- Department of Water, Atmosphere and Environment, Institute of Hydrobiology and Aquatic Ecosystem Management, University of Natural Resources and Life Sciences, Vienna, Austria
Tributaries play a vital role in fish spawning and recruitment, significantly influencing mainstem river fish populations. However, in the Anthropocene era, tributaries within river networks suffered ecological degradation due to fragmentation and channelization. This has led to reduced distribution ranges and declining populations of various riverine species, underscoring the urgency of conservation and rehabilitation efforts. Our study explores the potential for reintroducing fish from the mainstem Danube River into the Wien River, an urban tributary that has undergone partial rehabilitation. We assessed habitat use and movement patterns of 20 adult barbel (Barbus barbus), a species classified as ‘near threatened’ in Austria, collected from the Danube River during the spawning season. These barbel were tagged with radio telemetry tags and relocated into the upper reaches of the Wien River, surmounting several artificial barriers in the lower sections. Although spawning activity was not observed among the barbel, possibly due to prevailing water temperatures, our data suggest that the Wien River could function as a viable temporary habitat. Barbel were notably inclined to inhabit deeper pool and run habitats. Their daily movements and home ranges were relatively limited, ranging from 0.0 to 1.1 km and 100 m to 2.9 km, respectively. One contributing factor to restricted movement was the presence of beaver and knotweed dams, which created temporary migration obstacles. On average, the barbel remained within the system for more than a month. Most of these fish migrated downstream at the onset of a significant high-flow event. Our assessment suggests that while the Wien River may not serve as a permanent habitat, it could function as a temporary habitat for migratory fish. However, to ensure accurate assessments of the restored Wien River as a spawning habitat, it is imperative to reevaluate the findings under stable spawning conditions and gather comprehensive data on relevant abiotic factors. This study advocates for the restoration of longitudinal connectivity between tributaries and mainstem rivers as a means to counteract biodiversity loss in Anthropocene river ecosystems.
1 Introduction
Despite their relatively small size, tributaries can exert a disproportionately large influence on mainstem fish populations by providing critical habitats for spawning and recruitment, which are vital for the sustainability of the overall fish population (Schmutz and Jungwirth, 1999; Hitt and Angermeier, 2008). Previously widespread cyprinid species like the common barbel (Barbus barbus), nase (Chondrostoma nasus), or vimba bream (Vimba vimba) are known to migrate long distances upstream, even into tributaries, to spawn (Schiemer and Waidbacher, 1992; Panchan et al., 2022). After spawning, tributaries can serve as key nursery habitats for juvenile fish (Pollux et al., 2006) before they drift downstream into the mainstem (Pavlov and Mikheev, 2017). During the Anthropocene era, however, the ecological functioning of such river networks has often been limited due to diverse human stressors (Gosset et al., 2006; Dudgeon, 2019). River fragmentation, for example, can block the movements of aquatic biota, inhibiting fish from reaching their spawning grounds (Gosset et al., 2006; Mattocks et al., 2017). Channelization can degrade habitats needed for reproduction and recruitment (Brooker, 1985; Jurajda, 1995). Consequently, these anthropogenic disturbances have led to drastic declines in the distribution and population viability of some fish species (Hayes et al., 2022b).
River restoration by means of hydro-morphological improvements, fish passage installment, and weir removal can revitalize fluvial ecology (Kail et al., 2015) and increase ecosystem services provision (Kaiser et al., 2020). In the last decades, the rehabilitation of rivers has been gaining momentum, in Europe, largely motivated by the requirements of the EU Water Framework Directive (2000/60/EC). In large rivers, contrasting objectives of various stakeholders, such as flood protection, energy production, and nature conservation can stall management decisions. Notably, the rehabilitation of tributaries can constitute opportunities to enhance fish populations in the mainstem by providing a connected system (Bouska et al., 2023). Moreover, restoration efforts predominantly target urban systems due to their larger community benefits despite the higher associated costs (Findlay and Taylor, 2006; Guimarães et al., 2021; Hayes et al., 2022a).
The Wien River, a tributary to the Austrian Danube River, is an example of such a partly rehabilitated urban stream. Since the 1990s, the Wien River’s upper reaches boast freshwater habitats of high ecological value due to the creation of a flood protection zone with a 37 ha large wetland with self-forming river dynamics. Since 2014, an additional 1.8 km of near-natural riverbed, featuring sediment dynamics and diverse mesohabitats, has been re-established (Hohensinner, 2019). Despite these areas with corresponding fish ecological importance, many species belonging to the Danube River fish fauna, including the barbel, are absent (Keckeis et al., 2000; Holzer, 2014). According to the national reference standard (Leitbild), these species should, however, inhabit this section at least occasionally (BAW, 2007). The heavily channelized and partially culverted lower section, including several migration obstacles in the form of small weirs and riverbed sills in the Wien River near the mouth of the Danube River, are the reasons why typical Danube fish species cannot be found in the Wien River today. However, the climate-induced shift in fish regions would favor their spread (Melcher et al., 2013). These migration barriers are also the reason why the Wien River is at risk of failing European environmental objectives (Köck et al., 2008). If Danube fish species such as the barbel could overcome these obstacles and traverse the channelized section of the lower Wien River, this red-listed species could potentially re-colonize suitable areas, including habitats for spawning and juvenile rearing (Melcher and Schmutz, 2010; Britton and Pegg, 2011; Gruber et al., 2022). Considering the barbel’s preference for spawning in tributaries, restoring river network connectivity is likely to impact the resilience of mainstem populations positively (Nislow et al., 2011; Sun et al., 2022). Re-establishing the longitudinal connectivity can, therefore, expand the tributaries’ ability to contribute to the growth and expansion of mainstem fish populations, thereby reducing the harmful effects of river regulation (Sun et al., 2022).
In some cases, such as the Wien River, still-existing barriers prevent upstream fish migration to restored tributary stretches, effectively hindering recolonization efforts. In such circumstances, the translocation of adult fish can be a valuable method to support the natural dispersal of populations (Newhard et al., 2021). Indeed, a study on nase demonstrated that the relocation of adult fish over non-passable barriers can lead to successful spawning in reaches that feature suitable habitat (Ovidio et al., 2016). Also, the Wien River’s contribution to the rearing of 0+ nase, a rheophilic cyprinid with habitat preferences similar to the barbel, has been demonstrated through a stocking study in the rehabilitated flood protection basin, showing growth rates and abundance similar to other near-natural areas (Bartl and Keckeis, 2004). However, the evaluation of the success rate of such translocation experiments remains limited. Understanding the short-term behavioral responses of translocated fish in their new habitats is crucial for assessing the method’s feasibility on a larger scale (Ovidio et al., 2016). Unfortunately, this preliminary behavioral analysis has been largely neglected, leaving a significant knowledge gap regarding immediate translocation success mechanisms.
This study aims to evaluate the possibility of reintroducing barbel from the mainstem river to the upstream reaches of a restored urban tributary to encourage spawning (Ovidio et al., 2016) as well as to assess the potential of the Wien River as a summer residence habitat. To this aim, we tracked movement patterns and monitored habitat preferences of adult barbel translocated from the Danube River to the Wien River during the spawning season using radio telemetry and habitat mapping. The results of this study contribute to the management of threatened species by restoring fragmented, urban river-tributary networks.
2 Materials and methods
2.1 Target species
The common barbel is an example of a migratory species that has been significantly affected by hydro-morphological degradation (Britton and Pegg, 2011; Hayes et al., 2022b). Therefore, the barbel is classified as ‘near threatened’ in Austria (Wolfram and Mikschi, 2007) and is locally protected, for example, in the Federal State of Vienna according to the Vienna Nature Conservation Act (45/1998). At the European level, the barbel is included in Annex V of the Habitats Directive (92/43/EEC).
