- 1School of Agricultural, Environmental and Veterinary Sciences, Charles Sturt University, Albury, NSW, Australia
- 2Gulbali Institute, Charles Sturt University, Albury, NSW, Australia
- 3Department of Planning and Environment (NSW), Albury, NSW, Australia
Although intermittent and ephemeral rivers lack surface flow for part of the year, they provide vital refuges for biota in otherwise dry semi-arid and arid landscapes. The hydrology of many such rivers has been altered due to river regulation and climate change. Environmental flows can be delivered to address the negative impacts of regulated flows, however there is limited knowledge of how dry ephemeral ecosystems respond following environmental flows. This study examined changes in water quality of the ephemeral Thule Creek in the southern Murray-Darling Basin, Australia, following delivery of environmental water from an irrigation canal. We also examined how the environmental flow influenced water quality of Wakool River that receives inflows from Thule Creek. Six sites in Thule Creek, three in Wakool River, and one in Yarraman irrigation channel (source water) were monitored for dissolved organic carbon (DOC), nutrients and dissolved oxygen (DO) once per week over 15 weeks from October 2019 to January 2020. The environmental flow resulted in high DOC concentrations (4.4–76 mg/L). Although low DO levels at sites in Thule Creek were recorded on some dates below the threshold for fish stress (< 4 mg/L) there were no fish kills observed during the environmental flow. The carbon-rich and nutrient-rich water (DOC >10 mg/L, total phosphorus up to 94 μg/L, total nitrogen up to 1,125 μg/L) was detected in the Wakool River downstream of Thule Creek confluence compared to the Wakool River upstream of Thule Creek confluence (DOC 6.6 mg/L, total phosphorus up to 64 μg/L, total nitrogen up to 805 μg/L) during the period when the environmental flow in Thule Creek was connected with the Wakool River. This research provides an example of how irrigation canal networks can be used to deliver environmental water to an ephemeral river to maintain refuges and contribute to the productivity of a receiving river further downstream. Careful management of the timing, volume and duration of environmental flows in arid or semi-arid landscapes is needed to avoid the development of poor water quality during, or following, the delivery of environmental water.
1 Introduction
Intermittent and ephemeral rivers constitute over half the world’s river network (Datry et al., 2017; Messager et al., 2021). Although intermittent and ephemeral rivers lack permanent surface flow, they provide a vital source of water and refuge for biota in otherwise dry landscapes. It has been recognised that they support a high level of biodiversity, provide valuable habitat for endemic and rare species and contribute to biogeochemical processes of the ecosystems (Capon, 2003; Deil, 2005; Vorste et al., 2020). However, intermittent and ephemeral rivers are threatened by climate change and intensive anthropogenic pressures resulting in their rapid loss and degradation both globally (Dudgeon et al., 2006; Palmer et al., 2008; Pekel et al., 2016) and within Australia (Finlayson et al., 2011). Despite their prevalence throughout the world, intermittent and ephemeral rivers are less studied than perennial rivers.
The hydrological regimes of intermittent rivers are harder to characterise than those of permanent rivers (Graf and Lecce, 1988) because they are more variable as the rivers stop flowing or dry out at some point in time and space. These systems fluctuate in time and space in response to inter-annual hydrological variability, and seasonal hydrological variability in some circumstances (Zanor et al., 2012). Intermittent rivers receive inconsistent surface water flow and may cease to flow during dry seasons. Runoff from precipitation or upstream sources might be a supplement water source for these river systems (Zollitsch and Christie, 2014). Ephemeral rivers experience periodic wetting and drying cycles that substantially vary in terms of the timing, frequency and duration of their inundation events (Williams, 1996; Brock et al., 2003). The hydrology of many lowland river systems has been altered as a result of river regulation (e.g., Ward and Stanford, 1995; Nilsson and Berggren, 2000; Nilsson et al., 2005), with small to medium flood events being captured by upstream impoundments and water diverted out of river systems for irrigation and other uses. The flow regime of intermittent and ephemeral rivers has also been altered due to river regulation. Some intermittent and ephemeral rivers receive inflows from upstream permanent rivers. However, some inland intermittent and ephemeral rivers are situated high on floodplains and are only occasionally inundated by floodwater following large flow events and floods. Under unregulated conditions (modelled natural) these river systems would have received more frequent flows.
The hydrological connection between river channels and their floodplains promotes the transportation of carbon and nutrients, influencing in the functioning of the entire river system (Harris et al., 2017). The productivity and biodiversity of floodplain river ecosystems is closely linked to flows that can mobilise resources (e.g., carbon and nutrients) from low lying geomorphic features during in-channel flows and from floodplains during large flow events (Junk et al., 1989). These resources are subsequently utilised by a range of organisms, including bacteria, invertebrates, waterbirds and fish over a range of temporal and spatial scales. River regulation has disrupted river-floodplain connectivity through alterations in the volume, timing, and duration of high flow events (Ward and Stanford, 1995; Bunn and Arthington, 2002). Due to the loss of river-floodplain connectivity, the condition of terrestrial-aquatic habitats of intermittent and ephemeral rivers have declined and their biogeochemical processes have been altered.
Environmental water is water allocated and managed to improve and restore degraded river, wetland, and floodplain ecosystems (including the plants and animals that depend on these ecosystems), mitigate detrimental environmental outcomes of alteration of flow cycles (Konrad et al., 2011) and return a more natural cycle of flows to river and wetland ecosystems. Research on environmental flows has largely focussed on water delivered to perennial-permanent river systems and associated wetlands (e.g., Lind et al., 2007; Shafroth et al., 2010). The majority of environmental flows to rivers are delivered by the release of water from dams and weirs (Konrad et al., 2011; Opperman et al., 2019) to meet the requirements of water-dependant ecosystems further downstream. Due to the way in which river regulation infrastructure has been developed in many river systems, it may be difficult to deliver environmental water to intermittent and ephemeral rivers that are less connected to the main sources of water. Without environmental water, key refuges in these intermittent and ephemeral systems would dry up. In some circumstances and locations there may be the opportunity to use irrigation canal network infrastructures to deliver environmental water to rivers (e.g., Watts et al., 2018). However, there is limited knowledge of how dry intermittent and ephemeral ecosystems respond following environmental watering, and to what extent this can impact the water quality of river systems further downstream.
In this research we examined outcomes of the delivery of environmental water from an irrigation canal network on the water quality and instream productivity of the ephemeral Thule Creek in the southern Murray-Darling Basin (MDB), Australia. We hypothesised that the delivery of environmental flows to this ephemeral creek via the irrigation canal would result in the release of carbon and nutrients from leaves, organic matter and soil in the previously dry creek bed into the water, and this would influence the water quality of the receiving river system downstream. This research addressed two questions; 1) Does the delivery of environmental water via an irrigation canal to Thule Creek create detectable pulses of carbon and nutrients in this ephemeral creek?, and 2) Is the input of carbon and nutrients from the ephemeral creek detectable in the receiving Wakool River system?
2 Methods
2.1 Study area
The Edward/Kolety-Wakool River system is a large anabranch of the Murray River in the southern MDB, Australia. It is a complex network of permanent rivers, inter-connecting streams, creeks, wetlands and ephemeral and intermittent rivers. The Edward/Kolety-Wakool region has an extensive network of irrigation canals and channels which in some places can be used to release water into rivers and creeks via irrigation canal escape infrastructure, hereafter referred to as “irrigation escapes”. The irrigation escapes are traditionally used to drain excess water from the irrigation system at the end of the irrigation season or in times of heavy rainfall or floods. In more recent times they have also been to deliver water from environmental accounts to rivers and creeks which have suffered reduced frequency of flows due to reduced flood frequency as a result of the heavily modified flow regime that now persists in the Edward/Kolety-Wakool River system (Watts et al., 2020). The floodplain landscape in the region is a mosaic of agricultural land (irrigation, cropping and pasture for grazing) and native vegetation, including large river red gum floodplain forests and other riparian vegetation along river corridors. Like many other rivers of the MDB, the flow regimes of the Edward/Kolety-Wakool system have been considerably changed by river regulation (Green, 2001; Hale and SKM, 2011; Murray-Darling Basin Authority, 2018). Under regulated flow conditions the number of small to medium overbank flows have decreased in winter and early spring, and there have been fewer opportunities for carbon and nutrients to be exported from the floodplain to receiving creeks and rivers.
Flows in the permanent rivers and tributaries of the Edward/Kolety-Wakool system remain within the channel under regulated conditions, whereas during high unregulated flow events there is connectivity between the river channels, floodplains and several large forests including the Barmah-Millewa Forest, Koondrook-Perricoota Forest and Werai Forest that together form the NSW Central Murray Forests Ramsar site (Department of Climate Change, Energy, the Environment and Water, 2013). As some of the rivers in the Edward/Kolety-Wakool system are highly regulated and have low base flows during summer there is a risk of poor water quality developing in this system, particularly during warm weather.
Thule Creek is one of the many ephemeral rivers within the Edward/Kolety-Wakool River system (Figure 1). Flows in Thule Creek are reliant on Koondrook-Perricoota Forest being inundated from the Murray River. Water from the flooded forest then drains into Thule Creek and then flows downstream to the Wakool River (Murray-Darling Basin Authority, 2012). As with many ephemeral creeks in the MDB, river regulation has severely reduced the frequency of flows in Thule Creek, which threatens the ecosystem of the creek. The frequency of overbank flows that connect the Koondrook-Perricoota Forest to Thule Creek has been reduced by at least 50% due to reduced overbank flows in the Murray River as a result of river regulation (Tuteja, 2008; State of New South Wales and Department of Planning, Industry and Environment, 2020). The method for determining the declined frequency of overbank flows in Koondrook-Perricoota Forest was based on a comparison between frequency of events (as defined by environmental water requirement in long term water plan) under modelled natural (without development) and modelled current condition flow regimes. Environmental water requirement achievement over the long term was evaluated through statistical analysis of modelled or observed flow records (State of New South Wales and Department of Planning, Industry and Environment, 2020). Koondrook-Perricoota Forest would have received more frequent flows under modelled natural regime, and thus Thule Creek would have received more frequent outflows from Koondrook-Perricoota Forest under natural flow conditions. Whereas Thule Creek only experienced flows once in the 5 years prior to this study (J. Dyer, personal communication, 4 August 2023). Through the use of an irrigation channel, environmental water has been delivered directly to Thule Creek to restore a component of the natural flow regime of this ephemeral system by reducing the duration of dry periods. The environmental flows delivered to Thule Creek are not continuous throughout the year, so thus there continues to be a wetting/drying regime in this ephemeral system.
