- 1Institute of Environmental Engineering, Department of Ecological Health, Hangzhou Vocational and Technical College, Hangzhou, China
- 2Department of Design, Zongsheng Design Group Ltd. of Zhejiang Province, Wenzhou, China
The auto parts industry occupies an important strategic position in our national economy, which brings about the pollution problem in the processing of auto parts, particularly in soil polluted by heavy metals. Soil samples were collected from an automobile parts company in Jiaxing, China, and the data were evaluated using the land accumulation index method. The study found that the heavy metal pollution in the downwind direction of the Automobile Parts Co., Ltd. is mainly As, CD, and Zn mixed heavy metal pollution, and the distribution is uneven. The coefficient of variation of As was the largest, and the regional variation amplitude was large. The coefficient of variation of CD, Cr, and Ni is 50%, that of Zn is 39.38%, and that of PB is the lowest. The accumulative index of AS and CD was 6, which was a very serious pollution level. The content of As was 1994.7 mg/kg, exceeding the standard by more than 44 times, and the distribution of As in soil was uneven. The pollution level of Zn is 3, which belongs to the moderate level. The pollution degree of heavy metals in the soil decreases as the distance from the downwind outlet of the plant increases. According to the health risk assessment, the main route of heavy metals entering the body is through the mouth by breathing. Among exposure routes, oral exposure to heavy metals is the most harmful, so we need to pay special attention to farmland soil heavy metal pollution.
1 Introduction
Automotive parts are one of the automotive service industries, which have various units that make up the entire automobile and products that serve the automobile, collectively referred to as automotive parts. The upstream of the automotive parts industry includes the production of raw materials, such as steel, plastic, and rubber, whereas the downstream mainly targets the supporting market of the main engine factory and the after-sales service market (Liang et al., 2022). The automotive parts industry has many upstream- and downstream-related industries, particularly upstream, so this industry occupies an important strategic position in China’s national economy. In recent years, with the improvement of the living standards of Chinese residents, small cars have spread throughout every household. However, toxic metals, such as Pb, Hg, Cd6+, and Cr are found in automotive parts (Diwa et al., 2022). Lead is the most commonly used substance in automobiles among the four toxic metals. Lead mainly exists in seven forms in automotive materials, for example, instrument pointers for counterweights, seat belt sensors, and lead used as alloy components (Chen et al., 2019). The main applications of Hg in automobiles are lighting in instrument clusters, fluorescent lamps in cars, and others. Cd6+ can be used as an alloy material or as a coating component.
The harm of lead (Liverpool, 2021). Lead in automotive parts can enter the environment in multiple ways. Lead in automotive parts (1) evaporates into the atmosphere through steam and particles and (2) enters rivers and soil with rainwater after electrochemical corrosion. When people breathe lead-containing air or drink lead-containing water, lead enters the human body, which will lead to chronic lead poisoning.
The harm of mercury (Cheng et al., 2023). Mercury in scrapped car parts can quickly evaporate and enter the atmosphere and also be deposited in rivers and soil through electrochemical corrosion with rainwater. Mercury can enter the alveoli through the human respiratory tract, which can be completely absorbed by the human body. Long-term accumulation will lead to mercury poisoning.
Hazards of hexavalent chromium. The chromium metal in end-of-life auto parts can easily enter rivers and soil with rainwater through electrochemical corrosion, and sodium dichromate–containing hexavalent chromium will corrode sewers. When hexavalent chromium significantly exceeds the standard (Liverpool, 2021; Adotey et al., 2022), it will also lead to lung cancer.
The harm of cadmium. Cadmium metal in scrapped cars enters the atmosphere in the form of particles, and after electrochemical corrosion, it can also enter rivers and soil with rainwater. When people inhale air or consume water and food with a high cadmium content, cadmium metal accumulates in the human body, which can cause cancer over time. Therefore, high-pollution, low-end manufacturing enterprises will not be able to survive, and the development of the automotive parts industry must take the path of energy-saving, green, and sustainable development. Automotive parts contain considerable heavy metals, and waste automotive parts factories should promptly recycle them to avoid secondary pollution.
At present, some scholars have conducted investigations and studies on the heavy metal content in soil (LI et al., 2012; Lu et al., 2012; Shen et al., 2017; Zhao et al., 2017). However, no comprehensive and systematic investigations and studies exist on the heavy metal pollution situation in the soil around automobile parts factories. Hence, our research group systematically explores the current situation of heavy metal pollution in the surrounding agricultural land soil of automobile parts companies. This study also conducts risk assessments using considerable measured data to provide a basis for early warning of soil environmental quality. Starting from evaluating the ecological and environmental effects of heavy metal pollution in soil, this study uses the ground accumulation index and potential ecological hazard index of heavy metals in soil as pollution evaluation indicators to evaluate the level of heavy metal pollution in agricultural soil in the study area. This study contributes to controlling and regulating heavy metals in farmland soil and has guiding significance.
2 Materials and methods
2.1 Overview of the study area
The auto parts company was located in the Jiaxing Asia Pacific Science and Technology Industrial Park, Nanhu District, Jiaxing, Zhejiang Province, in the center of the Yangtze Delta metropolitan area. The downtown of Jiaxing was 25 km or 1 h from Shanghai, Hangzhou, and Suzhou. Zha Jia Su Expressway was connected to 07 km, 01 Provincial Highway through the town and Hangzhou Bay Bridge. The traffic was very convenient. The main business includeed producing and selling key automotive components, metal, and plastic handicrafts for automotive decoration (excluding gold jewelry). This company was a professional production and processing enterprise specializing in designing, researching, and developing key automotive components (excluding engines). The factory covered an area of 2000 m2 and specializes in processing services for various automotive components. The factory not only contributeed to the local economy but also had a significant impact on the surrounding environment. To make reasonable use of land resources and protect human health, this study focused on residents’ health and selects seven typical heavy metals (i.e., Cr, As, Pb, Ni, Cu, Zn, and Cd) for pollution assessment and health risk assessment.
