- 1Guangdong Energy Group Science and Technology Research Institute Co., Ltd., Guangzhou, China
- 2Key Laboratory of Low-Grade Energy Utilization Technologies and Systems of Ministry of Education, Chongqing University, Chongqing, China
- 3National-Regional Joint Engineering Research Center for Soil Pollution Control and Remediation in South China, Guangdong Key Laboratory of Integrated Agro-Environmental Pollution Control and Management, Institute of Eco-Environmental and Soil Sciences, Guangdong Academy of Sciences, Guangzhou, China
- 4College of Horticulture and Landscape Architecture, Zhongkai University of Agriculture and Engineering, Guangzhou, China
- 5Guangdong Energy Group Co., Ltd., Guangzhou, China
Cyanobacteria plays an important role in other ecological processes in paddy soils, particularly in terms of nitrogen input to the ecosystem. Organic fertilizer and biochar are common soil amendment materials used to preserve soil health in agricultural intensification background. However, the consequent increase in soil nutrition may inhibit soil cyanobacteria, therefore decreasing nitrogen fixation and changes other soil processes. To test this hypothesis, we established a 2 × 2 full factorial experiment in a paddy field in South China, which included four treatments: Ctr (control, receiving no organic fertilization or biochar addition), +OF (organic fertilizer application only), +BC (biochar application only), and +Mix (organic fertilizer and biochar applications). The soil cyanobacterial community was analyzed using metagenomics technology, and 14 soil property variables were measured. The results suggested that organic fertilizer was effective in enhancing nutrient levels, leading to a significant increase in extractable and soluble nitrogen, phosphorus, and potassium. In contrast, biochar application had a stronger effect on total soil carbon, potassium, and soil pH. However, both organic fertilizer and biochar applications induced significant decreases in overall cyanobacterial abundance and species number. Dominant cyanobacterial organisms, particularly the two most abundant genera, Leptolyngbya and Phormidium, experienced a greater decrease compared to others. Canonical correlation analyses and structural equation models indicated that organic fertilizer and biochar applications affected soil cyanobacterial community mainly through soil available nitrogen and pH. In total, the present study highlighted that both organic fertilizer and biochar applications in paddy soils notably change soil physicochemical traits, inhibiting rather than benefiting cyanobacterial microorganisms, especially the dominant ones, and potentially reducing nitrogen input. Our study reveals the impacts of oragnic fertilizer and biochar applications in paddies on soil cyanobacteria and how the consequent changes in soil properties mediate this impact, thereby enhancing our understanding of the responses of different soil microbial groups to soil improvement measures.
1 Introduction
Cyanobacteria is an important soil microbial guild in paddy soils (De, 1939; Dodds et al., 1995; Herridge et al., 2008). As a component of soil microflora, cyanobacteria acts as a reserve for plant nutrients, influences soil structure and the activities of other organisms, and plays a role in the incorporation of organic C and N through photosynthesis and N2 fixation (Pankratova, 2006; Hayat et al., 2010; Jassey et al., 2022). It is also a key part of the beneficial plant microbiome, helping plants to protect against biotic and abiotic stresses (Lee and Ryu, 2021). In rice production, moderate but constant yields have been obtained for decades when rice was grown without N fertilizer, and biological N2 fixation is the major exported N replacement mechanism (Roger et al., 1986). Hundreds of papers have been published on cyanobacterial use as biofertilizer (Roger and Kulasooriya, 1980). Recent studies also suggest there is high biodiversity in agricultural land soils, especially in paddy fields (Prasanna et al., 2012; Ma et al., 2019; Song J. et al., 2022). However, the ecology of cyanobacteria in paddy soils is imperfectly understood.
Organic fertilizer, including crop residues, livestock manure, and so on, has been widely used in rice production. Rice serves as the primary food supply for almost half of the global population and is cultivated across approximately 165 million hectares, accounting for roughly 11% of the world’s agricultural land (Liu et al., 2019; FAOSTAT, 2023). Excessive paddy cultivation degrades soil quality, leads to soil organic matter deficiency (Ren X. et al., 2020), and prevents the soil from receiving adequate supplies of essential nutrients, including nitrogen (N), and phosphorus (P), due to leaching and N gas emissions (Cao et al., 2014). Improving soil quality to enhance rice production while preserving the ecological balance of rice paddy ecosystems has become a crucial endeavor. Organic fertilizer increases crop yield and quality in ways similar to inorganic fertilizers (Bulluck et al., 2002; Sharma and Chetani, 2017; Wu et al., 2021), but it does not cause environmental pollution and soil degradation like inorganic fertilizer. Important advantages of organic fertilizer include improving soil nutrition, texture, water retention and resistance to erosion (Lima et al., 2009; Maltas et al., 2013; Wu et al., 2021). In rice paddies, organic fertilizer has been proven to increase soil quality and rice production (Siavoshi et al., 2011). Biochar is a carbon-rich substance obtained from the pyrolysis of waste feedstock. Biochar itself has a relatively lower nutrient content than organic fertilizer, however it has a high cation exchange capacity due to its porous structure and negative surface charge, which means it can adsorb and hold onto nutrients in the soil, preventing them from leaching away (Sohi et al., 2010; Novair et al., 2023). As a result, biochar has multiple benefits in promoting soil carbon sequestration, increasing plant nutrient uptake, improving soil aggregation and water holding capacity, enhancing soil nutrient availability, reducing GHGs emissions, benefiting crop growth and productivity, and being efficient in immobilizing harmful chemical pollutants (Sohi et al., 2010; Zhao et al., 2014). These traits make it a potential material in soil remediation and improvement. However, it is less used in paddy soil improvement, and little is known about how it will interact with organic fertilizer application.
Soil cyanobacteria may respond very differently to organic fertilizer and biochar applications from other microbial guilds. Metting (1981) summarized that soil cyanobacteria, as other soil algae, may be significantly influenced by a number of environmental factors: humidity, soil temperature, soil texture, рН, nutrition, and biotic interactions. As organic fertilization and biochar applications may significantly affect soil physicochemical property (Lima et al., 2009; Novair et al., 2023), they should have great impacts on soil cyanobacterial community. Generally, organic fertilizer provides a large amount of diverse organic C source, increases nutrient availability, improves soil structure, significantly influencing soil microbe (Werner and Dindal, 1990; Ponge et al., 2013; Viketoft et al., 2021). In contrast, biochar affects soil microbes by providing additional microbial habitat, nutrient retention, pH buffering, and so on (Lehmann et al., 2011; Palansooriya et al., 2019). Therefore, in paddy soils, both organic fertilizer (Lv et al., 2011; Yang et al., 2019; Liu et al., 2021) and biochar (Liu et al., 2022) applications increase microbial biomass and change microbial diversity, mostly through their effects on soil property. However, there are few studies reporting changes in soil cyanobacterial communities due to organic fertilization and biochar applications in agricultural lands (Werner and Dindal, 1990; Lehmann et al., 2011; Viketoft et al., 2021). Recent researches typically do not distinguish cyanobacteria from other microbial organisms, either look deep into changes in cyanobacterial assemblages. Indeed, the responses of soil cyanobacteria to organic fertilizer and biochar applications could be different from other soil microbes because of their distinct physiological characteristics (Metting, 1981; Whitton and Potts, 2012; Gaysina et al., 2019). Autotrophic cyanobacteria may be insensitive to increased soil organic matter due to soil remediation measures as their carbon source is CO2. In contrast, some cyanobacterial species are diazotrophs, and thus exerting distinct responses to increased nutrient availability from other microbial guild. Because diazotrophs is able to acquire atmospheric N, some cyanobacterial may be more responsive to P enhancement but not N. Moreover, cyanobacteria usually blooms in the early succession stage as typical pioneer organism in terrestrial ecosystem when habitat is oligotrophic. As organic fertilizer and biochar increase nutrient availability, the habitats may be more favorable to other organisms that may outcompete cyanobacterial organism. Nevertheless, empirical evidence in paddy soils supporting these interferences is largely lacking.
Collectively, we hypothesized that 1) organic fertilizer application would strongly increase soil nutrition, while biochar application mainly increased soil carbon and pH; 2) both organic fertilizer and biochar applications might decrease soil cyanobacterial abundance, and differently change soil cyanobacterial community structure.
