- Department of Agronomy, University of Fort Hare, Alice, South Africa
Prescribed fire frequencies have been widely used to reduce the risk of severe wildfire occurrences. In addition, several studies have been conducted to assess the impact of fire frequencies on vegetation, vertebrate, and invertebrate species, as well as soil physical and chemical properties. However, there is a lack of empirically based knowledge concerning the impact of fire frequency on soil microorganisms. This study assessed the effect of different fire frequencies on the diversity and composition of soil fungal communities in a semi-arid savanna rangeland. Soil samples were collected from an ongoing long-term trial at the University of Fort Hare (South Africa) on the following treatments: (i) no burning; (ii) annual burning (burned once every year); (iii) biennial burning (burned once every 2 years); (iv) triennial burning (burned once every 3 years); (v) quadrennial burning (burned once every 4 years); and (vi) sexennial burning (burned once every 6 years). Fungi were identified using high-throughput sequencing, with Shannon-Wiener and Inverse Simpson diversity indexes being used for diversity and network analysis. Principal coordinate analysis was used for Bray-Curtis distance matrices to visualise the relationships between treatments. The highest diversity was found in biennial burning, which was significantly different (p < 0.05) from the sexennial, quadrennial, and no burning treatments but was not different from the triennial and annual burning treatments. Regarding the taxa, Ascomycota and Basidiomycota were the phyla with the highest relative abundance, followed by Mortierellomycota, Chytridiomycota, and Rozellomycota. The different fire frequencies had an influence on soil fungi diversity and taxonomic composition in semi-arid savanna rangelands.
1 Introduction
Fire regimes are prevalent worldwide, and have been ascribed mostly to climate change, which results in rising temperatures and exacerbated droughts (Bowman et al., 2020; Bowd et al., 2022). Whitman et al. (2019) anticipated that the frequency and intensity of wildfires in various biomes would rise during the next 100 years. In South Africa, about 1.3 million ha of land are burnt annually (Russell-Smith et al., 2021), resulting in economic losses ranging between $123–215 million USD (Strydom and Savage, 2016). Consequently, a total of about 126, 000 fire incidences were recorded across South Africa in 2021, and fires mainly occurred in the Eastern Cape, North-West, and Mpumalanga provinces (Madondo et al., 2022). As a result, these incidences hamper the fulfilment of Sustainable Development Goals (SDG) 13 of Climate Action, which calls for immediate action to address climate change and its consequences, and SDG 15 of Life on Land, which aims to preserve the environment and save soils (United Nations, 2020).
Nevertheless, the extent of fire varies according to the type of biome. Among nine biomes found in South Africa, the savanna is the most fire-prone biome, constituting about 24.1% of the burnt area (Russell-Smith et al., 2021). It is imperative to note that fire is an important tool used in restructuring and managing savanna biomes due to their characterized mixture of grasses and scattered trees (Smith et al., 2013; Southworth et al., 2016). In this biome, fire is an important ecological factor for maintaining ecosystems and balancing competition among woody and herbaceous species (Ribeiro et al., 2019). Furley et al. (2008) deduced that savanna vegetation is typically fire-adapted and resilient. However, the increase in fire severity has resulted in the restructuring of vegetation and soil microbial communities. Thus, leading to long-lasting severe effects on ecosystem health and functions (de Groot et al., 2013).
Prescribed burning has been widely used to reduce the risk of severe wildfire occurrence (Fernández et al., 2013). Several studies have reported the effects of different prescribed burning frequencies on vegetation, vertebrate, and invertebrate species, as well as soil physical and chemical properties in the semi-arid savanna (Oluwole et al., 2008; Williams et al., 2012; Snyman, 2015; Holden et al., 2016; Madikana, 2022). For example, Madikana (2022) investigated the impact of various fire burning frequencies on the recovery of soil invertebrate communities in a semi-arid region of the Eastern Cape Province (South Africa) and observed a decrease in the abundance and diversity of earthworms, ants, and other ground-dwelling insect species. Another study conducted on savanna ecological zones in Ghana showed that anthropogenic fires increased soil pH, which had a positive impact on soil biological recovery (Amoako and Gambiza, 2019). Furthermore, it was demonstrated that fire critically altered soil physicochemical properties like changes in soil albedo and deterioration of nutrient pools (Magomani and van Tol, 2019). Fire can have a positive impact on soil nutrients P as burning transforms the organic P pool into orthophosphate, this is the only type of P accessible to biota, with P bioavailability being around the neutral pH range (Certain, 2005).
However, there is little knowledge concerning the impact of fire frequency on soil microorganisms (Fontúrbel et al., 2012; Certini et al., 2021). In all the soil microorganisms, fungi are the most susceptible to heat, and this could cause a change in ecosystem processes conducted by fungi (Dove et al., 2019). For instance, Vermeire et al. (2021) showed that species diversity was relatively lower in fungi than bacteria after various prescribed fire regimes. This is because microbes such as bacteria are relatively more tolerant of direct fire than fungi (Pulido-Chavez et al., 2023). However, fire causes a decrease in nutrient availability, such as carbon (C), nitrogen (N), and other organic substances like cellulose, which rapidly affect fungi (Adkins et al., 2020; Srikanthasamy et al., 2021). In terms of biomass and physiological activity, fungi are typically ranked as the most prevalent soil microorganisms (Kjoller and Struwe, 1982). They are abundant in the ecosystem and perform a variety of critical ecological tasks, such as nitrogen and carbon cycling and soil stabilization (Christensen, 1989; Boer et al., 2005; Janion-Scheepers et al., 2016). In addition to being decomposers, fungi are also saprobionts, mutualists, and parasites (Christensen, 1989; Challacombe et al., 2019). Fire regimes consequently affect nutrient cycling, and fungi play significant roles in nutrient cycles through nutrient transformations and enhancing plant-soil nutrient dynamics, mostly through symbiotic mycorrhizal fungi that enhance phosphorus absorption (Agbeshie et al., 2022).
Fire has direct effects on fungi through combustion and elevated temperatures, which cause mortalities (Dooley and Treseder, 2012; Nyamadzawo et al., 2013; Barreiro et al., 2016; Liu et al., 2023). Available evidence showed that savanna fires, which usually occur between 100°C and 700°C, are fatal to fungal communities, and their mortalities start happening as soon as the temperature reaches 60°C (Hansen et al., 2019). Indirect alteration of other soil properties makes it difficult for microbes to survive, which can happen through loss of habitat as well as food and energy (Singh et al., 2021). However, due to regular fire occurrence in savanna biomes, recurrent and frequent fires significantly shape the pre- and post-fire abundance of fungi in the soil (Hansen et al., 2019).
Considering the available evidence on how fire affects soil microbial activity, little is known about the effect of different prescribed fire frequencies on fungal diversity and community composition, particularly in the savanna rangeland. Also, the mechanisms that fungi use during their recovery process after fires require further research. In 1980, the University of Fort Hare in South Africa initiated a long-term trial aimed at evaluating the impact of different fire burning frequencies on various ecological indicators (Trollope, 1984). Since its establishment, the studies on this trial have been narrowly focused on the effect of fire on vegetation, with soil microorganisms such as fungi, which play a critical role in ecosystem function, being ignored. Therefore, the current study was conducted in a similar trial with the intention of assessing the effect of different fire burning frequencies on the diversity and taxonomic composition of soil fungal microbiota in a semi-arid savanna rangeland. It is hypothesized that soil microbial composition and diversity will be influenced by various fire frequencies.
2 Materials and methods
2.1 Site description, experimental design and sampling
The study was carried out during August 2022 (summer season) at the Honeydale part of the University of Fort Hare Research Farm, which is located 3 km outside of Alice town (32° 47′ 58″S and 26° 52′ 26″E) in South Africa’s Eastern Cape Province (Figure 1). This region is within the semi-arid savanna rangelands, with an annual average rainfall of between 450 and 550 mm (SAWS, 2021). The maximum temperatures range between 26°C and 41°C in July and January, respectively, while the minimum temperatures range from −5–11 °C in July and January, respectively (Metablue, 2022). The vegetation is characterized by the false thornveld of the Eastern Cape (Magomani and van Tol, 2019), which consists of grasses interspersed with Acacia karoo Hayne shrubs in some areas. The rangeland is a good environment for livestock production purposes and consists of dense sward that is dominated by Themeda triandra, Panicum maximum, Digitaria eriantha, and Sporobolus species (Acocks, 1975; Mucina and Rutherford, 2006). The soil is a Eutric Cambisol (IUSS Working Group WRB, 2022).
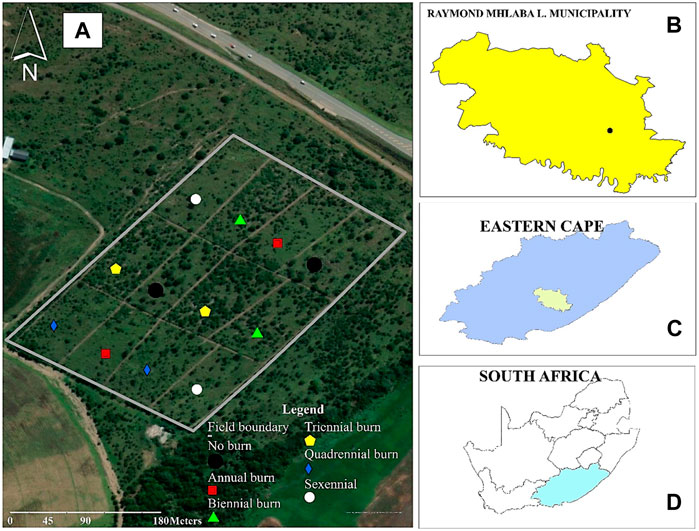
FIGURE 1. Experimental site layout with different fire treatments at the University of Fort Hare Research farm (Alice, South Africa). (A) = map of the experimental site showing burning frequencies; (B) = map of Raymond Mhlaba Municipality; (C) = map of Eastern Cape Province; (D) = map of South Africa.
