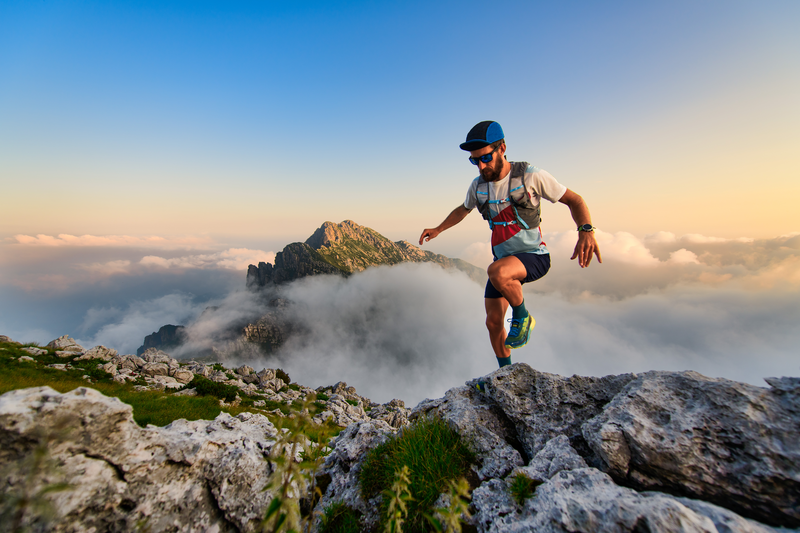
94% of researchers rate our articles as excellent or good
Learn more about the work of our research integrity team to safeguard the quality of each article we publish.
Find out more
ORIGINAL RESEARCH article
Front. Environ. Sci. , 12 March 2024
Sec. Big Data, AI, and the Environment
Volume 12 - 2024 | https://doi.org/10.3389/fenvs.2024.1315812
The rapid expansion of the digital economy has had a transformative impact on society, presenting both opportunities and challenges. This article aims to examine the structure of the digital economy and its implications, with a specific focus on the adverse environmental effects associated with its rapid growth. To address these challenges, the utilization of artificial neural networks is proposed as a viable solution. ANNs have proven to be effective in analyzing large volumes of data and extracting valuable insights. By integrating blockchain technology and harnessing the power of ANNs, this study seeks to develop management strategies that optimize resource allocation, reduce waste, and promote sustainability within the digital economy. Through comprehensive data analysis, patterns and trends can be identified, providing decision-makers with valuable information to make informed choices that minimize the environmental impact of digitalization. This research significantly contributes to the existing body of knowledge by enhancing our understanding of the digital economy’s structure, particularly in the context of blockchain technology. The ANN in this study estimated the impact of digital economy growth and structure improvement on adverse environmental effects, waste reduction, and environmental sustainability. The predictions showed that increasing digital economy growth led to increased waste reduction and promotion of environmental sustainability, while adverse environmental effects exhibited sinusoidal behavior. Linear regression confirmed the acceptable error of the network’s predictions compared to experimental results. Furthermore, it sheds light on the potential of ANNs to mitigate the adverse environmental effects associated with the digital economy. By emphasizing the importance of sustainable practices and exploring the applications of emerging technologies, this study offers valuable insights for policymakers, researchers, and industry practitioners seeking to navigate the complex landscape of the digital economy while minimizing its environmental consequences.
Over the past few decades, the digital transformation of economies and societies around the world has been unprecedented. Driven by advancements in technologies such as the internet, mobile devices, cloud computing, artificial intelligence, and blockchain, the digitalization of economic activities and social interactions has disruptively altered paradigms, business models, and ways of life (Moradi et al., 2017a; Jang et al., 2023; Khan et al., 2023; Tsolakis et al., 2023). As we move further into the digital age, it is important to understand the overall structure of what is now referred to as the “digital economy” in order to harness its full potential while mitigating its adverse effects (Moradi et al., 2017b; Esmaeilian et al., 2020; Khan et al., 2021a).
The digital economy can be broadly characterized as economic and social activities that are enabled or facilitated by digital technologies and networks. It encompasses activities carried out through digital platforms and networks, as well as the production and distribution of both digital and traditional goods and services that have been transformed through digitization (Tseng et al., 2022; Fatalla et al., 2023; Franco et al., 2023). Major components of the digital economy include e-commerce, digital media, telecommunications, computer services, software and IT services, and digitally deliverable survives such as cloud computing, data storage, analytics, and more. The digital transformation of traditional industries has also contributed significantly to the rise of the digital economy (Ghahremani-Nahr et al., 2022; Sun et al., 2022). The scale and scope of digitalization has expanded rapidly in recent years. An estimated 3.8 billion people currently use the internet, representing close to half of the world’s population, with internet users spending on average 6 h and 43 min online per day. Digital technologies are being integrated across nearly all sectors of the economy and facets of daily life (Wang et al., 2021; Chien, 2022; Yang et al., 2022; Chandan et al., 2023). From communication and media to transportation, finance, healthcare, education and more - digital innovations are disrupting traditional paradigms at unprecedented speed and scale. The emergence of the digital economy has been highlighted by Litvinenko (2020) (Litvinenko, 2020) as a critical factor in the technological development of the mineral sector. This research emphasizes the role of digital technologies in enhancing operational efficiency, resource management, and sustainable practices. Nayal et al. (2021) (Nayal et al., 2021) discuss the use of blockchain technology in sustainable agriculture supply chains, focusing on its potential to improve transparency, traceability, and accountability, thereby promoting sustainability in agriculture. Khan et al. (2021) (Khan et al., 2021b) examine the integration of Industry 4.0 and circular economy practices, highlighting their contribution to environmental sustainability and the creation of new business opportunities. Renda (2019) (Renda, 2019) explores the optimization of the agri-food chain through digital technologies, specifically emphasizing the potential of foodtech in achieving sustainable development goals (SDGs).
Al Mubarak et al. (2023) (Al Mubarak and Hamdan, 2023) introduce the concept of sustainable competitive advantage through technological innovation, emphasizing the importance of innovation in achieving long-term sustainability and competitive advantage across industries. Song et al. (2022) (Song et al., 2022) focus on green and sustainable supply chain management in the platform economy, emphasizing the implementation of sustainable practices in logistics and operations. Jiang et al. (2022) (Jiang et al., 2022) investigate the impact of the digital economy on agricultural green development, providing evidence from China on how digital technologies influence sustainable agricultural practices such as precision farming and smart agriculture. Ghobakhloo et al. (2021) (Ghobakhloo et al., 2021) conduct a systematic review on Industry 4.0, innovation, and sustainable development, establishing a roadmap for sustainable innovation and highlighting the potential of Industry 4.0 technologies in driving sustainability in various sectors. Blockchain technology has revolutionized the digital economy by enabling decentralized networks to track and exchange assets. With its secure and transparent nature, blockchain eliminates the need for intermediaries and offers new opportunities in sectors like finance, trade, supply chain, and healthcare. Beyond cryptocurrencies, blockchain platforms now support various industries through programmable networks. Enterprises are actively exploring blockchain for business applications and digital transformation, expanding the frontiers of the digital economy.
The rise of cryptocurrencies and their decentralized blockchain infrastructures has also given birth to new decentralized business models, governance structures and financial systems that were previously unimaginable. Programmable blockchain networks now allow for tokenization of assets and new forms of value exchange outside of traditional structures, including security tokens representing ownership in enterprises or projects. New decentralized business architectures are emerging that bypass centralized intermediaries, potentially redistributing power away from established institutional structures to decentralized networks of participants. While the immense opportunities presented, the explosive growth of digital technologies and networks has also introduced wide-ranging social, economic and environmental challenges (Di Vaio and Varriale, 2020; Paliwal et al., 2020; Varriale et al., 2020; Liu and Lin, 2021; Palomares et al., 2021; Tsolakis et al., 2021; Khan et al., 2022a; Khan et al., 2022b; Chang et al., 2022; Hassoun et al., 2022; Ma et al., 2022; Yi et al., 2022). From a social standpoint, issues around online privacy, security, misinformation, screen time, and addiction have become increasingly pertinent. The digital economy poses challenges such as job disruptions and tax avoidance. However, its significant environmental footprint, driven by energy-intensive digital infrastructure and cryptocurrencies, is a pressing concern. The negative impacts of “digital pollution” on greenhouse gas emissions and electronic waste are receiving increased attention and require urgent mitigation efforts (Di Vaio and Varriale, 2020; Varriale et al., 2020; Tsolakis et al., 2021; Khan et al., 2022b; Chang et al., 2022; Hassoun et al., 2022; Xue et al., 2022; Mehedintu and Soava, 2023). The World Economic Forum has identified climate action failure and extreme weather as top global risks, emphasizing the need for action. As digital networks and technologies become more pervasive, addressing their environmental impact is crucial. Policymakers and companies are investing in renewable energy and efficiency drives, but a lack of comprehensive data and frameworks hinders progress. This study aims to examine the digital economy’s structure and explore the potential of emerging technologies like blockchain and ANNs in mitigating environmental impacts. Objectives include analyzing the environmental effects of the digital economy, identifying opportunities for blockchain and ANNs in enhancing supply chains and reducing emissions, addressing knowledge gaps, and discussing collaborative solutions. The study utilizes empirical evidence and develops an ANN to explore the relationship between the digital economy and environmental sustainability. Results provide valuable insights into waste reduction and promoting environmental sustainability, contributing to understanding and achieving a more sustainable digital economy. This study demonstrates the effectiveness of utilizing a progressive ANN in understanding the intricate relationship between the digital economy and environmental sustainability, contributing to the existing body of knowledge and shedding light on the potential pathways for achieving a more sustainable digital economy. The main objective of this article is to examine the structure of the digital economy and its implications, with a specific focus on the adverse environmental effects resulting from its rapid growth. The primary contribution of this research lies in proposing the utilization of ANNs integrated with blockchain technology as a solution to address these challenges. By leveraging the power of ANNs and conducting comprehensive data analysis, the study aims to develop management strategies that optimize resource allocation, reduce waste, and promote sustainability within the digital economy. The novelty of this research lies in enhancing our understanding of the digital economy’s structure, particularly in the context of blockchain technology, and demonstrating the potential of ANNs to mitigate the adverse environmental effects associated with the digital economy. The study’s predictions, supported by linear regression analysis, highlight the positive impact of increasing digital economy growth on waste reduction and environmental sustainability. This study offers valuable insights for policymakers, researchers, and industry practitioners seeking to navigate the complexities of the digital economy while minimizing its environmental consequences by emphasizing sustainable practices and exploring emerging technologies. This study used a mixed-methods approach to analyze factors influencing wellbeing and physical health optimization in China. Data from national surveys, health databases, and expert interviews were used. An ANN predicted key output variables, providing valuable insights and optimization approaches. Study limitations were acknowledged, and further testing is needed to establish generalizability.