2.2 Sample collection and determination
2.2.1 Soil sampling and pretreatment
In this study, the grid method was used to arrange sampling points to investigate the pollution status of the topsoil in this block, and to determine the distribution of heavy metal pollution concentration in this key block. In addition, further investigation had be conducted on the depth of heavy metal pollution in the soil through additional cross-sectional sampling. Specifically, the southeast downwind area along the perennial wind direction of the factory was 100 m from the edge of the factory. We collected a total of 30 soil samples from top to bottom at vertical depths of 0–10, 10–20, and 20–30 cm, as shown in Figure 1. When sampling, we collected 1-2 kg of soil samples, placed them in a sampling bag, and labeled the sample information and quantity. We brought the samples back to the laboratory and place’d them in a well-ventilated place to dry naturally in the shade. The samples were ground and passed through a 100-m mesh nylon sieve before digestion.
2.2.2 Soil testing
The method of plate digestion was used to measure the total amount of metals in soil. Ground and sieved soil weighing accurately 0.2000 g was placed in a polytetrafluoroethylene crucible. The soil was wet with several drops of deionized water and added with 10 mL of HCl. Then, the soil was heated on an electric heating plate at medium temperature until it was almost dry, and 5 mL HNO3, 5 mL HF, and 3 mL HClO4 were added. The soil was heated at a high temperature until it was almost dry; if there was any residue left, we repeated adding three acids until the solution in the crucible was clear and transparent. Then, the soil was transferred to a 50-mL volumetric flask for constant volume filtration, and an inductively coupled plasma spectrometer was used to analyze soil elements (Xu et al., 2020). The reagents used in the experiment were of superior purity. Heavy metal content was analysis by ICP-MAS measurement. All experimental samples were soaked in 10% dilute nitric acid overnight and then washed with ultrapure water. Blank and parallel samples were conducted throughout the process as control. The recovery rates of each metal were within the allowable range of national standard reference substances. Detection and quality control methods for heavy metals in soil samples were shown in the reference (Hao, 2010; Heng, 2016).
2.3 Evaluation method
2.3.1 Index of geo-accumulation method
This method was proposed by Muller, a German scientist, and is used to quantitatively evaluate the degree of heavy metal pollution in sediments (LI et al., 2012; Lu et al., 2012). In addition to human pollution factors and environmental geochemical background values, the factors of background value changes caused by natural diagenesis are also considered in the evaluation process (Delplace et al., 2022; Zheng et al., 2023).
Where
in the equation:
K is a factor that takes into account possible changes in background values due to differences in rocks in different regions (usually taken as 1.5).
The calculation results are divided into pollution levels according to the evaluation criteria of the index of geo-accumulation (Table 1) (Muller, 1969).
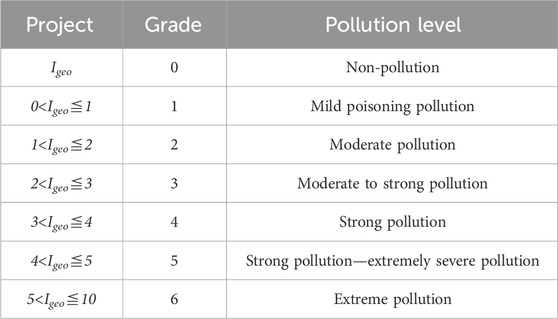
Table 1. Criteria for index of geo-accumulation (Igeo) (Förstner et al., 1993).
2.3.2 Human health risk assessment method
Heavy metals in soil pose a threat to human health through three main pathways: direct inhalation of soil dust into the air through oral and nasal breathing; transferred in the food chain, such as through fruits, vegetables, and grains, through contaminated soil; direct skin contact with contaminated soil with heavy metals (Arisekar et al., 2022; Siddique et al., 2023). The industrial process of surface treatment of this metal generates considerable smoke and dust, which is close to farmlands. All three pathways mentioned above may become the main pathways endangering human health. Therefore, in this study, the non-carcinogenic and carcinogenic risk assessments of heavy metals on human health are all included in the model (Goher et al., 2021).
2.3.2.1 Exposure assessment calculation
The amount of pollution ingested by inhaling soil dust through respiration:
Amount of pollution ingested through direct skin contact with soil:
Direct oral intake of soil pollution:
Total exposure:
In the formula, EDIbreathe, EDIskin, and EDI mouth refer to the total amount of pollutants in soil ingested through respiratory inhalation, skin contact, and direct oral intake, respectively, mg/kg per day; CS is the heavy metal content in the soil, mg/kg; IR soil refers to the soil intake rate, m3/d; IR air is the intake rate of air, m3/d; PEF is the soil dust generation factor m3/kg; SA is the skin contact surface area, cm2/d; AF is the skin’s adsorption coefficient, mg/cm2; ABS is the skin absorption rate, %; EF is the exposure frequency, d/a; ED is the exposure period/a; BW is the body mass, kg; AT is the average action time, d.
