- Centre for Research in Development, Social and Environment (SEEDS), Faculty of Social Sciences and Humanities, Universiti Kebangsaan, Bangi, Malaysia
The approach for monitoring forest health such as canopy layer, air quality, soil texture has evolved in tandem with the advancement of new technology such as lab analysis, remote sensing etc. The application of biomonitoring techniques for example species diversity and morphological observation, on the other hand, has been positive and has made its own contribution to forest management. Many studies have been conducted in the last decade (2011–2021), which use the biomonitoring techniques in assessing the forest health status. Therefore, this study aims to systematically review the forest health biomonitoring techniques in the last decade. This study used the PRISMA guidelines as the protocol to search and analyze all the papers. This study selected 72 out of 538 papers for a thematic analysis which eventually identified four main biomonitoring techniques, namely: 1) diversity distribution, 2) morphological observations, 3) trace elements, minerals and physiological measurements, and 4) behavioral observations. The biomonitoring techniques applied to monitor forest health has evolved with numerous ways that can support existing technologies, as well as help educate people on the necessity of protecting and safeguarding the natural forest environment. This also will give more options to the authority in monitoring the forest health and not only focusing on technology.
1 Introduction
Forest health has been defined by the production of forest conditions which directly satisfy human needs and by resilience, recurrence, persistence, and biophysical processes which lead to sustainable ecological conditions (Anderegg et al., 2020). The status of forest health has been declining globally, where global forest health has dropped nearly 60% from 1960 to 2019, mostly in the tropical region, due to urbanization and expansion of agricultural area (Williams et al., 2021). This is the same reason that brings forth climate change, which also affects forest health. The decline of forest health status signifies that forest ecosystems cannot provide the services or functions they normally supply to humans (Tebbett et al., 2021). The deterioration of forest ecosystem services implies a reduction in the forest’s ability to sustain local human populations through ecosystem services such as soil stability, water control, and local economic growth, as well as to offer recreational and cultural amenity value (Delgado and Marín 2020). Hence, this will eventually affect people’s quality of life and wellbeing. According to the Millennium Economic Assessment (MEA) (2005), human livelihoods are dependent on ecological services (particularly forest ecosystems), which help to support global employment and economic activity (food and timber production, marine fisheries and aquaculture, and recreation). Biodiversity loss, ecosystem degradation, and the resulting changes in ecosystem services have resulted in a fall in human wellbeing in some groups by intensifying poverty and increasing inequalities and inequities (Tallis et al., 2018). Therefore, it is important and critical for humans to be able to monitor, measure and determine the status of forest health. On the other hand, the techniques used to monitor and measure the forest health status are also important things to be considered. The monitoring techniques used must be holistic, practical, and able to act as an early alarm of any significant changes happening to the forest health (Abas 2021).
Forests are the most common terrestrial ecosystem on Earth and are found all across the world. Forests account for 75% of the biosphere’s gross primary output and comprise 80% of the planet’s plant biomass (Duncanson et al., 2019). Net primary output in tropical forests is predicted to be 21.9 gigatonnes of biomass per year, 8.1 gigatonnes in temperate forests, and 2.6 gigatonnes in boreal forests (Thakur et al., 2019). According to Newton et al. (2020), over 2 billion people rely on forests. Forests provide people with shelter, livelihoods, water, food, and fuel security. All these activities, directly or indirectly, involve forests. Some are easy to figure out—fruit, paper, and wood from trees, and so on. Others are less obvious, such as by-products that go into everyday items like medicines, cosmetics, and detergents. Therefore, it is important to monitor the forest health status so that the forest can always provide the service it has usually given and human livelihoods and wellbeing will always be protected (Diansyah et al., 2021). Forest health is usually defined as the production of forest conditions which directly satisfy human needs and have the resilience, recurrence, persistence, and biophysical processes which lead to sustainable ecological conditions. Numerous methods and parameters for monitoring biotic and abiotic forest components have been developed for example, the use of remote sensing (Lausch et al., 2018), measuring SO2 and NO2 concentrations (Davidson et al., 2020), satellite imaging (Woodward et al., 2018), and vegetative index (Rogers 2002). Biological indicators such as insects (Allen et al., 2019), fungi (Warwell et al., 2019), and plants (Finger et al., 2021) have also been used widely to monitor and assess the health of forests.
Although there are many ways to monitor forest health, the use of biological indicators is important due to several advantages such as: 1) reflecting the overall environmental integrity comprising physical, chemical, and biological monitoring; 2) imparting an integrated and holistic measure of ecological conditions by uniting stresses over a period of time; and 3) providing a better understanding of a healthy environment for the public than other methods (Medhi et al., 2020). Despite these advantages, efforts to review biomonitoring techniques systematically and constructively are still lacking, especially regarding forest health ecosystems (Swislowski et al., 2020). Rather than inferring exposure from chemical concentrations in air, water, or soil, biomonitoring analyses personal exposure to dangerous chemicals by detecting the substances or their metabolites in human specimens such as blood or urine (Bocca et al., 2019).
Bioaccumulation, biochemical modifications, morphological and behavioural observation, population- and community-level methods, and modelling are the most prevalent biomonitoring techniques (Abas 2021). The significance of collecting, searching, and reviewing the biomonitoring techniques used to monitor forest health will help to create a better understanding and facilitate the identification of the research gaps that exist in this particular area (Muhammad et al., 2018; Zulaini et al., 2019). Therefore, this study aims to systematically review the forest health biomonitoring techniques. In order to achieve this aim, three research objectives have been outlined which are: 1) to explore the spatial and temporal patterns of the studies; 2) to analyze the contextual issues discussed in the forest health biomonitoring techniques; and 3) to discover the biomonitoring techniques used to monitor forest health in the last decade between 2011–2021.
