- 1Sichuan Communication Surveying and Design Institute Co., Ltd., Chengdu, China
- 2Faculty of Geosciences and Environmental Engineering, Southwest Jiaotong University, Chengdu, China
The surface water and groundwater in the mountainous area are vulnerable to contamination from the mining and transportation construction in Sichuan Province, China. Pollutants produced by anthropogenic activities transport within the groundwater from mountains to rivers on the plain, transferring contamination to the surface water. This study investigates the process of groundwater flow and contaminant transport from mountains to the lowlands based on synthetic numerical models. Two key factors are considered: precipitation and the slope of the mountain. Based on the real situation in Sichuan Province, four rainfall recharge rates are defined as 600, 800, 1,000, and 1,200 mm/yr, and five slope angles are considered: 20°, 25°, 30°, 35°, and 40°. The simulation results reveal that the groundwater level and solute transport are strongly influenced by the precipitation amounts and slope angles. The mountains with lower slopes maintain a relatively higher groundwater level under steady-state rainfall conditions; for example, groundwater levels decrease from 340 m to 300 m as slope angles increase at a 1,200 mm/yr precipitation level. Contaminant transport from the source in the mountain to the surface river is faster with increasing precipitations and decreasing slope angles. The model with 20° slope angle and 1,200 mm/yr precipitation exhibits the fastest solute migration, with the contaminant arrival time of 65 years. Furthermore, the models with 35° and 40° slope angles at a 600 mm/yr precipitation level show the slow transport speed with the contaminant arrival time of more than 75 years. In addition, higher precipitation may lead to more contaminant transport to the river. The analysis and findings of this study offer valuable insights into groundwater protection at the boundaries of mountains and plains.
1 Introduction
The water resource is the valuable natural resource for human survival in the world. Surface water and groundwater are the primary constitutions of water resources on earth. Due to intensive anthropogenic activities including industrial, construction, agricultural, and sewage processes, the quality of surface water and groundwater is deteriorated (Paladino et al., 2018; Su et al., 2018). Accordingly, the quality of surface water and groundwater has been the focus of attention for water resource management (Li, 2020; Nasim et al., 2020).
So far, the hydrochemical monitoring of surface water and groundwater has been carried out for improving groundwater management in the world (Yin et al., 2021). Plenty of hydrochemical data provide a robust support for water quality evaluation in the spatial and temporal scale. Various water quality indices (e.g., the WQI and EWQI) have been used to evaluate the quality of surface water and groundwater (Amiri et al., 2014; Lapworth et al., 2017; Zhai et al., 2017). Hence, the spatial and temporal characteristics of the quality of surface water and groundwater have been clarified properly. However, the interaction between surface water and groundwater affecting water quality has remained unclear, failing to explain the dynamic evolution in the spatial and temporal scale.
The interaction between surface water and groundwater can be analyzed using the approaches of hydrochemistry, isotopy, and numerical simulation (Raiber et al., 2019; Marti et al., 2023; Wang et al., 2023). Hydrochemistry and D-O stable isotopes were used to reveal the hydrological relationship between surface water and groundwater in the Notwane River Catchment, Southeast Botswana (Modie et al., 2022). Multiple isotopic tracers (δD-H2O, δ18O–H2O, δ15N–NO3−, and δ18O–NO3−) indicated that shallow groundwater is contaminated with organic fertilizers and subsequently transferred to surface water (Le et al., 2023). The SWAT + gwflow model was constructed to investigate the long-term groundwater–surface water interactions in the Scheldt Basin (Liberoff and Poca, 2023).
