Corrigendum: A systematic review of factors influencing habitat connectivity and biodiversity along road and rail routes in temperate zones
- 1School of Engineering, University of Birmingham, Birmingham, United Kingdom
- 2Birmingham Institute of Forest Research (BIFoR), Birmingham, United Kingdom
- 3Network Rail, Milton Keynes, United Kingdom
This systematic review, part funded by Network Rail Ltd (UK), considers the role of transportation corridors in habitat connectivity within temperate climates, through verge habitat, surrounding matrix, movement along and across the corridor, the wider landscape context and management practices. PICO terms were developed for the bibliographic search on 15/11/22 using Web of Science (all databases), yielding 168 studies for review. The risk of bias was minimised by excluding non-peer reviewed papers. Large and exotic taxa were excluded due to a focus on temperate zones, as were studies on invasive species and climate change where the primary focus was not ecological connectivity. Emergent themes were used to structure the paper. Results indicate that transportation corridors have significant potential for habitat connectivity, especially for generalist and open-specialist species, which favour early to mid-successional habitats. However, physiology is a key determinant in dispersal ability. Vegetation management should consider representative communities rather than individual species. Gaps exist in the range of taxa studied, understanding of seasonal variations and lifecycle stages supported in verges, survival factors such as predation and disease and changes to community structure. Rail environments are under-represented and there is limited knowledge on the relative impacts of vegetation management regimes.
1 Introduction
Transport networks facilitate the movement of goods and people between population centres, through urban, suburban, peri-urban, and rural areas. Whilst these networks have socio-economic benefits for human populations, there are fewer benefits for the third pillar of sustainable development, the environment (Brundtland, 1987; Sultana et al., 2019). Ttransportation networks create discontinuities in the natural landscape from which anthropogenic development radiates out. This is known as habitat fragmentation and can impact natural ecosystems by reducing opportunities for reproduction outside the immediate gene pool as well as limiting the capability of animal and plant populations to relocate in response to environmental and ecological pressures (Holderegger and Di Giulio, 2010; Lomolino et al., 2017; Barrientos et al., 2019; Ouedraogo et al., 2020).
Wildlife Vehicle Collisions (WVCs) are a reminder of the need for wildlife to move between habitat patches and areas of high WVC frequency usually correlate with natural routes for animal movement (Grilo et al., 2011). Inability or reluctance to cross transport corridors isolates populations and can lead to their decline. Lighting, noise, chemical pollution, and other hazards combine to exacerbate this barrier effect (Gardiner et al., 2018).
Within human altered landscapes, fragments of natural habitat of different types frequently remain which can sustain ecological communities to varying degrees of success, depending upon fragment size, quality, and connectedness. The UK’s Biodiversity Action Plan (BRIG, 2011) identifies Priority Habitat types, which Natural England report as requiring better quality, larger and more numerous habitats, which are better connected (Crick et a;l., 2020).
In the context of US forestry, a Multiple Use Model (MUM) was proposed to balance production, biological diversity and landscape stability (Harris, 1984). This comprises a core reserve area, buffers of nature sensitive activity and land which is highly human altered. This concept is extended into a Multiple Use Landscape (MUL) by providing protected corridors between the MUMs (Perry et al., 2008). In the UK, a similar “Nature Network” approach is applied by Natural England to each priority habitat category. A nature network consists of primary habitat, associated habitat (i.e., other ecologically coherent habitat) and habitat restoration regions (to create or restore primary habitat). These are supported by “Network Enhancement Zones” which create connections between habitat nodes (Crick et al., 2020).
Figure 1 illustrates some key terms relevant to landscape ecology which are referenced in this paper and are typical of fragmented landscapes. The figure shows three population centres (1,2 and 3) with different habitat corridor types connecting these populations. The matrix is land considered broadly inhospitable for dispersal, such as urban or agricultural. Note that the landscape corridor shown between population 2 and 3 requires a railway crossing for successful dispersal. The UK government National Planning Policy Framework (2012) defines stepping-stones as ‘pockets of habitat that, while not necessarily connected, facilitate the movement of species across otherwise inhospitable landscapes’ and wildlife, or linear corridors as ‘areas of habitat connecting wildlife populations’. Edge habitat conditions tend to be less habitable to interior dwellers but are often areas of high biodiversity due to a mixture of open and interior species.
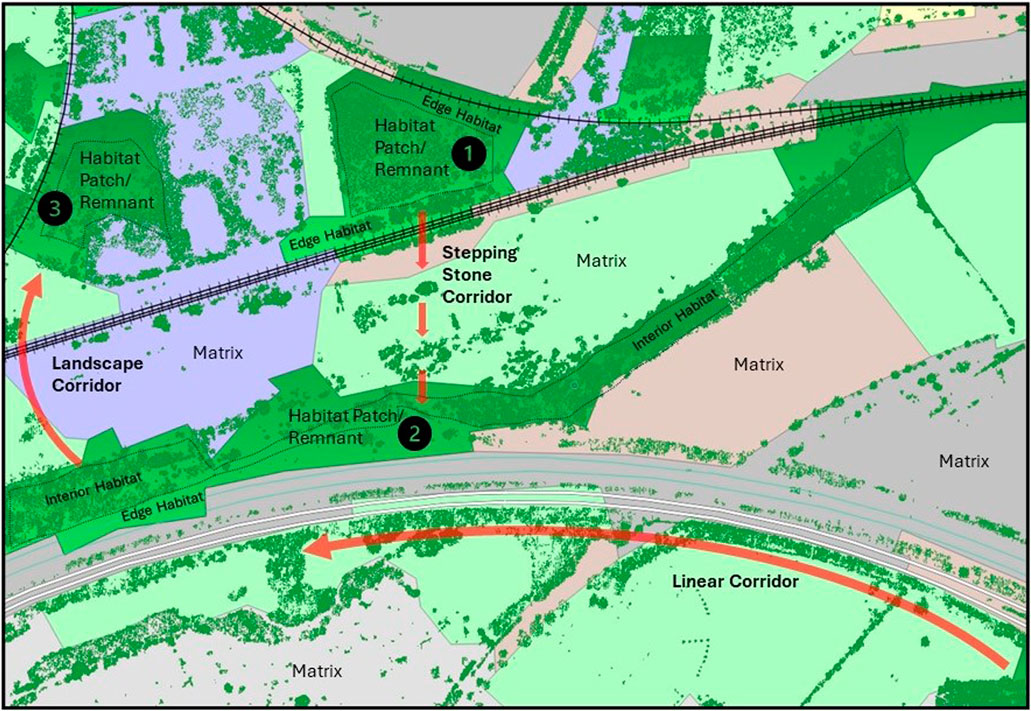
Figure 1. Illustration of Key Terms in Landscape Ecology. Data Sources: Environment Agency Vegetation Object Mode (Environment Agency, 2023). Based upon Land Cover Map 2021 © Marston et al., 2022. Contains Ordnance Survey data © Crown Copyright 2007, Licence number 100017572.
This Systematic Literature Review considers whether these principles can be applied to transportation corridors in temperate climatic regions. We hypothesise that roads and railway routes could serve as Network Enhancement Zones, because transportation providers are significant land holders and they manage continuous networks of linear corridors. For example, in the UK, Network Rail manages 20,000 route miles (on 52,000 hectares land) (Network_Rail, 2020) and National Highways manage 247,800 miles of highway, with 30,000 hectares of ‘soft estate’ (DfT, 2022; Highways_England, 2017). Indeed, an independent review of vegetation management practices notes the leading role of Network Rail in delivering targets in the UK Government’s 25 Year Environment Plan (Varley, 2018).
On a global scale, there is evidence that the transportation industry is beginning to recognise the potential for road and rail networks to support the biodiversity and habitat connectivity agenda. For example, the International Union of Railways (UIC) rEvERsE project (Ecological Effects of Railways on Wildlife) project sought “solutions and best practice to manage rail lineside in a way that can help halt and reverse the loss of biodiversity”. Other UIC initiatives include the TRISTRAM project (TRansItion STRategy on vegetAtion Management) resulting in a “UIC strategy on the Future of Vegetation Control” and the BISON project (Biodiversity and Infrastructure Synergies and Opportunities for European transport Networks) (UIC, 2021; UIC, 2022; UIC, 2023). In the UK, there are several high-profile examples of Network Enhancement Zones associated with transport corridors, including the ‘Green Corridor’ Initiative for HS2 (HS2, 2020), the ‘Green Ribs Initiative’ for the A30 Chiverton to Carland Cross scheme (National_Highways, 2023a) and the Lower Thames Crossing, which is building seven green bridges (National_Highways, 2023b).
This review presents state-of-the-art research and discusses methods and approaches to optimise the environmental value of transportation assets in supporting sustainable populations and biodiversity. In Section 2 we describe our methodology for the Systematic Review, including research question elaboration, search term development, article identification and the screening process. Section 3 presents the results of the review in terms of the themes developed in Section 2 and is broadly structured by taxonomic group (i.e., plants, invertebrates, and vertebrates). The discussion in Section 4 follows a similar structure and highlights gaps and opportunities for future research. Finally, Section 5 presents concluding statements, outlining key findings for transport practitioners, and pointing towards future research challenges.
2 Review methods
This research field is growing rapidly due to advances in remote sensing and digital data manipulation (e.g., Geographic Information Systems, GIS, and Machine Learning). For this reason, a Systematic Review approach has been adopted to provide a baseline of contemporary knowledge. This approach allows us to identify, select and critically appraise research to answer a clearly formulated question (Dewey and Drahota, 2016).
2.1 Research questions/PICO methodology
The objective of the review is to outline contemporary understanding of transportation verge ecosystems and their role in landscape scale habitat connectivity in a Temperate Zone context. The formulation of the research question applied a modified, or “universal PICO scheme” approach. This rephrases the PICO mnemonic as: the ‘problem’, or P; the ‘intervention’, or I; the ‘control’, or C; and the ‘outcome’, or O (Nishikawa-Pacher, 2022).
“How do biotic assemblages (P) subjected to Linear Transportation environments (I) respond in terms of movement along and across the corridor (O) compared to other environments (C)?”