2 Research methodology
2.1 PRISMA statement (Preferred reporting items for systematic reviews and meta-analyses)
The PRISMA statement (Moher et al., 2009) served as the primary guideline for this systematic review investigation. PRISMA is a minimal set of monitoring components based on evidence for systematic analyses and meta-analysis. The example of relevant studies that used PRISMA statement are; Abas et al. (2022) that analyzed the local wisdom of the indigenous people in nature conservation, Owen (2020) that explored the climate change adaptation globally, and Malek et al. (2021) that determined the social indicator for building a smart city. The PRISMA statement was chosen for this study because of its strict procedure emphasizing the reporting of randomized trial assessment reviews, which may also be used as a basis for publishing comprehensive reviews of other types of studies, including intervention evaluations (PRISMA 2021). PRISMA statement has been used to report the results of a systematic review evaluating the effects of an intervention, whether the review is limited to randomized controlled trials or includes other types of research.
2.2 Formulation of the research question
The study questions were developed using PICo. PICo is a key component of a question that developed to address knowledge gaps in a study. Caón and Buitrago-Gómez (2018) describe PICo as a tool that supports writers in developing appropriate research questions for review. PICo was often used in the field of health sciences and medical to develop an evidence-based clinical practice. PICo is built around three key concepts: population or problem, interest, and context. This study has incorporated three major features in the review based on these concepts: forest health monitoring (issue), forest health (interest), and biomonitoring approaches (context). Hence, this study asks the following questions: 1) What is the distribution pattern of biomonitoring techniques for forest health spatially and temporally? 2) What are the contextual issues discussed in this study? and 3) What is the theme that can be developed for the biomonitoring techniques used to assess and monitor forest health?
2.3 Systematic searching strategies
As shown in Figure 1, the systematic searching strategies included three main strategies: identification, screening, and eligibility.
2.3.1 Identification
Identification is the process of searching for the appropriate keywords depending on the research questions. Three keywords, as well as their synonyms, related phrases, and variants, were employed in this investigation. The buzzwords were <forest health> and <biomonitoring>. The keywords were produced based on Okoli’s (2015) research question, and the identification method relied on online thesauruses, keywords from previous studies, keywords recommended by Web of Science (WoS), and keywords suggested by experts. As demonstrated in Table 1, this study was able to enhance a series of keyword strings by searching for relevant publications in the WoS database and Scopus. WoS and Scopus are websites that offer subscription-based access to a variety of databases as well as detailed citation data for a wide range of academic subjects. The present researchers picked WoS and Scopus over other search engines since all journals in these two have gone through a rigorous editing procedure, assuring article quality before publication and preserving the publications’ impact factor rankings (Scopus 2021; WoS 2021). The search technique employing both databases yielded 538 articles that were relevant to the study’s research goals.
2.3.2 Screening
To eliminate duplicate records, all 538 articles were initially checked. Due to duplication, a total of 219 papers were removed from the database, leaving 319 articles. The remaining 319 papers were rescreened to confirm the review’s quality; only publications having empirical data and published in a journal were considered. Furthermore, only papers published in English were included in the review to minimize misunderstanding. This procedure eliminated 79 articles because they did not meet the inclusion requirements. The remaining 240 articles were employed in the third procedure, which was to determine eligibility.
2.3.3 Eligibility
The third procedure is eligibility, in which the authors personally monitored the retrieved articles to ensure that all of the remaining articles (after the screening process) met the requirements. This was accomplished by reading the titles and abstracts of the papers. A total of 144 articles were excluded because they focused on ecological processes, social monitoring, forest ecological studies, forest conservation outreach, social-ecological systems, forest ecosystem monitoring, ecosystem services valuation and assessment, assessment of sustainable forest management, non-forest biomonitoring, focus on review, did not use empirical data, or were published as a chapter in a book. Hence, only 96 articles were through for the next stage.
2.4 Quality appraisal
The remaining articles were provided to two specialists for quality assessment in order to ensure the quality of the material. These two specialists are academics who have undertaken several studies on forest health. According to Petticrew and Roberts (2006), the remaining papers were divided into three quality categories: good, moderate, and low. Only papers classified as high and moderate were examined. The specialists concentrated on the methodology of the articles in order to calculate the quality rating. Both experts had to concur that the paper’s quality had to be at least moderate in order for it to be included in the research. Before deciding whether or not to include or exclude articles from the review, they discussed any differences. Following this process, 50 articles were rated as high, 22 as moderate, and 24 as low. As a result, only 72 articles were eligible for review (refer to Annex 1).
2.5 Data abstraction and analysis
Thematic analysis was utilized in this study to create themes and sub-themes. According to Braun and Clarke (2006), thematic analysis is used to find the themes and sub-themes that exist within the abstracted data by noticing patterns and motifs, grouping, counting, and highlighting parallels and correlations. Any abstracted data that was comparable or related was grouped together. Following a thorough analysis, four topics emerged in this study: 1) diversity distribution, 2) morphological observation, 3) trace elements, minerals, and physiological measurements, and 4) behavioral observations.
