- College of Resources and Environment, Shanxi Agricultural University, Taigu, Shanxi, China
Public concern about polycyclic aromatic hydrocarbons (PAHs) is rising due to their potential carcinogenic, teratogenic, and mutagenic effects. This study assessed PAHs in Taiyuan City’s plain and mountain soil, investigating concentrations, distribution, sources, and carcinogenic risk. Σ21PAHs concentrations in plain topsoil ranged from 133.2 to 6,410.6 ng/g (mean 1,444.7 ng/g), and in mountain soil from 66.5 to 2,250.2 ng/g (mean 585.5 ng/g). Approximately 55.1% of plain and 19.0% of mountain soil samples had contamination levels exceeding 600 ng/g. In plain soil, 4-ring and 5-ring PAHs dominated, while 2-ring and 3-ring PAHs were prevalent in mountain soil. Polluted areas in Taiyuan were primarily centered in the central-north, with higher content closer to industrial or business districts. PAH isomer ratios and principal component analysis/multiple linear regression (PCA/MLR) indicated coal combustion as the main PAH source, followed by coke production, vehicle emissions, and biomass combustion. Incremental lifetime cancer risks (ILCRs) showed Taiyuan’s PAH-related cancer risks were generally low, though heavily contaminated areas exhibited moderate risks. Plain regions had three times higher cancer risk than mountains, with children facing higher risk than adults. These findings highlight the need to consider PAH pollution while enhancing urban environmental quality.
1 Introduction
In recent years, with the use of fossil fuels leading to adverse health effects, there has been growing public concern over deteriorating environmental problems, especially in urban areas, which are the main places where people work and live (Yan et al., 2019). Polycyclic aromatic hydrocarbons (PAHs) are ubiquitous organic pollutants and can lead to environmental problems due to their carcinogenic and mutagenic potential (Liu et al., 2021; Singh et al., 2021; Chen et al., 2022); in particular, PAHs lead to severe cardiovascular diseases in humans with long exposure (Xu et al., 2010; Bortey-Sam et al., 2017; Mirzababaei et al., 2021). PAHs are a group of organic compounds that contain at least two benzene rings with a fused structure, and sixteen PAHs have been classified as priority pollutants by the Environmental Protection Agency in the United States (USEPA) (Qu et al., 2020). Based on their molecular weights, PAHs can be further reclassified as low-molecular-weight PAHs (LMW, 2–3 rings) with noncarcinogenic properties, and high-molecular-weight PAHs (HMW, >4 rings), including seven carcinogenic PAHs according to the USEPA (Jia et al., 2021). In the environment, PAHs are derived mainly from the thermal alteration or incomplete combustion of organic carbon (C) and are widely found in various media, such as air, water, soil and food (Menzie et al., 1992; Duan et al., 2015; Liu et al., 2019).
Due to their volatility and semivolatility, PAHs may be transported far and accumulate in the environment through wet or dry deposition, especially in soils (Totten et al., 2004; Melymuk et al., 2012). The deposition of PAHs is influenced by the photochemical characteristics, atmospheric concentration, meteorological conditions, topography and emission density (Xing et al., 2016). One past report showed that nearly 90% of the total PAHs remained in the soil after being absorbed by soil organic matter (Choi et al., 2009). Considering their special characteristics, LMW PAHs are more likely to be reduced through volatilization, leaching, and degradation (Yan et al., 2019). HMW PAHs, with their heavy molecular weights, have strong accumulation characteristics, and over time, seven carcinogenic PAHs have shown significant growth trends (Lang et al., 2008). Moreover, due to multimedia transfer, contaminated soils can contaminate foods, leading to threats to ecosystems and human health (Duan et al., 2015). Therefore, many investigations aiming to identify PAHs distribution and assess their environmental effects in farmlands and mega-cities have been conducted (Devi et al., 2016; Liu et al., 2019).
Coal combustion and coke production are important sources of PAHs (Shen et al., 2013). Previous studies have shown that Shanxi Province in China is the largest coke production region in the world, and its provincial capital is Taiyuan city, which is one of the energy industry centers in China and has become the most polluted city in the nation (Xu et al., 2006; Duan et al., 2015; Yong et al., 2015; Mu et al., 2021; Wu et al., 2023). According to the Taiyuan statistical yearbook (TBS, 2019), the residential population was 3.888 million people in 2020 and the gross domestic product (GDP) was 415.3 billion CNY. The value added by the secondary industry, mainly including the coal, coking, and chemical industries, building materials, metallurgy and electric power, was 150.4 billion CNY, contributing 36.2% to the GDP. Moreover, Taiyuan City consumed 64.8 million tons of standard coal for industrial use, accounting for 38.1% of the total use of Shanxi Province, and approximately 89.6% of this industrial energy came from coal. Moreover, 80.8% of the coal was used for combustion by industrial production and thermal power, while 19.2% was used for coke production. Extensive coal combustion and coke production have caused serious environmental problems, especially PAHs contamination (Li H. et al., 2016).
In addition, influenced by the terrain, which is a basin surrounded by mountains in the eastern and western directions with a wide intermediary plain area, most the industrial and population activities are distributed in the plain area, leading to heterogeneity in the distribution of pollutants such as heavy metals and persistent organic pollutants (POPs) (Fu et al., 2009; Yong et al., 2015). However, information on soil contamination by PAHs in different the landforms of this region is limited. Therefore, this study investigated the background concentrations of PAHs in soil samples collected in the plain and mountainous areas of Taiyuan city. The specific objectives were 1) to compare the concentrations, compositions and sources of PAHs in plain and mountainous soils; 2) to determine the characteristics of the spatial heterogeneity distribution of PAHs in Taiyuan by ordinary kriging interpolation; and 3) to identify the potential risk associated with PAHs in the plain and mountainous regions using an incremental lifetime cancer risk (ILCR) assessment.
2 Materials and methods
2.1 Study area and site description
Taiyuan is in the northern part of China and on the eastern edge of the loess hilly region, and it belongs to the continental climate of the northern temperate zone. It is hot and rainy in the summer and cold and dry in the winter. The regional profile is bat-shaped, with hills surrounding the area in the west and east, while the southern area is a valley plain; the average elevation ranges from 800 to 900 m (Figure 1B). The annual mean temperature and precipitation are 9.5°C and 456 mm, respectively.
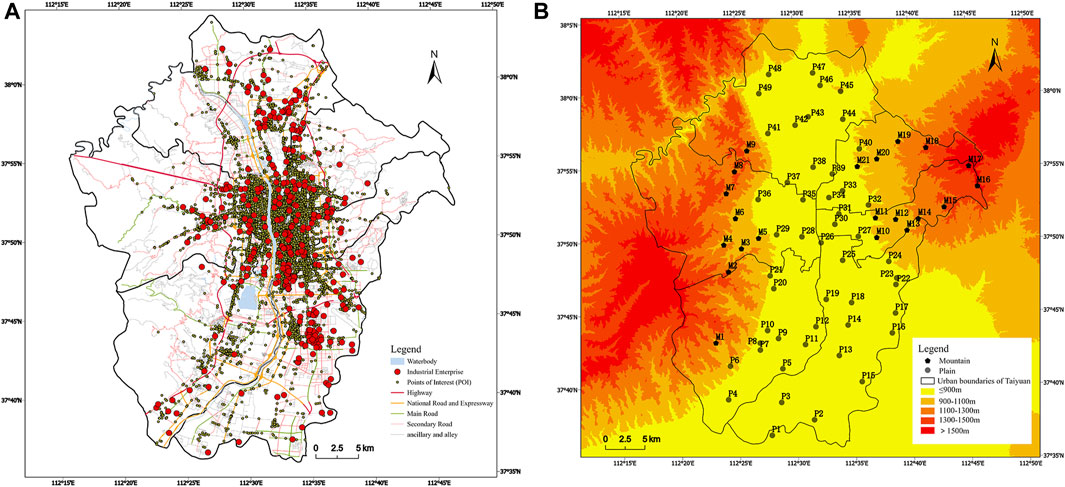
FIGURE 1. Study area anthropogenic activity (A) and soil sampling sites across various landforms (B).