Substantial amounts of population are living in basin areas in the world due to the advantages of warm climate and gentle terrain. The Sichuan Basin in southwestern China is the typically large-scale basin within more than 100 million people. The quality of surface water and groundwater has been investigated for decades. However, previous studies mostly focused on the hydrochemical and isotopic information of surface water and groundwater in the basin area (Zhang et al., 2020; Zhang et al., 2021a; Zhang et al., 2021b). Seldom studies investigate the dynamic interaction and transport processes between surface water and groundwater at the boundaries of plains and mountains. It is known that the contamination would be transferred between surface water and groundwater (Xu et al., 2022). Among those pollutions, the contamination produced by the mining projects and tunnel constructions in the mountainous area is a serious environmental problem (Tomiyama and Igarashi, 2022). The water system is interrupted by excavation, and the unpredicted groundwater flow may pollute the fresh groundwater in mining sites and surface water system in the plain (Wu et al., 2018), especially the transport of heavy metal in the wastewater (Santana et al., 2020). The recent investigation pointed out that mountainous water system in Sichuan Province is heavily contaminated by the regional mining and tunneling industry (Sun et al., 2023). Hence, a hydrological model of large-scale catchment from the basin margin to basin center is built in this study. Afterward, the dynamic interaction between surface water and groundwater is simulated under different conditions of climate precipitation and terrain slope. Finally, the contamination in surface water and groundwater can be evaluated under different conditions. The achievements of this study would significantly contribute to evaluating the quality of surface water and groundwater in the catchment of the Sichuan Basin.
2 Methods
To investigate the issue of groundwater contamination from mountains to lowland rivers, a 2D numerical model is constructed in this study. The groundwater flow is steady-state since our model is synthetic to represent the general situation. Flow is simulated using MODFLOW (Harbaugh, 2005) with the Darcy equation (Eq. 1), and the solute transport is simulated in MT3DMS (Zheng and Wang, 1999) to depict advection and dispersion (Eq. 2).
where K is the value of hydraulic conductivity [LT−1]; H is the hydraulic head [L]; and w is the volumetric flux per unit volume representing sources and/or sinks of water [T−1].
where θ is the porosity of porous media [dimensionless]; vi is the seepage velocity [LT−1]; C is the solute concentration [dimensionless]; Cs is the solute concentration of water entering from sources or flowing out from sinks [dimensionless]; Dij is the hydrodynamic dispersion coefficient tensor [L2T−1]; and qs is the volumetric flow rate per unit volume of aquifer representing the fluid source (positive) and sink (negative) [T−1].
2.1 Model setup and boundary conditions
The framework of this synthetic model is based on the general conditions of mountainous area around the Chengdu Plain in Sichuan Province, China. The total length of the model is 1,700 m, with the left side representing the mountainous area at a height of 500 m and the right side representing the plain area with dimensions of 200 m in length and 150 m in height (Figure 1A). The entire domain is discretized into numerous cells, with each cell measuring 10 m × 10 m in size.
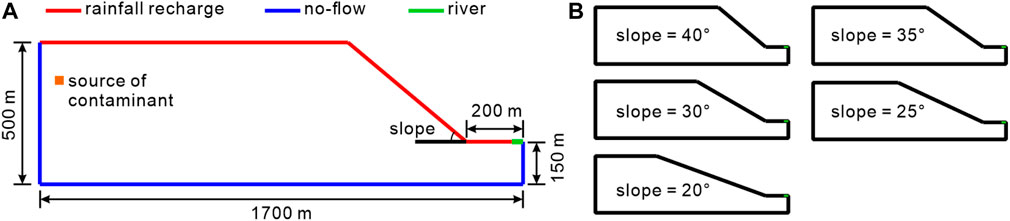
FIGURE 1. Model setup: (A) model domain and boundary conditions; (B) models with different slope angles.
The model was defined as an unconfined aquifer, with the left and right boundaries designated as no-flow boundaries, representing the water divide between the mountain and the river, respectively. The bottom also serves as a no-flow boundary, indicating the presence of impermeable rock beneath the model. The top is an active boundary, receiving the rainfall recharge at rates of 600, 800, 1,000, and 1,200 mm/year with an infiltration rate of 0.4. These settings are based on the precipitation data around the Chengdu Plain (Liu and Xu, 2016).