The environmental risk assessment standards for adults and children are quite different when conducting exposure assessments. Table 2 shows the values of each exposure assessment parameter in this assessment according to China’s site environmental assessment guidelines, the USEPA health risk assessment method, and the actual research conclusions at home and abroad in recent years (Hasanzadeh et al., 2022; Aly-Eldeen et al., 2023; Hou et al., 2023; Jin et al., 2023; Pandion et al., 2023; Siddique et al., 2023; Zheng et al., 2023).
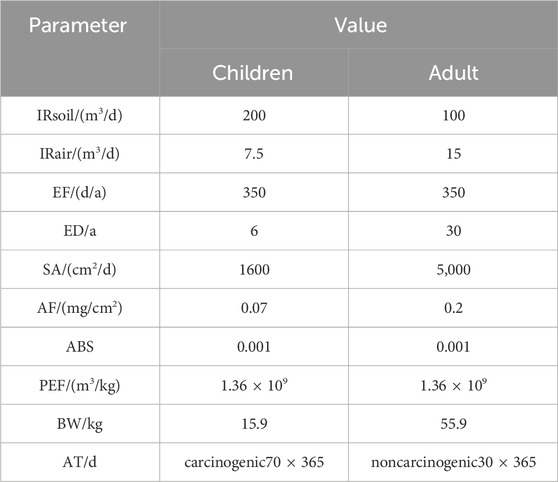
Table 2. The values of exposure parameter (Means, 1989; Palash et al., 2020; Sun et al., 2021).
2.3.2.2 Toxicity assessment and risk characterization
Toxicity assessment estimates the relationship between population exposure to pollutants and the likelihood of negative effects (Bandara and Pathiratne, 2023; Ghosh et al., 2023; Wang et al., 2023). Among the six heavy metals studied in this article, Cu, Pb, Cd, and Ni all have non-carcinogenic health risks, and Pb, Cd, and Ni also have carcinogenic risks (Sharma et al., 2022; Zheng et al., 2023). Table 3 presents the non-carcinogenic and carcinogenic toxicity parameters of four heavy metals, that is, Cu, Pb, Cd, and Ni. The non-carcinogenic toxicity parameters are the reference dose (RfDj) of heavy metals under each exposure pathway, and the carcinogenic effect reference number (SF) is the carcinogenic slope factor of Cd and Ni.
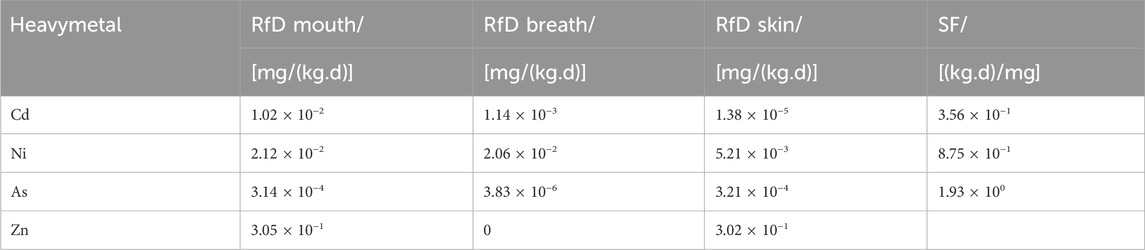
Table 3. RfD and SF of heavy metals for different exposure routes (Huang et al., 2022; Jin et al., 2023).
Each exposure route has carcinogenic and non-carcinogenic risks. The non-carcinogenic risk level can be calculated by dividing the daily exposure of heavy metals by the chronic reference dose of three routes, namely, oral, skin, and respiratory. The calculation formula is as follows:
where HI is the total non-carcinogenic risk level of soil heavy metals under three exposure routes: oral, respiratory, and skin contact; HQi represents the non-carcinogenic risk level of different intake pathways; EDIj is the average daily intake of pollutants from different pathways, mg/(kg·d), whereas RfDj is the chronic reference dose for each pathway, mg/(kg·d) (Table 3). When HQi <1 or HI <1, no significant non-carcinogenic health risk exists. When HQi >1 or HI >1, a non-carcinogenic health risk exists, and the higher the value, the more severe the risk.
The level of cancer risk is calculated by multiplying the average daily intake to the entire life cycle by the slope coefficient of carcinogenicity through oral, skin, or respiratory inhalation. The calculation formula is as follows:
where Riski is the carcinogenic risk index of soil heavy metals under different pathways; (Risk) T is the comprehensive risk index of heavy metal carcinogenesis in soil; EDI is the average daily intake of different pollutants, mg/(kg·d); SFi is the slope coefficient of cancer risk for various pathways, (kg·d)/mg (Table 3). Risk is the carcinogenic health index, usually represented by a certain number of recognized cancer patients. The acceptable risk value of carcinogens defined by the U.S. Environmental Protection Agency is 10−4–10−6, and the risk of cancer incidence in a lifetime exceeds the normal value. When the risk is < 1 × 10−6 h, no risk of cancer exists. When the risk is > 1 × 10−4 h, a risk of cancer exists. Then, when 1 × 10−6 ≤ risk ≤1 × 10−4 h, the risk of cancer is considered within an acceptable range.
3 Results and discussion
3.1 Current situation and source analysis of heavy metal pollution
In this survey, the downwind soil around the auto parts company in Jiaxing was collected in three layers, and a total of 30 sampling data were analyzed regionally. According to the average value of the soil in the Hangzhou–Jiaxing–Huzhou Plain as the background value and the standard values of various elements (Meng et al., 2022; Shao-cheng et al., 2023; Xu et al., 2023), specified in the secondary standard of the Soil Environmental Quality Standard GB 15618-1995 (pH < 6.5), the pollution degree of heavy metals can be simply and intuitively displayed by using the multiple of exceeding the standard. The coefficient of variation reflects the interference of human activities on heavy metal content (Xiaogang, 2014). The average values of heavy metal content in the downwind direction of the study area and the heavy metal content at three different sampling depths were measured (Halim, 2023). The objective is to investigate the overall pollution status of heavy metals around Jiaxing Automotive Parts Company and the impact of their location and distance from the pollution source. Table 4; Figure 2 show the results.