The Temperate Zone focus was addressed during screening (see 2.4). To comprehensively address the research question, a series of themes and sub-questions were developed from an assessment of the review papers, which provides structure when analysing the entire dataset. Similar approaches have been followed in the fields of sports science and educational research (Pickering and Byrne, 2014; Thomson et al., 2019). This approach enables exploration of emergent characteristics and themes across the dataset, and are presented in Table 1. The full literature search process is summarised in Figure 2.
2.2 Search terms
A Boolean sequence of search terms was developed for the bibliographic search using synonyms and related terms derived from PICO statement. Each column in Table 2 represents a block of similar terms and are captured using OR logic. These columns were combined using AND logic. Wildcard characters were employed to allow for variations in usage of terms. For example, “rail*” will select for railway, rail, or railroad.
2.3 Identification
This interdisciplinary systematic review spans fields of Engineering and Earth and Environmental Sciences. Within the Web of Science compendium of databases, the Core Collection (sciences, social sciences, and arts and humanities) and Zoological Record (animal biology and biodiversity) were considered to provide a good representation of papers from all relevant fields. An “All Databases” search was carried out on 15th November 2022.
An initial search yielded over 1,000 records. Due to the large dataset, a pragmatic approach was invoked from previous reviews which determined the date range applicable to the review (Okoli and Schabram, 2015; Hayrol et al., 2020). This process was refined by initially exploring the Review papers to determine key themes, then limiting the remainder to publication dates in or after 2000. The rationale behind this was that reviews would be able to summarise research prior to 2000.
The peer review status of a journal is a requirement in the journal evaluation process for inclusion in Web of Science. Since only review papers and research articles were selected, and not editorials or letters, utilisation of WoS guards against bias in publications.
2.4 Screening
Three screening stages were applied to both sets of records (review and non-reviews). In the first stage the titles were reviewed to screen out duplicates, datasets, or items without sufficient metadata. The second stage removed records which were off topic. Stage 3 screening entailed a detailed review of abstracts based on subject matter to determine eligibility. The review was framed within the context of transportation infrastructure in a temperate climate. Therefore, the screening process excluded records which were not analogous to temperate regions, or which focussed on large mammals. Also, since the objective was to review primarily habitat connectivity of verges, invasive species were screened out as were records which did not relate to the verge environment (see 4.2 for discussion on these limitations). Screening for eligibility based on the focus of the paper has precedent in other published Systematic Literature Reviews (Hayrol et al., 2020). The screening process yielded 24 review articles and 144 other articles.
2.5 Data collection
The Web of Science metadata was exported to EndNote Desktop refence management system and papers were imported for review and markup. Metadata was exported from EndNote to MS Excel where notes and comments were added during the review process. Notes were categorised into the themes outlined in Table 1 and Papers were classified to determine useful statistics. The 24 Review articles which made it through screening were reviewed in full and categorised against the themes, or outcomes identified in Table 1. The 144 non-review articles were first scrutinised by abstract and if the context was loosely applicable to the themes, results and conclusions were categorised from the abstract. If wholly relevant to the themes, the full paper was reviewed and detailed notes taken and categorised.
3 Results
This section is split into three parts. First, an analysis of metadata from the bibliographic search, then presentation of the review findings. These have been grouped thematically and address the sub-questions identified in Table 1. The last part covers gaps and limitations identified in the literature which help in the understanding of uncertainty and future challenges for research and vegetation management.
3.1 Descriptive analysis of publications
After screening, the literature search returned 168 articles for full analysis. 24 were reviews and 144 papers classed collectively as non-reviews. The upward trend for research in this topic can be seen in Figure 3.
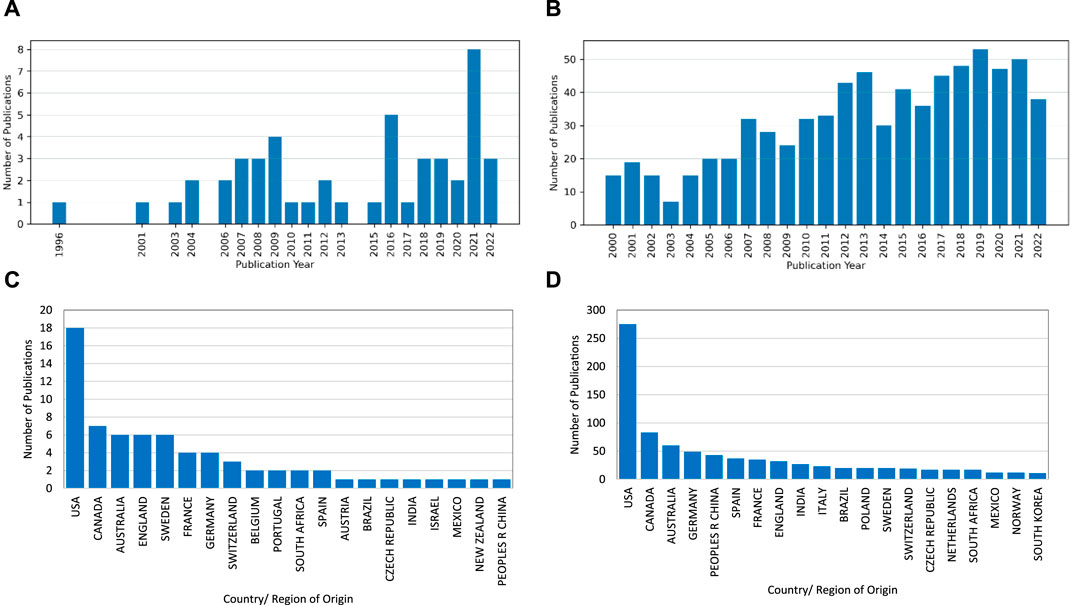
Figure 3. Year of publication and country/region of origin ((A,C) are reviews; (B,D) are non-reviews).
The Web of Science “Addresses” field is utilised to produce the “Countries/Regions” data shown in the two graphs in the lower half of Figure 3. Note that These graphs have been curtailed to the Top 20 regions.
Figure 4 provides insights into the range of taxa studied using keyword searches within titles or abstracts. Of the 144 non-review articles, 48% specifically referred to plants/trees, 25% to invertebrates and 42% to vertebrates. For the review articles this was 72%, 20% and 36% respectively. 23 out of the total 168 screened articles discussed pollinators.
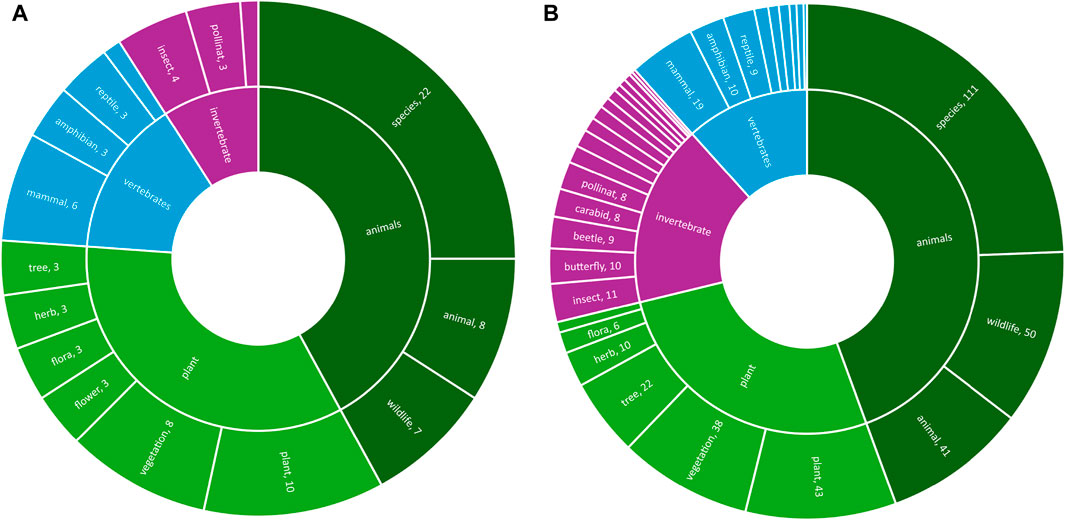
Figure 4. Range of taxa covered in bibliographic search ((A,B) are reviews and non-reviews respectively).
3.2 Findings of SLR based upon developed themes
3.2.1 Verge biodiversity
Verges provide critical habitat and linkage corridors between remaining patches of native habitat for dispersal of native flora and fauna. These functions help to sustain biodiversity and to mitigate against fragmentation of populations, genetic isolation and ultimately, population collapse and potentially local extinctions (New et al., 2021). However, if occupying a verge limits reproduction or dispersal, or impacts survivorship, and ultimately population persistence, then these habitats could represent ecological traps that act as population sinks. Also, due to compositional shifts, verge habitats could trigger changes in species interactions and co-evolutionary processes (Gardiner et al., 2018; Lazaro-Lobo and Ervin, 2019).
Verge vegetation can be highly diverse and can harbour remnant populations of rare and threatened flora and fauna as well as species excluded from the matrix, however this does not mean to say that such habitats can support viable populations (Roy and de Blois, 2006; Gardiner et al., 2018; New et al., 2021). The seed bank within verges is supportive of revegetation, and vegetated slopes contribute to the control of erosion (Lazaro-Lobo and Ervin, 2019). Verges are frequently prevented from progressing into mature forest by management interventions. These “early successional states” are open habitats supportive of many biomes and high biodiversity. Edge habitat conditions predominate which favour some plant communities over others due to altered biotic and abiotic conditions (Roy and de Blois, 2006; Suarez-Esteban et al., 2016). Vegetation management practices within verges also influence species composition.
Verges are key to maintaining native plant biodiversity in human modified landscapes (Lazaro-Lobo and Ervin, 2019), however, plant diversity is generally lower in verges within a natural landscape matrix (Suarez-Esteban et al., 2016). Verges can be unsupportive to the establishment of forest herbs which can be disturbance intolerant (Gardiner et al., 2018). Contiguous hedgerows generally contain fewer early flowering herbs and have a higher likelihood of, and susceptibility to, frost than native forest environments (Roy and de Blois, 2006). Generalists, ruderal, wind dispersed, high-seed-output, insect pollinated and plants which reproduce by vegetative propagation predominate in these locations (Roy and de Blois, 2006; de la Riva et al., 2011; Ranta et al., 2015).