According to research on the topography of Taiyuan city (Liang et al., 2000), plains and mountains were classified by an elevation of 900 m with SRTM-DEM (https://engine.piesat.cn/dataset-list) data. Forty-nine topsoil samples (obtained at 0–10-cm depths) from the plain area and 21 topsoil samples (at 0–10-cm depths) from the mountainous area were collected in July 2021 using wooden spades (Figure 1b). All sites were at least 50 m away from pollution sources and main roads, without anthropocentric interference activities. At each site, five topsoil subsamples were collected within a 5 m × 5 m (20 m × 20 m) grid and mixed to yield a mixed soil sample of approximately 500-1000 g. Then, one-quarter of each soil sample was collected, stored in a polyethylene bag, transported back to the laboratory to remove debris such as gravel and root debris, air-dried at room temperature and screened with a 70-mesh sieve (Tao et al., 2011; Duan et al., 2015). The treatment samples were mixed and reserved in clean brown bottles (Duan et al., 2015).
2.2 Laboratory analysis and quality control
Eight grams of each soil sample were weighed into a perfluoroalkoxy polymer extraction vessel with a Teflon-sealed, lip-tight cap and a polyetheretherketone liner. A microwave-accelerated system (MARS 240/50, CEM, United States) was used to extract PAHs from the soil samples using 20 mL of a hexane/acetone mixture (1:1, v/v) at 1200 W (100%). The extraction temperature was programmed to 110°C for 10 min and then held for an additional 10 min. After extraction, the soil and solvent were separated by filtration, and the solvent was decanted into a pear-shaped flask. The extracts were then concentrated to approximately 1 mL using a rotary vacuum evaporator (EYEL4, Japan) and transferred to a silica/alumina gel column (43 cm in length with 10 mm i.d.) filled (from bottom to top) with 12 cm silica gel, 12 cm alumina, and 1 cm anhydrous sodium sulfate for cleanup (Shen et al., 2012b). The column was first eluted with 20 mL hexane and then with 70 mL hexane/dichloromethane (1:1, v/v). The latter eluate was further concentrated to 1 mL and spiked with Nap-d8, Ant-d10, Ace-d10, Chr-d12, and Perylene-d12 (J&W Chemical Ltd., United States) for the chromatography analysis.
PAHs were measured using a gas chromatograph (GC 6890, Agilent, United States) connected to a mass spectrometer (MS5973, Agilent, United States) in electron ionization mode. An HP-5MS capillary column (30 m × 0.25 mm×0.25 μm) was used, and the oven temperature was held at 50°C for 1 min, increased to 150°C at a rate of 10°C/min, increased again to 240°C at a rate of 3°C/min, and then increased finally to 280°C for another 20 min. Helium was used as the carrier gas. Twenty-one parent PAHs were measured in this study, including naphthalene (NAP), acenaphthylene (ACY), acenaphthene (ACE), fluorene (FLO), phenanthrene (PHE), anthracene (ANT), fluoranthene (FLA), pyrene (PYR), retene (RET), cyclopenta [c,d]pyrene (CPP), benzo(a)anthracene (BaA), chrysene (CHR), benzo(b)fluoranthene (BbF), benzo(k)fluoranthene (BkF), benzo(a)pyrene (BaP), benzo(e)pyrene (BeP), perylene (PER), dibenz (a,h)anthracene (DahA), indeno (l, 2,3-cd)pyrene (IcdP), benzo (g,h,i)perylene (BghiP), and coronene (COR). The PAHs were identified by their retention time and qualifying ions from standards in selected ion-monitoring mode.
All glassware was cleaned in an ultrasonic cleaner and baked at 500°C for at least 10 h. The silica and alumina used were prebaked at 450°C for 4 h and reactivated at 130°C for 16 h prior to use. The solvents were purified by redistillation and checked for blanks. For each extraction, laboratory blanks were analyzed. Two field blanks (samplers transported to and from the field without significant exposure) were analyzed alongside the actual samples collected from different landforms.Procedural blanks were measured, and the PAHs concentration in the blanks were subtracted from the samples. Instrumental detection limits defined as 3 times the signal-to-noise ratio were established for the PAHs and ranged from 0.13 ng/g (ACY) to 0.92 ng/g (BghiP). Preliminary recovery tests showed that the recoveries of spiked PAHs standard ranged from 82% to 114%. The results of the reported PAHs were not corrected by the recoveries. The recoveries of the surrogates (2-fluoro-1,1-biphenyl and p-terphenyl-d14) added into 15 samples randomly selected before the extraction process were 81% ± 12% (64%–103% range) and 84% ± 25% (62%–137%) for 2-fluoro-1,1-biphenyl and p-terphenyl-d14, respectively. The statistical analysis was performed using SPSS with a significance level of 0.05. The spatial distributions of the PAHs contents were mapped with ArcGIS Pro software.
2.3 Human health risk assessment
The estimated incremental lifetime cancer risk (ILCR) metric of the United States Environmental Protection Agency (USEPA) was used to assess the potential health risks caused by PAHs in the soils in Taiyuan. Ingestion, dermal, and inhalation ILCR pathways were calculated using the following equations:
where CS is the ΣBaPeq concentration (mg/kg) with 21 PAHs; the individual BaPeq values were calculated by multiplying the measured component concentrations with the corresponding toxicity equivalent factors (TEFs, see Supplementary Table S7). CSFingestion, CSFdermal, and CSFinhalation are the carcinogenic slope factors of oral intake, skin contact and breath intake, with values of 7.3, 25, and 3.85, respectively (mg/kg/d) (Jia et al., 2021); BW is body weight (kg); AT is an average life (y); EF is the exposure frequency (d/y); ED is the exposure time (y); IRinhalation is the inhalation rate (m3/d); IRingestion is the soil intake rate (mg/d); SA is the skin exposure area (cm2); AF is the skin adhesion factor (mg/(cm2∙h)); and ABS is the skin adsorption fraction. The parameters of the ILCR model are listed in Supplementary Table S6.
3 Results and discussion
3.1 Contamination level
The soil sample concentrations of PAHs and the sums of the concentrations of 21 PAHs (Σ21PAHs), including the concentrations of the 16 USEPA priority PAHs (Σ16PAHs) and 7 carcinogenic PAHs (BaA, CHR, BbF, BkF, BaP, IcdP and DahA; Σ7CarPAHs), are shown in Figure 2, Supplementary Table S1, S2. The Σ21PAHs concentration in the plain soil samples ranged from 133.2 to 6,410.6 ng/g with a mean of 1,444.7 ng/g. The Σ16PAHs concentration were 100.1–5,448.3 ng/g (mean 1,240.0 ng/g), accounting for approximately 85.8% of Σ21PAHs. The Σ7CarPAHs contributed approximately 43.9% of the Σ16PAHs, with a mean of 544.8 ng/g (range 49.6–2,657.9 ng/g). The Σ21PAHs concentration in the mountain soil samples ranged from 66.5 to 2,250.2 ng/g with a mean of 585.5 ng/g. The Σ16PAHs concentration were 50.6–2065.8 ng/g (mean 500.3 ng/g ng/g), accounting for approximately 85.5% of Σ21PAHs. The Σ7CarPAHs contributed approximately 37.6% of the Σ16PAHs, with a mean of 188.0 ng/g (range 12.8–803.2 ng/g). A past study indicated that human activities are the main source of PAHs (Abdel-Shafy and Mansour, 2016; Li et al., 2020), and as shown in Figure 1B, the landform of Taiyuan city is a basin, with low elevations in the middle region and high elevations in the east and west. Human activities and heavy pollution enterprises are distributed mainly in the middle plain area at low elevations. This may be the reason why the Σ21PAHs in the plain soils were 2.47 times more concentrated than those in the mountainous soils and why the 7 carcinogenic PAHs in the plain soils were 3 times more concentrated than those in the mountain soils.