Table 4 shows that, compared with the soil environmental quality table issued in 2018, Cd, As, Pb, Cr, Cu, Ni, and Zn have all exceeded the standard, with exceeding rates of 100%, 100%, 63%, 10%, 13%, 57%, and 100%, respectively. Among them, the maximum coefficient of variation of As reached 63.38%, indicating that the distribution of As in the study area is very uneven and that the amplitude changes are significant. Second, the coefficient of variation of Cd, Cr, and Ni also reached 50%, whereas that of Zn was 39.38%. The distribution of several heavy metals was also uneven, whereas the coefficient of variation of Pb was the lowest. Relatively speaking, the distribution was relatively uniform.
Research has shown that heavy metals in soil are not only related to species but also to the relative location and distance of pollution sources. In this study, the As content of sampling points 1, 2, 3, 4, and 5 is particularly high, and these five points are located approximately 1 km below the air outlet of the factory area. On this basis, the closer you are to the factory area, the higher the concentration of heavy metals deposited in the soil. The content of As in all directions and locations exceeds the background value of soil in Jiaxing City (LI et al., 2012; Shen et al., 2017). Moreover, the content of As in the middle layer of 10–20-cm soil reaches the maximum value, with an average exceeding the standard of 79.39 times. This case may be caused by the disordered discharge of arsenic-containing wastewater and the atmospheric sedimentation of exhaust gas generated by the nearby automobile beauty enterprises in the production process. In addition, using organic arsenic pesticides to control rice diseases has caused a large amount of arsenic residue. The simple substance arsenic is non-toxic, and Arsenic compounds are toxic. Trivalent arsenic is approximately 60 times more toxic than pentavalent arsenic. The toxicity of organic arsenic is similar to that of inorganic arsenic. Moreover, long-term inhalation or oral administration in small amounts can lead to chronic poisoning (Li et al., 2023). Meanwhile, breathing arsenic-containing air for 5–10 min can lead to fatal poisoning. The presence of arsenic in agricultural soil is a major environmental and public health issue. Arsenic, an element that occurs naturally, can be introduced into soils by human activities such as mining, pesticide application, and industrial operations. Arsenic, when found in the soil, has the ability to build up in crops, posing a threat to the safety of our food. Prolonged exposure to arsenic through tainted agricultural produce has been associated with many health problems, such as dermatological abnormalities, cardiovascular disorders, and specific types of cancer (Dong-Yan, 2018). Arsenic pollution can cause toxicity in the gastrointestinal tract, liver, and kidneys and induce cancer. Hence, effective measures must be taken to prevent and treat it. In addition to As, the average value of Cd also exceeded 8.54 times, exhibiting the same distribution pattern as As, reaching its peak in the soil layer at 10–20 cm. Cd is a non-biological essential element that can enter the human body through the food chain and accumulate in the body, causing chronic poisoning, liver and kidney damage, and bone metabolism obstruction (Jin et al., 2023). Therefore, special attention is also needed. The exceeding standard rate of Zn reached 100%, which was higher than the soil background value in Jiaxing City. This situation may be due to proximity to highways, vehicle exhaust emissions, forum wear and tear, and corporate exhaust emissions exceeding the standard. Pb and Cu are similar in average bidding multiples, similar to the soil background values of Jiaxing City (Gao et al., 2023; Siddique et al., 2023). Cr has the lowest exceeding standard rate and average exceeding standard multiple among the seven tested heavy metals, which may be related to the layout of drainage channels (Zheng et al., 2023). The dominant wind direction in Jiaxing is southeast wind all year round, and in this study, the overall direction of heavy metal production and enrichment is 100 m southwest rather than in the downwind region. Zhao Renxin et al. (Zhao et al., 2017) concluded that the influence of wind direction on the distribution of heavy metals is not significant. The main reasons for this phenomenon may be threefold: interference from other enterprises’ emissions, interference from agricultural pesticides, and atmospheric sedimentation caused by wind power. The airflow disturbance caused by vehicles traveling to the west of the factory area weakens the role of natural wind direction.
3.2 Index of geo-accumulation
Figure 3 shows the overall evaluation of six heavy metals in the soil around the metal factory using the land accumulation index method and the evaluation results of each metal at different vertical depths.
From Figure 3, the cumulative index (Igeo) of heavy metals around the metal surface treatment plant is Cd > As > Cu > Zn > Pb > Ni > Cr in descending order. The ground accumulation index of the middle soil layer (10–20 cm) is mostly at a high level, which may be the result of surface infiltration downward. Many deep soil layers (20–30 cm) have a higher ground accumulation index than the surface soil, indicating that the deep soil is also severely polluted. The highest ground accumulation index of As and Cd is above 5.8, and all soils are contaminated with varying degrees of As and Cd. Surface and deep soils are at extremely severe pollution levels. Second, the overall pollution of Cu and Zn is between strong and moderate pollution levels, with a high degree of soil pollution in the layer (10–20 cm). The overall pollution level of Pb is at a moderate level. Compared with other heavy metals, the pollution level of Cr is the lightest. The pollution decreases with the deepening of soil depth, which may be because of the adsorption and diffusion of soil particles (Pandion et al., 2023). As shown in Figure 3, out of the seven heavy metals measured in this study, six are all at a moderate or higher pollution level. Considering their potential harm to surrounding residents, As, Zn, and Cd were selected as risk factors for human health risk assessment.