The research was carried out as part of a long-term ongoing burning trial initiated in 1980 at the University of Fort Hare in Alice (Figure 1) to evaluate the impact of burning frequency on species composition and biomass production (Trollope, 1984). The treatments used included i) no burning (K), ii) annual burning (B1), iii) biennial burning (B2), iv) triennial burning (B3), v) quadrennial burning (B4), and vi) sexennial burning (B6) (see Supplementary Table S1). The treatments are replicated twice on plots measuring 100 m × 50 m and are arranged in a completely randomized design. To minimize the edge effect, each plot was surrounded by a 5-m buffer. The plots are burnt after the first spring rains (September) (Trollope, 1984). It should be noted that this trial was originally intended for vegetative purposes, and the two replications employed were suitable for the design. Due to their size, the large field plots such as the current trial are difficult to replicate. Several articles have since been published using the improved sampling design and strategy (Materechera et al., 1998; Magomani and van Tol, 2019; Parwada et al., 2020) and this indicates the reproducibility of the experiment. The current trial sampling was completed prior to the initiation of the 42nd year of treatments in the experiment. The soil was collected using an auger at a depth of 0–30 cm. Three soil samples were randomly taken from each treatment plot (12 plots) to make a total of 36 soil samples. The soil samples were stored in an insulated box with ice and were sent to the Central Analytical Facilities (Stellenbosch University, South Africa) for analysis.
2.2 Soil DNA extraction, PCR amplifications and sequences
Total DNA was extracted from 0.25 g of soil for each of samples using a DNeasy PowerSoil Isolation Kit (QIAGEN, Germany) according to the manufacturer’s procedures. Metagenomic DNA (mgDNA) from these samples was quantified on the Qubit™ 4 Fluorometer using the Qubit Qubit™ 1X dsDNA High Sensitivity (HS) Assay Kit (ThermoFisher Scientific) according to the protocol, MAN0017455. To evaluate the DNA quality, the DNA samples were tested using a Nanodrop spectrophotometer (Thermo Fisher Scientific). Each soil sample was properly mixed and then stored at −20°C before analyzing soil fungal microbial diversity profiles.
The presence of mgDNA was confirmed by amplification of the eukaryotic internal transcribed spacer (ITS) region. Target ITS sequences were amplified using the universal primer set, ITS1F: 5′- CTT GGT CAT TTA GAG GAA GTA A- 3′ and ITS2: 5′- GCT GCG TTC TTC ATC GAT GC -3’. Fragments were amplified from 2 µL mgDNA in a final reaction volume of 20 µL containing 0.5 µM of each primer, 200 µM of each dNTP (ThermoFisher Scientific), 0.4 units of Phusion Hot Start II DNA Polymerase, and 1 x Phusion high-fidelity buffer with a final concentration of 1.5 mM MgCl2 (ThermoFisher Scientific). The PCRs were performed on the SimpliAmp™ Thermal Cycler (ThermoFisher Scientific). Initial template DNA denaturation was performed at 98°C for 30 s, followed by 25 cycles of denaturation at 98°C for 10 s, annealing at 58°C for 30 s, extension at 72°C for 30 s, and a final extension at 72°C for 10 min.
The presence of amplified products was verified on the LabChip GX Touch 24 Nucleic Acid Analyzer (PerkinElmer, Waltham, MA, United States), using the X-Mark DNA LabChip and HT DNA NGS 3K Reagent Kit according to the manufacturer’s protocol; NGS 3K Assay Quick Guide. Following verification, PCR products were purified with a 1.8x volume of AMPure XP reagent (Beckman Coulter, Brea CA, United States) and eluted in 25 µL of nuclease-free water (ThermoFisher Scientific). Purified amplicons were quantified on the Qubit™ 4 Fluorometer using the Qubit™ 1X dsDNA High Sensitivity (HS) Assay Kit (ThermoFisher Scientific) according to the protocol, MAN0017455.
Library preparation was performed from 50 ng purified PCR product for each sample using the Ion Plus Fragment Library Kit (ThermoFisher Scientific) according to the protocol (Ion Xpress™ Plus gDNA Fragment Library Preparation User Guide). Briefly, 79 µL of each purified PCR product was end-repaired at room temperature for 20 min using 1 µL end-repair enzyme and 20 µL of end-repair buffer in a final volume of 100 µL. The end-repaired products were purified with a 1.8x volume of AMPure XP reagent (Beckman Coulter). The end-repaired product was ligated to 1 µL of IonCode Barcode Adapters (ThermoFisher Scientific). The adapter-ligated, barcoded libraries were purified with a 1.4x volume of AMPure XP reagent (Beckman Coulter) and quantified using the Ion Library TaqMan™ Quantitation Kit according to the manufacturer’s protocol (Ion Library TaqMan Quantitation Kit User Guide).
Quantitative PCR amplification was performed using the StepOnePlus™ Real-Time PCR System (ThermoFisher Scientific). Library fragment size distributions were assessed on the LabChip GX Touch 24 Nucleic Acid Analyzer (PerkinElmer, Waltham, MA, United States), using the X-Mark DNA LabChip and HT DNA NGS 3K Reagent Kit according to the manufacturer’s protocol (NGS 3K Assay Quick Guide). Libraries were diluted to a target concentration of 60 p.m. The diluted, barcoded ITS libraries were combined in equimolar amounts for template preparation using the Ion 510™, Ion 520™ and Ion 530™ Chef Kit (ThermoFisher Scientific). In brief, 25 µL of the pooled library was loaded on the Ion Chef liquid handler using reagents, solutions, and supplies according to the protocol (Ion 510 & Ion 520 & Ion 530 Chef Kit User Guide). Enriched, template-positive ion sphere particles were loaded onto an Ion 530™ chip (ThermoFisher Scientific).
2.3 Sequencing data processing
Quality filtering and trimming: Based on the raw amplicon sequences, quality checking was done using FASTQC (v0.11.9), and the reports were amalgamated with MultiQC (v1.13). As a result, all the primers and adapters were removed from the amplicon sequences using the bbduk. sh script using the following parameter options: llumine ASAP/illumina-adapters-all.fasta, ktrim = r, k = 19, mink = 11, hdist = 1, minlen = 80, ottm = t, ordered = t, stats = samplename-fastqstats.txt, and statscolumns = 5. When these were removed, quality checking was done, and all the trimmed amplicon sequences for the samples passed the basic statistics: per base sequence quality score, per sequence quality, per base N content, and adapter content, whereas sequence length distribution passed with a warning.
2.4 Statistical analysis
Different R packages were used, including phyloseq, phangorn, and microbiome, for downstream analysis. R packages were useful for importing, storing, analyzing, and displaying graphs of complex phylogenetic sequencing data based on Operational Taxonomic Units (OTUs) trimming and ASV table construction. The UNITE database was used (https://unite.ut.ee/repository.php, specifically the UNITE + euk) to assign fungal taxonomic assignments. A permutational multivariate analysis of variance (PERMANOVA) was used to test the differences in species composition among different fire burning frequencies. This was done with 999 permutations on the dissimilarity matrices with the “adonis” function of the VEGAN package in R. Any significant differences were further investigated with the Turkey Honest Significant Difference (TurkeyHSD) test for multiple comparisons. Alpha diversity indices (observed, Chao1, Shannon-Weiner, and Inverse Simpson) were presented in boxplots, and the Shannon-Wiener’s and Inverse Simpson diversity indices means were further presented on a bar graph to show any trends given by the burning cycles.
Principal Coordinate Analysis (PCoA) was used for Bray-Curtis distance matrices to visualize the relationships between treatments. Relative abundance was used to group common fungi species into different phyla and genus levels. The analysis of similarity (ANOSIM) was used to determine whether there was a statistically significant difference between soil fungal microbial communities. Global R closer to 0 indicates high similarity, and closer to 1 indicates a large difference (Clarke et al., 2006). For instance, if the R values are less than 0.25, it implies that they are hardly separated. Whereas R values greater than 0.50 indicate that they are moderately separated, and values above 0.75 indicate a clear separation. Differential abundance analysis was done with DESeq2, and the DESeq2 results were presented on plots to show the genera that increased and/or decreased when comparing burnt to no burning treatments. All statistical analyses were performed using R software (version 4.1.2).
3 Results
3.1 Differential abundance analysis with DESeq2
Six taxa were the most altered by fire frequencies, and these included Mortierellomycota, Ascomycota, Basidiomycota, Chytridiomycota, Rozellomycota, and unknown (see Supplementary Material for taxa visualization and description of taxonomic classification). Figure 2 presents the genera that increased and/or decreased when comparing annual to no burning treatments, colored by phylum. The phylum Ascomycota had the largest representation of various genus, while the phylum Chytridiomycota had the lowest genus representation. The largest increase was observed in the genus Mycena under the phylum Basidiomycota, followed by Knufla and Papulaspora under the phylum Ascomycota and the other unidentified genera (NA). Conversely, the largest decline was observed, particularly in the phylum Ascomycota with the genus Venturiales gen incetate sedis and Coniochaetaceae gen Incertae sedis.
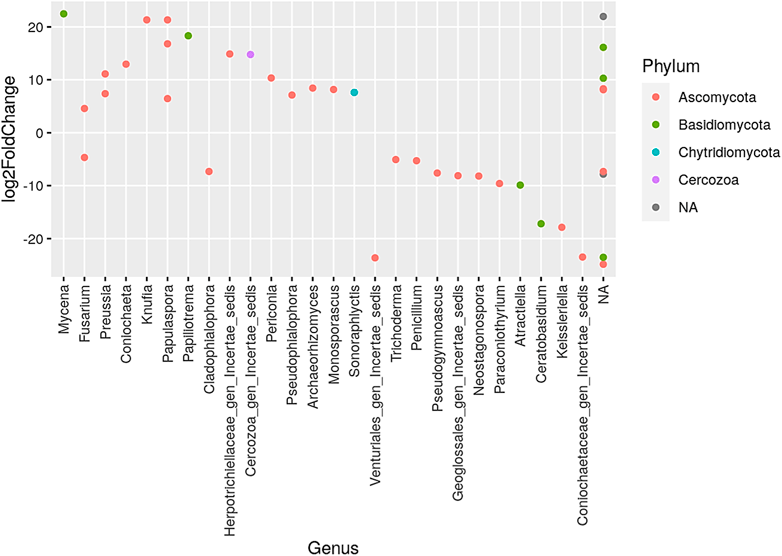
FIGURE 2. Presentation of the genera that increased and/or decreased when comparing annual to no burning treatments. NA denotes other unidentified genera; different color dots denote different dominant phyla.