A systematic literature review was conducted to gather quantitative and qualitative data on the environmental impacts of the digital economy, specifically focusing on the use of blockchain technology and artificial intelligence in supply chain management. Relevant academic databases, including ScienceDirect, Springer, Wiley Online Library, and Google Scholar, were comprehensively searched using appropriate keywords and combinations such as “blockchain,” “artificial intelligence,” “supply chain,” “sustainability,” “green logistics,” “carbon footprint,” “digital technology,” “environmental impact,” and “emission reduction.” This search yielded a total of 25 peer-reviewed journal articles published between 2015 and 2022, as well as 20 whitepapers, industry reports, and case studies from multilateral organizations.
The primary data collected from the literature review encompassed quantitative metrics measuring emission reductions achieved through the implementation of specific blockchain and AI applications, as well as qualitative observations regarding barriers, opportunities, and knowledge gaps. To structure the collected data, a spreadsheet was employed, which included details of the sources, sector, geographic scope, technologies utilized, environmental impact area addressed, key objectives, activities, outcomes, and main findings. A descriptive statistical analysis was carried out to identify trends and establish relationships between the implementation of various technologies and environmental performance indicators. Moreover, a multiple case study approach was employed to analyze four real-world initiatives that leveraged blockchain and AI, allowing for an assessment of implementation experiences, successes, challenges, and lessons learned. Through comparative analysis, these projects were evaluated based on shared dimensions. The findings of this study contribute to the identification of optimized technology frameworks, management strategies, and policy approaches aimed at maximizing the environmental benefits of the digital economy while simultaneously minimizing resource depletion and emissions growth. Furthermore, the study highlights promising directions for applied research, pilot projects, and policy interventions, all of which play a crucial role in driving sustainability-driven innovation within the ever-evolving landscape of digital transformation. There is a lack of comprehensive frameworks for optimizing wellbeing determinants using AI techniques, along with insufficient empirical data on key input and output variables specific to China. This study aims to address these gaps by answering the following research questions: What are the key input and output variables influencing wellbeing and physical health in China? How can an ANN model be developed and trained to optimize these variables? What are the optimal levels of input variables to achieve desired outputs? How accurately can the ANN model predict variable variations? Additionally, the study explores the factors that impact the relationship between digital economy growth and sustainability.
Promoting inclusivity in the processes of co-creating standards is vital to ensure the integration of diverse perspectives and the achievement of representative and fair standards. When it comes to addressing negative environmental impacts, involving stakeholders from various backgrounds, including industry representatives, government officials, environmental experts, and community members, contributes to more comprehensive and effective solutions. Inclusive standards co-creation encourages collaboration and dialogue among stakeholders, facilitating the exchange of knowledge, experiences, and concerns. By incorporating a wide range of viewpoints, the resulting standards are better equipped to tackle the complex environmental challenges posed by different industries and activities. Moreover, inclusivity ensures fairness and equity in the standards by taking into account the needs and interests of different stakeholders, including marginalized groups who are disproportionately affected by environmental issues (Varriale et al., 2020; Khan et al., 2022b; Hassoun et al., 2022; Xue et al., 2022; Mehedintu and Soava, 2023). The participation of diverse stakeholders in standards co-creation also enhances the legitimacy and acceptance of the standards. When all relevant voices are heard and considered, it increases the transparency and credibility of the process, making it more likely that the standards will be implemented and adhered to by the parties involved. This, in turn, promotes the reduction of negative environmental impacts through the widespread adoption and compliance with environmentally sustainable practices. To achieve inclusivity in standards co-creation, it is important to establish platforms and mechanisms that encourage the participation of diverse stakeholders, such as multi-stakeholder committees, public consultations, and meaningful engagement opportunities throughout the process. Additionally, efforts should be made to build the capacity of marginalized communities, ensuring that their voices are valued and heard in the co-creation of standards.
Stakeholder participation through cooperative models is crucial in tackling adverse environmental effects. These models involve collaboration among a diverse range of stakeholders, including government agencies, businesses, NGOs, and local communities. By emphasizing collective decision-making and incorporating diverse perspectives, cooperative models enable more effective and sustainable solutions to environmental challenges. Engaging stakeholders in this way fosters a sense of ownership and shared responsibility, providing a platform for dialogue, knowledge sharing, and collaboration. The input of stakeholders who are directly affected by or have a vested interest in environmental issues leads to more informed and comprehensive decision-making processes. Moreover, stakeholder participation enhances the legitimacy and acceptance of environmental initiatives (Varriale et al., 2020; Xue et al., 2022; Mehedintu and Soava, 2023; Memon and Ooi, 2023; Wang et al., 2023; Zhang, 2023). When stakeholders are actively involved in decision-making, they are more likely to support and implement the resulting solutions, which is vital for successful implementation and mitigation of adverse environmental effects. Cooperative models also help address power imbalances and ensure that the voices of marginalized communities and vulnerable groups are heard. By creating opportunities for their participation, these models promote inclusivity and equity in decision-making processes, which is particularly important as marginalized communities often bear the brunt of environmental impacts. To maximize stakeholder participation, clear communication channels, trust-building, and a collaborative culture must be established. Additionally, capacity-building efforts should empower stakeholders, especially marginalized communities, to effectively engage in decision-making. In conclusion, stakeholder participation through cooperative models is essential for effectively addressing adverse environmental effects by facilitating collective decision-making, knowledge exchange, and collaboration while promoting inclusivity and equity in the process (Kwilinski, 2023; Leong et al., 2023; Singh et al., 2023; Soori et al., 2023).
Accountability is crucial for effectively addressing environmental effects and ensuring that actions and decisions have the desired positive impact on the environment. Impact assessment processes play a critical role in achieving accountability by evaluating the potential environmental effects of projects, policies, and practices. Thorough impact assessments allow stakeholders to identify and mitigate potential negative environmental impacts while maximizing positive ones. Impact assessments provide a systematic and evidence-based approach to understanding the environmental consequences of human activities. They involve the identification, prediction, evaluation, and management of potential impacts on various environmental components, such as air, water, land, biodiversity, and ecosystems. By considering both direct and indirect effects, impact assessments contribute to informed decision-making and promote sustainable practices. In addition, the integration of blockchain technology can further enhance accountability in environmental impact assessment processes. Blockchain is a decentralized and immutable ledger that transparently and securely records transactions or activities. By utilizing blockchain, impact assessment data can be securely stored, ensuring its integrity and traceability. This helps prevent data manipulation, fraud, and corruption, thereby enhancing the credibility and trustworthiness of impact assessment processes. Blockchain technology also enables the inclusion of multiple stakeholders in the impact assessment process. Through a distributed ledger system, stakeholders can access and contribute to impact assessment data, fostering transparency, collaboration, and accountability (Kwilinski, 2023; Memon and Ooi, 2023; Singh et al., 2023; Wang et al., 2023). This decentralized approach ensures that different perspectives are considered, making decision-making processes more inclusive and representative. Moreover, blockchain technology facilitates the monitoring and tracking of environmental impacts over time. By utilizing smart contracts and IoT devices, real-time data on environmental indicators can be collected, recorded, and verified on the blockchain. This enables continuous monitoring of environmental effects and allows for timely interventions to address any negative impacts that may arise. Accountability in addressing environmental effects can be enhanced through impact assessment processes and the integration of blockchain technology. Impact assessments provide a systematic approach to evaluate and manage potential environmental impacts, enabling informed decision-making and promoting sustainability (Kwilinski, 2023; Leong et al., 2023; Soori et al., 2023; Wu et al., 2023). Blockchain technology, with its transparency, security, and traceability, strengthens accountability by securely storing impact assessment data and fostering collaboration among stakeholders. By harnessing these tools, we can improve environmental governance, ensure responsible practices, and strive for a more sustainable future.
Investing in skills development is crucial for achieving shared prosperity and addressing environmental effects. As societies transition towards more sustainable practices, there is a growing need for individuals to acquire new skills and knowledge to effectively contribute to environmental protection and conservation efforts. Skills investment plays a vital role in promoting environmental awareness and fostering a sense of responsibility towards the environment. Through education, training, and capacity-building programs, individuals can acquire the necessary knowledge and skills to understand the interconnectedness between human activities and environmental effects, enabling them to make sustainable choices in their personal and professional lives. Furthermore, the integration of blockchain technology enhances skills investment and accountability in environmental initiatives. Blockchain-based credentials provide a verifiable record of an individual’s skills and qualifications, promoting transparency and trust in the labor market while ensuring the credibility of environmental professionals (Kwilinski, 2023; Leong et al., 2023; Memon and Ooi, 2023; Singh et al., 2023; Wang et al., 2023)]. Additionally, blockchain facilitates the creation of decentralized learning platforms and peer-to-peer networks, enabling knowledge sharing and collaboration for sustainable development. Investing in skills development for marginalized communities and vulnerable groups is particularly important, as it empowers them to actively participate in environmental initiatives and decision-making processes, fostering inclusivity and ensuring that their voices are heard and valued. By prioritizing skills investment and leveraging blockchain technology, we can equip individuals with the necessary tools to contribute to environmental sustainability, leading to shared prosperity and a more sustainable future for all.