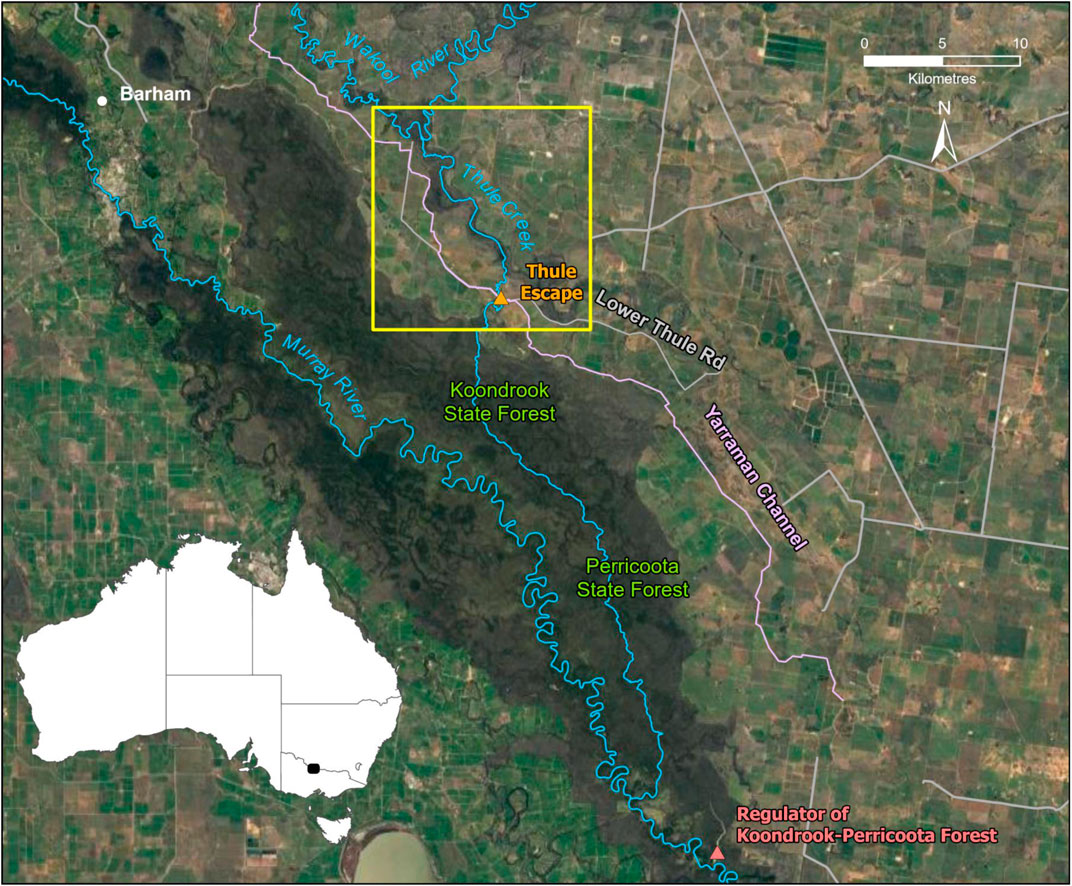
FIGURE 1. Yellow square indicates the location of the Thule Creek area within the mid-Murray River in the Murray–Darling Basin, Australia. (Source: ESRI, 2024).
Thule Creek and its associated fringing redgum wetlands areas provide a nursery and refuge habitat for a range of native fauna, particularly native fish and waterbirds that have significant cultural value in the area (Forestry Corporation of NSW, 2019). Thule Creek has been home to a relatively high diversity of small bodied native fish compared to other waterbodies in the area, including flathead gudgeon, carp gudgeon, Australian smelt, Murray rainbow fish and unspecked hardyhead (Gannon et al., 2019).
Ten sites were monitored for this study, including six in Thule Creek, three in the Wakool River, and one in Yarraman irrigation channel (source of environmental water) (Figure 2). Site 1 in Thule Creek was downstream of Perricoota Forest and upstream of the inflows of environmental water from Yarraman Channel. Sites 2 to 6 in Thule Creek were downstream of the environmental water delivery from Yarraman Channel. There is a small in-channel lagoon in Thule Creek between sites 2 and 3 and a larger in-channel lagoon in the creek between sites 3 and 4. Both of these lagoons were dry at the commencement of the environmental watering action. There was a sediment bank across Thule Creek between sites 5 and 6, that blocked the downstream flow of water until it was manually breached. In the Wakool River there was one monitoring site upstream of the Thule Creek confluence (Wakool upstream) and two sites in the Wakool River downstream of the Thule Creek confluence (Wakool downstream 1 and 2) (Figure 2).
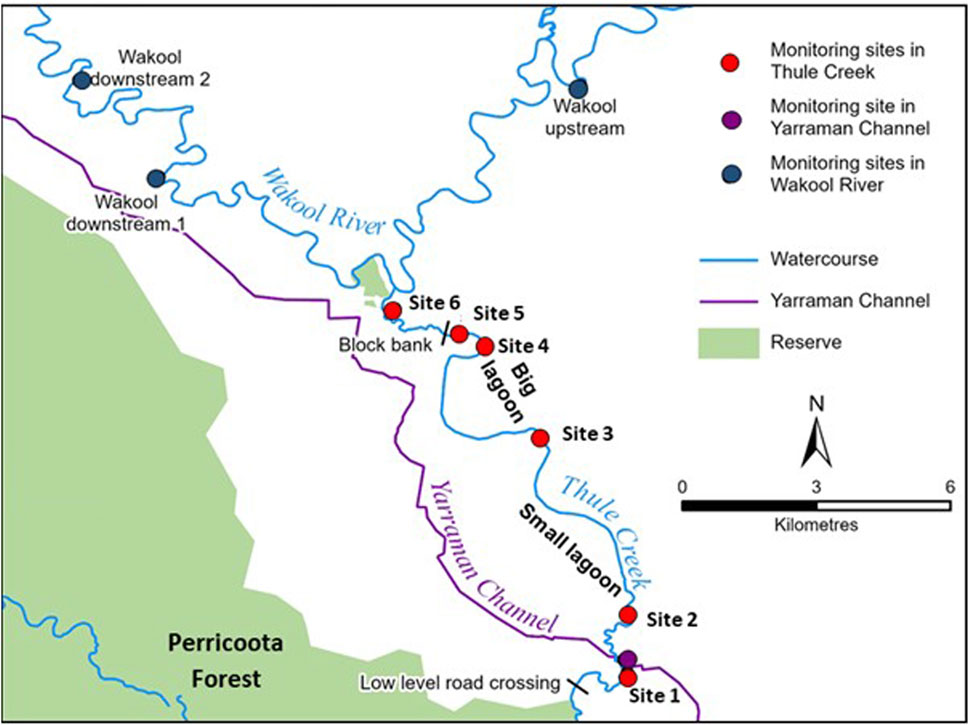
FIGURE 2. Location of monitoring sites along Thule Creek within the Edward/Kolety-Wakool River system. Numbered sites (red dots) show the six sites in Thule Creek from upstream to downstream. Dark blue dots are the three sites in Wakool River. The purple dot is the site within the Yarraman Channel (source water). The locations of a low-level road crossing, small and big lagoons and a block bank that influenced the progression of flow down Thule Creek are shown.
2.2 Environmental watering action
In many river systems, environmental water is released from dams to meet the requirements of water-dependant ecosystems further downstream. In the case of Thule Creek, environmental flows were delivered to the creek via an escape from a nearby irrigation channel, Yarraman Channel. In September 2019 Forestry Corporation of NSW and the NSW Department of Planning, Industry and the Environment (DPIE) planned an environmental watering action to deliver water via Thule Creek to Wakool River from Yarraman Channel via the Murray Irrigation Limited (MIL) Thule Escape (Commonwealth Environmental Water Office, 2019; Forestry Corporation of NSW, 2019).
The environmental watering action commenced to Thule Creek on 3rd October 2019 and ceased on 9th January 2020 (98 days) to achieve connectivity to the Wakool River and maximise wetted area in the wetland sections of the creek. Environmental flows ranged between 30 and 38 ML/day which was the maximum capacity of the Thule Escape, and total environmental water delivery was 3528.99 ML. Prior to the commencement of the environmental watering action to Thule Creek in 2019-20 there was a small refuge pool of water remaining in Thule Creek near site 1 from an environmental watering action delivered from the Thule Escape in 2018-2019. This refuge pool (hereafter referred to as the Thule Escape refuge pool) extended from upstream of the Lower Thule Road Bridge to approximately 3 km downstream of the Thule Escape, but it did not extend as far as the smaller lagoon on Thule Creek that was dry. There was also a pool of water in the lower section of Thule Creek backing up from Wakool River to just upstream of the Wakool-Barham Road Bridge. The bed of Thule Creek between these two pools was dry at the commencement of the environmental watering action.
2.3 Hydrological and weather data
Daily discharge (ML/day) and water level (m) for automated hydrometric gauges were obtained from the New South Wales Office of Water website (https://realtimedata.waternsw.com.au/water.stm). The daily discharge data for Thule Creek at Lower Thule Road was from gauge 409109. For this gauge there was no data for discharge available from 1/10/2019 and it is not representative of the Thule Escape flows as it is upstream of the escape with no flow. This gauge does however measure the effect of the escape deliveries on creek heights, so water level data have been used to describe hydrology. Daily discharge data for the release of water from the Thule Escape from Yarraman Channel Canal to Thule Creek was obtained from the Murray Irrigation Limited.
The temperature at weather station 80023 at Kerang, Victoria for the study period was obtained from the Australian Government Bureau of Meteorology website (http://www.bom.gov.au/climate/averages/tables/cw_080023.shtml).
2.4 Analysis of travel time of environmental flow down Thule Creek
The timing of the environmental water flowing down Thule Creek was documented through the analysis of Sentinel-2 satellite imagery from 3rd October 2019 to 11th March 2020. Initially the watercourse was digitised using satellite imagery and Light Detection and Ranging (LiDAR) (Digital Elevation Model, DEM derived) datasets as reference. Due to the very narrow width of channel in some parts of Thule Creek it was not possible to use an automated classification procedure to analyse the presence of water in the imagery. A basic imagery classification was to identify water presence and the imagery was manually assessed to determine the presence of water on each image. The pixels sizes of downloaded images ranged between 14 and 15 m. The analysis of imagery was undertaken by the Spatial Data Analysis Network (SPAN) at Charles Sturt University.
