- 1Centre for Interdisciplinary Scientific Research at UzhNU, Uzhhorod National University, Uzhhorod, Ukraine
- 2Institute for Sustainable and Circular Construction, Faculty of Civil Engineering, The Technical University of Košice, Košice, Slovakia
- 3Department of International Studies and Public Communications, Centre for Sustainable Development, Uzhhorod National University, Uzhhorod, Ukraine
- 4Branch for Physics of Mining Processes of the M. S. Poliakov Institute of Geotechnical Mechanics the National Academy of Sciences of Ukraine, Dnipro, Ukraine
This study assessed the possibility of using wood as a building material for the construction of houses. A comprehensive method was used, which consisted of analysing environmental management regulations, applying the life cycle assessment method to minimise the carbon footprint; using software to calculate the carbon footprint of a wooden house at different stages of the life cycle. The object of study is the carbon footprint of a house built of wood. The Life Cycle Assessment method was used as a methodology for assessing the life cycle. Using the One Click Life Cycle Assessment and Life Cycle Cost software, the carbon footprint of a log house was calculated for the product life cycle stage mentioned above (A1-A3). When calculating the carbon footprint of wood-based building materials, carbon emissions were taken into account not only from the finished products, but also from all other products obtained as a result of logging. When calculating the carbon footprint, greenhouse gas emissions from all activities are estimated. We have obtained data on the life cycle cost of a wooden house in terms of electricity use. Accordingly, we obtained a value of global warming potential (A1-A3) of 0.51 kg CO2 e/kWh. We also obtained data on the life cycle cost of a wooden house in terms of diesel consumption. According to the results of the LCA, the value of the global warming potential due to meeting the water supply/sewage needs is (A1-A3) 0.69 kg CO2 e/m3. The value of the global warming potential due to meeting the heat supply needs of production needs is (A1- A3) 0.13 kg CO2 e/kWh. Based on the information obtained, we can conclude that it is advisable to use a wooden log house as a building material, as the carbon footprint is smaller than that of a brick building. The final section presents the results of calculating the life cycle cost of a wooden house by discount factor and inflation, the results of the life cycle cost of a wooden house by percentage of energy costs, and the results of assessing the life cycle cost of a wooden house (displaying parameters according to the European energy certification scale). Based on the carbon footprint assessment (using the Life Cycle Assessment methodology), economic comparison (Life Cycle Cost and total construction costs), and expert assessment (based on technical and ergonomic parameters) of the two construction technologies, the feasibility and possibility of using wood as a building material was established. The study proves the feasibility of applying the LCA method in the construction industry.
1 Introduction
One of the most pressing and global issues of our time is the fight against climate change and overcoming the consequences of global climate change, which are among the central tasks in international cooperation of many countries and are being addressed at the highest level. Global climate change is a significant challenge to humanity and forces all conscious members of society to support the intensification of environmental research (Turner et al., 2016; Petlovanyi et al., 2023; Sun et al., 2023) with their subsequent industrial application, which is undoubtedly already one of the most important issues of national (Brancalion et al., 2022; Napathorn, 2022; Ross et al., 2022) and international development programmes in many highly developed countries (Gupta et al., 2020; Zimmerman and Groffman, 2021; Spagnoli, 2022). Global environmental problems have contributed to the development and implementation of effective laws, regulations (Philippopoulos-Mihalopoulos and Andreas, 2011a; Garver, 2013), directives (Evans, 2006; Francis et al., 2016), requirements and software (Vilčeková et al., 2020), which naturally encourage the decarbonisation of economies to achieve carbon neutrality in all spheres of life, where technological progress in the use of renewable energy sources and secondary material resources are key players.
Observations show that in Ukraine, the rate of change in global and European climate indicators has been exceeded. According to the National Oceanic and Atmospheric Administration (NOAA), the region to which Ukraine belongs has experienced one of the highest rates of temperature growth in the world over the past 30 years (Synetska and Hupková, 2023).
The change in climate indicators exceeds the global trend (Petrović et al., 2023), and in combination with the unfavourable domestic environmental situation, this will inevitably increase the impact of global warming on the territory of Ukraine and neighbouring countries (Borovska and Khokhlov, 2023). They lead to the intensification of adverse natural and man-made processes (Timoshuk et al., 2012; Khorolskyi et al., 2019), creating threats and risks to nature, the economy, socio-economic development, human life and health.
The global average temperature is the average of all annual temperatures on Earth. Usually, the data is calculated by region for each day, and then the arithmetic mean for the year is displayed for the entire planet. The increase in the global average temperature on Earth means that there are more hot days in a year and fewer cold days. This does not mean that each day has become almost 1° warmer compared to the corresponding day of the year in the pre-industrial era. According to observations, the average global temperature on Earth has already risen by 1.1°C since 1880. Global warming is uneven across the planet. The average temperature in the Arctic regions of the planet has already risen by 2°C.
In the forestry sector, climate change leads to changes in the indicators of optimal environmental conditions for forest ecosystems. Increasing extreme temperatures in summer, in particular, threatens the loss of certain species and the emergence of new (including invasive) species, which will affect the species composition and reduction of forest areas, as well as the expansion of the ranges of some pest species (Kittur et al., 2023).
Taking into account the impact of global climate change on energy supply, environment and economic security, the priority areas of state policy in this area include climate threats and natural disasters to ensure the gradual transition of Eastern European countries to low-carbon economic development, reduce the carbon intensity of the economy, improve the quality of greenhouse gas sinks and storage, adapt the country’s socio-economic system to climate change and strengthen the role of Eastern European countries in global climate change efforts. A key step in effective adaptation is a clear understanding of the expected impacts, vulnerabilities and risks to key economic sectors from short-, medium- and long-term climate change-related changes. A proper understanding of the impacts, risks and vulnerabilities will allow policymakers not only to determine the sequence of actions, but also to understand which areas need to be developed for appropriate interventions and programmes. To this end, a separate research programme should be developed to study the short- and long-term impacts of climate change.
The above data shows that climate change is a consequence of human activity. The key to overcoming negative trends (increased greenhouse gas emissions, deteriorating air quality, rising ambient temperatures, etc.) is low-carbon economic development. The construction sector is one of the most resource-intensive and innovative. Resource intensity means the use of a large number of materials and technologies, which leads to an increased man-made impact on the environment. That is why our study is aimed at a comparative assessment of the capabilities of the construction industries in Ukraine and Slovakia. To do this, we analysed the regulatory framework. After that, we can apply the Life Cycle Assessment methodology (Hellweg and Milà i Canals, 2014; Jolliet et al., 2014) to compare the use of different building materials and their environmental impact. All this will allow us to justify the feasibility of using environmentally preferable building materials for housing construction.
Eastern European countries have a high carbon intensity of their economies (Jolliet et al., 2014; Xiao et al., 2023). There are common features of the construction industries, which include the use of building materials whose production requires environmental changes. As previously mentioned, climate change poses a serious threat to the country’s sustainable development due to the high carbon intensity of the economy, the increase in the number of extreme weather events and the associated risks to the health and livelihoods of the population, natural ecosystems and economic sectors. That is why we have studied the construction industries of Ukraine and Slovakia. This choice is explained by the fact that the economies of these countries are carbon-intensive, which leads to carbon dioxide emissions into the atmosphere, causing extreme weather events. The application of the latest environmental protection measures helps to reduce the man-made burden on the environment. Ukraine is a party to the United Nations Framework Convention on Climate Change and the Kyoto Protocol and must ensure compliance with a number of obligations under these international agreements (da Costa et al., 2023).
