- 1State Key Laboratory of NBC Protection for Civilian, Beijing, China
- 2School of Environment, Nanjing Normal University, Nanjing, Jiangsu, China
- 3School of Chemistry and Chemical Engineering, Nanjing University of Science and Technology, Nanjing, Jiangsu, China
- 4Jiangsu Province Engineering Research Center of Environmental Risk Prevention and Emergency Response Technology, Nanjing, Jiangsu, China
Land and groundwater resources are fundamental pillars of sustainable human development. The negligent abandonment of ammunition and its fragments during range activities can result in severe contamination of range sites, thereby posing a significant risk to both the ecological environment and human health. Nevertheless, numerous uncertainties persist regarding the comprehension of range contaminated sites. In this study, the literature on the range of contaminated sites decommissioned after 2000 was systematically examined to consolidate basic information related to these sites, such as contaminant types, contamination status, and remediation measures. Considerable attention is devoted to investigating the advancement of diverse techniques, such as phytoremediation, chemical leaching, and solidification/stabilization, to remediate polluted areas within decommissioned firing ranges. Among the various types of remediation means, physical remediation and chemical remediation have higher remediation efficiency, but generally have higher costs and are prone to secondary pollution. Bioremediation is low cost and environmentally friendly, but has a long restoration cycle. The choice of remediation method should be based on actual needs. Additionally, this study puts forth prospective avenues for future research. Ultimately, this endeavor aims to attract the interest of scholars toward the remediation of contaminated sites within firing ranges, thereby making a valuable contribution to both human wellbeing and sustainable progress.
1 Introduction
The maintenance of operational readiness for troops necessitates military training involving live ammunition, which inevitably leads to soil and groundwater contamination in the surrounding areas. Due to the dispersion of training ranges across diverse soil types, the interaction of contaminants from range activities and their impact on specific receptors pose challenges in terms of prediction. The utilization of metallic lead is prevalent in the production of bullets and lead projectiles due to its exceptional attributes of high density, corrosion resistance, and ductility Dinake et al. (2019). The primary components of ammunition utilized for range training are a Pb-Sb core, which constitutes approximately 95% of the bullet, and a Cu-Zn casing, accounting for approximately 5% of the bullet. The core is primarily composed of Pb (>90%), Pb-Sb alloy (<5%), As (<0.5%), and Ni (<0.5%), whereas the casing is composed of a Cu-Zn (90%/10%) alloy (Dermatas et al., 2006a). Currently, firing ranges are located all over the world, including Asia, Europe, Africa and the Americas (Cao et al., 2003; Sorvari et al., 2006; Mathee et al., 2017; Khan et al., 2021). According to reports, approximately 100,000 outdoor and military firing ranges worldwide are utilized for shooting activities, resulting in an annual release of up to 72,600 tons of Pb from munitions onto the soils of these diverse national ranges (Rodríguez-Seijo et al., 2017). Furthermore, copper (Cu) is employed as a constituent in jacketed bullets alongside lead (Pb), antimony (Sb) serves as a hardening agent for lead, nickel (Ni) or zinc (Zn) are utilized as alloys in the copper jacketed bullets, arsenic (As) coexists within lead and is a constituent of lead shotgun shells, chromium (Cr) acts as an alloy material for certain bullet types, and clay targets contain a range of 3,000 to 40,000 mg of polycyclic aromatic hydrocarbons (PAHs) per kilogram (ITRC, 2003; Laporte-Saumure et al., 2011). The release of heavy metals and organic contaminants from firing ranges due to munitions weathering can result in their deposition in range soils and subsequent diffusion into the surrounding environment via pore space, surface or groundwater. Consequently, the detection of contamination of varying magnitudes has been observed in nearly all range environments. Pb, one of the priority metal contaminants in firing ranges, has been found in concentrations up to tens of thousands of mg/kg in contaminated ranges around the world, with the highest levels on berm (Hardison Jr et al., 2004; Mendes et al., 2023). Clay fragments piled up in the range contain large amounts of coal tar and petroleum asphalt, which can contain as much as 3,000–40,000 mg/kg of PAHs (Wolf et al., 2020). Some studies have shown that heavy metal concentrations in shooting range soils worldwide are much higher than background concentrations in agricultural soils, with Pb concentrations tens or even hundreds of times higher (Sanderson et al., 2018).
The bioavailability and bioaccessibility of pollutants at the target range determine the toxicity level of the pollutants, and higher bioavailability indicates higher levels of soil toxicity, which, to a certain extent, will lead to a decrease in the growth rate and level of plant growth, a decrease in the abundance of microbial communities, and an impediment to the cycling of soil nutrients, etc. (Fayiga and Saha, 2016a; Sanderson et al., 2018). Due to their inherent inertness in typical soil, groundwater, and surface water conditions, the persistence of contaminants in range sites can be prolonged in the absence of remediation efforts. Range pollution also has potential impacts on the natural environment and human health. For example, large quantities of metal fragments released into the environment as a result of shooting activities can be partially transformed into metal secondary minerals, thereby increasing the potential for the transport of heavy metals in the environment and expanding the scope of heavy pollution (Dermatas et al., 2006b). Inorganic heavy metals and organic pollutants, etc., in the range may pollute groundwater and nearby surface water through migration, etc., affecting the health of aquatic ecosystems (Mendes et al., 2023). In addition, direct exposure of humans to the environment around shooting ranges, inhalation of dust, or ingestion of heavy metals such as Pb, Zn, Ni, As and other (loid) heavy metals through the food chain can lead to a certain degree to cardiovascular disease, anemia, kidney damage and even cancer, etc. (Urrutia-Goyes et al., 2017; Kazery et al., 2021; Ni et al., 2023a). In contrast, only a small amount of Pb contamination ingested by adults through the various exposure routes enters their bloodstream, while the amount of lead that enters a child’s bloodstream can be much higher. Pollutants of all kinds (especially the heavy metal Pb) are particularly dangerous to infants and young children, and can cause irreversible damage to their developing brains and nervous systems (Fayiga and Saha, 2016b). Consequently, the remediation of contaminated range sites and the mitigation of hazards posed to surrounding soils and waters resulting from range activities hold paramount importance in enhancing the environmental quality and public health of such sites.
Based on our current understanding, a comprehensive assessment of the contamination levels and remediation efforts at range sites is lacking. In this study, we aim to provide a concise overview of the significant research developments over the past decades, followed by an examination of the present contamination status across various countries. Furthermore, we will emphasize the remediation strategies employed and the associated challenges encountered in this context. In addition, we emphasize gaps in future research with a view to harvesting better ways of managing pollution at range sites.
2 Bibliometric analysis of abandoned ranges
The detrimental impact of pollution stemming from military activities extends to both the environment and human health (Gómez-Sagasti et al., 2021). Of particular concern on a global scale is the escalating issue of heavy metal contamination in soils resulting from conventional shooting practices. Neglected ranges, lacking any form of remediation, pose a significant threat to environmental pollution (Sanderson et al., 2018; Reigosa-Alonso et al., 2021). The disintegration and deterioration of ammunition contribute to heightened levels of toxic metals within the soil, with the degree of contamination intensifying as the range ages (Fayiga and Saha, 2016a). In spite of the benefits offered by these metals in the production of ammunition, the presence of heavy metals, such as lead, can result in detrimental effects on the human nervous system, kidneys, and other bodily functions by interfering with the proper functioning of biological enzymes (Dinake and Kelebemang, 2019). Current research on the remediation of contamination at the range site focuses on soil flora characterization, hyper-enriched plants, health risks, and the uptake and transformation of pollutants (Lewińska et al., 2019; Wolf et al., 2020; Luo et al., 2023).
Figure 1 illustrates the quantity of publications pertaining to pollution remediation of target range sites from 2001 to the present. Since the onset of the 21st century, scholarly investigations in this domain have experienced a gradual upsurge, reaching their pinnacle during the period of 2017–2020, with an average annual publication count of 24.5. Subsequently, the number of publications in this field exhibited a decline. The 266 relevant literatures screened in the WOS database were used as the data source and imported into the bibliometric analysis software Citespace to draw the keyword clustering map with keywords as the emergence (Figure 2). There are 280 keyword nodes (N), and 507 connecting lines (E) in the keyword clustering map. Among them, the top five keywords with the highest frequency were heavy metals, remediation, lead, phytoremediation, and accumulation, and the five keywords with the highest centrality, i.e., the strongest connections to the rest of the keywords, were plants, shooting range soils, growth, phytoremediation and accumulation (Supplementary Table S3). The remaining research statistics in related fields are described in detail in the Supplementary Material.
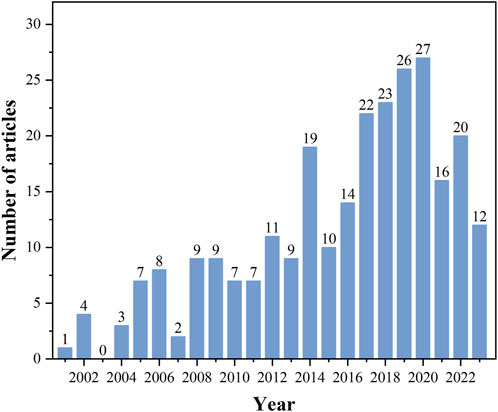
FIGURE 1. WOS database literature publications in the field related to remediation of contaminated sites at target ranges, 2001–2023.
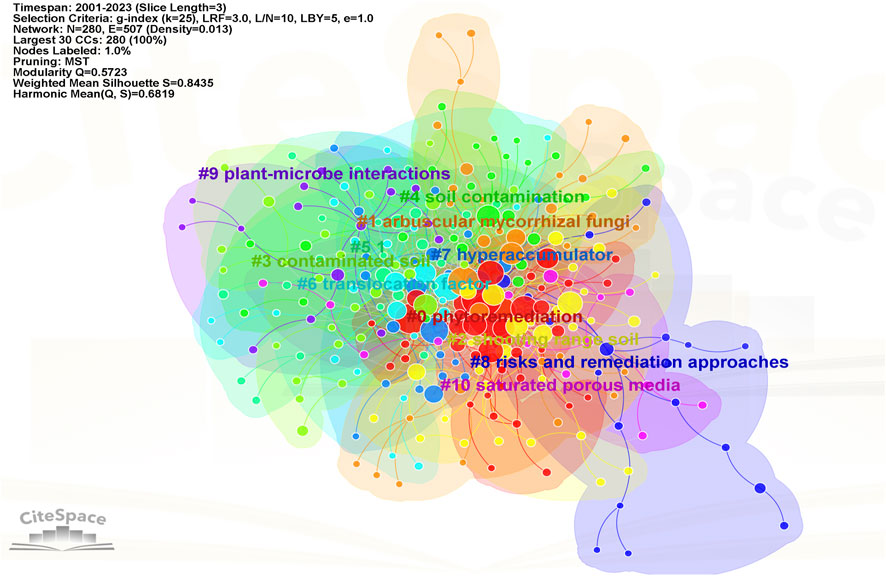
FIGURE 2. Keyword clustering of literature related to remediation of contaminated sites at the range, 2001–2023.
3 Contaminants in contaminated sites at firing ranges
The issue of soil contamination at firing ranges has garnered growing scholarly interest in recent years, primarily due to the substantial accumulation of pollutants resulting from shooting activities and the extensive global distribution of these ranges. Statistical data reveals that the U.S. Department of Defense is responsible for supervising over 3,000 military ranges dedicated to light weapons, alongside approximately 9,000 non-military outdoor ranges. According to the National Shooting Sports Foundation, it is estimated that there are approximately 10,000 firing ranges in the United States (Fayiga and Saha, 2016a). In comparison, Finland possesses around 2,000 firing ranges, which accounts for 10% of the overall number of contaminated sites. The range soils in these locations contain various contaminants such as lead (Pb), antimony (Sb), copper (Cu), selenium (As), zinc (Zn), nickel (Ni), chromium (Cr), and polycyclic aromatic hydrocarbons (PAHs). These contaminants primarily originate from warheads and warhead fragments, cartridges and cartridge fragments, targets, and various additives.
3.1 Heavy metals and metalloids
After being discharged, bullets make contact with the soil and undergo weathering, resulting in their fragmentation and pulverization. This process leads to the accumulation of substantial amounts of heavy metals in the soil, with firing ranges being recognized as a potential origin of soil contamination by diverse heavy metals (Sanderson et al., 2018). Lead constitutes the predominant element in lead bullets, comprising 93.1% of their overall mass (Laporte-Saumure et al., 2011). Lead bullets have been extensively employed worldwide over recent decades, establishing lead as the primary material utilized in bullet production (Pain et al., 2019). Consequently, firing ranges emerge as the second most significant source of lead (Pb) contamination in the environment, following the battery industry. Furthermore, copper (Cu) finds application in jacketed bullets, antimony (Sb) serves as a hardening agent for lead, nickel (Ni) or zinc (Zn) are employed as alloys in copper jacketed bullets, arsenic (As) coexists with lead in shotgun shells, and chromium (Cr) acts as an alloy in certain bullet varieties (ITRC, 2003; Laporte-Saumure et al., 2011). Despite constituting a minor proportion of a bullet’s overall mass, the persistent utilization of firing ranges is anticipated to lead to the gradual buildup of these metallic elements, particularly copper (Cu) and antimony (Sb), within range soils. The prevalence of heavy metal contamination in shooting range soils has garnered significant attention, with reports of such contamination emerging from diverse regions across the globe.
According to data published by the American Lead Industry Almanac, the utilization of lead in ammunition manufacturing amounted to 85,300 and 79,300 tons in 2014 and 2015, respectively. This accounted for 6% of the overall lead consumption mix in the United States, ranking second highest after the usage of lead-acid batteries (Guberman, 2017). The findings of a survey conducted by Bannon et al. (2009) on firing ranges in eight states in the U.S. revealed that the concentration of total Pb in topsoil ranged from 4,549 to 24,484 mg/kg, significantly surpassing the limit established by the USEPA, which is set at 400 mg/kg. Furthermore, the soil is also subjected to environmental risks due to elevated levels of Sb (ranging from 7 to 91 mg/kg), Cu (ranging from 223 to 2,936 mg/kg), Ni (ranging from 3 to 247 mg/kg), Zn (ranging from 102 to 284 mg/kg), Mn (ranging from 83 to 930 mg/kg), and As (ranging from 2.8 to 27.9 mg/kg).