The verge environment is associated with anthropogenic factors such as noise levels, lighting, and traffic density, which can have a detrimental impact on animal behaviour, resulting in higher energy expenditure and reduced fitness. Similarly, chemical pollutants can cause harm to natural functioning (Trombulak and Frissell, 2000; Shier et al., 2012; Gardiner et al., 2018; Barrientos et al., 2019; Ouedraogo et al., 2020). The introduction of artificial elements within the verge can have a detrimental effect on biodiversity through habitat loss and barrier effects leading to, amongst other things, broken food web linkages (Gardiner et al., 2018). Imbalance in community compositions and environmental factors may be detrimental to prolonged occupation of verges and combined with increased mortality, these areas may become ecological traps (Gardiner et al., 2018).
The high suitability of the verge environment for habitat generalist, open-specialist, early successional, disturbance tolerant or opportunistic animal and plant species (including invasive species) is well documented, as is the limiting effect on many habitat specialist species (Koivula, 2005; Van Rossum, 2009; Schaffers et al., 2012; Knapp et al., 2013; Bochet and Garcia-Fayos, 2015; Ranta et al., 2015; Gardiner et al., 2018; Villemey et al., 2018; Barrientos et al., 2019; Lazaro-Lobo and Ervin, 2019). Verge vegetation composition and condition is a key factor for invertebrate diversity and abundance, influencing movement and sometimes supporting life-processes, even reproduction and overwintering. Rare and functionally important insect species (e.g., pollinators or pest predators) can gain an essential foothold in remnant vegetation in an otherwise hostile landscape matrix (Noordijk et al., 2011; Schaffers et al., 2012; Gardiner et al., 2018; New et al., 2021).
In general, for human-altered environments, invertebrate diversity indicators were higher within verges compared to surrounding environments, even accounting for mortality from vehicles and pollution (Villemey et al., 2018). For some invertebrates, studies confirm that verges can support the full range of life processes (Koivula, 2005; Noordijk et al., 2011; Schaffers et al., 2012). Local factors (e.g., High nectar plants, tall vegetation, percentage litter) and regional factors (e.g., road density) were found to be key drivers for grassland pollinator species compositions (Saarinen et al., 2005; Davis et al., 2007; Phillips et al., 2020). Additionally, the presence of dead wood in the verge is a crucial resource for saproxylic and hole-nesting invertebrates (New et al., 2021).
Vertebrate presence in verges is influenced by the infrastructure type, vegetation cover and the surrounding matrix. Mammal predators such as foxes, badgers and snakes tend to be negatively affected by road verges. Studies indicate that small mammal species may therefore utilise verges as refugia (habitats where prey is relatively safe from predators) due partly to release from predation (Redon et al., 2015; Ouedraogo et al., 2020). A 2015 examination of the factors influencing the presence of bird groups in Natura 2000 sites in Cyprus found that road networks have negative effects on four of the five bird categories (Mammides et al., 2015).
Road noise is thought to have a detrimental effect on the activities of some birds by interrupting acoustic signals, causing stress, and impacting foraging activity (Ortega, 2012; Gardiner et al., 2018). Similarly, a study of the Stephen’s kangaroo rat found that road noise can lead to false responses that may be energetically costly (Shier et al., 2012). Artificial lighting is thought to have an overall negative impact on bat reproduction and commuting behaviour despite local benefits due to insects attracted to lights (Ouedraogo et al., 2020).
It is thought that railway verges in particular promote bird species richness due to the availability of remnants of diverse vegetation and the availability of food, shelter, perching, nesting, and resting (Ouedraogo et al., 2020; Dylewski and Tobolka, 2022). In addition, the presence of culverts and other transportation structures can also offer nesting opportunities (Dean et al., 2018).
3.2.2 Surrounding matrix
Plant abundance and diversity within verges is generally higher compared to adjacent areas when embedded in a human-altered landscape but lower compared to natural areas, although verges in natural areas are more biodiverse than verges in human altered areas (Suarez-Esteban et al., 2016; Lazaro-Lobo and Ervin, 2019). Verges can provide better conditions than adjacent habitats for plant dispersal and post-dispersal processes which contribute to success such as providing light, animal dispersers, high seedbank counts and less seed predation (Suarez-Esteban et al., 2016; Lazaro-Lobo and Ervin, 2019). For example, light favouring plant species can establish within verges, raising overall plant diversity in nearby forest areas (Avon et al., 2010).
In most instances invertebrate abundance is generally higher within the verge, compared with adjacent areas of similar composition. This is especially true amongst pollinators on railway embankments (Villemey et al., 2018). Verges in rural areas can provide shelter for pollinators and invertebrate predators of crop pests as well as resources and refuge habitats that are no longer available in the wider landscape. However they are susceptible to overspray from agricultural chemicals (New et al., 2021). A 2013 study of roadside invertebrates found that habitat generalists and open habitat specialists appeared to benefit from road proximity in both forest and open habitats, suggesting that roads could offer habitat and corridors for the benefit of non-specialist species (Knapp et al., 2013).
Vertebrate biodiversity within verges is dependent on the surrounding matrix. Urban areas tend to be detrimental to amphibian populations, shrubland birds and bats, whereas verges in natural areas have similar biodiversity to similar habitat in adjacent land (Ouedraogo et al., 2020). For fragmentation-intolerant birds such as passerine species (true perching birds, with three toes directed forward and one backward) as well as larger mammals, road verge occupancy is lower than surrounding areas. However, raptor biodiversity indicators were higher, suggesting that they can exploit the verge environment for hunting due to increased food availability (Gardiner et al., 2018; Ouedraogo et al., 2020). Studies suggest that in rural landscapes, roadside vegetation can attract birds for breeding, foraging, or resting, leading to collisions or conditions which are detrimental to survival (Gardiner et al., 2018).
3.2.3 Movement and dispersal along the transportation corridor
Transportation corridor verges tend to increase movement and dispersal of plants, vertebrates, and invertebrates between habitat patches, but overall effectiveness varies among taxa (Ouedraogo et al., 2020). Verges appear to assist in the dispersal of some moth species, but vegetation can hinder the movement of open-ground specialists such as carabid beetles (Butterweck and Ce, 2000; Do et al., 2017; Villemey et al., 2018; Elo et al., 2021). Many foodplants for invertebrates exist in verge environments, promoting dispersal over a prolonged period (Brunzel et al., 2004). Modelling has confirmed that highway verges played an important role in A. albopictus (mosquito) range expansion in mainland France, consistent with observed findings (Roques and Bonnefon, 2016).
Mammal movement along verges was found to be equivalent or higher to similar habitat elsewhere, and may function as both habitat and movement corridor (Ouedraogo et al., 2020). Meso-predators, including foxes, were found to frequently utilise road corridors within their home range for hunting (Frey and Conover, 2006). Also, hedgehogs were observed to stay close to roads when commuting (Doncaster et al., 2001). Similar can be inferred from genetic modelling for cottontail rabbits and a study on Virginia opossums (Beatty et al., 2014; Amaral et al., 2016). A study carried out in Melbourne found that bandicoots had longer home range areas in narrow strips than wider remnants (Maclagan et al., 2020). Using landscape genetic analysis, wood mice were found not to utilise road environs for dispersal (Wilson et al., 2016).
Studies into bat foraging behaviour around roadside verges found that traffic density was a determining factor, with detrimental effects for frequencies above 10 vehicles every 5 min and barrier effects at frequencies over 200 vehicles every 5 min (Bennett et al., 2013). Another study found that foraging near artificial lighting occurred predominantly around water bodies and well vegetated areas, but not around roads (Voigt et al., 2020). Studies also found that commuting bats favoured dark corridors and flew parallel to the edges of linear landscape features and that feeding along linear features is low, whilst other studies report no negative impact (Kalcounis-Rueppell et al., 2013; Vandevelde et al., 2014; Voigt et al., 2020). Additionally, it has been found that barn owls do not favour linear features when selecting roost sites (Ruiz et al., 2021).
Studies on reptile movements showed that remnant shape influenced ranges, with linear remnants being least preferred (Driscoll, 2004). One study in South Australia found that small linear fragments provided poor connectivity over larger distances (Williams et al., 2012). Alpine newts were observed to utilise road corridors in areas of adjacent low quality (Prunier et al., 2014).
Pollinated and early successional plants were found to benefit from open, linear landscape elements, which promoted long distance dispersal (Van Rossum, 2009). Seed dispersing animals also tended to move along verges promoting seed establishment (Suarez-Esteban et al., 2016). Forest herbs appear to benefit from the shelter and habitat provided by linear hedgerow features as well as facilitating dispersal (Roy and de Blois, 2006). Stepping-stone habitats (see Figure 1 for illustration) can offer sufficient conditions for dispersal, seed establishment and regeneration to disperse over multiple generations (Travers et al., 2021). Also, grassland ecosystems have been found to propagate effectively via transportation verges (Tikka et al., 2001).
3.2.4 Movement and dispersal across the transportation corridor
When considering animal and plant dispersal perpendicular to transportation corridors, barrier effects can be considerable leading to genetic isolation of populations over multiple generations and an overall fragmenting impact on biodiversity (Holderegger and Di Giulio, 2010; Barrientos et al., 2019; Ouedraogo et al., 2020). It is shown below that the magnitude of the barrier effect is dependent upon physiological and behavioural aspects as well as the composition and layout of the verge environment and surrounding land use. Mortality due to crossing attempts can also impact on population size to significant levels (Barrientos et al., 2019).
Research on animal mortality around transport networks focusses mainly on vehicular collisions, although electrocution, drowning and catenary line collisions have been studied (Holderegger and Di Giulio, 2010; Barrientos et al., 2019; Ouedraogo et al., 2020). A 2019 study suggested that current low mortality figures in some areas could also be indicative of massive past mortality, impacting populations and behaviours (Ascensao et al., 2019).