Ukraine has signed a joint agreement with the European Union and its Member States (UPA), which opens up new opportunities for reforming and creating new standards in all areas of public life, including environmental protection (Zhabotynska and Velivchenko, 2019). Sectoral issues of cooperation in the field of environmental protection are defined in Title V, Economic and Sectoral Cooperation, Chapter 6, Environment. The importance of climate change adaptation in Ukraine is of particular importance in the context of the EU-Ukraine Alliance Agreement, as Article 365 stipulates that the parties’ cooperation covers the formulation and implementation of climate change policy (Duleba, 2022).
The importance of EU adaptation to climate change was reaffirmed in 2013, when the European Commission adopted a communiqué on the EU Climate Change Adaptation Strategy, which included several elements to support EU member states in their efforts to address climate change (Kiforenko, 2022). Adaptation to climate change, including: providing advice and funding, facilitating the exchange of new knowledge and information, and strengthening the resilience of some of the most vulnerable sectors of life (Karabin et al., 2021). EU countries have also agreed to spend at least 20% of their budgets on climate change-related activities, including mitigation and adaptation, for the period 2014–2020. In addition, the EU has integrated climate change adaptation into numerous sectoral areas of EU policy in the form of recommendations for monitoring and analysis of necessary measures (Durman et al., 2021). The Strategy lists the impacts of climate change, as well as more specific climate change adaptation measures and actions, and calls for specific integrated or sectoral adaptation measures at the EU level.
Ensuring adaptation to climate change, building resilience and reducing climate change risks is part of Ukraine’s commitments under the ratified UNFCCC and the EU-Ukraine Association Agreement (Holubieva and Akulenko, 2020). The set of necessary measures to fulfil this task should include the integration of climate change adaptation and building resilience to climate-related risks and disasters into national development strategies, plans and programmes. The economy and its sectors, providing national support for the creation and continuous updating of practical assessments and modelling of expected climate change and its impacts, including regional distribution, identification of risks and vulnerabilities to climate change at the level of territorial communities, natural ecosystems, and the economic department.
At the same time, the implementation of EU environmental directives on climate change and ozone layer protection in Ukraine is extremely slow, as it cannot fully take advantage of the opportunity provided by the EU-Ukraine Association Agreement to improve the environment. This involves, first of all, the adoption of national legislation and the designation of authorised bodies as part of the implementation of Directive 2003/87/EC establishing a system for trading in emission allowances for GHG emissions (EC) No 842/2006 concerning certain fluorinated greenhouse gases, Regulation (EC) No 2037/2000 on substances that deplete the ozone layer (Dixon, 2011).
In August 2015, as part of the regulation of the negative anthropogenic impact on climate change and adaptation to its changes and fulfillment of the requirements of the United Nations Framework Convention on Climate Change and its Kyoto Protocol, Ukraine submitted to the UNFCCC Secretariat the National Inventory of Anthropogenic Emissions by Sources and Removal by Sinks of Greenhouse Gases in Ukraine for the period 1990–2013. In October 2015, it was reviewed by experts from the UNFCCC Secretariat and a report was published with comments to be taken into account when preparing the National Inventory of Anthropogenic Greenhouse Gas Emissions by Sources and Removal by Sinks in Ukraine for 2014–2016 (Marcinek et al., 2023). On 5 September 2022, the European Union published an annual report on Ukraine’s implementation of reforms under the EU-Ukraine Association Agreement for the period from the publication of the last report on 1 December 2020 to the beginning of Russia’s military aggression on 24 February 2022 (Kirsch, 2022).
We analysed the compliance of the current legislation of the countries with the environmental certification standards: Forest Stewardship Council forest certification (Bocci and Fortmann, 2023), Programme for the Endorsement of Forest Certification (N Doua, 2023), ISO 9001 and DSTU ISO 9001:2015 - management certification standard for a wide range of organisations (Camango and Cândido, 2023), ISO 14001 Environmental Management System (Fonseca et al., 2023), IS0 45,001 Standard for Management System, Health and Safety at Work (Arocena et al., 2023). Carbon standards PAS 2050, ISO/TS 14067 and the GHG protocol (Liu et al., 2023). As well as ISO 14040 standard for environmental management - life cycle assessment - principles and framework (Ai Lin Teo and Yean Yng Ling, 2006).
It has been found that buildings around the world consume up to 40% of energy and cause half of global greenhouse gas emissions. The introduction of life cycle assessment (LCA) in the construction industry is important because it systematically measures every environmental impact associated with each process. The implementation of LCA can identify and mitigate environmental impacts at the development stage, thereby contributing to the sustainability of the construction industry.
The total environmental impact of a building is the result of the environmental loads that occur over the life cycle of the building: the initial impact, the annualised recurrent impact and the deconstructed impact. Initial impacts occur during the design and construction of a building, including project management activities, material use, the construction process and waste management. Annualised recurrent impacts are those that recur each year and result from the use of energy for heating, lighting, ventilation and cooling, as well as repairs and renovations over long periods of time. The third stage of deconstruction occurs during the demolition of the building and is the final stage.
Thus, the analysis of regulatory documents for compliance with global standards (Bocci and Fortmann, 2023; N Doua, 2023; Camango and Cândido, 2023; Fonseca et al., 2023; Arocena et al., 2023; Liu et al., 2023; Ai Lin Teo and Yean Yng Ling, 2006) allowed us to make several generalisations that form the basis of the present study:
First, given the high carbon intensity of Ukraine’s economy, it is necessary to develop a nationwide system for managing risks caused by changes in the frequency and intensity of extreme weather events and natural disasters in Ukraine, as well as climate-related migration, and adaptation across borders with partner countries. The key to the implementation of this system is the development of climate change projects with border countries, as well as the development and implementation of national climate change adaptation plans, coordinated with the relevant plans implemented in districts, regions and regional communities. Slovakia, as a neighbouring country of Ukraine, has similar climatic conditions. That is why it is advisable to exchange experience and explore the possibilities of the construction industry, as the issue of reducing carbon emissions is not a national issue, but a regional one (in this case, Eastern Europe).
Secondly, certification and environmental management are the main trends in the field of environmental protection. That is why it is logical to use certified building materials. Currently, both Ukraine and Slovakia have FSC-certified forests, which makes it reasonable to consider using wood as a raw material for construction. In addition, existing wood treatment solutions allow for the use of not only wood from healthy trees, but also from trees affected by pests and fungi, which opens up new opportunities for integrated wood processing and, in the future, forest health.
Third, the hierarchy of approaches to waste management has many limitations. An analysis of regional conditions may reveal that the use of waste as energy is economically justified, as these conditions are provided by the current development of existing industrial complexes (steelmaking, for heating residential complexes; participation in the cement industry, etc.) Therefore, the selection of certain waste management methods should be based on studies of integrated waste management systems, taking into account regional conditions, such as the composition and nature of solid waste, which varies by season; annual solid waste generation; climatic conditions; demand for organic fertilisers, energy and secondary raw materials; and economic factors.
Fourthly, the assessment of socio-economic aspects allows us to consider the internal processes of the waste management system from the point of view of all parties involved, since the main obstacle to the development of the waste management sector is the refusal of investors to finance waste disposal, due to low tariffs of the population and the lack of guarantees that the state should provide on the basis of the legislative framework.
That is why a comparative assessment of the capabilities and success of the Slovak and Ukrainian wood construction industries, taking into account the Life Cycle Assessment certification standards, will justify the feasibility of using wood as a construction raw material.