In Korea, there exists a considerable number of active small arms firing ranges, totaling 1,400 (MOE, 2005). However, there is a dearth of information regarding the impact of heavy metals found in lead bullets on the soil within military firing ranges (Moon et al., 2013). Lee and Park, (2008) conducted a comparative analysis of heavy metal concentrations at the Maehyang-ri shooting range and other firing ranges in Korea. Their investigation revealed the predominant heavy metal contaminants at the Maehyang-ri shooting range. Notably, the copper concentration in the study area was found to be 23 times higher than the average soil concentration in Korea (114.4 ± 5.7 mg/kg), while the lead concentration exceeded the soil contamination standard (362.3 ± 20.5 mg/kg) by 1.2 times. In their study, (Moon et al., 2013), conducted an analysis of the geochemical properties of soils contaminated with heavy metals at a military shooting range in South Korea. The findings revealed significant contamination of Pb in the range soils, with evidence indicating varying degrees of anthropogenic Pb sources. Specifically, the surface (0–10 cm), subsurface (10–30 cm), and deep (30–50 cm) soils exhibited concentrations of Pb ranging from 5,127 to 28,040 mg/kg, 4,147–24,043 mg/kg, and 185–19,763 mg/kg, respectively. Additionally, trace amounts of Cu, Ni, Cd, and Zn were also detected.
According to Okkenhaug et al. (2018), Switzerland possesses a total of 2,000 firing ranges, which collectively contribute to the deposition of approximately 400–500 tons of lead bullets annually. In a study conducted by Knechtenhofer et al. (2003) in Losone, Ticino, Switzerland, the soil of these firing ranges was examined, revealing the presence of various heavy metals such as Pb (ranging from 22 to 12,533 mg/kg), Sb (ranging from 0.3 to 676 mg/kg), Cu (ranging from 9.8 to 148.9 mg/kg), Ni (ranging from 43.9 to 64 mg/kg), Zn (ranging from 57.4 to 87 mg/kg), and Mn (ranging from 180 to 550 mg/kg). In their study, Česynaitė et al. (2023) investigated a military range located in Alytus, Lithuania. Their findings revealed the presence of Pb concentrations reaching up to 54,600 ± 5,300 mg/kg in soils situated 45 m away from the firing line. Similarly, Česynaitė et al. (2021) conducted their own analysis on soils within the same area, at distances ranging from −5–45 m from the firing line. Their results indicated the presence of Pb concentrations ranging from 217 to 53,023 mg/kg, as well as varying concentrations of Sb, Cu, Ni, Zn, and Mn, with values reaching up to 59,978 mg/kg, respectively. The concentrations of antimony (Sb), copper (Cu), nickel (Ni), zinc (Zn), and manganese (Mn) were observed to range from 0 to 599.78, 6.1 to 24, 3.7 to 11.5, 1.7 to 2.7, and 170–215 mg/kg, respectively.
In their study, Wang et al. (2022) examined the attributes of heavy metal pollution in the uppermost layer of soil within a military training field for heavy weapons in Tibet, China. Additionally, the researchers assessed the potential ecological hazards associated with heavy metal contamination in the uppermost layer of soil within an artillery range situated at the aforementioned military training field. The findings indicate that the concentration of heavy metal elements, specifically As (ranging from 25.2 to 122 mg/kg) and Cu (ranging from 20.6 to 116 mg/kg), in the soil of the firing range exceeded the background levels observed in the surrounding regional soil. This disparity can be attributed to the dissimilar composition of gunpowder and metal materials used in heavy weapons compared to light weapons. The extensive use of copper-coated steel cartridges and arsenic-containing gunpowder during artillery landing and bombing operations has resulted in the contamination of heavy metal elements, namely As and Cu.
In Norway, there exists an estimated count of 3,000 firing ranges, encompassing both civilian and military facilities. Among these, 1,700 are currently operational. Furthermore, the production of bullets necessitates the annual consumption of approximately 700 tons of lead (Pb) and copper (Cu) (Okkenhaug et al., 2018). Consequently, the utilization of these bullets engenders an environmental hazard, impacting not only the soil within the ranges but also the surrounding aquatic ecosystem. The study conducted by Mariussen et al. (2017) examined the environmental conditions of mires and revealed relatively low concentrations of Pb (13 mg/kg), Sb (0.83 mg/kg), Cu (5.2 mg/kg), and Zn (1.1 mg/kg) in the soil. However, elevated concentrations of Pb (2,500 μg/L), Sb (150 μg/L), Cu (900 μg/L), and Zn (1,600 μg/L) were detected in the Subsurface soil water. These high concentrations of heavy metals in the Subsurface soil water are consistent with the findings of Okkenhaug et al. (2018), which also indicate heavy metal pollution in the groundwater and downstream surface water surrounding the range. Bullets used on firing ranges contain mainly Pb, Cu, Zn and Sb, while lead-free bullets contain steel (Johnsen and Aaneby, 2019).
Table 1 presents a comprehensive compilation of reports pertaining to the overall environmental heavy metal content found in firing ranges across various countries. The concentrations of copper and lead in the soils of these firing ranges exhibit significant variability, which can be attributed to factors such as range utilization, soil properties, and geographical location.
3.2 Organic substance
In contrast to the extensive attention given to the public health implications associated with heavy metals like Pb and Sb in shooting range soils, there exists a notable dearth of research conducted by scholars on the topic of organic matter in these soils. The concentrations of various organic contaminants at contaminated sites are provided in Table 2 for reference.
Clay targets were composed of coal tar pitch or petroleum (30–32 wt%), clay or limestone (67–70 wt%), and fluorescent paint. Each kilogram of clay targets contains 3,000 ∼ 40,000 mg of PAHs. Significant PAHs contamination was generated at the outdoor range due to the accumulation of clay target debris. Rodríguez-Seijo et al. (2017) investigated soil from an abandoned shooting range in Monforte de Lemos, NW Spain. PAHs detected included acenaphthylene (6.0 ∼ 56 mg/kg), acenaphthene + fluorene (0.58 ∼ 5.4 mg/kg), phenanthrene, anthracene (0.25 ∼ 2.4 mg/kg), fluoranthene (5.5 ∼ 30 mg/kg), pyrene (3.5 ∼ 28 mg/kg), Benzo [a]anthracene (2.8 ∼ 29 mg/kg), Chrysene (2.7 ∼ 27 mg/kg), Benzo[b]fluoranthene (6.8 ∼ 70 mg/kg), Benzo[k]fluoranthene (1.9 ∼ 19 mg/kg), benzo [a]pyrene (3 ∼ 35 mg/kg), Dibenzo[a,h]anthracene (2.1 ∼ 26 mg/kg) and Indeno[1,2,3-c,d]pyrene + benzo[g,h,i]perylene (1.8 ∼ 23 mg/kg). The total concentration of polycyclic aromatic hydrocarbons (PAHs) in the soil ranged from 38 to 360 mg/kg, significantly surpassing the background value for natural Spanish soils (0.61 mg/kg). Based on chemical and ecotoxicological data, the soils in this particular area pose a high risk. In a study conducted in California and Florida, United States, researchers investigated outdoor range soils and found total PAH concentrations of 2,431 and 1,324 mg/kg, respectively (Frakes et al., 2007). Wolf et al. (2020) demonstrated that decommissioned firing ranges in California, USA, had a total PAH concentration of 373 mg/kg, with high molecular weight PAHs accounting for 84% of the total.
Nitroglycerine (NG), 2,4-dinitrotoluene (2,4-DNT) and 2,6-Dinitrotoluene (2,6-DNT) are commonly used as energetics, stabilizing agents, or filler compounds in small arms ammunition. They are therefore deposited in the soil during military training. In their study, Clausen et al. (2011) examined the presence of NG, 2,4-DNT, and 2,6-DNT in soils at Camp Edwards, United States. The concentrations of these contaminants were found to be below 0.02 ∼ 69.64, below 0.014 ∼ 1.51, and below 0.18 mg/kg, respectively. Through their analysis of NG, 2,4-DNT, and the fate of 2,6-DNT, the authors established the existence of a potential threat to the groundwater at Camp Edwards, Massachusetts Military Reservation.
The release of chemical constituents and degradation intermediates from munitions occurs both during the manufacturing process and as a result of explosions in military activities. Examples of such compounds include RDX (1,3,5-trinitro-1,3,5-triazine), TNT (2,4,6-trinitrotoluene), HMX (1,3,5,7-tetranitro-1,3,5,7-tetrazacyclooctane), among others. These substances, even at low concentrations, have the potential to endanger the ecosystem. Robidoux et al. (2004) conducted an assessment of the toxicity levels of RDX, TNT, and HMX in soil samples obtained from a military contaminated site located in Western Canada. The concentrations of these substances were measured to be less than 0.25–2.8 mg/kg for RDX, less than 0.25–0.49 mg/kg for TNT, and 14–696 mg/kg for HMX. Additionally, the study also determined the presence of two metabolites of TNT, namely, 2-amino-4,6-dinitrotoluene (2-ADNT) and 4-amino-2,6-dinitrotoluene (4-ADNT), with concentrations ranging from less than 0.25–1.06 mg/kg for 2-ADNT and less than 0.25–6.68 mg/kg for 4-ADNT. The findings of Jugnia et al. (2019, 2018) revealed that soil from contaminated sites in Eastern Ontario, Canada, may potentially endanger soil organisms, as indicated by toxicity evaluations conducted on microorganisms, earthworms, and plants. The concentration of RDX, a military-related contaminant, was determined to range from 0 to 0.4 mg/kg in soil and 3.52 ∼ 196.7 μg/L in groundwater at the aforementioned site.
4 Contaminated site remediation technologies for decommissioned firing ranges
Currently, contaminated soils at range sites are remediated mainly by physical remediation, chemical remediation, and bioremediation (Table 3). Among them, physical remediation includes soil replacement, mechanical screening, electric remediation and vitrification. Physical remediation technology has a shorter remediation cycle and is suitable for short-term pollution remediation. Chemical remediation is mainly represented by curing/stabilization, chemical drenching and electrodialysis remediation, which can realize efficient remediation of highly polluted sites (Befkadu and Chen, 2018; Ni et al., 2023b). Bioremediation has become more popular in recent years, mainly including phytoremediation and microbial remediation, which is low-cost and a kind of environmentally friendly in-situ remediation technology with a broader development prospect (Xu et al., 2019; Raimondo et al., 2020).
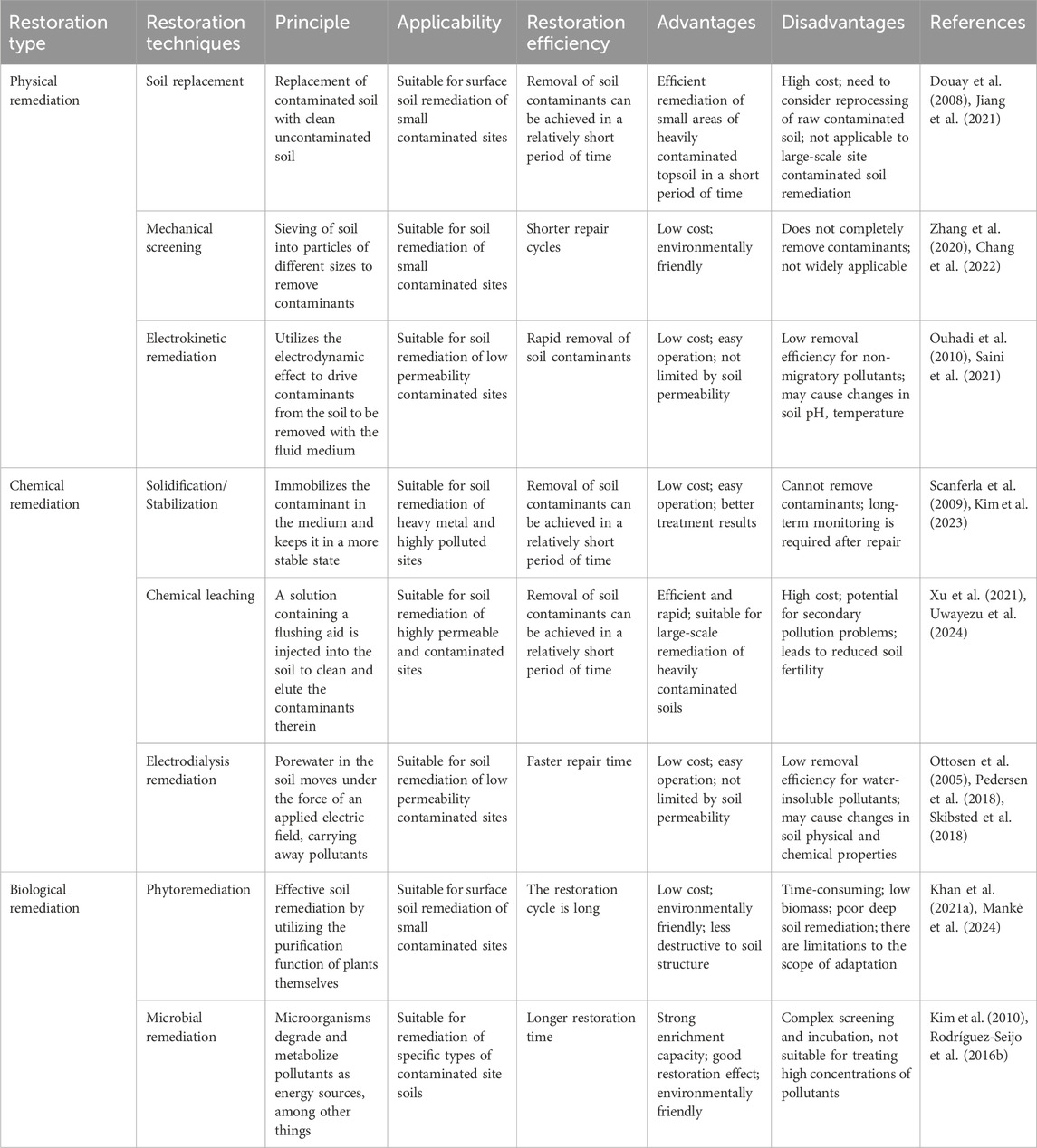
TABLE 3. Comparison of several major remediation technologies for contaminated sites at shooting ranges.