Adult barbel are primarily found in larger rivers, with water depths ranging from 2 to 6 m and flow velocities between 1.0 and 1.5 m/s (Banarescu and Bogutskaya, 2003; Vilizzi and Copp, 2005; Britton and Pegg, 2011; Panchan et al., 2022; Mawer et al., 2023). During the spawning season from May to July, when water temperatures exceed 10°C, barbel move upstream, often into tributaries, to spawn over gravel in fast-flowing areas (Ovidio et al., 2007; Melcher and Schmutz, 2010; Pelz, 2022). Flood events often prompt the upstream migration to suitable spawning sites. During the active spawning phase, at water temperatures of 14°C–16°C (Melcher and Schmutz, 2010; Meulenbroek et al., 2018), females occupy spawning sites, followed by the male barbel. Spawning sites are often used multiple times for spawning. During spawning pauses, the fish gather in deeper areas or under structures (Pelz, 2022). Spawning activity ceases when water temperatures exceed 16°C (Kottelat and Freyhof, 2007; Melcher and Schmutz, 2010; Pelz, 2022).
Young-of-the-year fish are known to drift downstream from the spawning grounds (Copp et al., 2002; Zitek et al., 2004) to overwintering habitats, predominantly found in the mainstem rivers (Banarescu and Bogutskaya, 2003; Britton and Pegg, 2011; Panchan et al., 2022). Given the ecology and habitat requirements of the barbel, conservation and restoration of large rivers and their tributaries (Pracheil et al., 2013) could improve the population status of this species, as well as others, as the barbel is a suitable indicator species for a river’s hydro-morphological state (Britton and Pegg, 2011).
2.2 Study area
The Wien River is the biggest tributary to the Danube River in the city of Vienna, Austria (Figure 1A). It originates in the western Wienerwald (Vienna Woods) at 520 m above sea level, flowing for 34 km to the Danube River confluence. With a watershed area of 230 km2, the Wien River basin extends from protected woodlands west of Vienna to urban areas. The catchment’s flysch geology leads to a highly variable and flashy flow regime. The mean discharge of the Wien River is around 2 m³/s. However, during short periods, such as after intense rainfall events, the discharge can be > 2,000 times higher compared to the regular flow conditions (Stadt Wien, 2023).
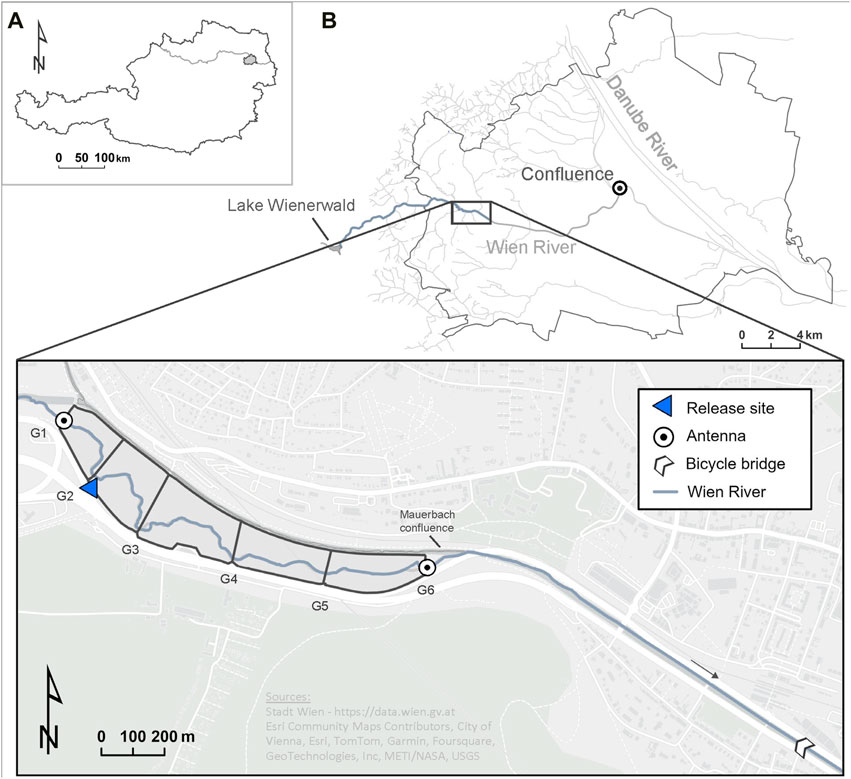
Figure 1. Location of the (A) city of Vienna within Austria, (B) the Wien River, and (C) the core study area between the bicycle bridge at Hütteldorf and gate one of the flood retention basins. The blue triangle marks the site where tagged fish were released. G1-G6 indicate the retention basins’ gate location.
The 12.6 km long river stretch relevant for this study extends from the bicycle bridge near Hütteldorf (48°11′44.9″N, 16°15′43.1″E) to the artificially constructed Vienna Woods Lake (Wienerwaldsee) (48°10′59.9″N, 16°07′24.0″E) (Figure 1B), and features a diverse array of mesohabitats. Downstream from Hütteldorf, the Wien River presents unsuitable fish habitat as the riverbed is heavily channelized.
Based on telemetry data, we designated a core study area where mesohabitat mapping was performed (Figures 1B, C). This 3.0 km long core study area stretches from the Hütteldorf bridge to the first gate of the Auhof flood retention basins (48°20′40.3″N, 16°23′26.3″E) as none of our study organisms was detected upstream of this point. The flood retention basin consists of six gates, referred to as gates 1-6 hereafter, which are only closed for flood protection (but this did not happen during our study); each gate has a width between 5 and 13 m. In between these gates, the Wien River has sufficient space for dynamic processes such as bank erosion and processes of in-river erosion and sediment transport. The substrate composition in the study area, as outlined by Gruber et al. (2022), consists mainly of fine and medium-sized gravel (2–63 mm), with cobbles (63–200 mm) and blocks (200–400 mm) also present (Schopf, 2009).
Throughout the study period, several beaver dams appeared intermittently between gates 3 and 5, posing a potential obstacle to movement. After passing through gate six of the retention basin, the Wien River is joined by the Mauerbach, a small creek also originating in the Wienerwald. Downstream of the basins, several restoration measures, including the removal of artificial riverbed and bank structures accompanied by bioengineering measures, were implemented along a 1.8 km long river stretch (Hohensinner, 2019), providing potentially suitable barbel habitats (Schopf, 2009; Gruber et al., 2022).
2.3 Telemetry
Radio telemetry is an internationally practiced standard method for investigating fish migratory behavior and habitat preferences (Huber and Kirchhofer, 1998; Boavida et al., 2015; Ovidio et al., 2016; Panchan et al., 2022). According to our study aims, barbel from the Danube River were captured during the spawning season in 2021, equipped with transmitters, and released into the Wien River.
We conducted electrofishing by boat to catch the target species at the Wien River mouth and nearby Danube River stretches. The barbel spawns between May and July at water temperatures of 14°C–16°C (Melcher and Schmutz, 2010; Meulenbroek et al., 2018). In 2021, low Danube River water temperatures delayed fish collections until June. We did not catch enough specimens at the first fishing date (8 June 2021); therefore, we conducted another campaign the following week (14 June 2021). The water temperature during the collection in the Danube was 17.7°C and 17.6°C on 8 and 14 June, respectively (Figure 2). Barbel did not initiate spawning movements or indicate typical spawning behavior before these dates.