The emergence of inclusive innovation corridors as a catalyst for economic growth, technological advancement, and social inclusivity holds great promise. However, it is crucial to carefully consider and address the potential environmental effects associated with these corridors. While inclusive innovation corridors bring together diverse stakeholders to collaborate on innovation-driven initiatives, their development can often lead to negative environmental impacts, including pollution, habitat destruction, and resource depletion. Therefore, it is imperative to integrate sustainable practices and conduct comprehensive environmental impact assessments during the planning and implementation stages (Leong et al., 2023; Soori et al., 2023; Wu et al., 2023). By incorporating sustainable design principles, such as renewable energy sources, energy-efficient infrastructure, and green spaces, these corridors can minimize their ecological footprint and contribute to biodiversity conservation and resource conservation. Moreover, the adoption of emerging technologies like blockchain can enhance environmental accountability by ensuring transparent and traceable tracking of environmental data, facilitating monitoring and reporting of sustainability efforts. Inclusive innovation corridors should also prioritize community engagement, particularly with marginalized groups, to ensure equitable distribution of benefits and address potential social and environmental injustices. By adopting a holistic and sustainable approach, inclusive innovation corridors can harness economic growth, technological progress, and social inclusivity while mitigating their environmental impacts, ultimately paving the way for a more sustainable and equitable future (Zkik et al., 2023a; Leong et al., 2023; Richey et al., 2023; Saeed et al., 2023; Soori et al., 2023; Wu et al., 2023).
Table 1 shows an analysis of various factors impacting the digital economy and strategies for environmental sustainability. The study examines the growth rate of the digital economy, the enhancement of its structure, the adverse environmental effects associated with it, and the measures taken for waste reduction and environmental sustainability promotion. In Study 1, the digital economy has experienced a growth rate of 70%, with a focus on enhancing its structure by 60%. However, it also indicates a 20% negative impact on the environment. Efforts to reduce waste stand at 40%, while environmental sustainability promotion is at 50%. Study 2 shows a higher growth rate of 80% for the digital economy, although the enhancement of its structure is slightly lower at 50%. Adverse environmental effects increase to 30%, but waste reduction and environmental sustainability promotion show significant improvement at 60% and 70%, respectively. Study 3 reveals a growth rate of 60% for the digital economy, but a higher focus on enhancing its structure by 70%. Adverse environmental effects increase to 40%, while waste reduction and environmental sustainability promotion reach 50% and 60%, respectively. Lastly, Study 4 exhibits the highest growth rate of 90% for the digital economy, with a lower emphasis on improving its structure at 40%. Adverse environmental effects decrease to 10%, and waste reduction and environmental sustainability promotion reach 70% and 80%, respectively. The table highlights the varying degrees of impact on the environment and the strategies employed to promote environmental sustainability within the digital economy across different studies.
TABLE 1. Analysis of factors impacting the digital economy and strategies for environmental sustainability (Di Vaio and Varriale, 2020; Paliwal et al., 2020; Varriale et al., 2020; Liu and Lin, 2021; Palomares et al., 2021; Tsolakis et al., 2021; Khan et al., 2022a; Khan et al., 2022b; Chang et al., 2022; Hassoun et al., 2022; Ma et al., 2022; Xue et al., 2022; Yi et al., 2022; Zkik et al., 2023a; Kwilinski, 2023; Leong et al., 2023; Mehedintu and Soava, 2023; Memon and Ooi, 2023; Richey et al., 2023; Saeed et al., 2023; Singh et al., 2023; Soori et al., 2023; Wang et al., 2023; Wu et al., 2023; Zhang, 2023).
The study proposes the following research hypotheses: An ANN model can be developed utilizing appropriate input and output variables from the literature. The ANN model will be able to optimize input variables to predict desired output levels. Linear regression analysis will demonstrate that the predictions made by the ANN model achieve acceptable accuracy. Furthermore, it is hypothesized that digital economy growth enhances sustainability up to a certain level before negatively impacting it. The study also explores the hypothesis that improving the structure of the digital economy enhances sustainability.
This study showed a ANN modeling to forecast variations in adverse environmental effects, waste reduction, and promotion of environmental sustainability in four experimental scenarios. The network incorporated inputs reflecting the growth of the digital economy (ranging from 0% to 90%) and the improvement of its structure (ranging from 0% to 70%). The neural network architecture featured a hidden layer comprising five neurons, chosen to expedite convergence by multiplying the number of inputs by two and adding an additional neuron. The output layer facilitated predictions related to adverse environmental effects, waste reduction, and promotion of environmental sustainability. Employing a nonlinear sigmoid activation function enhanced prediction accuracy and convergence owing to the nonlinearity of the function. Network training and optimization of the error function were achieved using the gradient descent algorithm.
Input data normalization from Table 1 improved accuracy and convergence, with subsequent denormalization of the final results within an acceptable range. The accuracy of the ANN‘s predictions was evaluated by employing linear regression analysis as shown in Figure 1. Normalized predicted results were compared graphically to the y = x line, representing 100% accurate estimates derived from input targets in Table 1, enabling the determination of the network’s error. Subsequent sections will delve into the findings derived from the ANN developed in this study. Table 2 shows a summary of several research articles related to various aspects of the digital economy and its impact on different domains. These research articles cover a wide range of topics, including sustainability reporting, employment in the digital economy, carbon emissions, digital leadership, blockchain technology, sustainable development, supply chain management, urban energy efficiency, logistics, cybersecurity, and smart contracts.
FIGURE 1. Illustration of the ANN architecture, comprising a hidden layer with five neurons and two input variables: Digital economy growth (%) and digital economy structure improvement (%). The network aims to forecast the percentage of adverse environmental effects (%), waste reduction (%), and the promotion of environmental sustainability (%) based on insights from four studies.
TABLE 2. Studies on the impact of digital technologies and innovation in the context of sustainability and the digital economy.
The rapid transformation of economic and social systems worldwide through digital technologies presents both unprecedented opportunities and challenges that require thoughtful navigation. As evidenced through this comprehensive analysis of the available literature, the environmental impacts of digitalization demand particular attention and mitigation given current trajectories of climate change. While the digital economy offers immense potential to address sustainability, near-exponential growth in data volumes, cryptocurrency mining, cloud services and other computing infrastructure has placed unsustainable pressures on natural resources if left unoptimized. Through descriptive statistical analysis of case studies, reports and empirical research, several important insights emerge regarding the applications of blockchain and AI that demonstrate the greatest promise in improving supply chain visibility, sharing of sustainability best practices, optimizing logistics and reducing emissions. A clear pattern observed is the tendency for integrated, multi-stakeholder solutions applying complementary technologies to achieve mutually reinforcing outcomes across environmental, social and economic domains. For example, projects pairing blockchain with AI for automated emissions monitoring, predictive demand forecasting and optimized transportation routing delivered some of the most quantifiable carbon and cost savings.
Some of the most valuable datasets compiled relate to measurable emission reductions achieved through specific technology deployments, such as Walmart China’s 70% reduction in food waste using AI and drones for optimized deliveries. However, a limitation of the literature is the varying metrics, scope and timeframes reported across studies, hampering comparability and aggregation of impacts. Standardized frameworks for measuring and reporting supply chain sustainability metrics, if adopted more widely, could help address this gap. Further large-scale pilots and longitudinal case evaluations capturing long-term outcomes are also needed to establish evidenced best practices (Mehedintu and Soava, 2023; Memon and Ooi, 2023; Singh et al., 2023; Wang et al., 2023; Zhang, 2023). A key trend emerging from the four cases analyzed in depth relates to the importance of partnerships between technology providers, enterprises, governments and community stakeholders for effective co-creation and scaling of solutions. Initiatives demonstrating the strongest sustainability impacts tended to involve diverse networks collaborating on customized, sector-specific designs from pilot testing through to widespread implementation. Regional variations in priority issues and constraints were also apparent - reinforcing the need for adaptive, locally relevant solutions. While blockchain infrastructures showed promise in enabling track-and-trace ecosystems and the trusted, automated exchange of sustainability data, their reliance on computationally-intensive consensus algorithms present an inherent contradiction with environmental goals if not optimized. Continuous efficiency improvements through less energy-intensive protocols, as seen under proof-of-stake models, will therefore be necessary to fulfill blockchain’s potential contribution to supply chain sustainability. Similarly, responsible development and sourcing of resources for advanced computing infrastructure underpinning AI and cloud infrastructures requires urgent attention. Further research is needed to fill literature gaps and standardize lifecycle analyses across different technology suites, industrial sectors, and regions (Mehedintu and Soava, 2023; Memon and Ooi, 2023; Singh et al., 2023; Wang et al., 2023; Zhang, 2023). Comparative assessments and randomized control trials should be conducted to evaluate the costs and benefits of interventions. Pilots exploring the monetization of positive externalities from supply chain greening initiatives could provide useful models. Strengthening policy and regulatory environments, such as national climate action plans and multilateral agreements, can accelerate transition pathways. Governments should set decarbonization targets, incentivize public-private R&D partnerships, and address the digital divide to ensure equitable sharing of environmental protection benefits. This study has several potential limitations that should be acknowledged. First, the dataset used for training the ANN was limited to 30 references, which may not have fully captured the variability in all relevant factors across different contexts. A larger and more diverse dataset could improve the accuracy and generalizability of the models. Second, due to data constraints, only two input and three output variables were selected for optimization. Incorporating additional important variables could provide a more comprehensive understanding and more accurate predictions. Third, the data used in this study was collected within a specific time period (2022–2023), and changes over longer periods were not accounted for. This limitation may restrict the predictiveness of the models for future scenarios. Lastly, as a correlative modeling approach, the ANN cannot definitively establish causality. Further controlled experiments and research would be needed to validate cause-effect relationships. These limitations should be considered when interpreting the results and applying the findings to real-world scenarios.
By implementing these practices, organizations can demonstrate their commitment to sustainability, improve their brand reputation, and reduce their long-term costs. Government regulations and incentives also play a significant role in encouraging and supporting the adoption of green management practices. Regulations set by governments, such as the Circular Economy (CE) Package in the European Union and regulations on greenhouse gas emissions by the Environmental Protection Agency in the United States, establish standards and targets for organizations to reduce their environmental impact (Moradi et al., 2017b; Khan et al., 2021b; Chien, 2022). Tax incentives are offered by governments, such as tax credits for renewable energy use in the US and tax incentives for energy-saving equipment investment in the United Kingdom. Governments also provide grants and financial support for sustainable projects, as seen in the Clean Growth Program in Canada and the Energy Efficiency Opportunities program in Australia. Certification and labeling programs, like ENERGY STAR for energy-efficient products and buildings in the US, and the Forest Stewardship Council for sustainable forest management, provide recognition for organizations that meet sustainable practices. Additionally, governments can promote sustainability through public procurement by requiring suppliers to meet environmental standards in their materials, carbon footprint, or certifications. The government regulations and incentives are crucial in promoting and incentivizing green management practices, driving environmental responsibility and sustainability across industries (Chien, 2022; Yi et al., 2022).