4.1 Physical restoration techniques
Common physical remediation techniques employed for contaminated soils at firing ranges encompass soil replacement, mechanical screening, electrokinetic remediation and vitrification (Figure 3).
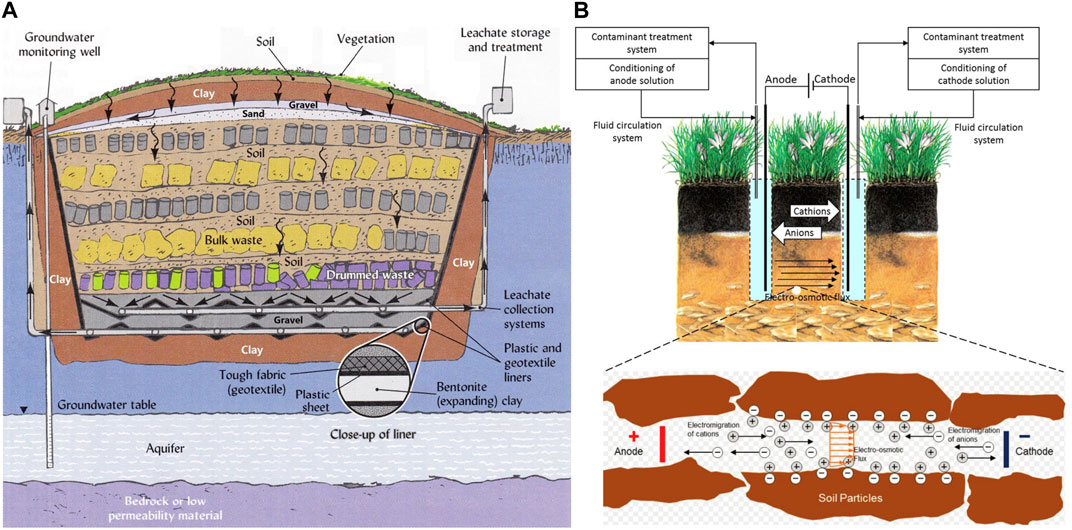
FIGURE 3. Physical restoration techniques. (A) Schematic cross-section of the soil filling process. This figure has been reprinted from Liu et al. (2018), with permission of the publisher. (B) Removal of ionic contaminants from soil by electrokinetic remediation techniques and its main mechanisms. This figure has been reprinted from Cameselle et al. (2013), with permission of the publisher.
4.1.1 Soil replacement
The conventional approach of directly excavating and transporting contaminated soil to a landfill is merely a customary method (Sorvari et al., 2006). Nevertheless, given the remote nature of range locations and their limited asset worth, the utilization of such mass transportation proves to be economically unviable and unsuitable for the remediation of contaminated soils originating from firing ranges (Allen, 2001).
4.1.2 Mechanical screening
Mechanical screening can be effective in removing large particle size contaminants such as bullets and their fragments. In their study, Yin et al. (2010) employed three effective management practices to address the detrimental environmental effects of lead bullets in range soils. These practices included the substitution of soil berm with sand berm, the application of lime to the sand berm, and the removal of lead bullets from berm soils through mechanical sieving. The replacement of soil berm with sand berm resulted in a reduction of the total soil Pb content from 277 to 57 mg/kg after a period of 11 months. The substitution of a soil berm with a liming sand berm resulted in a decrease in the overall concentration of lead (Pb) in the soil, reducing it from a range of 497–777 mg/kg to a range of 302–362 mg/kg after a period of 15 months. Despite the elimination of the initial source of soil Pb through mechanical sieving, the sieving process itself introduced metallic Pb into the soil particles smaller than 2 mm, leading to an increase in the total soil Pb content from 4,694 to 11,479 mg/kg. Thangavadivel et al. (2018) employed a heavy particle concentrator and orbital screening technique to eliminate lead particles. Subsequently, they subjected the treated soil to high-performance concrete as a secondary treatment. This combined approach successfully achieved a 91% removal of total lead content. Moreover, the resulting lead concentration in the treated soil fell below the threshold set by the Australian National Environmental Protection Measure Health Investigation Level for Soil Contaminant.
4.1.3 Electrokinetic remediation
Electrokinetic remediation is a class of in situ physical remediation technology, mainly by applying a small electric current between the anode and cathode in the contaminated soil to achieve the removal of pollution (Saini et al., 2021). Cai et al. (2022) found that both continuous power supply and intermittent power failure can effectively remove Cd contamination from soil through a comparative experiment of soil electrokinetic remediation, in which the efficiency of remediation with intermittent power failure is higher. In addition to effective remediation of inorganically contaminated soils, electrokinetic remediation technology can be used in combination with surfactants, nanoparticles and oxides for effective removal of soil organic matter (Cameselle and Gouveia, 2018).
4.1.4 Vitrification
In-situ vitrification technology converts heavily contaminated soils into inert and non-migratory vitrified briquettes, reducing the spread and dispersion of soil contamination (Shu et al., 2020). The technology can effectively control the contamination of soil with many heavy metals, including radionuclides (Buelt and Farnsworth, 1991; Yasunari et al., 2011). However, the soil vitrification treatments were relatively long and the soil immobilization was not homogeneous (Chen et al., 2019).
4.2 Chemical remediation technology
There are very extensive studies on chemical remediation techniques for remediating contaminated soils at the range, including solidification/stabilization, chemical leaching, electrodialysis, and others.
4.2.1 Solidification/stabilization
The process of solidifying/stabilization involves the incorporation of solidifying/stabilizing agents into the treated soil, resulting in the transformation of toxic heavy metals present in the soil into insoluble forms that exhibit reduced mobility and toxicity (Figure 4A). This process effectively hinders the leaching of heavy metals, thereby rendering it environmentally sustainable. Additionally, the treated soil can be reused, further enhancing the overall efficacy of this technology. Furthermore, the versatility of this approach allows for its application in various scenarios (Mulligan et al., 2001; Guo et al., 2006). Notably, in certain instances, this technology has demonstrated the capability to diminish soil lead concentrations to levels that comply with the maximum contamination limits established by both the U.S. Environmental Protection Agency (USEPA) and the World Health Organization (WHO) (Dinake and Kelebemang, 2019). Stabilization techniques can also be used in conjunction with other remediation tools to achieve higher removal results. Remediation of co-contaminated soil by extraction/degradation of organic contaminants and stabilization of Pb, Islam and Park, (2017) investigated the effect of hydrothermal treatment under subcritical conditions on the immobilization of lead in contaminated soil from a target range. The process resulted in a substantial conversion of the bioavailable portion of lead into a comparatively stable fraction, leading to a reduction in the bioavailable fraction from 41.33% to 14.66%. The application of high-temperature treatment appears to be effective in reducing the bioavailability and ecotoxicity of soils contaminated with Pb. Furthermore, the remediation of the affected range soils resulted in a shift from a high risk of pollution and ecological risk to a medium risk (Islam and Park, 2017). Commonly used solidification/stabilization materials include phosphorus-containing materials (poultry wastes, waste cattle bones, and gypsum wastes) (Cao et al., 2009), lime-containing materials (lime, eggshells, oyster shells, and mussel shells) (Cui et al., 2023), biochar materials (Shen et al., 2016), and iron-based materials (iron salts, nanoFe) (Azizi et al., 2017).
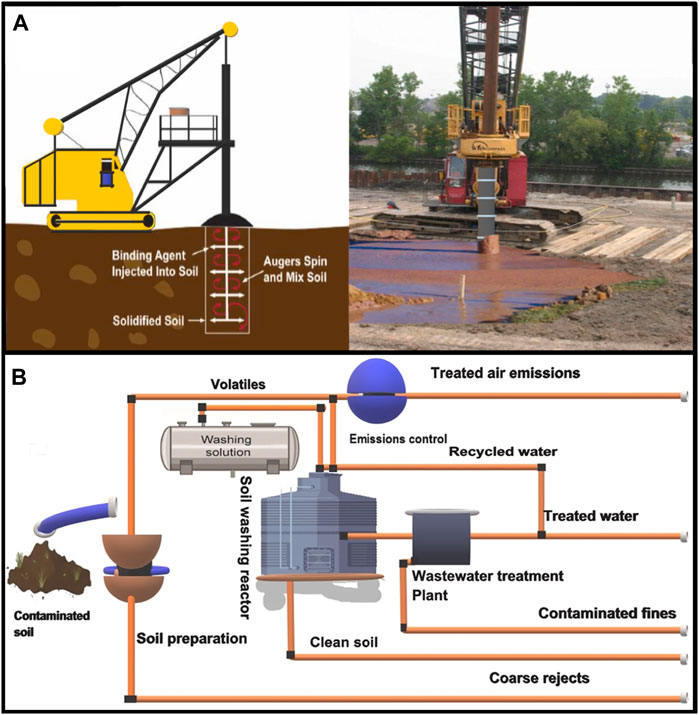
FIGURE 4. Examples of chemical remediation techniques. (A) Chemical immobilization technique; (B) Schematic diagram of the chemical leaching process. This figure has been reprinted from Khan et al. (2021), with permission of the publisher.
Phosphate-stabilized remediation has been implemented as a means of regulating the movement and accessibility of heavy metals within range soils, owing to its capacity to stabilize lead-phosphate deposits across a broad pH spectrum. The substances employed for phosphate stabilization encompass hydroxyapatite, phosphate rock, and phosphates (Ogawa et al., 2015; Han et al., 2022; Mi et al., 2023). The findings of the investigation conducted by Fayiga and Saha, (2016a) demonstrated a substantial decrease in Pb levels, from 800 mg/L to below 1 mg/L, subsequent to the application of phosphate rock and phosphoric acid in range soil. In their study, Sanderson et al. (2015) employed phosphate amendments for the remediation of polluted soils in a firing range. The application of phosphate resulted in a notable reduction of lead bioavailability in the soil, with a decrease of 55%. Furthermore, the leaching rate and weathering degree of lead bullets exhibited a significant decline, reaching up to 98%, following the application of phosphate onto the bullet surfaces. In their study, Ogawa et al. (2015) employed a combined application of hydroxyphosphate lime and ferrihydric compounds, resulting in the inhibition of 99.9% of water-soluble Pb and 95.5% of water-soluble Sb. These inhibitory effects were found to be superior to those observed when hydroxyphosphate lime or ferrihydric compounds were applied individually. Similarly, Yan et al. (2016) utilized alkali residues obtained during phosphorus adsorption to immobilize lead in soil within a limited area in Jiangsu, China. Phosphorus-loaded alkali slag and calcined phosphorus-loaded alkali slag exhibited conversion rates of over 60% and 90% for soil Pb into residual fractions, respectively. Conversely, raw alkali slag and calcined alkali slag without phosphorus only achieved conversion rates of 14% and 35% for soil Pb into residual fractions, respectively. It is important to acknowledge that the remediation of phosphate in range soils necessitates careful consideration of the potential eutrophication risk stemming from elevated phosphorus application rates.
Lime-based materials have proven effective in serving as remediation agents for contaminated soils at the range due to their ability to adsorb heavy metals and/or form insoluble minerals in the soil (Li et al., 2019; Sun et al., 2023). In a study conducted by Levonmäki and Hartikainen, (2007), the remediation efficiencies of two lime-based agents with varying reactivities, namely, calcium carbonate and blast furnace slag, were compared in relation to Pb remediation in range soils. After a 21-day reaction period, it was found that calcium carbonate exhibited greater mobility in reducing Pb compared to blast furnace slag. In their study, Conesa et al. (2010) effectively employed the application of Ca(OH)2 and humic acid amendment to extract metallic antimony from various soil samples in Switzerland. Ahmad et al. (2012), on the other hand, utilized eggshell waste as a means to immobilize Pb in a military firing soil in South Korea. Their findings revealed that the inclusion of 5% eggshells and calcinated eggshells resulted in a significant reduction of the TCLP-Pb content in the soil by 68.8%. Moreover, the Pb content and performance achieved with these additions were comparable to those achieved with pure CaCO3 and CaO, respectively. Therefore, these modifiers have the potential to be used as lead fixatives as alternatives to calcium carbonate and calcium oxide.
In recent years, there has been a growing interest in the utilization of natural or waste materials for the immobilization of trace metals, primarily due to their cost-effectiveness. One such material, biochar (BC), has been recognized as an effective adsorbent for the immobilization of trace metals (Zou et al., 2023). In a study conducted by Ahmad et al. (2012), highly contaminated military range soil in South Korea was treated using mussel shells, bovine bone, and biochar. The findings revealed that the bioavailability of lead in the soil treated with mussel shells, cow bones, and biochar was reduced by 92.5%, 84.8%, and 48.5%, respectively. In their study, Ahmad et al. (2016) conducted an investigation utilizing soybean straw and pine needle derived biochar to assess the immobilization of Pb and Cu in range soils. The results demonstrated that the soybean straw based biochar exhibited a higher efficacy in immobilizing Pb (88%) and Cu (87%) compared to the pine needle based biochar. In their study, Rajapaksha et al. (2015) examined the impact of various soil amendments, including trichocarp ragweed biomass and its derived biochar at different temperatures (300°C and 700°C), natural iron oxides (NRE), alumina trihydrate, and silver nanoparticles, on the immobilization of heavy metals in the soils of the designated site following 1 year of soil cultivation. The findings revealed that all of these soil amendments resulted in a reduction in the concentration of extractable Pb and Cu in the soil. Notably, the biochar produced at 300°C exhibited the highest efficacy in immobilizing the heavy metals. In their study, Vithanage et al. (2017) examined the impact of carbon nanotubes and biochar derived from soybean straw on the bio-efficacy of Pb, Cu, and Sb in range soils. The researchers discovered that biochar produced from soybean straw effectively immobilized bioeffective Pb and Cu in the range soils, resulting in reductions of 17.6% and 16.2% respectively. This immobilization was achieved at a lower cost. However, neither modifier proved effective in immobilizing Sb. In a separate study, Silvani et al. (2019) developed a waste wood biochar and a wood shrub iron enriched design biochar for the remediation of low TOC range soils. The findings indicate that the inclusion of 20% BC resulted in a 61% reduction in Pb leaching and a 12% reduction in Sb leaching, while the addition of Fe-BC led to a 99% decrease in Pb leaching and a 40% decrease in Sb leaching. These results demonstrate the efficacy of modified biomass waste in remediating contaminated soils within the desired range.