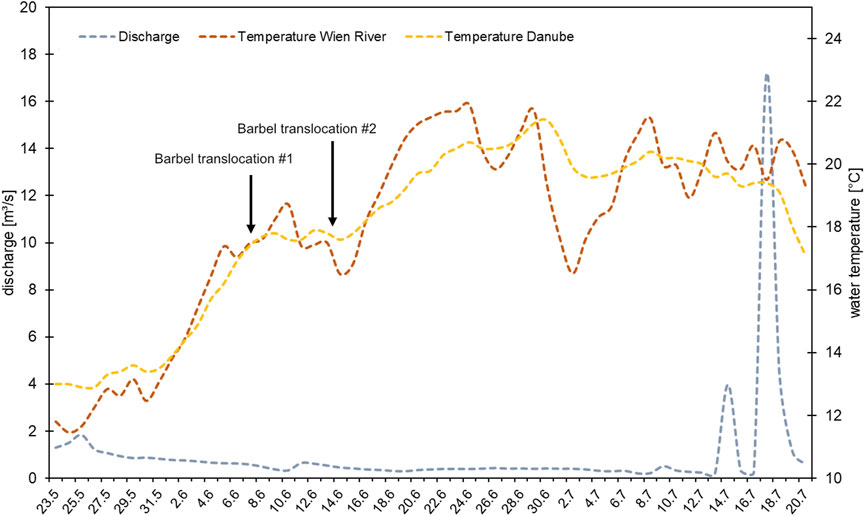
Figure 2. Water temperature in the Wien River and the Danube River, and discharge in the Wien River, before and during the study period. Dates of fish collection and relocation are marked with arrows.
Each barbel was measured to total length [mm] and weighed [g]. We only considered individuals for tagging if their body weight exceeded 300 g, as the radio telemetry tag should not weigh more than 2% of the fish’s body weight so as not to affect the swimming ability (Jepsen et al., 2002). Smaller specimens were released back into the Danube River. The maturity of the female barbel was assessed by gently palpating the abdomen. In mature females, the abdomen is bulging and very soft. For males, the presence of sperm emission from the vent was checked by applying the stripping method. In total, the sex of five females and two males could be identified, as the other fish showed no clear sign of ripeness (Table 1).
Tag implementation was done on the same day the fish were caught, in accordance with the Austrian animal welfare law (BGBI. I Nr. 114/2012). Barbel were anesthetized using clove oil. Following, a Lotek radio telemetry transmitter (1.5 g in air, 15 mm long and 8.2 mm wide) was inserted into the abdominal cavity through a 1 cm incision, with the antenna extending laterally outside. The surgical incision was closed via sutures. These Nano Tags of the NTF-5-2 type operate between 147 and 168 MHz with a burst interval set at 5 s. The tags were expected to last for 203 days. The antenna was led out laterally to the wound, while the opening was then sewn up with surgical thread. The fish were then placed into an oxygen-enriched tank to recover from the surgery. Following an approximately 3-h recovery period, the fish were driven to the Wien River in an oxygen-enriched water tank built for fish transportation. Barbel equipped with tags were released at the study site at the second gate (G2 in Figure 1C) of the retention basin (48°12′14.35″N, 16°13′57.53″E). Fifteen individuals (B01-B13, B21-B22) were released on the first day, and an additional seven fish (B14-B20) were released on the second day (Table 1). On 8 June, when the first fifteen barbel were released, the Wien River’s water temperature measured 17.6°C. On 14 June, the second release day, the water temperature was 16.5°C (Figure 2).
Following their translocation on 8 June and 14 June 2021, the tagged barbel were monitored with one mobile and three stationary antennas. Fixed antennas of the type Yagi were positioned at gate 1 (48°12′20.55″N, 16°13′52.48″E) and gate 6 (48°12′4.23″N, 16°14′47.48″E) of the retention basins, and the confluence of the Wien River with the Danube Canal (48°12′41.83″N, 16°23′3.37″E), a side arm of the Danube River, in order to observe movements beyond the retention basins and back into the Danube River (see Figures 1B, C). Mobile tracking using a hand-held antenna (Lotek SRX 800) was done daily between 7:00 and 8:00 a.m. for two to 3 hours to record the position of the tagged fish. Each individual was located and positioned with a GPS (positioning accuracy: 1–2 m in the longitudinal). Furthermore, verification of spawning activity was conducted through daily visual observations to ascertain the aggregation of barbel in potential spawning habitats (Pelz, 2022). Water temperature data measured at gate one of the retention basin was available for the entire study period. The telemetry monitoring continued until all tagged barbel had exited the study area by migrating downstream to the Danube River. The exit from the study area was determined by identifying the final day of detection within the study area before the individual’s detection at the confluence.
Telemetry data from the release day and the detections of two fish presumed deceased after release were excluded from the following analyses, as no tag movement or fish could be observed.
2.4 Habitat assessment
To determine the target species’ preferred habitat types, we performed a mesohabitat survey of the core study area (see Section 2.1). We mapped the following mesohabitat types based on expert judgment in the field, following the description of Fisher et al. (2012): (i) riffle, (ii) run, and (iii) pool.
For each mesohabitat unit, we recorded the depth, flow velocity, and vegetation overhang. The depth was assessed in multiple transects per mesohabitat using a measuring stick. The number of cross-sections and respective measurements varied between 2 and 8, contingent upon its size. We used the mean maximum depth measurements to describe water depth in the mesohabitats. Flow velocity was assigned to one of three classes – (1) stagnant, (2) slow, and (3) fast – according to expert judgment. Vegetation overhang was recorded as a binary variable (yes/no) per mesohabitat unit.
To assess barbel habitat use in the Wien River, we combined fish locations with mesohabitat mapping. We visualized concentration hotspots within the study area using a Kernel Density analysis in ArcGIS Pro with a 4 × 4 m raster size and a search radius of 100 m (Esri Inc, 2023). Additionally, we calculated a Habitat Use Index for each mesohabitat type (Ih) to assess habitat use on a scale from 0 to 1. This was done by calculating the number of detections relative to the total area of the respective mesohabitats (Eq. 1):
Where nh = the sum of detections in mesohabitat type h divided by the total area of the respective mesohabitat type; h = pool (P), riffle (RF), or run (RN).
3 Results
3.1 Mesohabitats
We mapped 2.08 ha of aquatic mesohabitats from gate one of the flood retention basins to the downstream end of the core study area (Figure 3). Riffles constitute the dominant habitat type, covering 55% of the mapped area. Pool habitats comprise 33% of the mapped area, while runs account for 12% percent (Table 2).
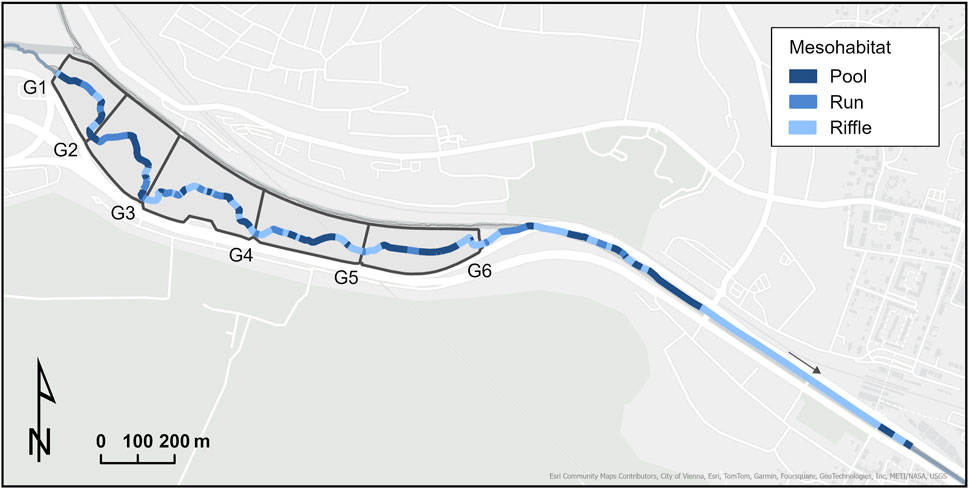
Figure 3. Distribution of mesohabitats in the core study area (see Figure 1). G1-G6 indicate the retention basins’ gate locations.