2 Methodology
2.1 Application of the product life cycle assessment method as a basis for comparative analysis of the wood construction industry capabilities
Life Cycle Assessment or LCA (also known as Life Cycle Analysis) is a method of assessing the environmental impacts of a commercial product, process or service at all stages of its life cycle. For example, in the case of industrial products, the environmental impact assessment extends from the extraction and processing of raw materials (the cradle), through the production, distribution and use of the product, to the treatment or final disposal of the product’s materials.
An environmental impact assessment study involves a thorough inventory of the energy and materials required for the entire industrial value chain of a product, process or service and the calculation of the corresponding environmental emissions. Life cycle assessment therefore assesses the cumulative potential environmental impact. The aim is to document and improve the overall environmental profile of a product.
Well-known LCA procedures are part of a series of environmental management standards. These include the International Organisation for Standardisation (ISO) 14,000, in particular ISO 14040 and ISO 14044. ISO 14040 defines the “principles and structure” of the standard, while ISO 14044 provides a general description of “requirements and guidance”. In general, ISO 14040 was written for a management audience, while ISO 14044 was written for practitioners. The introduction to ISO 14040 defines life cycle assessment as follows.
LCA examines environmental aspects and potential environmental impacts throughout the entire life cycle of a product (i.e., from cradle to grave), from raw material procurement to production, use and disposal. The general categories of environmental impact that need to be considered include resource use, human health and ecological impacts.
Life cycle assessment (LCA) can often be mentioned synonymously with life cycle analysis in the scientific literature and agency reports. In addition, due to the general nature of LCA, which is to study the impacts of the life cycle from extraction of raw materials (cradle) to disposal (grave), it is sometimes referred to as “cradle-to-grave analysis”. The term “life cycle” means that a fair, holistic assessment requires an assessment of raw material extraction, production, distribution, use and disposal, including all intermediate stages of transport necessary or caused by the product’s existence.
According to the EPA’s National Risk Management Research Laboratory, “LCA is a method of assessing the environmental aspects and potential impacts associated with a product, process, or service by using:
1) Compilation of an inventory of relevant energy and material resources and environmental emissions.
2) Assessment of potential environmental impacts associated with the identified resources and emissions.
3) Interpretation of the results to help you make a more informed decision."
In summary, LCA is a method of assessing the environmental impacts associated with all stages of a product’s life cycle, from raw material extraction to material processing, manufacturing, distribution, use, repair and maintenance, and disposal or recycling. This information is used to improve processes, support policy, and provide a sound basis for informed decision-making.
The results are used to help decision-makers select products or processes that result in the lowest environmental impact by considering the entire product system and avoiding sub-optimisation that would occur if only one process were used.
Thus, the goal of LCA is to compare the full range of environmental impacts that may be inherent in products and services by quantifying all inputs and outputs of material flows and assessing how these material flows affect the environment.
There are two main types of these methods: Attributive LCA and Contingent LCA. Attributional LCA attempts to determine the burden associated with the production and use of a product or a specific service or process over a specified time period.
Consequential LCA aims to determine the environmental consequences of a decision or proposed change in the system under study, and is therefore future-oriented and requires consideration of market and economic impacts. In other words, Attributional LCA “attempts to answer the question ‘how are the flows (i.e., pollutants, resources and exchanges between processes) occurring within the selected time window?’, while Consequential LCA attempts to answer the question ‘how will the flows change beyond the immediate impact on the system in response to the decisions made?
A third type of LCA, called social LCA, is also under development and is a separate approach designed to assess potential social and socio-economic impacts and consequences. Social Life Cycle Assessment (SLCA) is a useful tool for companies to identify and assess potential social impacts throughout the life cycle of a product or service on various stakeholders (e.g., employees, local communities, consumers). The SLCA is based on the UNEP/CETAC Guidelines for Social Life Cycle Assessment published in 2009 in Quebec. The tool is based on the ISO 26000:2010 Social Responsibility Guidelines and the Global Reporting Initiative (GRI) Guidelines.
The limitation of LCA to focusing solely on the environmental aspects of sustainability, rather than on economic or social aspects, distinguishes it from product line analysis (PLA) and similar methods. This limitation has been made intentionally to avoid overloading the method, but recognises that these factors should not be ignored when making product decisions.
Some commonly accepted procedures for LCA are included in the ISO 14000 series of environmental management standards, in particular ISO 14040 and 14,044. Life cycle assessment of products in terms of greenhouse gas (GHG) emissions can also be done in accordance with specifications such as the Publicly Available Specification (PAS) 2050 and the GHG Protocol Life Cycle Accounting and Reporting Standard.
According to the certification standard, there are 17 life cycle stages, which reflect the harvesting of raw materials, their production, the use of the building, and the end of life. The number of stages may vary, but assessments of stages A1-A3 (A1: Extraction and processing of raw materials, processing of incoming recycled materials (e.g., recycling processes; A2: Transport to the producer; A3: Production of raw materials). It is a holistic methodology that can assess the impact of a product (in our case, a house) on climate change. The methodology is described in more detail in EN ISO 14040–44.
Thus, the proposed methodology allows us to compare the capabilities of the wood construction industry. We will calculate the basic LCA indicators and conduct an economic assessment of the proposed solution.
2.2 Estimating life cycle costs with one click life cycle assessment software
One Click Life Cycle Assessment (Abdelaal et al., 2023; Almroth and Rehnberg, 2023; Athibaranan et al., 2023; Rana et al., 2023) allows to assess the life cycle of a product. In particular, the construction of a house from various building materials. The general algorithm was as follows:
1) First, we set the regionalisation of the cost by selecting the country and clicking “Load regional cost parameters”. If we have a licence with automatic costing, the costing method will already be defined. After that, we checked the generated parameters: exchange rate (expressed in relation to 1 euro); hourly labour rate of an employee (average hourly labour cost for a low-skilled worker); hourly labour rate of an artisan (average hourly labour cost for a high-skilled worker). It should be noted that the regional material cost index can also be changed, but it is filled with default values based on the urbanisation of your location. The discount factors and inflation rate can be edited if you know that these values are different for your project. If not, use the default values.
2) The next step involves setting the calculation period for the LCC study, as specified by the client/depending on the purpose of the study, in the “Calculation Period” input form. Please note: if the calculation for LCA is complete, LCC results will be displayed after the calculation period is set. However, we should adjust the LCC based on specific project cost information.
After that, we moved on to the life cycle assessment.
1) First, we have estimated the construction phase costs (A0) included in the questionnaire form “Other capital costs”. These costs include: land acquisition, permits and licences, planning, design and supervision costs, soil surveys and comparisons in specific lines if we wish to include these items in the scope.
2) Next, estimate the costs of construction materials (A1-A5) included in the input form “Construction materials”. The software provides a default unit cost.
3) After that, the maintenance, operation and repair costs (B1-B3) were estimated. These operating expenses are included in the “Other operating expenses” input form. Other operating expenses (cleaning, minor repairs, etc.) should be added to the “Other operating expenses” input form. The input data was calculated based on the area of the building.
4) In addition, the replacement/renovation costs (B4-B5) are calculated. The replacement/repair costs (B4-B5) will be calculated based on the building materials and their workload/investment costs, which are discounted to the net present value and service life of these materials. The service life of the building materials will be determined based on the service life values selected for the LCA calculations. They can be adjusted if necessary.
5) In the final step for this cycle, the operating energy and water costs were calculated (B6 and B7). The operating costs are calculated based on the operational energy and water consumption. As with the building materials, the energy and water consumption values are taken from the LCA calculations (and vice versa) or entered separately (if LCA integration is not used).