It is worth noting that iron-based materials, including iron hydrate and acicular ferrite, are commonly employed as amendments in contaminated soils within the range. These iron-based adsorbents function by immobilizing heavy metals through the process of adsorption (Zhao et al., 2023). The weathering and corrosion of zero-valent iron (Fe0) occurs when exposed to air and moisture, resulting in the formation of different iron (hydrogen) oxides. These oxides create additional adsorption and binding sites for heavy metals in the soil (Wang et al., 2022). Okkenhaug et al. (2016) successfully employed iron-based adsorbents to immobilize Pb and Sb, achieving immobilization rates of 89%–90% for Sb and 89%–99% for Pb in heavy metal-contaminated soil at the Norway Range. Tandy et al. (2017) conducted a study to assess the impact of two red mud amendments (ViroSoilTM 1 and 2) and two reductant treatments (zero-valent iron and ferrous sulfate) on the leaching of metal (loid) from the soil in the Switzerland range. The purpose of their research was to investigate the effects of these treatments. The findings of the study demonstrated that the utilization of ferrous sulfate resulted in the reduction of SbV to SbIII, effectively preventing Sb leaching. However, it was observed that the acidifying effect of ferrous sulfate, along with the reducing and dissolving impact of manganese oxides, had a detrimental influence on Pb leaching. Moreover, the combination of red mud amendment and ferrous sulfate exhibited comparable efficacy to FeSO4 alone in immobilizing Sb and reducing Pb leaching. In a separate study conducted by Mariussen et al. (2017), ferric hydroxide powder and zero-valent iron powder were mixed in range soils to assess their overall effectiveness as stabilizers for contaminated soils in the range. The concentration of Pb, Cu and Zn in soil leachate was reduced by 79%–99% when these two additives were mixed into acidic soil. In their study, Barker et al. (2023) performed soil column experiments to investigate the impact of two remediation treatments, namely, ferric chloride/calcium carbonate and nano-zero-valent iron (nZVI), on the immobilization of Pb and Sb in range soils. The results revealed that the incorporation of nZVI had minimal effect on the immobilization of Pb and Sb in the soils. On the other hand, the amendments of FeCl2 and CaCO3 led to a reduction of over 80% in the concentrations of Sb in the soil pore-water. In their study, Khan et al. (2023) conducted the preparation of biochar derived from pine-one waste, followed by the enrichment of biochar with Fe and Al salts to create engineered biochar. The results indicated that all three biochars effectively immobilized Sb (V) within the soil range. Notably, the Fe biochar exhibited superior immobilization capacity compared to the other two biochars, attributed to the heightened affinity of Sb (V) towards the Fe-binding functional groups present on its surface.
4.2.2 Chemical leaching
Chemical leaching, which involves the use of acids, bases, and chelating agents, is a commonly employed and effective method for treating heavy metals in range soils due to its rapid remediation capabilities and cost-effectiveness (Figure 4B and Figure 5). In the study conducted by Lafond et al. (2012), it was found that the application of 1 M sulfuric acid and 4 M sodium hydroxide effectively eliminated 75% of the Pb in the contaminated soil from a Canadian range, as well as Cu, Sb, and Zn. Lafond et al. (2013) employed chemical leaching techniques to eliminate heavy metal contaminants from a small Canadian military range. Through their investigation, they determined that the combination of sulfuric acid leaching and the introduction of sodium chloride proved to be the most efficient approach. Furthermore, when implemented alongside a continuous leaching process, this method achieved a removal rate of 96% for Cu, 99% for Pb, 84% for Sb, and 86% for Zn in Canadian military small ranges. Subsequently, a counter-current leaching process was employed, resulting in the removal of 93.2% ± 3.5%, 91.5% ± 5.7%, 82.2% ± 10.9%, and 30.0% ± 11.4% of Cu, Pb, Sb, and Zn, respectively, from the targeted range soils (Lafond et al., 2014).
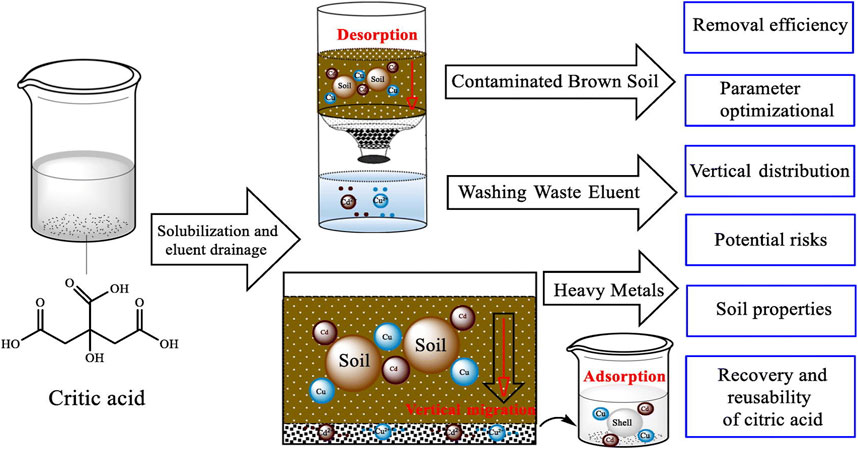
FIGURE 5. Removal of Cd and Cu from contaminated soil by combined solubilization and leachate drainage systems. This figure has been reprinted from Yang et al. (2022), with permission of the publisher.
Although ethylenediaminetetraacetic acid (EDTA) is a commonly used chelating agent, its toxicity and persistent presence in the environment make it unsuitable for long-term application (Wu et al., 2015). In their study, Ni et al. (2023a) investigated the substitution of [S,S]-ethylenediamine succinic acid, N,N-dicarboxymethyl glutamic acid, and 3-hydroxy-2,2-iminodisuccinic acid for EDTA in the Japanese Imari Range soil to facilitate cleaning and chemical immobilization. The authors found a positive correlation between the soil washing parameters and the Pb removal effect of the chelating agents. Furthermore, the application of FeCl3 and CaO as post-treatment significantly reduced the soluble Pb content in the soil that underwent chelator washing. This remediation strategy, which combines chemical leaching with curing/stabilization, can be a practical treatment method for extracting large amounts of lead from soil and inhibiting residual lead leaching. Etim, (2017) employed acetic acid in conjunction with varying concentrations of potassium chloride and the electrochemical reduction method to address soil remediation in Nigeria. Notably, the utilization of a 5% acetic acid and 5% KCL wash solution yielded the most favorable outcomes, with lead removal rates ranging from 74.9% to 86.9%. This particular solution exhibits potential as a viable alternative to potent acids and chelating agents. Moreover, subsequent treatment of the leachate containing Pb through the utilization of Al-Al and Al-Fe bipolar electrodes resulted in removal rates of 93.7% and 94.5% respectively.
The presence of high concentrations of strong acids in heavy metal-contaminated range soil, which is intended for reuse, poses a significant risk to soil microorganisms and can adversely affect the soil’s physicochemical properties. Additionally, chelating agents are not a viable option for treating range soil due to their costly nature and drawbacks, including limited treatment efficacy and the requirement for subsequent treatment.
4.2.3 Electrodialysis remediation technology
Electrodialysis remediation technology offers a solution by effectively extracting heavy metals from the soil, resulting in a final concentration below the established effects levels (Figure 6). In their study, Pedersen et al. (2019) employed multivariate analysis to concurrently evaluate the impacts of soil properties and experimental variables on the electrodialysis process for the elimination of Cu and Pb from soils at three designated locations. To represent in situ and ex situ remediation conditions, laboratory-scale fixed and agitated units were utilized. The agitation unit exhibited higher removal rates of Cu and Pb (ranging from 9% to 81%) compared to the fixed unit (ranging from 0% to 41%) within the identical experimental setting. The findings of the multivariate analysis (MVA) indicate that the impact of soil type on the effectiveness of remediation is contingent upon the specific metal being targeted and varies across different fixation and agitation configurations. Enhancing the removal of copper through adjustments to the experimental setup is more feasible in the agitation setup, which can be achieved by augmenting the current density. On the other hand, optimizing the removal of lead can be accomplished by prolonging the treatment duration and, in the agitation setup, by also increasing the current density.
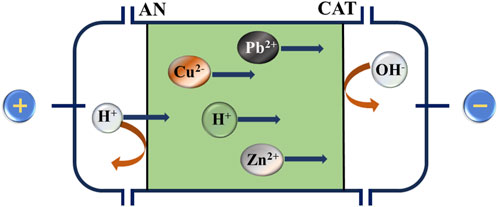
FIGURE 6. Schematic diagram of soil electrodialysis remediation technology. Where AN denotes an anion exchange membrane and CAT denotes a cation exchange membrane.
4.3 Bioremediation
Bioremediation is currently a popular remediation technology for site contamination remediation (Figure 7). This remediation method utilizes a biodegradation process to remove contaminants from the environment, thereby minimizing environmental risks (Seagren, 2024). For various types of inorganic metals and organic pollutants such as polycyclic aromatic hydrocarbons (PAHs) in the contaminated soil of the target range, common bioremediation methods include phytoremediation and microbial remediation techniques (Ni et al., 2023a; Ansari et al., 2023).
4.3.1 Phytoremediation
Certain plant species possess the capacity to assimilate and endure deleterious heavy metals present in the soil within a given area. These plants can be employed to hyperaccumulate the aforementioned heavy metals, thereby facilitating the utilization and regulation of soil contaminated with heavy metals in the above-mentioned area (Figure 8). Consequently, this procedure curtails the movement and percolation of heavy metals in the soil by immobilizing and stabilizing them, thereby diminishing their interaction with organisms, a phenomenon known as phytoremediation. Hyperaccumulating plants can transfer toxic heavy metals that they have absorbed to the above-ground parts of the plant, such as shoots and leaves, at concentrations significantly higher (ranging from 100 to 1,000 times) than those observed in non-hyperaccumulating plants. Additionally, it is noteworthy that the absorption of these high concentrations of heavy metals does not elicit any toxic effects on the plant (Singh et al., 2016). The ability of plants to accumulate metals is regulated by their growth rate and their capability to translocate metals to aboveground tissues. In situ phytoremediation can manifest in various forms, including phytoextraction, root filtration, phytostabilization, and phytovolatilization. However, two main forms of phytoextraction and phytostabilization have been used in the contaminated soil of the range.
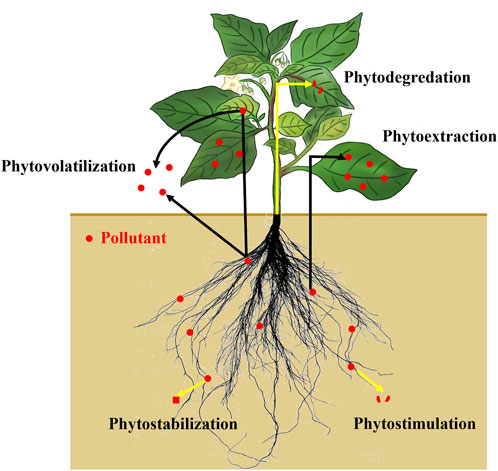
FIGURE 8. Phytoremediation mechanism diagram. Main pathways of Phytoremediation includes phytodegradation, phytovolatilization, phytoextraction, phytostabilization and phytostimulation.
Phytoextraction is the process of utilizing specific plant species to extract contaminants from the soil, thereby reducing the overall concentration of soil pollutants (Suresh and Ravishankar, 2004). Perennial herbaceous plants, due to their rapid growth, substantial biomass, extensive root systems, and moderate tolerance to pollutants, are generally considered suitable candidates for remediating heavy metal contamination (Yang et al., 2005). Numerous plant species, including various brassicas, clover (Pennisetum), trillium (Panikum), willow, and tobacco, have been extensively studied for their phytoextraction capabilities to different metals (Grispen et al., 2006; Abdel-Sabour and Al-Salama, 2007; Neugschwandtner et al., 2008; Purakayastha et al., 2008). Significant advancements have been achieved in the utilization of this technique for the improvement and restoration of range soil. In their research, Koelmel and Amarasiriwardena, (2012) employed laser ablation-inductively coupled plasma mass spectrometry (LA-ICP-MS) to investigate the spatial distribution of Cu, Pb, Sb, and Zn within the rhizomes of herbaceous ferns in range soils. The findings of their study indicated that the inner amyloplast cortex adjacent to the cortex exhibited elevated concentrations of Pb, Cu, and Zn within the rhizome tissue. In the outer amyloplast cortex, Pb was found to be concentrated, while Cu and Zn were evenly distributed in the cortex on both sides at the control site. This finding provides a basis for the effective implementation of phytoextraction techniques in the remediation of soils containing Pb and Sb. According to a study conducted by Tariq and Ashraf, (2016), which compared the uptake of Pb by oilseed rape, sunflower, pea, and maize in soils with high Pb concentrations (1,331 mg/kg), peas demonstrated the ability to uptake up to 96.23% of Pb and exhibited the highest Bioaccumulation Coefficient (BCF), indicating its hyper-enrichment properties. (Khan et al., 2021). examined the efficacy of phytoremediation in addressing soil contamination caused by firing ranges in Pakistan. Through conducting room temperature experiments, the researchers discovered that both dogbane and annual herb quinoa demonstrated the capacity to absorb Pb from the soil via their root systems and subsequently transport it above ground. Moreover, these plants exhibited resilience even when exposed to higher doses, thus qualifying them as Pb hyperaccumulating plants. However, it is worth noting that quinoa displayed necrotic/greening toxic effects. Consequently, dogbane emerges as a more effective candidate for remediating Pb in shooting range soils. In their study, Conesa et al. (2011) conducted potting experiments to investigate the uptake of three Swiss plants in Pb-contaminated range soils. The results indicated that common wheat exhibited a higher absorption of Pb in its root system (∼200 mg/kg) compared to perennial ryegrass (130 mg/kg) and longleaf plantain (110 mg/kg). Furthermore, the bioconcentration factors (BCFs) for all three plants were found to be less than 1, suggesting that the utilization of these plants in phytoremediation practices for range soil contamination can effectively mitigate the risk of lead transfer to the food chain. Hockmann et al. (2018) examined the uptake of Sb, Pb, Cu, and Zn by four commonly found forage grasses, namely, perennial ryegrass, white clover, longleaf plantain, and bluntleaf sourmilfoil. The investigation took place in the field, specifically on abandoned and aerobic range soils, and involved both flooded and drained conditions. The findings revealed significant variations in the uptake of Pb, Cu, and Zn among the different species, with Pb and Zn exhibiting the most pronounced impact on metal uptake. Interestingly, the levels of lead and zinc in blunt-leaved Rhodiola rosea were found to be 2–4 times higher under drained conditions compared to flooded conditions.