2 Materials and methods
2.1 Experimental design
The experiment was conducted in a paddy field in Zhanjiang City, Guangdong Province, China. The paddy field had been used for rice production for several years using chemical fertilizers before the experiment. We aimed to understand the effect of organic fertilizer and biochar applications (soil improvements) on the soil cyanobacterial community in paddy fields, and how the resulting changes in soil property mediated the effect. The experiment was a 2 × 2 full factorial design with four treatments: Ctr (control, no organic fertilization or biochar addition), +OF (organic fertilizer application only), +BC (biochar application only), and +Mix (organic fertilizer and biochar applications). The four treatments, each with three replicates, were randomly assigned to 12 rectangular plots (7 m × 8 m), separated by 40 cm wide ditches.
The quantity and frequency of organic fertilizer and biochar applications followed local conventional approachs, which involved application once every 3 years at a rate of 0.25 tons of biochar/0.5 tons organic fertilizer per 667 m2 each time. Specifically, organic fertilizer and biochar were applied by rotary tillage into 0–20 cm soil depth before rice planting in March 2018. Since then, the field was used for cultivating rice, with two crops planted annually. During the crop growth, all treatments received chemical fertilizers at regular doses and frequencies. The organic fertilizer was derived from fermented cow manure, while the biochar was pyrolyzed from discarded eucalyptus tree branches and leaves at temperatures between 550°C and 600°C. The basic characteristics of these two materials are shown in Supplementary Table S1.
2.2 Soil sampling
Soil sampling was conducted in June when water in paddy fields receded during the summer of 2019. In each plot, five soil cores (4.5 cm in diameter) were taken at a depth of 0–10 cm and mixed to form a pooled sample. The soil samples were stored in airtight polypropylene bags and placed in a cool box at 4°C during transportation to the laboratory. After the removal of visible litter, roots, worms, and other debris, the soils were sieved through a 2-mm mesh. Each sample was divided into three subsamples. Subsamples for determining the cyanobacterial community composition were stored at −80°C until cyanobacterial gene extraction. Subsamples for the analysis of dissolved organic carbon (DOC), dissolved nitrogen (DN), ammonium N, and nitrate N content were stored at 4°C and analyzed within 48 h. Subsamples used to measure soil pH, soil organic matter (SOM), soil alkali-hydrolysable N (Nalk), extractable P (Pextra), extractable K (Kextra), total soil N, P, K, Ca, and Mg content were air dried.
2.3 Soil property analysis
Soil pH was determined in a 1:2.5 soil: water slurry using a glass pH electrode (FiveGO™, METTLER TOLEDO, Zurich, Switzerland). SOM was determined using H2SO4-K2Cr2O7 oxidation method. Total soil N was quantified by the Kjeldahl acid digestion method. Total soil P was quantified using the molybdate blue method after acid digestion. To measure total soil K, Ca and Mg, air-dried soil were acid-digested. The solution after the digestion was analyzed following the method described in Liu et al. (1996). NH3 produced from 2 g air-dried soil mixed with 7 mL 1 M NaOH solution for 24 h was measured to determine the soil alkali-hydrolyzable N content. Soil Nextra was the sum of ammonium-N and nitrate-N. Soil ammonium-N and nitrate-N were extracted with 10 g fresh soil by 100 mL 2 M KCl for 30 min within 48 h after soil samplings. Soil Pextra was extracted with 5 g air-dried soil via the Bray 1 method (0.03 M NH4F and 0.025 M HCl, 50 mL) for 5 min. Soil Kextra was extracted with 2.0 g air-dried soil in 100 mL 1 M CH3COONH4 solution for 5 min. Dissolved soil organic C and dissolved soil N were extracted in K2SO4 solution and analyzed using a TOC analyzer (TOC-VCSH, Shimadzu GmbH, Duisburg, Germany).
2.4 Soil cyanobacterial metagenomic analysis
Soil cyanobacterial community genomic DNA extraction was performed using a E. Z.N.A. Soil DNA Kit (Omega, M5635-02, United States). The concentration of the DNA was measured using a Qubit 4.0 (Thermo, United States) to ensure that adequate amounts of high-quality genomic DNA had been extracted. Trimmomatic was used for filtering and evaluating the quality of sequenced data. Raw reads were filtered following these steps: 1) Removing adaptor sequence; 2) Removing low quality bases from reads 3′ to 5’ (Q < 20), using a sliding window method to remove the base value less than 20 of reads tail (window size is 4 bp); 3) Finding overlap of each pair of reads and properly correct inconsistent bases within the interval; 4) Removing reads with length less than 35 nt and its pairing reads. And the remaining clean data was used for further analysis. MEGAHIT was used to perform multi-sample mixed splicing to obtain preliminary spliced contig sequences. Clean reads were mapped back to the spliced results, unmapped reads were extracted, and spliced again using SPAdes to obtain low-abundance contigs. MetaWRAP was used to perform a series of binning, and bin identification are performed in sequence. After filtering, a draft genome of a single bacteria with high integrity and low contamination is obtained. We used DIAMOND to compare the gene set with NR databases to obtain species annotation information of genes. Screening conditions: e-value <1×e−5, Score >60. Based on gene set abundance information and annotation information, species abundance are obtained.
2.5 Statistical analysis
Structural equation model (SEM) analyses were carried out in Amos 21.0 (IBM SPSS Statistics). Other statistical analyses were conducted in R version 4.1.2 (R Core Team, 2020). Shannon’s diversity index (H) was calculated as diversity index using the function diversity () in R package “vegan” (Oksanen et al., 2018). We used two-way ANOVA to examine the effects of organic fertilizer and biochar applications on soil cyanobacterial abundance (gene copy number), species richness (observed OTUs number), Shannon’s H, gene copy number of each genus and soil property. To compare these characteristics among each treatment, one way ANOVA was carried out followed by Tukey’s HSD. Multivariate effects of soil improvement on soil cyanobacterial community structure were illustrated by plotting ordination structure of a canonical correlation analysis (CCA) dependent on organic fertilizer and biochar applications. Environmental factors were plotted on the ordination using envfit () function in “vegan”. Heatmaps of OTUs representing their abundances and relative abundance in each treatments were displayed use heatmap () in “pheatmap” package. Linear regression analyses were conducted to reveal the relationships between change rates due to soil improvements and gene copy number for each cyanobacterial genus. The change rates were the differences of gene copy number between the soil improvement treatments and Ctr treatment divided by gene copy number in the Ctr treatment. Linear regression analyses were also used to explore responses of soil cyanobacterial abundance, species richness and Shannon’s H to soil property changes. The best models including different explanatory variables number were obtained using the regsubsets () function in “leap” package. To avoid overfitting, models with more than 2 independent variables were not considered.
3 Results
3.1 Effect of organic fertilizer and biochar applications on soil cyanobacterial community
A one-way ANOVA indicated significant variations in soil cyanobacterial abundance (p = 0.004), species richness (p = 0.025), and Shannon’s H (p = 0.009) among Ctr, +OF, +BC, and +Mix (Figure 1). The soil cyanobacterial abundance in Ctr was the highest, significantly higher than those of soil improvement treatments (+OF, +BC, and +Mix) (all p < 0.017). In comparison to Ctr, both + OF (p = 0.031) and +BC (p = 0.048) treatments significantly reduced soil species richness. The + Mix treatment showed a tendency to decrease species (p = 0.070). Conversely, soil improvement practices led to an increase in Shannon’s H. The + OF treatment significantly elevated Shannon’s H (p = 0.006), the +BC exhibited a tendency to decrease it (p = 0.059), while the +Mix’s enhancement was not statistically significant (p = 0.327). A two-way ANOVA revealed significant interactive effects between organic fertilizer and biochar applications on soil cyanobacterial abundance (p = 0.005), species richness (p = 0.027), and Shannon’s H (p = 0.003) (Supplementary Table S2).