The PAHs concentration measured in plain and mountainous soils of other regions of China and other countries are shown in Supplementary Table S3. The concentrations of Σ16PAHs in the plain soils of Taiyuan were higher than those in Nanjing (China) (Yang et al., 2017), Harbin (Ma et al., 2009), Shanghai (Wang et al., 2015), Beijing-Tianjin (China) (Wang et al., 2010), Kurukshedra (India) (Kumar et al., 2013) and Ulsan (South Korea) (Kwon and Choi, 2014). Moreover, the Σ16PAHs concentration in the plain soils of Taiyuan were lower than those measured in Xi’an (China) (Wang et al., 2018), Chengdu (China) (Xing et al., 2011), Lisbon (Portugal) (Cachada et al., 2012) and Orlando (United States) (Liu et al., 2019).In addition, the Σ16PAHs concentration measured in mountainous soils were relatively high in other study regions; those measured in this study were higher than those of Changbai Mountain (China) (Zhao et al., 2015), Wang Swan (China) (Wang et al., 2019), and the eastern edge of the Himalayas (India) (Devi et al., 2016) and lower than those of the Qinling Mountains (China) (Chen et al., 2022) and British-Norwegian woodland (UK) (Nam et al., 2008).Drawing from the information provided, Taiyuan is characterized by its intensive industrial activities and coal combustion, which are recognized as significant sources of PAH emissions (Wu et al., 2023). This contributes to elevated concentrations of PAHs in the soil. Conversely, regions exhibiting lower PAH levels might be benefiting from stringent environmental policies, reduced dependency on heavy industries, or more efficacious pollution control strategies, particularly in large urban centers. Furthermore, the mountainous vicinities encircling Taiyuan, being in close proximity to urban activities, exhibit PAH concentrations surpassing those in areas with purely scenic and less affected by human activities. Nonetheless, these concentrations are marginally lower than those observed in the peripheries of more heavily industrialized mountainous regions.
3.2 Composition profiles
The concentrations of 21 individual PAHs in soil samples are shown in Supplementary Table S1, S2. In the plain area, FLA was dominant in Σ21PAHs, accounting for 13.0%, followed by BbF (10.3%), ANT (10.2%), PHE (8.3%) and PYR (8.1%). ANT was dominant in the Σ21PAHs of the mountainous soils, accounting for 13.0%, followed by FLA (11.9%), PHE (11.4%), BbF (9.2%), and PYR (7.1%). Therefore, FLA and ANT were the main PAHs, followed by PHE, BbF and PYR. A previous study (Duval and Friedlander, 1981) suggested that FLA, ANT, PHE and PYR are markers of coal combustion, while ANT and PHE are the main compounds of PAHs produced by coking, and the individual source of BbF is diesel-powered vehicles. From this information, the main sources of PAHs in Taiyuan may be coal combustion, followed by coke production and vehicle emissions.
The spatial distributions and triangle distributions by of PAHs composited with different numbers of rings in the plain and mountainous surface soils are shown in Figure 3. Among the soil samples collected in the plain area, the 4-ring and 5-ring PAHs were dominant in Σ21PAHs, accounting for 35.2% and 29.7%, respectively, followed by 3-ring (23.9%) and 6-ring (8.9%) PAHs. In addition, 2-ring and 7-ring PAHs were present at lower concentrations, contributing 1.5% and 0.8% of the Σ21PAHs, respectively. In the mountains, the 3-ring and 4-ring PAHs were dominant in Σ21PAHs, accounting for 32.7% and 31.2%, respectively, followed by 5-ring (25.3%) and 6-ring (7.8%) PAHs. In addition, 2-ring and 7-ring PAHs were present at lower concentrations, with contents of 2% and 0.9% of the Σ21PAHs, respectively.
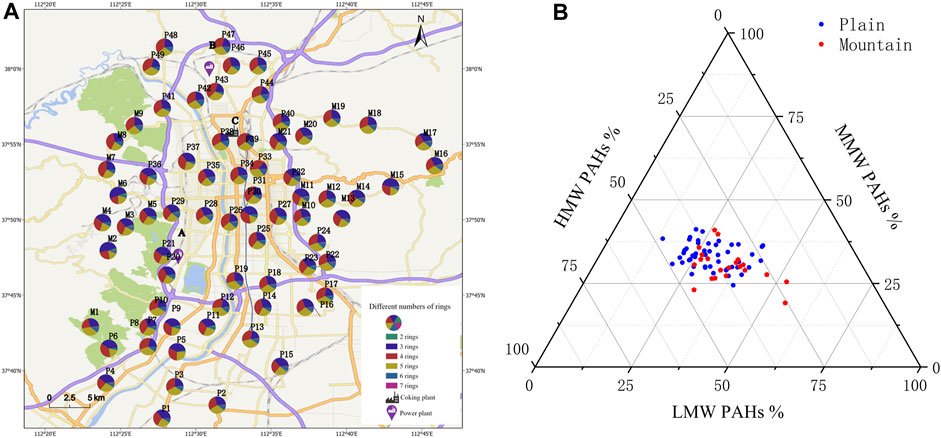
FIGURE 3. Spatial distributions (A) and triangle distributions (B) of the compmosition of PAHs with different numbers of rings in the surface soils of Taiyuan.
Investigations have revealed that 2-ring PAHs predominantly originate from the volatilization of petroleum products, whereas 3-ring and 4-ring PAHs are primarily generated through incomplete combustion processes, including those of coal, coke, and biomass, with coal combustion being a significant contributor to the presence of 4-ring PAHs. Conversely, PAHs encompassing more than 5 rings are typically associated with vehicular exhaust emissions (Guo et al., 2015; Li and Duan, 2015). In the context of Taiyuan, the analysis indicates a preponderance of 4-ring PAHs, which constitute over 32% of the Σ21PAHs, suggesting coal combustion as the principal source of pollution. This pattern aligns with findings from other Chinese urban centers such as Changzhi (Liu et al., 2017), Xinzhou (Zhao et al., 2014), and Lanzhou (Wang et al., 2016), where similar sources have been identified.However, this trend diverges in cities like Xi’an (Zhou and Lu, 2017) and Nanjing (Yang et al., 2017), where the dominant PAHs were observed to be in the 2–3 ring and 5–6 ring categories, respectively. Additionally, in the plains surrounding Taiyuan, high molecular weight (HMW) PAHs (5–7 rings) were detected at the second highest concentrations, implicating vehicle emissions as a significant contributor. In contrast, in the mountainous regions, low molecular weight (LMW) PAHs, possibly from biomass combustion (2–3 rings), were found to be the second most prevalent, highlighting the variegated sources of PAH pollution across different topographies and underscoring the complex interplay of urban and natural influences on the distribution of PAHs in the environment.
3.3 Spatial distributions of PAHs in soils
The average concentration of the 21 PAHs in all soil samples was 1,444.7 ng/g with a standard deviation of 1,451.3 ng/g, indicating significant spatial differences in the distribution of PAHs. The spatial distributions of the Σ21PAHs concentration obtained by the ordinary kriging interpolation method are shown in Figure 4. From Figures 1, 2, 4, the results demonstrated that the plain area was a hot spots for PAHs pollution. According to the (Maliszewska-Kordybach, 1996) pollution classification method that is widely used to estimate soil contamination by PAHs, PAH-contaminated soils were divided into four categories: uncontaminated (< 200 ng/g), weakly contaminated (200–600 ng/g), contaminated (600–1,000 ng/g), and heavily contaminated (> 1,000 ng/g). Accordingly, 96% of the soil in the plain was found to be contaminated by 16 priority PAHs at levels higher than 200 ng/g. Heavy contamination accounted for 40.8%, while weak contamination and contamination accounted for 40.8% and 14.3%, respectively. In the mountain, 38.1% of the sample soils were uncontaminated, and weak contamination, contamination and heavy contamination contributed 42.9%, 4.7% and 14.3%, respectively.