Phytostabilization encompasses the utilization of plants, fertilizers, and soil amendments to sequester and immobilize micronutrient-contaminated soils, thereby diminishing their bioavailability (Nsanganwimana et al., 2015). Notably, certain biofuel crops, namely, manzanita and willowherb, have exhibited promising capabilities for plant stabilization in Eastern Europe and the United States of America (Andrejić et al., 2019). Additionally, grass species offer advantages as phytostabilizing plants due to their tendency to accumulate fewer metals in their above-ground tissues compared to other species. Wilde et al. (2005) observed a noteworthy increase in Pb concentration within the root system of vetiver, while the leaves showed minimal enrichment. This accumulation of Pb in the root system highlights the potential of vetiver for phytostabilization, as it can effectively control Pb levels in the soil and subsequently reduce the risk of contaminant diffusion. Furthermore, the study showcased the significant potential of utilizing vetiver in conjunction with diverse amendments for the treatment of lead-contaminated range soils. Andrés Rodríguez-Seijo et al. (2016a) discovered that filamentous shearwaters have the potential to serve as an effective plant stabilizer for lead in the soils examined, as well as in soils with comparable attributes. The findings revealed that the roots of the grass exhibited high concentrations of Pb (1,107 mg/kg), while the branches displayed lower concentrations.
The incorporation of amendments in the phytoremediation process of range soils can effectively enhance the plant’s ability to remediate such soils. Liu et al. (2013) observed a noteworthy rise in the quantity of exchangeable Sb in range soils after biochar treatment, but a decrease in accumulation in corn plants. Hashimoto et al. (2008) discovered that incorporating amendments, specifically poultry waste, into Panicum maximum Jacq. plants effectively stabilized high levels of Pb (19,600 ± 730 mg/kg) in the soil within a designated target range. This approach not only mitigated the cumulative dissolution of Pb in leachate and the concentration of Pb in water within the soil profile but also minimized the potential risk of Pb contamination in the target area. The findings of Alasmary et al. (2021) demonstrated that the incorporation of manzanita along with organic and inorganic phosphorus amendments yielded favorable outcomes in terms of stabilizing Pb in range soils, while concurrently mitigating potential environmental hazards and enhancing soil health.
4.3.2 Microbial remediation
Furthermore, alongside plants, the utilization of certain microorganisms can also be considered for range restoration purposes. Studies have shown that in response to environmental stresses at contaminated sites, microbial communities in soil adopt different strategies to cope with such environmental changes (Liu et al., 2023). Jugnia et al. (2019) observed that microorganisms effectively reduced RDX levels in groundwater in the range to below 0.1 μg/L. Notably, Geobacter, Clostridium, Klebsiella and Bacteriodales exhibited predominant activity as active organisms, and the introduction of waste glycerol further augmented the biodegradation of RDX. The utilization of waste glycerol in range soils demonstrated comparable enhancement of RDX biodegradation, while exhibiting limited impact on the biodegradation of 2,4-DNT (Jugnia et al., 2018). According to Lee and Kim, (2010), the utilization of a microbial-mediated leaching process known as bioleaching has been suggested as a means to eliminate heavy metals from soils and sediments. Among the microorganisms commonly employed in this process, Thiobacillus thiophilus sulfoxidans stands out as a prominent choice due to its capacity to support growth through the oxidation of reduced sulfide and utilization of carbon dioxide as an energy source for cellular metabolism. The acidophilic oxidizing Thiobacillus thiophilus was employed by the researchers to augment the biological activity in the bioleaching process. Additionally, they integrated acid-enhanced and EDTA-enhanced electrokinetic techniques, which demonstrated a notable removal of lead (92.7%) compared to non-biological methods. Moreover, plant roots contribute nutrients to root microorganisms, facilitating a synergistic plant and microbial remediation approach that exhibits exceptional efficacy (Sas-Nowosielska et al., 2008). Wolf et al. (2020) conducted a study utilizing integrated bioremediation techniques to address the presence of polycyclic aromatic hydrocarbons (PAHs) in soils contaminated by outdoor ranges. The researchers evaluated the effectiveness of various remediation approaches, including phytoremediation, bioaugmentation with Mycobacterium wambachii PYR-1, and the use of surfactant modifiers, either individually or in combination. The investigation revealed that the dissipation of PAHs, particularly in the inter-root region, notably in dogbane plants, was accompanied by alterations in enzyme activities and enhancements in lettuce seed germination. Repeated applications of Mycobacterium avium subspecies PYR-1 resulted in a significant enhancement in the dissipation of high molecular weight PAHs when compared to the control group without inoculation. Conversely, the application efficacy of rhamnolipid biosurfactant or Brij-35 was found to be limited, necessitating further investigations to determine the optimal concentration of surfactant and the interval for repeat application.
Bioremediation offers an advantage over alternative soil remediation techniques due to its minimal disruption to the ecosystem (Tangahu et al., 2011), and its ability to preserve soil structure throughout the remediation process. Significantly, bioremediation is characterized by minimal chemical usage, cost-effectiveness, and environmental friendliness (Sanderson et al., 2018). Moreover, bioremediation encompasses additional advantageous processes, including the capacity of plants to mitigate heavy metal accumulation in the soil through root system immobilization, thereby reducing soil erosion and weathering-induced dust emissions, as well as mitigating the adverse effects of rainwater runoff on soil quality.
5 Prospects
This paper highlights the research gaps and technical challenges that are crucial for the advancement of efficient remediation technologies in addressing range sites. Current research on pollution remediation technologies for range sites still has certain deficiencies. For example, most of the current research only stays at the level of theoretical and laboratory validation of pollution control, and the feasibility of practical operation is not fully considered. Meanwhile, experimental validation generally selects only a small sample, limiting the generalizability of the results. In addition, most of the current studies do not pay enough attention to the correlation between environmental factors such as temperature and precipitation at the range site and factors such as anthropogenic activities and site contamination, which also makes the research perspective more one-sided. The following aspects can be considered in the future to optimize the current research on contamination-free management of range sites.
(1) It emphasizes the predominant presence of Pb as the primary pollutant in firing ranges and the limited focus of researchers on remediating this pollutant. However, it is important to recognize that the associated contaminants, such as Sb, Cu, Zn, Ni, As, PAHs, and RDX, which are introduced through the weathering of munitions and clay targets, may possess equal or greater ecotoxicity. This is because these pollutants have a higher proportion of bioavailable metals than Pb or are highly bioconcentrated. Simultaneously, the researchers’ remediation measures were exclusively aimed at addressing soil contamination within the range area. However, it has been reported that elevated levels of contamination have also been detected in the surrounding groundwater and surface water (Okkenhaug et al., 2018; Jugnia et al., 2019). Furthermore, it is noteworthy that mercury (Hg) compounds were historically utilized as primers for rifle and pistol ammunition (Stauffer et al., 2017). Despite the discontinuation of mercury-containing munitions in the 1960s, the resulting pollution cannot be disregarded. Regrettably, limited research has been conducted in this area, and the natural recovery processes tend to be sluggish. Hence, it is imperative to direct attention towards the comprehensive contamination level of the range site, prioritizing the overall efficacy of site remediation rather than solely pursuing high removal efficiency of individual matrices and contaminants.
(2) Emerging bioremediation technologies are being developed to offer economically feasible and environmentally sustainable solutions for the decontamination of firing range sites, capable of effectively addressing large-scale contamination. However, there is a lack of comprehensive understanding regarding the precise mechanisms of remediation. Therefore, it is imperative to integrate spectroscopic, imaging, genomics, metabolomics, and proteomics techniques to elucidate the fate of pollutants during the remediation process. This will enable a thorough comprehension of the purification mechanisms associated with each remediation measure employed at contaminated sites.
(3) It is important to note that while various remediation measures can mitigate the risks posed by elevated levels of contaminants in soils and decrease the ecotoxicity of metals and organics, their effectiveness may vary depending on the receptors and contaminants present at specific study sites. Even though these measures generally led to a decrease in exchangeable metals or the removal of contaminants from the site, the bioavailability of co-contaminants in the treated soils remained relatively elevated. To achieve equilibrium in the immobilization of various contaminants across diverse soil properties, it is imperative to use suitable models for contaminant removal that are tailored to the specific site, soil characteristics, contaminant quantity and composition, as well as the binding mechanisms of contaminants in the soil. In addition to the reduction of metals in the exchangeable state in the soil, due attention should also be given to the possibility of re-activation of stabilized metal fractions. Additionally, a comprehensive approach that combines existing remediation technologies should be considered to enhance the efficacy of remediation, while also taking into account cost-effectiveness. For phytoremediation techniques, the rational post-treatment of metal-accumulating plants is also a current and urgent consideration. Subsequently, pilot-scale treatability studies are conducted to evaluate the ecological risk posed by toxic contaminants and establish safety thresholds for environmental elements, thereby customizing the implementation of remediation strategies to individual sites.
(4) The majority of previous research about the remediation of range contamination has predominantly concentrated on the examination of contaminated area samples within controlled laboratory settings. Consequently, there exists a scarcity of information regarding the practical implementation of these remediation strategies for the treatment of contaminated soil and groundwater in their natural environment. Hence, it is imperative to devise in situ remediation technologies that possess distinct advantages, including reduced expenses, expedited remediation duration, and minimal adverse impact on the surrounding ecosystem.
(5) The utilization of artificial intelligence can enhance the comprehension of range site contamination and pollution, facilitating improved management of contaminated sites.
6 Conclusion
The issue of site contamination resulting from range activities has garnered heightened attention in recent years. Currently, worldwide range pollution is still a persistent environmental problem that needs to be solved. A number of studies have shown that range pollution is widespread and that the organic and inorganic pollutants produced have serious adverse effects on the surrounding soil and groundwater environment, and even on human beings in the vicinity. Starting from the current status of range contamination, this review systematically describes several major types of contamination remediation technologies and draws the following conclusions:
(1) Traditional military shooting methods result in a large number of toxic heavy metals and organic pollutants remaining in the shooting range soil environment, and the degree of contamination increases with the aging of the soil.
(2) Through bibliometric statistics, it was found that current research on the remediation of shooting range contamination is centered on several aspects, such as soil flora characteristics, human health risks, and pollution uptake and transformation.
(3) This review article systematically discusses measures taken to address contamination at firing ranges, with a specific emphasis on the effectiveness of bioremediation, physicoremediation, and chemoremediation in addressing a wide range of inorganic and organic pollutants found in water and soil. A substantial body of academic literature suggests that these remediation techniques hold significant promise for on-site remediation of contaminants in both soil and groundwater at contaminated sites.
(4) The review further identified several crucial areas for research and highlighted gaps in existing knowledge, which can serve as a valuable guide for the future implementation of these promising remediation strategies.
Further investigation in this field is anticipated to enhance our comprehension of the destiny, transportation, and remediation of contaminants at range sites, as well as improve our ability to assess and mitigate the risks associated with range site contamination. Moreover, it will enable us to effectively respond to the resultant contamination, thereby safeguarding the ecological security of range sites and human health, which holds immense importance.
Author contributions
YiZ: Conceptualization, Data curation, Formal Analysis, Methodology, Writing–original draft. RC: Data curation, Methodology, Writing–original draft. BT: Investigation, Methodology, Writing–original draft. JM: Investigation, Methodology, Writing–original draft. XL: Investigation, Writing–original draft. JL: Writing–review and editing. YoZ: Data curation, Funding acquisition, Writing–review and editing. FW: Funding acquisition, Supervision, Visualization, Writing–review and editing.
Funding
The author(s) declare that financial support was received for the research, authorship, and/or publication of this article. This work was financially supported by the State Key Laboratory of NBC Protection for Civilian (SKLNBC 2021-18), the Major Project of College Natural Science Foundation of Jiangsu Province (21KJA610005), and the special fund from the Key Laboratory for Soft Chemistry and Functional Materials of Ministry of Education (Project No. 2021–01).
Acknowledgments
All the authors are grateful to the reviewers and editors.
Conflict of interest
The authors declare that the research was conducted in the absence of any commercial or financial relationships that could be construed as a potential conflict of interest.
Publisher’s note
All claims expressed in this article are solely those of the authors and do not necessarily represent those of their affiliated organizations, or those of the publisher, the editors and the reviewers. Any product that may be evaluated in this article, or claim that may be made by its manufacturer, is not guaranteed or endorsed by the publisher.