2.5 Sample collection and laboratory analysis
Collection of water samples and spot measures of water quality was undertaken once per week over a period of 15 weeks between mid-October 2019 and the end of January 2020. On all sampling dates water quality parameters (temperature (oC), electrical conductivity (mS/cm), dissolved oxygen (mg/L), pH, and turbidity (NTU)) were measured as spot recordings using a hand-held Horiba U-50 multi-parameter water quality meter.
Two replicate water samples were collected at each site on each sampling occasion. Water samples could not be collected from some sites on all sampling occasions, because some of the sites were dry. Water quality parameters used to monitor the water quality responses to the environmental watering actions included dissolved organic carbon (DOC), nutrients (phosphorus and nitrogen were measured in their total forms (total phosphorus (TP) and total nitrogen (TN)) and also in the soluble/bioavailable forms (filterable reactive phosphorus (FRP), ammonia (NH3) and nitrates + nitrites (NOx)) and Chlorophyll-a (Chl a).
Water samples for TP and TN were collected using pre-rinsed syringes into sterile jars. Water samples for DOC, FRP, NOx and NH3 were filtered through 0.2-µm pore-size membrane syringe filters into sterile jars at the time of sampling. The water samples for chlorophyll analysis were vacuum filtered through GF/C filter papers (Whatman®) and these were immediately wrapped in aluminium foil. Two replicate water samples for each indicator were collected at each site per week. All collected samples were stored on ice until returned to the laboratory and were frozen for analysis later. All water samples then were sent to the National Association of Testing Authorities (NATA) accredited laboratory at CSIRO, Albury, for analysis, thereby ensuring the integrity of data and analysis procedures.
Water samples for TP and TN determination were analysed by simultaneous digestion ultraviolet spectrometry using an oxidising regent solution of NaOH-K2S2O8 (Hosomi and Sudo, 1986). The analyses of NH3 and NOx were undertaken by using flow injection analysis and DOC analysis was undertaken by using high-temperature conversion to CO2 followed by infrared detection. Water samples for chlorophyll analysis were collected and filtered using the method proposed by APHA (American Public Health Association, 2005) and analysed using spectrophotometry.
The monitoring results were assessed against the trigger levels for aquatic ecosystems from the ANZECC (2000) water quality guidelines. If the concentration of a particular water quality parameter exceeded the trigger level or falls outside of the acceptable range, the guidelines are written with the intention that further investigation of the ecosystem is “triggered” to establish whether the concentrations are causing ecological harm. Systems may vary in their sensitivity to various parameters and therefore exceeding a trigger level is not an absolute indicator of ecological harm. It is quite common for water quality parameters to briefly fall outside of guideline values during periods of very high flow, this is not necessarily a sign of poor ecosystem health. The ANZECC water quality guidelines do not provide trigger levels for total organic carbon (TOC) and dissolved organic carbon (DOC), and this reflects the expectation that there will be large variation in the “normal” concentrations of organic carbon between ecosystems and also in the chemical and biological reactivity of the mixture of organic compounds making up the DOC and TOC at a particular site. Given the variable make-up of organic carbon, and the possible range of ecological responses to this mixture, a trigger level for this parameter would not be appropriate. However, trigger levels are provided for a number of nutrients and these are discussed below.
2.6 Data analysis
The influence of outflows from Thule Creek on the dissolved organic carbon and nutrients flowing into Wakool River was assessed by two qualitative comparison approaches, including the comparison of time series within each site and the comparison of sites on each sampling occasion in the Wakool River upstream and downstream of the Thule confluence.
3 Results
3.1 Hydrology
The water level in Thule Creek at site 1 at Lower Thule Road (gauge 409109), shows that there was water retained in the Thule Escape refuge pool prior to the commencement of the environmental watering action. The pool was slowly drying down as the air temperature increased during August and September 2019 (Figure 3). Environmental water released from Yarraman Channel from the Thule Escape to Thule Creek commenced on 3rd October 2019 and ceased on 9th January 2020. Water delivered from Yarraman Channel to Thule Creek was approximately 37 ML/day and was consistent at that rate for 98 days. On 3rd October 2019 when the watering action commenced, the water level at the gauge increased from 0.40 m to approximately 1.58 m and remained at this level until the watering action ceased and the pool started to slowly dry down (Figure 3). Between 1st September 2019 and 29th February 2020, the discharge in Wakool River at Wakool-Barham Road (gauge 409045) was average 332 ML/day (minimum 212 ML/day and maximum 482 ML/day).
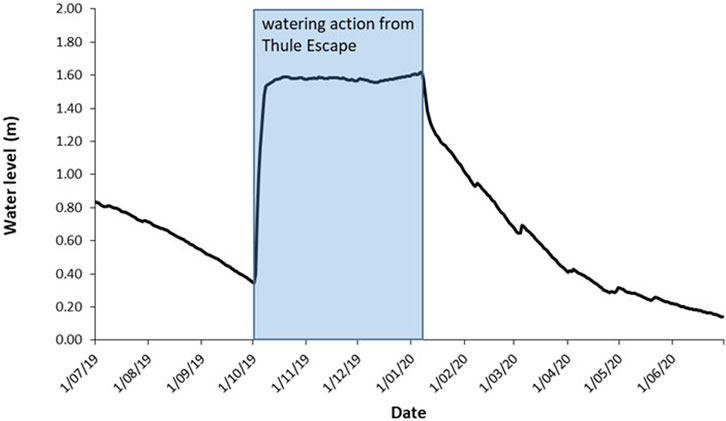
FIGURE 3. Water level (m) in Thule Creek at Lower Thule Road (gauge 409109) from 1 July 2019 to 30 June 2020. The shaded bar indicates the timing of the environmental watering action from Yarraman Channel via the Murray Irrigation Limited Thule Escape.
3.2 Air temperature
The daily maximum air temperature at Kerang, Victoria (weather station 80023) during the study period ranged from 16.3°C on 1st December to 46.6°C on 20th December 2019 (Figure 4).
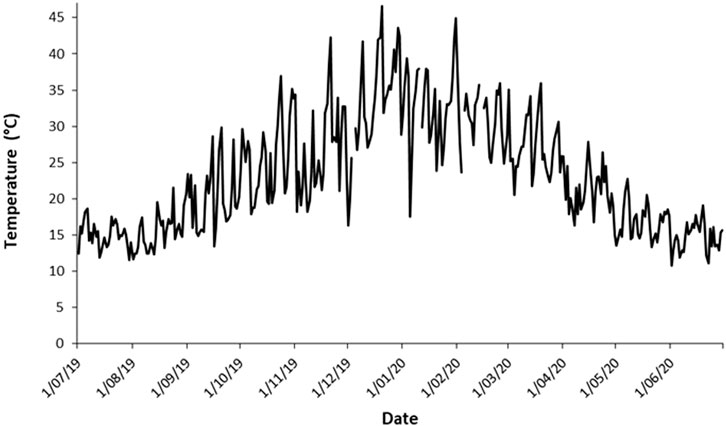
FIGURE 4. Daily maximum air temperature at Kerang, Victoria (weather station 80023) from 1 July 2019 to 30 June 2020 (Bureau of Meteorology, 2020).
3.3 Travel time of environmental flows down Thule Creek
The analysis of Sentinel-2 imagery showed that the environmental water took more than 80 days to travel approximately 11 km down the Thule Creek channel and reach the junction with Wakool River (Figure 5).
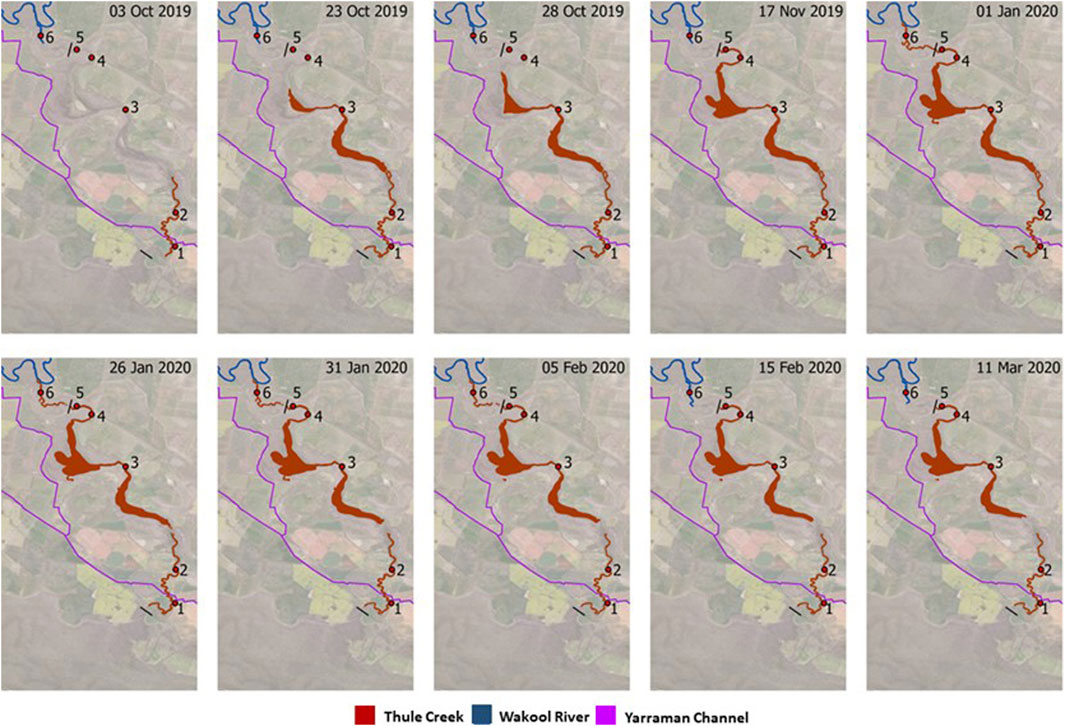
FIGURE 5. Annotated Sentinel-2 images showing changes in the presence of water in Thule Creek on selected dates from 3 October 2019 to 11 March 2020. Numbers indicate the location of six monitoring sites along Thule Creek. The blocked bank at site 5 influenced the flow of water down Thule Creek.
At the start of the watering action there was a remnant pool in Thule Creek near the escape (site 1), but the two in-channel lagoons downstream of the escape were dry. It took approximately 2 weeks for the water to reach and fill the smaller lagoon between sites 2 and 3 and approximately four more weeks to fill the larger second lagoon between sites 3 and 4. The progress of the water downstream to the Wakool River was obstructed by a block bank at site 5 for approximately a month until the bank was manually breached on 13th December 2019 to allow the flow to continue to flow downstream and connect with Wakool River (Figure 6). Approximately 1 week later, the environmental water connected with the Wakool River, between 19th and 26th December 2019. The real-time connection was not included in the Sentinel-2 images (Figure 5) due to extensive cloud cover making water detection impossible in images at that time. The water in Thule Creek started to dry back after the watering action ceased on 9th January 2020. Sentinel-2 imagery viewed on 1st August 2020 showed that water was retained in the two lagoons and in the refuge pool near the Thule Escape.