3 Results
3.1 Spatial and temporal distribution of selected articles
A total of 72 articles were analyzed through a detailed screening procedure. Based on Figure 2A, the distribution of studies on the forest health biomonitoring techniques in the last decade (2011–2021) has been fluctuated but slowly increasing temporally. Most papers were published in 2016 and 2018 with nine papers respectively. Based on Figure 2B, all of the 72 papers were distributed among 29 countries, of which the United States of America (United States) had the most with 23 papers, followed by Brazil, China and Spain each with four papers. Figure 2C shows the distribution of the selected papers based on their continent of origin. The most papers come from North America with 26 papers and followed by Europe with 20 papers.
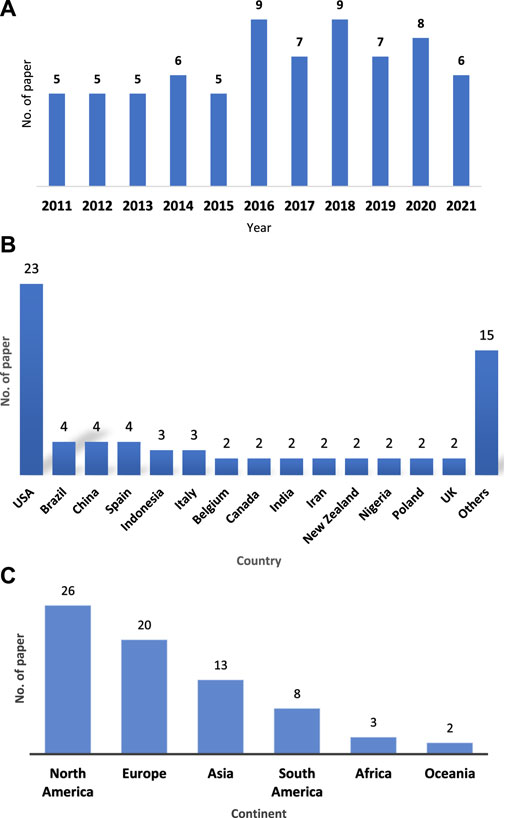
FIGURE 2. (A) Temporal distribution of the selected papers. (B) Country distribution of the selected papers. (C) Continent distribution of the selected articles.
3.2 The forest health biomonitoring technique—Contextual issues
This study discovered four main contextual issues which were: 1) method used from the selected articles, 2) type of forest, 3) sub-type of forest, and 4) type of biological indicators. Based on Figure 3A, most of the selected articles used experimental design-type methods (67%) to study the biomonitoring techniques used for forest health assessment. In terms of type of forest, based on Figure 3B, 74% of the selected articles were studies on temperate forests, 18% on tropical forests. For the sub-type of forest (Figure 3C), most of the selected articles (23%) focused on coniferous forests, followed by mixed forests with 18%, lowland evergreen rainforests and montane forests both with 11%. Based on Figure 3D, 16 out of the 72 articles used vascular plants as biological indicators to monitor forest health, followed by insects with 13 articles, mammals with nine articles.
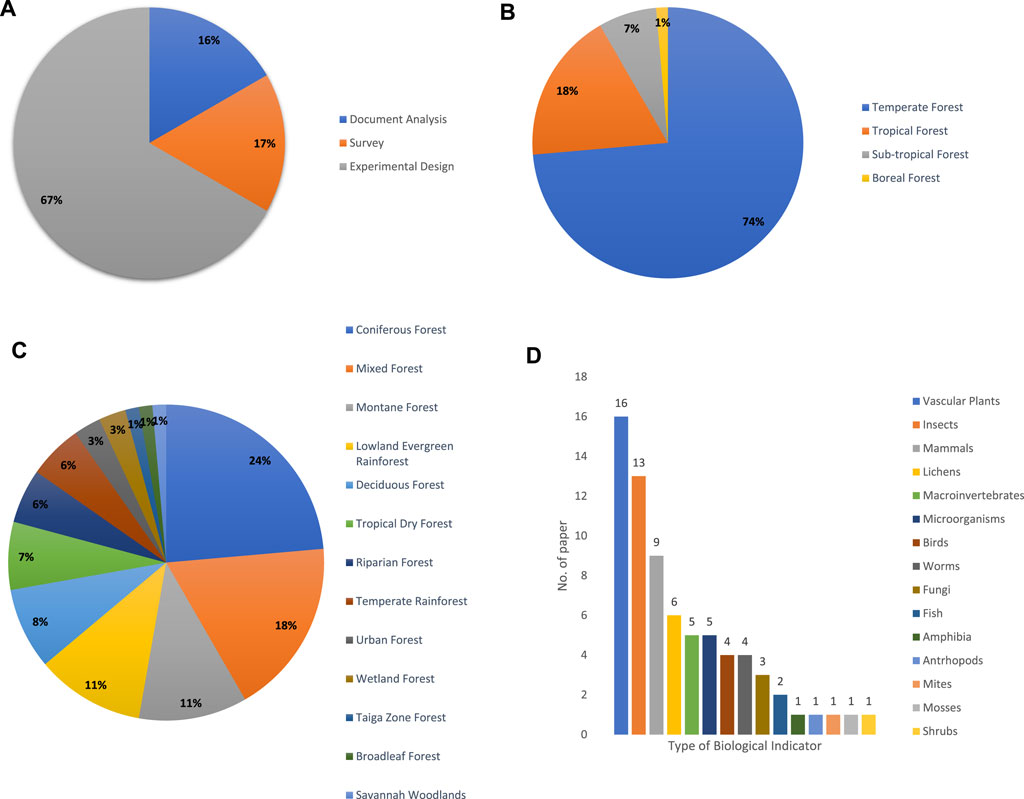
FIGURE 3. (A) Methods used from the selected articles. (B) Type of forest. (C) Sub-type of forest. (D) Type of biological indicator.