Areas of high functional connectivity, high traffic density and high road sinuosity (hence low visibility) were modelled to be high risk factors for animal mortality, as were road junctions in fenced highways and areas where roads bi-sect favourable cover, foraging, or breeding habitat (Grilo et al., 2011; Cserkesz et al., 2013). Gunson et al. (2011) provides an informative table of predictors for WVCs grouped by ungulates, carnivores, small-medium vertebrates, birds, and amphibians and reptiles (Gunson et al., 2011). The relatively infrequent traffic seen on railways (when compared with roads) is considered to introduce an element of unpredictability which can influence mortality risk (Dorsey et al., 2015).
Insect mortality is higher in grassland areas than forests and pollinator crossing likelihood appears to be linked to the availability of resources on the near verge (Daniel-Ferreira et al., 2022). Behavioural studies of two butterfly species revealed a reluctance of one species to cross habitat edges, suggesting that edge tolerant species are more likely to emigrate successfully across highly fragmented landscapes (Ries and Debinski, 2001). Reporting on invertebrate road mortality data, the UK based survey, “Buglife” has revealed a 63.7% decrease in “insect splat” events over 18 years up to 2022, suggesting marked declines in insect populations, which is consistent with a 2017 study in Germany which reported a 76% drop in flying insect biomass over 27 years (Ball, 2022).
Amphibian mortality is higher after rain events, and as well as crossing to reach favourable habitat, road crossing could serve other purposes including foraging, dispersal and regulation of body temperature (Timm and McGarigal, 2014). Studies on dormice and newts revealed no road barrier effect (Prunier et al., 2014; Friebe et al., 2018). A study in the Czech Republic found that forested areas appear to increase road mortality risk for medium sized carnivores such as the otter, marten, polecat, fox and badger (Cervinka et al., 2015). Genetic studies on wolverines demonstrated sex-bias in road crossing behaviours which may lead to isolation of populations and demographic fragmentation (Sawaya et al., 2019).
Over and under corridor crossing structures are most cited interventions to mitigate against Wildlife-vehicle collisions, but exclusion fencing, audible alarms, repellent odours, tree screening are also promoted in appropriate circumstances. Purpose built wildlife overpasses were found to be used significantly more than those shared with humans, and natural materials were preferred (Cserkesz et al., 2013; Denneboom et al., 2021).
Short under-road crossings are favoured over long ones and some studies found that central reservation, or median strips of vegetated land linking crossings are an effective remedy. Preferences for sizes and entry characteristics as well as spacing were different across taxa. The characteristic of adjacent vegetation is also important (Clevenger et al., 2001; McDonald and St Clair, 2004; Taylor and Goldingay, 2014).
3.2.5 Connectivity with the wider landscape
Connectivity models on the impact of tree loss to ecological connectivity demonstrate that roadside trees provide a disproportionately high contribution to landscape-scale dispersal ability (Henry et al., 2017). Spatial dynamic modelling of land use change models found that development resulting in 10% habitat losses could half populations of some species within the landscape (Aurambout et al., 2005). A study in Poland of non-specialised pollinators (butterflies, bees, hoverflies) along railway verges found that community composition on embankments were spatially dependant, indicating that railways potentially perform a dispersal corridor function. (Moron et al., 2017).
A 2011 study in Texas on small mammal movements across and along highways found that small mammals have been observed to utilise unfarmed crop corners, and so these features act as remnant habitat when linking up with transportation verges for habitat connectivity (Kuykendall and Keller, 2011). The State Acres for Wildlife Enhancement programme for small mammal conservation in United States of America, reported Vole abundance during the first year following restoration depended on spatial connectivity provided by linear habitats (roadside ditches and grass waterways) (Mulligan et al., 2013). In an urban setting, public green spaces were found to increase “urban-adapted” bird connectivity, although the cumulative effect of urban barriers, traffic volume, distance between vegetation and amount of canopy cover can impact this significantly (Tremblay and St Clair, 2009; 2011; Rycken et al., 2022). A study on possum populations in Australia found that canopy connectivity is an important factor as crossing at floor level does not occur (Wilson et al., 2007).
3.2.6 Verge management and interventions
Management actions may increase plant diversity by emulating natural disturbance regimes (Suarez-Esteban et al., 2016). However, this can create pronounced fluctuations in environmental conditions, including access to light and nutrients, which increases susceptibility to invasion by exotic species. Maintaining a perennial vegetation cover reduces this threat as well as enhancing nutrient cycling, reducing erosion and controlling noise. However, hydroseeding practices using non-native seed mixtures can displace native flora (Milton et al., 2015; Lazaro-Lobo and Ervin, 2019). Topsoil spreading to control erosion was found to accelerate establishment of a dense plant cover and ecosystem functioning (Lazaro-Lobo and Ervin, 2019). Embankments planted with woody vegetation did not promote natural plant colonisation and plant colonization occurs spontaneously in road verges, dependant on embankment age and surrounding vegetation (Arenas et al., 2015). A study of abiotic and biotic drivers in forest edges along railway lines in Sweden found that early successional productivity was influenced by the herb layer, soil moisture, humidity, and altitude, whilst late successional productivity was influenced by forest edge profile, canopy stratification and cover, forest continuity and the shape and contrast of edges in the surrounding landscape. (Wistrom and Nielsen, 2016).
A study into hedgerow planting found that a mosaic of hedgerows and grassland habitats increased spider and plant biodiversity at a landscape scale (Le Viol et al., 2008). Studies in Iowa which restored prairie habitat with the aim of increasing butterfly biodiversity found that the species richness of plants in flower had the strongest effect on richness and abundance of both generalist and habitat specialist butterfly species (Ries et al., 2001). Bee and dragonfly biodiversity was found to benefit from reduced mowing frequency, reduced artificial lighting, and replacement of invasive species with native nectar sources (Villemey et al., 2018; Phillips et al., 2020). Also, maintaining high flower densities and diversity in verges were found to reduce road crossing mortality of flower-visiting invertebrates (Daniel-Ferreira et al., 2022). Planting the larval foodplant in verges with many nectar plants was found to encourage the Oregon silver spot butterfly to remain and lay eggs on one side (Zielin et al., 2016).
Invertebrate mortality under differing mowing regimes were found to vary across taxa but was optimally two or less per annum, avoiding key life stage windows (Halbritter et al., 2015; Villemey et al., 2018; Parile et al., 2021). A study on meadow butterflies found that mid-summer mowing led to lower population density and delayed return to the area until the vegetation had regenerated (Valtonen and Saarinen, 2005). A study on monarch butterflies found that egg abundance was higher in mowed plots, and best results were seen in plots mowed once before egg-laying peaks (Knight et al., 2019). The use of eco-friendly mowing heads was found to reduce invertebrate mortality but effectiveness varied across taxa, with full mitigation for butterflies and moths and poor results for bees, wasps and beetles (Steidle et al., 2022).
Bat response to artificial lighting is species dependent. One review concluded that bat activity was highest in white light and lowest with orange (Ouedraogo et al., 2020). Bats appear to have an acoustic disturbance threshold, avoiding roads when noise levels reached around 88 dB for the studied species (Bennett and Zurcher, 2013).
Studies indicate that vegetated overpass crossing structures can benefit a broad range of taxonomic groups, including birds, amphibians, and reptiles (Jones and Pickvance, 2013; McGregor et al., 2015; Colley et al., 2017). A study in Brisbane found that forest specialist birds were also found to use wildlife overpasses designed for ground dwelling animals (Jones and Pickvance, 2013). Abundant vegetation and habitat restoration in the locality of crossing structures was found to increase the likelihood of use (Clevenger et al., 2001; Ng et al., 2004). Fencing has also been promoted in some locations where favourable habitat is located on both sides of the corridor (Ng et al., 2004), as is insulation of exposed electrical equipment for the protection of birds and arboreal animals (Laidlaw et al., 2021).
A pilot scheme in Switzerland installed “Amphibian plates” to the inside and outside of rails to facilitate crossing of rail tracks with some success (Chang et al., 2014; UIC, 2023). A study in Quebec found that a vegetated median strip improved the use of under-road crossings by providing foraging opportunities for some small mammals between culverts and by reducing the overall length of culvert (Martinig and McLaren, 2019). A pilot scheme was implemented in the Netherlands which utilised a modified hollow rail sleeper to enable small animals such as frogs, snakes and toads to safely cross the network below the tracks (UIC, 2023).
3.3 Gaps and limitations identified in research papers
Reviews found that there is limited understanding relating to the lifecycle stages which can be supported in verge environments across most taxa, with a bias towards large, charismatic species (Barrientos et al., 2019; New et al., 2021). It is also unclear how verges influence survival factors such as predation, parasitism and disease or community level interactions such as diet, competition, and mutualisms (Gardiner et al., 2018). Nor is there a satisfactory understanding of reproductive interactions such as calling, nesting, and other behaviours. Without a better understanding of these factors, it is difficult to conclude whether verges can perform the function of a habitat fragment, thereby adding to the overall habitat area in a region (Dawson, 1991; Gardiner et al., 2018; Barrientos et al., 2019).
Studies tend to focus on individual species, rather than the mechanisms within animal and plant communities such as surrounding habitats, underground composition, abiotic factors, and successional characteristics. Without more clarity, the conservation potential of verge habitats cannot be clearly determined (Roy and de Blois, 2006; Suarez-Esteban et al., 2016). Most studies collected data over a window of less than 2 years and inter-annual variation could yield different results. Seasonal fluctuation were also understudied (Ouedraogo et al., 2020).
The role of verges in functional connectivity at a landscape scale, whilst theoretically explainable and supported by models, is not yet underpinned with field measurements of dispersal ability across many taxa of plants and animals, although larger animals can benefit from radio tagging technology (Ouedraogo et al., 2020; New et al., 2021). Telemetry is a rapidly developing field and may soon be able to address this gap for some taxa (Dawson, 1991). Similarly, detailed mortality metadata and genetic studies would allow a better understanding of drivers for population dynamics at landscape scale (Gardiner et al., 2018; Barrientos et al., 2019). It is also unclear how shape factors such as verge width and interactions with surrounding landscape affect functional connectivity (Gardiner et al., 2018).