Analysis of Figure 1 revealed that the highest Σ21PAHs concentrations were predominantly detected in the plains within urban territories. Among these, notable pollution hotspots included sites P7 (6,410.6 ng/g), P39 (5,845.3 ng/g), P43 (4,457.1 ng/g), P31 (4,441.6 ng/g), P33 (3,530.7 ng/g), P49 (3,294.1 ng/g), P10 (3,248.5 ng/g), and P26 (3,217.4 ng/g), each recording Σ21PAHs concentrations exceeding 3,000 ng/g. Conversely, site P19 manifested the minimal pollution level at 133.2 ng/g. The spatial distribution of pollution, as depicted in Figures 1, 4, correlated with the clustering of major industrial facilities (such as thermal power stations, steel, and coking plants) and intensified human activities in the plain areas. Specifically, the highly contaminated sites P39 and P43 were situated to the southeast of the Taiyuan Iron and Steel Plant and the Taiyuan Second Thermal Power Plant, established in 1937 and 1956, respectively, signifying them as primary pollution emitters.Supporting data from Yang et al. (2017) and Supplementary Figure S1 indicated Taiyuan’s dominant northerly wind patterns, particularly during winter—a season marked by significant pollution and northwest winds. This meteorological factor, coupled with the sites’ proximity to pollution sources, likely exacerbated contamination levels at P39 and P43. Previous research (Li H. et al., 2016; Li et al., 2020) identified vehicular emissions as a significant contributor to Taiyuan’s PAH burden. This aligned with the elevated PAH concentrations at sites P31, P33, P26, and P49, which were proximal to high-traffic areas including the Taiyuan Railway Station, Liuxiang’s bustling commercial zones, Fenhe Park, and the North University of China, respectively. The dense human and vehicular activity in these locales offered a plausible explanation for the heightened soil PAH levels.The persistence and accumulation of non-volatile, decomposed, and degraded PAHs, particularly high molecular weight (HMW) PAHs, in soil could be attributed to their adsorption onto soil particles, with prolonged exposure leading to increased concentrations. This phenomenon might have accounted for the significant PAH levels at sites P7 and P10, situated near the historical Jinci Park. The long-standing pollution legacy from older industrial sites such as the Taiyuan First Power Plant (1953–2019), Taiyuan Chemical Fertilizer Plant (1960–2011), and Taiyuan Chemical Plant (1960–2011) (Wu and Yang, 1995) further compounded the issue. In contrast, the relatively low PAH levels at site P19 could be ascribed to its location within a newly established park, distanced from traditional pollution hotspots and with limited exposure duration to pollution sources, as construction commenced only in 2010 (Liu et al., 2010).
In the mountainous terrain, concentrations of Σ21PAHs at sites M6, M7, and M8 surpassed 1,000 ng/g, with the apex concentration observed at M8 (2,250.2 ng/g), succeeded by M6 (2,110.9 ng/g) and M7 (1,373.5 ng/g). The nadir was recorded at M2 with 66.5 ng/g, as depicted in Figure 2. According to Figure 1, Taiyuan’s mountainous expanse bifurcates into eastern and western sectors. The eastern sector exhibited lower pollution levels with a mean Σ21PAHs concentration of 303.2 ± 213.5 ng/g, indicating minimal pollution and spatial variance. Conversely, the western sector demonstrated elevated and variable concentrations, with a mean of 961.9 ± 782 ng/g. Notably, all Σ21PAHs concentrations exceeding 1,000 ng/g in the mountainous domain were situated in the northwestern quadrant of the western mountains. This sector has historically been a principal coal mining hub since 1933 (Qiao et al., 2011), which, alongside prolonged industrial and anthropogenic activities, likely contributed to the heightened soil contamination observed.
Spatial interpolation through ordinary kriging, as illustrated in Figure 4, pinpointed the central-northern region of Taiyuan as a primary locus for soil PAH pollution. Proximity to industrial zones or heavily trafficked areas corresponded with increased pollutant levels. Analysis of Figure 3A elucidated that in the plains, the Σ21PAHs profile was predominantly characterized by HMW PAHs, whereas the mountainous regions were chiefly influenced by LMW PAHs. Literature reviews (Lang et al., 2008), highlight that HMW PAHs tend to exhibit growth trends over time due to their low vapor pressure and propensity for accumulation. In contrast, LMW PAHs, primarily present in the gaseous phase, are more prone to photodegradation in soil environments. This may be the reason why HMW PAHs showed significant domination in the plain soil samples. Instead, the PAH concentrations in the mountainous soil samples dominated by LMW PAHs may have been partly caused by the local short-term emission of PAH characteristics in the surrounding countryside.
3.4 PAHs source identification
3.4.1 Source identification by isomer ratios
Performing a PAH source apportionment is important for evaluating the transport and fate of PAHs for controlling soil PAH contamination in the environment. To date, a number of studies have focused on methods for identifying the sources of PAHs. Among the many available methods, the isomer ratio characteristic is usually used to identify the sources of PAHs (Tobiszewski and Namieśnik, 2012). Much work has demonstrated that the possible sources of PAHs in the environment can often be identified by the diagnostic ratios of Fla/(Fla + Pyr) and BaA/(BaA + Chr). For Fla/(Fla + Pyr), ratios <0.4, 0.4–0.5 and > 0.5 indicate sources of oil, oil burning, and coal and biomass combustion, respectively. BaA/(BaA + Chr) ratios smaller than 0.2, 0.2–0.35 and greater than 0.35 indicate sources of oil, oil combustion, and coal and biomass combustion, respectively (Yunker et al., 2002; Du et al., 2021). In addition, LMW/HMW has also been used as an indicator to estimate the sources of PAHs. When LMW/HMW> 1, LMW PAHs dominate in the soil samples, and petrogenic PAHs such as biomass combustion are dominated by LMW PAHs (Qu et al., 2020). When LMW/HMW <1, HMW PAHs dominate in the soil samples, and the sources are mainly anthropogenic, such as thermal alteration (Soclo et al., 2000; Yan et al., 2019).
As shown in Supplementary Figure S2, Table S4, the mean Fla/(Fla + Pyr) and BaA/(BaA + Chr) ratios were 0.63 and 0.62 in the plain region and 0.45 and 0.47 in the mountainous region, respectively. These results suggest that the main sources of PAHs in the soils sampled in Taiyuan were coal combustion and biomass combustion. Moreover, the BaA/(BaA + Chr) ratios in some samples had values < 0.35, indicating that vehicle emissions were also a source of soil PAHs in Taiyuan. As shown in Figure 3B, the LMW/HMW ratios in both the plain and mountainous areas were smaller than 1, further indicating that the PAHs were thermally generated, especially through the combustion of coal.
3.4.2 Source identification by PCA/MLR
Principal component analysis (PCA) is a widely used method to proportion the sources of PAHs (Golobočanin et al., 2004). The rotation factors of 21 PAHs are shown in Supplementary Table S5. Prior studies have shown that RET has been used as a marker of biomass combustion (Shen et al., 2012a; Wang et al., 2016). FLO has been found to be a good marker of coke production (Ciaparra et al., 2009; Khalili et al., 1995). BbF, BkF, and CHR are derived mainly from coal combustion (Brown and Brown, 2012; Jia et al., 2021). IcdP and BghiP are enriched in vehicle tunnels and are thus signs of vehicle emissions (Khalili et al., 1995; Li J. et al., 2016).
From the plain samples, the PCA extracted 2 common factors that explained 87.6% of the total variance; PC1 and PC2 accounted for 59.3% and 28.3% of the total variance, respectively. Factor PC1 was dominated by NAP, PHE, ANT, FLA, PYR, BaA, CHR, BbF, BkF, BaP, DahA, PER, BeP, IcdP, and BghiP. PC1 represented mixed PAH sources from coal combustion and vehicle emissions. PC2 was predominantly loaded with ANY, ACE, FLO, RET, CPP, and COR. Thus, PC2 indicated a mixed PAH sources of coke production and biomass combustion.
For the mountainous samples, the PCA extracted three common factors that explained 97.5% of the total variance; PC1, PC2, and PC3 accounted for 58.1%, 33.7%, and 5.7% of the total variance, respectively. Factor PC1 was dominated by ACE, FLA, PYR, BaA, CHR, BbF, BkF, BaP, DahA, PER, BeP, IcdP, BghiP and COR. PC1 was thus designated as indicating mixed PAH sources from coal combustion and vehicle emissions. PC2 predominantly loaded with NAP, ANY, FLO, PHE, ANT, and CPP. PC2 indicated a PAH source from coke production. PC3 was dominated by RET and thus represented biomass emissions.