Table 2. Mesohabitat characteristics within the core study area of the Wien River (see Figures 1, 3).
Pool habitats reach a mean maximum depth of 0.27–1.05 m (mean = 0.55 m ± 0.15 SD). Riffles and runs exhibit a mean maximum depth of 0.10–0.40 m (mean = 0.24 m ± 0.60 SD) and 0.24–0.61 m (mean = 0.40 m ± 0.11 SD), respectively (Table 2).
Regarding vegetation overhang, pool habitats have the highest share, with 50% of all mesohabitat units shaded by overhanging vegetation. This is followed by run and riffle habitats with 44% and 24%, respectively (Table 2).
The velocity was variable across individual mesohabitat types (Table 2). Flow velocities in pool mesohabitats ranged from stagnant (36.1%) to slow (63.9%). Run habitats consisted of fast (16.7%), slow (72.2%), and stagnant (11.1%) flow velocities. Riffle habitats were mainly comprised of fast (67.6%) and slow (29.7%) flow velocities.
3.2 Fish mesohabitat use
The 22 tagged barbel weighed 300–1,710 g (mean: 704.1 g, ±427.0 SD), with a total length ranging from 278 to 805 mm (mean: 460 mm ± 135.4 SD) (Table 1). All fish that were translocated from the Danube River to the Wien River could be detected in the study area. Two individuals (B21 and B22) were excluded from further analysis due to indications in the telemetry data suggesting their demise.
Of the remaining 20 fish, a total of 573 detections were recorded. The daily count of detected barbel ranged from seven to 18 individuals, with a mean of 16.2 ± 4.2 SD fish detected per day (Figure 4). The tagged fish were detected on 4–37 days (mean: 29 days ± 11.2 SD). The mean detection rate per specimen, considering the days detected in relation to the maximum number of days fish spent in the study area, was 98.5% ± 1.8 SD. The highest mean detection rate per fish was 100% (for fish B02, B05-B10, B12, B16-B18), while the lowest was recorded at 93.8% (for fish B20).
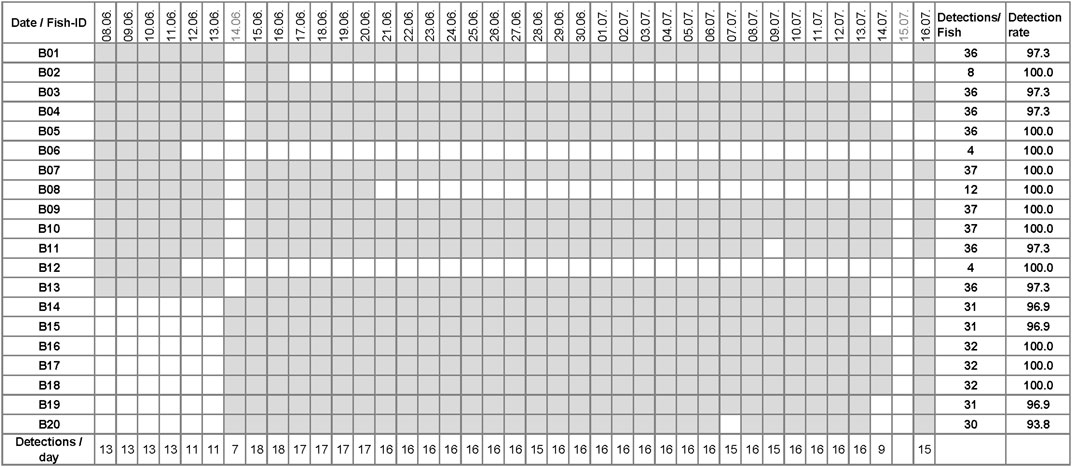
Figure 4. Daily detections of barbel in the Wien River core study area. Grey boxes indicate at least one detection per day; white boxes indicate no detection (excluding two dead fish: B21 and B22). No tracking was conducted on 14 June (the second release date) and on 15 July.
The mean residence time of barbel in the study area, including days when a fish was not detected, was 31.3 days ± 11.6 SD, with a minimum of four and a maximum of 39 days spent in the Wien River before migrating downstream into the Danube River (Figure 4).
Regarding preferred mesohabitats, barbel occupied pools 81.9% of the time, according to detected signals. In contrast, run and riffle habitats exhibited a lower use of 9.6% and 8.5% (Table 3). This preference for pool habitats was also displayed by a habitat use index of 0.72. With regards to the other two mesohabitat types, barbel prefer run over riffle habitats (Table 3).
Barbel were found in habitats with a mean maximum depth ranging from 0.15 to 1.10 m (mean: 0.60 m ± 0.18 SD). These habitats were characterized by a slow flow velocity. Regarding vegetation overhang, no preference could be detected, as 52% of all detections occurred in habitats with overhang, while 48% were in habitats without overhang.
The highest density of detected fish was located near gate 2 and in the upstream and downstream area of gate 6, including the confluence with the Mauerbach River downstream of gate 6 (Figure 5).
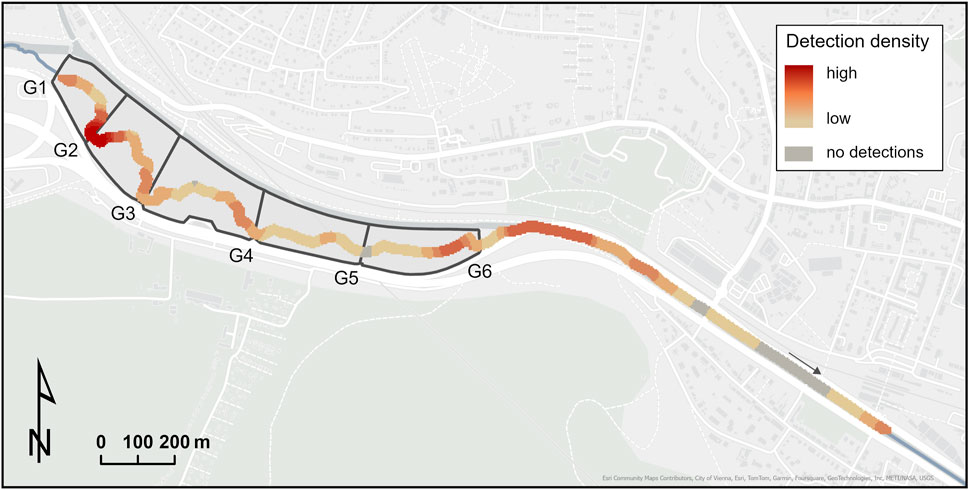
Figure 5. Fish detection density in the core study area (see Figure 1). G1-G6 indicate the retention basins’ gate locations.
3.3 Fish movements
Tagged barbel exhibited differences in daily movements (Figure 6A). The mean daily movement distance was 0.11 km ± 0.07 SD, with daily variations ranging from 0.0 to 1.1 km.