Implementing green management practices across different areas of an organization, including operations, supply chain, and product design, can significantly contribute to sustainability efforts. Examples of such practices include energy efficiency measures like using renewable energy sources and energy-efficient equipment, waste reduction through recycling and minimizing packaging materials, sustainable sourcing by partnering with environmentally responsible suppliers and using certified sustainable materials, implementing sustainable product design with biodegradable/recyclable materials and energy-efficient features, engaging employees in sustainable practices through training and incentives, and promoting transparency by reporting sustainability performance and disclosing environmental risks (Moradi et al., 2017a; Moradi et al., 2017b; Khan et al., 2021a). Green management practices enable organizations to demonstrate their commitment to sustainability, enhance brand reputation, and achieve long-term cost savings. It involves balancing economic, social, and environmental performance, and necessitates commitment from top management, involvement of stakeholders, continuous improvement, and innovation. By embracing green management, organizations contribute to environmental protection and the overall wellbeing of society (Moradi et al., 2017a; Moradi et al., 2017b).
Individuals have the power to make a substantial impact on green management efforts within their workplaces through various actions. First, they can reduce energy consumption by adopting simple practices such as turning off lights and electronics when not in use, adjusting thermostats for energy efficiency, and utilizing natural light whenever possible. Additionally, minimizing waste by opting for reusable containers, actively recycling paper and other materials, and reducing packaging usage can significantly contribute to waste reduction. Embracing sustainable transportation methods like carpooling, public transportation, or biking/walking to work helps lower carbon footprints and supports sustainable commuting (Moradi et al., 2017a; Moradi et al., 2017b; Khan et al., 2021a). Making sustainable purchasing choices, such as selecting environmentally friendly and sustainably sourced products and supplies, contributes to a culture of sustainable procurement. Individuals can also play a crucial role by educating and motivating their colleagues about the significance of green management practices and encouraging sustainable behaviors in the workplace. Active participation in green initiatives, such as volunteering for sustainability committees or engaging in energy audits and waste reduction programs, further amplifies the impact of individuals in promoting green management. By collectively fostering a culture of sustainability, individuals can effect positive change for both the environment and their organizations.
Green initiatives encompass diverse actions undertaken by organizations, governments, and individuals to advance environmental sustainability and mitigate environmental impact. These initiatives cover a broad spectrum of environmental concerns, including climate change, pollution, and resource depletion. Examples of green initiatives include promoting the utilization of renewable energy sources like solar, wind, and hydroelectric power to reduce greenhouse gas emissions and encourage sustainable energy practices. Energy efficiency initiatives aim to enhance energy efficiency by adopting energy-saving equipment and appliances, as well as implementing building retrofits to curtail energy consumption and associated costs. Waste reduction initiatives incorporate recycling programs, composting, and reduction of packaging materials to minimize landfill waste and mitigate environmental consequences (Yi et al., 2022).
By working together and taking action, organizations, governments, and individuals can make a significant contribution to creating a more sustainable future. Carbon pricing policies can incentivize businesses to reduce greenhouse gas emissions, while the CE model promotes resource reuse and closed-loop supply chains. Sustainable finance integrates ESG factors into investment decisions, and initiatives like green bonds and sustainability-linked loans are gaining traction (Khan et al., 2021b; Khan et al., 2022a). The transition to electric vehicles is gaining momentum, with targets set for phasing out gas-powered vehicles and investments in EVs and charging infrastructure. Sustainable agriculture practices reduce the environmental impact while promoting food security (Renda, 2019; Khan et al., 2021b; Al Mubarak and Hamdan, 2023). These initiatives address urgent environmental challenges and meet the growing demand for sustainable practices. Green bonds offer investors a way to support environmentally friendly projects, provide financial returns, transparency, access to capital, and enhance an issuer’s reputation as a socially responsible organization. Green bonds play a vital role in financing sustainable projects and promoting sustainability, aligning investments with environmental goals while generating financial returns.
Begin by identifying your financial goals, whether it is retirement, buying a house, or saving for education, as this will determine your investment time horizon. Estimate how long it will take to achieve your goals and consider any liquidity needs that may require accessing your investments before your goals are met. Regularly reassess your investment time horizon as your circumstances change and adjust your strategy accordingly (Paliwal et al., 2020; Palomares et al., 2021; Khan et al., 2022a; Ma et al., 2022; Yi et al., 2022). Remember that your investment time horizon influences the level of risk you can tolerate. Longer time horizons allow for more risk-taking to withstand short-term market fluctuations, while shorter time horizons may call for lower-risk investments with greater stability. Working with a financial advisor can provide valuable guidance in avoiding these mistakes and selecting an investment strategy that suits your risk tolerance and helps you achieve your financial goals.
Determining the investment time horizon is a crucial aspect of aligning investment strategies with financial goals and risk tolerance. In cases where individuals lack the expertise to establish their time horizon or select appropriate strategies, engaging with a financial advisor becomes imperative. The selection of a financial advisor holds significant implications for financial wellbeing. Several factors should be considered in this process, including the advisor’s credentials, qualifications (e.g., CFP or CFA designation), and experience working with comparable clients. Evaluating the services offered by the advisor, ensuring alignment with financial needs and goals, is essential (Palomares et al., 2021; Khan et al., 2022a; Ma et al., 2022; Yi et al., 2022). Transparency in the fee structure, encompassing upfront fees, ongoing charges, and commissions, is crucial. Effective communication, responsiveness to queries, and clear articulation of information are desirable traits in an advisor. Assessing whether the advisor adheres to a fiduciary duty, prioritizing the client’s best interests, is important. Additionally, considering the advisor’s reputation, track record, and client reviews contributes to making an informed decision (Di Vaio and Varriale, 2020; Paliwal et al., 2020; Liu and Lin, 2021; Tsolakis et al., 2021; Chang et al., 2022). Choosing a financial advisor necessitates a thorough assessment of individual financial needs, alongside qualifications, experience, and service offerings. By investing time and effort in selecting the right advisor, individuals can establish enduring relationships that facilitate the achievement of financial goals and secure their financial future.
Standards development plays a crucial role in shaping the trajectory of emerging ecosystems by establishing technical, economic, and social protocols. These protocols form the foundational framework for the ecosystem architecture. During this initial phase, it is essential to ensure broad-based participation to prevent the marginalization of vulnerable communities through non-inclusive models that distribute benefits unequally. While efficiency may be a driving factor for private sector leadership, it is democratically representative multi-stakeholders that ensures the inclusion of the needs and perspectives of less resourced groups in the co-design of solutions. Inclusive standards initiatives, such as the International Organization for Standardization (ISO), provide platforms for civil society organizations like the Inclusive Tech Lab and World Fair Trade Organization to advocate for the priorities of smallholder communities within technical committees that consist of representatives from industry, government, and academia (Di Vaio and Varriale, 2020; Varriale et al., 2020; Tsolakis et al., 2021; Khan et al., 2022b; Chang et al., 2022; Hassoun et al., 2022; Xue et al., 2022). These partnerships bridge the gap between different perspectives and help guide the development of protocols that consider accessibility, rights protection, and distributional concerns upfront. Impact assessments that take into account dimensions such as gender, poverty, and climate further inform the decision-making process. Shared governance roadmaps that incorporate SDG targets serve as constructive starting points (Moradi et al., 2017b; Renda, 2019; Chien, 2022; Chandan et al., 2023). They encourage the development of pathways that prioritize positive impact over purely profit-driven motives. By adopting these inclusive and impact-optimized approaches, the resulting protocols are more likely to prevent downstream social and environmental costs and contribute to a more equitable and sustainable future (Varriale et al., 2020; Hassoun et al., 2022; Xue et al., 2022; Mehedintu and Soava, 2023; Memon and Ooi, 2023; Wang et al., 2023; Zhang, 2023).
Collaborative arrangements that integrate technology contributors, knowledge holders, and communities impacted by applications into shared ownership and decision-making structures are vital for fostering responsive solutions that self-regulate toward public benefit. These arrangements incorporate built-in feedback mechanisms, allowing for continuous improvement and adaptation. Multi-stakeholder cooperatives are one example of such collaborative arrangements. These cooperatives pool resources and distribute benefits more equitably based on proportional capital or effort contributions, rather than adhering to winner-takes-all dynamics prevalent in traditional market systems (Kwilinski, 2023; Leong et al., 2023; Memon and Ooi, 2023; Singh et al., 2023; Wang et al., 2023). The Silicon Valley Startup Anthropic has partnered with traditional knowledge experts alongside AI modelers, recognizing the value of diverse perspectives in developing effective solutions. Similarly, the blockchain initiative Circular involves artisanal miners in project governance to ensure fair sourcing arrangements and empower local communities (Memon and Ooi, 2023; Wang et al., 2023). By granting rooted participation and ownership stakes, these collaborative arrangements provide a voice to those most affected by the processes and outcomes. This incentivizes sincere cooperation and problem-solving from indigenous perspectives, moving away from top-down approaches that may offer disconnected, piecemeal solutions. Incorporating local realities on the ground strengthens the relevance and effectiveness of the solutions developed. Policy support is crucial for scaling models that systematically account for social and environmental protections as cooperative investments (Kwilinski, 2023; Leong et al., 2023; Soori et al., 2023; Wu et al., 2023). By doing so, societies enable the development of just and regenerative innovations. This approach recognizes that cooperative efforts and investments in social and environmental safeguards are not only morally imperative but also yield long-term benefits for communities and the environment. Collaborative arrangements that integrate various stakeholders into shared ownership and decision-making structures foster more responsive, equitable, and sustainable solutions. By valuing diverse perspectives, empowering local communities, and implementing policies that support cooperative approaches, societies can drive positive change and ensure the development of innovative solutions that benefit the public at large (Kwilinski, 2023; Leong et al., 2023; Soori et al., 2023; Wu et al., 2023).