Furthermore, based on the slope inclination around Chengdu, we consider five slope angles at the interaction of mountainous area and lowlands, ranging from 20° to 40° (Figure 1B). Different slope angles may change the water infiltration rate since steep slopes bring more water flow on the surface. Although this is not considered in this synthetic study, we hope to investigate the effect of slopes on the groundwater flow. Additionally, we assume the lithology of the model as porous media, setting the hydraulic conductivity and porosity at 0.1 m/d and 0.2, respectively. Dispersity in porous media is set at 10 m, with a horizontal transverse ratio of 0.1 and a vertical transverse ratio of 0.01. A contaminant source is defined near the left boundary at the concentration of 1.0 (dimensionless), representing contamination produced by anthropogenic activities in the mountain area.
2.2 Solute transport assessment
To assess river contamination, we use two evaluation metrics to delineate solute transport from the mountainous area to the river: contaminant arrival time and the total mass of contamination over a 20-year period. Contaminant arrival time denotes the moment when the contaminant (concentration ≥0.01) first reaches the river from the beginning of the simulation, providing a measure of the solute transport speed from the mountainous region to the river. The total mass of contamination over a 20-year period represents the cumulative mass of solute flowing into the river in the 2 decades following the contaminant arrival time. For each scenario involving varying precipitation and slope angles, both metrics are derived from simulation results to quantify the vulnerability of the river to mountainous contamination.
3 Results and discussion
This study aims to investigate the effect of slope and precipitation on the transport of contaminants from the mountainous area to lowland river. Figure 3 illustrates examples of simulation results for steady-state groundwater levels. At a precipitation level of 1200 mm/yr, the highest groundwater head level is approximately 300 m for the 40° slope angle, 320 m for the 30° slope angle, and 340 m for the 20° slope angle (Figures 2A–C). It indicates that the groundwater head level increases with a steeper slope angle. On the other hand, the precipitation also controls the groundwater level, in which the groundwater head increases with precipitation from 600 mm/yr to 1,000 mm/yr (Figures 2D–F). Hence, it can be inferred that groundwater head levels possessed a positive relationship with precipitation while a negative relationship with the slope angle.
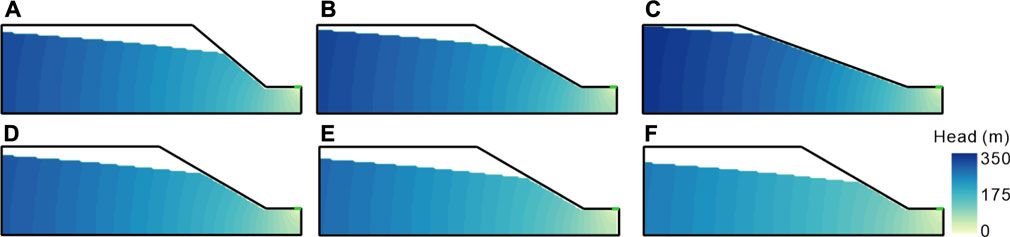
FIGURE 2. Examples of head distribution with different slope angles and precipitation levels: (A) slope angle is 40° and precipitation is 1200 mm/yr; (B) slope angle is 30° and precipitation is 1200 mm/yr; (C) slope angle is 20° and precipitation is 1200 mm/yr; (D) slope angle is 30° and precipitation is 1000 mm/yr; (E) slope angle is 30° and precipitation is 800 mm/yr; (F) slope angle is 30° and precipitation is 600 mm/yr. For the head distribution of all models, see Supplementary Figure S1.