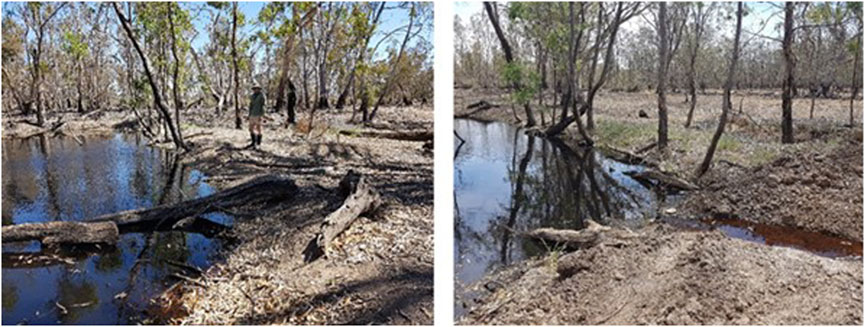
FIGURE 6. Left: Water was blocked by a bank in Thule Creek. Right: Water flowing over the blocked bank on 14th December 2019 after it was manually breached on 13th December 2019. (Photos Xiaoying Liu).
3.4 Dissolved organic carbon and nutrients in Thule Creek during and after the environmental watering action
The water in Yarraman Channel had DOC concentration between 4 and 5 mg/L throughout the study, which is in the normal range observed in this system (Watts et al., 2019). The water released from the Thule Escape increased in DOC concentration as it travelled downstream in Thule Creek (Figure 7). The concentration of DOC in water samples collected from sites 1 and 2 was similar to that from the Yarraman Channel. At sites 3, 4, and 5 the water inundated shallow areas of small red gum trees, grass, leaf litter and bare soil (Supplementary Figure S1). At site 3, downstream of the smaller in-channel lagoon, the concentration of DOC increased to 10–12 mg/L. The concentration of DOC increased to about 50 mg/L at site 4 after the water travelled through the larger in-channel lagoon. At site 5 it increased to concentration between 70 and 80 mg/L after the water had pooled in the creek for several weeks behind the blocked bank (Figure 7).
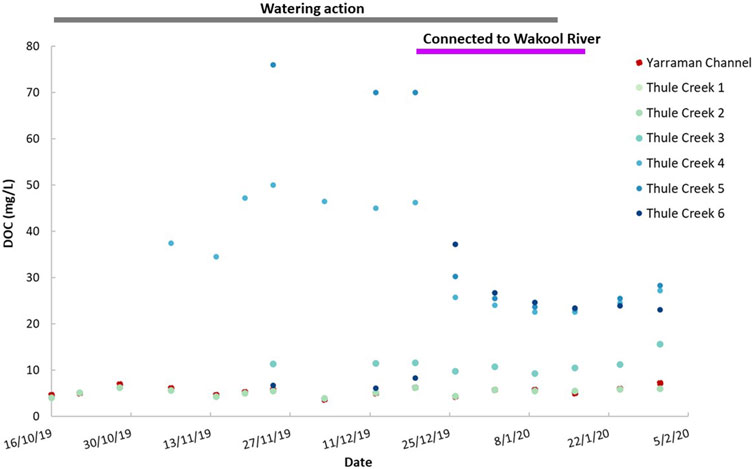
FIGURE 7. Dissolved organic carbon (DOC, mg/L) concentrations at all study sites in Thule Creek and Yarraman Channel from mid-October 2019 to the end of January 2020. The bold grey line indicates when environmental watering commenced and ceased, and the purple line indicates the period when Thule Creek was connected with the Wakool River.
The block bank between Thule Creek sites 5 and 6 was breached on 13th December 2019 enabling the water in Thule Creek to flow downstream through the dry creek bed that had a thick cover of leaf litter and sticks which had accumulated for 3 years since the last major flow event (Supplementary Figure S2). The soil was very dry and the leading edge of the flow progressed slowly downstream. The colour of the water at the leading edge of the flow was extremely dark (Supplementary Figure S3, top), suggesting it contained a very high concentration of DOC.
After the water commenced to flow downstream the concentration of DOC at sites 4 and 5 reduced to between 30 and 40 mg/L and the colour of the water became lighter (Supplementary Figure S3, bottom). At the end of December 2019 when the Thule Creek flow connected with the Wakool River, the concentration of DOC at Thule Creek site 6 increased and was similar to Thule Creek sites 4 and 5 (Figure 7). On 26th January 2020 when the water ceased to flow downstream and the air temperature was over 35°C, the concentration of DOC at sites 3, 4, and 5 started to increase (Figure 7).
Similar to the patterns of DOC concentration, the water released from the MIL Thule Escape increased in concentration of nutrients as it travelled downstream. There were particularly high concentrations of nutrients at sites 4 and 5 (Figure 8) after the water had travelled through the larger lagoon and resided in the system for several weeks behind the block bank.
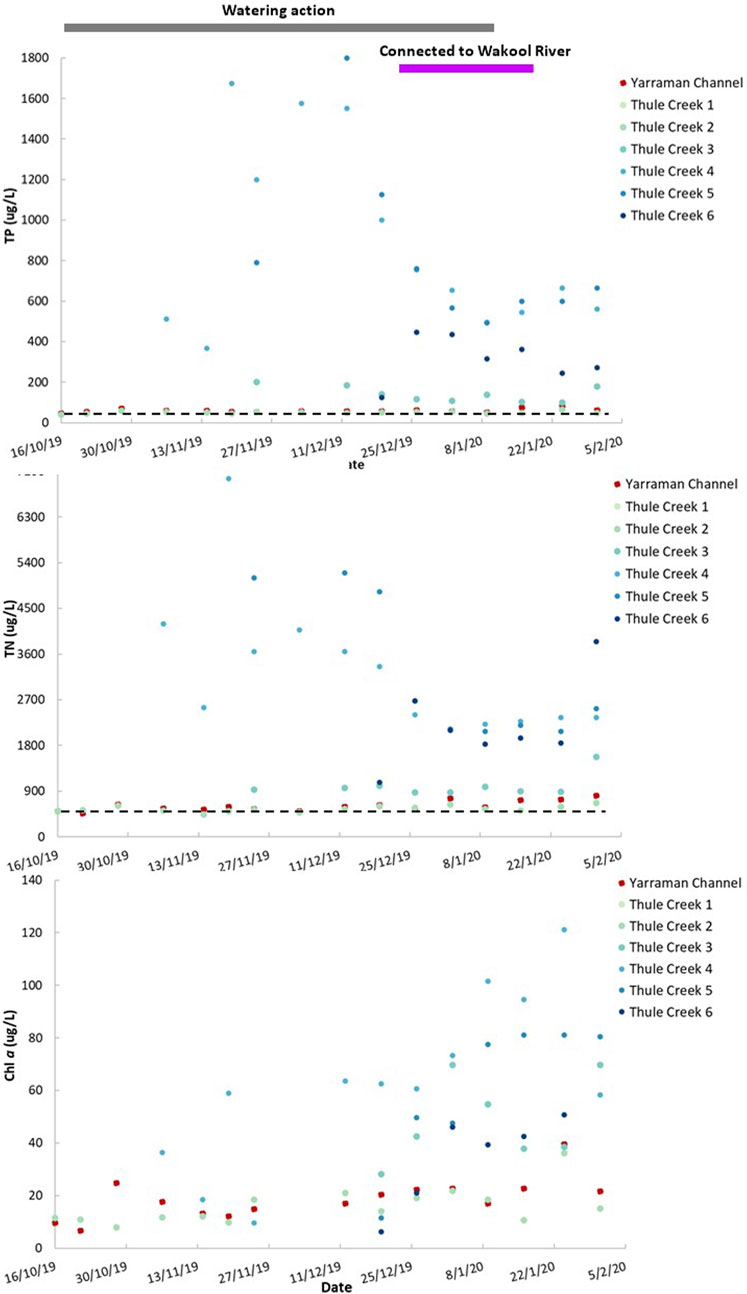
FIGURE 8. Total phosphorus (TP), total nitrogen (TN) and chlorophyll a (Chl a) concentrations (µg/L) at sites in Thule Creek and Yarraman Channel from mid-October 2019 to the end of January 2020. The bold grey line indicates when environmental watering commenced and ceased, the purple line indicates the period when Thule Creek was connected with the Wakool River, and the black dashed lines indicate ANZECC trigger values for TP and TN.
Total phosphorus (TP, 45–86 μg/L) was at low concentrations in the source water from Yarraman Channel. TP concentrations were elevated at downstream sites of Thule Creek during the watering action, suggesting a considerable amount of phosphorous was leached from soil, leaf litter and organic matter as the water flowed down the Thule Creek channel. Most of the TP was released between site 4 (368–1,675 μg/L) and site 5 (493–1,800 μg/L) and highest TP concentration was measured at site 5 on 19th November 2019 with a concentration of 1800 μg/L (Figure 8). The pattern observed for TN was similar to TP. The total nitrogen concentration (TN, 465–815 μg/L) was low in the source water from Yarraman Channel. The majority of TN was leached between site 4 (2,125–7,050 μg/L) and site 5 (2,075–5,200 μg/L) (Figure 8). During and after the watering action, TP and TN concentrations at most of the study sites were above the ANZECC trigger value of 50 μg/L and 500 μg/L respectively.
Chlorophyll a (Chl a) concentrations were closely associated with nutrient concentrations. At sites 4 and 5 concentrations of Chl a were considerably higher than all other sites in the study area and increased over time as water temperature was increased over December 2019 and January 2020 (Figure 8).
3.5 Dissolved organic carbon and nutrients in the Wakool River during and after connection with outflow of Thule Creek
The outflow of Thule Creek connected with the Wakool River water between 19th and 26th December 2019, resulting in a carbon-rich pulse detected in the Wakool River system (Figure 9). The concentration of DOC in the Wakool River downstream of Thule Creek increased in early January 2020 when the DOC-rich water from Thule Creek was flowing into Wakool River. DOC in the Wakool River downstream of Thule Creek, Wakool River downstream site 1 was 10.1 mg/L and Wakool River downstream site 2 was 8.3 mg/L compared to the site in the Wakool River upstream of Thule Creek confluence with 6.6 mg/L on 2nd January 2020. On 9th January the DOC concentration at Wakool River downstream site 1 (7.3 mg/L) and Wakool River downstream site 2 (7.6 mg/L) continued to be higher than that observed at Wakool River upstream site (5.2 mg/L).
