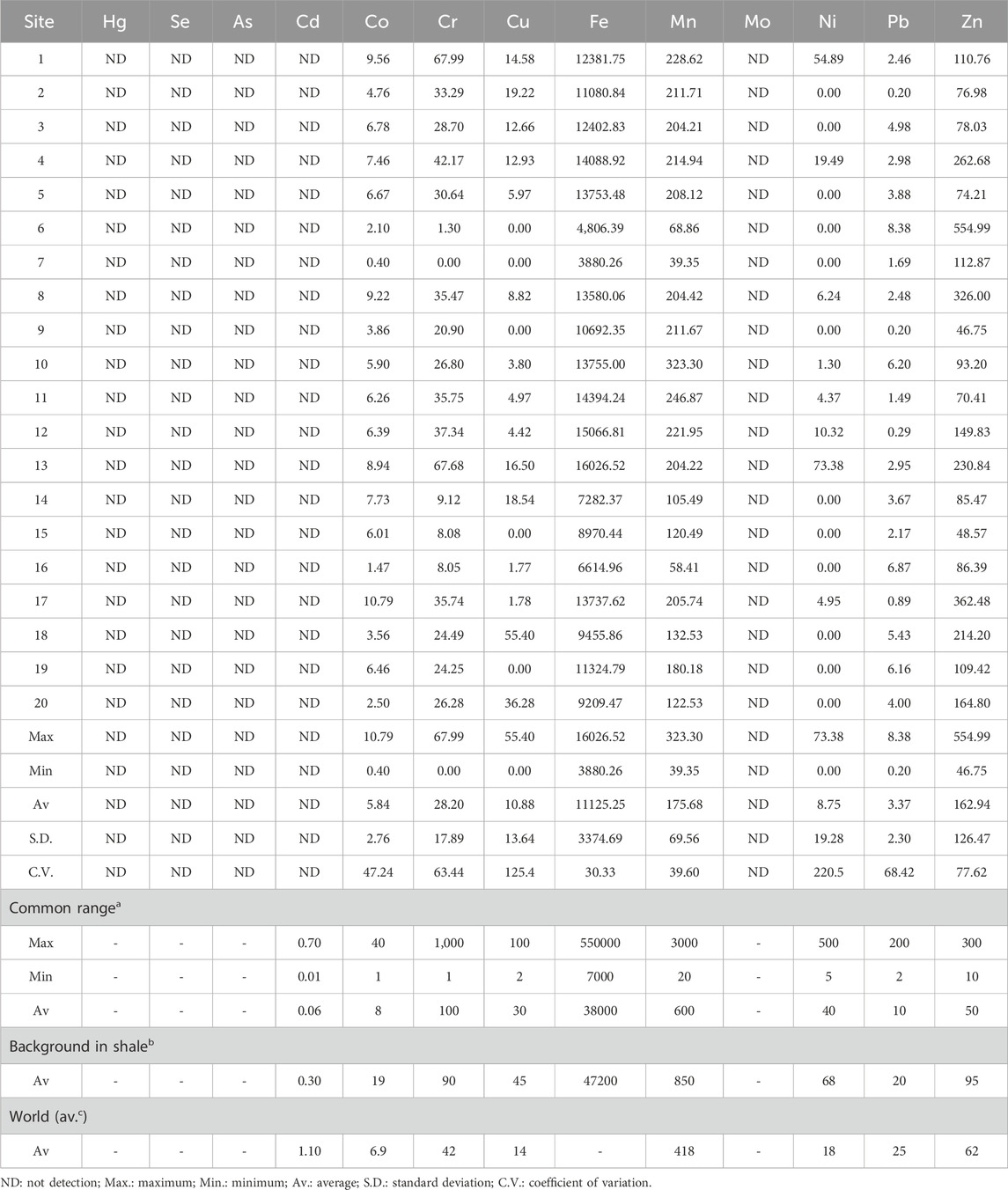
Table 2. The average concentrations of heavy metals (mg kg-1) in the dust samples collected from Riyadh city, Saudi Arabia.