The process of solute transport was simulated from the mountainous area to the river based on the steady-state flow field (Figure 3). Figure 4 presents the arrival time and total contaminant mass to the river for each simulation case. The arrival time analysis reveals that pollutants take approximately 70 years to reach the river. Specifically, the case of 20° slope angle at a 1,200 mm/yr rainfall recharge rate exhibits the earliest arrival time at ∼65 years. In contrast, the case of 35° and 40° slope angles at a 600 mm/yr rainfall recharge rate show the slowest solute transport with the arrival time at ∼75 years. Arrival time decreases with increasing precipitation, indicating that higher rainfall recharge rates promote the speed of solute transport. Furthermore, it increases with slope angles, highlighting that the gentler slopes are more conducive to contaminant transport. On the other hand, the total mass of pollutants discharged into the river is greatly influenced by the precipitation. The mass of contaminants discharged into the river increases from 45 to 67 with the increasing rainfall recharge rate over a 20-year period. However, the relationship between total mass and slope angle is less apparent, suggesting that slope angles have a limited impact on the mass of contaminants transported into the river.

FIGURE 3. Example of solute transport with time (slope angle is 30° and precipitation is 1000 mm/yr): (A) 25 years; (B) 50 years; (C) 75 years. Other plots of contaminant transport are shown in Supplementary Figures S2–6.
The findings of this study indicate the importance of evaluating contaminant transport between the mountainous area and basin plain, suggesting that a comprehensive analysis with multiple factors are needed in studying the contamination interaction between groundwater and surface water. In addition, the protection of groundwater resources in the mining sites and tunnels should be stressed as the contaminants possibly transport with groundwater from mountains to the surface water in the basin. Engineers should pay more attention to the groundwater flow system and water–rock interaction around the mining sites and tunnels, as well as techniques of water drainage and pollutant deposition in the underground engineering.
4 Conclusion
This study conducts simulations to analyze contaminant transport from the mountainous area to the lowland river. The investigation considers multiple cases with varying precipitation and slope angles along the gradient from the mountain to the plain, with a focus on calculating the total mass and arrival time of contamination. The simulation results reveal that both groundwater level and solute transport are influenced by precipitation and slope angles. The main findings are listed below:
(1) The general groundwater head demonstrates an increase with higher precipitation and a decrease with steeper slope angles, ranging from 300–340 m.
(2) The arrival time of contaminants from the mountain to the river exhibits a decrease with higher precipitation and an increase with steeper slope angles, with the minimum arrival time of approximately 65 years and the maximum arrival time of more than 75 years.
(3) The total mass of contaminants discharging into the river in 20 years shows an increase with precipitation. Although the trend is less clear with slope angles, slope angles have an unclear impact on the mass of contaminants transported into the river.
Data availability statement
The original contributions presented in the study are included in the article/Supplementary Material; further inquiries can be directed to the corresponding author.
Author contributions
XX: investigation, methodology, and writing–original draft. MZ: funding acquisition, project administration, validation, and writing–original draft. LZ: data curation, formal analysis, investigation, and writing–original draft. MM: methodology, software, and writing–original draft. JH: investigation, methodology, and writing–original draft. LL: formal analysis and writing–original draft. WQ: software and writing–original draft. ZX: conceptualization, validation, and writing–review and editing.
Funding
The author(s) declare that financial support was received for the research, authorship, and/or publication of this article. This work was supported by the National Natural Science Foundation of China (No. 42207074), Natural Science Foundation of Sichuan Province (No. 2023NSFSC0788), and Sichuan Transportation Science and Technology Program (2023-B-15).
Conflict of interest
Authors XX, MZ, LZ, MM, JH, LL, and WQ were employed by Sichuan Communication Surveying and Design Institute Co., Ltd.
The remaining author declares that the research was conducted in the absence of any commercial or financial relationships that could be construed as a potential conflict of interest.
Publisher’s note
All claims expressed in this article are solely those of the authors and do not necessarily represent those of their affiliated organizations, or those of the publisher, the editors, and the reviewers. Any product that may be evaluated in this article, or claim that may be made by its manufacturer, is not guaranteed or endorsed by the publisher.