6) In addition, end-of-life costs (C1-C4) are calculated. These costs are automatically calculated based on the capital costs (A0-A5) using a proportion of the total capital costs. The percentage value can be set in the calculation parameters.
After that, we analyzed the generated LCC file. This allowed us to conduct a comparative analysis of log houses and traditional materials.
The results of the calculations are presented in the Results and Discussion section.
3 Result and discussion
In accordance with the research objective, we assessed the possibilities of building from wood. Compared to traditional brick houses, wooden houses are a more environmentally friendly and economical option. Not only is wood a part of nature, but its use is also good for the environment. Building with wood has less negative environmental impact and a lower carbon footprint than traditional construction methods.
The most obvious factor in building a house out of wood is its environmental friendliness. Regardless of the fact that wood is one of the few renewable resources in construction, it helps to reduce the carbon footprint. A gas that absorbs harmful carbon dioxide from the atmosphere and stores it as carbon. Wood and modern building materials based on it are subjected to greater stress than steel; the production of building elements made of wood or wood products requires significantly less energy than the production of similar elements made of steel (Cherubini et al., 2012). Given the concern of the international community and the world’s leading countries about energy conservation and cleansing the atmosphere of carbon dioxide, the relevance of the Forest Europe plan, which is being actively discussed on the construction site today, is 80% in 2020. Many European countries have already approached this target. For example, in Finland, wooden houses account for about 40% of the total housing stock, in Germany - 20%, and in Austria - 30% (Churkina et al., 2020).
Throughout Europe, Ukraine has preserved the largest and most valuable examples of wooden buildings. Consequently, great interest in Ukrainian wooden architecture arose in Slavic and other national literature that emerged in the early 19th century, and it has become especially intense in Western Europe in recent years. Thanks to favorable climatic and economic conditions, Ukraine has long had a large number of wood species used in construction, from soft conifers to beautiful hardwoods such as maple, sycamore, aspen, ash, and finally linden, pear, yew, beech, and oak.
Log houses are built faster than traditional brick houses and require less maintenance. Construction costs 30% less than concrete and brick houses. The latter is due to the cheapness and lightness of wood construction. A log house does not require a huge foundation. It will stand perfectly on a shallow, columnar or pile foundation. This ensures the speed of building construction, so the construction can be completed within 2–4 weeks, especially if a screw pile is chosen as the foundation, no earthworks and concrete structures are required (Brienen et al., 2015).
When calculating the cost of building a log house, it is necessary to take into account not only the cost of materials, but also the work to be performed during the construction process. Accordingly, the main factors affecting the cost of a house are as follows:
1. Project. The price of any house starts with the project. Development of an exclusive project can cost up to 10% of the final construction costs. The construction of a house from timber according to a standard project is cheaper, there is no need to draw up fundamentally new working documentation, carry out architectural work and complex calculations.
2. Foundation. The more complex the foundation structure and the deeper it is, the more expensive it is. Building a house from timber just makes it possible to save on the foundation, because such a house is relatively lightweight and does not require a complex and deep foundation.
3. Walls and number of floors. The price of a house directly depends on the type and size of the building material, as well as on the complexity of the structure - the number of floors, walls and partitions.
4. Roofing. The cost of a house is affected by the complexity of its roof structure and the type of roofing material.
5. Windows. The more windows in the house, and the area of glazing, the more expensive the delivery of windows and installation will be. Also, the price will depend on the quality of double-glazed windows, window frames with mechanisms that prevent shrinkage.
6. Doors. The cost of building a house includes the cost of installing doors. The price is influenced by the number and type of doors, transportation and installation of doors and door frames with mechanisms that prevent shrinkage.
7. Floors. The cost of building a house includes the cost of laying floors. The price is affected by the area of the flooring and its type, the cost of installation work.
8. Ceilings. The cost of building a house includes the cost of arranging ceilings. The price is affected by the area and type of ceilings, the cost of installation work.
9. Communications. The price of the house depends on the cost of work on the installation of electrical, heating, water supply, ventilation and sewage systems.
10. Finishing work. The price of the house depends on the cost of interior finishing and exterior painting works.
11. Transportation. The cost of construction must take into account the cost of delivery of building material to the site of assembly of the house from timber, removal, cleaning of construction waste. In the case of remote construction, the cost of accommodation of the construction team shall be taken into account.
Our study analyzed the modern construction market, which specializes in the production of log houses and traditional materials. The calculations include data related exclusively to the process of preparation, installation, and related processes to ensure normal working conditions for employees, but exclude interior work.
The carbon footprint of a house built of wood is considered as the object of study. Based on the carbon footprint assessment, it is possible to compare a house made of wood with an alternative (brick) house and analyze the possibilities and prospects of the construction industry using wood materials. The house had the following parameters: the area of the house was 100 square meters, the number of floors was 1, the type of wood was “Spruce”, the use of antiseptic was provided for the treatment of finished logs during the construction phase, natural linen insulation in rolls was used for sealing between the logs, and linseed oil was also used to treat the interior of the house. The parameters of the house and measures to prevent deterioration (mold, pest infestation, shrinkage) are typical for the conditions in Ukraine and Slovakia. A state examination was conducted and conclusions were obtained on the safety of using varnishes, emulsions, and paints in the construction of wooden houses.
For a house of about 100 m2, 35 m3 of spruce wood with a diameter of 24–28 cm is required. It is best to use winter forest, as the logs will not emit resin in warm temperatures. During this period, all wood is drier, and therefore less prone to rotting and infection by fungal diseases. Timber harvested in winter is usually less prone to deformation and cracking. Natural drying makes timber stronger and more durable. In addition, harvesting timber in winter at low temperatures contributes to the excellent preservation of its light natural color. The finished logs were treated during the construction phase with Adler antiseptic to prevent them from being affected by blue, bark beetle and woodworm. Natural linen insulation in rolls was used for caulking between the logs. For exterior painting, we used water-based paint by Adler (2 coats). Natural linseed oil was used to finish the interior of the house. The areas between the logs, already on the assembled house after “shrinkage” were tightened with a sealant as people call “warm seam”. The cost of such a house, including all materials and labor, will be about 45 thousand USD.
We calculated the need for bricks for a brick house (Table 1).
Based on the data in Table 1, we calculated the cost and time of building the walls (without interior work) of a brick house (approximately 100 m2 in area). According to these calculations, the construction period for a 100 m2 house will be 1 month (assuming a continuous 6-day working week of 8–10 h). During this time, approximately 600 kWh of electricity will be used to operate the two kWh concrete mixer alone. The amount of water for concrete will be at least 3 tons. In addition, such houses require significant costs to build the foundation. Despite a number of advantages, brick, gas block, foam block, cinder block and other building materials for the construction of houses are more in demand among the population. Table 2 shows the results of the survey among the population.