Based on the PCA identification of the possible sources of PAHs, multiple linear regression (MLR) was used to calculate the PAHs concentrations and the contribution rate of each PAH source. The MLR equation applied to the plain equations in Eq. 4 and to the equations for mountainous areas in Eq. 5. are as follows:
According to Figure 5the largest potential pollution sources in the plain area were coal combustion and vehicle emissions, contributing an average of 65.3% of the PAH emissions, followed by mixed sources of coke production and biomass combustion, which accounted for 34.5% of the PAH emissions. In the mountainous areas, the largest potential sources of pollution were coal combustion and vehicle emissions, contributing an average of 54.1% of the PAH emissions, followed by coke production and biomass combustion, which accounted for 43.0% and 3.1% of the PAH emissions, respectively. To summarize the results, in the mountainous area, the average contribution rate of coal burning and vehicle emissions was reduced by 10.2%, and RET was separated as a principal component. According to a past study (Shen et al., 2012b), RET is a unique marker of soft wood combustion. Compared to the plain, the mountainous regions had less anthropogenic emissions and more opportunities to use more soft wood as fuel for heating, cooking and other activities. This may be the reason that the PAH sources of coal combustion and vehicle emissions decreased while biomass combustion increased in the mountainous region. On the basis of the isomer ratios and PCA/MLR results, we concluded that the main sources of PAHs in the Taiyuan soils were coal combustion, coke production, vehicle emission and biomass combustion, and these sources were consistent with the findings of previously published studies (Jiang et al., 2013; Cheng et al., 2018; Li et al., 2020).
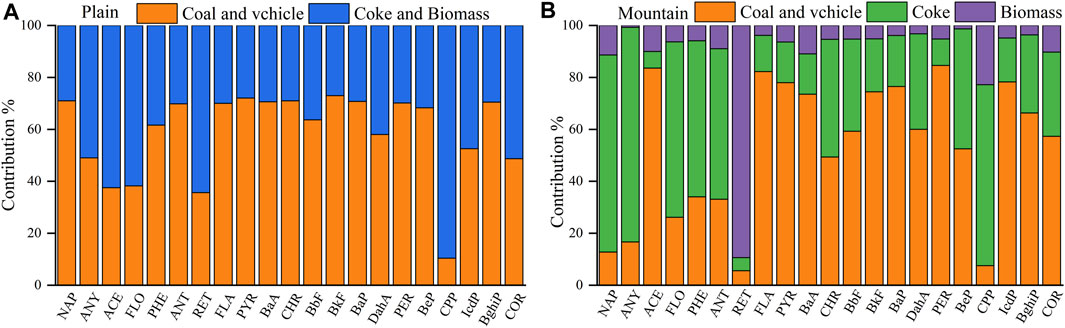
FIGURE 5. Contributions and average contributions of the selected pollution source variables in the study area: (A) the plain and (B) mountain regions.
3.5 Human health risk assessment
The Lifelong Incremental Cancer Risk (ILCR) model was used to assess the incremental cancer risks resulting from inhalation, ingestion and dermal exposure to PAHs, and the ILCR values for children and adults corresponding to the soils in the plain and mountainous regions were calculated using Eqs 1–3.
As shown in Figure 6 and Supplementary Table S8, the inhalation ILCR values of children and adults in the mountainous and plain regions were approximately 10−12, much lower than the ingestion and dermal exposure values, which had the same order of magnitude of approximately 10−7, indicating that the inhalation of heavy particles through the mouth and nose was negligible in terms of the carcinogenic risk posed by soil-induced PAHs; this finding was similar to previously published results (Jia et al., 2021). As shown in Supplementary Table S8, in the plain soils, the ingestion and dermal ILCR values for children were 3.4 × 10−8–1.8 × 10−6 (mean 3.4 × 10−7) and 4.2 × 10−8–2.2 × 10−6 (mean 4.3 × 10−7), respectively. Adults’ ingestion and dermal ILCR values were 4.2 × 10−8–2.2 × 10−6 (mean 2.7 × 10−7) and 4.7 × 10−8–2.4 × 10−6 (mean 4.8 × 10−7), respectively. These results demonstrated that children had a 1.3 times greater ingestion ILCR and 0.9 times greater dermal ILCR than adults. According to previous studies (Zhang et al., 2020; Jia et al., 2021), the main reason for these differences is that children are more likely to ingest soils and dust through hand-matched activities than adults, and adults should surpass children in terms of their skin exposure area. The ILCR differences for the ingestion and dermal contact between children and adults in the mountainous region were similar to those in the plain region. However, in the mountainous region, the cancer risk value was lower than that in the plain area; children’s ingestion and dermal exposure were both 0.32 times greater than those in the plain area, and adults had ingestion and dermal exposure risks 0.32 and 0.31 times greater than those in the plain area, respectively.
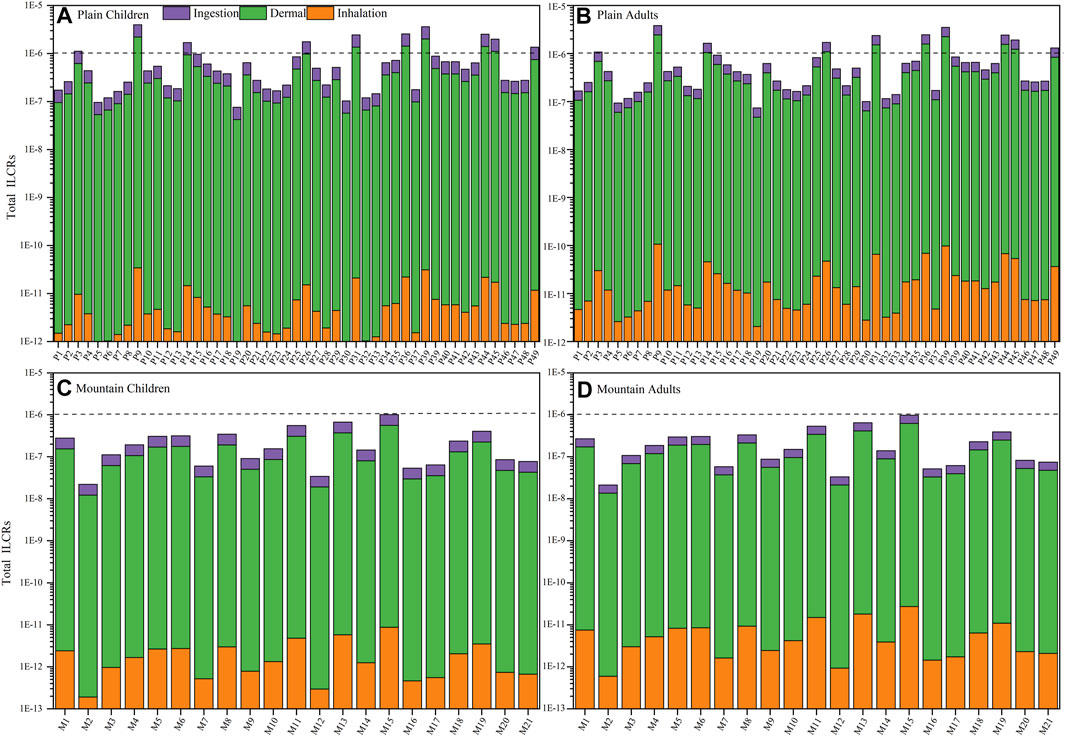
FIGURE 6. Incremental lifetime cancer risks (ILCRs) associated with the soils in the plain and mountainous regions.
According to the USEPA standard, ILCRs below 106 represent a safe levels with negligible carcinogenicity, while ILCRs between 106 and 104 or greater than 104 represent a potential and high carcinogenic risks, respectively. As shown in Supplementary Table S8, the mean total ILCR values were 7.7 × 10−7 and 7.5 × 10−7 for children and adults in the plain region, respectively. The values in the mountains were 2.5 × 10−7 and 2.4 × 10−7, respectively. These results indicate that the cancer risks of PAHs in Taiyuan were at a safe level, with children more likely to have higher exposure risks than adults, and those in the plain region were more easily exposed to PAH risk than those in the mountains. However, some samples showed potential risk points at all sites except point M8 located in the plain area, such as sites P7, P39, P33, P43, P31, P49, P26, and P10. According to the previous analysis, these sites were mainly located near industrial areas, commercial areas, urban parks and coal mining areas. The frequent human activities near these sites lead to the high PAH concentrations in these areas, especially of carcinogenic PAHs such as BaP and DahA.