Supplementary material
The Supplementary Material for this article can be found online at: https://www.frontiersin.org/articles/10.3389/fenvs.2024.1352603/full#supplementary-material
References
Abdel-Sabour, M. F., and Al-Salama, Y. J. (2007). Zinc and cobalt phytoextraction by different plant species. Remediation 18, 109–119. doi:10.1002/rem.20155
Ahmad, M., Hashimoto, Y., Moon, D. H., Lee, S. S., and Ok, Y. S. (2012). Immobilization of lead in a Korean military shooting range soil using eggshell waste: an integrated mechanistic approach. J. Hazard. Mater. 209 (210), 392–401. doi:10.1016/j.jhazmat.2012.01.047
Ahmad, M., Ok, Y. S., Rajapaksha, A. U., Lim, J. E., Kim, B.-Y., Ahn, J.-H., et al. (2016). Lead and copper immobilization in a shooting range soil using soybean stover- and pine needle-derived biochars: chemical, microbial and spectroscopic assessments. J. Hazard. Mater. 301, 179–186. doi:10.1016/j.jhazmat.2015.08.029
Alasmary, Z., Hettiarachchi, G. M., Roozeboom, K. L., Davis, L. C., Erickson, L. E., Pidlisnyuk, V., et al. (2021). Phytostabilization of a contaminated military site using Miscanthus and soil amendments. J. Environ. Qual. 50, 1220–1232. doi:10.1002/jeq2.20268
Allen, A. (2001). Containment landfills: the myth of sustainability. Eng. Geol. 60, 3–19. doi:10.1016/S0013-7952(00)00084-3
Andrejic, G., Šinžar-Sekulić, J., Prica, M., Dželetović, Ž., and Rakić, T. (2019). Phytoremediation potential and physiological response of Miscanthus × giganteus cultivated on fertilized and non-fertilized flotation tailings. Environ. Sci. Pollut. Res. 26, 34658–34669. doi:10.1007/s11356-019-06543-7
Ansari, F., Momina, A., and Rafatullah, M. (2023). Review on bioremediation technologies of polycyclic aromatic hydrocarbons (PAHs) from soil: mechanisms and future perspective. Int. Biodeterior. Biodegrad. 179, 105582. doi:10.1016/j.ibiod.2023.105582
Azizi, S., Mahdavi Shahri, M., and Mohamad, R. (2017). Green synthesis of zinc oxide nanoparticles for enhanced adsorption of lead ions from aqueous solutions: equilibrium, kinetic and thermodynamic studies. Molecules 22, 831. doi:10.3390/molecules22060831
Bannon, D. I., Drexler, J. W., Fent, G. M., Casteel, S. W., Hunter, P. J., Brattin, W. J., et al. (2009). Evaluation of small arms range soils for metal contamination and lead bioavailability. Environ. Sci. Technol. 43, 9071–9076. doi:10.1021/es901834h
Barker, A. J., Douglas, T. A., Spaleta, K. J., and Trainor, T. P. (2023). Attenuation of Pb and Sb in shooting range soils by Fe amendments. Chemosphere 318, 137899. doi:10.1016/j.chemosphere.2023.137899
Befkadu, A. A., and Chen, Q. (2018). Surfactant-enhanced soil washing for removal of petroleum hydrocarbons from contaminated soils: a review. Pedosphere 28, 383–410. doi:10.1016/S1002-0160(18)60027-X
Buelt, J. L., and Farnsworth, R. K. (1991). In situ vitrification of soils containing various metals. Nucl. Technol. 96, 178–184. doi:10.13182/NT91-A34603
Cai, Z., Sun, Y., Deng, Y., Zheng, X., Sun, S., Sinkkonen, A., et al. (2022). Enhanced electrokinetic remediation of cadmium (Cd)-Contaminated soil with interval power breaking. Int. J. Environ. Res. 16, 31. doi:10.1007/s41742-022-00409-6
Cameselle, C., and Gouveia, S. (2018). Electrokinetic remediation for the removal of organic contaminants in soils. Curr. Opin. Electrochem. 11, 41–47. doi:10.1016/j.coelec.2018.07.005
Cameselle, C., Gouveia, S., Akretche, D. E., Belhadj, B., Cameselle, C., Gouveia, S., et al. (2013). Advances in electrokinetic remediation for the removal of organic contaminants in soils. Org. Pollut. - Monit. Risk Treat. (IntechOpen). doi:10.5772/54334
Cao, X., Ma, L. Q., Chen, M., Hardison, D. W., and Harris, W. G. (2003). Lead transformation and distribution in the soils of shooting ranges in Florida, USA. Sci. Total Environ. 307, 179–189. doi:10.1016/S0048-9697(02)00543-0
Cao, X., Wahbi, A., Ma, L., Li, B., and Yang, Y. (2009). Immobilization of Zn, Cu, and Pb in contaminated soils using phosphate rock and phosphoric acid. J. Hazard. Mater. 164, 555–564. doi:10.1016/j.jhazmat.2008.08.034
Česynaitė, J., Praspaliauskas, M., Pedišius, N., and Sujetovienė, G. (2021). Biological assessment of contaminated shooting range soil using earthworm biomarkers. Ecotoxicology 30, 2024–2035. doi:10.1007/s10646-021-02463-w
Česynaitė, J., Praspaliauskas, M., and Sujetovienė, G. (2023). Bioaccumulation of trace metal(loid)s and toxic response of Lactuca sativa grown in shooting range soil. Toxicol. Environ. Chem. 105, 28–41. doi:10.1080/02772248.2023.2199996
Chang, J.-H., Wang, Y.-L., Dong, C.-D., and Shen, S.-Y. (2022). The particle-size sieving technique to remediate Pb- and Cu-contaminated agricultural soil. Environ. Geotech. 9, 124–132. doi:10.1680/jenge.19.00162
Chen, S., Shu, X., Tang, H., Mao, X., Xu, C., and Lu, X. (2019). Microwave vitrification of uranium-contaminated soil for nuclear test site and chemical stability. Ceram. Int. 45, 13334–13339. doi:10.1016/j.ceramint.2019.04.026
Christou, A., Hadjisterkotis, E., Dalias, P., Demetriou, E., Christofidou, M., Kozakou, S., et al. (2022). Lead contamination of soils, sediments, and vegetation in a shooting range and adjacent terrestrial and aquatic ecosystems: a holistic approach for evaluating potential risks. Chemosphere 292, 133424. doi:10.1016/j.chemosphere.2021.133424
Clausen, J. L., Scott, C., and Osgerby, I. (2011). Fate of nitroglycerin and dinitrotoluene in soil at small arms training ranges. Soil Sediment Contam. An Int. J. 20, 649–671. doi:10.1080/15320383.2011.594108
Conesa, H. M., Wieser, M., Gasser, M., Hockmann, K., Evangelou, M. W. H., Studer, B., et al. (2010). Effects of three amendments on extractability and fractionation of Pb, Cu, Ni and Sb in two shooting range soils. J. Hazard. Mater. 181, 845–850. doi:10.1016/j.jhazmat.2010.05.090
Conesa, H. M., Wieser, M., Studer, B., and Schulin, R. (2011). Effects of vegetation and fertilizer on metal and Sb plant uptake in a calcareous shooting range soil. Ecol. Eng. 37, 654–658. doi:10.1016/j.ecoleng.2010.11.001
Cui, W., Li, X., Duan, W., Xie, M., and Dong, X. (2023). Heavy metal stabilization remediation in polluted soils with stabilizing materials: a review. Environ. Geochem Health 45, 4127–4163. doi:10.1007/s10653-023-01522-x
Dermatas, D., Cao, X., Tsaneva, V., Shen, G., and Grubb, D. G. (2006a). Fate and behavior of metal(loid) contaminants in an organic matter-rich shooting range soil: implications for remediation. Water Air Soil Pollut. Focus 6, 143–155. doi:10.1007/s11267-005-9003-4
Dermatas, D., Menounou, N., Dadachov, M., Dutko, P., Shen, G., Xu, X., et al. (2006b). Lead leachability in firing range soils. Environ. Eng. Sci. 23, 88–101. doi:10.1089/ees.2006.23.88
Dinake, P., and Kelebemang, R. (2019). Critical assessment of mechanistic pathways for chemical remediation techniques applied to Pb impacted soils at shooting ranges – a review. Environ. Pollut. Bioavailab. 31, 282–305. doi:10.1080/26395940.2019.1676170
Dinake, P., Kelebemang, R., and Sehube, N. (2019). A comprehensive approach to speciation of lead and its contamination of firing range soils: a review. Soil Sediment Contam. An Int. J. 28, 431–459. doi:10.1080/15320383.2019.1597831
Dinake, P., Maphane, O., Sebogisi, K., and Kamwi, O. (2018). Pollution status of shooting range soils from Cd, Cu, Mn, Ni and Zn found in ammunition. Cogent Environ. Sci. 4, 1528701. doi:10.1080/23311843.2018.1528701
Dinake, P., Mokgosi, S. M., Kelebemang, R., Kereeditse, T. T., and Motswetla, O. (2022). Pollution assessment of antimony in shooting range soils. South Afr. J. Chem. 76, 72–78. doi:10.17159/0379-4350/2022/v76a11
Douay, F., Roussel, H., Pruvot, C., Loriette, A., and Fourrier, H. (2008). Assessment of a remediation technique using the replacement of contaminated soils in kitchen gardens nearby a former lead smelter in Northern France. Sci. Total Environ. 401, 29–38. doi:10.1016/j.scitotenv.2008.03.025
Etim, E. U. (2017). Lead removal from contaminated shooting range soil using acetic acid potassium chloride washing solutions and electrochemical reduction. J. Health Pollut. 7, 22–31. doi:10.5696/2156-9614-7-13.22
Fayiga, A. O., and Saha, U. K. (2016a). Soil pollution at outdoor shooting ranges: health effects, bioavailability and best management practices. Environ. Pollut. 216, 135–145. doi:10.1016/j.envpol.2016.05.062
Fayiga, A. O., and Saha, U. K. (2016b). Soil pollution at outdoor shooting ranges: health effects, bioavailability and best management practices. Environ. Pollut. 216, 135–145. doi:10.1016/j.envpol.2016.05.062
Frakes, R. A., Boughner, E. A., Boggs, J. F., Tutton, J., and Bargar, T. A. (2007). Delineation of the nature and extent of contamination at the former NAS key west skeet club on great white heron national wildlife refuge. Fish Wildl. Serv. Available at: https://ecos.fws.gov/ServCat/DownloadFile/21637?Reference=23118.
Gómez-Sagasti, M. T., Anza, M., Hidalgo, J., Artetxe, U., Garbisu, C., and Becerril, J. M. (2021). Recent trends in sustainable remediation of Pb-contaminated shooting range soils: rethinking waste management within a circular economy. Processes 9, 572. doi:10.3390/pr9040572
Grispen, V. M. J., Nelissen, H. J. M., and Verkleij, J. A. C. (2006). Phytoextraction with Brassica napus L.: a tool for sustainable management of heavy metal contaminated soils. Environ. Pollut. 144, 77–83. doi:10.1016/j.envpol.2006.01.007
Guberman (2017). Lead statistics and information | U.S. Geological survey. Available at: https://www.usgs.gov/centers/national-minerals-information-center/lead-statistics-and-information (Accessed October 25, 2023).
Guo, G., Zhou, Q., and Ma, L. Q. (2006). Availability and assessment of fixing additives for the in situ remediation of heavy metal contaminated soils: a review. Environ. Monit. Assess. 116, 513–528. doi:10.1007/s10661-006-7668-4
Han, L.-J., Li, J.-S., Xue, Q., Guo, M.-Z., Wang, P., and Poon, C. S. (2022). Enzymatically induced phosphate precipitation (EIPP) for stabilization/solidification (S/S) treatment of heavy metal tailings. Constr. Build. Mater. 314, 125577. doi:10.1016/j.conbuildmat.2021.125577
Hardison, D. W., Ma, L. Q., Luongo, T., and Harris, W. G. (2004). Lead contamination in shooting range soils from abrasion of lead bullets and subsequent weathering. Sci. Total Environ. 328, 175–183. doi:10.1016/j.scitotenv.2003.12.013
Hashimoto, Y., Matsufuru, H., and Sato, T. (2008). Attenuation of lead leachability in shooting range soils using poultry waste amendments in combination with indigenous plant species. Chemosphere 73, 643–649. doi:10.1016/j.chemosphere.2008.07.033
He, Y., Joseph, B. H., and Sung, S. H. (2007). Biodegradation of 2,4- and 2,6-dinitrotoluene in a pilot-scale system for soil contaminated with explosive compounds. Environ. Sci. 28, 613–616. doi:10.3321/j.issn:0250-3301.2007.03.030
Hockmann, K., Tandy, S., Studer, B., Evangelou, M. W. H., and Schulin, R. (2018). Plant uptake and availability of antimony, lead, copper and zinc in oxic and reduced shooting range soil. Environ. Pollut. 238, 255–262. doi:10.1016/j.envpol.2018.03.014
Islam, M. N., and Park, J.-H. (2017). Immobilization and reduction of bioavailability of lead in shooting range soil through hydrothermal treatment. J. Environ. Manag. 191, 172–178. doi:10.1016/j.jenvman.2017.01.017
ITRC (2003). Characterization and remediation of soils at closed small arms firing ranges. Interstate Technology and Regulatory Council. Available at: https://itrcweb.org/home.