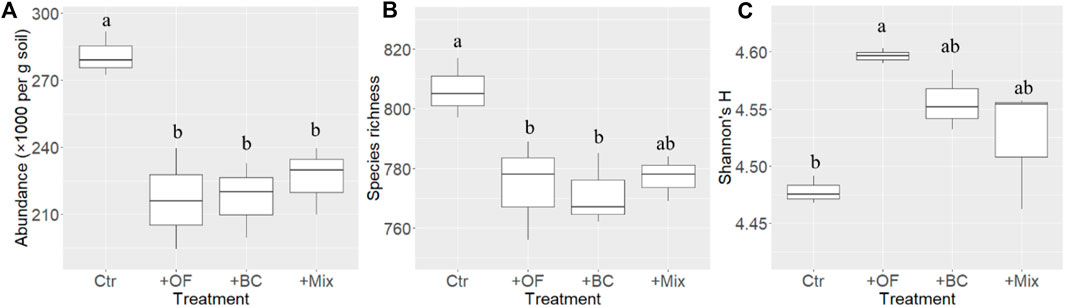
Figure 1. Soil cyanobacterial (A) abundance, (B) species richness, and (C) Shannon’ H index in Ctr (control), +OF (organic fertilizer application), +BC (biochar application) and +Mix (simultaneous application of organic fertilizer and biochar). Different letters indicate significant differences (p < 0.050).
Canonical correlation analysis (CCA) indicated a significant interactive effect between organic fertilizer (OF) and biochar (BC) application (p = 0.035, Figure 2). The ordination plot of the CCA further illustrated that both organic fertilizer (p = 0.029) and biochar (p = 0.007) applications had a substantial impact on soil cyanobacterial structure. All soil improvement plots were distinct from Ctr plots, while + OF plots showed a tendency to differ from +BC and +Mix.
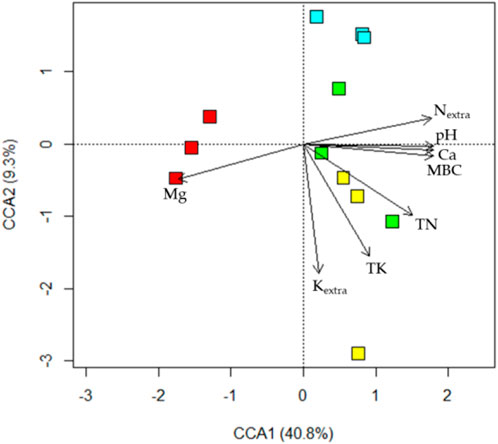
Figure 2. The ordination plot depicts the outcomes of a canonical correlation analysis (CCA) that explores the impact of organic fertilizer and biochar application on the soil cyanobacterial gene matrix. In the graph, Ctr plots are represented by red squares, +OF plots by cyan, +BC plots by green, and +Mix plots by yellow. The CCA model exhibits an adjusted r2 of 0.350 with a significant p-value of 0.007. Fixed factor effects include organic fertilizer application (p = 0.028), biochar application (p = 0.007), and the interaction between organic fertilizer and biochar (p = 0.035). Soil variables were incorporated into the ordination using the envfit () function, and arrows representing vectors significantly correlated with the data are presented (p < 0.050). pH: soil pH; TN: total soil N; TK: total soil K; Nextra: soil extractable N; Kextra: soil extractable K; Ca: total soil Ca; Mg: total soil Mg; MBC: microbial biomass carbon.
A heatmap featuring all Operational Taxonomic Units (OTUs) also indicated that the 12 plots could be broadly grouped into two major clusters, labeled as A and B (Supplementary Figure S1), aligning with the Canonical Correlation Analysis (CCA) results. Cluster A consisted of 3 Ctr plots, while cluster B encompassed soil improvement plots. In Figure 3, the abundance and relative abundance of the top 200 abundant cyanobacterial OTUs in each treatment were illustrated. Soil improvement led to a decline in the majority of OTUs (Figure 3A). Interestingly, the relative abundance of several of the most abundant OTUs in Ctr was higher than those in other treatments, whereas most other OTUs were generally more prevalent in soil improvement treatments (Figure 3B). These response patterns suggested that the most abundant OTUs experienced a more significant decrease compared to other OTUs due to soil improvements, contributing to the alterations in the soil cyanobacterial community. The heatmap analysis involving all OTUs indicated that, for those OTUs with very low abundance, there were no apparent differences in abundance or relative abundance between Ctr and soil improvement treatments. However, owing to their extremely low read numbers, they exerted minimal influence on the overall size of the community.
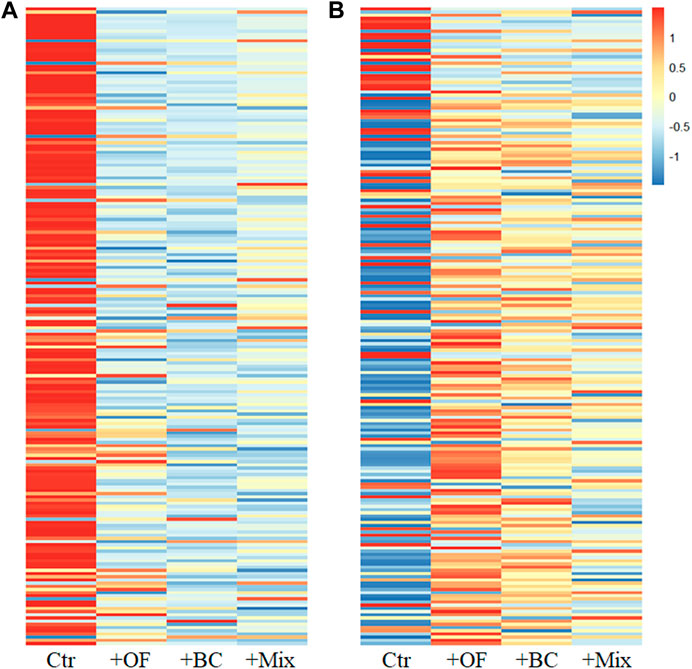
Figure 3. Heatmaps depicting (A) abundance and (B) relative abundance of the top 200 most abundant cyanobacterial Operational Taxonomic Units (OTUs) in each treatment. The values have been row-standardized, with colors in the figure corresponding to relative values. In the heatmap, rows represent cyanobacterial OTUs, and columns represent treatments. The OTUs are arranged in descending order based on absolute abundance, from top to bottom. For a comprehensive view of heatmaps with all OTUs, refer to the Supplementary Material (Supplementary Figure S1).
At the genus level, notable differences were observed among the four treatments, particularly between Ctr and soil improvement treatments. In Ctr plots, the six most abundant cyanobacterial genera, in descending order, were Leptolyngbya, Microcoleus, Phormidium, Nostoc, Calothrix and Scytonema. Conversely, in the soil improvement plots, the order shifted to Leptolyngbya, Microcoleus, Nostoc, Phormidium, Calothrix and Hassallia. Differences in the abundance of each cyanobacterial genus among the four treatments were examined through one-way ANOVA followed by Tukey’s HSD test (Table 1), and the results were consistent with the heatmap analyses. The relative abundance of Leptolyngbya and Phormidium, the two most abundant cyanobacterial genera, decreased due to soil improvements, while the majority of others increased. This finding aligns with the results obtained from the analysis of OTUs data, providing insights into specific changes at the cyanobacterial genus level. Linear regressions suggested that the more abundant a genus was, the more it decreased due to soil improvements (Supplementary Figure S2).
3.2 Effects of organic fertilizer and biochar applications on soil property
The applications of organic fertilizer and biochar exerted significant effects on soil properties. Organic fertilizer application resulted in notable changes in soil pH, TP, Pextra, Kextra, Ca, DN and Nextra (all p < 0.050) (Table 2). In comparison, biochar application demonstrated a more pronounced impact on soil properties than organic fertilization, significantly altering soil pH, SOM, TN, TP, TK, Pextra, Kextra, Ca, DN and Nextra (all p < 0.050). Furthermore, significant interactive effects between organic fertilizer and biochar application were observed concerning soil pH, Pextra, Kextra, Ca, DOC and Nextra (all p < 0.050). Detailed changes and the results of Tukey’s HSD can be found in Supplementary Table S3 in the Supplementary Material.