3.3 The forest health biomonitoring technique—Thematic analysis
This study discovered four main themes for the biomonitoring techniques used to assess forest health which were: 1) diversity distribution, 2) morphological observation, 3) trace elements and physiological measurement and 4) behavioral observation. Based on Figure 4, 56.94% of the selected articles studied the diversity distribution techniques, while 19.44% focused on morphological observation.
4 Discussions
4.1 Biomonitoring technique of the forest health—Substantive issue
4.1.1 Diversity distribution
The diversity distribution monitoring techniques is used to assess and measure forest health by calculating the frequency of individuals of certain species, identifying species diversity, analyzing by using the existing indices, etc. Vascular plants have been used in several countries, such as Indonesia (Wulffraat and Morrison 2013; Fujiki et al., 2016), the United States (Shearman et al., 2015; Guyon and Battaglia 2018) and Brazil (Poorter et al., 2015) to monitor forest health through the forest communities stand index, canopy cover analysis through satellite imagery and forest inventory and analysis (FIA). Besides that, the diversity of insects has also been used to monitor forest health. For example, the diversity of dung beetles (Sullivan et al., 2018; Somay et al., 2021) and bark beetles (Rassati et al., 2016; Morris et al., 2018) have been analyzed and monitored to determine the soil moisture in the forest. Other than that, orchid bees (Suni, 2017; Allen et al., 2019), moths (Highland et al., 2013) and dragonflies (Dolný et al., 2013) were used to observe the forest fertility and humidity. The individual frequency of small mammal species such as rodents (Ahumada et al., 2011) and squirrels (De La Sancha 2014) have been used to monitor the forest humidity and temperature. A specific index (the Living Planet Index) using the diversity of mammals such as deer (Fisichelli and Miller, 2018) and forest specialists (Green et al., 2020) has been developed to monitor forest health. On the other hand, a study by López-Baucells et al. (2017) used fruit bats as an indicator of good forest condition due to their need for fruit to survive. The diversity distribution of lichens and fungi were also used to monitor the forest health status. A study by Coyle and Hurlbert (2016) used macrolichen richness to measure the environmental heterogeneity and a study by Song et al. (2012) used the epiphytic lichen species distribution to determine the impact of climate change on forest health. In terms of the use of fungi, the existence of fungi usually brings negative signals to the forest health status. Numerous studies, such as Xu et al. (2012), Warwell et al. (2019) and Zgrablić et al. (2016), have shown that the existence of fungi such as Armillaria spp., Sphaeropsis sapinea, etc. indicate that the forest health status is in a very poor condition. Macroinvertebrates are one of the most notable biological indicators for freshwater quality, especially for river ecosystems. However, there are a few studies such as Deborde et al. (2016), Kim et al. (2016), Brogna et al. (2018), Foomani et al. (2020), and Edegbene et al. (2021) which have shown a significant relationship between forest health and river water quality. There are also studies that use other biological indicator distributions such as birds (Laiolo et al., 2011; Wade et al., 2013; Gnass Giese et al., 2015), nematodes (D’Errico et al., 2014; Nisa et al., 2021), amphibians (Jongsma et al., 2014), microorganisms (Hermans et al., 2020), fish (Gottesman et al., 2020) and mites (Meehan et al., 2019).
Diversity distribution has been the most notable method used to monitor forest health through biological indicators because of the dynamics of living things that always strive in order to survive. Living things always seek to evolve and adapt to their surroundings which will lead to variations of species. This process has been happening since the beginning of living things on Earth. Vascular plants such as gymnosperms, angiosperms, ferns, etc. have the highest numbers in forests, which means vascular plants are the most efficient and practical biological indicator to use for diversity distribution (Moradi et al., 2019). Other than vascular plants, fungi and lichens are also favorites to be used as biological indicators to monitor forest health. Lichens are very dynamic in terms of species diversity distribution; they have a vast range in terms of sensitive species and tolerant species. Based on which species dominate the forest, indications of forest health can be determined (Abas et al., 2019). Forests are also known as the home of megafauna such as mammals, insects, birds, amphibians, etc. The range of diversity of animals in the forest is often used to determine its condition. Mammals, insects, birds, and amphibians always respond to the forest conditions such as forest humidity, soil moisture, and light intensity (Mohd-Taib et al., 2020).
However, organisms such as non-vascular plants, fish, and amphibians are still lacking in terms of studies for biomonitoring of forest health. According to Norhazrina et al. (2016), non-vascular plant diversity distribution, such as mosses, liverworts, and hornworts, have a significant relationship with the forest humidity and the light intensity under the forest canopy. Therefore, they can act as good biological indicators for forest health. Other than that, fish and amphibian diversity distribution are highly dependent on the river water quality, which significantly correlates with the soil fertility of the forest (Faruk et al., 2013). Fish and amphibians usually respond to the dissolved oxygen (DO) and pH values of the river water, where certain species such as toads and salmon can only be found in the upstream river due to its richness in terms of DO and neutral pH value (Faudzi et al., 2021).