The phylum Ascomycota dominated the representation of genera when comparing biennial to no burning treatments, while phylum such as Glomeromycota and Morterollomycota had fewer genus representations (Figure 3). The largest increased genera were Papulaspora under the phylum Ascomycota, while the most decreased genus was Papulaspora and Glomus under the phylum Glomeromycota. The phylum Ascomycota had the largest representation of genera in the comparison of triennial to no burning treatment, whereas Basidiomycota, Mortlerellomycota, Cercozoa, Chytridiomycota, and Kickxellomycota had fewer genera. The most increase was observed in the genus Pulvinullaceae gen Incertae sedis, Herpotrichiellaceae gen Incertae sedls, Megacapitula, and Cladophlalophora, which were under the phylum Ascomycota. On the contrary, the greatest decrease was in the genus Montagnula and Polyscytalum of the phylum Ascomycota and the genus Sonoraphlyctis of the phylum Chytridiomycota (Figure 4).
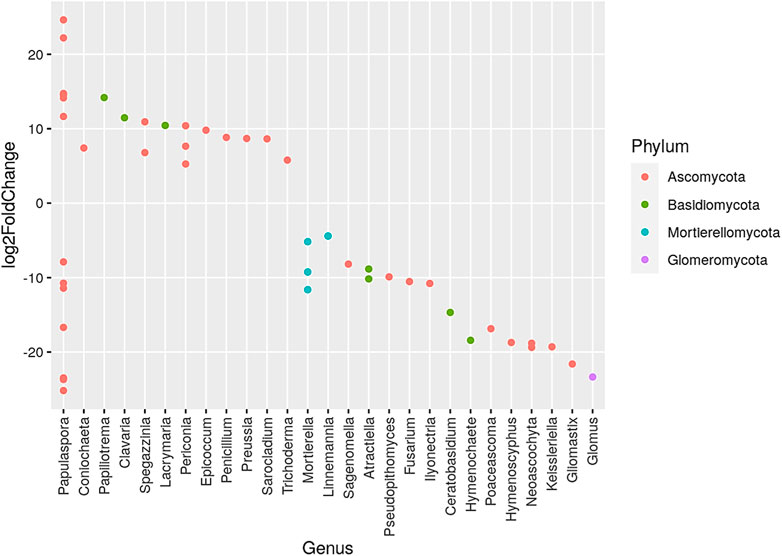
FIGURE 3. Presentation of the genera that increased and/or decreased when comparing biennial to no burning treatment. Different color dots denote different dominant phyla.
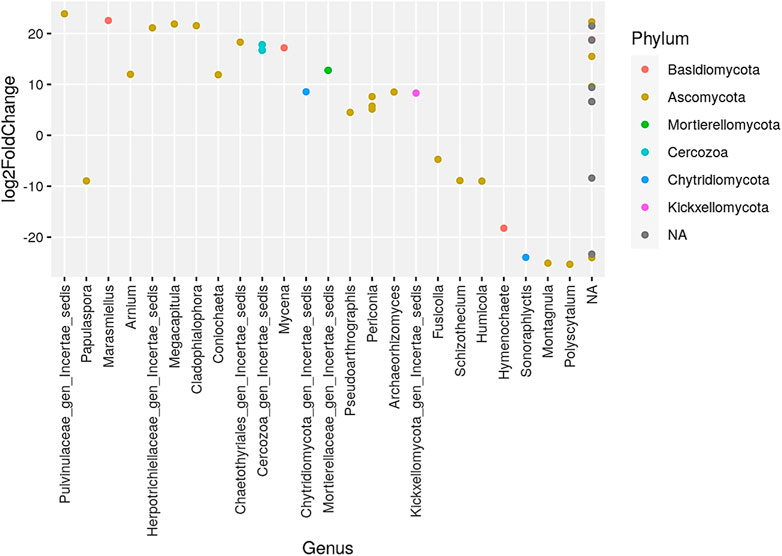
FIGURE 4. Presentation of the genera that increased and/or decreased when comparing triennial to no burning treatments. NA denotes other unidentified genera.
Quadrennial and no-burning treatments showed a decrease in the number of genus when compared to annual, biennial, and triennial burning treatments. The phylum Ascomycota had the largest representation of various genus while the phylum Chytrldiomycota, Cercozoa, and Kickxellomycota had the fewest genera. The increase was highest in the genus Chytridiomycota gen Incertae sedls under the phylum Chytrldiomycota and Cercozoa gen Incertae sedls under the phylum Cercozoa. The greatest decrease was observed in the genus Chaetosphaeria and Polyscytalum, both under the phylum Ascomycota (Figure 5).
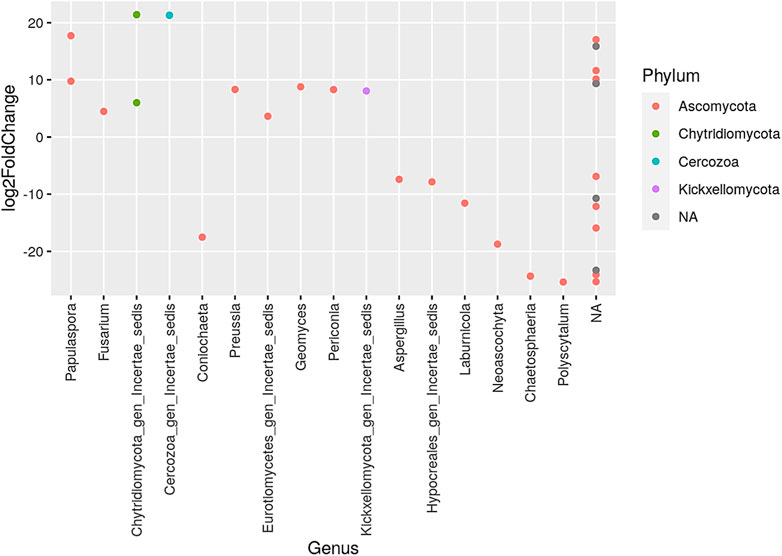
FIGURE 5. Presentation of the genera that increased and/or decreased when comparing quadrennial to no burning treatments. NA denotes other unidentified genera; different color dots denote different dominant phyla.
The phylum Ascomycota had the largest representation of genera in the comparison of sexennial burning to no burning treatments, whereas Basidiomycota and Chytridiomycota had fewer genera. The most increase was observed in the genus Papulaspora, which is under the phylum Ascomycota. On the contrary, the greatest decrease was in the genus Venturturiales gen incertae sedis in the phylum Ascomycota and the genus Spizellomycetales gen incertae sedis in the phylum Chytridlmycota (Figure 6).
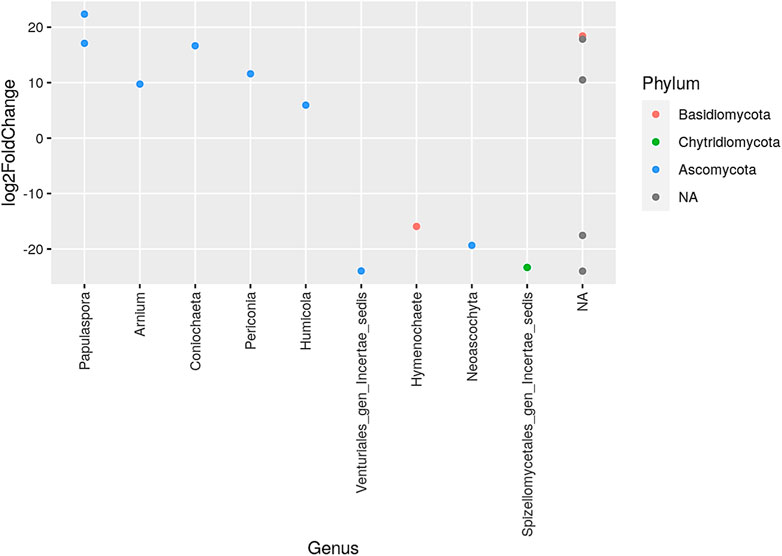
FIGURE 6. Presentation of the genera that increased and/or decreased when comparing sexennial burning to no burning treatments. NA denotes other unidentified genera; different color dots denote different dominant phyla.
3.2 Effect of burning treatments on alpha-diversity measures
There was no significant effect (F(11, 1) = 2.36; p > 0.05) among six fire-burning treatments on the Shannon-Wiener diversity index. The InvSimpson diversity index of soil fungi was found to be significantly different (F(11, 1) = 5.65; p = 0.029) among treatments (Table 1). In Table 2, the results showed that within treatments, the highest diversity was found in biennial burning, which was significantly different from the sexennial, quadrennial, and no burning treatments but not different from the triennial and annual burning treatments. In addition, there was no significant difference between the sexennial, quadrennial, triennial, annual, and no burning treatments (Table 2). The treatment groups that differed significantly were further assessed to see the comparisons between each other (see Supplementary Table S2). It was shown that the distribution of the InvSimpson diversity index differed for biennial versus no burning, biennial versus quadrennial burning, and biennial versus sexennial burning.