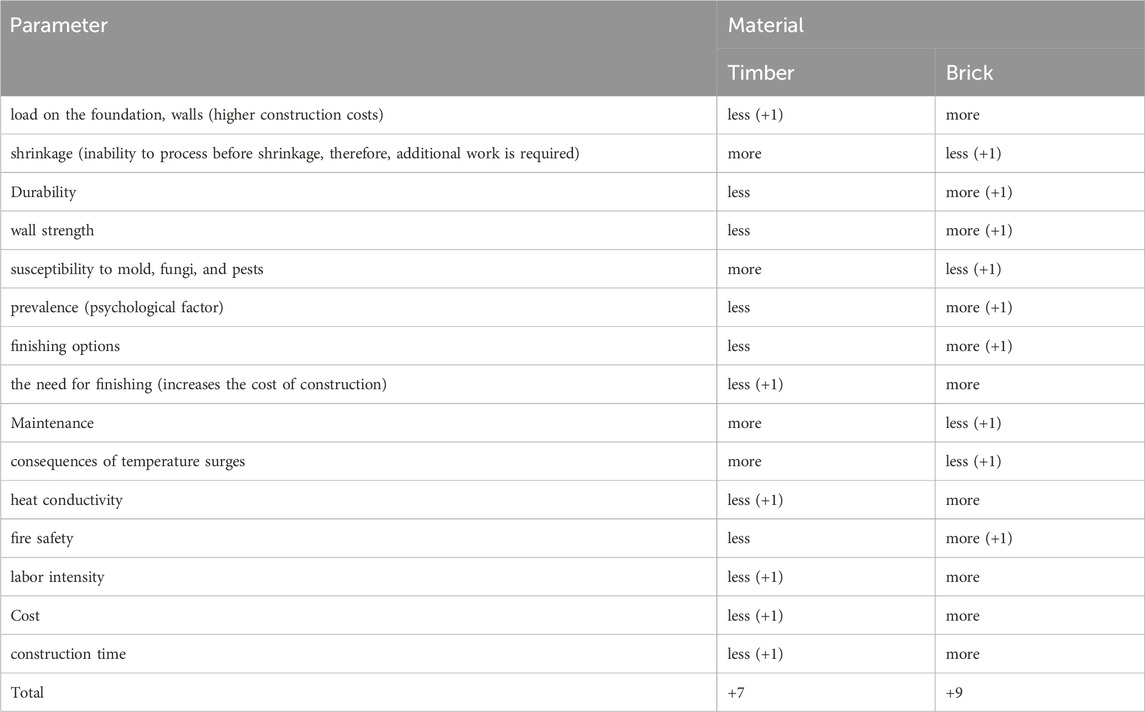
Table 2. Advantages and disadvantages of brick and log houses (based on the population’s perceptions).
As Table 2 shows, the population has a perception that brick houses are more reliable and safe. However, this is only a misconception, as the cost of building brick houses (excluding the cost of interior work) is 25% higher (45 thousand USD for wood and 60 thousand USD for brick), and if we take into account part of the cost of labor and interior work, the advantages in favor of wood houses will become even more convincing. In addition, more harmful materials are used to decorate brick houses: plastic, metal profiles, etc., which significantly increases the carbon footprint. It is worth noting that both hardwood and composite wood materials can be used in the construction and decoration of eco-houses, but it is important that no toxic substances, such as phenol, formaldehyde, benzene, and toluene, are released throughout its life cycle. Only a certificate of appropriate quality can provide confidence in the environmental friendliness of the material. Figure 1 shows a typical house made of wood.
After analyzing the time and cost of building houses, as well as the public’s perceptions of the advantages and disadvantages of wood and log houses, basic carbon footprint indicators can be calculated.
When calculating the carbon footprint of wood-based building materials, carbon emissions should be taken into account not only from finished products, but also from all other products obtained as a result of logging (Brienen et al., 2015). The methodology for calculating the ecological footprint is developed and adjusted by the international organization Global Footprint Network (van den Bergh and Grazi, 2015).
We calculated the life cycle cost at stages A1-A3 for all types of resources used. Accordingly, the value of the global warming potential (A1-A3) is 0.51 kg CO2 e/kWh.
It has been determined that housing construction accounts for approximately 29% of total material consumption, which includes all raw materials and resources required for construction and maintenance, including materials that become necessary for demolition at the end of the building’s life cycle.
After that, we calculated the life cycle cost of a wooden house by the parameter of diesel use. Construction companies often use vehicles not only to deliver building raw materials to an industrial site, but also to ensure the production process itself, up to the stage of final work. According to the results of the LCA program, the global warming potential from the use of the required amount of diesel is (A1-A3) 3.24 kg of CO2 e/l.
The LCA value of the global warming potential resulting from the provision of water supply/wastewater disposal needs is (A1-A3) 0.69 kg CO2 e/m3.
It is worth noting that this method of manufacturing log houses does not require a large amount of water and is mainly about ensuring normal working conditions for the work crew.
According to the results obtained from the LCA program, the value of the global warming potential due to the heat supply needs of production needs is (A1- A3) 0.13 kg CO2 e/kWh.
Table 3 shows the indicators for calculating the carbon footprint for a wooden house at life cycle stages A1-A3.

Table 3. Indicators for calculating the carbon footprint of a wooden house at life cycle stages A1-A3.
The advantages of wooden houses include the fact that the main life cycle costs occur at the construction stage (Figure 2). In other words, from an environmental perspective, wooden houses have a smaller carbon footprint in the long run. In economic terms, maintenance costs are lower than those of brick houses.
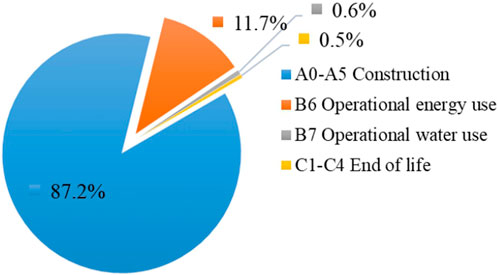
Figure 2. Results of the life cycle cost of a wooden house by discount factor and inflation (where A0-A5, B6, B7, C1-C4 are modules of the life cycle stages).
The data in Figure 3 make it possible to estimate the results of assessing the life cycle cost of a wooden house by the percentage of energy costs. The energy efficiency aspect is relevant today from many perspectives, not only economic, social, and environmental. The Ukrainian real estate market is seeing more and more “healthy” construction projects, which is a trend in European countries. Energy-efficient technologies are designed to reduce energy consumption and carbon dioxide emissions, thereby reducing the negative impact on the environment.
By comparing the One Click LCA data obtained from the cost of building a brick and cement house per 100 m2, it becomes clear not only the economic but also the environmental cost of the construction industry (Figure 4).
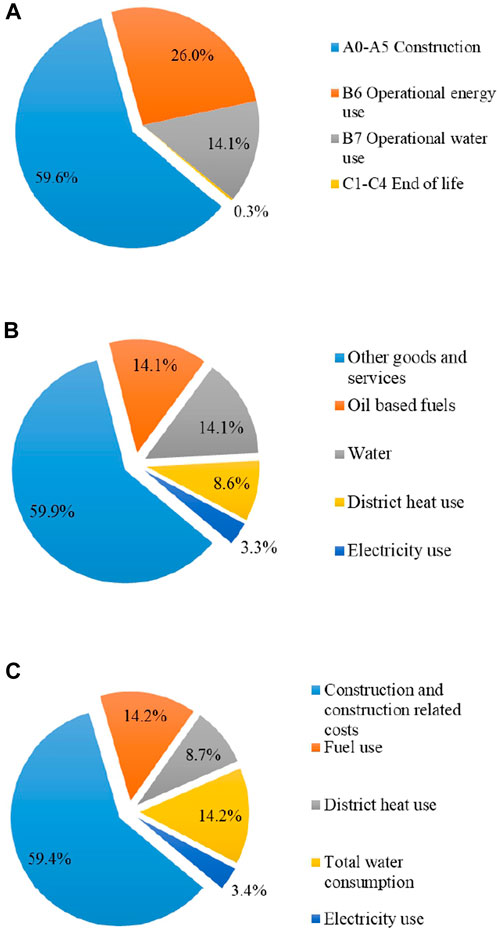
Figure 4. Results of life-cycle cost estimation of a brick and cement house (display of parameters according to the European energy certification scale), by percentage of energy costs (A), discount (B) and inflation coefficient (C).