3.4 Assessment of pollution, ecological, and human risks of heavy metals
The maximum, minimum, and mean EF, PI, IPI, Ei, and RI parameters for all heavy metals in dust samples are shown in Table 3. The all calculated date is presented in Supplementary Table S2-4. Among all heavy metals in dust samples, the greatest EF values were recorded for Zn (57.37) at site 6, indicating extremely high enrichment. In addition, EF values at all sites recorded in two categories two to five and 5–20, indicating moderate and significant enrichment. Furthermore, EF values showed moderate enrichment for Cr (2.88) and Ni (3.08) at site-1, Pb (4.12) at site 6, Ni (3.18) at site 13, Co (2.64) and Cu (2.67) at site 14, Pb (2.45) at site 16, and Cu (6.15 and 4.16) at sites 16 and 20. Previously, it has been indicated that the EF index could be applied to identify the heavy metals source. Therefore, the EF index being less than two pointed out that the heavy metals originated from natural sources, and some previous studies reported that the EF values of the heavy metals less than two were not a major concerned contaminates (Yongming et al., 2006; Ye et al., 2011). In this context, the EF index for Zn in dust samples at all sites was more than 2, indication a major contamination concern and anthropogenic sources responsible for the existence of Zn in dust. Moreover, the aforementioned metals, such as Zn at all sites and Co (at site 14), Cr (at site 1), Cu (at sites 14, 18, and 20), Ni (at sites 1 and 13), and Pb (at sites 6 and 16), had EF values higher than 2, indicating that these metals were a major concern contaminant at these sites. Therefore, the anthropogenic influence was responsible, including tire tread, brake dust, and emission from the vehicles (Al-Swadi et al., 2022; Alotaibi et al., 2022).
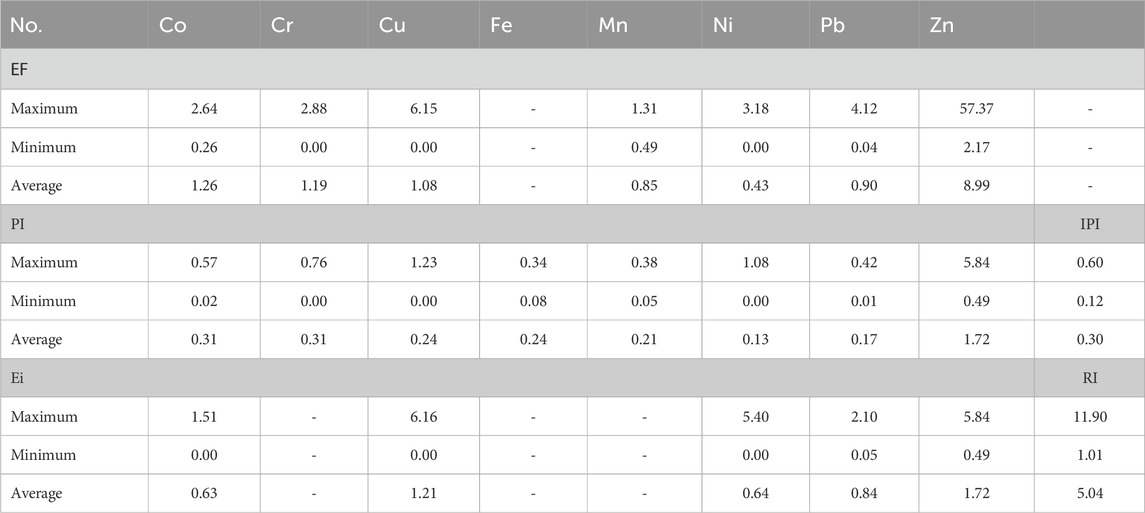
Table 3. The calculated enrichment factor (EF), pollution index (PI), integrated pollution index (IPI), monomial potential ecological risk factor (Ei), and potential ecological risk index (RI) values for heavy metals in dust samples collected from Riyadh city, Saudi Arabia.
The results revealed that the maximum, minimum, and mean PI index for most heavy metals in dust samples were <1, suggesting a low pollution level. On the contrary, the PI values of Cu at site 18, Ni at site 13, and Zn at sites 1, 4, 7, 12, 13, 18, 19, and 20 were in the range of 1 < PI ≤ 3, indicating a middle level of pollution. On the other hand, the PI value of Zn at sites 6, 8, and 17 was more than 3, indicating a high level of pollution. Likewise, the maximum, minimum, and mean IPI index of heavy metals in dust samples in all sites were less than 1, indicating a low level of pollution. Additionally, the evaluation of potential ecological risk was investigated in this study for Co, Cu, Ni, Pb, and Zn. The single Ei and integrated potential ecological risk (RI) are shown in Table 3; Supplementary Table S4. The findings showed that the maximum, minimum, and mean of RI index in all dust roads samples were classified by low ecological risk (RI ≤ 150). Generally, since each heavy metal pollution index has advantages and disadvantages of its own, no one approach can be suggested for risk evaluation. For instance, the PI index is simple to employ and has a precise scale, but it excludes the variations in natural sources for its calculations and ignores the available ability of heavy metals. Similarly, EF index can estimate the anthropogenic impacts of heavy metals and evaluate pollution caused by individual heavy metals, but it needs a reference value for its calculation.