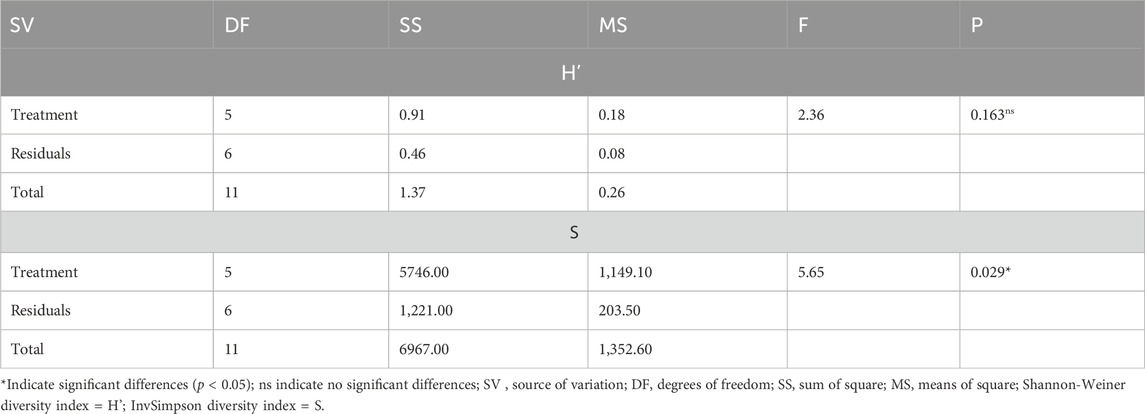
TABLE 1. Effect of burning treatments on the α−diversity indices of Shannon-Wiener and InvSimpson measures of soil fungi (one-way ANOVA).
3.3 Species richness and diversity estimates plots
Results on alpha diversity indices showed that both Observed and Chao1 followed a similar pattern (Figures 7A, B). On both indices, the highest counts of the number of taxa present (richness) were observed with sexennial burning treatments, followed by no burning and annual burning plots. The lowest counts were observed in the quadrennial burning treatments. In addition, the counts on the Observed ranged between 1,362 and 2,018, while those on Chao1 ranged between 1585.13 and 2385.59. Chao1 accounts for the likelihood of having more undiscovered species in the treatment. It is important to note that richness did not take into account the abundances of the species types. A sample with an even distribution of species is more diverse than a sample with the same number of species, yet one of the species dominates. Similar to the pattern between Observed and Chao1, the Shannon and InvSimpson indices also showed almost similar trends. The biennial plots had the highest diversity on both indices, whereas the lowest diversity was observed on the no-burning plots (Figures 7A, B). Both Shannon and InvSimpson indices account for abundance, richness, and evenness of species (Figures 7C, D). Unlike Shannon, Simpson is less sensitive to richness than it is to evenness, hence the use of InvSimpson. The abundance, richness, and evenness of fungal species indicated by Shannon-Wiener and InvSimpson means were higher in the biennial burning treatment than in other treatments (Figures 8A, B).
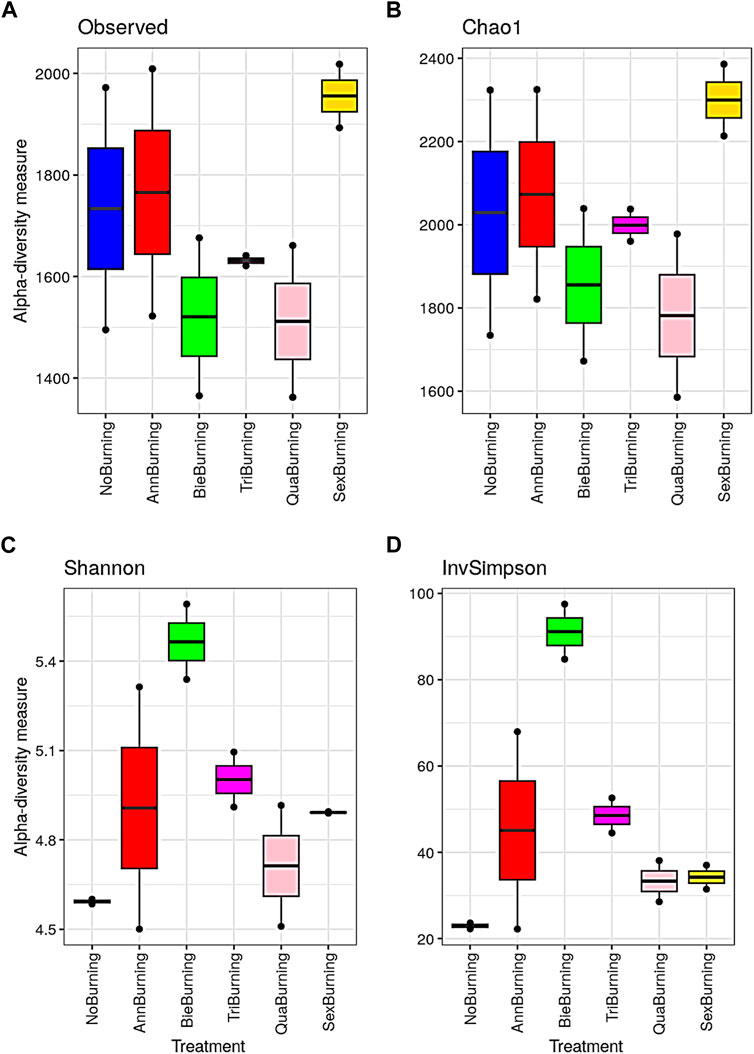
FIGURE 7. The effect of different fire frequencies on alpha-diversity estimates of soil fungal species. (A) = Observed; (B) = Chao1; (C) = Shannon-Wiener and (D) = InvSimpson indexes.
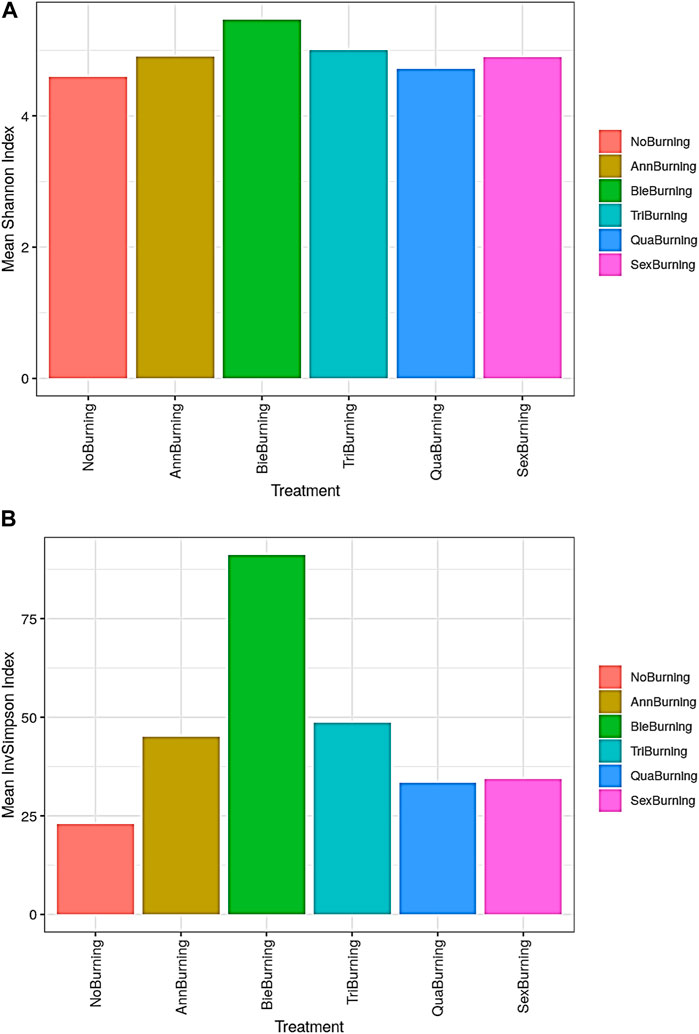
FIGURE 8. The effect of different fire frequencies on the diversity of soil fungal species. Indicated by (A) mean Shannon-Wiener and (B) mean InvSimpson indices.
The correlation relationship between the alpha diversity indices is represented by and a correlation matrix (Supplementary Figure S2). For both the scatter plot of measures and the correlation matrix, it was demonstrated that the Observed measure was highly correlated to Chao1 (R = 0.99). On the other hand, InvSimpson was not correlated to both Observed and Chao1. This confirmed that Observed and Chao1 gave similar information about the treatment samples, and the same applied to Shannon and InvSimpson.
3.4 Determining beta-diversity and analysis of similarity (ANOSIM) among treatments
The total distance that is captured by the eigenvalues was determined using a variation captured by the principal coordinate analysis. The PCoA1 had the largest variation, followed by PCoA2. This implied that the two PCoAs had the largest variation since they had the largest eigenvalues, and these were enough to explain the total variation. About 23% and 13.8% of the variations were explained by PCoA1 and PCoA2, respectively, both accounting for 37% of the variance between the samples. The PCoA1 was mostly influenced by annual, biennial, triennial, quadrennial, and sexennial burning treatments, which clustered together, while the PCoA2 was influenced by no burning treatment. Although it was easy to differentiate no burning and biennial burning samples in the PCoA using the Bray-Curtis method, it was difficult to differentiate all other considered treatment samples. The PCoA in Figure 9 showed that the annual, biennial, quadrennial, sexennial, and no burning treatments were correlated since they influenced PCoA1.
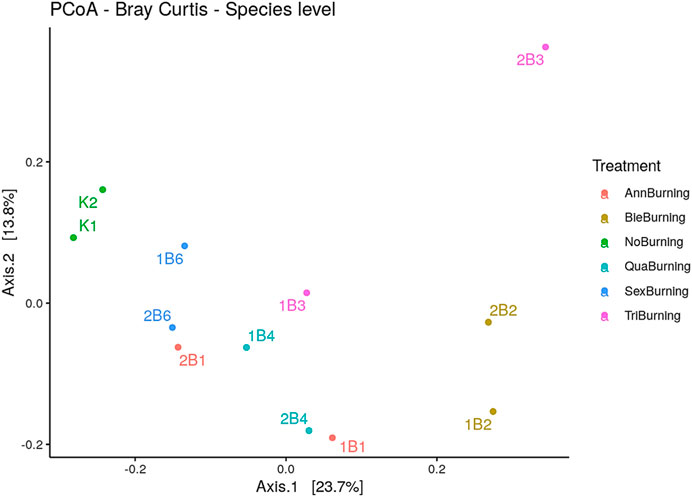
FIGURE 9. The distance between burning treatments determined using principal component analysis (Bray Curtis, species level).