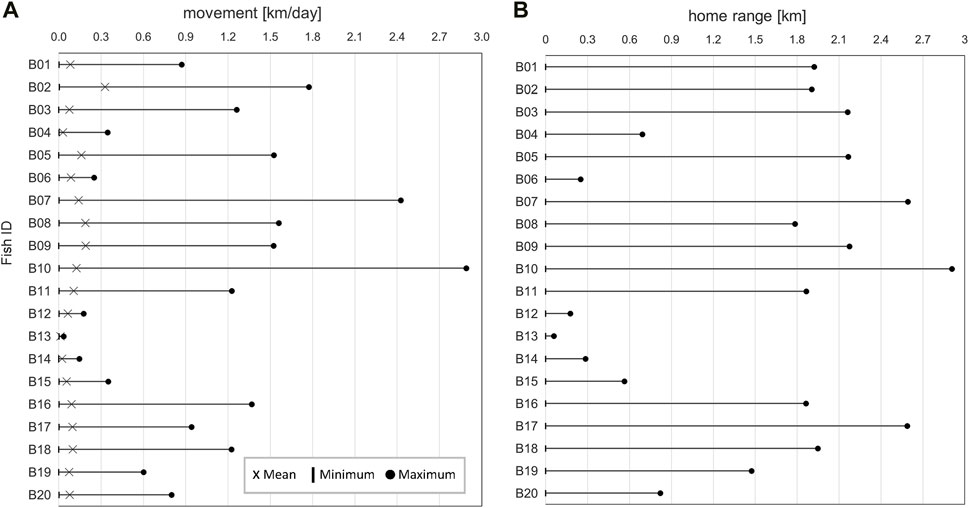
Figure 6. (A) Daily movement distances and (B) home range of the tagged barbel in the core study area (see Figure 1).
The home range of tagged fish along the river corridor varied between 0.1 km and 2.9 km, with a mean of 1.5 km ± 0.9 SD. Fish B13 remained at the release spot for the entire time it was in the study area. Fish B10 was the most active, occupying a stretch of 2.91 km (Figure 6B).
Movement patterns of tagged barbel were variable (Figure 7), showing single (e.g., B16) and stepwise downstream movements (e.g., B03). Larger upstream migrations, as demonstrated by B09 and B10, were relatively infrequent. Certain individuals, such as B13-B14, underwent only minimal downstream and upstream movement before departing the river system in a coherent, single migration event.
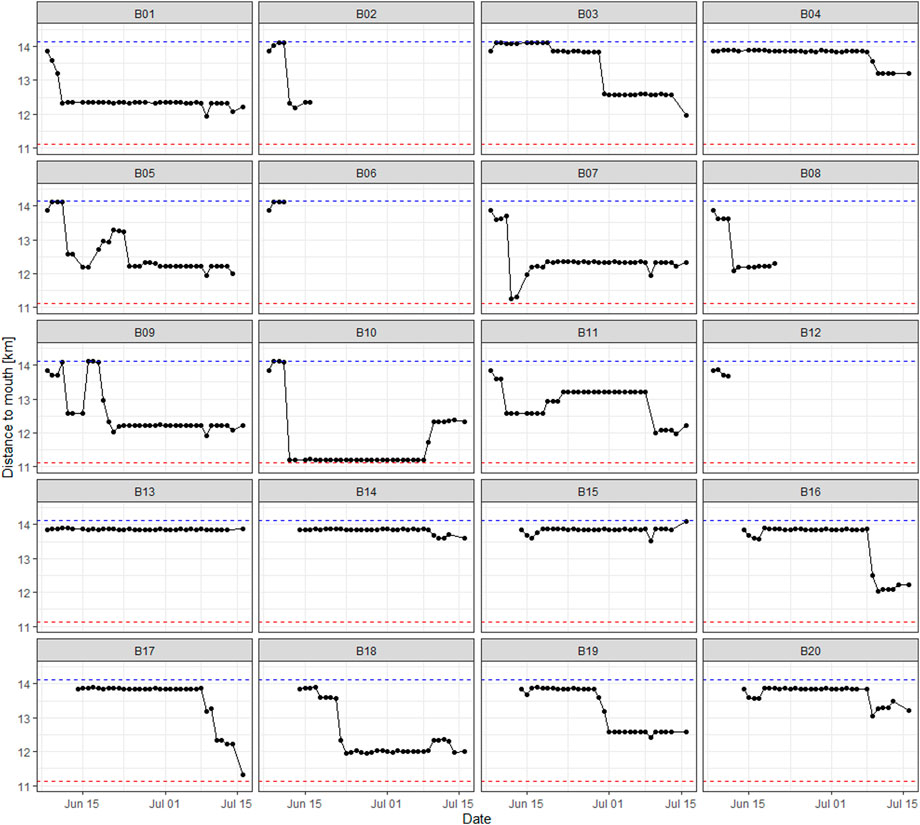
Figure 7. Fish movements in the core study area (see Figure 1). The x-axis corresponds to the date of detection, while the y-axis represents the river kilometers.
In summary, 72.4% of all daily movements occurred in a downstream direction, whereas 27.6% occurred in an upstream direction.
The tagged fish migrated out of the study area 4–39 days after translocation (Figure 4; Figure 8). Eighteen of the 20 tagged fish were detected at the permanent station located at the river mouth 11.1 km downstream from the core study area. This antenna detected the first returning fish (B06) on 16 June and the last fish (B20) on 20 July. An overview of the first detection of barbel at the confluence is depicted in Figure 8. The data suggests a mean migration time from the study area to the Danube confluence of 1.7 days ± 1.1 SD, with a minimum of one and a maximum of 5 days.
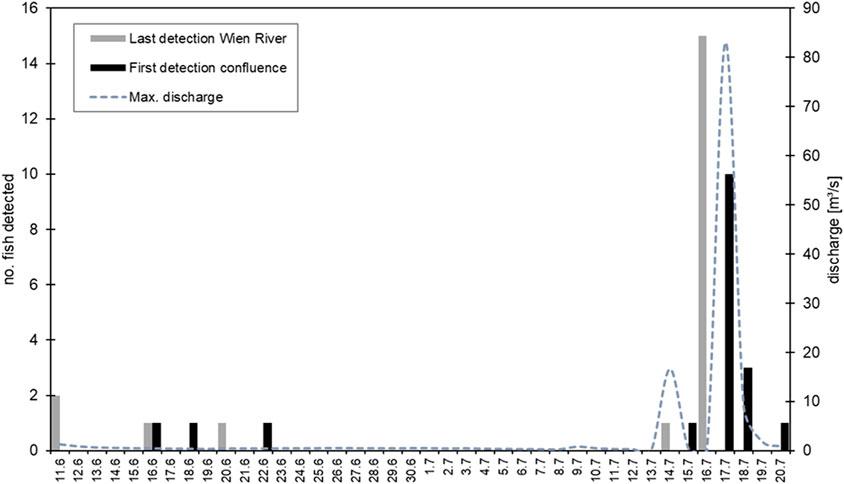
Figure 8. The last detection of each fish in the core study area (grey bar) and the first detection at the Danube confluence (black bar), including the maximum daily discharge of the Wien River (dotted line).
In the early morning of 14 July, a high flow event occurred, reaching a peak discharge of 16.6 m³/s. One fish (B05) left the study area on this day (Figures 7, 8). Three days later, on 16–17 July, a flood with a maximum discharge of 83.0 m³/s was recorded; none of the 15 remaining fish were detected in the study area anymore during the daily tracking routing in the morning of 17 July. Eleven fish were first detected at the confluence within 24 h, on 17–18 July (Figure 8). The average time difference of the initial detection at the confluence station among these eleven individuals was 1.2 h (±0.9 SD), displaying a close temporal proximity in the downstream migration.