Road settings are studied far more than rail and the impact of disruptive factors associated with transportation networks on animal behaviour and ecosystem function require further study. These include the effects of noise, lighting, vibration, chemical pollution, and heat. (Gardiner et al., 2018; Barrientos et al., 2019; Ouedraogo et al., 2020). Whilst studies have been carried out for vegetation management practices and their associated impact on biodiversity, this is limited and skewed towards mowing and pollinator impact. This research should be widened in terms of taxa, method, habitat and infrastructure type (Villemey et al., 2018).
4 Discussion
4.1 Discussion of results
This review has found that research is heavily focussed on roadside environments, but it seems reasonable to draw parallels to the rail environment in most cases. One key difference between the two modes of transport is the much lower density of traffic on railways which one would imagine significantly reduces the likelihood and frequency of animal collisions, even considering the increased mortality risk due to train infrequency highlighted in some literature, e.g., Dorsey et al., 2015.
4.1.1 Verge biodiversity
Whilst the loss of total habitat area is understood to consistently reduce biodiversity and negatively impact populations, research reveals that verge environments which are functionally similar to adjacent habitat patches can increase the overall regional habitat area. In this capacity, verges may function as stepping-stone habitats. The extensive reach of transportation verges throughout the landscape provides an opportunity to utilise these features for biodiversity gains. Also, studies indicate that overall biodiversity can be increased by eco-sensitive management interventions of the verge where it is an ecotone between open and dense habitats. Ecotones have been described in literature as having potential to support greater biodiversity (Perry et al., 2008).
We have seen that habitat specialists suffer greatest harm from habitat loss and fragmentation, and therefore stand to gain more from increased efforts to provide suitable habitat conditions. There is therefore a risk that a management focus on biodiversity indicators of richness and abundance alone would fail to address the needs of habitat specialists. It would therefore be beneficial to consider habitat specialist species within the list of target species for habitat restoration. A review of the IUCN Red List across a range of taxa for the region under consideration could yield candidate species for special consideration.
4.1.2 Surrounding matrix
In human-altered environments, we have seen that verges offer significant opportunities to maintain biodiversity by providing suitable conditions for plant and animal refuge and dispersal between habitat patches, which may be prohibitive in adjacent areas. It follows that verges can provide a valuable ecosystem function at landscape scale. Improvements in verge condition and vegetation continuity between habitat patches will therefore promote ecological recovery. Additionally, other valuable ecosystem services such as pollination and crop pest control can result from these improvements. Therefore, local context should be considered when making decisions on verge management practices.
4.1.3 Movement and dispersal along the transportation corridor
We have seen that the physiological characteristics of an organism greatly influence dispersal ability. This organism-specific response precludes a “one size fits all” approach to optimising the verge environment for ecological connectivity. Therefore assessment of interventions consider representative communities rather than individual species.
Also, when considering dispersal and range-expansion a key consideration is the rate of movement. In many animals this may be expressed by speed across the terrain, but for smaller and less mobile organisms such as plants it is often more appropriate to consider dispersal over generations (Beier et al., 2008). In the latter case, the verge environment would need to support all life cycle stages and is therefore likely to resemble native habitat for slow-dispersing species.
4.1.4 Movement and dispersal across the transportation corridor
The network of roads and railways bisecting the landscape is a significant fragmentation factor and studies suggest that this can create physiological and behavioural barriers to movement, potentially leading to genetic isolation and eventually population decline. A key question is how to facilitate ecological connectivity to beneficial levels whilst at the same time managing the infrastructure for safe and cost-effective operations. This would involve raising awareness within all the business functions able to contribute and having a structure in place to coordinate, implement, monitor, and evaluate such activity.
We can draw a general conclusion that mortality risk is highest where roads bisect areas of natural functional connectivity (Grilo et al., 2011). This indicates that an efficient strategy to both reduce losses and to facilitate functional connectivity is to prioritise crossing safety at these locations.
Crossing structures can range from simple pipes within the ground through to elaborate vegetated overbridges. However, studies suggest that installing culverts and pipes of varying sizes at frequent intervals at crossing locations would benefit a large range of taxa from invertebrates up to small mammals (Clevenger et al., 2001). For larger animals, crossing prevention may help to limit mortality.
4.1.5 Connectivity with the wider landscape
Climate change and land use change will influence the effectiveness of connectivity measures over time due to shifts in vegetation assemblies (IPCC, 2001). Therefore, measures to maintain and improve connectivity along verges should allow for consideration of these temporal factors on species distributions and the effectiveness of proposals over time. Successful implementation at landscape scale depends upon effective collaboration with stakeholders and coordinated design (Weber and Allen, 2010; Apfelbaum et al., 2012).
Research indicates that, where multiple habitat types co-exist in an area, verges containing stepping-stone patches of habitat at suitable intervals for the focal species will provide permeability for multiple habitat ecosystems. Also, since studies confirm the benefits of transportation verges in functional connectivity for pollinators and woodland/forest species it seems reasonable to adopt a strategy for protection and restoration of these habitat types where they exist along verges today.
4.1.6 Verge management/interventions
Verge management practices need to consider safety aspects related to the operational infrastructure, such as route visibility or windthrow, and some treatments may be prohibitive due to regular access requirements (Milton et al., 2015; Villemey et al., 2018). The methods used would also need to consider risks and hazards to all focal species (Beier et al., 2008). The challenge for management is therefore to manage vegetation to enhance the positive impacts and reduce their negative effects (Milton et al., 2015).
Given the reach of road and railway networks throughout the landscape, it would be prudent to identify and prioritise locations on the network which are best able to support the function of habitat connectivity at a landscape scale. With this knowledge, management action can be coordinated in such a way as to promote biodiversity and habitat connectivity at the same time as prioritising for transportation delivery. A body of knowledge should be collated on vegetation management methods and interventions which promote biodiversity and reduce harm within given habitat types and given taxa. Whilst research is available to support this, we have seen that there are still many gaps across taxa.
Appropriate siting and design of crossing structures will be influenced by habitat distribution across the landscape and the taxa under consideration as well as commercial and operational constraints. Priority is likely to be given to underpass rather than overpass designs where appropriate due to cost and operational factors, especially in railway environments. When designing crossings, careful consideration is to be given towards vegetation composition around crossing points as research indicates that composition and layout can both help and hinder safe crossing (Cserkesz et al., 2013; Nevrelova et al., 2022). Culverts should be frequently spaced and of mixed sized openings to benefit a wide range of taxa (Clevenger et al., 2001; Jones and Pickvance, 2013). Similarly, livestock/wildlife fencing design requires a careful balance of preventing collisions and promoting dispersal (Ng et al., 2004; Holderegger and Di Giulio, 2010; Cserkesz et al., 2013; Ouedraogo et al., 2020).
4.2 Discussion of limitations of the review
The decision to limit the search to the bibliographic databases within the Web of Science compendium is not considered to be a major weakness in terms of peer-reviewed academic research. However, since this paper is aimed at transport professionals primarily, a review of trade journals and other transport-related literature would add a valuable strand to these findings. Of the 237 review and other articles screened out for not being transport-related, many of these addressed landscape scale habitat connectivity. It is likely that some of these papers could provide valuable insights to the characteristics of habitat corridors which have not been picked up in this review.
To create a focus on temperate climatic zones, the review has screened out 184 articles which focussed on the movement of large mammals. However, it is appreciated that there are large mammals within temperate regions, and this review therefore under-represents these taxa. By extending this review to include larger mammals, more detail would be available for key sections. Whilst climate change impacts and invasive species were screened out due to a focus on habitat connectivity and space limitations, they do have relevance in discussions around transportation verge habitats. More detail on these two important subjects would provide transport practitioners with valuable insights into the propagation of unwanted species along transport corridors as well as the challenge of accommodating a changing climate into the assessment of functional connectivity.
It is also noted that search expression omitted some terms such as “mammal” and “wildlife” and, whilst mammals are still well represented in the dataset, further insights may be by using specific terms.
4.3 Discussion of next steps/future research
Section 3.3 identifies gaps and limitations identified within review papers, with suggestions for future research. In addition to these, the following points are also made which would help to map out next steps for successful implementation by transport professionals:
It is recommended that transportation infrastructure providers map out processes for promoting habitat connectivity across the network, and that methods and tools are developed to rapidly assess functional connectivity “hotspots” along the network at regional level for focal species and ecological communities, considering national habitat improvement/connectivity proposals. It is also recommended that the verge vegetation in “hotspot” areas is assessed for both functional connectivity and habitat potential and that this forms the baseline for decision making on vegetation recovery and future interventions.
It is recommended that an inventory of verge vegetation is made in functional connectivity “hotspot” areas which is managed alongside other infrastructure assets in a systematic manner, facilitating risk identification and assessment to both infrastructure and natural assets.
5 Conclusion
The literature review reveals that the extensive reach of transportation network verges can provide a vital resource for landscape scale habitat connectivity and biodiversity by functioning as both connecting corridors and remnant habitat. However, this potential is both under-exploited and not fully understood. When combined with a national and regional strategy for habitat networks (which includes restoration and creation of primary habitat), targeted investments in verge vegetation can maximise effectiveness with limited expenditure. Moreover, erges are particularly important in human altered environments such as urban and agricultural areas, for they provide refuges and habitat fragments which can assist in the functional connectivity of plants and animals across an otherwise hostile environment. Habitat improvements in these areas would significantly improve local biodiversity. Lastly, animal and plant physiology determines dispersal ability, which precludes a “one size fits all” approach to optimising the verge environment for dispersal. Interventions should therefore consider representative communities rather than individual species.
Data availability statement
The raw data supporting the conclusion of this article will be made available by the authors, without undue reservation.