The results of the ANOSIM test showed a significant difference (p = 0.014), which implied that treatment is a factor in predicting clustering. The ANOSIM value of R = 0.372, which is less than 1, depicts that fire burning treatments were fairly dissimilar (Figure 10). The dissimilarity ranks between and within classes of burning treatment are shown in Table 3.
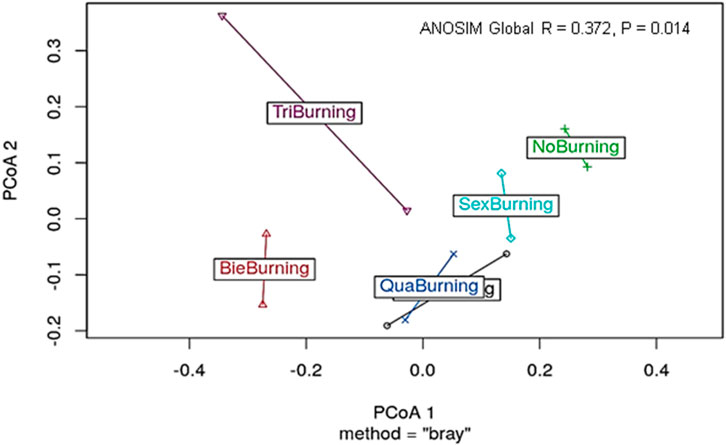
FIGURE 10. Difference in dispersion among the treatment groups. PCoA = Principal coordinate analysis.
3.5 Determination of treatment groups difference using PERMANOVA
The permutation analysis of variance (PERMANOVA) test was done to test if groups are different with respect to centroid and dispersion and the results showed that the treatment groups were significantly different (R2 = 0.54; F(11, 1) = 1.44; p = 0.002) (Table 4). We used PERMANOVA since it is a semi-parametric model that allows for adjustable confounder corrections and multi-distance encoding. As a result, PERMANOVA can investigate relationships including directional soil fungal community shift that ANOSIM cannot detect. Based on the PERMANOVA test, treatments explain 54.5% of the variability and, therefore suggesting that the treatment groups had a significant effect on soil fungal species composition. Since the p-value is significant (Table 4), this implied that there was a difference in dispersion among the treatment groups. The centroids of the groups, as defined in the space of the chosen resemblance measure, were not equivalent for all treatment groups. It was indicated that, within-treatment variation of triennial burning and sexennial burning samples was smaller than the within-treatment variation given in other treatments including no burning, quadrennial, annual and biennial (see Supplementary Figure S1A). A split plot was used instead of a biplot to elucidate the PCoA of taxa alongside labelled treatments because the biplot was not clear due to the large amounts of data points. The taxa were much more concentrated in all treatments except biennial, which was relatively low. See Supplementary Figure S1 for fungi taxonomy found on different fire treatments with respect to centroid and dispersion (A biplot and (B) split plot.

TABLE 4. The difference in treatment groups with respect to centroid and dispersion (PERMNOVA) test.
4 Discussion
The current study showed that fire increased soil fungi diversity, which was evident by higher diversity in burnt plots than unburnt (control) plots. The increase in fungi diversity and richness observed in this study after fire burning is attributed to various factors. The comparison of burnt treatment plots showed that frequently burnt treatment plots (annual, biennial, and triennial treatments) had higher diversity than the less frequently burnt treatment plots (quadrennial and sexennial treatments). This depicts that fungi diversity differs with the frequency of burning. The findings of this study were comparable to those made by Bowd et al. (2022), which reported high increases in fungi communities due to high fire frequency in the forest ecosystem.
The high diversity found in frequently burnt plots might be because repeated burning can create more resistance to fire and intensify the support of spore gemination (Hopkins et al., 2021). In frequently burnt plots, the influence can be reduced due to less vegetation recovery, such that when burning occurs, less heat is produced, which decreases its belowground dissemination. Conversely, in less frequently burnt plots, there is elevated vegetative recovery than in frequently burnt plots, and high heat amounts are produced, which increase fungi diversity in the soil (Semenova-Nelsen et al., 2019). Correspondingly, a previous study conducted on the same site demonstrated higher vegetative recovery on triennial, quadrennial, and sexennial burning frequencies when compared to frequently burnt treatments such as annual and biennial (Mopipi, 2012). Also, the conditions in less frequently burnt plots could be worsened by the indirect effects of fire. For instance, high heat loads result in large alterations of soil ecosystem features such as habitat loss, nutrient loss, and carbon loss, which provide shelter, food, and energy, and their recovery takes time (Alem et al., 2020).
In the current study, six taxa that were most altered by fire frequency included Mortierellomycota, Ascomycota, Basidiomycota, Chytridiomycota, Rozellomycota, and unknown. The largest representation of phyla was generally found in Ascomycota, which plays a critical role in litter decomposition, especially cellulose decomposition (Huffman and Madritch, 2018). In arid environments, Ascomycota fungi play a key role in the cycling of carbon and nitrogen (Challacombe et al., 2019). Fungi serves important roles in soil stability, plant biomass breakdown, and plant endophytic interactions. This large representation of this phyla has been reported in other fire frequency trials, including the study by Egidi et al. (2016), with a representation of 40% under grassland ecosystems, and Vázquez-Veloso et al. (2022), with a representation of 43% under Mediterranean ecosystems. Moreover, Bowd et al. (2022) also reported similar results, with a representation of 55% in the dry-sclerophyll forest ecosystem. This is because Ascomycota is the largest phylum in the fungi kingdom (Alem et al., 2020; Martín-Pinto et al., 2023), so it is usually expected to dominate in many studies. When the burnt plots were compared to the unburnt, the Ascomycota phyla showed the greatest resilience to fire because the abundance of its genus on all burnt plots increased, despite the fire frequency. Thus, on annual, biennial, triennial, and sexennial treatment, the Ascomycota phyla had the greatest representation of genus, which showed the utmost increase after fire burning. In addition to the Ascomycota phyla, the most common genus was Papulaspora, which increased more in annual, biennial, and sexennial burning.
The increase in Ascomycota phyla reflects its resistance to fire, especially with increased burning frequency, specifically in its genus Papulaspora. In the literature, a study conducted by Martín-Pinto et al. (2023) reported that post-fire burning, the altered soil environmental status results in the instant dominance of Pyrophilous (fire-loving) ascomycetous fungi, which ultimately results in Basidiomycota-Ascomycota formation. Salo et al. (2019) deduced that the Pyrophilous species in Ascomycota are highly attracted to burnt forests, which in this study could probably relate to the high numbers of Ascomycota in frequently burnt treatment plots. Pyrophilous species are attracted to burns. This is further facilitated by the substantial mineralizable matter presence post-fire burning, which brings into play the significance of organic matter influencing soil microbes (Bowd et al., 2022).
A study done in natural temperate grassland in Australia reported that the genus Dothideomycetes was mainly found under the phylum Ascomycota (Egidi et al., 2016). Another study in a dry Afromontane Forest in Ethiopia found Hypocreales, Pleosporales, and Chaetothyriales genus. In the savanna biomes, there is limited documentation on this aspect, which prompted the undertaking of the current study. The Ascomycota phyla are associated with carbon and nitrogen cycling, specifically in arid regions where they drive litter decomposition and plant endophytic interactions. In addition, it was reported that the Ascomycota fungi are associated with symbiotic associations as well as being saprotrophs or pathogens hosted in plant tissues (Challacombe et al., 2019).
The Coniochaeta species, which belong to the Ascomycota phylum, were also recorded in various burning treatments in the current study. Coniochaeta species can degrade lignocellulose in several woody substrates, including corn stover, sawdust, switchgrass, wheat straw, and coffee residues (van Heerden et al., 2011; Ravindran et al., 2012; Weber et al., 2015; de Lima Brossi et al., 2016). In addition, Coniochaeta species tend to have poor virulence on most hosts, and they usually colonize dead tissue or occupy already infected, injured, or dead plant tissues (Leonhardt et al., 2018).
Basidiomycota phyla was the second dominant after Ascomycota in the current study, and this dominance has also been reported in studies done in other ecosystems like dry-sclerophyll forest (Bowd et al., 2022), Mediterranean (Vázquez-Veloso et al., 2022), and pine savanna (Hopkins et al., 2021). Basidiomycota can also be Pyrophilous (same as Ascomycota), implying that they are capable of sporulating and forming colonies prior to fire subjection; therefore, such fungi sprout and become dominant soon after fire (Hopkins et al., 2021; Wang et al., 2023). Several classes under this phylum were higher under frequently burnt treatment plots (annual, biennial, and triennial treatments) than under less frequently burnt treatment plots (quadrennial and sexennial treatments). In addition, Basidiomycota classes such as Mycena, Papillotrema, Clavaria, and Lactimaria increased while Attractella and Ceratobasmidium decreased under these frequently burnt treatment plots. Basidiomycota are detritivores that play a critical role in ecosystem functioning. They contribute to carbon cycling through their nutrient absorption abilities during litter degradation (Taylor et al., 2014).
In this study, the Chytridiomycota phyla had less abundance in comparison to other phyla, which was evident by the presence of a few classes. This phylum (Chytridiomycota) is known saprophytically and is dominant in grassland environments. In these grassland ecosystems, it was found to be higher in burnt than unburnt plots (Egidi et al., 2016; Vázquez-Veloso et al., 2022). In the current study, the classes identified consist of Sonography lactis, which increased under annual and decreased under triennial burning, implying they are more tolerant of high than low frequent fire burning. Another class identified was C. gen. Incertae sedis, which increased under both triennial and quadrennial burning, implying that this class can be more tolerant in less frequently burnt soils. Chytridiomycota are known to make use of organic residues from fire burning in search of nutrients. Also, Chytridiomycota can increase when there is less competition with other fungi (Vázquez-Veloso et al., 2022). Glomus, a genus in the phylum Glomeromycota, was the most reduced when comparing biannual to no burning treatments. The phylum Glomeromycota contains an important fungus known as arbuscular mycorrhizal fungi (AMF) (Stürmer, 2012). The AMF form symbiotic relationships with the roots of many terrestrial plants, allowing them access to nutrients in the soil in exchange for C (Mpongwana et al., 2023). Longo et al. (2014) reported that fire occurrence negatively affected AMF spore communities in the Mountain Chaco Forest.