4.1.2 Morphological observation
The monitoring techniques using morphological observation of the biological component in the forest ecosystem includes observing physical changes such as color, size, and texture. Numerous studies have observed physical changes in vascular plants, such as the decolorization of leaves due to the lack of water and low humidity (Kleinman et al., 2012; Kamoske et al., 2019; Finger et al., 2021) or disease and infection (Smith 2012; Feldpausch et al., 2016; Donald et al., 2020). Besides that, a study by Sánchez-Salguero et al. (2017) found a significant correlation between the radial growth of trees and the soil moisture in the forest ecosystem. Mulvey and Bisbing (2016) also found a significant relationship between leaf size and CO2 concentration in the forest, where excessive CO2 would increase the size of tree leaves. On the other hand, there are several studies that used the physical changes in insects such as ants (Krapfl et al., 2011; Skaldina and Sorvari 2017), elongate hemlock scale (Gómez et al., 2015), and termites (Kashian et al., 2018) to determine the soil moisture, soil pH and tree health. A study by Bal et al. (2018) observed nematode body moisture to determine the soil fertility in forests. Peterson et al. (2014) observed oomycete body color to assess the water quality of the river in the forest ecosystem.
The change of morphology of organisms such as color, texture, or size of a certain structure usually happens when there are sudden changes in the surroundings. This is similar to when the human skin turns red after sunbathing. According to Osman et al. (2021), due to excessive CO2, vascular plants such as angiosperms usually adapt their structure by increasing the leaf size and wingless seeds. Besides that, due to decreasing forest humidity, some vascular plants will change the color of their leaves or bark as a sign of lack of water (Nizam et al., 2009). Animals also have the ability to change morphological characteristics such as their skin color, body parts size, etc. However, according to Li et al. (2021), changes in morphological characteristics of animals take a long time to happen and, in terms of monitoring forest health, this is only practical for long term monitoring.
4.1.3 Trace elements, minerals, and physiological measurement
Biological components can be monitored by analyzing trace elements, minerals, heavy metals, PAH, etc., and also by assessing physiological changes such as the integrity of the cell membrane, the efficiency of chlorophyll, etc. Through these techniques, lichens have been widely used as the biological indicator to determine forest health. Lichens from forests were collected and analyzed to measure the concentrations of heavy metals such as Cr, Ni, Cu, Zn, Cd, and Pb (Kłos et al., 2011), concentrations of S and N (Cleavitt et al., 2015), the efficiency of the chlorophyll (Loppi and Baragatti 2011) and the integrity of the cell membrane in the lichen (Will-Wolf et al., 2018). Also, monitoring has been carried out on heavy metals such as K, Fe, Mg, Ca, Zn, Pb, Cd, and Cu from the internal organs of mammals, such as the liver of wild boar (Kasprzyk et al., 2020), the colon of rats (Naderi et al., 2017) and the blood of livestock (Amadi et al., 2020). Physiological changes of microorganisms have been observed to understand and assess forest health using the integrity of their cell membrane (Dhyani et al., 2019) and their genetic sequences (Shi et al., 2019). Besides that, other studies analyzed the content of heavy metals in mosses (Berisha et al., 2017), used fish blood to evaluate the genotoxicity (Bühler et al., 2014), and vascular plants to determine the iodine concentration (Roulier et al., 2018).
According to Abas et al. (2020), trace elements can be found in some biological indicators, such as lichens. Lichens have unique features, such as the absence of a waxy cuticle on the thallus, allowing elements to enter the thallus. This makes lichens very good biological indicators that can accumulate air borne particles from their surroundings. Trace elements can also be found in animal’s internal organs such as the liver, brain, lungs, intestines, etc. (Rahman et al., 2020). Besides that, according to Becklin et al. (2016), a plant’s physiological features such as chlorophyll and cell membrane usually have reduced capacity under the stress of pollutants which can be one way to assess forest health.
4.1.4 Behavioral observation
The change in the behavior of certain organisms or biological components, such as reproductive rate, mortality rate, fatality rate, etc., can determine the current status of forest health. The fecundity rate of vascular plants has been observed to aid understanding of how forest health status has changed (de la Cruz et al., 2014; McMahon et al., 2019). On the other hand, the fatality rate of birds has been monitored to assess the temperature and humidity of a forest (Bianchi et al., 2016). The growth pattern of shrubs has been mapped and analyzed to determine the soil fertility in forests, which also correlates with forest health (Myron et al., 2021). Besides that, the zigzag sawfly eating pattern has been studied to analyze forest fertility and health (Zúbrik et al., 2017).
The relationship between a change in behavior of an organism and the status of forest health has been found to be significant. According to Xu et al. (2021), living things such as large mammals, reptiles and birds usually react and respond to sudden changes in their surroundings. A rise in temperature in an area, especially a forest, typically will significantly change the mortality and fatality rate of the vascular plants due to their dependency on soil moisture and air humidity to grow and survive (Osman et al., 2021). Other than that, animals such as insects are usually less active in reproduction in rain and wet seasons but become more active when it is bright and in the warm season (Alston et al., 2020). However, when it comes to large animals, it is quite difficult to monitor their behavior because there are no significant changes over short periods, and longer periods of monitoring are needed to observe any significant changes in their behavior (Rahman et al., 2020).