Continuous feedback loops that evaluate the long-term socioeconomic and environmental performance of distributed technologies are crucial for stewarding aligned outcomes based on stakeholder priorities. These feedback loops should be based on standardized indicator frameworks that allow for consistent measurement and comparison. One key aspect of these feedback loops is identifying unintended harms that may arise in different geographical and demographic contexts, as well as recognizing marginalized groups that may be overlooked. Mitigation action plans should be developed in collaboration with affected communities to address these unintended harms and ensure equitable outcomes (Zkik et al., 2023a; Richey et al., 2023; Saeed et al., 2023; Soori et al., 2023; Wu et al., 2023). It is important to capture the contributions of distributed technologies towards the SDGs and sustainability drivers such as resource efficiency, pollution prevention, and biodiversity conservation (Moradi et al., 2017b; Renda, 2019; Chien, 2022; Chandan et al., 2023). This information can guide impact-focused process improvements and inform future research and development efforts. To prevent a narrow “solutionism” mentality that overlooks contextual nuances, citizen involvement is essential. Participatory budgeting and multi-perspective impact weighting, using deliberative democratic methods that incorporate indigenous epistemologies, can ensure that diverse perspectives are considered in decision-making processes (Zkik et al., 2023a; Zkik et al., 2023b; Richey et al., 2023; Saeed et al., 2023; Wu et al., 2023). Transparency is a vital component of these assessments. Open registries that log activity flows, revenue streams, and outcomes should be established and made accessible to all parties. Transparent review cycles allow for scrutiny and accountability. Independent third-party certifiers play a critical role in appraising the accuracy and integrity of assessments. Their involvement bolsters accountability and helps preserve public trust as technologies mature and operate at increasingly larger scales.
To achieve inclusive progress through frontier technologies, it is essential to ensure universal access and opportunities for talent development across socioeconomic classes. Bridging digital divides and providing freely available online learning materials in multiple languages can enhance education and skills development, particularly for vulnerable groups impacted by automation (Leong et al., 2023; Soori et al., 2023; Wu et al., 2023). Combining vocational retooling programs with basic income schemes can alleviate adoption burdens and facilitate participation. Interdisciplinary education promotes a holistic understanding of technology’s impacts, fostering problem-solving skills that consider complexity and interconnectedness. Engaging marginalized communities through participatory training, respecting consent and benefit-sharing, integrates their unique perspectives and expertise. Coordinated multi-level actions are necessary to invest in knowledge and skills that benefit diverse constituents, aligning innovation pathways with needs and aspirations (Zkik et al., 2023a; Richey et al., 2023; Saeed et al., 2023). By prioritizing universal access, vocational retooling, interdisciplinary education, participatory engagement, and coordinated actions, inclusive prosperity can be achieved through frontier technologies. These approaches empower individuals and communities, ensuring that the benefits of technology are accessible to all (Zkik et al., 2023a; Zkik et al., 2023b; Saeed et al., 2023). Significant advancements in question answering models have yielded notable improvements in the accuracy and efficiency of natural language processing tasks. PAL-BERT, an advanced question answering model, integrates pre-training and fine-tuning techniques to augment the model’s comprehension of context, thereby enhancing its capacity to generate precise responses (Li et al., 2020; Guo et al., 2023; Zheng et al., 2023; Hu et al., 2024; Jin et al., 2024). A study in the field of energy strategy examined the evolutionary trajectory of China’s photovoltaic technology innovation network, offering valuable insights into its maturation and progression through an analysis of patents. The study underscored the network’s pivotal role in propelling technological advancements within the energy sector. Additionally, research explored the influence of institutional investor ESG activism on promoting environmentally friendly innovation in corporations as a means to address climate change, with particular attention to the disparities between digital and non-digital firms (Xu et al., 2021; Hu et al., 2023; Xu et al., 2023; Yang et al., 2023; Chen et al., 2024). The findings shed light on the potential of ESG activism to stimulate sustainable practices and innovation in the corporate domain (Chen and Pan, 2019; Biliavska et al., 2022; Chen and Su, 2022; Luo et al., 2023; Shen et al., 2023; Ali et al., 2024; Luo et al., 2024).
Knowledge exchange platforms play a crucial role in cultivating understanding and cooperation across different paradigms. One example of such platforms is the concept of “living labs.” Living labs serve as testbeds for collaborative models, conducting action-oriented experimentation and engaging stakeholders at the community level. These labs enable the co-creation and iterative refinement of solutions that address hyperlocal needs and impacts, involving technology teams, local experts, policymakers, residents, and impact investors. These demonstration projects serve as examples of “innovation corridors” where frontier research and development performers, public/private investors, and citizen oversight bodies work together to pilot inclusive models. The localized learnings from these projects help inform upscaling programs and avoid the one-size-fits-all fallacy. To synchronize multi-level actions, cross-sector collaboration is essential, bridging the realms of policy, finance, community, and technology. International cooperation arrangements that pool regional expertise and resources toward shared prosperity priorities help elevate incremental efforts to achieve systemic impacts. The United Nations (UNs) sustainable development framework provides a constructive rallying point for aligning public and private initiatives across scales and domains, using common impact benchmarks (Varriale et al., 2020; Xue et al., 2022; Mehedintu and Soava, 2023; Memon and Ooi, 2023; Richey et al., 2023; Saeed et al., 2023; Singh et al., 2023; Soori et al., 2023; Wang et al., 2023; Wu et al., 2023; Zhang, 2023). Coalitions such as the World Economic Forum’s Global Future Council on Blockchain and the OECD’s AI Policy Observatory convene multi-stakeholder working groups to chart interlinkages and mitigation pathways, considering technology-society interactions holistically from socio-technical perspectives. Cooperation between standardization bodies, social engineers, impact investors, and governments creates synergy and unlocks comprehensive, co-designed pathways that fully leverage emerging innovations while proactively safeguarding vulnerable communities and natural environments.
Sustainable investment has gained significant traction as investors increasingly prioritize environmental and social considerations. The rising awareness of sustainability issues and the demand for sustainable products and services have propelled sustainable investment into the mainstream. Companies now recognize the significance of sustainability for attracting capital and maintaining their social license to operate. Sustainable investment offers several benefits: Firstly, it can have a positive social and environmental impact by supporting companies and projects that promote sustainability. Secondly, sustainable investments can yield financial returns on par with or even surpassing traditional investments (Renda, 2019; Khan et al., 2021b; Song et al., 2022; Al Mubarak and Hamdan, 2023). Thirdly, sustainable investment helps manage risks associated with environmental and social issues, such as climate change and labor practices. Additionally, it enhances an investor’s reputation as a socially responsible organization, attracting customers, employees, and stakeholders. As sustainability continues to grow in importance, sustainable investment will become increasingly crucial for both investors and companies. Sustainability criteria encompass environmental, social, and governance (ESG) factors that investors employ to evaluate companies’ sustainability performance and determine their investment suitability. These criteria may include aspects such as carbon emissions, energy consumption, labor practices, human rights, and governance practices (Ghobakhloo et al., 2021; Khan et al., 2022a; Jiang et al., 2022). Sustainable investment approaches encompass screening, positive selection, and engagement. Screening excludes investments in environmentally or socially harmful activities, positive selection actively seeks investments in high-performing sustainable companies, and engagement involves actively encouraging companies to adopt sustainable practices and improve their sustainability performance. Sustainability criteria have gained significant importance among investors, who seek to align their investments with their values and drive positive social and environmental outcomes. Companies are increasingly recognizing the significance of sustainability, taking measures to enhance their sustainability performance in order to attract investment and maintain their social license to operate. Sustainability criteria serve as a vital tool for investors to assess the sustainability performance of companies and make investment decisions that align with their values and promote positive social and environmental impacts (Paliwal et al., 2020; Palomares et al., 2021; Ma et al., 2022; Yi et al., 2022). Investment strategies, on the other hand, are plans or approaches employed by investors to guide their investment decisions. These strategies consider factors such as financial goals, risk tolerance, investment horizon, and more, all aimed at achieving investment objectives. Various investment strategies exist, including growth investing, value investing, income investing, index investing, sustainable investing, and tactical asset allocation (Di Vaio and Varriale, 2020; Liu and Lin, 2021; Tsolakis et al., 2021; Khan et al., 2022b; Chang et al., 2022; Hassoun et al., 2022).
Blockchain registries and AI-driven supply/demand matching can mitigate risks of supply-demand mismatches by dynamically reconfiguring production lines. Anthropos’s renewable energy investment vehicle leverages these functions to direct capital efficiently based on local conditions and policies. Additionally, AI-based predictive maintenance optimizes asset performance by analyzing IoT sensor data, extending maintenance cycles and reducing carbon-intensive activities. Historic maintainer records can also provide insights for more accurate part and labor estimations, improving repair budgeting. Route optimization is enhanced through AI simulations that consider factors such as weather, traffic, regulations, and node inventories to determine hyper-efficient multi-modal route planning. Platforms like Project Vesta can significantly reduce miles and hours from global routes, thus reducing carbon footprints. Bayer’s platform Kirchhoff goes beyond route optimization by incorporating dynamic pricing data and even production/demand shifts to maximize resource allocation within the circulation of goods. These applications demonstrate how the combination of blockchain and AI technologies can optimize supply chains by improving supply-demand matching, enhancing asset performance and maintenance, and optimizing route planning (Zkik et al., 2023a; Richey et al., 2023; Saeed et al., 2023; Wu et al., 2023).
By leveraging these technologies, organizations can achieve greater efficiency, reduced environmental impact, and improved resource allocation in their supply chain operations. Digital technologies show promise for optimizing resource flows through supply chain coordination, yet prudent policymaking remains crucial to realizing inclusive sustainability gains at scale. Blockchain uniquely addresses inter-organizational trust and coordination issues inhibiting cleaner production globally through incentivizing desirable behaviors while regulations safeguard accessibility. Distributed governance oversees impact accountability via participatory processes inclusive of all affected (Zkik et al., 2023a; Richey et al., 2023; Saeed et al., 2023; Soori et al., 2023; Wu et al., 2023). Multi-level actions synchronize recommended efforts including low-carbon industry funds conditioned on transparent participation according lifecycle assessments and localized benefit reviews co-designed democratically. Strategic data protocols underpin collaborative solutions balancing ecological modernization with fairness concerns. International arrangements pool capacities on shared sustainability priorities through matched demonstratable pilots’ upscaling regionally-tailored lessons avoiding singular approaches. Continuous stewardship across intersecting policy domains coordinates frontier opportunities steering prosperity within planetary boundaries equitably for current and future inhabitants.
Sustainable supply chain finance integrates sustainability into financial decisions, supporting environmentally friendly and socially responsible practices. Connectivity enables real-time communication and collaboration among stakeholders through digital technologies, facilitating information flow and coordination. Together, these concepts promote environmentally responsible and socially inclusive business practices.