Mortierellomycota phyla were also less abundant in this study, and this was observed mostly on biennial and triennial burning treatments. The classes identified were Mortierella and Linnemania under biennial burning and Mortierellaceae_gen_Incertae_sedls under triennial burning. In addition, all these classes increased under both burning frequencies, which implied that this phylum could survive and increase under highly frequent fire regimes. This phylum has been identified in other fire studies done in dry sclerophyll forest (Bowd et al., 2022), subtropical monsoon (Wang et al., 2023), and boreal forest (Whitman et al., 2019). This phylum is known to inhabit several environments, such as the rhizosphere and plant tissues, where it mediates the carbon cycle and organic matter mineralization. In addition, Mortierellomycota are also plant-growth-promoting fungi (Muneer et al., 2021).
The current study showed that various fire frequencies had a positive impact on soil fungal abundance and diversity. Fire results in the alteration of the soil ecosystem, which facilitates the spore germination of numerous fungal species (Alem et al., 2020). If the fire is of low intensity, it may have little consequence on the fungi’s mortality (Egidi et al., 2016; Dove and Hart, 2017). Fungi communities make use of post-burning changes that increase abundance (Pressler et al., 2019; Vázquez-Veloso et al., 2022). For instance, from a biome perspective, savanna biomes in South Africa are characterized by a sparse population of trees rather than a high wood density responsible for high fire intensity. Another study has reported that after fire, ash deposits can be beneficial to the fungi and there can be less competition for resources with other species (Alem et al., 2020). The current study implies that, despite the devastation effects of fire frequency in savanna ecosystems, fire may increase soil fungi diversity and abundance, which can be significant in post-fire recovery due to the promotion of fungi, which play critical roles in soil functioning.
Generally, fungal metabarcoding studies that employ short-read high-throughput sequencing (HTS) technologies, like Ion Torrent, Illumina, and 454 Pyrosequencing, typically focus on the rDNA internal transcribed spacer regions (ITS1 or ITS2) (Lindahl et al., 2013; Furneaux et al., 2021; Cheng et al., 2023). In the current study, we used Ion Torrent sequencer which is a semiconductor-based next generational sequence (NGS) platform. Similarly to the Roche/454 system, the sensor’s pH change has poor linearity in proportion to the total number of nucleotides integrated in a single reaction cycle, decreasing its ability to identify homopolymer regions (Pu and Xiao, 2017; Cheng et al., 2023). In comparison to other platforms, the Illumina platform has the highest market share for sequencing tools. It can entirely address concerns in homopolymer sequencing, albeit there is a tendency for substitution errors in AT-rich and CG-rich sections (Dohm et al., 2008; Minoche et al., 2011; Cheng et al., 2023). In Illumina data, all sequence reads generated during a single experiment are the same length, however Ion Torrent reads vary. Furthermore, the latest generation of Illumina instruments can produce sequence reads from both ends of a fragment (“paired-end” reads), but Ion Torrent cannot (Brown et al., 2013). Unlike Illumina sequencing, multiple nucleotides may be incorporated during a single Ion Torrent sequencing cycle, and it is recognized that errors in quantitating the length of homopolymer repeats are common (Dickie, 2010; Wydro, 2022). As such, to better understand these discrepancies in sequence quality, further systematic comparisons between Ion Torrent and other HTS technologies are required, as have been done for various other platforms (Luo et al., 2012; Brown et al., 2013). Comparing these is crucial since different sequencing technologies differ in their capacity to sequence at different depths and lengths, as well as in terms of their quality profiles and possible biases (Furneaux et al., 2021).
In the current study, we noted that the quality of the sequences was not good as almost half of the samples failed the per base sequence quality, while the remainder passed with a warning. To improve the sequences, bbmap and DADA2 were used to remove the adapters and trim and filter quality. However, it is acknowledged that strict quality filtering could skew our assessment of the significance of particular fungal groups by eliminating them before the analysis. This study was limited to one geographical location by design; therefore, analyzing multiple rangelands within the same savanna biome in South Africa and elsewhere may provide a more complete picture of the effect of fire rates on soil fungal diversity and composition. Also, the distance between the burning treatments was 5 m, which could make it difficult to control the fire and as such the distance between treatments could be increased in future studies. It is necessary to point out that the techniques utilized in this study, (like all others), have inherent biases. As such, there is a potential for under representation of soil fungal diversity and strains that were beneath detected levels and were unable to develop under the fire frequency environments used. Therefore, it is critical to understand the benefits and drawbacks of employing next-generation sequence metabarcoding to detect and monitor significant functional groups on an ecological scale (Makiola et al., 2019).
5 Conclusion
The current study showed that different fire frequencies had an influence on soil fungi diversity and taxonomic composition in a semi-arid savanna rangeland. It was shown that the highest soil fungal microbial diversity was found in frequently burnt treatment plots (annual, biennial, and triennial treatments) than in less frequently burnt treatment plots (quadrennial and sexennial treatments). This could suggest fungi may survive more frequently occurring fires than less frequent ones. Regarding the taxa, Ascomycota and Basidiomycota were the phyla with the highest relative abundance followed by Mortierellomycota, Chytridiomycota, and Rozellomycota. Although there was heterogeneity in terms of how they were influenced by different fire frequencies, it was demonstrated that numerous phyla increased their communities with high fire frequencies over those with low fire frequencies. Although this is a long-term trial, the data in the current study was collected over one season, and it is recommended that future studies should collect data over a longer duration to assess the impact of prescribed fire frequencies on soil microorganisms over time. Such studies should also consider climatic conditions and environmental alterations in order to make informed decisions regarding conservation of fungi species in semi-arid savanna rangelands.
Data availability statement
The original contributions presented in the study are included in the article/Supplementary Material, further inquiries can be directed to the corresponding author.
Author contributions
SB: Writing–review and editing, Conceptualization, Data curation, Formal Analysis, Investigation, Methodology, Visualization, Writing–original draft. AM: Conceptualization, Funding acquisition, Investigation, Project administration, Resources, Supervision, Validation, Writing–review and editing. CM: Conceptualization, Investigation, Methodology, Supervision, Validation, Writing–review and editing.
Funding
The author(s) declare financial support was received for the research, authorship, and/or publication of this article. This research work was supported by Govan Mbeki Research and Development Centre of the University of Fort Hare (South Africa) under the Sustainability Agriculture and Food Security research niche area (Project P744).
Acknowledgments
Special thanks to Dr Ephifania Geza and DIPLOMICS team (South Africa) for assistance and guidance regarding data analyses. We thank the National Research Foundation (Grant No: 149806) for providing a bursary to Sanele Poswa.
Conflict of interest
The authors declare that the research was conducted in the absence of any commercial or financial relationships that could be construed as a potential conflict of interest.
Publisher’s note
All claims expressed in this article are solely those of the authors and do not necessarily represent those of their affiliated organizations, or those of the publisher, the editors and the reviewers. Any product that may be evaluated in this article, or claim that may be made by its manufacturer, is not guaranteed or endorsed by the publisher.
Supplementary material
The Supplementary Material for this article can be found online at: https://www.frontiersin.org/articles/10.3389/fenvs.2024.1355278/full#supplementary-material
References
Acocks, J. P. H. (1975). Veld types of South Africa memoirs of botanical survey of South Africa, No. 40. 2nd edition. Pretoria, South Africa: Botanical Research Institute, Department of Agriculture.