4.2 Biomonitoring technique of the forest health—Current progress and practices
The present trend in biomonitoring technology has demonstrated a major influence as an alternate tool for measuring forest health status. The species richness of flora and wildlife in the forest itself is the most commonly used biomonitoring approach. According to Nisa et al. (2021), the composition of the forest’s flora and wildlife may simply monitor its health state. For example, a forest with a large diversity of plants would have more fertile soil and will attract a greater range of animals (Naderi et al., 2017). Aside from that, a large diversity of species in a forest indicates that the ecosystem service and function are optimum. According to Abas (2021), biodiversity is commonly acknowledged as the primary driving factor in ecosystem function. Numerous of studies have been conducted to investigate the impact of tree species variety on several forest ecosystem services, including primary production (Moradi et al., 2019). Furthermore, according to Finger et al. (2021), a large diversity of fungi and lichens in the forest indicates that the forest is in a very active and healthy state. According to Hermans et al. (2020), animal and fungal diversity play an important role in forest health, where the diversity of animal and fungal species affects many important processes, such as the availability of nutrients for tree growth, promoting timber growth, preventing soil erosion, recycling nutrients, and controlling pest populations.
4.3 The challenge for the biomonitoring technique of the forest health
The biomonitoring technology is still relatively new in comparison to other existing monitoring techniques for detecting forest health (Faruk et al., 2013). The biomonitoring approach has a lot of promise, and it is not just limited to the utilisation of species diversity in the forest ecosystem. Several studies use changes in behaviour and appearance of organisms to measure forest health status (Shearman et al., 2015). In addition, the trace element concentration inside the organism and its physiological changes may be tracked to evaluate how the forest health has altered (Berisha et al., 2017). However, these techniques are currently insufficient for use in biomonitoring research, with the majority of studies focusing on species variety in the forest to establish the health status (Kashian et al., 2018). This might be due to a shortage of botanists or zoologists who utilise the research topic to establish the forest health condition, as well as the observation process taking a very long time to conduct and finish, so the study will exhaust their money budget, time, and people resources (Brogna et al., 2018).
4.4 Strength of the studied articles
This study has found three main strengths from the studied articles which firstly, the studied articles were coming from all over the continents. This show that the results that this study has shown are inclusive and cover all type of ecosystem around the globe. Secondly, the studied articles have covered and explored every type of forest that exist on the Earth biosphere. This means that every possible type of forest ecosystem has been represented in this study. This is very important to ensure that the themes that have been developed can be applied onto every type of forest that exist on Earth. Lastly, the type biological indicator that has been used to monitor the forest health is well-diverse and has explored every possible biological indicator that can used to determine the environmental quality. This means that the themes that have been developed are relevant, precise and inclusive. Determining the type of biological indicator that used to monitor the forest health is the main aim of this study, therefore it is important for it to be inclusive and well-represented.
4.5 Limitation of the studied articles
The study on the biomonitoring technique for forest health has shown several strengths and advantages. However, it also has several limitations where it can be improved in the future study. Firstly, the studied articles were mostly coming from the United States. The domination of article from United States proved that the study from other countries especially countries from the Asia region, South America and Oceania are still lacking and scientist from those countries are encouraged to explore the biomonitoring technique for the forest health in their particular locality. Secondly, all of the studied articles were written and published in English language and also been searched and screened from the Scopus and Web of Science database. Some of the articles that study on the biomonitoring technique of their local forest health may written in their native language and published on the local publisher database only. This is due to the language boundary and also the requirement from PRISMA statement which the article that selected for the study must published in the open access database with rigorous reviewing process.
4.6 Future research direction in forest health biomonitoring technique
As a result, for the limitation of the studied articles, also acknowledging the strength of it, this study found several future research directions in forest health biomonitoring technique that can explored by scientist and researcher in this field. Firstly, researcher and scientist from Asia, North America and Oceania should explore and do more study on this particular field where there are still lacking in term of number of the study. Especially for the country that have the tropical rainforest where the biological component is provenly much diverse and rich. Secondly, the method uses in assessing forest health through biomonitoring technique can be diversified where research method such as observation can be used also though it will consume much more energy and time. Lastly, the effort on translating the biomonitoring technique into the standard forest health monitoring guidelines should be taken seriously by the authorities and researcher.
5 Conclusion
This study confirms that there are several limitations and challenges to maximizing the use of biomonitoring to determine the forest health status. Firstly, information on the biomonitoring techniques used for the boreal forest type is lacking. Secondly, the biomonitoring techniques are dominated by plants, fungi, or small animals such as insects. However, studies of biomonitoring using large animals such as reptiles or mammals are still lacking due to the long period it takes to achieve significant results. Lastly, the decline of biological indicators in the forest due to anthropogenic activities and climate change has limited the choice of biological indicator for biomonitoring techniques to assess forest health status. We recommend that scientists and researchers explore boreal forest biological indicators for future studies. Plus, an established method for long term biomonitoring needs to be explored that can use large animals such as reptiles and mammals to assess forest health status. Also, there is an urgent need to conserve and preserve forest ecosystems to ensure the sustainability of biological indicators in the forest. Lastly, it is critical to have a standard parameter using biological indicators to determine the forest health quality globally. All these discoveries show that this study has succeeded in filling the gap and answering the questions raised earlier. It also opens a new dimension for research and discovery in the study of biomonitoring techniques for forest health.
Data availability statement
The original contributions presented in the study are included in the article/Supplementary Material, further inquiries can be directed to the corresponding author.
Author contributions
AA is the only author for this article and all the works in this article is done by AA. AA also approved and read the final manuscript.
Funding
This study has been supported by Universiti Kebangsaan Malaysia through research grant (SK-2021-025).
Acknowledgments
This study acknowledges the contribution by Universiti Kebangsaan Malaysia through providing online database in searching for all the articles. Also, Rose Norman for her generous assistance in conducting the proofreading for this article.
Conflict of interest
The author declares that the research was conducted in the absence of any commercial or financial relationships that could be construed as a potential conflict of interest.