Author contributions
NC: Conceptualization, Data curation, Formal Analysis, Investigation, Methodology, Writing–original draft. RF: Conceptualization, Writing–review and editing, Supervision. NS: Conceptualization, Writing–review and editing. EF: Conceptualization, Writing–review and editing, Funding acquisition, Project administration, Supervision. AQ: Conceptualization, Writing–review and editing, Funding acquisition, Project administration, Supervision.
Funding
The author(s) declare that financial support was received for the research, authorship, and/or publication of this article. This work was supported by the University of Birmingham and Network Rail Limited. EF acknowledges EPSRC Fellowship Award: EP/R007365/1.
Conflict of interest
Author NS was employed by Network Rail.
The remaining authors declare that the research was conducted in the absence of any commercial or financial relationships that could be construed as a potential conflict of interest.
Publisher’s note
All claims expressed in this article are solely those of the authors and do not necessarily represent those of their affiliated organizations, or those of the publisher, the editors and the reviewers. Any product that may be evaluated in this article, or claim that may be made by its manufacturer, is not guaranteed or endorsed by the publisher.
References
Amaral, K. E., Palace, M., Obrien, K. M., Fenderson, L. E., and Kovach, A. I. (2016). Anthropogenic habitats facilitate dispersal of an early successional obligate: implications for restoration of an endangered ecosystem. Figshare 11, e0148842. doi:10.1371/journal.pone.0148842
Apfelbaum, S., Rock, R., and Zoli, T. (2012). A simple structure supports a complex habitat in wildlife crossing design. Ecol. Restor. 30 (4), 341–344. doi:10.3368/er.30.4.341
Arenas, J. M., Escudero, A., Magro, S., Salaguer, L., and Casado, M. A. (2015). Woody colonization of road embankments: a large spatial scale survey in central Spain. Landsc. Urban Plan. 141, 52–58. doi:10.1016/j.landurbplan.2015.04.009
Ascensao, F., Kindel, A., Teixeira, F. Z., Barrientos, R., D'Amico, M., Borda-de-Agua, L., et al. (2019). Beware that the lack of wildlife mortality records can mask a serious impact of linear infrastructures. Glob. Ecol. Conservation 19, e00661. doi:10.1016/j.gecco.2019.e00661
Aurambout, J. P., Endress, A. G., and Deal, B. M. (2005). A spatial dynamic model to simulate population variations and movements within fragmented landscapes, international congress on modelling and simulation (MODSIM05). Melbourne, AUSTRALIA: MODSIM.
Avon, C., Berges, L., Dumas, Y., and Dupouey, J. L. (2010). Does the effect of forest roads extend a few meters or more into the adjacent forest? A study on understory plant diversity in managed oak stands. For. Ecol. Manag. 259 (8), 1546–1555. doi:10.1016/j.foreco.2010.01.031
Barrientos, R., Ascensao, F., Beja, P., Pereira, H. M., and Borda-de-Agua, L. (2019). Railway ecology vs. road ecology: similarities and differences Eur. J. Wildl. Res. 65 (1), 12. doi:10.1007/s10344-018-1248-0
Beatty, W. S., Beasley, J. C., and Rhodes, O. E. (2014). Habitat selection by a generalist mesopredator near its historical range boundary. Can. J. Zoology 92 (1), 41–48. doi:10.1139/cjz-2013-0225
Beier, P., Majka, D. R., and Spencer, W. D. (2008). Forks in the road: choices in procedures for designing wildland linkages. Conserv. Biol. 22 (4), 836–851. doi:10.1111/j.1523-1739.2008.00942.x
Bennett, V. J., Sparks, D. W., and Zollner, P. A. (2013). Modeling the indirect effects of road networks on the foraging activities of bats. Landsc. Ecol. 28 (5), 979–991. doi:10.1007/s10980-013-9874-0
Bennett, V. J., and Zurcher, A. A. (2013). When corridors collide: road-related disturbance in commuting bats. J. Wildl. Manag. 77 (1), 93–101. doi:10.1002/jwmg.467
Bochet, E., and Garcia-Fayos, P. (2015). Identifying plant traits: a key aspect for species selection in restoration of eroded roadsides in semiarid environments. Ecol. Eng. 83, 444–451. doi:10.1016/j.ecoleng.2015.06.019
Brundtland, G. H. (1987). Our common future—call for action. Environ. Conserv. 14 (4), 291–294. doi:10.1017/s0376892900016805
Brunzel, S., Elligsen, H., and Frankl, R. (2004). Distribution of the Cinnabar moth Tyria jacobaeae L. at landscape scale: use of linear landscape structures in egg laying on larval hostplant exposures. Landsc. Ecol. 19 (1), 21–27. doi:10.1023/b:land.0000018354.77405.0e
Butterweck, M. D., and Ce, C. E. (2000). Do corridors increase movements between forest patches? A study on between habitat migration of ground beetles (Coleoptera: carabidae), Workshop on Ecological Corridors for Invertebrates. Switzerland: Neuchatel.
Cervinka, J., Riegert, J., Grill, S., and Salek, M. (2015). Large-scale evaluation of carnivore road mortality: the effect of landscape and local scale characteristics. Mammal Res. 60 (3), 233–243. doi:10.1007/s13364-015-0226-0
Chang, Y.-H., Wu, B.-Y., and Lu, H.-L. (2014). Using ecological barriers for the conservation of frogs along roads. Ecol. Eng. 73, 102–108. doi:10.1016/j.ecoleng.2014.09.009
Clevenger, A. P., Chruszcz, B., and Gunson, K. (2001). Drainage culverts as habitat linkages and factors affecting passage by mammals. J. Appl. Ecol. 38 (6), 1340–1349. doi:10.1046/j.0021-8901.2001.00678.x
Colley, M., Lougheed, S. C., Otterbein, K., and Litzgus, J. D. (2017). Mitigation reduces road mortality of a threatened rattlesnake. Wildl. Res. 44 (1), 48–59. doi:10.1071/wr16130
Crick, H. Q. P., Crosher, I. E., Mainstone, C. P., Taylor, S. D., Wharton, A., Langford, P., et al. (2020). Nature networks evidence handbook. USA: Natural England.
Cserkesz, T., Ottlecz, B., Cserkesz-Nagy, A., and Farkas, J. (2013). Interchange as the main factor determining wildlife-vehicle collision hotspots on the fenced highways: spatial analysis and applications. Eur. J. Wildl. Res. 59 (4), 587–597. doi:10.1007/s10344-013-0710-2
Daniel-Ferreira, J., Berggren, A., Wissman, J., and Ockinger, E. (2022). Road verges are corridors and roads barriers for the movement of flower-visiting insects. Ecography 2022 (2). doi:10.1111/ecog.05847
Davis, J. D., Debinski, D. M., and Danielson, B. J. (2007). Local and landscape effects on the butterfly community in fragmented Midwest USA prairie habitats. Landsc. Ecol. 22 (9), 1341–1354. doi:10.1007/s10980-007-9111-9
Dawson, D. (1991). Are habitat corridors conduits for animals and plants in a fragmented landscape? A review of the scientific evidence. England: ENRR094 England.
Dean, W. R. J., Seymour, C. L., and Joseph, G. S. (2018). Linear structures in the Karoo, South Africa, and their impacts on biota. Afr. J. Range Forage Sci. 35 (3-4), 223–232. doi:10.2989/10220119.2018.1514530
de la Riva, E. G., Casado, M. A., Jimenez, M. D., Mola, I., Costa-Tenorio, M., and Balaguer, L. (2011). Rates of local colonization and extinction reveal different plant community assembly mechanisms on road verges in central Spain. J. Veg. Sci. 22 (2), 292–302. doi:10.1111/j.1654-1103.2010.01248.x
Denneboom, D., Bar-Massada, A., and Shwartz, A. (2021). Factors affecting usage of crossing structures by wildlife-A systematic review and meta-analysis. Sci. Total Environ., 777. doi:10.1016/j.scitotenv.2021.146061
DfT (2022). Road lengths in great britain: 2021. Available at: https://www.gov.uk/government/statistics/road-lengths-in-great-britain-2021/road-lengths-in-great-britain-2021.
Do, Y., Kim, J. Y., Kim, H. W., and Joo, G.-J. (2017). Distribution of Carabid beetles within wildlife corridors connecting fragmented forests. Landsc. Ecol. Eng. 13 (2), 279–286. doi:10.1007/s11355-016-0319-1
Doncaster, C. P., Rondinini, C., and Johnson, P. C. D. (2001). Field test for environmental correlates of dispersal in hedgehogs Erinaceus europaeus. J. Animal Ecol. 70 (1), 33–46. doi:10.1046/j.1365-2656.2001.00471.x
Driscoll, D. A. (2004). Extinction and outbreaks accompany fragmentation of a reptile community. Ecol. Appl. 14 (1), 220–240. doi:10.1890/02-5248
Dylewski, L., and Tobolka, M. (2022). Unused railway lines as a contributor to bird abundance, species richness and diversity in intensively managed farmland. Agric. Ecosyst. Environ., 326. doi:10.1016/j.agee.2021.107820
Elo, M., Ketola, T., and Komonen, A. (2021). Species co-occurrence networks of ground beetles in managed grasslands. Community Ecol. 22 (1), 29–40. doi:10.1007/s42974-020-00034-3
Environment Agency (2023). LIDAR Vegetation Object Model (VOM). Available at: https://www.data.gov.uk/dataset/227ab487-e8f2-4cbb-b26a-9e6d3b662265/lidar-vegetation-object-model-vom#licence-info.