Thus, having carried out a comparative analysis of the life cycle of two popular and fundamentally different construction methods, the data clearly indicate the construction of log houses in terms of a number of environmental characteristics and carbon footprint parameters.
As can be seen from Figure 5, the main costs of wooden houses are incurred at the construction stage (A0-A5) - 87.2%, i.e., in the long term, wooden houses are more advantageous in terms of economic factor.
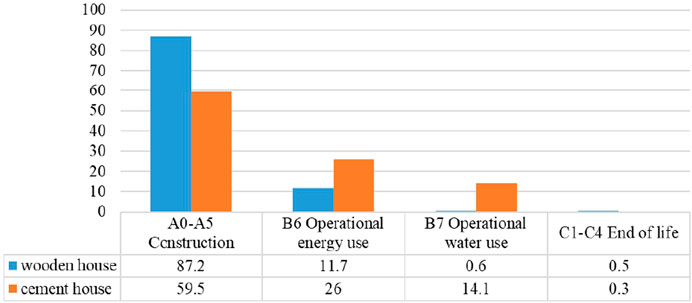
Figure 5. Comparative characteristics of wood and brick houses by life cycle cost by discount factor and inflation.
In our opinion, it is important to compare brick and wood houses by the types of energy resources used during the life cycle. We have compared the houses by the percentage of resources in energy consumption (Figure 6). As can be seen from the comparative analysis, the share of resources that pollute the environment is much higher in brick houses (42.2% in brick houses, 12.3% in wooden houses). That is, brick houses use more fuel, water, heat, and electricity during their life cycle. In addition, it is worth noting that wood is a renewable resource used for the construction of wooden houses. Trees affected by pests or fungal diseases can also be used as raw materials. Treatment with solutions and mechanical cleaning allow the use of raw materials that were previously used exclusively as fuel.
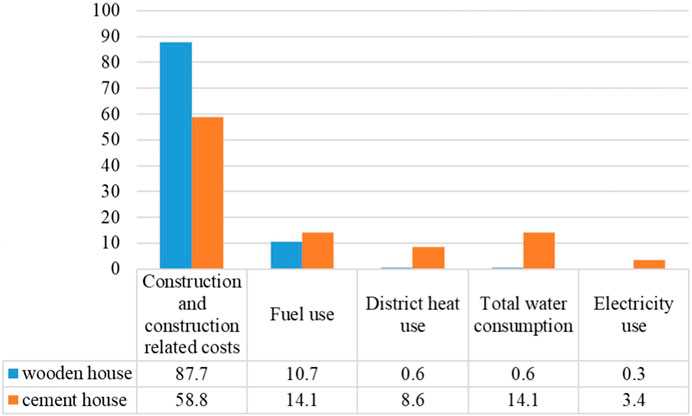
Figure 6. Comparative characteristics of wood and brick houses in terms of life cycle cost by percentage of energy consumption.
The above calculations referred to the study of the life cycle of buildings and opportunities at the design stage. The aspect of studying sanitary conditions is also important. Works (Burdova and Vilcekova, 2021; Budajova et al., 2023) investigate the lifespan of buildings and their environmental and social factors. Three houses were considered as the object of study. They are They are built of aerated concrete blocks (family house 1), wood (family house 2) and prefabricated reinforced concrete (family house 3). The results of the LCA analysis show that a family house made of aerated concrete blocks emits 23.27 kg CO2e/m2/year, a wooden house 8.87 kg CO2e/m2/year, and a ceramic composite house 16.81 kg CO2e/m2/year. We conclude that wood as a building material has a number of advantages, among which the main ones are lower carbon footprint and a smaller share of non-renewable resources in the construction process. It is worth noting that houses built of wood comply with the building codes of Ukraine and Slovakia, and the practical data obtained on the sanitary conditions of living in houses made of wood allow us to assert their safety.
It can be stated that the prospects of the construction industries in Ukraine and Slovakia using wood are the main directions in preserving the environment and transitioning the economy to a low-carbon level. The use of environmentally preferable materials allows to accelerate the pace of housing construction, reduce construction costs, and minimize maintenance costs.
4 Conclusion and prospects for further studies
A building’s total environmental impact is the result of environmental loads that occur over the life cycle of the building: initial impact, annual recurring impact, and deconstructed impact. Initial impacts occur during the design and construction of a building, including project management activities, material use, the construction process, and waste management. Annualized recurring impacts are those that recur every year and result from energy use for heating, lighting, ventilation and cooling, as well as repairs and renovations over long periods of time. The third stage of deconstruction occurs during the demolition of the building and is the final stage.
The Life Cycle Assessment method was used as a methodology for assessing the life cycle. This is an analytical method that allows to assess the potential environmental impact associated with the life cycle of a product. In accordance with the certification standard, there are 17 life cycle stages that reflect the procurement of raw materials, their production, building use, and end of life. The number of stages may vary, but assessments of stages A1-A3 (A1: Extraction and processing of raw materials, processing of incoming secondary materials (e.g., recycling processes; A2: Transportation to the producer; A3: Production of raw materials). This is a holistic methodology that can assess the impact of a product (in our case, a house) on climate change. The methodology is described in more detail in the EN ISO 14040–44 standard.
Using the One Click Life Cycle Assessment and Life Cycle Cost software, the carbon footprint of a log house was calculated for the three life cycle stages (A1-A3) described above. When calculating the carbon footprint of wood-based building materials, we took into account carbon emissions not only from finished products, but also from all other products obtained as a result of logging. When calculating the carbon footprint, greenhouse gas emissions from all types of activities are estimated.
Based on the information obtained, it is possible to draw conclusions about the feasibility of using a wooden log house as a building material, since the amount of carbon footprint is less than that of a brick house. The final part of the paper presents the results of calculating the life cycle cost of a wooden house by discount factor and inflation, the results of the life cycle cost of a wooden house by percentage of energy costs, and the results of assessing the life cycle cost of a wooden house (displaying parameters according to the European energy certification scale).
After that, the analytical data for estimating the life cycle cost of a house built of brick and cement were presented. The diagrams reflect the level of energy efficiency of the house according to the European energy certification scale, the percentage of energy costs, as well as the discount and inflation coefficient. Thus, having carried out a comparative analysis of the life cycle of two popular and fundamentally different construction methods, the data clearly indicate in favor of the construction of log houses in terms of a number of environmental characteristics and carbon footprint parameters.
It was found that the cost of building houses made of wood is 20%–25% less than brick. In addition, significant savings can be made on the development of project documentation, as wooden houses are mostly built according to standard designs. The advantage of wooden houses is that they use renewable raw materials for construction. The share of costs in the structure of the building’s life cycle in stages (B1-B7) related to the use of the building is lower for wooden houses; in stages (C1-C4) related to waste processing, wooden houses are also lower. Based on the carbon footprint assessment (using the Life Cycle Assessment methodology), economic comparison (Life Cycle Cost and total construction costs), and expert evaluation (by technical and ergonomic parameters) of the two construction technologies, the feasibility and possibility of using wood as a building raw material was established.
Data availability statement
The raw data supporting the conclusion of this article will be made available by the authors, without undue reservation.
Ethics statement
Ethical review and approval was not required for the study on human participants in accordance with the local legislation and institutional requirements. Written informed consent from the (patients/ participants or patients/participants legal guardian/next of kin) was not required to participate in this study in accordance with the national legislation and the institutional requirements.