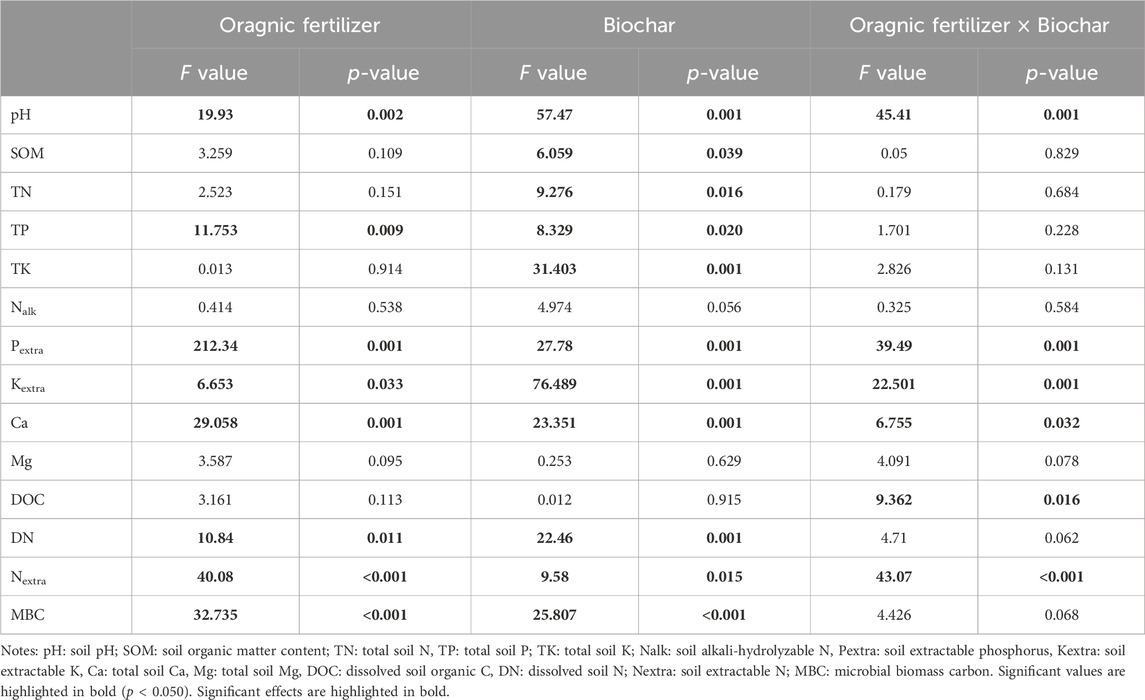
Table 2. Results of two-way ANOVA examining the effect of organic fertilizer and biochar applications on soil property.
3.3 Relationship between soil cyanobacterial community and soil property
A model included only Nextra explained as high as 78% variation of soil cyanobacterial abundance (p < 0.001, Table 3). The model also suggested soil cyanobacterial abundance was negatively correlated with Nextra (p < 0.001). Soil cyanobacterial species richness was negatively affected by Nextra (R2 = 0.709, p < 0.001). Incorporating Nalk into the model slightly improve the model’s r2 (R2 = 0.841, p < 0.001). Nextra explained 52% variation of Shannon’s H (p = 0.005), while pH and TK together explained 79% (p < 0.001). Shannon’s H was positively correlated with Nextra (p = 0.005) and pH (p < 0.001), but negatively correlated with TK (p = 0.018). The present study found that Nextra, TK, pH, Ca, TN, MBC, Kextra, and Mg were significantly correlated with soil cyanobacterial community structure (all p < 0.016) (Figure 2), and Nextra had the highest R2.
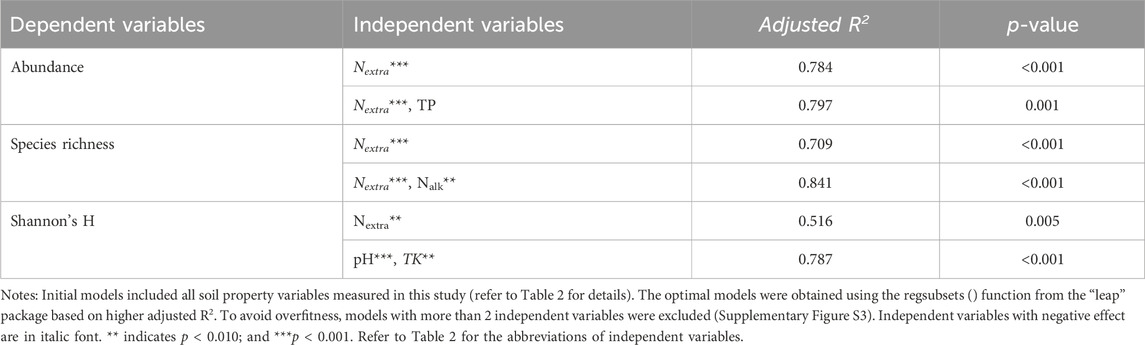
Table 3. Results from all-subset linear regression analysis relating soil cyanobacterial abundance, species number and Shannon’s H to soil physicochemical characteristic.
3.4 Structural equation models explaining how organic fertilizer and biochar applications influenced soil cyanobacteria through soil physicochemical property
Our structural equation models (SEM) revealed how organic fertilizer and biochar applications affected soil cyanobacterial abundance, species number and Shannon’s H (Figure 4) through influencing soil physicochemical characteristic. Both organic fertilizer and biochar applications affect cyanobacterial abundance through its effects on soil pH and the consequent Nextra changes (p = 0.468, χ2 = 3.569, RMSEA <0.001) (Figure 4A). The model indicated Nextra was strongly and negatively impacted cyanobacterial abundance (r = −0.90, p < 0.001). The change of Nextra could be directly influenced by organic fertilizer (r = 0.27, p = 0.055) and biochar (r = −0.30, p = 0.081) applications. However, Nextra was more closely correlalated with soil pH (r = 0.92, p < 0.001) which was postively affected by both organic fertilizer (r = 0.39, p = 0.043) and biochar (r = 0.66, p < 0.001) applications. The SEM for soil cyanobacterial species richness revealed a mechanism illustrating the impact of soil improvements on it, similar to soil cyanobacterial abundance (p = 0.497, χ2 = 3.375, RMSEA <0.001) (Figure 4B). In contrast, organic fertilizer and biochar applications influenced soil cyanobacterial Shannon’s H diveristy mostly through soil pH and K (p = 0.878, χ2 = 2.417, RMSEA <0.001) (Figure 4C). The model suggested organic fertilizer mostly influenced cyanobacterial diveristy through increasing soil pH (r = 0.81, p = 0.009). Interestingly, the application of biochar led to an increase in soil pH, thereby enhancing cyanobacterial diversity, but, it also improved soil K availability (r = 0.86, p < 0.001), which in turn suppressed cyanobacterial diversity (r = −0.73, p < 0.001). Although soil Nextra was contained in the final model, it was not the principle factor mediating the effects of organic fertilizer and biochar applications (all p > 0.050).
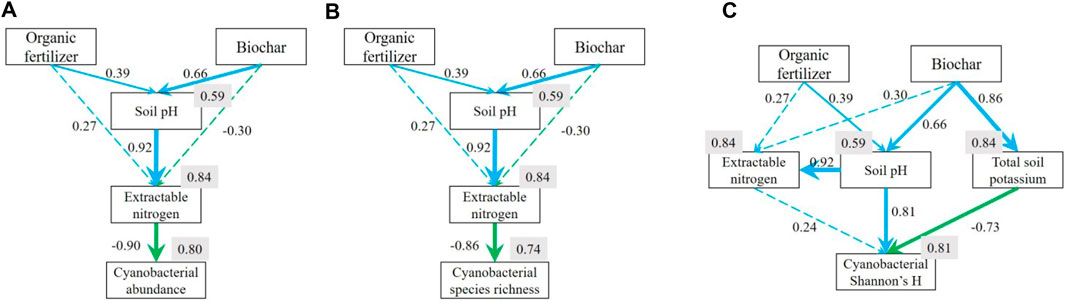
Figure 4. Structural equation models (SEMs) explaining how organic fertilizer and biochar applications affected soil cyanobacterial (A) abundance (p = 0.468, χ2 = 3.569, RMSEA <0.001), (B) species number (p = 0.497, χ2 = 3.375, RMSEA <0.001) and (C) Shannon’s H (p = 0.878, χ2 = 2.417, RMSEA <0.001) through soil physicochemical characteristics. Numbers adjacent to arrows are standardized path coefficients, analogous to partial regression weights and indicative of the effect size of the relationship. Continuous and dashed arrows indicate significant (p < 0.050) and non-significant (p ≥ 0.050) relationship, and blue and green indicate positive and negative relationships, respectively. Width of arrows is proportional to the strength of path coefficients. R2 indicating the proportion of variance explained appears close to every response variable in the model.