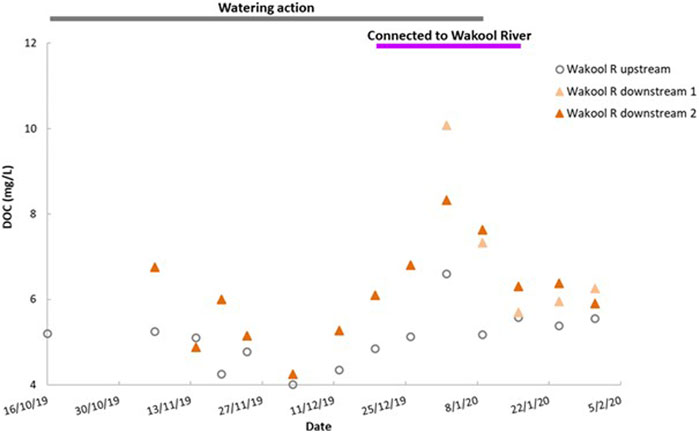
FIGURE 9. Dissolved organic carbon (DOC, mg/L) concentrations at sites in Wakool River from mid-October 2019 to the end of January 2020. The bold grey line indicates when environmental watering commenced and ceased, and the purple line indicates the period when Thule Creek was connected with the Wakool River.
There was a noticeable difference in the colour of the carbon-rich Thule Creek water and the turbid Wakool River water at the junction of these two systems (Supplementary Figure S4). On 16th January there was no difference in DOC concentration between the upstream and downstream Wakool River sites, as flows from Thule Creek to Wakool River had stopped flowing.
The flow in Thule Creek connected with Wakool River resulting in a nutrient-rich pulse detected in the Wakool River downstream of Thule Creek compared to the Wakool River upstream of Thule Creek (Figure 10). For example, on 2nd January 2020, TP and TN at the Wakool River downstream site 1 of Thule Creek confluence were 94 μg/L and 1,125 μg/L at Wakool River downstream site 2 were 103 μg/L and 980 μg/L compared to the Wakool River site upstream of Thule Creek with 64 μg/L and 805 μg/L. Higher Chl a levels were measured at the Wakool River downstream site 1 of Thule Creek with 44.2 μg/L and Wakool River downstream site 2 with 45.6 μg/L, compared to the Wakool River site upstream of Thule Creek with 29.6 μg/L when flow from Thule Creek connected with Wakool River.
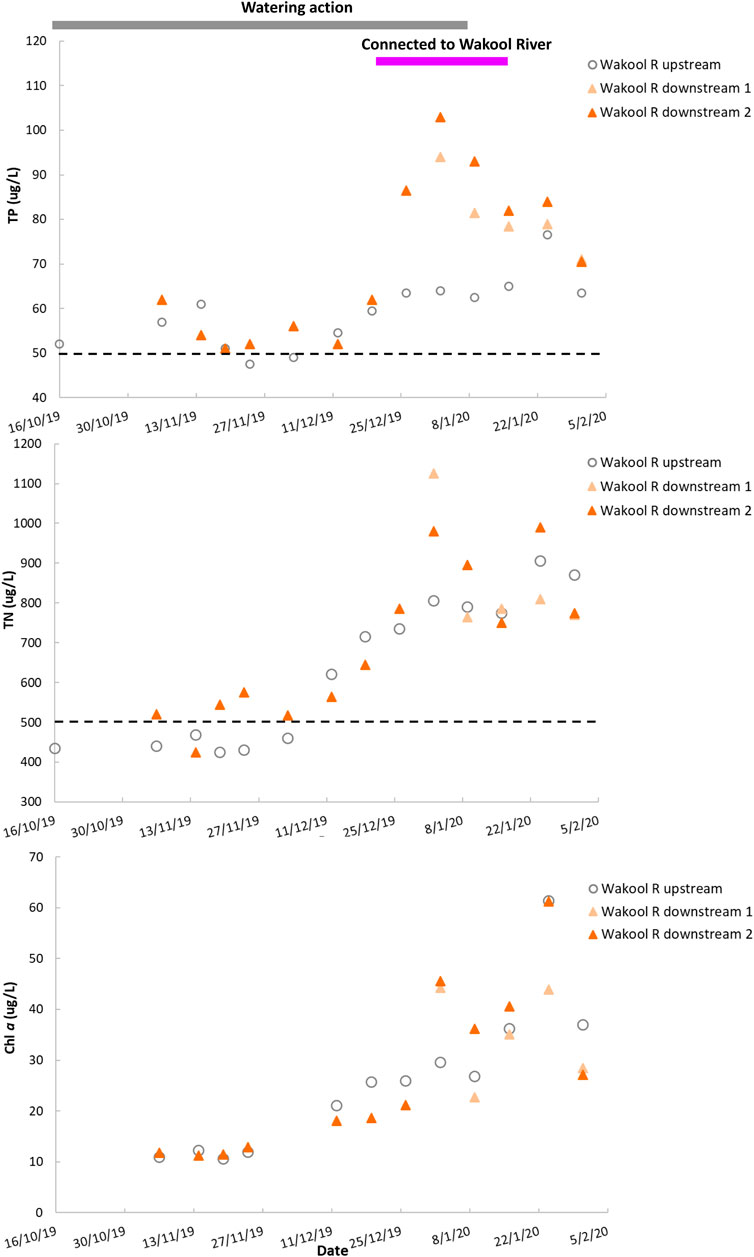
FIGURE 10. Total phosphorus (TP), total nitrogen (TN) and chlorophyll a (Chl a) concentrations (µg/L) at sites in Wakool River from mid-October 2019 to the end of January 2020. The bold grey line indicates when environmental watering commenced and ceased, the purple line indicates the period when Thule Creek was connected with the Wakool River, and the black dashed lines indicate ANZECC trigger values for TP and TN.
There was minimal difference in nutrient concentrations between the upstream and downstream Wakool River sites on 16th January 2020, as flows from Thule Creek to Wakool River had stopped flowing.
3.6 Dissolved oxygen
Spot dissolved oxygen (DO) measures were taken at different times of the day. The time of day will affect DO readings: early morning spot measures of DO are often lower than measurements taken at the same site later in the day. While these measurements do not provide the same high resolution indication of changes with time, the data presented here provide an indication of the extent of hypoxia at each site at the time of sampling.
The DO concentration was less than 4 mg/L (threshold for fish stress, Gehrke, 1988) between sites 3 and site 6 in Thule Creek during the environmental watering action. At times the concentration of DO at sites 4 and 5 dropped into the range of lethal to fish populations (below 2 mg/L) (Table 1). On 8th January 2020 before the environmental watering action ceased, at most sites the DO concentrations were above 4 mg/L and DO levels at sites 3 and 4 were above 2 mg/L which were a little higher (better) than observed in previous weeks. However, after the Thule Creek ceased to flow on 9th January 2020 and air temperatures were very high, the DO levels at sites 3, 4, and 5 were below 2 mg/L again.
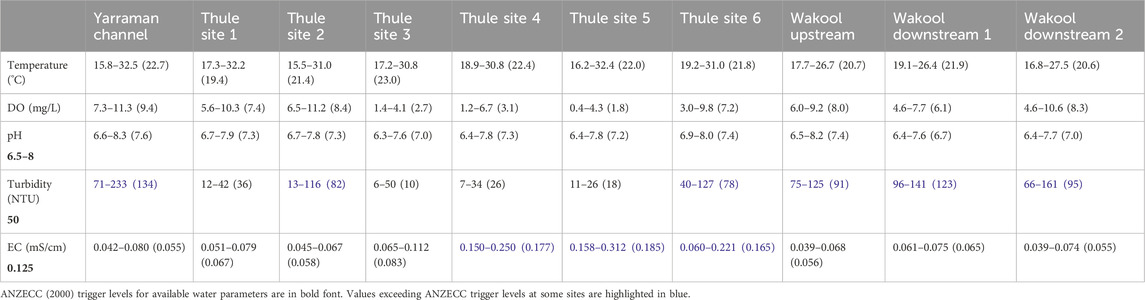
TABLE 1. Range and mean values of water physico-chemical parameters measured using handheld water quality meter for all study sites in Yarraman Channel, Thule Creek and Wakool River from mid-October 2019 to the end of January 2020. Mean values are shown in brackets.
In general, a 3–4°C increase in water temperature was observed at all sites on 12th December 2019 coinciding with the time when rapid onset of hypoxia was observed. Temperatures at all sites were similar although wider daily fluctuations were recorded at sites in Thule Creek than at the sites in the Wakool River. This may have been due to the shallower water depth at the sites in Thule Creek.
4 Discussion
4.1 Outcomes of delivery of environmental water from an irrigation canal to Thule Creek
The inundation of a range of floodplain land uses (including redgum forests, crops, pastures and bare soil) during floods can be a major source of DOC and nutrients and a major contributor to dissolved oxygen depletion in rivers (Liu et al., 2019). Drying of sediments followed by re-wetting events in inland ephemeral systems can lead to a flush of nutrients (such as phosphorus and nitrogen) released to the water column (Twinch, 1987; De Groot and Van Wijck, 1993; Qiu and McComb, 1994; Mitchell and Baldwin, 1998; Liu, 2017). The results of detected flushes of DOC and nutrients in the Thule Creek channel support our hypothesis that a considerable amount of carbon and nutrients would be leached into the water column as during the environmental flow as the water flowed down Thule Creek.
Previously studies have shown that large amounts of organic matter accumulated in the bed of ephemeral rivers in the period preceding inundation can be mobilised by the high flow events (Sabo et al., 1999; Junk and Wantzen, 2004; Kobayashi et al., 2009). In our study we observed that a substantial amount of organic matter had accumulated on the bed of Thule Creek over the preceding years the creek had been dry. As sites 3 to 6 in Thule Creek (the lower part of the creek) had not been flooded or inundated since 2016, substantial inputs of DOC and nutrients derived from small red gum trees, grass, leaf litter and bare soils were released to the water column during the delivery of environmental water. For example, although the DOC concentration in the water released from Yarraman Channel was in the normal range observed in this system (Watts et al., 2019), the DOC concentration increased considerably after the water had travelled downstream and inundated shallow areas within in the Thule Creek channel.