3.3 Health risk assessment
3.3.1 Exposure assessment analysis
The exposure assessment of As, Zn, and Cd on children and adults on non-carcinogenic days was carried out in this study, and Table 5 shows the results. From Table 5, the intake of heavy metals through oral intake is much higher than that through skin contact and respiratory inhalation. The daily intake of heavy metals in soil through the three pathways is in descending order: EDI through mouth > EDI through skin > EDI through respiration. Children’s intake of heavy metals through oral intake is higher than that of adults, but the content of heavy metals through skin contact and respiratory inhalation is lower than that of adults. The exposure dose reaches its maximum when children ingest As through mouth, 3.05 × 10−3 mg/(kg/d), and the minimum exposure dose appears in children who inhale Cd through breathing, with a minimum value of 2.85 × 10−11 mg/(kg/d). The doses of the other two metals Zn and Cd ingested by mouth, skin contact, and respiratory inhalation in adults are greater than those in children.
3.3.2 Healthy risk assessment
In this study, non-carcinogenic daily exposure assessment of three heavy metals, As, Zn, and Cd, to children and adults was carried out, and the results are shown in Table 6. From Table 6, the non-carcinogenic risk index (HQi) of the surrounding soil of Jiaxing Minhui Automotive Parts Company is partially greater than 1. Relatively speaking, the risk of oral intake is the highest, and the non-carcinogenic risk of direct skin contact and respiratory inhalation is relatively small. These results are consistent with the health risk assessment conclusion of heavy metals in subway station dust, which is the research background of Yang Xiaozhi (Yang et al., 2022). The maximum occurrence of HQi is in children’s oral intake of As, with a maximum value of 12.34, whereas the minimum value is the respiratory intake of heavy metal Zn by children, with a minimum value of 7.56 × 10−9. In addition, the doses of Cd and Zn for oral intake, skin contact, and respiratory inhalation in adults are higher than those in children. This result is consistent with the conclusions of the exposure assessment, indicating that the non-carcinogenic risk index is related to the exposure route (Meng et al., 2022). Regardless of the exposure pathway, the non-carcinogenic health risk assessment of As is higher than that of the other two heavy metals. Therefore, As has the greatest potential for carcinogenic health risk, with a total HQi of 12 for non-carcinogenic health risks, followed by Zn. Therefore, the prevention and control of these two elements should be strengthened. In addition, the total non-carcinogenic health risk HQi of Cd for adults and children is less than 1, indicating that these elements do not pose a non-carcinogenic health risk to residents around the factory area. Fadel et al. (Fadel et al., 2022) studied the soil pollution situation around electroplating plants and also found that the carcinogenic risk of As and Cr in the soil was greater than 10−4, which was higher than the maximum acceptable risk level, similar to the results of this study.
The maximum values of the carcinogenic health risk index (RISK) of As for adults and children under the exposure pathway of oral intake are 6.30 × 10−4 and 8.83 × 10−4, with a risk of cancer. The minimum value of the carcinogenic health risk index of Cd is 1.46 × 10−10, occurring in children through respiratory exposure pathways, within an acceptable range of carcinogenic risk. The maximum values of Cd all occur through the oral intake pathway in children, with values of 5.28 × 10−6. Zn does not have a carcinogenic risk. Overall, the total cancer risk index of As through three pathways is relatively high, and risks in children are higher than in adults, which should be highly valued and strengthened for prevention and control. The excessive levels of As may be related to the application of pesticides in farmland or to nearby agricultural companies (Fan et al., 2022; Hou et al., 2023; Shao-cheng et al., 2023). As for Cd, risks in adults are higher than those in children, which is consistent with the results of the non-carcinogenic risk index. The health risk index for cancer caused by oral intake in adults is 5.28 × 10−6, has exceeded the warning value, and if not controlled, will pose a threat to the health of surrounding residents.
4 Conclusion
1) Many kinds of heavy metal pollution were detected in the leeward soil of Jiaxing Minhui Auto Parts Factory; the soil was extremely polluted by As and Cd, and the exceeding standard rates are 100% and 37%, respectively. Moreover, the As content can reach 1994.7 mg/kg, exceeding the standard by 34.287 times. Such pollution is unevenly distributed in the soil. The pollution level of Zn is 3, belonging to the moderate pollution level. The average exceeding multiple of Zn is not high, but the exceeding rate reaches 100%. The level of heavy metal pollution in the soil decreases as the distance from the downwind mouth of the factory increases, but some do not follow this pattern. Overall, this area is polluted by a mixture of As, Cd, and Zn, similar to the heavy metal pollution in agricultural soil in Zhejiang Province. The coefficient of variation of As reached 63.38%, possibly because of the use of arsenic-containing fertilizers and pesticides causing metal residues.
2) The conclusion drawn from the ground accumulation index method is that As and Cd pollution is the most severe, with a grading result of 6 levels, belonging to the extremely strong pollution level. Pb, Cu, and Zn are at levels 3, 4, and 3, respectively, belonging to the medium strong pollution level. The ground accumulation index of Cr and Ni is at level 2, belonging to the moderate pollution level. When the distance between the lower air vents in the factory area is close and the pollution level high, the soil in this area is highly polluted with As and Cd, accompanied by moderate to high levels of Pb, Cu, and Zn pollution. Moreover, the relevant parts should be taken seriously, and efforts should be made to address the pollution of heavy metals on the soil to ensure people’s lives and health.