4 Discussion
4.1 Organic fertilizer and biochar applications positively but differently affected soil property
Consistent with our hypothesis, the application of organic fertilizer and the addition of biochar, although exhibiting somewhat different effects, both positively contribute to the enhancement of soil nutrition. As shown in previous studies (Maltas et al., 2013; Ren H. et al., 2020; Viketoft et al., 2021), organic fertilizer excels in elevating nutrient levels, leading to a significant and rapid increase in extractable and soluble N, P, and K. In contrast, biochar has higher levels of C and K, but lower levels of N and P. Due to its slow decomposition, biochar made notable contributions to soil structure through its inactive structure (Sohi et al., 2010; Novair et al., 2023). As expected, compared to organic fertilizer application, biochar application had stronger effects on total soil C and K, as well as soil pH. On the other hand, organic fertilizer had a more pronounced impact on soluble soil nutrients, such as extractable NPK, DOC and DN. The distinct effects between organic fertilizer and biochar applications suggested combining biochar and organic fertilizer may offer a comprehensive approach, harnessing the nutrient-rich attributes of organic fertilizer and the structural enhancements provided by biochar. Our results also suggested that there were significant interactive effects between biochar and organic fertilizer applications on soil pH, extractable N, P and K, and other soil characteristics, indicating a combination of these two amendments could provide valuable insights into optimizing soil health and fertility. Therefore, exploring their synergistic potential emerges as a promising avenue for future research.
4.2 Higher-abundance cyanobacteria declined more due to organic fertilizer and biochar applications
Although the effects on paddy soil property between the applications of organic fertilizer and biochar were different, both of them induced significant decreases in overall cyanobacterial abundance and species numbers. In paddy fields, the application of organic fertilizer (Lv et al., 2011; Ponge et al., 2013; Yang et al., 2019; Liu et al., 2021) and biochar (Lehmann et al., 2011; Liu et al., 2022) increased total microbial biomass, which were consistent with the findings of the present study. However, such studies treated the entire soil microbiota as a whole, ignoring the distinctions between cyanobacteria and other microorganisms. The present study revealed that cyanobacterial abundance’s response to organic fertilizer application was contrary to total soil microbial biomass. There were only a few studies studying the effects on soil cyanobacteria (Li et al., 2020). In line with the present study, most of them found organic fertilizer application depressed soil cyanobacterial abundance (Zhang et al., 2017; O'Brien et al., 2018; Li et al., 2020; Song W. et al., 2022). In a 5-year organic fertilization experiment in paddy soils, Song W. et al. (2022) suggested that replacement of replacing chemical fertilizers with organic fertilizers turned cyanobacteria to be non-dominant, suggesting the organic fertilizer appliaction inhibited the soil cyanobacteria. In a cabbage field, rhizosphere cyanobacterial abundance in synthetically fertilized soils also delined relative to control (O'Brien et al., 2018). As a result, we concluded that organic fertilizer application will decreased soil cyanobacteria. The impact of biochar application on soil cyanobacterial communities was seldomly studied and remained controversial (Kholssi et al., 2018; Cheng et al., 2019; Ren H. et al., 2020). Consistent with the present study, in rapeseed and maize fields in karst regions of southwestern China, long-term biochar addition decreased soil cyanobacterial abundance (Cheng et al., 2019). In contrast, in a eucalyptus seedling plantation in the neigbour province, biochar application increased the relative abundance of cyanobacteria (Ren H. et al., 2020). And a 20 days of incubation suggested the growth of filamentous cyanobacteria was increased on biochar solid supports (Kholssi et al., 2018). These contrasted results suggested that the impacts of biochar application on soil cyanobacteria may be dependent on specific ecosystem. In crop lands, as in our study, biochar application seemed to inhibit soil cyanobacteria, while in forests and lab incubation, biochar application tended to favour soil cyanobacteria.
While the majority of cyanobacterial species declined, the prominent cyanobacterial species, particularly the two most abundant genera, Leptolyngbya and Phormidium, exhibited more pronounced decreases. The responsiveness of soil microbial species to environmental changes can vary, influenced by the nature and extent of changes, as well as the specific characteristics of a microbial community and a ecosystem (Fierer and Jackson, 2006; Allison and Martiny, 2008; Rousk et al., 2010). It is important to acknowledge that microbial communities are highly diverse, and their responses to environmental changes are complex and context-dependent. Previous studies have yielded inconsistent results regarding whether dominant species are more sensitive to environmental changes. For examples, a field study in subtropical forest suggested the most dominant bacteria phyla were more responsive to N deposition (Dai et al., 2021). In contrary, in a maize filed, there were not obvious response difference between dominant and other microbes (Sun et al., 2019). The present study indicated that applications of organic fertilizer and biochar inhibited dominant soil cyanobacteria more than other species in paddy fields. However, the underlying mechanism remained unclear. Nevertheless, these divergent responses among cyanobacterial species should be considered in accounting for changes of the soil cyanobacterial community structure.
4.3 Soil N availability and soil pH mediated the effect of soil remediation on soil cyanobacterial community
Soil available N and pH were the key soil property influencing soil cyanobacterial abundance, species richness and community structure due to the organic fertilizer and biochar applications.
Both organic fertilizer and biochar applications significantly afftected soil physicochemical property, potentially accounting for their effects on soil cyanobacterial community. In our experimental paddy field, the predominant factor influencing soil cyanobacterial community appeared to be the N availability. In terrestrial environments, oligotrophic conditions is more favorable for cyanobacterial growth. Soil cyanobacteria was usually the pioneer species in N-poor bare soil and weathered rock surface (Belnap, 2002; Housman et al., 2006). The copiotrophic hypothesis suggests that N addition reduces the relative abundance of oligotrophic taxa as relieved N limitation allows them to be outcompeted by more copiotrophic (Ramirez et al., 2010; Ramirez et al., 2012). As a consequence, the competitive advantage of N-fixing cyanobacteria is expected to wane with N supplementation. As the ecosystem undergoes succession, the dominance of cyanobacteria was gradually replaced by other species, usually coinciding with the increase in N availability within the ecosystem (Benavent-Gonzalez et al., 2019; Cano-Diaz et al., 2022). Notably, some previous studies suggested that increasing nutrient levels due to fertilizer application, particularly N availability, inhibited soil cyanobacteria (Sun et al., 2019; Sido, 2021), aligning with our findings.
Soil pH also strongly mediated the effects of organic fertilizer and biochar on soil cyanobacteria. Soil pH was commonly an important factor influencing soil microbial community in soil improvement experiments (Zhang et al., 2017; Li et al., 2020). In the present study, both organic fertilizer and biochar additions elevated soil pH to nearly neutral levels. Typically, aquatic cyanobacteria prefers neutral to alkaline pH conditions (Metting, 1981), and thus the increase in pH due to soil improvement should significantly and positively impact the cyanobacterial community. Consistent with our hypothesis, the increased soil pH directly enhanced soil cyanobacterial diveristy. Although soil pH did not directly impacted soil cyanobacterial abundance and species richness, it strongly influenced them through affecting soil Nextra. Surprisingly, the cyanobacterial abundance and richness in the paddy soil did not benefit from the increased soil pH. Conversely, the cyanobacteria were even less abundant in the soil improvement plots. Interestingly, a previous study suggested that cyanobacteria was more abundant in acidic soil (Zhang et al., 2017), indicating the cyanobacterial preference on neutral to alkaline pH may not be universal in soil ecosystem. In the present study, our SEMs suggested that the applications of organic fertilizer and biochar increased soil pH, thus increasing soil Nextra, which, in turn, inhibited soil cyanobacterial abundance and species richness.