Author contributions
SD: Conceptualization, Data curation, Investigation, Methodology, Project administration, Supervision, Validation, Visualization, Writing–original draft, Writing–review and editing. SV: Conceptualization, Funding acquisition, Project administration, Resources, Software, Writing–review and editing. HM: Formal Analysis, Investigation, Resources, Software, Validation, Visualization, Writing–review and editing. EK: Formal Analysis, Software, Validation, Visualization, Writing–review and editing. AK: Conceptualization, Data curation, Formal Analysis, Methodology, Supervision, Writing–original draft, Writing–review and editing.
Funding
The author(s) declare financial support was received for the research, authorship, and/or publication of this article. This research was funded by Erasmus +, grant number No 2021-1-SK01-KA220-HED-000023274 “Support of higher education system in a context of climate change mitigation through regional-level of carbon footprint caused by a product, building and organization”.
Acknowledgments
The team of authors expressed their gratitude to the reviewers for valuable recommendations that have been taken into account to significantly improve the quality of this paper.
Conflict of interest
The authors declare that the research was conducted in the absence of any commercial or financial relationships that could be construed as a potential conflict of interest.
Publisher’s note
All claims expressed in this article are solely those of the authors and do not necessarily represent those of their affiliated organizations, or those of the publisher, the editors and the reviewers. Any product that may be evaluated in this article, or claim that may be made by its manufacturer, is not guaranteed or endorsed by the publisher.
References
Abdelaal, M. A., Seif, S. M., El-Tafesh, M. M., Bahnas, N., Elserafy, M. M., and Bakhoum, E. S. (2023). Sustainable assessment of concrete structures using BIM–LCA–AHP integrated approach. Environ. Dev. Sustain., 1–20. doi:10.1007/s10668-023-03701-3
Ai Lin Teo, E., and Yean Yng Ling, F. (2006). Developing a model to measure the effectiveness of safety management systems of construction sites. Build. Environ. 41 (11), 1584–1592. doi:10.1016/j.buildenv.2005.06.005
Almroth, A., and Rehnberg, I. (2023) Life cycle assessment of an active safety radar system an environmental assessment based on ISO 14040 and 14044 in conjunction with the GHG protocol.
Arocena, P., Orcos, R., and Zouaghi, F. (2023). The scope of implementation of ISO 14001 by multinational enterprises: the role of liabilities of origin. J. Environ. Manag. 327, 116844. doi:10.1016/j.jenvman.2022.116844
Athibaranan, S., Manasa, K. L., Vamsi, G. R., Madhunandhan, K., Sharmila, K., and Koushik, A. (2023). Life cycle assessment for G+ 4 building using BIM and one Click LCA. J. Constr. Build. Mater. Eng. 29–43. doi:10.1007/s41024-021-00149-8
Bocci, C., and Fortmann, L. (2023). Community and industrial forest concessions: are they effective at reducing forest loss and does FSC certification play a role? World Dev. 170, 106315. doi:10.1016/j.worlddev.2023.106315
Borovska, H., and Khokhlov, V. (2023). Climate data for Odesa, Ukraine in 2021– 2050 based on EURO- CORDEX simulations. Geoscience Data J. 00, 148–159. doi:10.1002/gdj3.197
Brancalion, P. H. S., de Siqueira, L. P., Amazonas, N. T., Rizek, M. B., Mendes, A. F., Santiami, E. L., et al. (2022). Ecosystem restoration job creation potential in Brazil. People Nat. 4 (6), 1426–1434. doi:10.1002/pan3.10370
Brienen, R. J., Phillips, O. L., Feldpausch, T. R., Gloor, E., Baker, T. R., Lloyd, J., et al. (2015). Long-term decline of the Amazon carbon sink. Nature 519 (7543), 344–348. doi:10.1038/nature14283
Budajova, J., Vilcekova, S., Burdova, E. K., Harcarova, K., and Mesaros, P. (2023). Lifespan of wooden houses and analysis of their environmental and social indicators. Pollack Period. 18 (3), 39–45. doi:10.1556/606.2023.00801
Burdova, E. K., and Vilcekova, S. (2021). Assessment of environmental impacts and costs during life cycle stages of selected family houses. IOP Conf. Ser. Earth Environ. Sci. 900 (1), 012017. doi:10.1088/1755-1315/900/1/012017
Camango, C., and Cândido, C. J. (2023). ISO 9001 maintenance, decertification and recertification: a systematic literature review. Total Qual. Manag. Bus. Excell. 34, 1764–1796. doi:10.1080/14783363.2023.2203379
Cherubini, F., Guest, G., and Strømman, A. H. (2012). Application of probability distributions to the modeling of biogenic <scp>CO</scp>2 fluxes in life cycle assessment. GCB Bioenergy 4 (6), 784–798. doi:10.1111/j.1757-1707.2011.01156.x
Churkina, G., Organschi, A., Reyer, C. P. O., Ruff, A., Vinke, K., Liu, Z., et al. (2020). Buildings as a global carbon sink. Nat. Sustain. 3 (4), 269–276. doi:10.1038/s41893-019-0462-4
da Costa, J. P., Silva, A. L., Barcelò, D., Rocha-Santos, T., and Duarte, A. (2023). Threats to sustainability in face of post-pandemic scenarios and the war in Ukraine. Sci. Total Environ. 892, 164509. doi:10.1016/j.scitotenv.2023.164509
Dixon, R. K. (2011). Global Environment Facility investments in the phase-out of ozone-depleting substances. Mitig. Adapt. Strategies Glob. Change 16, 567–584. doi:10.1007/s11027-011-9281-2
Duleba, A. (2022). Differentiated European integration of Ukraine in comparative perspective. East Eur. Polit. Soc. 36 (2), 359–377. doi:10.1177/08883254211005179
Durman, O., Durman, M., Mazievich, T., Zhiliaieva, O., and Arkhypenko, S. (2021). European Union–Ukraine association agreement: challenges and prospects for cooperation. J. East. Eur. Central Asian Res. 8 (3), 349–361. doi:10.15549/jeecar.v8i3.780
Evans, D. (2006). The habitats of the European Union habitats directive. Biology and environment: Proceedings of the royal Irish academy. (China: Royal Irish Academy), 106B(3), 167–173.
Fonseca, L., Domingues, P., Nóvoa, H., Simpson, P., and Sá, J. D. G. (2023). ISO 9001:2015: the view from the conformity assessment community. Total Qual. Manag. Bus. Excell. 34 (5–6), 558–579. doi:10.1080/14783363.2022.2073212
Francis, R. A., Millington, J. D., and Chadwick, M. A. (Editors) (2016). Urban landscape ecology. New York, NY: Routledge: Abingdon.