A study conducted to examine the contribution of water delivery through Koondrook-Perricoota Forest to the productivity of Wakool River in spring of 2016 (Watts et al., 2017b) recorded DOC values ranging from 10 to 32.5 mg/L in Thule Creek. The carbon and nutrients concentrations observed in current study were even more elevated compared to the results of the 2016 study, but were similar to results of a glasshouse experiment where DOC was examined following inundation of soil and vegetation from a lowland river floodplain (Liu et al., 2019), simulating the leaching of carbon in standing water on a floodplain. Both the results of the glasshouse experiment and the current study suggest that high concentrations of DOC can develop when water flow is standing on the floodplain or, in the case of the current study, held up behind a block bank that obstructed the flow in the creek. In contrast, when the water is flowing through a system the DOC can increase but not to the same extent as in standing water.
Blackwater occurs when large quantities of organic material (carbon-based substances), such as sticks, leaves, grass or crops are washed off the floodplain and into rivers and creeks resulting in a dark tea color in the water. Blackwater becomes hypoxic (low dissolved oxygen concentration) when large amounts of organic material in rivers are broken down by bacteria, consuming dissolved oxygen in the water (Department of Climate Change, Energy, the Environment and Water, 2023). The likelihood of the occurrence of hypoxic blackwater events is increased if a large volume of organic matter has built-up on the floodplain or water temperatures are high. The high water temperatures at the time of this study may have accelerated respiration beyond the capacity of the system to maintain DO in the water column, combined with lower solubility at higher temperatures (Howitt et al., 2007; Kerr et al., 2013; Whitworth et al., 2014; Pasco et al., 2016). DO concentrations below the 2 mg/L threshold associated with fish deaths (Gehrke, 1988) occurred only at sites where water was held up behind the block bank with high DOC concentrations. Thus, the poor water quality was only in a relatively small section of Thule Creek for a brief period during very hot weather conditions. A study of fish monitoring was done in the refuge pool near site 1 prior to this study and it has documented fish community in Thule Creek (Gannon et al., 2019) and the landholder who owned the property at sites 3 and 4 had noted fish moving around in the water in Thule Creek because the water was clear and fish could be seen through the water. Despite the high DOC concentration and hot weather, the researchers and landholders did not observe any dead fish in Thule Creek at any time during the study.
After the blocked bank was breached and water started to flow downstream to Wakool River, the DO concentrations at most sites were a little higher than in the previous weeks, possibly because the blackwater was diluted by the canal source water. Furthermore, there may have been less carbon leaching out of the soil and grasses as the creek had already been wet for several weeks. Thus, the risk to fish was localised in the vicinity of Thule site 5 and the increased connectivity from the flow action provided fish with the opportunity to move upstream to a section of the creek that had higher DO concentration near the Thule Escape, or downstream to higher DO water in Wakool River.
4.2 Contribution of delivery of environmental water via an ephemeral system to Wakool River
In the current study, the carbon-rich and nutrient-rich water was detected in the Wakool River downstream of Thule Creek after the environmental flow in Thule Creek connected with Wakool River. The discharge in Wakool River was sufficient to dilute the inputs from Thule Creek in hot weather, so there was no evidence of low DO in Wakool River that would increase the risk of the development of hypoxia or fish deaths. Excessive inputs of nutrients and organic carbon can result in poor water quality with the development of algal blooms or hypoxic blackwater events resulting in very low DO concentrations (Howitt et al., 2007; Hladyz et al., 2011). Particularly during hot weather, the temperature affects the rates of microbial processes and organic matter leaching (Howitt et al., 2007; Whitworth et al., 2014). In 2016 there was a large flood in the southern Murray-Darling Basin that resulted in hypoxic blackwater events that extended throughout the Murray River system, including the Edward/Kolety-Wakool River system. Fish kills were reported in many areas with very low DO levels because large amounts of floodplain-derived carbon and nutrients were present in the water throughout the system (Watts et al., 2017a; 2018). The main issue with that large hypoxic event was that it was extremely widespread throughout the Murray River system and there were very few refuges having higher DO concentration that fish could migrate to.
In contrast, during the environmental watering action in 2019, only a short length of Thule Creek experienced high DOC and low DO concentrations, thus fish and other aquatic organisms had the opportunity to move upstream or downstream to areas where there was water with higher concentration of DO. This suggests that when delivering environmental water to create pulses of carbon to improve productivity it is essential to maintain connectivity with other parts of the system to allow fish and other aquatic organisms to move to reaches having better water quality.
Inputs of nutrients and organic carbon can have a positive influence on river systems through the stimulation of productivity and increased food availability for downstream (Robertson et al., 1999). Studies have shown that the connection between a river and its floodplain can create essential carbon stores to sustain the system through drier periods (Baldwin et al., 2013). Our study indicates that the delivery of environmental flows via the Thule Creek resulted in the addition of nutrients and organic carbon that could support microbial productivity and create food for aquatic organisms such as fish in the Wakool River.
The delivery of environmental flows has been shown to provide ecological benefits to permanent river systems and associated wetlands. Environmental flows to permanent river systems have been delivered to help prevent loss of critical taxa from droughts by creating refuges (Rayner et al., 2009), to promote hydrological connection and primary productivity (Chester and Norris, 2006), support spawning activity and recruitment of fish (King et al., 2010), sustain breeding of colonial waterbirds (Kingsford and Auld, 2005) and flush excessive growth of nuisance biofilms (Watts et al., 2010) by creating pulsed flows or flood events. Our study shows that a small discharge of environmental water delivered to a previously dry ephemeral river can result in the release of carbon and nutrients from leaves, grass and bare soils from the ephemeral waterway and influence the productivity of a receiving permanent river system. This possibly achieved a greater response than could have been achieved by delivering the same small amount of environmental water to a permanently river.
Our study suggests that careful planning of timing, magnitude and duration of delivery of environmental water through Thule Creek could accelerate ecosystem processes and increase the input of floodplain-derived carbon and nutrients to the Wakool River when it is under regulated flows, but at the same time minimise the risk of hypoxia, particularly in hot weather.
4.3 Other ecological benefits of the environmental watering action in Thule Creek
Environmental flows to Thule Creek also provided other visually assessed ecological benefits. Apart from facilitating nutrient and carbon transport between Thule Creek and the Wakool River there were several other ecosystem responses observed during the watering action. Along Thule Creek channel, the water was very clear with low turbidity, and there was a large amount of productivity. There were a high abundance of aquatic plants emerging on the riverbed that could be seen through the clear water. In amongst the plants there a high abundance of aquatic invertebrates observed in the shallow edges of the creek, suggesting it was creating a pulse of “fish food” as the flow moved downstream. The response of the riparian vegetation along the creek was also very notable, with trees adding a thick cover of new leaves. In addition, a lot of waterbirds were observed including pelicans, darters and cormorants in Thule Creek, and there were over 50 colonial waterbird nests observed by researchers, including darters nesting at the small lagoon and little black cormorants nesting in thick red gum regrowth between the two lagoon sites. Thus, the delivery of the environmental water to Thule Creek was visually observed to improve the condition of riparian vegetation, stimulate emergence and growth of aquatic plants, increase aquatic invertebrate activity, and support waterbird feeding and nesting in the Thule Creek channel. There were no fish kills observed during the environmental flow, and fish were observed moving around at sites in the creek.
4.4 Using irrigation infrastructure to deliver environmental flows to ephemeral rivers
In the Edward/Kolety-Wakool River system, the presence of an extensive irrigation canal network infrastructure provides an opportunity to deliver environmental water to ephemeral creeks. In this study the environmental flow from Yarraman Channel via the Murray Irrigation Limited (MIL) Thule Escape continued for 98 days and it took more than 80 days for the water to travel down Thule Creek and reach the junction with Wakool River. The reason the flow took a long time to reach the Wakool River was due to the very flat landscape, small-scale environmental watering action, some of the water soaking into the dry creek bed along the way, and hot weather resulting in high rate of water evaporation. Despite the long travel time, the environmental flow in Thule Creek did reach the Wakool River and created a small pulse of carbon and nutrients, contributing to the productivity of that system.
In May/June 2019 an upgrade to the MIL Thule Escape was completed, increasing the discharge that can be released from 30 ML/day to 120 ML/day. This would considerably reduce travel times between the Thule Escape and the Wakool River, reducing time for hypoxia to develop. The upgrade on MIL infrastructure to increase the water delivery capacity provides an opportunity to increase the discharge in Thule Creek of future environmental watering actions, thus increasing the opportunity for water managers to manage water deliveries to increase carbon inputs to stimulate productivity and at the same time avoid the risk of hypoxia. Further flow trials could be undertaken under different conditions to improve our understanding of how to balance the flows from Koondrook-Perricoota Forest regulator and the MIL Thule Escape with flows in Wakool River to achieve the best outcomes for the river ecosystem.
One challenge in many arid or semi-arid landscapes is that there will be limited options to deliver environmental water to ephemeral systems (Hughes, 2005). It may not be possible to create an environmental flow of sufficient discharge in permanent river systems that will connect to ephemeral or intermittent tributaries. The use of irrigation canal network infrastructure is an option that can be explored and trialled elsewhere worldwide.
This study focussed on hydrology and water chemistry outcomes of the environmental flow, combined with a visual assessment of ecological benefits. Future similar studies would be benefit from a more comprehensive integrated evaluation, including foodweb and ecological responses to environmental flows, to extend our knowledge of ephemeral river systems.
5 Conclusion
Due to the high evaporation rates of ephemeral systems in semi-arid and/or arid areas, careful management of the timing, volume and duration of environmental flows is required to avoid the development of poor water quality during, or following, the delivery of environmental water. This research provides an example of how an environmental flow delivered via an irrigation canal network infrastructure provided ecosystem benefits to an ephemeral creek and also contributed to productivity of a permanent river further downstream. The flow in the Wakool River at the time was sufficient to dilute the inputs from Thule Creek so at no time was there any risk of hypoxia or fish deaths. In general, the outcome of this small blackwater pulse was positive rather than negative. The small pulse increased hydrological connectivity and also improved vegetation condition including fringing vegetation and emergent/submerged aquatic plants in Thule Creek. Despite there being some sections of the creek that had low DO levels, no fish deaths were observed in Thule Creek. The pulse also provided opportunities for movement, reproduction and recruitment of invertebrates, frogs and native fish and other propagules.
Historically, before river regulation and agricultural modifications to the landscape, ephemeral systems would have flowed during large unregulated flow events. However, many of these ephemeral systems are now disconnected for extended periods of time from the rivers and permanent creeks that would have once supplied them with water and, without environmental water, would be dry in most years. In many landscapes there will be limited options to deliver environmental water to ephemeral systems. This research provides an example of the important contribution of delivering environmental water from irrigation canal network infrastructures toward promoting instream productivity in ephemeral creeks and permanent rivers and preventing loss of critical taxa. Water delivery options should be explored to find ways to increase river-floodplain connectivity and increase river productivity through the input of small pulses of dissolved inorganic and organic matter as seen during this study.