Other soil properties might have only minor effects on cyanobacterial community. Cyanobacteria was sensitive to increased P availability both in aquatic and terrestrial ecosystems (Correll, 1998; Conley et al., 2009; Zou et al., 2023), and sometimes blooms with P enrichment, especially in aquatic environments (Reinl et al., 2021). As a result, the increased P availability induced by the soil improvements, especially the soil fertilizer application, was expected to increase soil cyanobacterial abundance. However, soil cyanobacterial abundance decreased with the organic fertilizer and biochar applications, and was not significantly correlated to soil P availability. The decreased soil cyanobacterial abundance and species number might be also attributed to altered specie interaction resulting from the soil improvements. Consistent with many other studies, the applications of organic fertilizer and biochar led to increases in total microbial biomass, predominantly comprising heterotrophic microorganisms (Werner and Dindal, 1990; Lehmann et al., 2011; Zhang et al., 2018; Viketoft et al., 2021). These heterotrophic microorganisms may compete with cyanobacteria for mineral nutrients or produce inhibitory substances on cyanobacteria (Wang et al., 2021), inhibiting soil cyanobacterial growth. On the other hand, other phototrophic microorganisms, such as green algae, tend to proliferate extensively with increasing N availability, covering the surface of soil and water, subsequently hindering cyanobacteria from obtaining light energy (Hu and Liu, 2003). Moreover, the heightened soil nutrient levels due to soil improvements could increase rice biomass, reducing the light penetration to soil surface. This reduction in light availability may also contribute to the decrease of soil cyanobacterial abundance, particularly those with photosynthetic capabilities (Rejmankova et al., 2008; Cerna et al., 2009; Santruckova et al., 2010).
4.4 Limitations of this study
The soil sampling in the present study was carried out only once, which limits the generalizability of the results. The response of soil cyanobacteria to soil improvements may be seasonally dependent. The temperature in the research area exhibited only a small seasonal variation, with the lowest temperature in January reaching approximately 5 degrees Celsius while the highest temperature reaches around 40 degrees Celsius in either December or January. In contrast, there was a significant alternation between wet and dry seasons in terms of precipitation, which may lead to pronounced seasonal differences in the response of cyanobacterial community to soil improvements. Studies on other soil microorganisms have indeed shown this possibility (Yu et al., 2022; Zhu et al., 2023). Since our samplings were conducted during the wet season, it should be noted that the response of cyanobacteria to soil improvements during the dry season may be different. Furthermore, the response of cyanobacterial community to soil improvements may change over years as the materials produce their effects and then gradually decline (Rinnan et al., 2007; Bell et al., 2014). Our samplings were conducted 2 years after the improvements, a time considered optimal for soil improvement effects in practical productions. Therefore, the impact of soil improvement measures on cyanobacteria may lessen if it is shorter or longer than 2 years. These factors represent limitations of our study, and we urge readers to consider them when interpreting the results of this study.
Data availability statement
The original contributions presented in the study are included in the article/Supplementary Material, further inquiries can be directed to the corresponding author.
Author contributions
XZ: Writing–review and editing, Writing–original draft, Investigation. XJ: Writing–review and editing, Writing–original draft, Investigation. JG: Writing–original draft, Investigation. SH: Writing–original draft, Investigation. CC: Writing–review and editing, Project administration, Funding acquisition, Conceptualization. TZ: Writing–review and editing, Supervision, Methodology. CK: Writing–review and editing, Supervision, Conceptualization. JY: Writing–review and editing, Methodology, Formal Analysis. TL: Writing–review and editing, Methodology, Investigation. JC: Writing–review and editing, Funding acquisition. SC: Writing–review and editing, Conceptualization. SY: Writing–review and editing, Project administration, Conceptualization.
Funding
The author(s) declare that financial support was received for the research, authorship, and/or publication of this article. This research was supported by Key-Area Research and Development Program of Guangdong Province, China (2022B0111130003); National Key R&D Program of China (2021YFF0601001), and National Natural Science Foundation of China (42201057, 42177305).
Conflict of interest
Authors XZ, CC, TZ, CK, and JY were employed by Guangdong Energy Group Science and Technology Research Institute Co., Ltd. Author CK was employed by Guangdong Energy Group Co., Ltd.
The remaining authors declare that the research was conducted in the absence of any commercial or financial relationships that could be construed as a potential conflict of interest.
Publisher’s note
All claims expressed in this article are solely those of the authors and do not necessarily represent those of their affiliated organizations, or those of the publisher, the editors and the reviewers. Any product that may be evaluated in this article, or claim that may be made by its manufacturer, is not guaranteed or endorsed by the publisher.
Supplementary material
The Supplementary Material for this article can be found online at: https://www.frontiersin.org/articles/10.3389/fenvs.2024.1376147/full#supplementary-material
References
Allison, S. D., and Martiny, J. B. H. (2008). Resistance, resilience, and redundancy in microbial communities. Proc. Natl. Acad. Sci. U. S. A. 105, 11512–11519. doi:10.1073/pnas.0801925105
Bell, C. W., Tissue, D. T., Loik, M. E., Wallenstein, M. D., Acosta - Martinez, V., Erickson, R. A., et al. (2014). Soil microbial and nutrient responses to 7 years of seasonally altered precipitation in a Chihuahuan Desert grassland. Glob. Change Biol. 20, 1657–1673. doi:10.1111/gcb.12418
Belnap, J. (2002). Impacts of off road vehicles on nitrogen cycles in biological soil crusts: resistance in different US deserts. J. Arid Environ. 52, 155–165. doi:10.1006/jare.2002.0991
Benavent-Gonzalez, A., Raggio, J., Villagra, J., Manuel Blanquer, J., Pintado, A., Rozzi, R., et al. (2019). High nitrogen contribution by Gunnera magellanica and nitrogen transfer by mycorrhizas drive an extraordinarily fast primary succession in sub-Antarctic Chile. New Phytol. 223, 661–674. doi:10.1111/nph.15838
Bulluck, R., Brosius, M., Evanylo, G., and Ristaino, J. (2002). Organic and synthetic fertility amendments influence soil microbial, physical and chemical properties on organic and conventional farms. Appl. Soil Ecol. 19, 147–160. doi:10.1016/s0929-1393(01)00187-1
Cano-Diaz, C., Maestre, F. T., Wang, J., Li, J., Singh, B. K., Ochoa, V., et al. (2022). Effects of vegetation on soil cyanobacterial communities through time and space. New Phytol. 234, 435–448. doi:10.1111/nph.17996
Cao, D., Cao, W., Fang, J., and Cai, L. (2014). Nitrogen and phosphorus losses from agricultural systems in China: a meta-analysis. Mar. Pollut. Bull. 85, 727–732. doi:10.1016/j.marpolbul.2014.05.041
Cerna, B., Rejmankova, E., Snyder, J. M., and Santruckova, H. (2009). Heterotrophic nitrogen fixation in oligotrophic tropical marshes: changes after phosphorus addition. Hydrobiologia 627, 55–65. doi:10.1007/s10750-009-9715-y
Cheng, J. Z., Lee, X., Tang, Y., and Zhang, Q. H. (2019). Long-term effects of biochar amendment on rhizosphere and bulk soil microbial communities in a karst region, southwest China. Appl. Soil Ecol. 140, 126–134. doi:10.1016/j.apsoil.2019.04.017
Conley, D. J., Paerl, H. W., Howarth, R. W., Boesch, D. F., Seitzinger, S. P., Havens, K. E., et al. (2009). Controlling eutrophication: nitrogen and phosphorus. Science 323, 1014–1015. doi:10.1126/science.1167755
Correll, D. L. (1998). The role of phosphorus in the eutrophication of receiving waters: a review. J. Environ. Qual. 27, 261–266. doi:10.2134/jeq1998.00472425002700020004x
Dai, Y., Wang, H., Chen, M., Wang, D., Cao, X., Chu, B., et al. (2021). Response of soil bacterial communities to nitrogen and phosphorus additions in an age-sequence of subtropical forests. Iforest-Biogeosciences For. 14, 71–79. doi:10.3832/ifor3655-013
De, P. K. (1939). The role of blue-green algae in nitrogen fixation in rice-fields. Proc. R. Soc. Ser. B-Biological Sci. 127, 121–139. doi:10.1098/rspb.1939.0014
Dodds, W. K., Gudder, D. A., and Mollenhauer, D. (1995). The ecology of Nostoc. J. Phycol. 31, 2–18. doi:10.1111/j.0022-3646.1995.00002.x
Faostat, (2023). Statistical databases. Rome, Italy: Food and agriculture organization of the United Nations. Available at http://www.fao.org/faostat/en/ Accessed: 9 10 2023.