Transparent tracking of sustainability metrics promotes accountability and drives waste reduction, lower carbon footprints, improved working conditions, and increased trust. Integrating sustainable supply chain finance and connectivity aligns financial investments and supply chain practices with broader sustainability goals, addressing climate change and poverty reduction. Figure 2 illustrates the alignment of green management goals and principles, emphasizing poverty alleviation and renewable energy adoption in developing countries, and low-carbon circular economies and environmental protection in developed countries. Both contexts prioritize regulatory frameworks, standards, incentives, public-private partnerships, and innovation to drive sustainable practices. They strive to align their green management principles with international agreements such as the Paris Agreement and the UNs SDGs (Moradi et al., 2017b; Renda, 2019; Chien, 2022). This includes sharing knowledge, expertise, and financial resources to support capacity-building efforts and technology transfer in developing countries. While there are similarities in the goals and principles of green management between developing and developed countries, it is crucial to acknowledge the differing contexts and priorities. Developing countries often face resource constraints, limited infrastructure, and socio-economic disparities, while developed countries grapple with the need for sustainable consumption patterns and addressing legacy environmental issues. The collective pursuit of green management goals across countries can foster a more sustainable and resilient global future.
FIGURE 2. Examining the goals and principles of green management in developing and developed countries.
To successfully implement green management in China’s industries, a comprehensive approach is needed as presented in Figure 3. This involves setting clear goals, developing supportive policies, fostering collaboration, promoting resource efficiency and circular economy practices, investing in research and development, providing training, ensuring transparency, engaging stakeholders, and monitoring progress.
FIGURE 3. Key steps and strategies for successful implementation of green management in Chinese industries.
By adopting these strategies, China can achieve sustainable development, reduce environmental impact, and contribute to global efforts in combating climate change and promoting green practices. In developed countries, green management principles revolve around environmental protection, energy efficiency, waste reduction, and sustainable consumption. These countries have established regulatory frameworks, standards, and incentives to drive sustainability. They prioritize public-private partnerships, innovation, and research and development for environmental technologies. Developing and developed countries acknowledge the importance of international cooperation to tackle global environmental challenges.
Government regulations and incentives are crucial in driving green management practices in industries for sustainability and success.
Regulations enforce environmental laws, rules, and standards, guiding responsible practices like emissions control and waste management. Incentives, such as financial support and tax benefits, motivate industries to adopt green practices, enhancing competitiveness and market reputation. This combination creates a supportive environment for integrating sustainability, fostering resource efficiency, reducing environmental footprints, and ensuring long-term industry viability while addressing environmental challenges. Figure 4 shows the government regulations and incentives play a significant role in driving green management practices, resulting in various benefits and impacts. These include the reduction of pollution, conservation of resources, improved public health, enhanced competitiveness, job creation, and progress towards global sustainability goals. Moreover, this approach fosters innovation and the development of sustainable technologies, facilitating a transition to a greener and more sustainable economy.
Green bonds are a popular sustainable investment option, but they should be compared to other alternatives. Socially responsible investing (SRI) allows investors to align their portfolios with social and environmental criteria, though it may lack the specificity and transparency of green bonds. Impact investing generates measurable social and environmental benefits alongside financial returns, but it may not offer the same liquidity and marketability.
Sustainable mutual funds and exchange-traded funds (ETFs) show diversification across sustainable companies, including green bonds, SRI companies, and other assets, with professional management and broader exposure. Factors to consider when comparing these options include risk, return potential, liquidity, transparency, and alignment with specific environmental goals. Green bonds are designed for environmentally friendly projects, offering transparency and stable returns. The choice depends on individual investment goals, risk tolerance, and preferences, with some favoring the targeted impact of green bonds and others opting for a diversified approach through funds.
The objective of this article is to predict changes in adverse environmental effects, waste reduction, and promotion of environmental sustainability by enhancing the growth and structural improvement of the digital economy. A feedforward neural network was constructed based on the information provided in Table 1. The neural network’s outputs include the reduction of adverse environmental effects, waste reduction, and promotion of environmental sustainability, while the inputs correspond to the growth of the digital economy (ranging from 0% to 90%) and the improvement of its structure (ranging from 0% to 70%). Figure 5 shows the predicted results for the reduction of adverse environmental effects obtained from the neural network. It is noteworthy that the behavior of adverse environmental effects exhibits a sinusoidal pattern, not always maintaining a stable trend, and fluctuating in a sinusoidal manner. The maximum reduction is achieved when the improvement of the digital economy’s structure is at its peak and the growth of the digital economy is at its lowest. Figure 6 shows the estimated results for waste reduction by the neural network.
FIGURE 5. The results obtained from the ANN in order to predict the (A) front view and (B) side view adverse effects of the environment tested in this study.
FIGURE 6. The results obtained from the ANN in order to predict the reduction of lesions (A) front view and (B) side view.
The analysis reveals that waste reduction is positively correlated with the growth of the digital economy. As the digital economy expands, waste reduction also increases. Conversely, the improvement of the digital economy’s structure does not exert a significant influence on waste reduction. In the absence of digital economic growth, waste tends to rise. Furthermore, Figure 7 demonstrates the estimated results for promoting environmental sustainability. Similar to waste reduction, the promotion of environmental sustainability shows a positive association with the growth of the digital economy.
FIGURE 7. The results obtained from the ANN in order to predict the promotion of environmental sustainability (A) front view and (B) side view.
The improvement of the digital economy’s structure alone does not yield a discernible impact on enhancing environmental sustainability. However, when combined with digital economic growth, it contributes to improved environmental sustainability. The maximum level of environmental sustainability is attained when the growth of the digital economy is at its peak and the improvement of its structure is at its minimum. Figure 8 illustrates the results obtained from linear regression analysis. The ANN shows a high level of accuracy, with an error rate of less than 1% when compared to the targets specified in Table 1, namely, adverse environmental effects, waste reduction, and promotion of environmental sustainability.
FIGURE 8. Linear regression charts to check the error of the ANN formed in this study, (A) adverse environmental effects (%), (B) reduction of waste (%) (C) and promotion of environmental sustainability (%).
The results affirm that by fostering the growth of the digital economy and improving its structure, adverse environmental effects can be mitigated, waste can be reduced, and environmental sustainability can be promoted. Adverse environmental effects reach their maximum when the improvement of the digital economy’s structure is at its peak and the growth of the digital economy is at its lowest. Improving the structure of the digital economy does not exert a significant influence on waste reduction, and waste tends to increase in the absence of digital economic growth. Similarly, the promotion of environmental sustainability is maximized when the growth of the digital economy is at its peak and the improvement of its structure is at its minimum.
Investment strategies with green financial goals and risk tolerance are driven by the desire to align investments with environmental objectives while managing risk appropriately. These strategies seek to generate financial returns while also considering the impact on the environment. By incorporating green financial goals, investors aim to support sustainable initiatives, promote clean technologies, and contribute to the transition towards a low-carbon economy. Risk tolerance plays a crucial role in determining the investment approach, as it influences the balance between potential returns and associated risks. Sustainable investment options such as green bonds, impact investing, and sustainable funds provide avenues for investors to allocate capital towards environmentally responsible projects and companies. These investment strategies can have positive environmental effects by facilitating the financing of renewable energy projects, energy-efficient infrastructure, sustainable agriculture, and other environmentally friendly initiatives. By directing capital towards these sectors, investors can help drive positive change and contribute to the preservation of the natural environment.
As digital networks and technologies become further embedded in every facet of modern life and economies, addressing their adverse environmental repercussions may be imperative going forward. Policymakers worldwide are grappling with regulatory solutions, while companies are investing in renewable energy sourcing and efficiency drives. However, a lack of comprehensive data on emissions inventories and best practices has posed a hindrance. With no standard frameworks to measure and mitigate “digital carbon”, optimized solutions have remained elusive. This study aims to comprehensively examine the structure of the digital economy and investigate the potential of emerging technologies such as blockchain and ANNs in mitigating its adverse environmental impacts. This research has several objectives such as to conduct a literature review to analyze the environmental effects of the expanding digital economy, including topics such as supply chain management, sustainable innovation, and technology applications in sectors like agriculture, mining, and manufacturing. Secondly, to identify opportunities where blockchain and ANNs have been effectively employed to enhance supply chain transparency, optimize logistics, reduce emissions, and promote circularity, based on the examination of case studies and reported data. Thirdly, to address knowledge gaps related to quantifying and reporting the environmental footprint of the digital economy, and recommend the adoption of uniform frameworks for consistent measurement. Lastly, to discuss the potential of standardized methodologies, collaborative action, targeted research, and optimized multi-stakeholder solutions in addressing challenges within the digital economy through the integration of these emerging technologies across various sectors. This research is innovative in its comprehensive investigation of the digital economy’s structure and its exploration of specific applications of blockchain and AI technologies, supported by empirical evidence of their measurable sustainability benefits. By evaluating technology combinations that yield quantifiable environmental outcomes, this study aims to advance knowledge in this relatively unexplored field, providing valuable insights for decision-makers to design appropriate interventions for responsible digital transformation. In this study, a progressive ANN of shallow type with a hidden layer was developed to explore the relationship between the digital economy and environmental sustainability. The network was trained and tested using various cases, including adverse environmental effects, waste reduction, and promotion of environmental sustainability. By incorporating a wider range of experimental samples, the network provided estimations with enhanced accuracy and reliability. The error of the ANN‘s predictions was evaluated using linear regression to assess its performance. The results generated by the network were carefully analyzed and documented, providing valuable insights into the potential impact of digital economy growth and structural improvements on adverse environmental effects, waste reduction, and the promotion of environmental sustainability. The process of estimation was thoroughly evaluated to ensure the robustness and validity of the results. A potential future direction for this study involves collecting longitudinal data over several years in order to capture the dynamic changes in determinants that influence wellbeing and physical health in China. By incorporating longitudinal data, the predictive power of the ANN model can be significantly improved. Additionally, it is important to consider incorporating additional relevant input and output variables from a wider range of sources to provide a more comprehensive analysis and to capture a more holistic understanding of the factors impacting wellbeing and physical health. Furthermore, to enhance the generalizability of the ANN model beyond the China context, it would be valuable to validate the model using data from multiple countries. This would allow for a more robust evaluation of the model’s effectiveness and applicability in different settings. In terms of the ANN architecture, further refinement can be explored by experimenting with additional hidden layers, different activation functions, and optimization algorithms. These modifications can potentially improve the convergence and predictive accuracy of the model. Lastly, conducting experiments with larger sample sizes would enable a more rigorous assessment of the ANN model’s performance and provide more robust conclusions regarding its effectiveness in predicting wellbeing and physical health outcomes.