3) The daily intake of heavy metals in the soil through three pathways, in descending order, is EDI through mouth > EDI through skin > EDI through respiration. The non-carcinogenic risk index is related to the exposure pathway. The total carcinogenic risk index of As in the study area through three exposure pathways is relatively high, whereas the carcinogenic harm of Zn is within an acceptable range. However, a potential carcinogenic risk that should be highly valued and prevented already exists.
Data availability statement
The original contributions presented in the study are included in the article/Supplementary material, further inquiries can be directed to the corresponding author.
Author contributions
TTL: Writing–original draft, Writing–review and editing. SYN: Data curation, Formal Analysis, Methodology, Writing–review and editing. ZW: Funding acquisition, Investigation, Resources, Visualization, Writing–review and editing.
Funding
The author(s) declare that financial support was received for the research, authorship, and/or publication of this article. I would like to express my gratitude to the supported by the Open Fund of the Key Laboratory of Waste minimisation technology Research of Zhejiang Province (No. 2021ZEKL10).
Conflict of interest
Author SN was employed by Zongsheng Design Group Ltd. of Zhejiang Province.
The remaining authors declare that the research was conducted in the absence of any commercial or financial relationships that could be construed as a potential conflict of interest.
Publisher’s note
All claims expressed in this article are solely those of the authors and do not necessarily represent those of their affiliated organizations, or those of the publisher, the editors and the reviewers. Any product that may be evaluated in this article, or claim that may be made by its manufacturer, is not guaranteed or endorsed by the publisher.
References
Adotey, E. K., Burkutova, L., Tastanova, L., Bekeshev, A., Balanay, M. P., Sabanov, S., et al. (2022). Quantification and the sources identification of total and insoluble hexavalent chromium in ambient PM: a case study of Aktobe, Kazakhstan. Chemosphere 307, 136057. doi:10.1016/j.chemosphere.2022.136057
Aly-Eldeen, M. A., Shreadah, M. A., and Abdel Ghani, S. A. (2023). Distribution, bioavailability, and ecological risk assessment of potentially toxic heavy metals in El-Burullus Lake sediments, Egypt. Mar. Pollut. Bull. 191, 114984. doi:10.1016/j.marpolbul.2023.114984
Arisekar, U., Shakila, R. J., Shalini, R., Jeyasekaran, G., Keerthana, M., Arumugam, N., et al. (2022). Distribution and ecological risk assessment of heavy metals using geochemical normalization factors in the aquatic sediments. Chemosphere 294, 133708. doi:10.1016/j.chemosphere.2022.133708
Bábek, O., Grygar, T. M., Faměra, M., Hron, K., Nováková, T., and Sedláček, J. (2015). Geochemical background in polluted river sediments: how to separate the effects of sediment provenance and grain size with statistical rigour? CATENA 135, 240–253. doi:10.1016/j.catena.2015.07.003
Bandara, S., and Pathiratne, A. (2023). Concentrations of trace metals in Siganus javus captured in Negombo estuary, Sri Lanka: human health risk assessment through dietary exposure. Mar. Pollut. Bull. 188, 114639. doi:10.1016/j.marpolbul.2023.114639
Chen, Y., Zhang, Q., Luo, T., Xing, L., and Xu, H. (2019). Occurrence, distribution and health risk assessment of organophosphate esters in outdoor dust in Nanjing, China: urban vs rural areas. Chemosphere 231, 41–50. doi:10.1016/j.chemosphere.2019.05.135
Cheng, S., Liu, P., Hou, X., Guo, X., Li, G., Yang, F., et al. (2023). Copper and mercury exposure alters rectum microbiota in female adult mice. J. King Saud Univ. - Sci. 35 (6), 102776. doi:10.1016/j.jksus.2023.102776
Delplace, G., Viers, J., and Oliva, P. (2022). This letter is a response to the comment submitted to chemosphere by Melleton et al. on our paper (Delplace et al., 2022), entitled “pedo-geochemical background and sediment contamination of metal(loid)s in the old mining-district of Salsigne (Orbiel valley, France)” by Gauthier Delplace, Jérôme Viers, Eva Schreck, Priscia Oliva and Philippe Behra (2022), published online in Chemosphere in September 2021. Chemosphere 307, 135766. doi:10.1016/j.chemosphere.2022.135766
Diwa, R. R., Elvira, M. V., Deocaris, C. C., Fukuyama, M., and Belo, L. P. (2022). Transport of toxic metals in the bottom sediments and health risk assessment of Corbicula fluminea (Asiatic clam) collected from Laguna de Bay, Philippines. Sci. Total Environ. 838, 156522. doi:10.1016/j.scitotenv.2022.156522
Dong-Yan, R. (2018). Association between arsenic exposure and diabetes mellitus in rats. J. Environ. Occup. Med. 2018.