Fierer, N., and Jackson, R. B. (2006). The diversity and biogeography of soil bacterial communities. Proc. Natl. Acad. Sci. U. S. A. 103, 626–631. doi:10.1073/pnas.0507535103
Gaysina, L. A., Saraf, A., and Singh, P. (2019). “Chapter 1 - cyanobacteria in diverse habitats,” in Cyanobacteria. Editors A. K. Mishra, D. N. Tiwari, and A. N. Rai Cambridge, MA, USA (Academic Press), 1–28.
Hayat, R., Ali, S., Amara, U., Khalid, R., and Ahmed, I. (2010). Soil beneficial bacteria and their role in plant growth promotion: a review. Ann. Microbiol. 60, 579–598. doi:10.1007/s13213-010-0117-1
Herridge, D. F., Peoples, M. B., and Boddey, R. M. (2008). Global inputs of biological nitrogen fixation in agricultural systems. Plant Soil 311, 1–18. doi:10.1007/s11104-008-9668-3
Housman, D. C., Powers, H. H., Collins, A. D., and Belnap, J. (2006). Carbon and nitrogen fixation differ between successional stages of biological soil crusts in the Colorado Plateau and Chihuahuan Desert. J. Arid Environ. 66, 620–634. doi:10.1016/j.jaridenv.2005.11.014
Hu, C., and Liu, Y. (2003). Primary succession of algal community structure in desert soil. Acta Bot. Sin. 45, 917–924.
Jassey, V. E. J., Walcker, R., Kardol, P., Geisen, S., Heger, T., Lamentowicz, M., et al. (2022). Contribution of soil algae to the global carbon cycle. New Phytol. 234, 64–76. doi:10.1111/nph.17950
Kholssi, R., Marks, E. A. N., Montero, O., Maté, A. P., Debdoubi, A., and Rad, C. (2018). The growth of filamentous microalgae is increased on biochar solid supports. Biocatal. Agric. Biotechnol. 13, 182–185. doi:10.1016/j.bcab.2017.12.011
Lee, S.-M., and Ryu, C.-M. (2021). Algae as new kids in the beneficial plant microbiome. Front. Plant Sci. 12, 599742. doi:10.3389/fpls.2021.599742
Lehmann, J., Rillig, M. C., Thies, J., Masiello, C. A., Hockaday, W. C., and Crowley, D. (2011). Biochar effects on soil biota - a review. Soil Biol. Biochem. 43, 1812–1836. doi:10.1016/j.soilbio.2011.04.022
Li, X., Deng, S., Raun, W. R., Wang, Y., and Teng, Y. (2020). Bacterial community in soils following century-long application of organic or inorganic fertilizers under continuous winter wheat cultivation. Agronomy-Basel 10, 1497. doi:10.3390/agronomy10101497
Lima, D. L., Santos, S. M., Scherer, H. W., Schneider, R. J., Duarte, A. C., Santos, E. B., et al. (2009). Effects of organic and inorganic amendments on soil organic matter properties. Geoderma 150, 38–45. doi:10.1016/j.geoderma.2009.01.009
Liu, F., Ji, M., Xiao, L., Wang, X., Diao, Y., Dan, Y., et al. (2022). Organics composition and microbial analysis reveal the different roles of biochar and hydrochar in affecting methane oxidation from paddy soil. Sci. Total Environ. 843, 157036. doi:10.1016/j.scitotenv.2022.157036
Liu, G., Jiang, N., Zhang, L., and Liu, Z. (1996). “Soil physical and chemical analysis and description of soil profiles,” in Chineses (Beijing, China: Standards Press of China).
Liu, H. Y., Xu, W., Li, J. Y., Yu, Z. Y., Zeng, Q. C., Tan, W. F., et al. (2021). Short-term effect of manure and straw application on bacterial and fungal community compositions and abundances in an acidic paddy soil. J. Soils Sediments 21, 3057–3071. doi:10.1007/s11368-021-03005-x
Liu, X., Zhou, J., Chi, Z., Zheng, J., Li, L., Zhang, X., et al. (2019). Biochar provided limited benefits for rice yield and greenhouse gas mitigation six years following an amendment in a fertile rice paddy. CATENA 179, 20–28. doi:10.1016/j.catena.2019.03.033
Lv, M. R., Li, Z. P., Che, Y. P., Han, F. X., and Liu, M. (2011). Soil organic C, nutrients, microbial biomass, and grain yield of rice (Oryza sativa L.) after 18 years of fertilizer application to an infertile paddy soil. Biol. Fertil. Soils 47, 777–783. doi:10.1007/s00374-011-0584-y
Ma, J., Bei, Q., Wang, X., Liu, G., Cadisch, G., Lin, X., et al. (2019). Paddy system with a hybrid rice enhances cyanobacteria Nostoc and Increases N2 fixation. Pedosphere 29, 374–387. doi:10.1016/s1002-0160(19)60809-x
Maltas, A., Charles, R., Jeangros, B., and Sinaj, S. (2013). Effect of organic fertilizers and reduced-tillage on soil properties, crop nitrogen response and crop yield: results of a 12-year experiment in Changins, Switzerland. Switz. Soil Tillage Res. 126, 11–18. doi:10.1016/j.still.2012.07.012
Metting, B. (1981). The systematics and ecology of soil algae. Botanical Rev. 47, 195–312. doi:10.1007/bf02868854
Novair, S. B., Cheraghi, M., Faramarzi, F., Lajayer, B. A., Senapathi, V., Astatkie, T., et al. (2023). Reviewing the role of biochar in paddy soils: an agricultural and environmental perspective. Ecotoxicol. Environ. Saf. 263, 115228. doi:10.1016/j.ecoenv.2023.115228
O'Brien, F. J. M., Dumont, M. G., Webb, J. S., and Poppy, G. M. (2018). Rhizosphere bacterial communities differ according to fertilizer regimes and cabbage (Brassica oleracea var. capitata L.) harvest time, but not aphid herbivory. Front. Microbiol. 9, 1620. doi:10.3389/fmicb.2018.01620
Oksanen, J., Blanchet, F. G., Friendly, M., Kindt, R., Legendre, P., McGlinn, D., et al. (2018). Vegan: Community Ecology Package. R package version 2.5-6. Available at https://cran.r-project.org/package=vegan
Palansooriya, K. N., Wong, J. T. F., Hashimoto, Y., Huang, L., Rinklebe, J., Chang, S. X., et al. (2019). Response of microbial communities to biochar-amended soils: a critical review. Biochar 1, 3–22. doi:10.1007/s42773-019-00009-2
Pankratova, E. M. (2006). Functioning of cyanobacteria in soil ecosystems. Eurasian Soil Sci. 39, 118–127. doi:10.1134/s1064229306130199
Ponge, J.-F., Pérès, G., Guernion, M., Ruiz-Camacho, N., Cortet, J., Pernin, C., et al. (2013). The impact of agricultural practices on soil biota: a regional study. Soil Biol. Biochem. 67, 271–284. doi:10.1016/j.soilbio.2013.08.026
Prasanna, R., Nain, L., Pandey, A. K., and Saxena, A. K. (2012). Microbial diversity and multidimensional interactions in the rice ecosystem. Archives Agron. Soil Sci. 58, 723–744. doi:10.1080/03650340.2010.537325
Ramirez, K. S., Craine, J. M., and Fierer, N. (2012). Consistent effects of nitrogen amendments on soil microbial communities and processes across biomes. Glob. Change Biol. 18, 1918–1927. doi:10.1111/j.1365-2486.2012.02639.x
Ramirez, K. S., Lauber, C. L., Knight, R., Bradford, M. A., and Fierer, N. (2010). Consistent effects of nitrogen fertilization on soil bacterial communities in contrasting systems. Ecology 91, 3463–3470. doi:10.1890/10-0426.1
R Core Team (2020). R: a language and environment for statistical computing. Vienna, Austria: R Foundation for Statistical Computing.