The utilization of innovative digital technologies holds promise for optimizing production systems and economic activity, while also prioritizing sustainability as a common goal across interconnected societies. The adoption of distributed technologies has the potential to reduce environmental impacts by addressing information imbalances in global supply chains. However, it is crucial to ensure inclusive governance that incorporates democratic participation to prevent the exacerbation of existing inequities when deploying these technologies, as vulnerable communities need protection. Collaborative policymaking that encompasses diverse perspectives provides a sensible approach to guiding responsible innovation that benefits all present and future generations. Establishing multi-stakeholder cooperatives that embrace local viewpoints can serve as constructive platforms for scaling effective solutions globally, requiring ongoing cooperation to navigate the rapid changes we face. By steering these tools with careful consideration for wellbeing as the primary objective, emerging technologies can facilitate the transition to post-carbon economies while ensuring long-term shared prosperity within the limits of our planet.
The original contributions presented in the study are included in the article/Supplementary material, further inquiries can be directed to the corresponding author.
TC: Formal analysis, Investigation, Writing–original draft, Writing–review and editing. ZH: Investigation, Supervision, Writing–original draft, Writing–review and editing.
The author(s) declare that no financial support was received for the research, authorship, and/or publication of this article.
The authors declare that the research was conducted in the absence of any commercial or financial relationships that could be construed as a potential conflict of interest.
All claims expressed in this article are solely those of the authors and do not necessarily represent those of their affiliated organizations, or those of the publisher, the editors and the reviewers. Any product that may be evaluated in this article, or claim that may be made by its manufacturer, is not guaranteed or endorsed by the publisher.
Ali, T. A. A., Xiao, Z., Jiang, H., and Li, B. (2024). A class of digital integrators based on trigonometric quadrature rules. IEEE Trans. Industrial Electron. 71 (6), 6128–6138. doi:10.1109/tie.2023.3290247
Al Mubarak, M., and Hamdan, A. (2023). “Sustainable competitive advantage through technological innovation: an introduction,” in Technological sustainability and business competitive advantage (Cham: Springer International Publishing), 3–8.
Biliavska, V., Castanho, R. A., and Vulevic, A. (2022). Analysis of the impact of artificial intelligence in enhancing the human resource practices. J. Intell. Manag. Decis. 1, 128–136. doi:10.56578/jimd010206
Chandan, A., John, M., and Potdar, V. (2023). Achieving UN SDGs in food supply chain using blockchain technology. Sustainability 15 (3), 2109. doi:10.3390/su15032109
Chang, X., Su, J., and Yang, Z. (2022). The effect of digital economy on urban green transformation—an empirical study based on the yangtze river delta city cluster in China. Sustainability 14 (21), 13770. doi:10.3390/su142113770
Chen, C., and Pan, J. (2019). The effect of the health poverty alleviation project on financial risk protection for rural residents: evidence from Chishui City, China. Int. J. Equity Health 18 (1), 79. doi:10.1186/s12939-019-0982-6
Chen, L., and Su, S. (2022). Optimization of the trust propagation on supply chain network based on blockchain plus. J. Intell. Manag. Decis. 1 (1), 17–27. doi:10.56578/jimd010103
Chen, Y., Wang, Y., and Zhao, C. (2024). From riches to digitalization: the role of AMC in overcoming challenges of digital transformation in resource-rich regions. Technol. Forecast. Soc. Change 200, 123153. doi:10.1016/j.techfore.2023.123153
Chien, F. (2022). The mediating role of energy efficiency on the relationship between sharing economy benefits and sustainable development goals (Case of China). J. Innovation Knowl. 7 (4), 100270. doi:10.1016/j.jik.2022.100270
Di Vaio, A., and Varriale, L. (2020). Blockchain technology in supply chain management for sustainable performance: evidence from the airport industry. Int. J. Inf. Manag. 52, 102014. doi:10.1016/j.ijinfomgt.2019.09.010
Esmaeilian, B., Sarkis, J., Lewis, K., and Behdad, S. (2020). Blockchain for the future of sustainable supply chain management in Industry 4.0. Resour. Conservation Recycl. 163, 105064. doi:10.1016/j.resconrec.2020.105064
Fatalla, A. A., Arzani, S., Veseli, E., Khademi, A., Khandan, A., Fahmy, M. D., et al. (2023). Revolutionizing systematic reviews and meta-analyses: the role of artificial intelligence in evidence synthesis. Dent. Hypotheses 14 (4), 93–94. doi:10.4103/denthyp.denthyp_122_23
Franco, R., Taghizadeh, M., Iranmanesh, P., Mirmohammadi, H., Hasselgren, G., Bang, H., et al. (2023). Whether enough attention is being paid to the ethical concerns regarding the use of artificial intelligence in dentistry? Dent. Hypotheses 14 (3), 69–70. doi:10.4103/denthyp.denthyp_96_23
Ghahremani-Nahr, J., Aliahmadi, A., and Nozari, H. (2022). An IoT-based sustainable supply chain framework and blockchain. Int. J. Innovation Eng. 2 (1), 12–21. doi:10.59615/ijie.2.1.12
Ghobakhloo, M., Iranmanesh, M., Grybauskas, A., Vilkas, M., and Petraitė, M. (2021). Industry 4.0, innovation, and sustainable development: a systematic review and a roadmap to sustainable innovation. Bus. Strategy Environ. 30 (8), 4237–4257. doi:10.1002/bse.2867
Guo, Y., Zhang, C., Wang, C., and Jia, X. (2023). Towards public verifiable and forward-privacy encrypted search by using blockchain. IEEE Trans. Dependable Secure Comput. 20 (3), 2111–2126. doi:10.1109/tdsc.2022.3173291
Hassoun, A., Prieto, M. A., Carpena, M., Bouzembrak, Y., Marvin, H. J., Pallares, N., et al. (2022). Exploring the role of green and Industry 4.0 technologies in achieving sustainable development goals in food sectors. Food Res. Int. 162, 112068. doi:10.1016/j.foodres.2022.112068
Hu, F., Mou, S., Wei, S., Qiu, L., Hu, H., and Zhou, H. (2024). Research on the evolution of China's photovoltaic technology innovation network from the perspective of patents. Energy Strategy Rev. 51, 101309. doi:10.1016/j.esr.2024.101309
Hu, F., Qiu, L., Wei, S., Zhou, H., Bathuure, I. A., and Hu, H. (2023). The spatiotemporal evolution of global innovation networks and the changing position of China: a social network analysis based on cooperative patents. R&D Manag. doi:10.1111/radm.12662
Jang, H. W., Yoo, J. J. E., and Cho, M. (2023). Resistance to blockchain adoption in the foodservice industry: moderating roles of public pressures and climate change awareness. Int. J. Contemp. Hosp. Manag. doi:10.1108/ijchm-09-2022-1127
Jiang, Q., Li, J., Si, H., and Su, Y. (2022). The impact of the digital economy on agricultural green development: evidence from China. Agriculture 12 (8), 1107. doi:10.3390/agriculture12081107
Jin, C., Monfort, A., Chen, F., Xia, N., and Wu, B. (2024). Institutional investor ESG activism and corporate green innovation against climate change: exploring differences between digital and non-digital firms. Technol. Forecast. Soc. Change 200, 123129. doi:10.1016/j.techfore.2023.123129
Khan, A. A., Laghari, A. A., Li, P., Dootio, M. A., and Karim, S. (2023). The collaborative role of blockchain, artificial intelligence, and industrial internet of things in digitalization of small and medium-size enterprises. Sci. Rep. 13 (1), 1656. doi:10.1038/s41598-023-28707-9
Khan, S. A. R., Godil, D. I., Jabbour, C. J. C., Shujaat, S., Razzaq, A., and Yu, Z. (2021a). Green data analytics, blockchain technology for sustainable development, and sustainable supply chain practices: evidence from small and medium enterprises. Ann. Operations Res., 1–25. doi:10.1007/s10479-021-04275-x
Khan, S. A. R., Piprani, A. Z., and Yu, Z. (2022a). Digital technology and circular economy practices: future of supply chains. Operations Manag. Res. 15 (3-4), 676–688. doi:10.1007/s12063-021-00247-3
Khan, S. A. R., Razzaq, A., Yu, Z., and Miller, S. (2021b). Retracted: industry 4.0 and circular economy practices: a new era business strategies for environmental sustainability. Bus. Strategy Environ. 30 (8), 4001–4014. doi:10.1002/bse.2853
Khan, S. A. R., Umar, M., Muhammad Zia-ul-haq, H., and Yu, Z. (2022b). Technological advancement and circular economy practices in food supply chain. Agri-Food 4.0 27, 65–75. doi:10.1108/s1877-636120220000027005
Kwilinski, A. (2023). The relationship between sustainable development and digital transformation: bibliometric analysis. Virtual Econ. 6 (3), 56–69. doi:10.34021/ve.2023.06.03(4)
Leong, L. Y., Hew, J. J., Lee, V. H., Tan, G. W. H., Ooi, K. B., and Rana, N. P. (2023). An SEM-ANN analysis of the impacts of Blockchain on competitive advantage. Industrial Manag. Data Syst. 123 (3), 967–1004. doi:10.1108/imds-11-2021-0671
Li, Q., Lin, H., Tan, X., and Du, S. (2020). H∞ consensus for multiagent-based supply chain systems under switching topology and uncertain demands. IEEE Trans. Syst. Man, Cybern. Syst. 50 (12), 4905–4918. doi:10.1109/tsmc.2018.2884510
Litvinenko, V. S. (2020). Digital economy as a factor in the technological development of the mineral sector. Nat. Resour. Res. 29 (3), 1521–1541. doi:10.1007/s11053-019-09568-4
Liu, K. S., and Lin, M. H. (2021). Performance assessment on the application of artificial intelligence to sustainable supply chain management in the construction material industry. Sustainability 13 (22), 12767. doi:10.3390/su132212767
Luo, J., Zhuo, W., Liu, S., and Xu, B. (2024). The optimization of carbon emission prediction in low carbon energy economy under Big data. IEEE Access.