The average daily dose of heavy metals for children and adults are shown in Supplementary Table S5, 6, while the HQ values are shown in Supplementary Table S7, 8. The results indicated that the HQ values for the investigated heavy metals in dust samples were higher for children than those of adults (Table 4). However, all the HQ values for heavy metals for all dust samples were <1, suggesting that there were no adverse health risks of the heavy metals on children or adults.
4 Conclusion
Dust samples were collected from several locations in Riyadh city, Saudi Arabia. The occurrence of MPs and heavy metals were analyzed and their associated human exposure risks were explored. Red and black colored MPs were dominant in all the studied dust samples, whereas fragments shaped MPs were most common, followed by granules, fibers, and lines. Also, the majority of the detected MPs were in size range above 1,000 µm. The MPs found in the collected dust samples were as high as 1,052 particles g-1. In addition, our results showed that toddlers were more susceptible for MPs intake via dust inhalation. It was estimated that toddlers can inhale on average of 0.86 MPs particles kg-1 day-1 and 312.93 particles kg-1 year-1. The dust samples were also studied for the presence of heavy metals. The highest concentration of heavy metals varied from site to site; however, Fe (16026.52 mg kg-1) and Ni (73.38 mg kg-1) were highest at site 13, Zn (554.99 mg kg-1) and Pb (8.38 mg kg-1) were the highest at site 6, Mn (323.30 mg kg-1) was the highest at site 10, and Co (10.79 mg kg-1), Cr (67.99 mg kg-1), and Cu (55.40 mg kg-1) were highest at sites 17, 1, and 18, respectively. The high EF values for Zn indicates extremely high enrichment. The hazard quotient of the dust samples indicates no adverse impacts of the heavy metals on children and adults. Therefore, it is recommended to monitor MPs and heavy metals pollution in dust samples for human health and sustainable ecosystem.
Data availability statement
The original contributions presented in the study are included in the article/Supplementary Material, further inquiries can be directed to the corresponding author.
Author contributions
MAh: Conceptualization, Resources, Software, Writing–original draft, Writing–review and editing. HA-S: Investigation, Methodology, Writing–original draft, Writing–review and editing. JA: Investigation, Methodology, Writing–review and editing. MAk: Data curation, Validation, Writing–review and editing. MM: Data curation, Methodology, Writing–review and editing. NL: Data curation, Formal Analysis, Writing–review and editing. MA-W: Conceptualization, Resources, Software, Writing–review and editing. AA-F: Supervision, Visualization, Writing–review and editing.
Funding
The author(s) declare that financial support was received for the research, authorship, and/or publication of this article. The authors extend their appreciation to the Deputyship for Research and Innovation, “Ministry of Education” in Saudi Arabia for funding this research work through the project number (IFKSUDR-F161).
Conflict of interest
The authors declare that the research was conducted in the absence of any commercial or financial relationships that could be construed as a potential conflict of interest.
Publisher’s note
All claims expressed in this article are solely those of the authors and do not necessarily represent those of their affiliated organizations, or those of the publisher, the editors and the reviewers. Any product that may be evaluated in this article, or claim that may be made by its manufacturer, is not guaranteed or endorsed by the publisher.