Reinl, K. L., Brookes, J. D., Carey, C. C., Harris, T. D., Ibelings, B., Morales-Williams, A. M., et al. (2021). Cyanobacterial blooms in oligotrophic lakes: shifting the high-nutrient paradigm. Freshw. Biol. 66, 1846–1859. doi:10.1111/fwb.13791
Rejmankova, E., Macek, P., and Epps, K. (2008). Wetland ecosystem changes after three years of phosphorus addition. Wetlands 28, 914–927. doi:10.1672/07-150.1
Ren, H., Huang, B., Fernandez-Garcia, V., Miesel, J., Yan, L., and Lv, C. (2020a). Biochar and rhizobacteria amendments improve several soil properties and bacterial diversity. Microorganisms 8, 502. doi:10.3390/microorganisms8040502
Ren, X., Chen, F., Ma, T., and Hu, Y. (2020b). Soil quality characteristics as affected by continuous rice cultivation and changes in cropping systems in South China. Agriculture 10, 443. doi:10.3390/agriculture10100443
Rinnan, R., Michelsen, A., Baath, E., and Jonasson, S. (2007). Fifteen years of climate change manipulations alter soil microbial communities in a subarctic heath ecosystem. Glob. Change Biol. 13, 28–39. doi:10.1111/j.1365-2486.2006.01263.x
Roger, P. A., and Kulasooriya, S. A. (1980). Biue-green algae and rice. Manila, Philippines: The International Rice Research Institute, 29–30.
Roger, P. A., Tirol, A., Ardales, S., and Watanabe, I. (1986). Chemical composition of cultures and natural samples of N2-fixing blue-green algae from rice fields. Biol. Fertil. Soils 2, 131–146. doi:10.1007/bf00257592
Rousk, J., Baath, E., Brookes, P. C., Lauber, C. L., Lozupone, C., Caporaso, J. G., et al. (2010). Soil bacterial and fungal communities across a pH gradient in an arable soil. Isme J. 4, 1340–1351. doi:10.1038/ismej.2010.58
Santruckova, H., Rejmankova, E., Pivnickova, B., and Snyder, J. M. (2010). Nutrient enrichment in tropical wetlands: shifts from autotrophic to heterotrophic nitrogen fixation. Biogeochemistry 101, 295–310. doi:10.1007/s10533-010-9479-5
Sharma, A., and Chetani, R. (2017). A review on the effect of organic and chemical fertilizers on plants. Int. J. Res. Appl. Sci. Eng. Technol. 5, 677–680. doi:10.22214/IJRASET.2017.2103
Siavoshi, M., Nasiri, A., and Laware, S. L. (2011). Effect of organic fertilizer on growth and yield components in rice (Oryza sativa L.). J. Agric. Sci. 3, 217–224. doi:10.5539/jas.v3n3p217
Sido, M. Y. (2021). Consistent application of inorganic N-fertilizer caused retrogressive succession of cyanobacteria in an upland agricultural soil of China. Microbiology 90, 538–541. doi:10.1134/s0026261721040135
Sohi, S. P., Krull, E., Lopez-Capel, E., and Bol, R. (2010). “Chapter 2 - a review of biochar and its use and function in soil,” in Advances in agronomy Cambridge, MA, USA (Academic Press), 47–82.
Song, J., He, X., Wang, S., Yang, X., Wu, L., Li, S., et al. (2022). Community composition specificities of cyanobacteria in paddy soil under different ecological conditions. Agronomy-Basel 12, 3090. doi:10.3390/agronomy12123090
Song, W., Shu, A., Liu, J., Shi, W., Li, M., Zhang, W., et al. (2022). Effects of long-term fertilization with different substitution ratios of organic fertilizer on paddy soil. Pedosphere 32, 637–648. doi:10.1016/s1002-0160(21)60047-4
Sun, R., Zhang, P., Riggins, C. W., Zabaloy, M. C., Rodriguez-Zas, S., and Villami, M. B. (2019). Long-term N fertilization decreased diversity and altered the composition of soil bacterial and archaeal communities. Agronomy-Basel 9, 574. doi:10.3390/agronomy9100574
Viketoft, M., Riggi, L. G. A., Bommarco, R., Hallin, S., and Taylor, A. R. (2021). Type of organic fertilizer rather than organic amendment per se increases abundance of soil biota. Peerj 9, e11204. doi:10.7717/peerj.11204
Wang, Z., Akbar, S., Sun, Y., Gu, L., Zhang, L., Lyu, K., et al. (2021). Cyanobacterial dominance and succession: factors, mechanisms, predictions, and managements. J. Environ. Manag. 297, 113281. doi:10.1016/j.jenvman.2021.113281
Werner, M. R., and Dindal, D. L. (1990). Effects of conversion to organic agricultural practices on soil biota. Am. J. Altern. Agric. 5, 24–32. doi:10.1017/s0889189300003192
Whitton, B. A., and Potts, M. (2012). Introduction to the cyanobacteria. Dordrecht, Netherland: Springer Netherlands.
Wu, J., Sha, C., Wang, M., Ye, C., Li, P., and Huang, S. (2021). Effect of organic fertilizer on soil bacteria in maize fields. Land 10, 328. doi:10.3390/land10030328
Yang, Y., Wang, P., and Zeng, Z. (2019). Dynamics of bacterial communities in a 30-year fertilized paddy field under different organic-inorganic fertilization strategies. Agronomy-Basel 9, 14. doi:10.3390/agronomy9010014
Yu, S., Sayer, E. J., Li, Z., Mo, Q., Wang, M., Li, Y., et al. (2022). Delayed wet season increases soil net N mineralization in a seasonally dry tropical forest. Sci. Total Environ. 823, 153314. doi:10.1016/j.scitotenv.2022.153314
Zhang, K., Cheng, X., Shu, X., Liu, Y., and Zhang, Q. (2018). Linking soil bacterial and fungal communities to vegetation succession following agricultural abandonment. Plant Soil 431, 19–36. doi:10.1007/s11104-018-3743-1
Zhang, Y., Shen, H., He, X., Thomas, B. W., Lupwayi, N. Z., Hao, X., et al. (2017). Fertilization shapes bacterial community structure by alteration of soil pH. Front. Microbiol. 8, 1325. doi:10.3389/fmicb.2017.01325
Zhao, X., Wang, J., Wang, S., and Xing, G. (2014). Successive straw biochar application as a strategy to sequester carbon and improve fertility: a pot experiment with two rice/wheat rotations in paddy soil. Plant Soil 378, 279–294. doi:10.1007/s11104-014-2025-9
Zhu, Y., Yu, K., Wu, Q., Cheng, X., Li, Z., Wang, Z., et al. (2023). Seasonal precipitation and soil microbial community influence plant growth response to warming and N addition in a desert steppe. Plant Soil 482, 245–259. doi:10.1007/s11104-022-05684-y
Keywords: blue algae, soil improvement, rice field, soil biodiversity, soil bacteria
Citation: Zou X, Jiang X, Guan J, Huang S, Chen C, Zhou T, Kuang C, Ye J, Liu T, Cheng J, Chen S and Yu S (2024) Both organic fertilizer and biochar applications enhanced soil nutrition but inhibited cyanobacterial community in paddy soils. Front. Environ. Sci. 12:1376147. doi: 10.3389/fenvs.2024.1376147
Received: 25 January 2024; Accepted: 11 March 2024;
Published: 18 April 2024.
Edited by:
Dima Chen, Inner Mongolia University, ChinaReviewed by:
Yuhui Meng, China Three Gorges University, ChinaShengjie Liu, Chinese Academy of Sciences (CAS), China
Copyright © 2024 Zou, Jiang, Guan, Huang, Chen, Zhou, Kuang, Ye, Liu, Cheng, Chen and Yu. This is an open-access article distributed under the terms of the Creative Commons Attribution License (CC BY). The use, distribution or reproduction in other forums is permitted, provided the original author(s) and the copyright owner(s) are credited and that the original publication in this journal is cited, in accordance with accepted academic practice. No use, distribution or reproduction is permitted which does not comply with these terms.
*Correspondence: Shiqin Yu, sqyu@soil.gd.cn
†These authors have contributed equally to this work