Supplementary material
The Supplementary Material for this article can be found online at: https://www.frontiersin.org/articles/10.3389/fenvs.2024.1343903/full#supplementary-material
References
Harbaugh, A. W. (2005). MODFLOW-2005, the US Geological Survey modular ground-water model: the ground-water flow process. US Geological Survey Scientific Investigations Report, 253.
Lapworth, D. J., Krishan, G., MacDonald, A. M., and Rao, M. S. (2017). Groundwater quality in the alluvial aquifer system of northwest India: new evidence of the extent of anthropogenic and geogenic contamination. Sci. Total Environ. 599-600, 1433–1444. doi:10.1016/j.scitotenv.2017.04.223
Le, F., Li, R., Ruan, X., and Liu, C. (2023). Isotopic tracing of nitrogen source and interaction between surface water and groundwater of a small valley plain in the Zhangxi watershed. Appl. Geochem. 151, 105615. doi:10.1016/j.apgeochem.2023.105615
Liberoff, A. L., and Poca, M. (2023). Groundwater-surface water interactions in a semi-arid irrigated agricultural valley: a hydrometric and tracer-aided approach. Sci. Total Environ. 903, 166625. doi:10.1016/j.scitotenv.2023.166625
Liu, L., and Xu, Z. (2016). Regionalization of precipitation and the spatiotemporal distribution of extreme precipitation in southwestern China. Nat. Hazards 80, 1195–1211. doi:10.1007/s11069-015-2018-x
Marti, E., Leray, S., Villela, D., Maringue, J., Yáñez, G., Salazar, E., et al. (2023). Unravelling geological controls on groundwater flow and surface water-groundwater interaction in mountain systems: a multi-disciplinary approach. J. Hydrology 623, 129786. doi:10.1016/j.jhydrol.2023.129786
Modie, L. T., Kenabatho, P. K., Stephens, M., and Mosekiemang, T. (2022). Investigating groundwater and surface water interactions using stable isotopes and hydrochemistry in the Notwane River Catchment, South East Botswana. J. Hydrology Regional Stud. 40, 101014. doi:10.1016/j.ejrh.2022.101014
Nasim, S., Helfand, S., and Dinar, A. (2020). Groundwater management under heterogeneous land tenure arrangements. Resour. Energy Econ. 62, 101203. doi:10.1016/j.reseneeco.2020.101203
Paladino, O., Seyedsalehi, M., and Massabò, M. (2018). Probabilistic risk assessment of nitrate groundwater contamination from greenhouses in Albenga plain (Liguria, Italy) using lysimeters. Sci. Total Environ. 634, 427–438. doi:10.1016/j.scitotenv.2018.03.320
Raiber, M., Lewis, S., Cendón, D. I., Cui, T., Cox, M. E., Gilfedder, M., et al. (2019). Significance of the connection between bedrock, alluvium and streams: a spatial and temporal hydrogeological and hydrogeochemical assessment from Queensland, Australia. J. Hydrology 569, 666–684. doi:10.1016/j.jhydrol.2018.12.020
Santana, C. S., Olivares, D. M. M., Silva, V. H. C., Luzardo, F. H. M., Velasco, F. G., and de Jesus, R. M. (2020). Assessment of water resources pollution associated with mining activity in a semi-arid region. J. Environ. Manag. 273, 111148. doi:10.1016/j.jenvman.2020.111148
Su, H., Kang, W., Xu, Y., and Wang, J. (2018). Assessing groundwater quality and health risks of nitrogen pollution in the shenfu mining area of shaanxi Province, northwest China. Expo. Health 10, 77–97. doi:10.1007/s12403-017-0247-9
Sun, H., Hu, X., Yang, X., Wang, H., and Cheng, J. (2023). Estimating water pollution and economic cost embodied in the mining industry: an interprovincial analysis in China. Resour. Policy 86 (B), 104284. doi:10.1016/j.resourpol.2023.104284
Tomiyama, S., and Igarashi, T. (2022). The potential threat of mine drainage to groundwater resources. Curr. Opin. Environ. Sci. Health 27, 100347. doi:10.1016/j.coesh.2022.100347
Wang, W., Chen, Y., Wang, W., Zhu, C., Chen, Y., Liu, X., et al. (2023). Water quality and interaction between groundwater and surface water impacted by agricultural activities in an oasis-desert region. J. Hydrology 617, 128937. doi:10.1016/j.jhydrol.2022.128937
Wu, Q., Dong, S., Li, B., and Zhou, W. (2018). “Mine water inrush,” in Encyclopedia of sustainability science and technology. Editor R. A. Meyers (New York: Springer).