Data availability statement
The original contributions presented in the study are included in the article/Supplementary Material, further inquiries can be directed to the corresponding author.
Author contributions
XL, RW, and JD contributed to design of the study. XL wrote the first draft of the manuscript. All authors contributed to the article and approved the submitted version.
Acknowledgments
Authors acknowledge the funding of this project by the Forestry Corporation of NSW with in-kind contributions from Charles Sturt University. Thanks to Linda Broekman and Sarah Treby (Forestry Corporation NSW) for project management. Thanks to landholders who own properties along Thule Creek and Wakool River for providing access to the waterways for collection of water samples. Thanks to Sam Brouwer, Dale Campbell, Reg Griffiths, Jarryd McGowan, Cameron McGregor, Matt Pihkanen, Allen Brook, Mitchell Cowan and John Trethewie for their assistance with field sampling. Maps and Sentinel-2 imagery analysis was undertaken by Craig Poynter and Deanna Duffy, Charles Sturt University Spatial Data Analysis Network. Carbon and nutrient samples were analysed by John Pengelly, NATA Laboratory, CSIRO Land and Water, Albury.
Conflict of interest
The authors declare that the research was conducted in the absence of any commercial or financial relationships that could be construed as a potential conflict of interest.
Publisher’s note
All claims expressed in this article are solely those of the authors and do not necessarily represent those of their affiliated organizations, or those of the publisher, the editors and the reviewers. Any product that may be evaluated in this article, or claim that may be made by its manufacturer, is not guaranteed or endorsed by the publisher.
Supplementary material
The Supplementary Material for this article can be found online at: https://www.frontiersin.org/articles/10.3389/fenvs.2024.1213001/full#supplementary-material
SUPPLEMENTARY FIGURE S1 | Inundated vegetation and organic matter in Thule Creek during delivery of environmental water. (Photos Xiaoying Liu).
SUPPLEMENTARY FIGURE S2 | Leading edge of the water flowing downstream of site 5 block bank on 14th December 2019 (left) and 19th December 2019 (right). (Photos Xiaoying Liu).
SUPPLEMENTARY FIGURE S3 | Water samples collected on 19th December 2019 (top) and 8th January 2020 (bottom), showing color differences of sampling sites on two dates during the environmental watering action. (Photos Xiaoying Liu).
SUPPLEMENTARY FIGURE S4 | Photo taken at the junction of Thule Creek and Wakool River on 2nd January 2020. The darker coloured carbon rich water from Thule Creek on the left of the photo is flowing into the turbid water in the Wakool River on the right. (Photo Xiaoying Liu).
References
ANZECC (2000). Australian and New Zealand guidelines for fresh and marine water quality. Sydney, NSW, Australia, and Auckland, New Zealand: Australian and New Zealand Environment and Conservation Council, Agriculture and Resource Management Council of Australia and New Zealand.
APHA (2005). Standard methods for the examination of water and wastewater. Washington DC, USA: American Public Health Association/American Water Works Association/Water Environment Federation.
Baldwin, D., Rees, G., Wilson, J., Colloff, M., Whitworth, K., Pitman, T., et al. (2013). Provisioning of bioavailable carbon between the wet and dry phases in a semi-arid floodplain. Oecologia 172 (2), 539–550. doi:10.1007/s00442-012-2512-8
Brock, M. A., Nielsen, D. L., Shiel, R. J., Green, J. D., and Langley, J. D. (2003). Drought and aquatic community resilience: the role of eggs and seeds in sediments of temporary wetlands. Freshw. Biol. 48 (7), 1207–1218. doi:10.1046/j.1365-2427.2003.01083.x
Bunn, S. E., and Arthington, A. H. (2002). Basic principles and ecological consequences of altered flow regimes for aquatic biodiversity. Environ. Manag. 30, 492–507. doi:10.1007/s00267-002-2737-0
Bureau of Meteorology (2020). Climate statistics for Kerang (station 080023), Victoria, Australia. Available at: http://www.bom.gov.au/climate/averages/tables/cw_080023.shtml.
Capon, S. J. (2003). Plant community responses to wetting and drying in a large arid floodplain. River Res. Appl. 19 (5-6), 509–520. doi:10.1002/rra.730
Chester, H., and Norris, R. (2006). Dams and flow in the cotter river, Australia: effects on instream trophic structure and benthic metabolism. Hydrobiologia 572, 275–286. doi:10.1007/s10750-006-0219-8
Commonwealth Environmental Water office (2019). Southern spring flow 2019 fact sheet. Commonwealth of Australia. https://www.environment.gov.au/water/cewo/publications/southern-spring-flow-2019-fact-sheet.
Datry, T., Bonada, N., and Boulton, A. J. (2017). Intermittent rivers and ephemeral streams, Ecology and management. Cambridge, Massachusetts, United States: Academic Press.
De Groot, C. J., and Van Wijck, C. (1993). The impact of desiccation of a freshwater marsh (Garcines Nord, Camargue, France) on sediment-water-vegetation interactions. Hydrobiologia 252 (1), 83–94. doi:10.1007/BF00000130
Deil, U. (2005). A review on habitats, plant traits and vegetation of ephemeral wetlands—a global perspective. Phytocoenologia 35 (2-3), 2–3. doi:10.1127/0340-269X/2005/0035-0533
Department of Climate Change Energy the Environment and Water (2013). Australia’s ramsar sites. Accessed: https://www.dcceew.gov.au/water/wetlands/publications/factsheet-australias-ramsar-sites.
Department of Climate Change Energy the Environment and Water (2023). Low-oxygen blackwater and water for the environment. Retrieved on: https://www.dcceew.gov.au/water/cewo/catchment/hypoxic-blackwater#:∼:text=What%20is%20low%2Doxygen%20blackwater,water%20%E2%80%93%20oxygen%20that%20fish%20need.
Dudgeon, D., Arthington, A. H., Gessner, M. O., Kawabata, Z. I., Knowler, D. J., Lévêque, C., et al. (2006). Freshwater biodiversity: importance, threats, status and conservation challenges. Biol. Rev. 81 (2), 163–182. doi:10.1017/S1464793105006950
Finlayson, C. M., Davidson, N., Pritchard, D., Milton, G. R., and MacKay, H. (2011). The Ramsar Convention and ecosystem-based approaches to the wise use and sustainable development of wetlands. J. Int. Wildl. Law Policy 14 (3-4), 176–198. doi:10.1080/13880292.2011.626704
Forestry Corporation of NSW (2019). Banked water delivers crucial flows to Koondrook-Perricoota State Forests. https://www.forestrycorporation.com.au/about/releases/2019/banked-water-delivers-crucial-flows-to-koondrook-perricoota-state-forests.
Gannon, R., Wood, D., Young, C., and Whiterod, N. (2019). Baseline survey of fish communities in Thule Creek and Pollack Lagoon to inform wetland management. Hindmarsh Valley, Australia: Aquasave-NGT.
Gehrke, P. C. (1988). Response surface analysis of teleost cardiorespiratory responses to temperature and dissolved oxygen. Comp. Biochem. Physiology, A 89 (4), 587–592. doi:10.1016/0300-9629(88)90837-7
Graf, W. L., and Lecce, S. A. (1988). Fluvial processes in dryland rivers. New York, NY, USA: Springer-Verlag.
Green, D. (2001). The Edward-Wakool system: river regulation and environmental flows. Unpublished report. Deniliquin, NSW: Department of Land and Water Conservation.
Hale, J.SKM (2011). “Environmental water delivery: Edward-Wakool,” in Prepared for commonwealth environmental water, department of sustainability, environment, water, population and communities (Parkes, Australia: Department of Climate Change, Energy, the Environment and Water).
Harris, J., Kingsford, R., Peirson, W., and Baumgartner, L. (2017). Mitigating the effects of barriers to freshwater fish migrations: the Australian experience. Mar. Freshw. Res. 68, 614–628. doi:10.1071/MF15284
Hladyz, S., Watkins, S. C., Whitworth, K. L., and Baldwin, D. S. (2011). Flows and hypoxic blackwater events in managed ephemeral river channels. J. Hydrology 401 (1-2), 117–125. doi:10.1016/j.jhydrol.2011.02.014
Hosomi, M., and Sudo, R. (1986). Simultaneous determination of total nitrogen and total phosphorus in freshwater samples using persulfate digestion. Int. J. Environ. Stud. 27 (3-4), 267–275. doi:10.1080/00207238608710296
Howitt, J. A., Baldwin, D. S., Rees, G. N., and Williams, J. L. (2007). Modelling blackwater: predicting water quality during flooding of lowland river forests. Ecol. Model. 203 (3-4), 229–242. doi:10.1016/j.ecolmodel.2006.11.017
Hughes, D. A. (2005). Hydrological issues associated with the determination of environmental water requirements of ephemeral rivers. River Res. Appl. 21, 899–908. doi:10.1002/rra.857
Junk, W. J., Bayley, P. B., and Sparks, R. E. (1989). The flood pulse concept in river-floodplain systems. Can. special Publ. Fish. aquatic Sci. 106, 110–127.
Junk, W. J., and Wantzen, K. W. (2004). “The flood pulse concept: new aspects, approaches, and applications e an update,” in Proceedings of the second international symposium on the management of large rivers for fisheries. Food and agriculture organization & mekong river commission. Editors R. L. Welcomme, and T. Petr (Bangkok: RAP Publication), 117–140.
Kerr, J. L., Baldwin, D. S., and Whitworth, K. L. (2013). Options for managing hypoxic blackwater events in river systems: a review. J. Environ. Manag. 14, 139–147. doi:10.1016/j.jenvman.2012.10.013
King, A. J., Ward, K. A., O’Connor, P., Green, D., Tonkin, Z., and Mahoney, J. (2010). Adaptive management of an environmental watering event to enhance native fish spawning and recruitment. Freshw. Biol. 55, 17–31. doi:10.1111/j.1365-2427.2009.02178.x
Kingsford, R. T., and Auld, K. M. (2005). Waterbird breeding and environmental flow management in the Macquarie Marshes, arid Australia. River Res. Appl. 21, 187–200. doi:10.1002/rra.840
Kobayashi, T., Ryder, D. S., Gordon, G., Shannon, I., Ingleton, T., Carpenter, M., et al. (2009). Short-term response of nutrients, carbon and planktonic microbial communities to floodplain wetland inundation. Aquat. Ecol. 43, 843–858. doi:10.1007/s10452-008-9219-2
Konrad, C. P., Olden, J. D., Lytle, D. A., Melis, T. S., Schmidt, J. C., Bray, E. N., et al. (2011). Large-scale flow experiments for managing river systems. BioScience 61 (12), 948–959. doi:10.1525/bio.2011.61.12.5
Lind, P. R., Robson, B. J., and Mitchell, B. D. (2007). Multiple lines of evidence for the beneficial effects of environmental flows in two lowland rivers in Victoria, Australia. River Res. Appl. 23, 933–946. doi:10.1002/rra.1016
Liu, X. (2017). Applying a transdisciplinary approach to improve the understanding of current and future states of inland ephemeral wetlands: an Australian case study. PhD thesis. Albury, Australia: Charles Sturt University.