Fadel, M., Ledoux, F., Afif, C., and Courcot, D. (2022). Human health risk assessment for PAHs, phthalates, elements, PCDD/Fs, and DL-PCBs in PM2.5 and for NMVOCs in two East-Mediterranean urban sites under industrial influence. Atmos. Pollut. Res. 13 (1), 101261. doi:10.1016/j.apr.2021.101261
Fan, T., Pan, J., Wang, X., Wang, S., and Lu, A. (2022). Ecological risk assessment and source apportionment of heavy metals in the soil of an Opencast mine in xinjiang. Int. J. Environ. Res. Public Health 19 (23), 15522. doi:10.3390/ijerph192315522
Förstner, U., Ahlf, W., and Calmano, W. (1993). Sediment quality objectives and criteria development in Germany. Water Sci. Technol. 28 (8-9), 307–316. doi:10.2166/wst.1993.0629
Gao, X., Zhou, Y., Fan, M., Jiang, M., Zhang, M., Cai, H., et al. (2023). Environmental risk assessment near a typical spent lead-acid battery recycling factory in China. Environ. Res. 233, 116417. doi:10.1016/j.envres.2023.116417
Ghosh, S., Banerjee, S., Prajapati, J., Mandal, J., Mukherjee, A., and Bhattacharyya, P. (2023). Pollution and health risk assessment of mine tailings contaminated soils in India from toxic elements with statistical approaches. Chemosphere 324, 138267. doi:10.1016/j.chemosphere.2023.138267
Goher, M. E., Mangood, A. H., Mousa, I. E., Salem, S. G., and Hussein, M. M. (2021). Ecological risk assessment of heavy metal pollution in sediments of Nile River, Egypt. Environ. Monit. Assess. 193 (11), 703. doi:10.1007/s10661-021-09459-3
Halim, T. (2023). Comments on “Economics of natural disasters and technological innovations in Africa: an empirical evidence” by Okolo, Chukwuemeka et al., DOI (10.1007/s11356-022-22989-8). Environ. Sci. Pollut. Res. Int. 30 (10), 122974–122975. doi:10.1007/s11356-023-30873-2
Hao, C. (2010). The monitoring process of heavy metals in soils and its quality control measures. Environ. Monit. China. doi:10.3724/SP.J.1088.2010.00432
Hasanzadeh, M., Malakootian, M., Nasiri, A., Oliveri Conti, G., Ferrante, M., and Faraji, M. (2022). Ecological and probabilistic health risk assessment of heavy metals in topsoils, southeast of Iran. Bull. Environ. Contam. Toxicol. 108 (4), 737–744. doi:10.1007/s00128-021-03389-z
Hou, Y., Zhao, Y., Lu, J., Wei, Q., Zang, L., and Zhao, X. (2023). Environmental contamination and health risk assessment of potentially toxic trace metal elements in soils near gold mines – a global meta-analysis. Environ. Pollut. 330, 121803. doi:10.1016/j.envpol.2023.121803
Huang, Y., Han, R., Qi, J., Duan, H., Chen, C., Lu, X., et al. (2022). Health risks of industrial wastewater heavy metals based on improved grey water footprint model. J. Clean. Prod. 377, 134472. doi:10.1016/j.jclepro.2022.134472
Jin, J., Zhao, X., Zhang, L., Hu, Y., Zhao, J., Tian, J., et al. (2023). Heavy metals in daily meals and food ingredients in the Yangtze River Delta and their probabilistic health risk assessment. Sci. Total Environ. 854, 158713. doi:10.1016/j.scitotenv.2022.158713
Kwon, J. C., Lee, J., and Jung, M. C. (2012). Arsenic contamination in agricultural soils surrounding mining sites in relation to geology and mineralization types. Appl. Geochem. 27 (5), 1020–1026. doi:10.1016/j.apgeochem.2011.11.015
Li, N., Li, Y., Wei, J., Liu, K., Wang, G., Zhang, H., et al. (2023). Source-oriented ecological risk assessment of heavy metals in sediments of West Taihu Lake, China. Environ. Sci. Pollut. Res. Int. 30 (6), 13909–13919. doi:10.1007/s11356-022-24766-z
Liang, Q., Tian, K., Li, L., He, Y., Zhao, T., Liu, B., et al. (2022). Ecological and human health risk assessment of heavy metals based on their source apportionment in cropland soils around an e-waste dismantling site, Southeast China. Ecotoxicol. Environ. Saf. 242, 113929. doi:10.1016/j.ecoenv.2022.113929
Liverpool, L. (2021). Legal levels of lead in US tap water can still cause harm. New Sci. 251 (3344), 21. doi:10.1016/s0262-4079(21)01285-9
Li, X., Yin, H., and Su, J. (2012). An attempt to quantify Cu-resistant microorganisms in a paddy soil from jiaxing, China. Pedosphere 22 (2), 201–205. doi:10.1016/s1002-0160(12)60006-x
Lu, J., Jiang, L., Chen, D., Toyota, K., Strong, P. J., Wang, H., et al. (2012). Decontamination of anaerobically digested slurry in a paddy field ecosystem in Jiaxing region of China. Agric. Ecosyst. Environ. 146 (1), 13–22. doi:10.1016/j.agee.2011.10.011
Means, B. (1989). Risk-assessment guidance for superfund. Human Health Evaluation Manual. Part A. Interim report (Final) 1. Available at: https://www.osti.gov/biblio/350784.
Meng, C., Wang, P., Hao, Z., Gao, Z., Li, Q., Gao, H., et al. (2022). Ecological and health risk assessment of heavy metals in soil and Chinese herbal medicines. Environ. Geochem Health 44 (3), 817–828. doi:10.1007/s10653-021-00978-z
Muller, G. (1969). Index of geoaccumulation in sediments of the rhine river. GeoJournal 2 (3), 109–118.