Luo, J., Zhuo, W., and Xu, B. (2023). The bigger, the better? Optimal NGO size of human resources and governance quality of entrepreneurship in circular economy. Management Decision.
Ma, J. Y., Shi, L., and Kang, T. W. (2022). The effect of digital transformation on the pharmaceutical sustainable supply chain performance: the mediating role of information sharing and traceability using structural equation modeling. Sustainability 15 (1), 649. doi:10.3390/su15010649
Mehedintu, A., and Soava, G. (2023). Approach to the impact of digital technologies on sustainability reporting through structural equation modeling and artificial neural networks. Electronics 12 (9), 2048. doi:10.3390/electronics12092048
Memon, K. R., and Ooi, S. K. (2023). Identifying digital leadership's role in fostering competitive advantage through responsible innovation: a SEM-Neural Network approach. Technol. Soc. 75, 102399. doi:10.1016/j.techsoc.2023.102399
Moradi, J., Shahinzadeh, H., and Khandan, A. (2017b). A cooperative dispatch model for the coordination of the wind and pumped-storage generating companies in the day-ahead electricity market. Int. J. Renew. Energy Res. (IJRER) 7 (4), 2057–2067. doi:10.1016/j.energy.2017.11.088
Moradi, J., Shahinzadeh, H., Khandan, A., and Moazzami, M. (2017a). A profitability investigation into the collaborative operation of wind and underwater compressed air energy storage units in the spot market. Energy 141, 1779–1794. doi:10.1016/j.energy.2017.11.088
Nayal, K., Raut, R. D., Narkhede, B. E., Priyadarshinee, P., Panchal, G. B., and Gedam, V. V. (2021). Antecedents for blockchain technology-enabled sustainable agriculture supply chain. Ann. operations Res. 327, 293–337. doi:10.1007/s10479-021-04423-3
Paliwal, V., Chandra, S., and Sharma, S. (2020). Blockchain technology for sustainable supply chain management: a systematic literature review and a classification framework. Sustainability 12 (18), 7638. doi:10.3390/su12187638
Palomares, I., Martínez-Cámara, E., Montes, R., García-Moral, P., Chiachio, M., Chiachio, J., et al. (2021). A panoramic view and swot analysis of artificial intelligence for achieving the sustainable development goals by 2030: progress and prospects. Appl. Intell. 51, 6497–6527. doi:10.1007/s10489-021-02264-y
Renda, A. (2019). The age of foodtech: optimizing the agri-food chain with digital technologies. in Achieving the sustainable development goals through sustainable food systems, 171–187.
Richey, R. G., Chowdhury, S., Davis-Sramek, B., Giannakis, M., and Dwivedi, Y. K. (2023). Artificial intelligence in logistics and supply chain management: a primer and roadmap for research. J. Bus. Logist. 44 (4), 532–549. doi:10.1111/jbl.12364
Saeed, S., Altamimi, S. A., Alkayyal, N. A., Alshehri, E., and Alabbad, D. A. (2023). Digital transformation and cybersecurity challenges for businesses resilience: issues and recommendations. Sensors 23 (15), 6666. doi:10.3390/s23156666
Shen, J., Sheng, H., Wang, S., Cong, R., Yang, D., and Zhang, Y. (2023). Blockchain-based distributed multi-agent reinforcement learning for collaborative multi-object tracking framework. IEEE Trans. Comput., 1–11. doi:10.1109/tc.2023.3343102
Singh, A. K., Kumar, V. P., Shoaib, M., Adebayo, T. S., and Irfan, M. (2023). A strategic roadmap to overcome blockchain technology barriers for sustainable construction: a deep learning-based dual-stage SEM-ANN approach. Technol. Forecast. Soc. Change 194, 122716. doi:10.1016/j.techfore.2023.122716
Song, M., Fisher, R., de Sousa Jabbour, A. B. L., and Santibañez Gonzalez, E. D. (2022). Green and sustainable supply chain management in the platform economy. Int. J. Logist. Res. Appl. 25 (4-5), 349–363. doi:10.1080/13675567.2022.2045763
Soori, M., Arezoo, B., and Dastres, R. (2023). Artificial neural networks in supply chain management, A review. J. Econ. Technol. 1, 179–196. doi:10.1016/j.ject.2023.11.002
Sun, X., Chen, Z., Shi, T., Yang, G., and Yang, X. (2022). Influence of digital economy on industrial wastewater discharge: evidence from 281 Chinese prefecture-level cities. J. Water Clim. Change 13 (2), 593–606. doi:10.2166/wcc.2021.447
Tseng, M. L., Ha, H. M., Tran, T. P. T., Bui, T. D., Chen, C. C., and Lin, C. W. (2022). Building a data-driven circular supply chain hierarchical structure: resource recovery implementation drives circular business strategy. Bus. Strategy Environ. 31 (5), 2082–2106. doi:10.1002/bse.3009
Tsolakis, N., Niedenzu, D., Simonetto, M., Dora, M., and Kumar, M. (2021). Supply network design to address United Nations Sustainable Development Goals: a case study of blockchain implementation in Thai fish industry. J. Bus. Res. 131, 495–519. doi:10.1016/j.jbusres.2020.08.003
Tsolakis, N., Schumacher, R., Dora, M., and Kumar, M. (2023). Artificial intelligence and blockchain implementation in supply chains: a pathway to sustainability and data monetisation? Ann. Operations Res. 327 (1), 157–210. doi:10.1007/s10479-022-04785-2
Varriale, V., Cammarano, A., Michelino, F., and Caputo, M. (2020). The unknown potential of blockchain for sustainable supply chains. Sustainability 12 (22), 9400. doi:10.3390/su12229400
Wang, H., Yang, G., and Yue, Z. (2023). Breaking through ingrained beliefs: revisiting the impact of the digital economy on carbon emissions. Humanit. Soc. Sci. Commun. 10 (1), 609–613. doi:10.1057/s41599-023-02126-7
Wang, L., Chen, Y., Ramsey, T. S., and Hewings, G. J. (2021). Will researching digital technology really empower green development? Technol. Soc. 66, 101638. doi:10.1016/j.techsoc.2021.101638
Wu, J., Lin, K., and Sun, J. (2023). Improving urban energy efficiency: what role does the digital economy play? J. Clean. Prod. 418, 138104. doi:10.1016/j.jclepro.2023.138104
Xu, A., Qiu, K., and Zhu, Y. (2023). The measurements and decomposition of innovation inequality: based on Industry − University − Research perspective. J. Bus. Res. 157, 113556. doi:10.1016/j.jbusres.2022.113556
Xu, X., Wang, C., and Zhou, P. (2021). GVRP considered oil-gas recovery in refined oil distribution: from an environmental perspective. Int. J. Prod. Econ. 235, 108078. doi:10.1016/j.ijpe.2021.108078
Xue, Q., Feng, S., Chen, K., and Li, M. (2022). Impact of digital finance on regional carbon emissions: an empirical study of sustainable development in China. Sustainability 14 (14), 8340. doi:10.3390/su14148340
Yang, J., Yang, K., Xiao, Z., Jiang, H., Xu, S., and Dustdar, S. (2023). Improving commute experience for private car users via blockchain-enabled multitask learning. IEEE Internet Things J. 10 (24), 21656–21669. doi:10.1109/jiot.2023.3317639
Yang, Q., Ma, H., Wang, Y., and Lin, L. (2022). Research on the influence mechanism of the digital economy on regional sustainable development. Procedia Comput. Sci. 202, 178–183. doi:10.1016/j.procs.2022.04.025
Yi, M., Liu, Y., Sheng, M. S., and Wen, L. (2022). Effects of digital economy on carbon emission reduction: new evidence from China. Energy Policy 171, 113271. doi:10.1016/j.enpol.2022.113271
Zhang, Z. (2023). The impact of the artificial intelligence industry on the number and structure of employments in the digital economy environment. Technol. Forecast. Soc. Change 197, 122881. doi:10.1016/j.techfore.2023.122881
Zheng, W., Lu, S., Cai, Z., Wang, R., Wang, L., and Yin, L. (2023). PAL-BERT: an improved question answering model. Computer Modeling in Engineering and Sciences. doi:10.32604/cmes.2023.046692Moradi
Zkik, K., Sebbar, A., Fadi, O., Kamble, S., and Belhadi, A. (2023a). Securing blockchain-based crowdfunding platforms: an integrated graph neural networks and machine learning approach. Electron. Commer. Res., 1–37. doi:10.1007/s10660-023-09702-8
Zkik, K., Sebbar, A., Fadi, O., Mustapha, O., and Belhadi, A. (2023b). “A graph neural network approach for detecting smart contract anomalies in collaborative economy platforms based on blockchain technology,” in 2023 9th international conference on control, decision and information technologies (CoDIT) (IEEE), 1285–1290.
Keywords: digital economy, blockchain technology, adverse environmental effects, artificial neural networks, management
Citation: Cai T and Hong Z (2024) Exploring the structure of the digital economy through blockchain technology and mitigating adverse environmental effects with the aid of artificial neural networks. Front. Environ. Sci. 12:1315812. doi: 10.3389/fenvs.2024.1315812
Received: 10 October 2023; Accepted: 08 February 2024;
Published: 12 March 2024.
Edited by:
Željko Stević, University of East Sarajevo, Bosnia and HerzegovinaReviewed by:
Adis Puška, University of Bijeljina, Bosnia and HerzegovinaCopyright © 2024 Cai and Hong. This is an open-access article distributed under the terms of the Creative Commons Attribution License (CC BY). The use, distribution or reproduction in other forums is permitted, provided the original author(s) and the copyright owner(s) are credited and that the original publication in this journal is cited, in accordance with accepted academic practice. No use, distribution or reproduction is permitted which does not comply with these terms.
*Correspondence: Tengwei Cai, Y2FpdDAwMTNAZS5udHUuZWR1LnNn
Disclaimer: All claims expressed in this article are solely those of the authors and do not necessarily represent those of their affiliated organizations, or those of the publisher, the editors and the reviewers. Any product that may be evaluated in this article or claim that may be made by its manufacturer is not guaranteed or endorsed by the publisher.
Research integrity at Frontiers
Learn more about the work of our research integrity team to safeguard the quality of each article we publish.