4 Conclusion
This study analyzed the concentrations, types, distribution, potential sources and risks of 21 PAHs in soil samples from the plain and mountain regions of Taiyuan. The results showed that Σ16PAHs accounted for approximately 82% of Σ21PAHs. Moreover, the total PAHs measured on the plain were 2.47 times more prevalent than those in the mountainous area, and the carcinogenic PAHs were approximately 3 times more prevalent than those in the mountains. The soil Σ16PAHs contamination in Taiyuan was at a moderately high level compared to the rest of China. In the component distribution results, FLA and ANT were found to be the main PAHs, and 4-ring PAHs were the dominant PAHs in Taiyuan, indicating that the main source of PAHs was coal burning, followed by coke production. In the Σ21PAHs spatial distribution results, the overall soil PAHs pollution characteristics of Taiyuan were high in the central-northern area. Two soil PAHs contamination accumulation areas were identified in the northern area centered on the Taiyuan Iron and Steel Plant and Taiyuan Second Thermal Power Plant and in the southwest area with Jinci Park as the center. The isomer ratio and PCA/MLR results showed that the PAHs concentrations were derived mainly from coal combustion, coke production, vehicle emissions and biomass combustion. The incremental lifetime cancer risks (ILCR) indicated that the soil PAHs carcinogenicity in Taiyuan was at a safe level, though some samples located in heavily polluted areas showed potential risks. The cancer risk in the plain region was 3 times greater than that in the mountainous area. The risks posed to children were higher than those of adults, and children were more likely to obtain cancer risks through ingestion. Overall, this study of PAHs contamination in soils under different terrain types in Taiyuan can provide a scientific basis for further regional environmental quality protection, for adjusting the energy structure to reduce the emission of pollutants through activities such as coal combustion, and for protecting human health.
Data availability statement
The original contributions presented in the study are included in the article/Supplementary Material, further inquiries can be directed to the corresponding author.
Author contributions
LL: Data curation, Funding acquisition, Investigation, Software, Writing–original draft. XC: Data curation, Formal Analysis, Writing–original draft. YD: Funding acquisition, Supervision, Validation, Writing–review and editing. ZW: Conceptualization, Data curation, Formal Analysis, Investigation, Writing–review and editing. LX: Supervision, Writing–review and editing.
Funding
The author(s) declare financial support was received for the research, authorship, and/or publication of this article. This research was supported by the Research Innovation Program for College Graduates of Shanxi Province (Grant No. 2020BY047) and National Natural Science Foundation of China (Project No. 41271507).
Acknowledgments
We thank the editor and three reviewers for their valuable comments on the manuscript.
Conflict of interest
The authors declare that the research was conducted in the absence of any commercial or financial relationships that could be construed as a potential conflict of interest.
Publisher’s note
All claims expressed in this article are solely those of the authors and do not necessarily represent those of their affiliated organizations, or those of the publisher, the editors and the reviewers. Any product that may be evaluated in this article, or claim that may be made by its manufacturer, is not guaranteed or endorsed by the publisher.
Supplementary material
The Supplementary Material for this article can be found online at: https://www.frontiersin.org/articles/10.3389/fenvs.2024.1363297/full#supplementary-material
References
Abdel-Shafy, H. I., and Mansour, M. S. (2016). A review on polycyclic aromatic hydrocarbons: source, environmental impact, effect on human health and remediation. Egypt J. Pet. 25, 107–123. doi:10.1016/j.ejpe.2015.03.011
Bortey-Sam, N., Ikenaka, Y., Akoto, O., Nakayama, S. M., Asante, K. A., Baidoo, E., et al. (2017). Oxidative stress and respiratory symptoms due to human exposure to polycyclic aromatic hydrocarbons (PAHs) in Kumasi, Ghana. Environ. Pollut. 228, 311–320. doi:10.1016/j.envpol.2017.05.036
Brown, A. S., and Brown, R. J. (2012). Correlations in polycyclic aromatic hydrocarbon (PAH) concentrations in UK ambient air and implications for source apportionment. J. Environ. Monit. 14, 2072–2082. doi:10.1039/c2em10963h
Cachada, A., Pato, P., Rocha-Santos, T., da Silva, E. F., and Duarte, A. (2012). Levels, sources and potential human health risks of organic pollutants in urban soils. Sci. Total Environ. 430, 184–192. doi:10.1016/j.scitotenv.2012.04.075
Chen, Z., Tian, Z., Liu, X., and Sun, W. (2022). The potential risks and exposure of Qinling giant pandas to polycyclic aromatic hydrocarbon (PAH) pollution. Environ. Pollut. 292, 118294. doi:10.1016/j.envpol.2021.118294
Cheng, M., Zhang, L., He, Q., Guo, X., Zhang, G., and Wang, X. (2018). Pollution characteristics of polycyclic aromatic hydrocarbons in park soil in Taiyuan,China. Environ. Chem. 37, 8.
Choi, S.-D., Shunthirasingham, C., Daly, G. L., Xiao, H., Lei, Y. D., and Wania, F. (2009). Levels of polycyclic aromatic hydrocarbons in Canadian mountain air and soil are controlled by proximity to roads. Environ. Pollut. 157, 3199–3206. doi:10.1016/j.envpol.2009.05.032
Devi, N. L., Yadav, I. C., Shihua, Q., Dan, Y., Zhang, G., and Raha, P. (2016). Environmental carcinogenic polycyclic aromatic hydrocarbons in soil from Himalayas, India: implications for spatial distribution, sources apportionment and risk assessment. Chemosphere 144, 493–502. doi:10.1016/j.chemosphere.2015.08.062
Du, W., Wang, J., Zhuo, S., Zhong, Q., Wang, W., Chen, Y., et al. (2021). Emissions of particulate PAHs from solid fuel combustion in indoor cookstoves. Sci. Total Environ. 771, 145411. doi:10.1016/j.scitotenv.2021.145411
Duan, Y., Shen, G., Tao, S., Hong, J., Chen, Y., Xue, M., et al. (2015). Characteristics of polycyclic aromatic hydrocarbons in agricultural soils at a typical coke production base in Shanxi, China. Chemosphere 127, 64–69. doi:10.1016/j.chemosphere.2014.12.075
Duval, M., and Friedlander, S. (1981). Source resolution of polycyclic aromatic hydrocarbons in the Los Angeles atmosphere application of a CMB with first-order decay. Washington, DC, USA, Control Technol Cent: U S Environ Prot Agency EPA.
Fu, S., Cheng, H.-X., Liu, Y.-H., and Xu, X.-B. (2009). Levels and distribution of organochlorine pesticides in various media in a mega-city, China. Chemosphere 75, 588–594. doi:10.1016/j.chemosphere.2009.01.061
Golobočanin, D. D., Škrbić, B. D., and Miljević, N. R. (2004). Principal component analysis for soil contamination with PAHs. Chemom. Intell. Lab. Syst. 72, 219–223. doi:10.1016/j.chemolab.2004.01.017
Guo, L., Cui, Y., Zhang, G., Yan, Y., He, Q., and Wang, X. (2015). Pollution characteristics of polycyclic aromatic hydrocarbons in PM2.5 during the heating season in taiyuan city,China. Earth Environ. 43, 1672–9250.
Jia, T., Guo, W., Xing, Y., Lei, R., Wu, X., Sun, S., et al. (2021). Spatial distributions and sources of PAHs in soil in chemical industry parks in the Yangtze River Delta, China. Environ. Pollut. 283, 117121. doi:10.1016/j.envpol.2021.117121
Jiang, Q., Li, y., Hu, x., Lu, B., Tao, S., and Wong, Y. (2013). Estimation of annual emission and distribution characteristics of polycyclic aromatic hydrocarbons (PAHs) in Taiyuan. China Environ. Sci. 33, 14–20.