Adkins, J., Docherty, K. M., Gutknecht, J. L., and Miesel, J. R. (2020). How do soil microbial communities respond to fire in the intermediate term? Investigating direct and indirect effects associated with fire occurrence and burn severity. Sci. Total Environ. 745, 140957. doi:10.1016/j.scitotenv.2020.140957
Agbeshie, A. A., Abugre, S., Atta-Darkwa, T., and Awuah, R. (2022). A review of the effects of forest fire on soil properties. J. For. Res. 33, 1419–1441. doi:10.1007/s11676-022-01475-4
Alem, D., Dejene, T., Oria-de-Rueda, J. A., Geml, J., Castano, C., Smith, J. E., et al. (2020). Soil fungal communities and succession following wildfire in Ethiopian dry Afromontane forests, a highly diverse underexplored ecosystem. For. Ecol. Manag. 474, 118328. doi:10.1016/j.foreco.2020.118328
Amoako, E. E., and Gambiza, J. (2019). Effects of anthropogenic fires on some soil properties and the implications of fire frequency for the Guinea savanna ecological zone, Ghana. Sci. Afr. 6, 00201. doi:10.1016/j.sciaf.2019.e00201
Barreiro, A., Martín, A., Carballas, T., and Díaz-Raviña, M. (2016). Long-term response of soil microbial communities to fire and fire-fighting chemicals. Biol. Fertil. Soils 52, 963–975. doi:10.1007/s00374-016-1133-5
Boer, W. D., Folman, L. B., Summerbell, R. C., and Boddy, L. (2005). Living in a fungal world: impact of fungi on soil bacterial niche development. FEMS Microbiol. Rev. 29, 795–811. doi:10.1016/j.femsre.2004.11.005
Bowd, E. J., Egidi, E., Lindenmayer, D. B., Wardle, D. A., Kardol, P., Cary, G. J., et al. (2022). Direct and indirect effects of fire on microbial communities in a pyrodiverse dry-sclerophyll forest. J. Ecol. 110, 1687–1703. doi:10.1111/1365-2745.13903
Bowman, D. M., Kolden, C. A., Abatzoglou, J. T., Johnston, F. H., van der Werf, G. R., and Flannigan, M. (2020). Vegetation fires in the anthropocene. Nat. Rev. Earth Environ. 1, 500–515. doi:10.1038/s43017-020-0085-3
Brown, S. P., Callaham, M. A., Oliver, A. K., and Jumpponen, A. (2013). Deep Ion Torrent sequencing identifies soil fungal community shifts after frequent prescribed fires in a southeastern US forest ecosystem. FEMS Microbiol. Ecol. 86, 557–566. doi:10.1111/1574-6941.12181
Certain, G. (2005). Effects of fire on properties of forest soils: a review. Oecologia 143, 1–10. doi:10.1007/s00442-004-1788-8
Certini, G., Moya, D., Lucas-Borja, M. E., and Mastrolonardo, G. (2021). The impact of fire on soil-dwelling biota: a review. For. Ecol. Manag. 488, 118989. doi:10.1016/j.foreco.2021.118989
Challacombe, J. F., Hesse, C. N., Bramer, L. M., McCue, L. A., Lipton, M., Purvine, S., et al. (2019). Genomes and secretomes of Ascomycota fungi reveal diverse functions in plant biomass decomposition and pathogenesis. BMC Genom 20, 976–1027. doi:10.1186/s12864-019-6358-x
Cheng, C., Fei, Z., and Xiao, P. (2023). Methods to improve the accuracy of next-generation sequencing. Front. Bioeng. Biotechnol. 11, 982111. doi:10.3389/fbioe.2023.982111
Clarke, K. R., Somerfield, P. J., Airoldi, L., and Warwick, R. M. (2006). Exploring interactions by second-stage community analyses. J. Exp. Mar. Biol. Ecol. 338, 179–192. doi:10.1016/j.jembe.2006.06.019
De Groot, W. J., Flannigan, M. D., and Cantin, A. S. (2013). Climate change impacts on future boreal fire regimes. For. Ecol. Manag. 294, 35–44. doi:10.1016/j.foreco.2012.09.027
De Lima Brossi, M. J., Jiménez, D. J., Cortes-Tolalpa, L., and van Elsas, J. D. (2016). Soil-derived microbial consortia enriched with different plant biomass reveal distinct players acting in lignocellulose degradation. Microb. Ecol. 71, 616–627. doi:10.1007/s00248-015-0683-7
Dickie, I. A. (2010). Insidious effects of sequencing errors on perceived diversity in molecular surveys. New Phytol. 188, 916–918. doi:10.1111/j.1469-8137.2010.03473.x
Dohm, J. C., Lottaz, C., Borodina, T., and Himmelbauer, H. (2008). Substantial biases in ultra-short read data sets from high-throughput DNA sequencing. Nucleic Acids Res. 36, e105. doi:10.1093/nar/gkn425
Dooley, S. R., and Treseder, K. K. (2012). The effect of fire on microbial biomass: a meta-analysis of field studies. Biogeochem 109, 49–61. doi:10.1007/s10533-011-9633-8
Dove, N. C., and Hart, S. C. (2017). Fire reduces fungal species richness and in situ mycorrhizal colonization: a meta-analysis. Fire Ecol. 13, 37–65. doi:10.4996/fireecology.130237746
Dove, N. C., Stark, J. M., Newman, G. S., and Hart, S. C. (2019). Carbon control on terrestrial ecosystem function across contrasting site productivities: the carbon connection revisited. Ecology 100, e02695. doi:10.1002/ecy.2695
Egidi, E., McMullan-Fisher, S., Morgan, J. W., May, T., Zeeman, B., and Franks, A. E. (2016). Fire regime, not time-since-fire, affects soil fungal community diversity and composition in temperate grasslands. FEMS Microbiol. Lett. 363, 196. doi:10.1093/femsle/fnw196
Fernández, C., Vega, J. A., and Fonturbel, T. (2013). Does fire severity influence shrub resprouting after spring prescribed burning? Acta Oecol 48, 30–36. doi:10.1016/j.actao.2013.01.012
Fontúrbel, M. T., Barreiro, A., Vega, J. A., Martín, A., Jiménez, E., Carballas, T., et al. (2012). Effects of an experimental fire and post-fire stabilization treatments on soil microbial communities. Geoderma 191, 51–60. doi:10.1016/j.geoderma.2012.01.037
Furley, P. A., Rees, R. M., Ryan, C. M., and Saiz, G. (2008). Savanna burning and the assessment of long-term fire experiments with particular reference to Zimbabwe. Prog. Phys. Geogr. 32, 611–634. doi:10.1177/0309133308101383
Furneaux, B., Bahram, M., Rosling, A., Yorou, N. S., and Ryberg, M. (2021). Long-and short-read metabarcoding technologies reveal similar spatiotemporal structures in fungal communities. Mol. Ecol. Resour. 21, 1833–1849. doi:10.1111/1755-0998.13387
Hansen, P. M., Semenova-Nelsen, T. A., Platt, W. J., and Sikes, B. A. (2019). Recurrent fires do not affect the abundance of soil fungi in a frequently burned pine savanna. Fungal Ecol. 42, 100852. doi:10.1016/j.funeco.2019.07.006
Holden, S. R., Rogers, B. M., Treseder, K. K., and Randerson, J. T. (2016). Fire severity influences the response of soil microbes to a boreal forest fire. Environ. Res. Lett. 11, 035004. doi:10.1088/1748-9326/11/3/035004
Hopkins, J. R., Semenova-Nelsen, T., and Sikes, B. A. (2021). Fungal community structure and seasonal trajectories respond similarly to fire across pyrophilic ecosystems. FEMS Microbiol. Ecol. 97, 219. doi:10.1093/femsec/fiaa219
Huffman, M. S., and Madritch, M. D. (2018). Soil microbial response following wildfires in thermic oak-pine forests. Biol. Fertil. Soils 54, 985–997. doi:10.1007/s00374-018-1322-5
IUSS Working Group WRB (2022). World Reference Base for Soil Resources, International soil classification system for naming soils and creating legends for soil maps. 4th edition. Vienna, Austria: International Union of Soil Sciences IUSS, 234.
Janion-Scheepers, C., Measey, J., Braschler, B., Chown, S. L., Coetzee, L., Colville, J. F., et al. (2016). Soil biota in a megadiverse country: current knowledge and future research directions in South Africa. Pedobiologia 59, 129–174. doi:10.1016/j.pedobi.2016.03.004
Kjoller, A., and Struwe, S. (1982). Microfungi in ecosystems: fungal occurrence and activity in litter and soil. Oikos 39, 391–422. doi:10.2307/3544690
Leonhardt, S., Büttner, E., Gebauer, A. M., Hofrichter, M., and Kellner, H. (2018). Draft genome sequence of the sordariomycete lecythophora (Coniochaeta) hoffmannii CBS 245.38. Genome announc. 6, 015100–e11128. doi:10.1128/genomea.01510-17
Lindahl, B. D., Nilsson, R. H., Tedersoo, L., Abarenkov, K., Carlsen, T., Kjøller, R., et al. (2013). Fungal community analysis by high-throughput sequencing of amplified markers–a user’s guide. New Phytol. 199, 288–299. doi:10.1111/nph.12243
Liu, W., Zhang, Z., Li, J., Wen, Y., Liu, F., Zhang, W., et al. (2023). Effects of fire on the soil microbial metabolic quotient: a global meta-analysis. Catena 224, 106957. doi:10.1016/j.catena.2023.106957
Longo, S., Nouhra, E., Goto, B. T., Berbara, R. L., and Urcelay, C. (2014). Effects of fire on arbuscular mycorrhizal fungi in the Mountain Chaco Forest. For. Ecol. Manag. 315, 86–94. doi:10.1016/j.foreco.2013.12.027
Luo, C., Tsementzi, D., Kyrpides, N., Read, T., and Konstantinidis, K. T. (2012). Direct comparisons of Illumina vs. Roche 454 sequencing technologies on the same microbial community DNA sample. PLoS ONE 7, e30087. doi:10.1371/journal.pone.0030087
Madikana, A. (2022). Investigations into the impact of fire frequency on the recovery of soil invertebrate communities in semi-arid savanna biome of the Eastern Cape, South Africa. MSc Thesis. Alice, South Africa: University of Fort Hare.
Madondo, R., Mutingwende, N., Shwababa, S., Bayne, R. J., Restás, Á., and Tandlich, R. (2022). Analyses of trends in the fire losses and the fire-brigade call-outs in South Africa between 2004 and 2017. Geogr. Tech. 17, 54–68. doi:10.21163/gt_2022.172.06
Magomani, M. I., and van Tol, J. J. (2019). The impact of fire frequency on selected soil physical properties in a semi-arid savannah Thornveld. Acta Agric. Scand. B Soil Plant Sci. 69, 43–51. doi:10.1080/09064710.2018.1495253
Makiola, A., Dickie, I. A., Holdaway, R. J., Wood, J. R., Orwin, K. H., Lee, C. K., et al. (2019). Biases in the metabarcoding of plant pathogens using rust fungi as a model system. MicrobiologyOpen 8, e00780. doi:10.1002/mbo3.780
Martín-Pinto, P., Fernández, C., Santos, M., Fontúrbel, T., Oria-de-Rueda, J. A., Vázquez-Veloso, A., et al. (2023). Unaltered fungal community after fire prevention treatments over widespread Mediterranean rockroses (Halimium lasianthum). Sci. Rep. 13, 608. doi:10.1038/s41598-023-27945-1
Materechera, S. A., Mandiringana, O. T., Mbokodi, P. M., and Nyamapfene, K. (1998). Organic matter, pH and nutrient distribution in soil layers of a savanna Thornveld subjected to different burning frequencies at Alice in the Eastern Cape. S. Afr. J. Plant Soil 15, 109–115. doi:10.1080/02571862.1998.10635127
Metablue (2022). Simulated historical climate and weather data for Alice. Eastern Cape, South Africa. Available at: https://www.meteoblue.com/en/weather/historyclimate/climatemodelled/alice_south-africa_1023332 (Accessed October 12, 2023).