Supplementary material
The Supplementary Material for this article can be found online at: https://www.frontiersin.org/articles/10.3389/fenvs.2024.1377811/full#supplementary-material
References
Abbasi, S., Keshavarzi, B., Moore, F., Delshab, H., Soltani, N., and Sorooshian, A. (2017). Investigation of microrubbers, microplastics and heavy metals in street dust: a study in bushehr city, Iran. Environ. Earth Sci. 76, 798–819. doi:10.1007/s12665-017-7137-0
Abbasi, S., Keshavarzi, B., Moore, F., Turner, A., Kelly, F. J., Dominguez, A. O., et al. (2019). Distribution and potential health impacts of microplastics and microrubbers in air and street dusts from Asaluyeh County, Iran. Environ. Pollut. 244, 153–164. doi:10.1016/j.envpol.2018.10.039
Ahmad, M., Lubis, N. M., Usama, M., Ahmad, J., Al-Wabel, M. I., Al-Swadi, H. A., et al. (2023). Scavenging microplastics and heavy metals from water using jujube waste-derived biochar in fixed-bed column trials. Environ. Pollut. 335, 122319. doi:10.1016/j.envpol.2023.122319
Alloway, B., Centeno, J. A., Finkelman, R. B., Fuge, R., Lindh, U., and Smedley, P. (2005). in Essentials of medical geology. Editor O. Selinus (Selinus: Academic Press).
Alotaibi, M. O., Albedair, L. A., Alotaibi, N. M., Elobeid, M. M., Al-Swadi, H. A., Alasmary, Z., et al. (2022). Pollution indexing and health risk assessment of heavy-metals-laden indoor and outdoor dust in elementary school environments in Riyadh, Saudi Arabia. Atmosphere 13 (3), 464. doi:10.3390/atmos13030464
Alsabhan, A. H., Perveen, K., and Alwadi, A. S. (2022). Heavy metal content and microbial population in the soil of Riyadh Region, Saudi Arabia. J. King Saud. Univ.-Sci. 34, 101671. doi:10.1016/j.jksus.2021.101671
Al-Swadi, H. A., Usman, A. R. A., Al-Farraj, A. S., Al-Wabel, M. I., Ahmad, M., and Al-Faraj, A. (2022). Sources, toxicity potential, and human health risk assessment of heavy metals-laden soil and dust of urban and suburban areas as affected by industrial and mining activities. Sci. Rep. 12, 8972. doi:10.1038/s41598-022-12345-8
Andrady, A. L. (2011). Microplastics in the marine environment. Mar. Pollut. Bull. 62, 1596–1605. doi:10.1016/j.marpolbul.2011.05.030
Besseling, E., Quik, J. T., Sun, M., and Koelmans, A. A. (2017). Fate of nano-and microplastic in freshwater systems: a modeling study. Environ. Pollut. 220, 540–548. doi:10.1016/j.envpol.2016.10.001
Borrelle, S. B., Ringma, J., Law, K. L., Monnahan, C. C., Lebreton, L., McGivern, A., et al. (2020). Predicted growth in plastic waste exceeds efforts to mitigate plastic pollution. Science 369 (6510), 1515–1518. doi:10.1126/science.aba3656
Chen, T. B., Zheng, Y. M., Lei, M., Huang, Z. C., Wu, H. T., Chen, H., et al. (2005). Assessment of heavy metal pollution in surface soils of urban parks in Beijing, China. Chemosphere 60 (4), 542–551. doi:10.1016/j.chemosphere.2004.12.072
Cheng, Z., Chen, L. J., Li, H. H., Lin, J. Q., Yang, Z. B., Yang, Y. X., et al. (2018). Characteristics and health risk assessment of heavy metals exposure via household dust from urban area in Chengdu, China. China. Sci. Total Environ. 619, 621–629. doi:10.1016/j.scitotenv.2017.11.144
Cox, K. D., Covernton, G. A., Davies, H. L., Dower, J. F., Juanes, F., and Dudas, S. E. (2019). Human consumption of microplastics. Environ. Sci. Technol. 53 (12), 7068–7074. doi:10.1021/acs.est.9b01517
Dehghani, S., Moore, F., and Akhbarizadeh, R. (2017). Microplastic pollution in deposited urban dust, Tehran metropolis, Iran. Environ. Sci. Pollut. Res. 24, 20360–20371. doi:10.1007/s11356-017-9674-1
Dris, R., Gasperi, J., Mirande, C., Mandin, C., Guerrouache, M., Langlois, V., et al. (2017). A first overview of textile fibers, including microplastics, in indoor and outdoor environments. Environ. Pollut. 221, 453–458. doi:10.1016/j.envpol.2016.12.013
Gasperi, J., Wright, S. L., Dris, R., Collard, F., Mandin, C., Guerrouache, M., et al. (2018). Microplastics in air: are we breathing it in? Curr. Opin. Environ. Sci. Health 1, 1–5. doi:10.1016/j.coesh.2017.10.002
Guo, J. J., Huang, X. P., Xiang, L., Wang, Y. Z., Li, Y. W., Li, H., et al. (2020). Source, migration and toxicology of microplastics in soil. Environ. Int. 137, 105263. doi:10.1016/j.envint.2019.105263
Hakanson, L. (1980). An ecological risk index for aquatic pollution control. A sedimentological approach. Water Res. 14 (8), 975–1001. doi:10.1016/0043-1354(80)90143-8
Ho, K. F., Lee, S. C., Chow, J. C., and Watson, J. G. (2003). Characterization of PM10 and PM2. 5 source profiles for fugitive dust in Hong Kong. Atmos. Environ. 37 (8), 1023–1032. doi:10.1016/s1352-2310(02)01028-2
Hossner, L. R. (1996). in Dissolution for total elemental analysis. Editor D. L. Sparks (Madison, WI, USA: Soil Science Society of America Inc).