Garver, G. (2013). The rule of ecological law: the legal complement to degrowth economics. Sustainability 5 (1), 316–337. doi:10.3390/su5010316
Gupta, S., Dharmaraj, T., Reddy, K. M., and Ravisankar, T. (2020). Spatial-temporal analysis and visualization of rural development works implemented under world’s largest social safety programme in India—a case study. J. Geovisualization Spatial Analysis 4 (2), 21. doi:10.1007/s41651-020-00062-7
Hellweg, S., and Milà i Canals, L. (2014). Emerging approaches, challenges and opportunities in life cycle assessment. Science 344 (6188), 1109–1113. doi:10.1126/science.1248361
Holubieva, V., and Akulenko, V. (2020). Correlation of the international and national institutional and legal regulations of applying some aspects of technical barriers in practices of Ukraine and the European Union. Eur. Stud. Rev. Eur. Law, Econ. Polit. 7, 325–359. doi:10.2478/eustu-2022-0060
Jolliet, O., Frischknecht, R., Bare, J., Boulay, A. M., Bulle, C., Fantke, P., et al. (2014). Global guidance on environmental life cycle impact assessment indicators: findings of the scoping phase. Int. J. Life Cycle Assess. 19, 962–967. doi:10.1007/s11367-014-0703-8
Karabin, T., Bilash, O., Fridmanskyy, R., and Tymchak, V. (2021). Local government transfer into the process of Ukraine’s European integration: achievements of communities and losses of the executive branch of power. Lex. Localis. 19 (3), 781–803. doi:10.4335/19.3.781-803(2021)
Khorolskyi, A., Hrinov, V., and Kaliushenko, O. (2019). Network models for searching for optimal economic and environmental strategies for field development. Procedia Environ. Sci. Eng. Manag. 6 (3), 463–471.
Kiforenko, O. (2022). Ukraine-EU trade relations: statistical analysis of the total trade turnover. Int. J. Econ. Bus. Res. 23 (1), 1–18. doi:10.1504/IJEBR.2022.119364
Kirsch, W. (2022). The distribution of power within the EU: perspectives on a Ukrainian accession and a Turkish accession. Int. Econ. Econ. Policy 19 (2), 401–409. doi:10.1007/s10368-022-00541-w
Kittur, B. H., Raj, A., Upadhyay, A. P., Jhariya, M. K., and Banerjee, A. (2023). Eco-Restoration of degraded forest ecosystems for sustainable development. Land Environ. Manag. through For., 273–291. doi:10.1002/9781119910527.ch11
Liu, X., Liu, Y., Li, H., and Wen, D. (2023). Identification and analysis of barriers to the effectiveness of ISO 45001 certification in Chinese certified organisations: a DEMATEL-ISM approach. J. Clean. Prod. 383, 135447. doi:10.1016/j.jclepro.2022.135447
Marcinek, K., Balagna, J., and Holynska, K. (2023) The trade-offs of Ukraine's recovery: fighting for the future.
Napathorn, C. (2022). The development of green skills across firms in the institutional context of Thailand. Asia-Pacific J. Bus. Adm. 14 (4), 539–572. doi:10.1108/APJBA-10-2020-0370
N Doua, B. D. (2023). The impact of forest management certification on exports in the wood sector: evidence from French firm-level data. J. Clean. Prod. 418, 138032. doi:10.1016/j.jclepro.2023.138032
Petlovanyi, M., Sai, K., Malashkevych, D., Popovych, V., and Khorolskyi, A. (2023). Influence of waste rock dump placement on the geomechanical state of underground mine workings. IOP Conf. Ser. Earth Environ. Sci. 1156 (1), 012007. doi:10.1088/1755-1315/1156/1/012007
Petrović, S. N., Larsen, M. A. D., Colangelo, A., Diachuk, O., Podolets, R., Semeniuk, A., et al. (2023). Effect of localisation and climate change on operation of data centres in Ukraine towards 2050. Energy Strategy Rev. 45, 101049. doi:10.1016/j.esr.2022.101049
Philippopoulos-Mihalopoulos, A., and Andreas, (2011a). Towards critical environmental law. In law and ecology: Law and ecology: new environmental foundations (Routledge/Glasshouse: Abingdom).
Rana, R. L., Bux, C., and Lombardi, M. (2023). Carbon footprint of the globe artichoke supply chain in Southern Italy: from agricultural production to industrial processing. J. Clean. Prod. 391, 136240. doi:10.1016/j.jclepro.2023.136240
Ross, E., Day, M., Ivanova, C., McLeod, A., and Lockshin, J. (2022). Intersections of disadvantaged communities and renewable energy potential: data set and analysis to inform equitable investment prioritization in the United States. Renew. Energy Focus 41, 1–14. doi:10.1016/j.ref.2022.02.002
Spagnoli, L. (2022). Supporting the “sustainability” of inland (rural) areas in Basilicata. Tools and place-based strategies for implementing local development processes in the Lagonegrese-Pollino subregion. BELGEO 4. doi:10.4000/belgeo.57141
Sun, K., Sun, R., Li, Y., Ji, H., Jia, B., and Xu, Z. (2023). Plant economic strategies in two contrasting forests. BMC Plant Biol. 23 (1), 366. doi:10.1186/s12870-023-04375-9
Synetska, Y., and Hupková, D. (2023). Effects of climate change on the dynamics of crops yield—case of Ukraine. Univers. J. Agric. Res. 11 (1), 32–45. doi:10.13189/ujar.2023.110104
Timoshuk, V., Tishkov, V., Inkin, O., and Sherstiuk, E. (2012). Influence of coal layers gasification on bearing rocks. Geomechanical Process. Dur. Undergr. Mining–Proceedings Sch. Undergr. Min., 109–113.
Turner, K. G., Anderson, S., Gonzales-Chang, M., Costanza, R., Courville, S., Dalgaard, T., et al. (2016). A review of methods, data, and models to assess changes in the value of ecosystem services from land degradation and restoration. Ecol. Model. 319, 190–207. doi:10.1016/j.ecolmodel.2015.07.017
van den Bergh, J. C. J. M., and Grazi, F. (2015). Reply to the first systematic response by the Global Footprint Network to criticism: a real debate finally? Ecol. Indic. 58, 458–463. doi:10.1016/j.ecolind.2015.05.007
Vilčeková, S., Harčárová, K., Moňoková, A., and Burdová, E. K. (2020). Life cycle assessment and indoor environmental quality of wooden family houses. Sustainability 12 (24), 10557. doi:10.3390/su122410557
Xiao, Y., Ma, D., Zhang, F., Zhao, N., Wang, L., Guo, Z., et al. (2023). Spatiotemporal differentiation of carbon emission efficiency and influencing factors: from the perspective of 136 countries. Sci. Total Environ. 879, 163032. doi:10.1016/j.scitotenv.2023.163032
Zhabotynska, S., and Velivchenko, V. (2019). New media and strategic narratives: the Dutch referendum on Ukraine–EU Association Agreement in Ukrainian and Russian Internet blogs. Eur. Secur. 28 (3), 360–381. doi:10.1080/09662839.2019.1648253
Keywords: eco-labelling, environmentally friendly building materials, environmental safety, LCA, wood
Citation: Delehan S, Vilčeková S, Melehanych H, Krídlová Burdová E and Khorolskyi A (2024) A comparative assessment of the capabilities and success of the wood construction industry in Slovakia and Ukraine based on life cycle assessment certification standards. Front. Environ. Sci. 12:1319823. doi: 10.3389/fenvs.2024.1319823
Received: 11 October 2023; Accepted: 30 April 2024;
Published: 05 June 2024.
Edited by:
Vojtech Vaclavik, VSB-Technical University of Ostrava, CzechiaReviewed by:
Agata Matarazzo, University of Catania, ItalySérgio António Neves Lousada, University of Madeira, Portugal
Copyright © 2024 Delehan, Vilčeková, Melehanych, Krídlová Burdová and Khorolskyi. This is an open-access article distributed under the terms of the Creative Commons Attribution License (CC BY). The use, distribution or reproduction in other forums is permitted, provided the original author(s) and the copyright owner(s) are credited and that the original publication in this journal is cited, in accordance with accepted academic practice. No use, distribution or reproduction is permitted which does not comply with these terms.
*Correspondence: Svitlana Delehan, svitlana.delehan-kokaiko@uzhnu.edu.ua