Khalili, N. R., Scheff, P. A., and Holsen, T. M. (1995). PAH source fingerprints for coke ovens, diesel and, gasoline engines, highway tunnels, and wood combustion emissions. Atmos. Environ. 29, 533–542. doi:10.1016/1352-2310(94)00275-p
Kumar, B., Verma, V. K., Kumar, S., and Sharma, C. S. (2013). Probabilistic health risk assessment of polycyclic aromatic hydrocarbons and polychlorinated biphenyls in urban soils from a tropical city of India. J. Environ. Sci. Health, Part A Environ. Sci. Eng. 48, 1253–1263. doi:10.1080/10934529.2013.776894
Kwon, H.-O., and Choi, S.-D. (2014). Polycyclic aromatic hydrocarbons (PAHs) in soils from a multi-industrial city, South Korea. Sci. Total Environ. 470, 1494–1501. doi:10.1016/j.scitotenv.2013.08.031
Lang, C., Tao, S., Liu, W., Zhang, Y., and Simonich, S. (2008). Atmospheric transport and outflow of polycyclic aromatic hydrocarbons from China. Environ. Sci. Technol. 42, 5196–5201. doi:10.1021/es800453n
Li, H., Guo, L., Cao, R., Gao, B., Yan, Y., and He, Q. (2016a). A wintertime study of PM2. 5-bound polycyclic aromatic hydrocarbons in Taiyuan during 2009–2013: assessment of pollution control strategy in a typical basin region. Atmos. Environ. 140, 404–414. doi:10.1016/j.atmosenv.2016.06.013
Li, J., Dillon, W., Xu, Y., Li, X., Wang, X., Zeng, C., et al. (2016b). Recent distribution and sources of polycyclic aromatic hydrocarbons in surface soils from Yangtze River Delta. Environ. Sci. 9.
Li, R., Cheng, M., Cui, Y., He, Q., Guo, X., Chen, L., et al. (2020). Distribution of the soil PAHs and health risk influenced by coal usage processes in taiyuan city, northern China. Int. J. Environ. Res. Public Health 17, 6319. doi:10.3390/ijerph17176319
Li, Y., and Duan, X. (2015). Polycyclic aromatic hydrocarbons in sediments of China Sea. Environ. Sci. Pollut. Res. 22, 15432–15442. doi:10.1007/s11356-015-5333-6
Liang, W., Li, H. a., and Yang, C. (2000). Edge geomorphology and neotectonic movement in taiyuan basin and their meanings. J. Shanxi Univ. 23.
Liu, G., Guo, W., Niu, J., An, X., and Zhao, L. (2017). Polycyclic aromatic hydrocarbons in agricultural soils around the industrial city of Changzhi, China: characteristics, spatial distribution, hotspots, sources, and potential risks. J. Soils Sediments 17, 229–239. doi:10.1007/s11368-016-1490-6
Liu, Q., Wu, Y., Zhou, Y., Li, X., Yang, S., Chen, Y., et al. (2021). A novel method to analyze the spatial distribution and potential sources of pollutant combinations in the soil of Beijing urban parks. Environ. Pollut. 284, 117191. doi:10.1016/j.envpol.2021.117191
Liu, S., Xia, X., Yang, L., Shen, M., and Liu, R. (2010). Polycyclic aromatic hydrocarbons in urban soils of different land uses in Beijing, China: distribution, sources and their correlation with the city's urbanization history. J. Hazard Mater 177, 1085–1092. doi:10.1016/j.jhazmat.2010.01.032
Liu, Y., Gao, P., Su, J., da Silva, E. B., de Oliveira, L. M., Townsend, T., et al. (2019). PAHs in urban soils of two Florida cities: background concentrations, distribution, and sources. Chemosphere 214, 220–227. doi:10.1016/j.chemosphere.2018.09.119
Ma, W.-L., Li, Y.-F., Sun, D.-Z., and Qi, H. (2009). Polycyclic aromatic hydrocarbons and polychlorinated biphenyls in topsoils of Harbin, China. Arch. Environ. Contam. Toxicol. 57, 670–678. doi:10.1007/s00244-009-9314-y
Maliszewska-Kordybach, B. (1996). Polycyclic aromatic hydrocarbons in agricultural soils in Poland: preliminary proposals for criteria to evaluate the level of soil contamination. Appl. Geochem 11, 121–127. doi:10.1016/0883-2927(95)00076-3
Melymuk, L., Robson, M., Helm, P. A., and Diamond, M. (2012). PCBs, PBDEs, and PAHs in Toronto air: spatial and seasonal trends and implications for contaminant transport. Sci. Total Environ. 429, 272–280. doi:10.1016/j.scitotenv.2012.04.022
Menzie, C. A., Potocki, B. B., and Santodonato, J. (1992). Exposure to carcinogenic PAHs in the environment. Environ. Sci. Technol. 26, 1278–1284. doi:10.1021/es00031a002
Mirzababaei, A., Daneshzad, E., Moradi, S., Abaj, F., Mehranfar, S., Asbaghi, O., et al. (2021). The association between urinary metabolites of polycyclic aromatic hydrocarbons (PAHs) and cardiovascular diseases and blood pressure: a systematic review and meta-analysis of observational studies. Environ. Sci. Pollut. Res. 29, 1712–1728. doi:10.1007/s11356-021-17091-4
Mu, L., Li, X., Liu, X., Bai, H., Peng, L., Li, Y., et al. (2021). Characterization and emission factors of carbonaceous aerosols originating from coke production in China. Environ. Pollut. 268, 115768. doi:10.1016/j.envpol.2020.115768
Nam, J. J., Thomas, G. O., Jaward, F. M., Steinnes, E., Gustafsson, O., and Jones, K. C. (2008). PAHs in background soils from Western Europe: influence of atmospheric deposition and soil organic matter. Chemosphere 70, 1596–1602. doi:10.1016/j.chemosphere.2007.08.010
Qiao, X., Li, G., Li, M., Zhou, J., Du, J., Du, C., et al. (2011). Influence of coal mining on regional karst groundwater system: a case study in West Mountain area of Taiyuan City, northern China. Environ. Earth Sci. 64, 1525–1535. doi:10.1007/s12665-010-0586-3
Qu, Y., Gong, Y., Ma, J., Wei, H., Liu, Q., Liu, L., et al. (2020). Potential sources, influencing factors, and health risks of polycyclic aromatic hydrocarbons (PAHs) in the surface soil of urban parks in Beijing, China. Environ. Pollut. 260, 114016. doi:10.1016/j.envpol.2020.114016
Shen, G., Tao, S., Chen, Y., Zhang, Y., Wei, S., Xue, M., et al. (2013). Emission characteristics for polycyclic aromatic hydrocarbons from solid fuels burned in domestic stoves in rural China. Environ. Sci. Technol. 47, 14485–14494. doi:10.1021/es403110b
Shen, G., Tao, S., Wei, S., Zhang, Y., Simonich, S. L. M., Wang, B., et al. (2012a). Retene emission from residential solid fuels in China and evaluation of retene as a unique marker for soft wood combustion. Environ. Sci. Technol. 46, 4666–4672. doi:10.1021/es300144m
Shen, G., Tao, S., Wei, S., Zhang, Y., Wang, R., Wang, B., et al. (2012b). Emissions of parent, nitro, and oxygenated polycyclic aromatic hydrocarbons from residential wood combustion in rural China. Environ. Sci. Technol. 46, 8123–8130. doi:10.1021/es301146v
Singh, B. P., Kumar, K., and Jain, V. K. (2021). Distribution of ring PAHs in particulate/gaseous phase in the urban city of Delhi, India: seasonal variation and cancer risk assessment. Urban Clim. 40, 101010. doi:10.1016/j.uclim.2021.101010
Soclo, H., Garrigues, P., and Ewald, M. (2000). Origin of polycyclic aromatic hydrocarbons (PAHs) in coastal marine sediments: case studies in Cotonou (Benin) and Aquitaine (France) areas. Mar. Pollut. Bull. 40, 387–396. doi:10.1016/s0025-326x(99)00200-3
Tao, S., Wang, W., Liu, W., Zuo, Q., Wang, X., Wang, R., et al. (2011). Polycyclic aromatic hydrocarbons and organochlorine pesticides in surface soils from the Qinghai-Tibetan plateau. J. Environ. Monit. 13, 175–181. doi:10.1039/c0em00298d
TBS (2019). Statistics bulletin of the national economic and social development of Taiyuan. Taiyuan, China: Taiyuan city Bureau of Statistics.