Minoche, A. E., Dohm, J. C., and Himmelbauer, H. (2011). Evaluation of genomic high-throughput sequencing data generated on Illumina HiSeq and genome analyzer systems. Genome Biol. 12, R112. doi:10.1186/gb-2011-12-11-r112
Mopipi, K. (2012). The roles of competition, disturbance and nutrients on species composition, light interception and biomass production in a South African semi-arid savanna. PhD Dissertation. Pietermaritzburg, South Africa: University of KwaZulu-Natal.
Mpongwana, S., Manyevere, A., Mupangwa, J., Mpendulo, C. T., and Mashamaite, C. V. (2023). Optimising biomass yield of three herbaceous forage legumes through dual inoculation of arbuscular mycorrhizal fungi and Rhizobia. S. Afr. J. Bot. 159, 61–71. doi:10.1016/j.sajb.2023.06.006
Mucina, L., and Rutherford, M. C. (2006). The vegetation of South Africa, Lesotho and Swaziland Strelitzia 19. Pretoria, South Africa: South African National Biodiversity Institute.
Muneer, M. A., Huang, X., Hou, W., Zhang, Y., Cai, Y., Munir, M. Z., et al. (2021). Response of fungal diversity, community composition, and functions to nutrients management in red soil. J. Fungi 7, 554. doi:10.3390/jof7070554
Nyamadzawo, G., Gwenzi, W., Kanda, A., Kundhlande, A., and Masona, C. (2013). Understanding the causes, socio-economic and environmental impacts, and management of veld fires in tropical Zimbabwe. Fire Sci. Rev. 2, 2–13. doi:10.1186/2193-0414-2-2
Oluwole, F. A., Sambo, J. M., and Sikhalazo, D. (2008). Long-term effects of different burning frequencies on the dry savannah grassland in South Africa. Afr. J. Agric. Res. 3, 147–153.
Parwada, C., Magomani, M. I., and van Tol, J. J. (2020). Impacts of different prescribed fire frequencies on selected soil chemical properties in a semi-arid savannah thornveld. Cogent Environ. Sci. 6, 1868171. doi:10.1080/23311843.2020.1868171
Pressler, Y., Moore, J. C., and Cotrufo, M. F. (2019). Belowground community responses to fire: meta-analysis reveals contrasting responses of soil microorganisms and mesofauna. Oikos 128, 309–327. doi:10.1111/oik.05738
Pu, D., and Xiao, P. F. (2017). A real-time decoding sequencing technology – new possibility for high throughput sequencing. RSC Adv. 7, 40141–40151. doi:10.1039/c7ra06202h
Pulido-Chavez, M. F., Randolph, J. W., Zalman, C., Larios, L., Homyak, P. M., and Glassman, S. I. (2023). Rapid bacterial and fungal successional dynamics in first year after chaparral wildfire. Mol. Ecol. 32, 1685–1707. doi:10.1111/mec.16835
Ravindran, A., Adav, S. S., and Sze, S. K. (2012). Characterization of extracellular lignocellulolytic enzymes of Coniochaeta sp. during corn stover bioconversion. Process Biochem. 47, 2440–2448. doi:10.1016/j.procbio.2012.10.003
Ribeiro, N., Ruecker, G., Govender, N., Macandza, V., Pais, A., Machava, D., et al. (2019). The influence of fire frequency on the structure and botanical composition of savanna ecosystems. Ecol. Evol. 9, 8253–8264. doi:10.1002/ece3.5400
Russell-Smith, J., Yates, C., Vernooij, R., Eames, T., van der Werf, G., Ribeiro, N., et al. (2021). Opportunities and challenges for savanna burning emissions abatement in southern Africa. J. Environ. Manage. 288, 112414. doi:10.1016/j.jenvman.2021.112414
Salo, K., Domisch, T., and Kouki, J. (2019). Forest wildfire and 12 years of post-disturbance succession of saprotrophic macrofungi (Basidiomycota, Ascomycota). For. Ecol. Manag. 451, 117454. doi:10.1016/j.foreco.2019.117454
Semenova-Nelsen, T. A., Platt, W. J., Patterson, T. R., Huffman, J., and Sikes, B. A. (2019). Frequent fire reorganizes fungal communities and slows decomposition across a heterogeneous pine savanna landscape. New Phytol. 224, 916–927. doi:10.1111/nph.16096
Singh, D., Sharma, P., Kumar, U., Daverey, A., and Arunachalam, K. (2021). Effect of forest fire on soil microbial biomass and enzymatic activity in oak and pine forests of Uttarakhand Himalaya, India. Ecol. Process. 10, 29. doi:10.1186/s13717-021-00293-6
Smith, M. D., van Wilgen, B. W., Burns, C. E., Govender, N., Potgieter, A. L., Andelman, S., et al. (2013). Long-term effects of fire frequency and season on herbaceous vegetation in savannas of the Kruger National Park, South Africa. J. Plant Ecol. 6, 71–83. doi:10.1093/jpe/rts014
Snyman, H. A. (2015). Short-term responses of southern African semi-arid rangelands to fire: a review of impact on soils. Arid. Land Res. Manag. 29, 222–236. doi:10.1080/15324982.2014.944244
South Africa Weather Service (2021). Climate South Africa. Available at: http://ftp.weathersa.co.za (Accessed August 02, 2023).
Southworth, J., Zhu, L., Bunting, E., Ryan, S. J., Herrero, H., Waylen, P. R., et al. (2016). Changes in vegetation persistence across global savanna landscapes, 1982–2010. J. Land Use Sci. 11, 7–32. doi:10.1080/1747423X.2015.1071439
Srikanthasamy, T., Barot, S., Koffi, F. K., Tambosco, K., Marcangeli, Y., Carmignac, D., et al. (2021). Short-term impact of fire on the total soil microbial and nitrifier communities in a wet savanna. Ecol. Evol. 11, 9958–9969. doi:10.1002/ece3.7661
Strydom, S., and Savage, M. J. (2016). A spatio-temporal analysis of fires in South Africa. S. Afr. J. Sci. 112, 1–8. doi:10.17159/sajs.2016/20150489
Stürmer, S. L. (2012). A history of the taxonomy and systematics of arbuscular mycorrhizal fungi belonging to the phylum Glomeromycota. Mycorrhiza 22, 247–258. doi:10.1007/s00572-012-0432-4
Trollope, W. S. W. (1984). “Fire in savanna,” in Ecological effects of fire in South African ecosystems (Berlin Heidelberg: Springer), 149–175.
United Nations (2020). The sustainable development goals report. Available at: https://unstats.un.org/sdgs/report/2020/The-Sustainable-Development-Goals-Report (Accessed July 26, 2023).
Van Heerden, A., van Zyl, W. H., Cruywagen, C. W., Mouton, M., and Botha, A. (2011). The lignicolous fungus Coniochaeta pulveracea and its interactions with syntrophic yeasts from the woody phylloplane. Microb. Ecol. 62, 609–619. doi:10.1007/s00248-011-9869-9
Vázquez-Veloso, A., Dejene, T., Oria-de-Rueda, J. A., Guijarro, M., Hernando, C., Espinosa, J., et al. (2022). Prescribed burning in spring or autumn did not affect the soil fungal community in Mediterranean Pinus nigra natural forests. For. Ecol. Manag. 512, 120161. doi:10.1016/j.foreco.2022.120161
Vermeire, M. L., Thoresen, J., Lennard, K., Vikram, S., Kirkman, K., Swemmer, A. M., et al. (2021). Fire and herbivory drive fungal and bacterial communities through distinct above-and belowground mechanisms. Sci. Total Environ. 785, 147189. doi:10.1016/j.scitotenv.2021.147189
Wang, L. L., Zhou, Q. Q., Su, W. H., Xu, Y. J., Qian, Q. D., Yang, X., et al. (2023). Responses of fungal community to forest fire are species-specific in Yunnan Plateau, southwest China. J. Plant Ecol. 16, 043. doi:10.1093/jpe/rtac043
Weber, C. F., King, G. M., and Aho, K. (2015). Relative abundance of and composition within fungal orders differ between cheatgrass (Bromus tectorum) and sagebrush (Artemisia tridentata)-associated soils. PLoS One 10 (1), 0117026. doi:10.1371/journal.pone.0117026
Whitman, T., Whitman, E., Woolet, J., Flannigan, M. D., Thompson, D. K., and Parisien, M. A. (2019). Soil bacterial and fungal response to wildfires in the Canadian boreal forest across a burn severity gradient. Soil Biol. biochem. 138, 107571. doi:10.1016/j.soilbio.2019.107571
Williams, R. J., Hallgren, S. W., and Wilson, G. W. (2012). Frequency of prescribed burning in an upland oak forest determines soil and litter properties and alters the soil microbial community. For. Ecol. Manag. 265, 241–247. doi:10.1016/j.foreco.2011.10.032
Keywords: ascomycota, ASVs, climate change, fire-prone biome, species richness, species diversity
Citation: Poswa SB, Manyevere A and Mashamaite CV (2024) Responses of fungal diversity and community composition after 42 years of prescribed fire frequencies in semi-arid savanna rangelands. Front. Environ. Sci. 12:1355278. doi: 10.3389/fenvs.2024.1355278
Received: 14 December 2023; Accepted: 19 February 2024;
Published: 29 February 2024.
Edited by:
Jianshuang Wu, Chinese Academy of Agricultural Sciences, ChinaReviewed by:
Rentao Liu, Ningxia University, ChinaHelene Niculita-Hirzel, University Center of General Medicine and Public Health, Switzerland
Copyright © 2024 Poswa, Manyevere and Mashamaite. This is an open-access article distributed under the terms of the Creative Commons Attribution License (CC BY). The use, distribution or reproduction in other forums is permitted, provided the original author(s) and the copyright owner(s) are credited and that the original publication in this journal is cited, in accordance with accepted academic practice. No use, distribution or reproduction is permitted which does not comply with these terms.
*Correspondence: Alen Manyevere, AManyevere@ufh.ac.za