4 Discussion
This study aimed to assess the feasibility of reintroducing a wild fish species from the mainstem to the upstream reaches of a restored urban tributary to encourage spawning. Additionally, it sought to evaluate the Wien River’s potential as a summer residence habitat for the barbel. A habitat modeling study indicated the presence of potentially suitable spawning and juvenile habitats in the Wien River (Gruber et al., 2022). Therefore, we hypothesized that barbel translocated to the Wien River during the spawning season would utilize the tributary for spawning. To test this hypothesis, we assessed habitat use and movement patterns of 20 adult barbel caught in the Danube River during the spawning season and relocated into the upper reaches of the Wien River.
The tagged barbel remained in the Wien River for about a month, with a maximum stay of 39 days. The question of why the fish were not detected further upstream from gate one of the retention basin still needs to be answered, as to the expert judgement, sediment and water depth were suitable and no barriers to migration were present. It is noticeable that 75% of tagged fish emigrated in a single day. This collective downstream movement must be related to a flood pulse with water levels rising in the early morning of 17 July (Figure 8). At the time of tracking (ca. 07:00 a.m.), all remaining fish had apparently already left the core study area. Therefore, we hypothesize that the onset of the flood event facilitated the outmigration of all 15 fish remaining in the study area at that time, as barbel prefer moving with the flow (Mawer et al., 2023). Eleven of these fish that departed the system with the flood pulse on 17 July displayed a remarkably swift outmigration, likely reaching the Danube River in less than 24 h. This contrasts with the mean migration time of 2.6 days observed among fish that left the system before this flood pulse. Considering that the initial flood pulse on 17 July occurred before the daily mobile tracking, it is likely that certain fish migrated downstream in less than 24 h. Pelz (2022) also observed similar downstream migration behavior of barbel, noting that the fish left the spawning sites during high flood events to migrate into the mainstem river.
Our results suggest that the rather small Wien River is likely unsuitable as a permanent habitat. These findings are supported by literature which states that barbel’s permanent habitat is mostly found in the mainstem and larger tributaries with high flow velocities and greater water depths (Banarescu and Bogutskaya, 2003; Vilizzi and Copp, 2005; Britton and Pegg, 2011; Panchan et al., 2022; Mawer et al., 2023). Nonetheless, the results indicate that potential spawning habitats exist in the Wien River, particularly in runs. The hydraulic characteristics of the mesohabitat type run indicate an alignment with the barbel’s spawning habitat preferences observed in other rivers (Melcher and Schmutz, 2010; Panchan et al., 2022; Pelz, 2022). A study at the Traisen River, a Danube River tributary upstream of the city of Vienna, found that barbel spawned in areas with flow velocities between 0.4 and 1.0 m/s in water depths of 0.3–0.5 m (Pelz, 2022). A similar preference regarding flow velocity (0.65 m/s ± 0.18 SD) and water depth (0.37 m ± 0.16 SD) was found in the Pielach River, another Danube tributary (Melcher and Schmutz, 2010). The run mesohabitats, with a mean maximum depth of 0.40 m ± 0.11 SD and moderate flow velocity, representing around one-tenth of the total core study area (Table 2), fit those observations fairly well. However, these findings need validation through fieldwork involving the assessment of quantitative data at a high resolution, particularly regarding bottom flow velocity and substrate composition. Additionally, the hypothesis is supported by the findings of Gruber et al. (2022), who evaluated the ecological benefits of the Wien River through hydro-dynamic habitat modeling to assess the habitat suitability of different barbel life stages for typical flow magnitudes. The results of this modeling study showed that, at a discharge of 1.5 m³/s, around 15%–30% of the study site area offers valuable temporary habitats for key life stages of barbel, including spawning and juvenile rearing.
In our study, barbel occupied pool habitats most frequently, followed by runs. The observed home range of the barbel was comparatively smaller than in other studies. For instance, Ovidio et al. (2007) reported a maximum home range of 12.8 km. In our study, conducted in a study area approximately half the length of the one from Ovidio et al. (2007), the maximum observed home range was 2.9 km. The mean daily movement of barbel in the Wien River ranged from zero to 1 km, with a maximum recorded movement of around 3 km per day. Similarly, the tagged fish’ home range varied, ranging from 0.1 km to 2.9 km. Overall, the daily movement and home range variability and extent are less pronounced than described in other studies (Lucas and Batley, 1996), for example, with home ranges of 18–47 km in a 58 km long river system (Panchan et al., 2022) or 340 m to around 13 km in a 27 km long river stretch (Ovidio et al., 2007). The reason for the relatively small home range and daily movement distances could be the short observation period, the preference for certain habitats, or the presence of multiple beaver dams, regularly changing locations between gates 3 and 5 of the flood retention basins, effectively blocking fish movements. The basins also boast a high density of the invasive Japanese knotweed (Fallopia japonica), which was frequently observed to become washed up and stuck in the river corridor, forming temporal migration barriers.
We could not detect any spawning activity of the tagged barbel in the Wien River. This could be attributed to a highly irregular spawning season in 2021, with water temperatures in the Danube River below the barbel’s preference for reproduction (Melcher and Schmutz, 2010; Pelz, 2022). This circumstance could have resulted in delayed or even interrupted spawning activities of barbel in the region, particularly considering that none of the tagged fish showed significant egg development. Other studies from Austria observed reproduction beginning at a water temperature of 14°C (Melcher and Schmutz, 2010; Pelz, 2022), a threshold not reached in the Danube River until the start of June, in contrast to preceding years when it was reached a month earlier. Moreover, the water temperature in the Wien River had exceeded 17°C prior to the translocation of barbel (Figure 2), surpassing the maximum temperature range outlined in the literature (Kottelat and Freyhof, 2007; Melcher and Schmutz, 2010; Pelz, 2022). Additionally, shortly after the release, water temperatures rose to 21.9°C (Figure 2), a value which lies substantially outside of the spawning temperature range. Still, with the barbel remaining in the system for several weeks, the study’s findings suggest that the Wien River should not be ruled out as a suitable temporary habitat.
In conclusion, this study indicates that the Wien River is unlikely to be suitable as a permanent habitat. However, given the fact that the barbel stayed in the relocation area for some weeks and moved actively in the system, the Wien River may serve as a temporary fluvial habitat for fish that undergo migrations to smaller tributaries. However, there is a need to reevaluate the results during times of stable spawning conditions while providing in-detail information on the biological prerequisites. Even though the Wien River’s function as a barbel spawning habitat remains to be confirmed, our hydro-morphological assessment suggests that this restored urban tributary could benefit Danube River fishes if instream connectivity were re-established, thereby reducing the harmful effects of river regulation (Sun et al., 2022). Therefore, this study supports the notion of restoring the longitudinal tributary-mainstem river connectivity as a means to reverse biodiversity loss in Anthropocene river systems (Tickner et al., 2020).
Data availability statement
The raw data supporting the conclusion of this article will be made available by the authors, without undue reservation.
Ethics statement
The animal study was approved by the Magistratsabteilung (MA) 22, MA45, and MA58. The study was conducted in accordance with the local legislation and institutional requirements.
Author contributions
SP: Formal Analysis, Validation, Visualization, Writing–original draft, Writing–review and editing. KP: Conceptualization, Funding acquisition, Methodology, Project administration, Supervision, Validation, Writing–original draft, Writing–review and editing. CS: Data curation, Formal Analysis, Investigation, Writing–review and editing. DH: Conceptualization, Formal Analysis, Funding acquisition, Methodology, Project administration, Supervision, Validation, Visualization, Writing–original draft, Writing–review and editing.