Publisher’s note
All claims expressed in this article are solely those of the authors and do not necessarily represent those of their affiliated organizations, or those of the publisher, the editors and the reviewers. Any product that may be evaluated in this article, or claim that may be made by its manufacturer, is not guaranteed or endorsed by the publisher.
Supplementary material
The Supplementary Material for this article can be found online at: https://www.frontiersin.org/articles/10.3389/fenvs.2023.970730/full#supplementary-material
References
Abas, A. (2021). A systematic review on biomonitoring using lichen as the biological indicator: A decade of practices, progress and challenges. Ecol. Indic. 121, 107197. doi:10.1016/j.ecolind.2020.107197
Abas, A., Awang, A., and Aiyub, K. (2020). Analysis of heavy metal concentration using transplanted lichen Usnea misaminensis at Kota Kinabalu, Sabah (Malaysia). Appl. Ecol. Environ. Res. 18 (1), 1175–1182. doi:10.15666/aeer/1801_11751182
Abas, A., Aziz, A., and Awang, A. (2022). A systematic review on the local wisdom of indigenous people in nature conservation. Sustainability 14 (6), 3415. doi:10.3390/su14063415
Abas, A., Khalid, R. M., Rosandy, A. R., and Sulaiman, N. (2019). Lichens of pulau pangkor, perak, Malaysia. Malays. For. 82 (1), 59–66.
Ahumada, J. A., Silva, C. E. F., Gajapersad, K., Hallam, C., Hurtado, J., Martin, E., et al. (2011). Community structure and diversity of tropical forest mammals: Data from a global camera trap network. Philosophical Trans. R. Soc. B Biol. Sci. 366 (1578), 2703–2711. doi:10.1098/rstb.2011.0115
Allen, L., Reeve, R., Nousek-McGregor, A., Villacampa, J., and MacLeod, R. (2019). Are orchid bees useful indicators of the impacts of human disturbance? Ecol. Indic. 103, 745–755. doi:10.1016/j.ecolind.2019.02.046
Alston, J. M., Joyce, M. J., Merkle, J. A., and Moen, R. A. (2020). Temperature shapes movement and habitat selection by a heat-sensitive ungulate. Landsc. Ecol. 35 (9), 1961–1973. doi:10.1007/s10980-020-01072-y
Amadi, C. N., Frazzoli, C., and Orisakwe, O. E. (2020). Sentinel species for biomonitoring and biosurveillance of environmental heavy metals in Nigeria. J. Environ. Sci. Health, Part C Toxicol. Carcinog. 38 (1), 21–60. doi:10.1080/26896583.2020.1714370
Anderegg, W. R., Trugman, A. T., Badgley, G., Konings, A. G., and Shaw, J. (2020). Divergent forest sensitivity to repeated extreme droughts. Nat. Clim. Change 10 (12), 1091–1095. doi:10.1038/s41558-020-00919-1
Bal, T. L., Storer, A. J., and Jurgensen, M. F. (2018). Evidence of damage from exotic invasive earthworm activity was highly correlated to sugar maple dieback in the Upper Great Lakes region. Biol. Invasions 20 (1), 151–164. doi:10.1007/s10530-017-1523-0
Becklin, K. M., Anderson, J. T., Gerhart, L. M., Wadgymar, S. M., Wessinger, C. A., and Ward, J. K. (2016). Examining plant physiological responses to climate change through an evolutionary lens. Plant Physiol. 172 (2), 635–649. doi:10.1104/pp.16.00793
Berisha, S., Skudnik, M., Vilhar, U., Sabovljević, M., Zavadlav, S., and Jeran, Z. (2017). Trace elements and nitrogen content in naturally growing moss Hypnum cupressiforme in urban and peri-urban forests of the Municipality of Ljubljana (Slovenia). Environ. Sci. Pollut. Res. 24 (5), 4517–4527. doi:10.1007/s11356-016-8201-0
Bianchi, M. A. F., Mello, R. H., Bianchi, H., Bermond, J. L., and Ibrahim, P. A. F. (2016). Restrain of birds with bottles of polyethylene terephthalate, tested in red-browed from the Atlantic Forest. Arq. Bras. Med. Veterinaria Zootec. 68 (1), 136–140. doi:10.1590/1678-4162-8604
Bocca, B., Ruggieri, F., Pino, A., Rovira, J., Calamandrei, G., Martínez, M. Á., et al. (2019). Human biomonitoring to evaluate exposure to toxic and essential trace elements during pregnancy. Part A. concentrations in maternal blood, urine and cord blood. Environ. Res. 177, 108599. doi:10.1016/j.envres.2019.108599
Braun, V., and Clarke, V. (2006). Using thematic analysis in psychology. Qual. Res. Psychol. 3 (2), 77–101. doi:10.1191/1478088706qp063oa
Brogna, D., Dufrêne, M., Michez, A., Latli, A., Jacobs, S., Vincke, C., et al. (2018). Forest cover correlates with good biological water quality. Insights from a regional study (Wallonia, Belgium). J. Environ. Manag. 211, 9–21. doi:10.1016/j.jenvman.2018.01.017
Bühler, D., Marinowic, D. R., De Barros, M. P., and Da Silva, L. B. (2014). Genetic damage induced by water pollutants in the freshwater fish Hyphessobrycon luetkenii (Characidae) in a reservoir of the Canela National Forest, Brazil. J. Freshw. Ecol. 29 (2), 295–299. doi:10.1080/02705060.2013.879539