Jiang, S., Duan, L., Dai, G., and Shu, Y. (2021). Immobilization of heavy metal(loid)s in acid paddy soil by soil replacement-biochar amendment technology under normal wet condition. Environ. Sci. Pollut. Res. 28, 68886–68896. doi:10.1007/s11356-021-14757-x
Johnsen, I. V., and Aaneby, J. (2019). Soil intake in ruminants grazing on heavy-metal contaminated shooting ranges. Sci. Total Environ. 687, 41–49. doi:10.1016/j.scitotenv.2019.06.086
Jugnia, L.-B., Manno, D., Dodard, S., Greer, C. W., and Hendry, M. (2019). Manipulating redox conditions to enhance in situ bioremediation of RDX in groundwater at a contaminated site. Sci. Total Environ. 676, 368–377. doi:10.1016/j.scitotenv.2019.04.045
Jugnia, L. B., Manno, D., Drouin, K., and Hendry, M. (2018). In situ pilot test for bioremediation of energetic compound-contaminated soil at a former military demolition range site. Environ. Sci. Pollut. Res. 25, 19436–19445. doi:10.1007/s11356-018-2115-y
Kazery, J. A., Proctor, G., Larson, S. L., Ballard, J. H., Knotek-Smith, H. M., Zhang, Q., et al. (2021). Distribution and fractionation of uranium in weapon tested range soils. ACS Earth Space Chem. 5, 356–364. doi:10.1021/acsearthspacechem.0c00326
Kelebemang, R., Dinake, P., Sehube, N., Daniel, B., Totolo, O., and Laetsang, M. (2017). Speciation and mobility of lead in shooting range soils. Chem. Speciat. Bioavailab. 29, 143–152. doi:10.1080/09542299.2017.1349552
Khan, A. Z., Khan, S., Muhammad, S., Baig, S. A., Khan, A., Nasir, M. J., et al. (2021a). Lead contamination in shooting range soils and its phytoremediation in Pakistan: a greenhouse experiment. Arab. J. Geosci. 14, 4. doi:10.1007/s12517-020-06301-x
Khan, B. A., Ahmad, M., Iqbal, S., Ullah, F., Bolan, N., Solaiman, Z. M., et al. (2023). Adsorption and immobilization performance of pine-cone pristine and engineered biochars for antimony in aqueous solution and military shooting range soil: an integrated novel approach. Environ. Pollut. 317, 120723. doi:10.1016/j.envpol.2022.120723
Khan, S., Naushad, Mu., Lima, E. C., Zhang, S., Shaheen, S. M., and Rinklebe, J. (2021b). Global soil pollution by toxic elements: current status and future perspectives on the risk assessment and remediation strategies – a review. J. Hazard. Mater. 417, 126039. doi:10.1016/j.jhazmat.2021.126039
Kim, S., Baek, K., and Lee, I. (2010). Phytoremediation and microbial community structure of soil from a metal-contaminated military shooting range: comparisons of field and pot experiments. J. Environ. Sci. Health, Part A 45, 389–394. doi:10.1080/10934520903467832
Kim, S., Choi, J., and Jeong, S.-W. (2023). Changes in the health of metal-contaminated soil before and after stabilization and solidification. Environ. Pollut. 331, 121929. doi:10.1016/j.envpol.2023.121929
Knechtenhofer, L. A., Xifra, I. O., Scheinost, A. C., Flühler, H., and Kretzschmar, R. (2003). Fate of heavy metals in a strongly acidic shooting-range soil: small-scale metal distribution and its relation to preferential water flow. J. Plant Nutr. Soil Sci. 166, 84–92. doi:10.1002/jpln.200390017
Koelmel, J., and Amarasiriwardena, D. (2012). Imaging of metal bioaccumulation in Hay-scented fern (Dennstaedtia punctilobula) rhizomes growing on contaminated soils by laser ablation ICP-MS. Environ. Pollut. 168, 62–70. doi:10.1016/j.envpol.2012.03.035
Lafond, S., Blais, J.-F., Martel, R., and Mercier, G. (2012). Chemical leaching of antimony and other metals from small arms shooting range soil. Water Air Soil Pollut. 224, 1371. doi:10.1007/s11270-012-1371-6
Lafond, S., Blais, J.-F., Mercier, G., and Martel, R. (2013). Counter-current acid leaching process for the removal of Cu, Pb, Sb and Zn from shooting range soil. Environ. Technol. 34, 2377–2387. doi:10.1080/09593330.2013.770560
Lafond, S., Blais, J.-F., Mercier, G., and Martel, R. (2014). A counter-current acid leaching process for the remediation of contaminated soils from a small-arms shooting range. Soil Sediment Contam. An Int. J. 23, 194–210. doi:10.1080/15320383.2014.808171
Laporte-Saumure, M., Martel, R., and Mercier, G. (2011). Characterization and metal availability of copper, lead, antimony and zinc contamination at four Canadian small arms firing ranges. Environ. Technol. 32, 767–781. doi:10.1080/09593330.2010.512298
Lee, J.-H., and Park, K.-S. (2008). Heavy metal distribution in soils from the maehyang-ri Inland shooting range area. J. Korean Soc. Water Environ. 24, 407–414.
Lee, K.-Y., and Kim, K.-W. (2010). Heavy metal removal from shooting range soil by hybrid electrokinetics with bacteria and enhancing agents. Environ. Sci. Technol. 44, 9482–9487. doi:10.1021/es102615a
Levonmäki, M., and Hartikainen, H. (2007). Efficiency of liming in controlling the mobility of lead in shooting range soils as assessed by different experimental approaches. Sci. Total Environ. 388, 1–7. doi:10.1016/j.scitotenv.2007.07.055
Lewińska, K., Karczewska, A., Siepak, M., Szopka, K., Gałka, B., and Iqbal, M. (2019). Effects of waterlogging on the solubility of antimony and arsenic in variously treated shooting range soils. Appl. Geochem. 105, 7–16. doi:10.1016/j.apgeochem.2019.04.005
Li, W., Ni, P., and Yi, Y. (2019). Comparison of reactive magnesia, quick lime, and ordinary Portland cement for stabilization/solidification of heavy metal-contaminated soils. Sci. Total Environ. 671, 741–753. doi:10.1016/j.scitotenv.2019.03.270
Liu, L., Li, W., Song, W., and Guo, M. (2018). Remediation techniques for heavy metal-contaminated soils: principles and applicability. Sci. Total Environ. 633, 206–219. doi:10.1016/j.scitotenv.2018.03.161
Liu, S., Shi, Y., Sun, M., Huang, D., Shu, W., and Ye, M. (2023). The community assembly for understanding the viral-assisted response of bacterial community to Cr-contamination in soils. J. Hazard. Mater. 441, 129975. doi:10.1016/j.jhazmat.2022.129975
Liu, Z., Chen, L., Zhang, Z., Li, Y., Dong, Y., and Sun, Y. (2013). Synthesis of multi-walled carbon nanotube–hydroxyapatite composites and its application in the sorption of Co(II) from aqueous solutions. J. Mol. Liq. 179, 46–53. doi:10.1016/j.molliq.2012.12.011
Luo, J., Li, Y., Cao, H., Zhu, Y., Liu, X., Li, H., et al. (2023). Variations of microbiota in three types of typical military contaminated sites: diversities, structures, influence factors, and co-occurrence patterns. J. Hazard. Mater. 443, 130290. doi:10.1016/j.jhazmat.2022.130290
Mankė, J., Praspaliauskas, M., Pedišius, N., and Sujetovienė, G. (2024). Evaluation of phytoremediation efficiency of shooting range soil using the bioaccumulation potential and sensitivity of different plant species. Ecol. Eng. 198, 107134. doi:10.1016/j.ecoleng.2023.107134
Mariussen, E., Johnsen, I. V., and Strømseng, A. E. (2017). Distribution and mobility of lead (Pb), copper (Cu), zinc (Zn), and antimony (Sb) from ammunition residues on shooting ranges for small arms located on mires. Environ. Sci. Pollut. Res. 24, 10182–10196. doi:10.1007/s11356-017-8647-8
Mathee, A., de Jager, P., Naidoo, S., and Naicker, N. (2017). Exposure to lead in South African shooting ranges. Environ. Res. 153, 93–98. doi:10.1016/j.envres.2016.11.021
Mendes, G. P., Soares, L. C., Viegas, R. M. A., Chiavone-Filho, O., and do Nascimento, C. A. O. (2023). Lead (Pb) in shooting range soil: a systematic literature review of contaminant behavior, risk assessment, and remediation options. Water Air Soil Pollut. 235, 1. doi:10.1007/s11270-023-06783-x
Mi, R., Zhang, Z., Ji, W., Liu, S., Kai, M. F., lin, K., et al. (2023). Solidification/stabilisation behaviours of Zn2+ in magnesium potassium phosphate cement: experiments and density functional theory study. Environ. Res. 231, 116247. doi:10.1016/j.envres.2023.116247
MOE (2005). The development of hybrid electrokinetic remediation technique using solar energy on shooting range soils contaminated by heavy metals, Gwachun, Kyunggi Republic of Korea. Korea: MOE: Gwachun, 40–63.
Moon, D. H., Cheong, K. H., Khim, J., Wazne, M., Hyun, S., Park, J.-H., et al. (2013). Stabilization of Pb2+ and Cu2+ contaminated firing range soil using calcined oyster shells and waste cow bones. Chemosphere 91, 1349–1354. doi:10.1016/j.chemosphere.2013.02.007
Moon, I., Kim, H., Jeong, S., Choi, H., Park, J., and Lee, I. (2021). Chemical properties of heavy metal-contaminated soils from a Korean military shooting range: evaluation of Pb sources using Pb Isotope ratios. Appl. Sci. 11, 7099. doi:10.3390/app11157099
Mulligan, C. N., Yong, R. N., and Gibbs, B. F. (2001). An evaluation of technologies for the heavy metal remediation of dredged sediments. J. Hazard. Mater. 85, 145–163. doi:10.1016/S0304-3894(01)00226-6
Neugschwandtner, R. W., Tlustoš, P., Komárek, M., and Száková, J. (2008). Phytoextraction of Pb and Cd from a contaminated agricultural soil using different EDTA application regimes: laboratory versus field scale measures of efficiency. Geoderma 144, 446–454. doi:10.1016/j.geoderma.2007.11.021
Ni, S., Rahman, S., Kasai, S., Yoshioka, S., Wong, K. H., Mashio, A. S., et al. (2023a). Remediation of lead-contaminated shooting range soil: biodegradable chelator-assisted washing and subsequent post-treatment using FeCl3 and CaO. Environ. Technol. Innovation 31, 103172. doi:10.1016/j.eti.2023.103172
Ni, S., Rahman, S., Kasai, S., Yoshioka, S., Wong, K. H., Mashio, A. S., et al. (2023b). Remediation of lead-contaminated shooting range soil: biodegradable chelator-assisted washing and subsequent post-treatment using FeCl3 and CaO. Environ. Technol. Innovation 31, 103172. doi:10.1016/j.eti.2023.103172
Nsanganwimana, F., Pourrut, B., Waterlot, C., Louvel, B., Bidar, G., Labidi, S., et al. (2015). Metal accumulation and shoot yield of Miscanthus×giganteus growing in contaminated agricultural soils: insights into agronomic practices. Agric. Ecosyst. Environ. 213, 61–71. doi:10.1016/j.agee.2015.07.023
Ogawa, S., Katoh, M., and Sato, T. (2015). Simultaneous lead and antimony immobilization in shooting range soil by a combined application of hydroxyapatite and ferrihydrite. Environ. Technol. 36, 2647–2656. doi:10.1080/09593330.2015.1042071
Okkenhaug, G., Grasshorn Gebhardt, K.-A., Amstaetter, K., Lassen Bue, H., Herzel, H., Mariussen, E., et al. (2016). Antimony (Sb) and lead (Pb) in contaminated shooting range soils: Sb and Pb mobility and immobilization by iron based sorbents, a field study. J. Hazard. Mater. 307, 336–343. doi:10.1016/j.jhazmat.2016.01.005
Okkenhaug, G., Smebye, A. B., Pabst, T., Amundsen, C. E., Sævarsson, H., and Breedveld, G. D. (2018). Shooting range contamination: mobility and transport of lead (Pb), copper (Cu) and antimony (Sb) in contaminated peatland. J. Soils Sediments 18, 3310–3323. doi:10.1007/s11368-017-1739-8
Ottosen, L. M., Pedersen, A. J., Ribeiro, A. B., and Hansen, H. K. (2005). Case study on the strategy and application of enhancement solutions to improve remediation of soils contaminated with Cu, Pb and Zn by means of electrodialysis. Eng. Geol. 77, 317–329. doi:10.1016/j.enggeo.2004.07.021
Ouhadi, V. R., Yong, R. N., Shariatmadari, N., Saeidijam, S., Goodarzi, A. R., and Safari-Zanjani, M. (2010). Impact of carbonate on the efficiency of heavy metal removal from kaolinite soil by the electrokinetic soil remediation method. J. Hazard. Mater. 173, 87–94. doi:10.1016/j.jhazmat.2009.08.052
Pain, D. J., Mateo, R., and Green, R. E. (2019). Effects of lead from ammunition on birds and other wildlife: a review and update. Ambio 48, 935–953. doi:10.1007/s13280-019-01159-0
Pedersen, K. B., Jensen, P. E., Ottosen, L. M., and Barlindhaug, J. (2018). Influence of electrode placement for mobilising and removing metals during electrodialytic remediation of metals from shooting range soil. Chemosphere 210, 683–691. doi:10.1016/j.chemosphere.2018.07.063
Pedersen, K. B., Jensen, P. E., Ottosen, L. M., and Barlindhaug, J. (2019). Applying multivariate analysis for optimising the electrodialytic removal of Cu and Pb from shooting range soils. J. Hazard. Mater. 368, 869–876. doi:10.1016/j.jhazmat.2018.10.014
Purakayastha, T. J., Viswanath, T., Bhadraray, S., Chhonkar, P. K., Adhikari, P. P., and Suribabu, K. (2008). Phytoextraction of zinc, copper, nickel and lead from a contaminated soil by different species of Brassica. Int. J. Phytoremediation 10, 61–72. doi:10.1080/15226510701827077