Funding
The author(s) declare that financial support was received for the research, authorship, and/or publication of this article. This study was funded by MA45—Wiener Gewässer, Wiener Hochschuljubiläumsfonds [H-288161/2020], Verband der österr. Arbeiter- und Fischereivereine (VÖAFV), Fischereiverein Wiental, BV Penzing, Wiener Fischereiausschuss, and Fischereirevierverband II.
Acknowledgments
Thanks to Jakob Neuburg, Michi Grohmann, and Gabriel Gruber for their help during fieldwork. Thanks to the city of Vienna, MA58 and MA22, for providing sampling permits [MA 58-228353-2021-33, MA22-440862/2021, LF1-TVG-60/001-2018]. Thanks to the reviewers for providing critical comments to improve the quality of the article.
Conflict of interest
The authors declare that the research was conducted in the absence of any commercial or financial relationships that could be construed as a potential conflict of interest.
Publisher’s note
All claims expressed in this article are solely those of the authors and do not necessarily represent those of their affiliated organizations, or those of the publisher, the editors and the reviewers. Any product that may be evaluated in this article, or claim that may be made by its manufacturer, is not guaranteed or endorsed by the publisher.
References
Banarescu, P., and Bogutskaya, N. (2003). The freshwater Fishes of Europe. Cyprinidae 2. Part II: Barbus. AULA-verlag. Available at: https://www.abebooks.com/9783891046593/Freshwater-Fishes-Europe-Cyprinidae-Part-3891046596/plp (Accessed January 11, 2024).
Bartl, E., and Keckeis, H. (2004). Growth and mortality of introduced fish larvae in a newly restored urban river. J. Fish Biol. 64, 1577–1592. doi:10.1111/j.0022-1112.2004.00412.x
BAW (2007). Fisch index Austria (FIA) leitbildkatalog. Available at: https://www.baw.at/wasser-fische-IGF/abteilungen/gewaesseroekologie-igf/eu-wasserrahmenrichtlinie-eu-wrrl-/fisch-index-austria--fia-.html.
Boavida, I., Santos, J. M., Ferreira, T., and Pinheiro, A. (2015). Barbel habitat alterations due to hydropeaking. J. Hydro-Environment Res. 9, 237–247. doi:10.1016/j.jher.2014.07.009
Bouska, K. L., Healy, B. D., Moore, M. J., Dunn, C. G., Spurgeon, J. J., and Paukert, C. P. (2023). Diverse portfolios: investing in tributaries for restoration of large river fishes in the Anthropocene. Front. Environ. Sci. 11. doi:10.3389/fenvs.2023.1151315
Britton, J. R., and Pegg, J. (2011). Ecology of European barbel Barbus barbus: implications for river, fishery, and conservation management. Rev. Fish. Sci. 19, 321–330. doi:10.1080/10641262.2011.599886
Brooker, M. P. (1985). The ecological effects of channelization. Geogr. J. 151, 63–69. doi:10.2307/633280
Copp, G. H., Faulkner, H., Doherty, S., Watkins, M. S., and Majecki, J. (2002). Diel drift behaviour of fish eggs and larvae, in particular barbel, Barbus barbus (L.), in an English chalk stream. Fish. Manag. Ecol. 9, 95–103. doi:10.1046/j.1365-2400.2002.00286.x
Dudgeon, D. (2019). Multiple threats imperil freshwater biodiversity in the Anthropocene. Curr. Biol. 29, R960–R967. doi:10.1016/j.cub.2019.08.002
Findlay, S. J., and Taylor, M. P. (2006). Why rehabilitate urban river systems? Area 38, 312–325. doi:10.1111/j.1475-4762.2006.00696.x
Gosset, C., Rives, J., and Labonne, J. (2006). Effect of habitat fragmentation on spawning migration of brown trout (Salmo trutta L.). Ecol. Freshw. Fish 15, 247–254. doi:10.1111/j.1600-0633.2006.00144.x
Gruber, G., Hayes, D. S., Lett, A., Zeiringer, B., and Pinter, K. (2022). Was tun mit urbanen Gewässern? Evaluierung des ökologischen Nutzens am Wienfluss mittels Habitatmodellierung. Österr Wasser- Abfallw 74, 433–440. doi:10.1007/s00506-022-00890-5
Guimarães, L. F., Teixeira, F. C., Pereira, J. N., Becker, B. R., Oliveira, A. K. B., Lima, A. F., et al. (2021). The challenges of urban river restoration and the proposition of a framework towards river restoration goals. J. Clean. Prod. 316, 128330. doi:10.1016/j.jclepro.2021.128330
Hayes, D. S., Muhar, S., Popp, S., Becsi, R., Mühlmann, H., Ofenböck, G., et al. (2022a). Evaluierung kultureller Ökosystemleistungen renaturierter Fließgewässer. Österr Wasser- Abfallw 74, 486–500. doi:10.1007/s00506-022-00895-0
Hayes, D. S., Schaufler, G., Schmutz, S., Unfer, G., Führer, S., Auer, S., et al. (2022b). Hydro-morphological stressors reduce distribution range and affect population status of cyprinid fishes in Austria. Front. Environ. Sci. 10, 991722. doi:10.3389/fenvs.2022.991722
Hitt, N. P., and Angermeier, P. L. (2008). River-stream connectivity affects fish bioassessment performance. Environ. Manag. 42, 132–150. doi:10.1007/s00267-008-9115-5
Hohensinner, S. (2019). “Neue Rollen: Ausbau und Rückbau seit 1918,” in Wasser Stadt Wien. Editor Zentrum für Umweltgeschichte (Vienna: Universität für Bodenkultur Wien, Technische Universität Wien), 122–143.
Huber, M., and Kirchhofer, A. (1998). Radio telemetry as a tool to study habitat use of nase (Chondrostoma nasus L.) in medium-sized rivers. Hydrobiologia 371 (372), 309–319. doi:10.1007/978-94-011-5090-3_35
Jepsen, N., Koed, A., Thorstad, E. B., and Baras, E. (2002). “Surgical implantation of telemetry transmitters in fish: how much have we learned?,” in Aquatic Telemetry: Proceedings of the Fourth Conference on Fish Telemetry in Europe (Netherlands: Springer), 239–248.
Jurajda, P. (1995). Effect of channelization and regulation on fish recruitment in a flood plain river. Regul. Rivers Res. Manag. 10, 207–215. doi:10.1002/rrr.3450100215
Kail, J., Brabec, K., Poppe, M., and Januschke, K. (2015). The effect of river restoration on fish, macroinvertebrates and aquatic macrophytes: a meta-analysis. Ecol. Indic. 58, 311–321. doi:10.1016/j.ecolind.2015.06.011
Kaiser, N. N., Feld, C. K., and Stoll, S. (2020). Does river restoration increase ecosystem services? Ecosyst. Serv. 46, 101206. doi:10.1016/j.ecoser.2020.101206
Keckeis, H., Bartl, E., Ratschan, C., and Weissenbacher, A. (2000). Evaluierung des Erfolges von Revitalisierungen im Wiener Schutzwasserbau. Modul Fischökologie – Jahresbericht 2000. Im Auftrag der MA 45 und des Bundesministeriums für Land- und Forstwirtschaft.
Köck, J., Altenhofer, M., Eberstaller-Fleischanderl, , and Eberstaller, J. (2008). Wiederherstellung der Durchgängigkeit des Wienflusses zwischen den Rückhaltebecken Auhof und dem Wienerwaldsee. Im Auftrag der MA 45 Abteilung Wasserbau Amt der NÖ Landesregierung, Abt. Wasserbau WA 3.