Huang, S., Tu, J., Liu, H., Hua, M., Liao, Q., Feng, J., et al. (2009). Multivariate analysis of trace element concentrations in atmospheric deposition in the Yangtze River Delta, East China. Atmos. Environ. 43 (36), 5781–5790. doi:10.1016/j.atmosenv.2009.07.055
Jeyasanta, K. I., Sathish, N., Patterson, J., and Edward, J. P. (2020). Macro-meso-and microplastic debris in the beaches of Tuticorin district, Southeast coast of India. Mar. Pollut. Bull. 154, 111055. doi:10.1016/j.marpolbul.2020.111055
Kang, H., Park, S., Lee, B., Kim, I., and Kim, S. (2022). Concentration of microplastics in road dust as a function of the drying period—a case study in G City, Korea. Sustainability 14 (5), 3006. doi:10.3390/su14053006
La Porta, E., Exacoustos, O., Lugani, F., Angeletti, A., Chiarenza, D. S., Bigatti, C., et al. (2023). Microplastics and kidneys: an update on the evidence for deposition of plastic microparticles in human organs, tissues and fluids and renal toxicity concern. Int. J. Mol. Sci. 24 (18), 14391. doi:10.3390/ijms241814391
Lehner, R., Weder, C., Petri-Fink, A., and Rothen-Rutishauser, B. (2019). Emergence of nanoplastic in the environment and possible impact on human health. Environ. Sci. Technol. 53 (4), 1748–1765. doi:10.1021/acs.est.8b05512
Li, W. C., Tse, H. F., and Fok, L. (2016). Plastic waste in the marine environment: a review of sources, occurrence and effects. Sci. Total Environ. 566, 333–349. doi:10.1016/j.scitotenv.2016.05.084
Liao, C., Liu, F., Guo, Y., Moon, H. B., Nakata, H., Wu, Q., et al. (2012). Occurrence of eight bisphenol analogues in indoor dust from the United States and several Asian countries: implications for human exposure. Environ. Sci. Technol. 46 (16), 9138–9145. doi:10.1021/es302004w
Liu, C., Li, J., Zhang, Y., Wang, L., Deng, J., Gao, Y., et al. (2019). Widespread distribution of PET and PC microplastics in dust in urban China and their estimated human exposure. Environ. Int. 128, 116–124. doi:10.1016/j.envint.2019.04.024
Manasreh, W. A. (2010). Assessment of trace metals in street dust of Mutah city, Karak, Jordan. Carpath. J. Earth Environ. Sci. 5 (1), 5–12.