Frey, S. N., and Conover, M. R. (2006). Habitat use by meso-predators in a corridor environment. J. Wildl. Manag. 70 (4), 1111–1118. doi:10.2193/0022-541x(2006)70[1111:hubmia]2.0.co;2
Friebe, K., Steffens, T., Schulz, B., Valqui, J., Reck, H., and Hartl, G. (2018). The significance of major roads as barriers and their roadside habitats as potential corridors for hazel dormouse migration - a population genetic study. Folia Zool. 67 (2), 98–109. doi:10.25225/fozo.v67.i2.a10.2018
Gardiner, M. M., Riley, C. B., Bommarco, R., and Ockinger, E. (2018). Rights-of-way: a potential conservation resource. Front. Ecol. Environ. 16 (3), 149–158. doi:10.1002/fee.1778
Grilo, C., Ascensao, F., Santos-Reis, M., and Bissonette, J. A. (2011). Do well-connected landscapes promote road-related mortality? Eur. J. Wildl. Res. 57 (4), 707–716. doi:10.1007/s10344-010-0478-6
Gunson, K. E., Mountrakis, G., and Quackenbush, L. J. (2011). Spatial wildlife-vehicle collision models: a review of current work and its application to transportation mitigation projects. J. Environ. Manag. 92 (4), 1074–1082. doi:10.1016/j.jenvman.2010.11.027
Halbritter, D. A., Daniels, J. C., Whitaker, D. C., and Huang, L. (2015). Reducing mowing frequency increases floral resource and butterfly (Lepidoptera: hesperioidea and Papilionoidea) abundance in managed roadside margins. Fla. Entomol. 98 (4), 1081–1092. doi:10.1653/024.098.0412
Harris, L. D. (1984). The fragmented forest: island biogeography Theory and the Preservation of biotic Diversity. Chicago, United States: University of Chicago Press.
Hayrol, A. M. S., Ahmad, N., Samsul, F. S., Asnarulkhadi, A. S., and Mas, E. H. (2020). Systematic literature review on adaptation towards climate change impacts among indigenous people in the Asia Pacific regions. J. Clean. Prod. 258, 120595. doi:10.1016/j.jclepro.2020.120595
Henry, R. C., Palmer, S. C. F., Watts, K., Mitchell, R. J., Atkinson, N., and Travis, J. M. J. (2017). Tree loss impacts on ecological connectivity: developing models for assessment. Ecol. Inf. 42, 90–99. doi:10.1016/j.ecoinf.2017.10.010
Holderegger, R., and Di Giulio, M. (2010). The genetic effects of roads: a review of empirical evidence. Basic Appl. Ecol. 11 (6), 522–531. doi:10.1016/j.baae.2010.06.006
HS2 (2020). Green corridor prospectus. Available at: https://www.hs2.org.uk/about-us/our-documents/green-corridor-prospectus/.
Jones, D. N., and Pickvance, J. (2013). FOREST BIRDS USE VEGETATED FAUNA OVERPASS TO CROSS MULTI-LANE ROAD. Oecologia Aust. 17 (1), 147–156. doi:10.4257/oeco.2013.1701.12
Kalcounis-Rueppell, M. C., Briones, K. M., Homyack, J. A., Petric, R., Marshall, M. M., and Miller, D. A. (2013). Hard forest edges act as conduits, not filters, for bats. Wildl. Soc. Bull. 37 (3), 571–576. doi:10.1002/wsb.289
Knapp, M., Saska, P., Knappova, J., Vonicka, P., Moravec, P., Kurka, A., et al. (2013). The habitat-specific effects of highway proximity on ground-dwelling arthropods: implications for biodiversity conservation. Biol. Conserv. 164, 22–29. doi:10.1016/j.biocon.2013.04.012
Knight, S. M., Norris, D. R., Derbyshire, R., and Flockhart, D. T. T. (2019). Strategic mowing of roadside milkweeds increases monarch butterfly oviposition. Glob. Ecol. Conservation 19, e00678. doi:10.1016/j.gecco.2019.e00678
Koivula, M. J. (2005). Effects of forest roads on spatial distribution of boreal carabid beetles (Coleoptera: carabidae). Coleopt. Bull. 59 (4), 465–487. doi:10.1649/815.1
Kuykendall, M. T., and Keller, G. S. (2011). IMPACTS OF ROADS AND CORRIDORS ON ABUNDANCE AND MOVEMENT OF SMALL MAMMALS ON THE LLANO ESTACADO OF TEXAS. Southwest. Nat. 56 (1), 9–16. doi:10.1894/clg-33.1
Laidlaw, K., Broadbent, E., and Eby, S. (2021). Effectiveness of aerial wildlife crossings: do wildlife use rope bridges more than hazardous structures to cross roads? Rev. De. Biol. Trop. 69 (3), 1138–1148. doi:10.15517/rbt.v69i3.47098
Lazaro-Lobo, A., and Ervin, G. N. (2019). A global examination on the differential impacts of roadsides on native vs. exotic and weedy plant species Glob. Ecol. Conservation 17, e00555. doi:10.1016/j.gecco.2019.e00555
Le Viol, I., Julliard, R., Kerbiriou, C., de Redon, L., Carnino, N., Machon, N., et al. (2008). Plant and spider communities benefit differently from the presence of planted hedgerows in highway verges. Biol. Conserv. 141 (6), 1581–1590. doi:10.1016/j.biocon.2008.04.003
Maclagan, S. J., Coates, T., Hradsky, B. A., Butryn, R., and Ritchie, E. G. (2020). Life in linear habitats: the movement ecology of an endangered mammal in a peri-urban landscape. Anim. Conserv. 23 (3), 260–272. doi:10.1111/acv.12533
Mammides, C., Kadis, C., and Coulson, T. (2015). The effects of road networks and habitat heterogeneity on the species richness of birds in Natura 2000 sites in Cyprus. Landsc. Ecol. 30 (1), 67–75. doi:10.1007/s10980-014-0100-5
Martinig, A. R., and McLaren, A. A. D. (2019). Vegetated highway medians as foraging habitat for small mammals. Wildl. Soc. Bull. 43 (2), 317–322. doi:10.1002/wsb.967
Marston, C., Rowland, C. S., O’Neil, A. W., and Morton, R. D. (2022). UKCEH (2021). Land Cover Map (LCM2021) (land parcels, GB). NERC EDS Environmental Information Data Centre. Available at: https://doi.org/10.5285/398dd41e-3c08-47f5-811f-da990007643f.
McDonald, W., and St Clair, C. C. (2004). Elements that promote highway crossing structure use by small mammals in Banff National Park. J. Appl. Ecol. 41 (1), 82–93. doi:10.1111/j.1365-2664.2004.00877.x
McGregor, M. E., Wilson, S. K., and Jones, D. N. (2015). Vegetated fauna overpass enhances habitat connectivity for forest dwelling herpetofauna. Glob. Ecol. Conservation 4, 221–231. doi:10.1016/j.gecco.2015.07.002
Milton, S. J., Dean, W. R. J., Sielecki, L. E., and van der Ree, R. (2015). The function and management of roadside vegetation.
Moron, D., Skorka, P., Lenda, M., Celary, W., and Tryjanowski, P. (2017). Railway lines affect spatial turnover of pollinator communities in an agricultural landscape. Divers. Distributions 23 (9), 1090–1097. doi:10.1111/ddi.12600
Mulligan, M. P., Schooley, R. L., and Ward, M. P. (2013). Effects of connectivity and regional dynamics on restoration of small mammal communities in midwestern grasslands. Restor. Ecol. 21 (6), 678–685. doi:10.1111/rec.12039
National_Highways (2023a). A30 Chiverton to Carland cross. Available at: https://nationalhighways.co.uk/our-roads/south-west/a30-chiverton-to-carland-cross/.
Nevrelova, M., Lehotska, B., and Ruzickova, J. (2022). Methodology of wildlife underpasses attractiveness assessment. Ekol. Bratisl. 41 (2), 172–182. doi:10.2478/eko-2022-0018
New, T. R., Sands, D. P. A., and Taylor, G. S. (2021). Roles of roadside vegetation in insect conservation in Australia. Austral Entomol. 60 (1), 128–137. doi:10.1111/aen.12511
Ng, S. J., Dole, J. W., Sauvajot, R. M., Riley, S. P. D., and Valone, T. J. (2004). Use of highway undercrossings by wildlife in southern California. Biol. Conserv. 115 (3), 499–507. doi:10.1016/s0006-3207(03)00166-6
Nishikawa-Pacher, A. (2022). Research questions with PICO: a universal mnemonic. Publications 10 (3), 21. doi:10.3390/publications10030021
Noordijk, J., Schaffers, A. P., Heijerman, T., and Sykora, K. V. (2011). Using movement and habitat corridors to improve the connectivity for heathland carabid beetles. J. Nat. Conservation 19 (5), 276–284. doi:10.1016/j.jnc.2011.05.001
Okoli, C., and Schabram, K. (2015). A guide to conducting a standalone systematic literature review. Commun. Assoc. Inf. Syst. 37 (1), 879–910. doi:10.17705/1cais.03743
Ortega, C. P. (2012). Effects of noise pollution on birds: a brief review of our knowledge. Ornithol. Monogr. 74, 6–22.