Xu, Z., Hariharan, J., Passalacqua, P., Steel, E., Chadwick, A., Paola, C., et al. (2022). Effects of geologic setting on contaminant transport in deltaic aquifers. Water Resour. Res. 58, e2022WR031943. doi:10.1029/2022wr031943
Yin, Z., Luo, Q., Wu, J., Xu, S., and Wu, J. (2021). Identification of the long-term variations of groundwater and their governing factors based on hydrochemical and isotopic data in a river basin. J. Hydrology 592, 125604. doi:10.1016/j.jhydrol.2020.125604
Zhai, Y., Zhao, X., Teng, Y., Li, X., Zhang, J., Wu, J., et al. (2017). Groundwater nitrate pollution and human health risk assessment by using HHRA model in an agricultural area, NE China. Ecotoxicol. Environ. Saf. 137, 130–142. doi:10.1016/j.ecoenv.2016.11.010
Zhang, H., Xu, Y., Cheng, S., Li, Q., and Yu, H. (2020). Application of the dual-isotope approach and Bayesian isotope mixing model to identify nitrate in groundwater of a multiple land-use area in Chengdu Plain, China. Sci. Total Environ. 717, 137134. doi:10.1016/j.scitotenv.2020.137134
Zhang, Y., Dai, Y., Wang, Y., Huang, X., Xiao, Y., and Pei, Q. (2021a). Hydrochemistry, quality and potential health risk appraisal of nitrate enriched groundwater in the Nanchong area, southwestern China. Sci. Total Environ. 784, 147186. doi:10.1016/j.scitotenv.2021.147186
Zhang, Y., He, Z., Tian, H., Huang, X., Zhang, Z., Liu, Y., et al. (2021b). Hydrochemistry appraisal, quality assessment and health risk evaluation of shallow groundwater in the Mianyang area of Sichuan Basin, southwestern China. Environ. Earth Sci. 80, 576. doi:10.1007/s12665-021-09894-y
Keywords: mountain–plain area, groundwater contamination, river pollution, solute transport, MODFLOW simulation
Citation: Xu X, Zhu M, Zhou L, Ma M, Heng J, Lu L, Qu W and Xu Z (2024) The impact of slope and rainfall on the contaminant transport from mountainous groundwater to the lowland surface water. Front. Environ. Sci. 12:1343903. doi: 10.3389/fenvs.2024.1343903
Received: 24 November 2023; Accepted: 19 January 2024;
Published: 05 February 2024.
Edited by:
Lei Xia, KU Leuven, BelgiumReviewed by:
Jin Wu, Beijing University of Technology, ChinaHongji Wang, Southwestern University of Finance and Economics, China
Liting Hao, Beijing University of Civil Engineering and Architecture, China
Copyright © 2024 Xu, Zhu, Zhou, Ma, Heng, Lu, Qu and Xu. This is an open-access article distributed under the terms of the Creative Commons Attribution License (CC BY). The use, distribution or reproduction in other forums is permitted, provided the original author(s) and the copyright owner(s) are credited and that the original publication in this journal is cited, in accordance with accepted academic practice. No use, distribution or reproduction is permitted which does not comply with these terms.
*Correspondence: Zhongyuan Xu, zyxu@swjtu.edu.cn