Maqsoom, A., Aslam, B., Hassan, U., Kazmi, Z. A., Sodangi, M., Tufail, R. F., et al. (2020). Geospatial assessment of soil erosion intensity and sediment yield using the revised universal soil loss equation (RUSLE) model. ISPRS Int. J. Geo-Inf. 9 (6), 356. doi:10.3390/ijgi9060356
Monira, S., Bhuiyan, M. A., Haque, N., Shah, K., Roychand, R., Hai, F. I., et al. (2021). Understanding the fate and control of road dust-associated microplastics in stormwater. Process Saf. Environ. Prot. 152, 47–57. doi:10.1016/j.psep.2021.05.033
Narmadha, V. V., Jose, J., Patil, S., Farooqui, M. O., Srimuruganandam, B., Saravanadevi, S., et al. (2020). Assessment of microplastics in roadside suspended dust from urban and rural environment of Nagpur, India. Int. J. Environ. Res. 14, 629–640. doi:10.1007/s41742-020-00283-0
Nematollahi, M. J., Zarei, F., Keshavarzi, B., Zarei, M., Moore, F., Busquets, R., et al. (2022). Microplastic occurrence in settled indoor dust in schools. Sci. Total Environ. 807, 150984. doi:10.1016/j.scitotenv.2021.150984
O’Brien, S., Okoffo, E. D., Rauert, C., O’Brien, J. W., Ribeiro, F., Burrows, S. D., et al. (2021). Quantification of selected microplastics in Australian urban road dust. J. Hazard. Mater. 416, 125811. doi:10.1016/j.jhazmat.2021.125811
Opher, T., and Friedler, E. (2010). Factors affecting highway runoff quality. Urban Water J. 7, 155–172. doi:10.1080/15730621003782339
Plastic Europe (2023). Plastics – the fast facts 2023. Available at: https://plasticseurope.org/knowledge-hub/plastics-the-fast-facts-2023/(Accessed on March 18, 2024).
Prabhakar, G., Sorooshian, A., Toffol, E., Arellano, A. F., and Betterton, E. A. (2014). Spatiotemporal distribution of airborne particulate metals and metalloids in a populated arid region. Atmos. Environ. 92, 339–347. doi:10.1016/j.atmosenv.2014.04.044
Rahman, A., Sarkar, A., Yadav, O. P., Achari, G., and Slobodnik, J. (2021). Potential human health risks due to environmental exposure to nano-and microplastics and knowledge gaps: a scoping review. Sci. Total Environ. 757, 143872. doi:10.1016/j.scitotenv.2020.143872
Rezaei, M., Riksen, M. J., Sirjani, E., Sameni, A., and Geissen, V. (2019). Wind erosion as a driver for transport of light density microplastics. Sci. Total Environ. 669, 273–281. doi:10.1016/j.scitotenv.2019.02.382
Rillig, M. C., Kim, S. W., Kim, T. Y., and Waldman, W. R. (2021). The global plastic toxicity debt. Environ. Sci. Technol. 55 (5), 2717–2719. doi:10.1021/acs.est.0c07781
Saeedi, M., Li, L. Y., and Salmanzadeh, M. (2012). Heavy metals and polycyclic aromatic hydrocarbons: pollution and ecological risk assessment in street dust of Tehran. J. Hazard. Mater. 227, 9–17. doi:10.1016/j.jhazmat.2012.04.047
Sarker, A., Deepo, D. M., Nandi, R., Rana, J., Islam, S., Rahman, S., et al. (2020). A review of microplastics pollution in the soil and terrestrial ecosystems: a global and Bangladesh perspective. Sci. Total Environ. 733, 139296. doi:10.1016/j.scitotenv.2020.139296
Siegfried, M., Koelmans, A. A., Besseling, E., and Kroeze, C. (2017). Export of microplastics from land to sea. A modelling approach. Water Res. 127, 249–257. doi:10.1016/j.watres.2017.10.011
Stanton, T., Johnson, M., Nathanail, P., MacNaughtan, W., and Gomes, R. L. (2019). Freshwater and airborne textile fibre populations are dominated by ‘natural’, not microplastic, fibres. Sci. Total Environ. 666, 377–389. doi:10.1016/j.scitotenv.2019.02.278
Sutherland, R. A., Tack, F. M. G., Tolosa, C. A., and Verloo, M. G. (2000). Operationally defined metal fractions in road deposited sediment, Honolulu, Hawaii. Am. Soc. Agron. Crop Sci. Soc. Am. Soil Sci. Soc. Am. 29 (5), 1431–1439. doi:10.2134/jeq2000.00472425002900050009x
Thiele, C. J., Hudson, M. D., Russell, A. E., Saluveer, M., and Sidaoui-Haddad, G. (2021). Microplastics in fish and fishmeal: an emerging environmental challenge? Sci. Rep. 11 (1), 2045. doi:10.1038/s41598-021-81499-8
Thompson, R. C., Olsen, Y., Mitchell, R. P., Davis, A., Rowland, S. J., John, A. W., et al. (2004). Lost at sea: where is all the plastic? Science 304 (5672), 838. doi:10.1126/science.1094559
Turekian, K. K., and Wedepohl, K. H. (1961). Distribution of the elements in some major units of the earth's crust. Geol. Soc. Am. Bull. 72 (2), 175–192. doi:10.1130/0016-7606(1961)72[175:doteis]2.0.co;2
Us Epa, U. (2011). Exposure factors handbook. United States: United States Environmental Protection Agency.