Ouedraogo, D.-Y., Villemey, A., Vanpeene, S., Coulon, A., Azambourg, V., Hulard, M., et al. (2020). Can linear transportation infrastructure verges constitute a habitat and/or a corridor for vertebrates in temperate ecosystems? A systematic review. Environ. Evid. 9 (1), 13. doi:10.1186/s13750-020-00196-7
Parile, E., Piccini, I., and Bonelli, S. (2021). A demographic and ecological study of an Italian population of Polyommatus ripartii: the ESU Polyommatus exuberans. J. Insect Conservation 25 (5-6), 783–796. doi:10.1007/s10841-021-00344-5
Phillips, B. B., Wallace, C., Roberts, B. R., Whitehouse, A. T., Gaston, K. J., Bullock, J. M., et al. (2020). Enhancing road verges to aid pollinator conservation: a review. Biol. Conserv. 250, 108687. doi:10.1016/j.biocon.2020.108687
Pickering, C., and Byrne, J. (2014). The benefits of publishing systematic quantitative literature reviews for PhD candidates and other early-career researchers. High. Educ. Res. Dev. 33 (3), 534–548. doi:10.1080/07294360.2013.841651
Prunier, J. G., Kaufmann, B., Lena, J.-P., Fenet, S., Pompanon, F., and Joly, P. (2014). A 40-year-old divided highway does not prevent gene flow in the alpine newt Ichthyosaura alpestris. Conserv. Genet. 15 (2), 453–468. doi:10.1007/s10592-013-0553-0
Ranta, P., Kesulahti, J., Tanskanen, A., Viljanen, V., and Virtanen, T. (2015). Roadside and riverside green - urban corridors in the city of Vantaa, Finland. Urban Ecosyst. 18 (2), 341–354. doi:10.1007/s11252-014-0402-z
Redon, L., Viol, I. L., Jiguet, F., Machon, N., Scher, O., and Kerbiriou, C. (2015). Road network in an agrarian landscape: potential habitat, corridor or barrier for small mammals? Acta Oecologica-International J. Ecol. 62, 58–65. doi:10.1016/j.actao.2014.12.003
Ries, L., and Debinski, D. M. (2001). Butterfly responses to habitat edges in the highly fragmented prairies of Central Iowa. J. Animal Ecol. 70 (5), 840–852. doi:10.1046/j.0021-8790.2001.00546.x
Ries, L., Debinski, D. M., and Wieland, M. L. (2001). Conservation value of roadside prairie restoration to butterfly communities. Conserv. Biol. 15 (2), 401–411. doi:10.1046/j.1523-1739.2001.015002401.x
Roques, L., and Bonnefon, O. (2016). Modelling population dynamics in realistic landscapes with linear elements: a mechanistic-statistical reaction-diffusion approach. Figshare 11, e0151217. doi:10.1371/journal.pone.0151217
Roy, V., and de Blois, S. (2006). Using functional traits to assess the role of hedgerow corridors as environmental filters for forest herbs. Biol. Conserv. 130 (4), 592–603. doi:10.1016/j.biocon.2006.01.022
Ruiz, M. D. M., Ramsden, D., Roper, S., Cresswell, B., and Skuse, J. (2021). Juvenile Barn Owl Tyto alba dispersal: a radio-tracking study of roost site selection in relation to landscape features. Bird. Study 68 (2), 245–257. doi:10.1080/00063657.2021.2021141
Rycken, S. J. E., Warren, K. S., Yeap, L., Donaldson, R., Mawson, P., Dawson, R., et al. (2022). Forest specialist species in the urban landscape: do different levels of urbanization affect the movements of Forest Red-tailed Black Cockatoos (Calyptorhynchus banksii naso)? Avian Conservation Ecol. 17 (1), art11. doi:10.5751/ace-02061-170111
Saarinen, K., Valtonen, A., Jantunen, J., and Saarnio, S. (2005). Butterflies and diurnal moths along road verges: does road type affect diversity and abundance? Biol. Conserv. 123 (3), 403–412. doi:10.1016/j.biocon.2004.12.012
Sawaya, M. A., Clevenger, A. P., and Schwartz, M. K. (2019). Demographic fragmentation of a protected wolverine population bisected by a major transportation corridor. Biol. Conserv. 236, 616–625. doi:10.1016/j.biocon.2019.06.030
Schaffers, A. P., Raemakers, I. P., and Sykora, K. V. (2012). Successful overwintering of arthropods in roadside verges. J. Insect Conservation 16 (4), 511–522. doi:10.1007/s10841-011-9437-0
Shier, D. M., Lea, A. J., and Owen, M. A. (2012). Beyond masking: endangered Stephen's kangaroo rats respond to traffic noise with footdrumming. Biol. Conserv. 150 (1), 53–58. doi:10.1016/j.biocon.2012.03.007
Steidle, J. L. M., Kimmich, T., Csader, M., and Betz, O. (2022). Negative impact of roadside mowing on arthropod fauna and its reduction with 'arthropod-friendly' mowing technique. J. Appl. Entomology 146 (5), 465–472. doi:10.1111/jen.12976
Suarez-Esteban, A., Fahrig, L., Delibes, M., and Fedriani, J. M. (2016). Can anthropogenic linear gaps increase plant abundance and diversity? Landsc. Ecol. 31 (4), 721–729. doi:10.1007/s10980-015-0329-7
Sultana, S., Salon, D., and Kuby, M. (2019). Transportation sustainability in the urban context: a comprehensive review. Urban Geogr. 40 (3), 279–308. doi:10.1080/02723638.2017.1395635
Taylor, B. D., and Goldingay, R. L. (2014). Use of highway underpasses by bandicoots over a 7-year period that encompassed road widening. Aust. Mammal. 36 (2), 178–183. doi:10.1071/am13034
Thomson, A., Graham, C., Toohey, K., Kennelly, M., Burton, P., and Fredline, L. (2019). Sport event legacy: a systematic quantitative review of literature. Sport Manag. Rev. 22 (3), 295–321. doi:10.1016/j.smr.2018.06.011
Tikka, P. M., Hogmander, H., and Koski, P. S. (2001). Road and railway verges serve as dispersal corridors for grassland plants. Landsc. Ecol. 16 (7), 659–666. doi:10.1023/a:1013120529382
Timm, B. C., and McGarigal, K. (2014). Fowler's toad (Anaxyrus fowleri) activity patterns on a roadway at cape cod national seashore. J. Herpetology 48 (1), 111–116. doi:10.1670/12-202
Travers, E., Haerdtle, W., and Matthies, D. (2021). Corridors as a tool for linking habitats ? Shortcomings and perspectives for plant conservation. J. Nat. Conservation 60, 125974. doi:10.1016/j.jnc.2021.125974
Tremblay, M. A., and St Clair, C. C. (2009). Factors affecting the permeability of transportation and riparian corridors to the movements of songbirds in an urban landscape. J. Appl. Ecol. 46 (6), 1314–1322. doi:10.1111/j.1365-2664.2009.01717.x
Tremblay, M. A., and St Clair, C. C. (2011). Permeability of a heterogeneous urban landscape to the movements of forest songbirds. J. Appl. Ecol. 48 (3), 679–688. doi:10.1111/j.1365-2664.2011.01978.x
Trombulak, S. C., and Frissell, C. A. (2000). Review of ecological effects of roads on terrestrial and aquatic communities. Conserv. Biol. 14 (1), 18–30. doi:10.1046/j.1523-1739.2000.99084.x
UIC (2021). Future vegetation control of European railways - state-of-the-art report (TRISTRAM final report).
Valtonen, A., and Saarinen, K. (2005). A highway intersection as an alternative habitat for a meadow butterfly: effect of mowing, habitat geometry and roads on the ringlet (Aphantopus hyperantus) Ann. Zool. Fenn. 42 (5), 545–556. doi:10.1016/j.biocon.2004.12.012
Vandevelde, J.-C., Bouhours, A., Julien, J.-F., Couvet, D., and Kerbiriou, C. (2014). Activity of European common bats along railway verges. Ecol. Eng. 64, 49–56. doi:10.1016/j.ecoleng.2013.12.025
Van Rossum, F. (2009). Pollen dispersal and genetic variation in an early-successional forest herb in a peri-urban forest. Plant Biol. 11 (5), 725–737. doi:10.1111/j.1438-8677.2008.00176.x
Villemey, A., Jeusset, A., Vargac, M., Bertheau, Y., Coulon, A., Touroult, J., et al. (2018). Can linear transportation infrastructure verges constitute a habitat and/or a corridor for insects in temperate landscapes? A systematic review. Environ. Evid. 7 (1), 5. doi:10.1186/s13750-018-0117-3
Voigt, C. C., Scholl, J. M., Bauer, J., Teige, T., Yovel, Y., Kramer-Schadt, S., et al. (2020). Movement responses of common noctule bats to the illuminated urban landscape. Landsc. Ecol. 35 (1), 189–201. doi:10.1007/s10980-019-00942-4
Weber, T. C., and Allen, W. L. (2010). Beyond on-site mitigation: an integrated, multi-scale approach to environmental mitigation and stewardship for transportation projects. Landsc. Urban Plan. 96 (4), 240–256. doi:10.1016/j.landurbplan.2010.04.003
Williams, J. R., Driscoll, D. A., and Bull, C. M. (2012). Roadside connectivity does not increase reptile abundance or richness in a fragmented mallee landscape. Austral Ecol. 37 (3), 383–391. doi:10.1111/j.1442-9993.2011.02292.x
Wilson, A., Fenton, B., Malloch, G., Boag, B., Hubbard, S., and Begg, G. (2016). Urbanisation versus agriculture: a comparison of local genetic diversity and gene flow between wood mouse Apodemus sylvaticus populations in human-modified landscapes. Ecography 39 (1), 87–97. doi:10.1111/ecog.01297
Wilson, R. F., Marsh, H., and Winter, J. (2007). Importance of canopy connectivity for home range and movements of the rainforest arboreal ringtail possum (Hemibelideus lemuroides). Wildl. Res. 34 (3), 177–184. doi:10.1071/wr06114
Wistrom, B., and Nielsen, A. B. (2016). Decisive environmental characteristics for woody regrowth in forest edges - patterns along complex environmental gradients in Southern Sweden. For. Ecol. Manag. 363, 47–62. doi:10.1016/j.foreco.2015.12.025
Keywords: sustainable development, environmental value, transportation infrastructure, vegetation management, biodiversity, habitat networks
Citation: Cork NA, Fisher RS, Strong N, Ferranti EJS and Quinn AD (2024) A systematic review of factors influencing habitat connectivity and biodiversity along road and rail routes in temperate zones. Front. Environ. Sci. 12:1369072. doi: 10.3389/fenvs.2024.1369072
Received: 11 January 2024; Accepted: 26 February 2024;
Published: 12 March 2024.
Edited by:
Eliane Ceccon, National Autonomous University of Mexico, MexicoReviewed by:
Thomas Göttert, Eberswalde University for Sustainable Development, GermanyFrancesco Bisi, University of Insubria, Italy
Copyright © 2024 Cork, Fisher, Strong, Ferranti and Quinn. This is an open-access article distributed under the terms of the Creative Commons Attribution License (CC BY). The use, distribution or reproduction in other forums is permitted, provided the original author(s) and the copyright owner(s) are credited and that the original publication in this journal is cited, in accordance with accepted academic practice. No use, distribution or reproduction is permitted which does not comply with these terms.
*Correspondence: Nicholas A. Cork, nac287@student.bham.ac.uk
†These authors have contributed equally to this work and share senior authorship