Liu, X., Watts, R. J., Howitt, J. A., and McCasker, N. (2019). Carbon and nutrient release from experimental inundation of agricultural and forested floodplain soil and vegetation: influence of floodplain land use on the development of hypoxic blackwater during floods. Mar. Freshw. Res. 71, 213–228. doi:10.1071/MF18452
Messager, M. L., Lehner, B., Cockburn, C., Lamouroux, N., Pella, H., Snelder, T., et al. (2021). Global prevalence of non-perennial rivers and streams. Nature 594, 391–397. doi:10.1038/s41586-021-03565-5
Mitchell, A., and Baldwin, D. S. (1998). Effects of desiccation/oxidation on the potential for bacterially mediated P release from sediments. Limnol. Oceanogr., 481–487. doi:10.4319/lo.1998.43.3.0481
Murray-Darling Basin Authority (2012). Assessment of environmental water requirements for the proposed basin plan: Edward-Wakool River System. Canberra: Murray-Darling Basin Authority.
Murray-Darling Basin Authority (2018). River flows and connectivity: 2017 basin plan evaluation. Canberra: Murray-Darling Basin Authority.
Nilsson, C., and Berggren, K. (2000). Alterations of riparian ecosystems caused by river regulation dam operations have caused global-scale ecological changes in riparian ecosystems. How to protect river environments and human needs of rivers remains one of the most important questions of our time. Bioscience 50, 783–792. doi:10.1641/0006-3568(2000)050[0783:AORECB]2.0.CO;2
Nilsson, C., Reidy, C. A., Dynesius, M., and Revenga, C. (2005). Fragmentation and flow regulation of the world’s large river systems. Science 308, 405–408. doi:10.1126/science.11078
Opperman, J. J., Kendy, E., and Barrios, E. (2019). Securing environmental flows through system reoperation and management: lessons from case studies of implementation. Front. Environ. Sci. 7, 104. doi:10.3389/fenvs.2019.00104
Palmer, M. A., Reidy Liermann, C. A., Nilsson, C., Flörke, M., Alcamo, J., Lake, P. S., et al. (2008). Climate change and the world's river basins: anticipating management options. Front. Ecol. Environ. 6, 81–89. doi:10.1890/060148
Pasco, T. E., Kaller, M. D., Harlan, R., Kelso, W. E., Rutherford, D. A., and Roberts, S. (2016). Predicting floodplain hypoxia in the Atchafalaya River, Louisiana, USA, a large, regulated southern floodplain river system. River Res. Appl. 32, 845–855. doi:10.1002/rra.2903
Pekel, J. F., Cottam, A., Gorelick, N., and Belward, A. S. (2016). High-resolution mapping of global surface water and its long-term changes. Nature 540, 418–422. doi:10.1038/nature20584
Qiu, S., and McComb, A. J. (1994). Effects of oxygen concentration on phosphorus release from reflooded air-dried wetland sediments. Mar. Freshw. Res. 45 (7), 1319–1328. doi:10.1071/MF9941319
Rayner, T. S., Jenkins, K. M., and Kingsford, R. T. (2009). Small environmental flows, drought and the role of refugia for freshwater fish in Macquarie Marshes, arid Australia. Ecohydrology 2, 440–453. doi:10.1002/eco.73
Robertson, A. I., Bunn, S. E., Boon, P. I., and Walker, K. F. (1999). Sources, sinks and transformations of organic carbon in Australian floodplain rivers. Mar. Freshw. Res. 50, 813–829. doi:10.1071/MF99112
Sabo, M. J., Bryan, C. F., Kelso, W. E., and Rutherford, D. A. (1999). Hydrology and aquatic habitat characteristics of a riverine swamp: I. Influence of flow on water temperature and chemistry. Regul. Rivers Res. Manag. 15, 505–523.
Shafroth, P. B., Wilcox, A. C., Lytle, D. A., Hickey, J. T., Andersen, D. C., Beauchamp, V. B., et al. (2010). Ecosystem effects of environmental flows: modelling and experimental floods in a dryland river. Freshw. Biol. 55, 68–85. doi:10.1111/j.1365-2427.2009.02271.x
State of New South Wales and Department of Planning, Industry and Environment (2020). Murray–lower darling long term water plan Part B: murray–lower darling planning units. https://www.environment.nsw.gov.au/-/media/OEH/Corporate-Site/Documents/Water/Water-for-the-environment/long-term-water-plans/murray-lower-darling-long-term-water-plan-part-b-planning-units-200081.pdf.
Tuteja, N. K. (2008). Koondrook-Perricoota Forest flood enhancement project: hydraulic modelling. https://www.environment.nsw.gov.au/-/media/OEH/Corporate-Site/Documents/Land-and-soil/koondrook-perricoota-forest-flood-enhancement-project-080561.pdf.
Twinch, A. J. (1987). Phosphate exchange characteristics of wet and dried sediment samples from a hypertrophic reservoir: implications for the measurements of sediment phosphorus status. Water Res. 21 (10), 1225–1230. doi:10.1016/0043-1354(87)90174-6
Vorste, R. V., Sarremejane, R., and Datry, T. (2020). “Intermittent Rivers and Ephemeral Streams: a unique biome with important contributions to biodiversity and ecosystem services,” in Encyclopedia of the world's biomes. Editors s), M. I. Goldstein, and D. A. DellaSala (Amsterdam, Netherlands: Elsevier).
Ward, J. V., and Stanford, J. A. (1995). Ecological connectivity in alluvial river ecosystems and its disruption by flow regulation. River Res. Appl. 11 (1), 105–119. doi:10.1002/rrr.3450110109
Watts, R. J., Bond, N. R., Grace, M. R., Healy, S., Howitt, J. A., Liu, X., et al. (2019). Commonwealth environmental water Office long term intervention monitoring project: Edward/Kolety-Wakool River System selected area summary report, 2018-19. https://www.dcceew.gov.au/sites/default/files/documents/edward-wakool-ltim-summary-report-2018-19.pdf.
Watts, R. J., Bond, N. R., Healy, S., Liu, X., McCasker, N. G., Siebers, A., et al. (2020). Commonwealth environmental water Office monitoring, evaluation and research project: Edward/Kolety-Wakool River System selected area technical report, 2019-20. https://www.dcceew.gov.au/sites/default/files/documents/cewo-mer-edward-kolety-wakool-technical-report-2019-20.pdf.
Watts, R. J., Kopf, R. K., McCasker, N., et al. (2018). Adaptive management of environmental flows: using irrigation infrastructure to deliver environmental benefits during a large hypoxic blackwater event in the southern Murray–Darling Basin, Australia. Environ. Manag. 61, 469–480. doi:10.1007/s00267-017-0941-1
Watts, R. J., McCasker, N., Howitt, J. A., Thiem, J., Grace, M., Kopf, R. K., et al. (2017a). Commonwealth environmental water Office long term intervention monitoring project: Edward-Wakool River System selected area evaluation report, 2016-17. https://www.dcceew.gov.au/water/cewo/publications/edward-wakool-ltim-annual-report-2016-17.
Watts, R. J., Ryder, D. S., Allan, C., and Commens, S. (2010). Using river-scale experiments to inform variable flow releases from large dams: a case study of emergent adaptive management. Mar. Freshw. Res. 61, 786–797. doi:10.1071/MF09190
Watts, R. J., Wolfenden, B., Howitt, J. A., Jenkins, K., McCasker, N., and Blakey, R. (2017b). Contribution of koondrook-perricoota floodplain runoff to the productivity of the Wakool River. Report prepared for Forestry corporation of New South Wales. https://researchoutput.csu.edu.au/ws/portalfiles/portal/22010199/21480640_published_report.pdf.
Whitworth, K. L., Baldwin, D. S., and Kerr, J. L. (2014). The effect of temperature on leaching and subsequent decomposition of dissolved carbon from inundated floodplain litter: implications for the generation of hypoxic blackwater in lowland floodplain rivers. Chem. Ecol., 1–10. doi:10.1080/02757540.2014.885019
Williams, D. D. (1996). Environmental constraints in temporary fresh waters and their consequences for the insect fauna. J. North Am. Benthol. Soc., 634–650. doi:10.2307/1467813
Zanor, G. A., Piovano, E. L., Ariztegui, D., and Vallet-Coulomb, C. (2012). A modern subtropical playa complex: salina de Ambargasta, central Argentina. J. S. Am. Earth Sci. 35 (0), 10–26. doi:10.1016/j.jsames.2011.10.007
Zollitsch, B., and Christie, J. (2014). Report on state definitions, jurisdiction and mitigation requirements in state programs for ephemeral, intermittent and perennial streams in the United States. https://www.nawm.org/stream_mitigation/streams_in_the_us.pdf.
Keywords: environmental flows, intermittent and ephemeral rivers, irrigation infrastructure, instream productivity, dissolved organic carbon, dissolved oxygen, refuge, Murray-Darling Basin
Citation: Liu X, Watts RJ and Dyer J (2024) An environmental flow to an ephemeral creek increases the input of carbon and nutrients to a downstream receiving river. Front. Environ. Sci. 12:1213001. doi: 10.3389/fenvs.2024.1213001
Received: 27 April 2023; Accepted: 12 January 2024;
Published: 06 February 2024.
Edited by:
Maria Ilhéu, University of Evora, PortugalReviewed by:
João Oliveira, University of Lisbon, PortugalFrancisco Godinho, Hidroerg, Portugal
Rui Manuel Vitor Cortes, University of Trás-os-Montes and Alto Douro, Portugal
Copyright © 2024 Liu, Watts and Dyer. This is an open-access article distributed under the terms of the Creative Commons Attribution License (CC BY). The use, distribution or reproduction in other forums is permitted, provided the original author(s) and the copyright owner(s) are credited and that the original publication in this journal is cited, in accordance with accepted academic practice. No use, distribution or reproduction is permitted which does not comply with these terms.
*Correspondence: Xiaoying Liu, eGlsaXVAY3N1LmVkdS5hdQ==