Kottelat, M., and Freyhof, J. (2007). Handbook of European freshwater fishes. Germany, Berlin: Kottelat, Cornol, Switzerland and Freyhof.
Lucas, M. C., and Batley, E. (1996). Seasonal movements and behaviour of adult barbel Barbus barbus, a riverine cyprinid fish: implications for river management. J. Appl. Ecol. 33, 1345–1358. doi:10.2307/2404775
Mattocks, S., Hall, C. J., and Jordaan, A. (2017). Damming, lost connectivity, and the historical role of anadromous fish in freshwater ecosystem dynamics. BioScience 67, 713–728. doi:10.1093/BIOSCI/BIX069
Mawer, R., Bruneel, S., Pauwels, I., Elings, J., Pickholtz, E., Pickholtz, R., et al. (2023). Individual variation in the habitat selection of upstream migrating fish near a barrier. Mov. Ecol. 11, 49. doi:10.1186/s40462-023-00414-0
Melcher, A., Pletterbauer, F., Kremser, H., and Schmutz, S. (2013). Temperaturansprüche und Auswirkungen des Klimawandels auf die Fischfauna in Flüssen und unterhalb von Seen. Österreichische Wasser- Abfallwirtsch. 65, 408–417. doi:10.1007/s00506-013-0118-y
Melcher, A. H., and Schmutz, S. (2010). The importance of structural features for spawning habitat of nase Chondrostoma nasus (L.) and barbel Barbus barbus (L.) in a pre-Alpine river. River Syst. 19, 33–42. doi:10.1127/1868-5749/2010/019-0033
Meulenbroek, P., Drexler, S., Nagel, C., Geistler, M., and Waidbacher, H. (2018). The importance of a constructed near-nature-like Danube fish by-pass as a lifecycle fish habitat for spawning, nurseries, growing and feeding: a long-term view with remarks on management. Mar. Freshw. Res. 69, 1857–1869. doi:10.1071/MF18121
Newhard, J. J., Devers, J., Minkkinen, S., and Mangold, M. (2021). Assessment of reintroduction of American eel into Buffalo creek (susquehanna river, Pennsylvania). J. Fish Wildl. Manag. 12, 422–433. doi:10.3996/JFWM-20-021
Nislow, K. H., Hudy, M., Letcher, B. H., and Smith, E. P. (2011). Variation in local abundance and species richness of stream fishes in relation to dispersal barriers: implications for management and conservation. Freshw. Biol. 56, 2135–2144. doi:10.1111/j.1365-2427.2011.02634.x
Ovidio, M., Hanzen, C., Gennotte, V., Michaux, J., Benitez, J.-P., and Dierckx, A. (2016). Is adult translocation a credible way to accelerate the recolonization process of Chondrostoma nasus in a rehabilitated river? Cybium 40, 43–49.
Ovidio, M., Parkinson, D., Philippart, J. C., and Baras, E. (2007). Multiyear homing and fidelity to residence areas by individual barbel (Barbus barbus). Belg. J. Zoology 137, 183–190.
Panchan, R., Pinter, K., Schmutz, S., and Unfer, G. (2022). Seasonal migration and habitat use of adult barbel (Barbus barbus) and nase (Chondrostoma nasus) along a river stretch of the Austrian Danube River. Environ. Biol. Fish. 105, 1601–1616. doi:10.1007/s10641-022-01352-3
Pavlov, D. S., and Mikheev, V. N. (2017). Downstream migration and mechanisms of dispersal of young fish in rivers. Can. J. Fish. Aquat. Sci. 74, 1312–1323. doi:10.1139/cjfas-2016-0298
Pelz, D. (2022). Characterization of spawning habitat and spawning ecology of three rheophilic fish species (Chondrostoma nasus, Barbus barbus and Vimba vimba) at the river Traisen. Austria.
Pollux, B. J. A., Pollux, P. M. J., Korosi, A., Verberk, W. C. E. P., and van der Velde, G. (2006). “Reproduction, growth, and migration of fishes in a regulated lowland tributary: potential recruitment to the river Meuse,” in Living rivers: trends and challenges in science and management. Editors R. S. E. W. Leuven, A. M. J. Ragas, A. J. M. Smits, and G. van der Velde (Dordrecht: Springer Netherlands), 105–120. doi:10.1007/1-4020-5367-3_7
Pracheil, B. M., McIntyre, P. B., and Lyons, J. D. (2013). Enhancing conservation of large-river biodiversity by accounting for tributaries. Front. Ecol. Environ. 11 (3), 124–128.
Schiemer, F., and Waidbacher, H. (1992). “Stratgies for conservation of a Danubian fish fauna,” in River conservation and management (Chichester: Wiley), 363–382.
Schmutz, S., and Jungwirth, M. (1999). Fish as indicators of large river connectivity: the Danube and its tributaries. Large Rivers 11, 329–348. doi:10.1127/lr/11/1999/329
Schopf, T. (2009). Räumliche Verteilung und Habitatwahl von Fischen in einer Versuchsstrecke des. Vienna, Austria: University of Vienna.
Stadt Wien (2023). Wienfluss - Schutz vor Hochwasser. Available at: https://www.wien.gv.at/umwelt/gewaesser/hochwasserschutz/wienfluss/index.html (Accessed November 23, 2023).
Sun, J., Tummers, J. S., Galib, S. M., and Lucas, M. C. (2022). Fish community and abundance response to improved connectivity and more natural hydromorphology in a post-industrial subcatchment. Sci. Total Environ. 802, 149720. doi:10.1016/j.scitotenv.2021.149720
Tickner, D., Opperman, J. J., Abell, R., Acreman, M., Arthington, A. H., Bunn, S. E., et al. (2020). Bending the curve of global freshwater biodiversity loss: an emergency recovery plan. BioScience, 1–13. doi:10.1093/biosci/biaa002
Vilizzi, L., and Copp, G. H. (2005). An analysis of 0+ barbel (Barbus barbus) response to discharge fluctuations in a flume. River Res. Appl. 21, 421–438. doi:10.1002/rra.806
Wolfram, G., and Mikschi, E. (2007). “Rote Liste der Fische (Pisces) Österreichs,” in Rote listen gefährdeter tiere österreichs teil 2. (Bundesministerium für Land-und Forstwirtschaft, Umwelt und Wasserwirtschaft). Editor K.-P. Zulka, 61–198.
Keywords: river restoration, fragmentation, resettlement, Cyprinidae, home range, trans-location
Citation: Popp S, Pinter K, Schwayer C and Hayes DS (2024) Habitat use of barbel (Barbus barbus) in a restored urban Danube tributary. Front. Environ. Sci. 12:1371150. doi: 10.3389/fenvs.2024.1371150
Received: 15 January 2024; Accepted: 08 April 2024;
Published: 26 April 2024.
Edited by:
Andrya Whitten, Illinois Natural History Survey (INHS), United StatesReviewed by:
Katelyn Lawson, Auburn University, United StatesStefan Tucker, Illinois Natural History Survey (INHS), United States
Copyright © 2024 Popp, Pinter, Schwayer and Hayes. This is an open-access article distributed under the terms of the Creative Commons Attribution License (CC BY). The use, distribution or reproduction in other forums is permitted, provided the original author(s) and the copyright owner(s) are credited and that the original publication in this journal is cited, in accordance with accepted academic practice. No use, distribution or reproduction is permitted which does not comply with these terms.
*Correspondence: Daniel S. Hayes, ZGFuaWVsLmhheWVzQGJva3UuYWMuYXQ=
†These authors have contributed equally to this work