Vianello, A., Jensen, R. L., Liu, L., and Vollertsen, J. (2019). Simulating human exposure to indoor airborne microplastics using a Breathing Thermal Manikin. Sci. Rep. 9 (1), 8670. doi:10.1038/s41598-019-45054-w
Vogelsang, C., Lusher, A., Dadkhah, M. E., Sundvor, I., Umar, M., Ranneklev, S. B., et al. (2019). Microplastics in road dust–characteristics, pathways and measures. NIVA-rapport.
Wang, L., Lu, X., Ren, C., Li, X., and Chen, C. (2014). Contamination assessment and health risk of heavy metals in dust from Changqing industrial park of Baoji, NW China. Environ. Earth Sci. 71, 2095–2104. doi:10.1007/s12665-013-2613-7
Wong, C. S., Li, X., and Thornton, I. (2006). Urban environmental geochemistry of trace metals. Environ. Pollut. 142 (1), 1–16. doi:10.1016/j.envpol.2005.09.004
Wong, J. K. H., Lee, K. K., Tang, K. H. D., and Yap, P. S. (2020). Microplastics in the freshwater and terrestrial environments: prevalence, fates, impacts and sustainable solutions. Sci. Total Environ. 719, 137512. doi:10.1016/j.scitotenv.2020.137512
Ye, C., Li, S., Zhang, Y., and Zhang, Q. (2011). Assessing soil heavy metal pollution in the water-level-fluctuation zone of the Three Gorges Reservoir, China. J. Hazard. Mater. 191 (1-3), 366–372. doi:10.1016/j.jhazmat.2011.04.090
Yongming, H., Peixuan, D., Junji, C., and Posmentier, E. S. (2006). Multivariate analysis of heavy metal contamination in urban dusts of Xi'an, Central China. Sci. Total Environ. 355 (1-3), 176–186. doi:10.1016/j.scitotenv.2005.02.026
Yukioka, S., Tanaka, S., Nabetani, Y., Suzuki, Y., Ushijima, T., Fujii, S., et al. (2020). Occurrence and characteristics of microplastics in surface road dust in kusatsu (Japan), da nang (Vietnam), and kathmandu (Nepal). Environ. Pollut. 256, 113447. doi:10.1016/j.envpol.2019.113447
Zhang, J., Wang, L., and Kannan, K. (2020). Microplastics in house dust from 12 countries and associated human exposure. Environ. Int. 134, 105314. doi:10.1016/j.envint.2019.105314
Zhang, S., Yang, X., Gertsen, H., Peters, P., Salánki, T., and Geissen, V. (2018). A simple method for the extraction and identification of light density microplastics from soil. Sci. Total Environ. 616, 1056–1065. doi:10.1016/j.scitotenv.2017.10.213
Keywords: microplastics pollution, heavy metals, estimated intake, human exposure, risk assessment
Citation: Ahmad M, Al-Swadi HA, Ahmad J, Akanji MA, Mousa MA, Lubis NMA, Al-Wabel MI and Al-Farraj ASF (2024) Pollution and health risk assessment of co-existing microplastics and heavy metals in urban dust of Riyadh city, Saudi Arabia. Front. Environ. Sci. 12:1377811. doi: 10.3389/fenvs.2024.1377811
Received: 28 January 2024; Accepted: 28 March 2024;
Published: 16 April 2024.
Edited by:
Mezbaul Bahar, The University of Newcastle, AustraliaReviewed by:
Hassan Taghipour, Tabriz University of Medical Sciences, IranMahbub Alam, Bangladesh University of Professionals, Bangladesh
Copyright © 2024 Ahmad, Al-Swadi, Ahmad, Akanji, Mousa, Lubis, Al-Wabel and Al-Farraj. This is an open-access article distributed under the terms of the Creative Commons Attribution License (CC BY). The use, distribution or reproduction in other forums is permitted, provided the original author(s) and the copyright owner(s) are credited and that the original publication in this journal is cited, in accordance with accepted academic practice. No use, distribution or reproduction is permitted which does not comply with these terms.
*Correspondence: Munir Ahmad, YW11bmlyQGtzdS5lZHUuc2E=