Tobiszewski, M., and Namieśnik, J. (2012). PAH diagnostic ratios for the identification of pollution emission sources. Environ. Pollut. 162, 110–119. doi:10.1016/j.envpol.2011.10.025
Totten, L. A., Gigliotti, C. L., VanRy, D. A., Offenberg, J. H., Nelson, E. D., Dachs, J., et al. (2004). Atmospheric concentrations and deposition of polychorinated biphenyls to the Hudson River Estuary. Environ. Sci. Technol. 38, 2568–2573. doi:10.1021/es034878c
Wang, J., Ho, S. S. H., Huang, R., Gao, M., Liu, S., Zhao, S., et al. (2016). Characterization of parent and oxygenated-polycyclic aromatic hydrocarbons (PAHs) in Xi’an, China during heating period: an investigation of spatial distribution and transformation. Chemosphere 159, 367–377. doi:10.1016/j.chemosphere.2016.06.033
Wang, L., Zhang, P., Wang, L., Zhang, W., Shi, X., Lu, X., et al. (2018). Polycyclic aromatic hydrocarbons in urban soil in the semi-arid City of Xi’an, Northwest China: composition, distribution, sources, and relationships with soil properties. Arch. Environ. Contam. Toxicol. 75, 351–366. doi:10.1007/s00244-018-0522-1
Wang, W., Simonich, S. L. M., Xue, M., Zhao, J., Zhang, N., Wang, R., et al. (2010). Concentrations, sources and spatial distribution of polycyclic aromatic hydrocarbons in soils from Beijing, Tianjin and surrounding areas, North China. Environ. Pollut. 158, 1245–1251. doi:10.1016/j.envpol.2010.01.021
Wang, X.-T., Chen, L., Wang, X.-K., Lei, B.-L., Sun, Y.-F., Zhou, J., et al. (2015). Occurrence, sources and health risk assessment of polycyclic aromatic hydrocarbons in urban (Pudong) and suburban soils from Shanghai in China. Chemosphere 119, 1224–1232. doi:10.1016/j.chemosphere.2014.10.019
Wang, Z., Liu, S., and Zhang, T. (2019). Characteristics of polycyclic aromatic hydrocarbons (PAHs) in soil horizon from high-altitude mountains in Northeastern China. Chemosphere 225, 93–103. doi:10.1016/j.chemosphere.2019.03.001
Wu, Z., Duan, Y., Liu, L., Xu, L., Yao, X., Chen, X. J. J. o. S., et al. (2023). Characteristics of polycyclic aromatic hydrocarbons in the soils of different functional areas of a typical industrial capital city, Taiyuan, Shanxi province, China. Taiyuan, Shanxi Prov. China 23, 1315–1331. doi:10.1007/s11368-022-03379-6
Wu, Z., and Yang, J. (1995). Study on atmospheric particulate pollution in Jinci tourist point in Taiyuan. J. Shanxi Med. Univ. 26, 15–16.
Xing, X., Qi, S., Zhang, J., Wu, C., Zhang, Y., Yang, D., et al. (2011). Spatial distribution and source diagnosis of polycyclic aromatic hydrocarbons in soils from Chengdu Economic Region, Sichuan Province, western China. J. Geochem Explor 110, 146–154. doi:10.1016/j.gexplo.2011.05.001
Xing, X., Zhang, Y., Yang, D., Zhang, J., Chen, W., Wu, C., et al. (2016). Spatio-temporal variations and influencing factors of polycyclic aromatic hydrocarbons in atmospheric bulk deposition along a plain-mountain transect in western China. Atmos. Environ. 139, 131–138. doi:10.1016/j.atmosenv.2016.05.027
Xu, S., Liu, W., and Tao, S. (2006). Emission of polycyclic aromatic hydrocarbons in China. Environ. Sci. Technol. 40, 702–708. doi:10.1021/es0517062
Xu, X., Cook, R. L., Ilacqua, V. A., Kan, H., Talbott, E. O., and Kearney, G. (2010). Studying associations between urinary metabolites of polycyclic aromatic hydrocarbons (PAHs) and cardiovascular diseases in the United States. Sci. Total Environ. 408, 4943–4948. doi:10.1016/j.scitotenv.2010.07.034
Yan, D., Wu, S., Zhou, S., Tong, G., Li, F., Wang, Y., et al. (2019). Characteristics, sources and health risk assessment of airborne particulate PAHs in Chinese cities: a review. Environ. Pollut. 248, 804–814. doi:10.1016/j.envpol.2019.02.068
Yang, J., Yu, F., Yu, Y., Zhang, J., Wang, R., Srinivasulu, M., et al. (2017). Characterization, source apportionment, and risk assessment of polycyclic aromatic hydrocarbons in urban soil of Nanjing, China. J. Soils Sediments 17, 1116–1125. doi:10.1007/s11368-016-1585-0
Yong, L., Huifeng, W., Xiaoting, L., and Jinchang, L. (2015). Heavy metal contamination of agricultural soils in Taiyuan, China. Pedosphere 25, 901–909. doi:10.1016/s1002-0160(15)30070-9
Yunker, M. B., Macdonald, R. W., Vingarzan, R., Mitchell, R. H., Goyette, D., and Sylvestre, S. (2002). PAHs in the Fraser River basin: a critical appraisal of PAH ratios as indicators of PAH source and composition. Org. Geochem 33, 489–515. doi:10.1016/s0146-6380(02)00002-5
Zhang, J., Liu, F., Huang, H., Wang, R., and Xu, B. (2020). Occurrence, risk and influencing factors of polycyclic aromatic hydrocarbons in surface soils from a large-scale coal mine, Huainan, China. Ecotoxicol. Environ. Saf. 192, 110269. doi:10.1016/j.ecoenv.2020.110269
Zhao, L., Hou, H., Shangguan, Y., Cheng, B., Xu, Y., Zhao, R., et al. (2014). Occurrence, sources, and potential human health risks of polycyclic aromatic hydrocarbons in agricultural soils of the coal production area surrounding Xinzhou, China. Ecotoxicol. Environ. Saf. 108, 120–128. doi:10.1016/j.ecoenv.2014.05.034
Zhao, X., Kim, S.-K., Zhu, W., Kannan, N., and Li, D. (2015). Long-range atmospheric transport and the distribution of polycyclic aromatic hydrocarbons in Changbai Mountain. Chemosphere 119, 289–294. doi:10.1016/j.chemosphere.2014.06.005
Keywords: polycyclic aromatic hydrocarbon, spatial distributions, sources, risk assessment, Taiyuan
Citation: Liu L, Chen X, Duan Y, Wu Z and Xu L (2024) Distribution, sources and health risk assessment of polycyclic aromatic hydrocarbons in urban soils under different landform conditions of Taiyuan, China. Front. Environ. Sci. 12:1363297. doi: 10.3389/fenvs.2024.1363297
Received: 05 January 2024; Accepted: 02 February 2024;
Published: 21 February 2024.
Edited by:
Sudhir Kumar Pandey, Guru Ghasidas Vishwavidyalaya, IndiaReviewed by:
Arup Giri, Baba Mastnath University, IndiaSema Yurdakul, Süleyman Demirel University, Türkiye
Copyright © 2024 Liu, Chen, Duan, Wu and Xu. This is an open-access article distributed under the terms of the Creative Commons Attribution License (CC BY). The use, distribution or reproduction in other forums is permitted, provided the original author(s) and the copyright owner(s) are credited and that the original publication in this journal is cited, in accordance with accepted academic practice. No use, distribution or reproduction is permitted which does not comply with these terms.
*Correspondence: Yonghong Duan, yhduanpku@126.com