Palash, M. A. U., Islam, M. S., Bayero, A. S., Taqui, S. N., and Koki, I. B. (2020). Evaluation of trace metals concentration and human health implication by indigenous edible fish species consumption from Meghna River in Bangladesh. Environ. Toxicol. Pharmacol. 80, 103440. doi:10.1016/j.etap.2020.103440
Pandion, K., Arunachalam, K. D., Rajagopal, R., Ali, D., Alarifi, S., Chang, S. W., et al. (2023). Health risk assessment of heavy metals in the seafood at Kalpakkam coast, Southeast Bay of Bengal. Mar. Pollut. Bull. 189, 114766. doi:10.1016/j.marpolbul.2023.114766
Pojar, I., Kochleus, C., Dierkes, G., Ehlers, S. M., Reifferscheid, G., and Stock, F. (2021). Quantitative and qualitative evaluation of plastic particles in surface waters of the Western Black Sea. Environ. Pollut. 268, 115724. doi:10.1016/j.envpol.2020.115724
Shao-cheng, S., Yu-cheng, W., Yuan, L., Shuai, Y., Xiao-hong, P., and Yong-ming, L. (2023). Divergent soil health responses to long-term inorganic and organic fertilization management on subtropical upland red soil in China. Ecol. Indic. 154, 110486. doi:10.1016/j.ecolind.2023.110486
Sharma, K., Janardhana Raju, N., Singh, N., and Sreekesh, S. (2022). Heavy metal pollution in groundwater of urban Delhi environs: pollution indices and health risk assessment. Urban Clim. 45, 101233. doi:10.1016/j.uclim.2022.101233
Shen, L., Wang, H., Lü, S., Zhang, X., Yuan, J., Tao, S., et al. (2017). Influence of pollution control on air pollutants and the mixing state of aerosol particles during the 2nd World Internet Conference in Jiaxing, China. J. Clean. Prod. 149, 436–447. doi:10.1016/j.jclepro.2017.02.114
Siddique, S., Chaudhry, M. N., Ahmad, S. R., Nazir, R., Zhao, Z., Javed, R., et al. (2023). Ecological and human health hazards; integrated risk assessment of organochlorine pesticides (OCPs) from the Chenab River, Pakistan. Sci. Total Environ. 882, 163504. doi:10.1016/j.scitotenv.2023.163504
Sun, X., Zhang, L., and Lv, J. (2021). Spatial assessment models to evaluate human health risk associated to soil potentially toxic elements. Environ. Pollut. 268, 115699. doi:10.1016/j.envpol.2020.115699
Wang, J., Chen, Y., Pan, D., Zhang, J., Zhang, Y., and Lu, Z. (2023). Source and health risk assessment of soil polycyclic aromatic hydrocarbons under straw burning condition in Changchun City, China. Sci. Total Environ. 894, 165057. doi:10.1016/j.scitotenv.2023.165057
Xiaogang, Y. (2014). Variation of heavy metal concentrations in hyporheic sediments for the Weihe River of Shaanxi Province. Acta Sci. Circumstantiae.
Xu, D., Chen, S., Xu, H., Wang, N., Zhou, Y., and Shi, Z. (2020). Data fusion for the measurement of potentially toxic elements in soil using portable spectrometers. Environ. Pollut. 263, 114649. doi:10.1016/j.envpol.2020.114649
Xu, G., Liu, J., Pei, S., Hu, G., and Kong, X. (2016). Sources and geochemical background of potentially toxic metals in surface sediments from the Zhejiang coastal mud area of the East China Sea. J. Geochem. Explor. 168, 26–35. doi:10.1016/j.gexplo.2016.06.003
Xu, H., Huang, Y., Xiong, X., Zhu, H., Lin, J., Shi, J., et al. (2023). Changes in soil Cd contents and microbial communities following Cd-containing straw return. Environ. Pollut. 330, 121753. doi:10.1016/j.envpol.2023.121753
Yang, J., Sun, F., Su, H., Tao, Y., and Chang, H. (2022). Multiple risk assessment of heavy metals in surface water and sediment in taihu lake, China. Int. J. Environ. Res. Public Health 19 (20), 13120. doi:10.3390/ijerph192013120
Zhang, J., Peng, W., Lin, M., Liu, C., Chen, S., Wang, X., et al. (2023). Environmental geochemical baseline determination and pollution assessment of heavy metals in farmland soil of typical coal-based cities: a case study of Suzhou City in Anhui Province, China. Heliyon 9 (4), e14841. doi:10.1016/j.heliyon.2023.e14841
Zhao, B., Han, L., Pilz, J., Wu, J., Khan, F., and Zhang, D. (2017). Metallogenic efficiency from deposit to region–A case study in western Zhejiang Province, southeastern China. Ore Geol. Rev. 86, 957–970. doi:10.1016/j.oregeorev.2016.10.003
Keywords: automobile industry, heavy metal contamination, index of geoaccumulation, human health risk assessment, risk assessment
Citation: Liu T, Ni SY and Wang Z (2024) Contamination and health risk assessment of heavy metals in soil surrounding an automobile industry factory in Jiaxing, China. Front. Environ. Sci. 12:1362366. doi: 10.3389/fenvs.2024.1362366
Received: 28 December 2023; Accepted: 25 March 2024;
Published: 09 April 2024.
Edited by:
Peng Shi, Xi’an University of Technology, ChinaReviewed by:
Bayram Yuksel, Giresun University, TürkiyeYuanpeng Wang, Xiamen University, China
Jiang Yu, Sichuan University, China
Copyright © 2024 Liu, Ni and Wang. This is an open-access article distributed under the terms of the Creative Commons Attribution License (CC BY). The use, distribution or reproduction in other forums is permitted, provided the original author(s) and the copyright owner(s) are credited and that the original publication in this journal is cited, in accordance with accepted academic practice. No use, distribution or reproduction is permitted which does not comply with these terms.
*Correspondence: Tingting Liu, bGl1c2hhQHpqdS5lZHUuY24=