Raimondo, E. E., Saez, J. M., Aparicio, J. D., Fuentes, M. S., and Benimeli, C. S. (2020). Coupling of bioaugmentation and biostimulation to improve lindane removal from different soil types. Chemosphere 238, 124512. doi:10.1016/j.chemosphere.2019.124512
Rajapaksha, A. U., Ahmad, M., Vithanage, M., Kim, K.-R., Chang, J. Y., Lee, S. S., et al. (2015). The role of biochar, natural iron oxides, and nanomaterials as soil amendments for immobilizing metals in shooting range soil. Environ. Geochem Health 37, 931–942. doi:10.1007/s10653-015-9694-z
Reigosa-Alonso, A., Lorenzo Dacunha, R., Arenas-Lago, D., Vega, F. A., and Rodríguez-Seijo, A. (2021). Soils from abandoned shooting range facilities as contamination source of potentially toxic elements: distribution among soil geochemical fractions. Environ. Geochem Health 43, 4283–4297. doi:10.1007/s10653-021-00900-7
Robidoux, P. Y., Gong, P., Sarrazin, M., Bardai, G., Paquet, L., Hawari, J., et al. (2004). Toxicity assessment of contaminated soils from an antitank firing range. Ecotoxicol. Environ. Saf. 58, 300–313. doi:10.1016/j.ecoenv.2003.11.004
Rodríguez-Seijo, A., Alfaya, M. C., Andrade, M. L., and Vega, F. A. (2016a). Copper, chromium, nickel, lead and zinc levels and pollution degree in firing range soils. Land Degrad. Dev. 27, 1721–1730. doi:10.1002/ldr.2497
Rodríguez-Seijo, A., Cachada, A., Gavina, A., Duarte, A. C., Vega, F. A., Andrade, M. L., et al. (2017). Lead and PAHs contamination of an old shooting range: a case study with a holistic approach. Sci. Total Environ. 575, 367–377. doi:10.1016/j.scitotenv.2016.10.018
Rodríguez-Seijo, A., Lago-Vila, M., Andrade, M. L., and Vega, F. A. (2016b). Pb pollution in soils from a trap shooting range and the phytoremediation ability of Agrostis capillaris L. Environ. Sci. Pollut. Res. 23, 1312–1323. doi:10.1007/s11356-015-5340-7
Saini, A., Bekele, D. N., Chadalavada, S., Fang, C., and Naidu, R. (2021). Electrokinetic remediation of petroleum hydrocarbon contaminated soil (I). Environ. Technol. Innovation 23, 101585. doi:10.1016/j.eti.2021.101585
Sanderson, P., Naidu, R., Bolan, N., Bowman, M., and Mclure, S. (2012). Effect of soil type on distribution and bioaccessibility of metal contaminants in shooting range soils. Sci. Total Environ. 438, 452–462. doi:10.1016/j.scitotenv.2012.08.014
Sanderson, P., Naidu, R., Bolan, N., Lim, J. E., and Ok, Y. S. (2015). Chemical stabilisation of lead in shooting range soils with phosphate and magnesium oxide: synchrotron investigation. J. Hazard. Mater. 299, 395–403. doi:10.1016/j.jhazmat.2015.06.056
Sanderson, P., Qi, F., Seshadri, B., Wijayawardena, A., and Naidu, R. (2018). Contamination, fate and management of metals in shooting range soils—a review. Curr. Pollut. Rep. 4, 175–187. doi:10.1007/s40726-018-0089-5
Sas-Nowosielska, A., Galimska-Stypa, R., Kucharski, R., Zielonka, U., Małkowski, E., and Gray, L. (2008). Remediation aspect of microbial changes of plant rhizosphere in mercury contaminated soil. Environ. Monit. Assess. 137, 101–109. doi:10.1007/s10661-007-9732-0
Scanferla, P., Ferrari, G., Pellay, R., Volpi Ghirardini, A., Zanetto, G., and Libralato, G. (2009). An innovative stabilization/solidification treatment for contaminated soil remediation: demonstration project results. J. Soils Sediments. 9, 229–236. doi:10.1007/s11368-009-0067-z
Seagren, E. A. (2024). “Bioremediation,” in Encyclopedia of Toxicology. Editor P. Wexler Fourth Edition (Oxford: Academic Press), 145–159. doi:10.1016/B978-0-12-824315-2.00413-9
Shen, Z., Som, A. M., Wang, F., Jin, F., McMillan, O., and Al-Tabbaa, A. (2016). Long-term impact of biochar on the immobilisation of nickel (II) and zinc (II) and the revegetation of a contaminated site. Sci. Total Environ. 542, 771–776. doi:10.1016/j.scitotenv.2015.10.057
Shu, X., Li, Y., Huang, W., Chen, S., Xu, C., Zhang, S., et al. (2020). Rapid vitrification of uranium-contaminated soil: effect and mechanism. Environ. Pollut. 263, 114539. doi:10.1016/j.envpol.2020.114539
Silvani, L., Cornelissen, G., Botnen Smebye, A., Zhang, Y., Okkenhaug, G., Zimmerman, A. R., et al. (2019). Can biochar and designer biochar be used to remediate per- and polyfluorinated alkyl substances (PFAS) and lead and antimony contaminated soils? Sci. Total Environ. 694, 133693. doi:10.1016/j.scitotenv.2019.133693
Singh, S., Parihar, P., Singh, R., Singh, V. P., and Prasad, S. M. (2016). Heavy metal tolerance in plants: role of transcriptomics, proteomics, metabolomics, and ionomics. Front. Plant Sci. 6, 1143. doi:10.3389/fpls.2015.01143
Skibsted, G., Ottosen, L. M., Elektorowicz, M., and Jensen, P. E. (2018). Effect of long-term electrodialytic soil remediation on Pb removal and soil weathering. J. Hazard. Mater. 358, 459–466. doi:10.1016/j.jhazmat.2018.05.033
Sorvari, J. (2007). Environmental risks at Finnish shooting ranges—a case study. Hum. Ecol. Risk Assess. An Int. J. 13, 1111–1146. doi:10.1080/10807030701506124
Sorvari, J., Antikainen, R., and Pyy, O. (2006). Environmental contamination at Finnish shooting ranges—the scope of the problem and management options. Sci. Total Environ. 366, 21–31. doi:10.1016/j.scitotenv.2005.12.019
Stauffer, M., Pignolet, A., and Corcho Alvarado, J. A. (2017). Persistent mercury contamination in shooting range soils: the legacy from former primers. Bull. Environ. Contam. Toxicol. 98, 14–21. doi:10.1007/s00128-016-1976-3
Sujetoviene, G., and Cesynaite, J. (2020). Changes in physicochemical properties and contamination with lead in outdoor shooting range soils. Environ. Eng. Manag. J. 19, 1025–1031. doi:10.30638/eemj.2020.097
Sun, Z., Chen, W.-B., Zhao, R.-D., Shen, P., Yin, J.-H., and Chen, Y. (2023). Effect of seawater on solidification/stabilisation treatment of marine soft soil slurry by lime-activated ISSA and GGBS. Eng. Geol. 323, 107216. doi:10.1016/j.enggeo.2023.107216
Suresh, B., and Ravishankar, G. A. (2004). Phytoremediation—a novel and promising approach for environmental clean-up. Crit. Rev. Biotechnol. 24, 97–124. doi:10.1080/07388550490493627
Tandy, S., Meier, N., and Schulin, R. (2017). Use of soil amendments to immobilize antimony and lead in moderately contaminated shooting range soils. J. Hazard. Mater. 324, 617–625. doi:10.1016/j.jhazmat.2016.11.034
Tangahu, B. V., Sheikh Abdullah, S. R., Basri, H., Idris, M., Anuar, N., and Mukhlisin, M. (2011). A review on heavy metals (as, Pb, and Hg) uptake by plants through phytoremediation. Int. J. Chem. Eng. 2011, 9391611–e939231. doi:10.1155/2011/939161
Tariq, S. R., and Ashraf, A. (2016). Comparative evaluation of phytoremediation of metal contaminated soil of firing range by four different plant species. Arabian J. Chem. 9, 806–814. doi:10.1016/j.arabjc.2013.09.024
Thangavadivel, K., Ranganathan, S., Sanderson, P., Chadalavada, S., Naidu, R., and Bowman, M. (2018). Case study of testing heavy-particle concentrator-aided remediation of lead-contaminated rifle shooting range soil. Remediat. J. 28, 67–74. doi:10.1002/rem.21561
Urrutia-Goyes, R., Mahlknecht, J., Argyraki, A., and Ornelas-Soto, N. (2017). Trace element soil contamination at a former shooting range in Athens, Greece. Geoderma Reg. 10, 191–199. doi:10.1016/j.geodrs.2017.08.002
Uwayezu, J. N., Ren, Z., Sonnenschein, S., Leiviskä, T., Lejon, T., van Hees, P., et al. (2024). Combination of separation and degradation methods after PFAS soil washing. Sci. Total Environ. 907, 168137. doi:10.1016/j.scitotenv.2023.168137
Vithanage, M., Herath, I., Almaroai, Y. A., Rajapaksha, A. U., Huang, L., Sung, J.-K., et al. (2017). Effects of carbon nanotube and biochar on bioavailability of Pb, Cu and Sb in multi-metal contaminated soil. Environ. Geochem Health 39, 1409–1420. doi:10.1007/s10653-017-9941-6
Wang, L., Li, H., Li, A., and Deng, J. (2022a). Study on characteristics and ecological risks of heavy metal pollution in soil of projectile range in military training ground - a case of a training ground in Tibet. Environ. Chem., 2646–2654. doi:10.7524/j.issn.0254-6108.2022012705
Wang, P., Shen, F., Li, R., Guo, D., Liang, W., Liu, T., et al. (2022b). Remediation of Cd and Zn contaminated soil by zero valent iron (Fe0): a field trial. Environ. Technol. Innovation 28, 102603. doi:10.1016/j.eti.2022.102603
Wilde, E. W., Brigmon, R. L., Dunn, D. L., Heitkamp, M. A., and Dagnan, D. C. (2005). Phytoextraction of lead from firing range soil by Vetiver grass. Chemosphere 61, 1451–1457. doi:10.1016/j.chemosphere.2005.04.059
Wolf, D. C., Cryder, Z., Khoury, R., Carlan, C., and Gan, J. (2020). Bioremediation of PAH-contaminated shooting range soil using integrated approaches. Sci. Total Environ. 726, 138440. doi:10.1016/j.scitotenv.2020.138440
Wu, Q., Cui, Y., Li, Q., and Sun, J. (2015). Effective removal of heavy metals from industrial sludge with the aid of a biodegradable chelating ligand GLDA. J. Hazard. Mater. 283, 748–754. doi:10.1016/j.jhazmat.2014.10.027
Xu, D.-M., Fu, R.-B., Wang, J.-X., Shi, Y.-X., and Guo, X.-P. (2021). Chemical stabilization remediation for heavy metals in contaminated soils on the latest decade: available stabilizing materials and associated evaluation methods-A critical review. J. Clean. Prod. 321, 128730. doi:10.1016/j.jclepro.2021.128730
Xu, L., Xing, X., Liang, J., Peng, J., and Zhou, J. (2019). In situ phytoremediation of copper and cadmium in a co-contaminated soil and its biological and physical effects. RSC Adv. 9, 993–1003. doi:10.1039/C8RA07645F
Yan, Y., Qi, F., Seshadri, B., Xu, Y., Hou, J., Ok, Y. S., et al. (2016). Utilization of phosphorus loaded alkaline residue to immobilize lead in a shooting range soil. Chemosphere 162, 315–323. doi:10.1016/j.chemosphere.2016.07.068
Yang, S., Li, Y., Si, S., Liu, G., Yun, H., Tu, C., et al. (2022). Feasibility of a combined solubilization and eluent drainage system to remove Cd and Cu from agricultural soil. Sci. Total Environ. 807, 150733. doi:10.1016/j.scitotenv.2021.150733
Yang, X., Feng, Y., He, Z., and Stoffella, P. J. (2005). Molecular mechanisms of heavy metal hyperaccumulation and phytoremediation. J. Trace Elem. Med. Biol. 18, 339–353. doi:10.1016/j.jtemb.2005.02.007
Yasunari, T. J., Stohl, A., Hayano, R. S., Burkhart, J. F., Eckhardt, S., and Yasunari, T. (2011). Cesium-137 deposition and contamination of Japanese soils due to the Fukushima nuclear accident. Proc. Natl. Acad. Sci. 108, 19530–19534. doi:10.1073/pnas.1112058108
Yin, X., Saha, U. K., and Ma, L. Q. (2010). Effectiveness of best management practices in reducing Pb-bullet weathering in a shooting range in Florida. J. Hazard. Mater. 179, 895–900. doi:10.1016/j.jhazmat.2010.03.089
Zhang, J., Wang, L., Wang, M., Xiao, Y., and Han, Y. (2020). Mechanochemical remediation of petroleum hydrocarbons contaminated soil and its effects on soil properties. Chem. Industry Eng. Prog. 39, 4726–4733. doi:10.16085/j.issn.1000-6613.2020-0246
Zhao, X., Sang, L., Song, H., Liang, W., Gong, K., Peng, C., et al. (2023). Stabilization of Ni by rhamnolipid modified nano zero-valent iron in soil: effect of simulated acid rain and microbial response. Chemosphere 341, 140008. doi:10.1016/j.chemosphere.2023.140008
Keywords: shooting range, heavy metal, polycyclic aromatic hydrocarbon (PAH), pollution, remediation
Citation: Zhu Y, Che R, Tu B, Miao J, Lu X, Li J, Zhu Y and Wang F (2024) Contamination and remediation of contaminated firing ranges—an overview. Front. Environ. Sci. 12:1352603. doi: 10.3389/fenvs.2024.1352603
Received: 08 December 2023; Accepted: 19 February 2024;
Published: 12 March 2024.
Edited by:
Qi Liao, Central South University, ChinaReviewed by:
John Yang, Lincoln University, United StatesQuanqing Zhang, University of California, Riverside, United States
Copyright © 2024 Zhu, Che, Tu, Miao, Lu, Li, Zhu and Wang. This is an open-access article distributed under the terms of the Creative Commons Attribution License (CC BY). The use, distribution or reproduction in other forums is permitted, provided the original author(s) and the copyright owner(s) are credited and that the original publication in this journal is cited, in accordance with accepted academic practice. No use, distribution or reproduction is permitted which does not comply with these terms.
*Correspondence: Fenghe Wang, d2FuZ2ZlbmdoZUBuanVzdC5lZHUuY24=
†These authors have contributed equally to this work and share first authorship