Cañón, M., and Buitrago-Gómez, Q. (2018). The research question in clinical practice: A guideline for its formulation. Rev. Colomb. Psiquiatr. 47 (3), 193–200. doi:10.1016/j.rcp.2016.06.004
Cleavitt, N. L., Hinds, J. W., Poirot, R. L., Geiser, L. H., Dibble, A. C., Leon, B., et al. (2015). Epiphytic macrolichen communities correspond to patterns of sulfur and nitrogen deposition in the northeastern United States. Bryologist 118 (3), 304–324. doi:10.1639/0007-2745-118.3.304
Coyle, J. R., and Hurlbert, A. H. (2016). Environmental optimality, not heterogeneity, drives regional and local species richness in lichen epiphytes. Glob. Ecol. Biogeogr. 25 (4), 406–417. doi:10.1111/geb.12420
Davidson, C. J., Foster, K. R., and Tanna, R. N. (2020). Forest health effects due to atmospheric deposition: Findings from long-term forest health monitoring in the Athabasca Oil Sands Region. Sci. Total Environ. 699, 134277. doi:10.1016/j.scitotenv.2019.134277
de la Cruz, A. C., Gil, P. M., Fernández-Cancio, Á., Minaya, M., Navarro-Cerrillo, R. M., Sánchez-Salguero, R., et al. (2014). Defoliation triggered by climate induced effects in Spanish ICP Forests monitoring plots. For. Ecol. Manag. 331, 245–255. doi:10.1016/j.foreco.2014.08.010
De La Sancha, N. U. (2014). Patterns of small mammal diversity in fragments of subtropical Interior Atlantic Forest in eastern Paraguay. Mammalia 78 (4), 1–13. doi:10.1515/mammalia-2013-0100
Deborde, D. D. D., Hernandez, M. B. M., and Magbanua, F. S. (2016). Benthic macroinvertebrate community as an indicator of stream health: The effects of land use on stream benthic macroinvertebrates. Sci. Diliman 28 (2), 5–26.
Delgado, L. E., and Marín, V. H. (2020). Ecosystem services and ecosystem degradation: Environmentalist’s expectation? Ecosyst. Serv. 45, 101177. doi:10.1016/j.ecoser.2020.101177
D’Errico, G., Carletti, B., Schroder, T., Mota, M., Vieira, P., and Roversi, P. F. (2014). An update on the occurrence of nematodes belonging to the genus Bursaphelenchus in the Mediterranean area. Forestry 88 (5), 509–520. doi:10.1093/forestry/cpv028
Dhyani, A., Jain, R., and Pandey, A. (2019). Contribution of root-associated microbial communities on soil quality of Oak and Pine forests in the Himalayan ecosystem. Trop. Ecol. 60 (2), 271–280. doi:10.1007/s42965-019-00031-2
Diansyah, W., Abas, A., and Sakawi, Z. (2021). A systematic review on community forest management in southeast Asia: Current practices and impacts on biodiversity conservation and livelihood quality of local communities. Hum. Ecol. Rev. 27 (1), 3–21. doi:10.22459/her.27.01.2021.01
Dolný, A., Harabiš, F., Bártaa, D., Lhota, S., and Drozd, P. (2013). Aquatic insects indicate terrestrial habitat degradation: Changes in taxonomical structure and functional diversity of dragonflies in tropical rainforest of East Kalimantan. Trop. Zool. 25 (3), 141–157. doi:10.1080/03946975.2012.717480
Donald, F., Green, S., Searle, K., Cunniffe, N. J., and Purse, B. V. (2020). Small scale variability in soil moisture drives infection of vulnerable juniper populations by invasive forest pathogen. For. Ecol. Manag. 473, 118324. doi:10.1016/j.foreco.2020.118324
Duncanson, L., Armston, J., Disney, M., Avitabile, V., Barbier, N., Calders, K., et al. (2019). The importance of consistent global forest aboveground biomass product validation. Surv. Geophys. 40 (4), 979–999. doi:10.1007/s10712-019-09538-8
Edegbene, A. O., Arimoro, F. O., Odume, O. N., Ogidiaka, E., and Keke, U. N. (2021). Can macroinvertebrate traits Be explored and applied in biomonitoring riverine systems draining forested catchments? Front. Water 3. doi:10.3389/frwa.2021.607556
Faruk, A., Belabut, D., Ahmad, N., Knell, R. J., and Garner, T. W. J. (2013). Effects of oil-palm plantations on diversity of tropical Anurans. Conserv. Biol. 27 (3), 615–624. doi:10.1111/cobi.12062
Faudzi, R., Abas, A., Othman, N. W., and Mazlan, S. M. (2021). Effect of water quality on the abundance of firefly populations at Cherating River, Pahang, Malaysia. Environ. Asia 14 (1), 69–79. doi:10.14456/ea.2021.8
Feldpausch, T. R., Phillips, O. L., Brienen, R. J. W., Gloor, E., Lloyd, J., Lopez-Gonzalez, G., et al. (2016). Amazon forest response to repeated droughts. Glob. Biogeochem. Cycles 30 (7), 964–982. doi:10.1002/2015GB005133
Finger, D. J. I., McPherson, M. L., Houskeeper, H. F., and Kudela, R. M. (2021). Mapping bull kelp canopy in northern California using Landsat to enable long-term monitoring. Remote Sens. Environ. 254, 112243. doi:10.1016/j.rse.2020.112243
Fisichelli, N. A., and Miller, K. M. (2018). Weeds, worms, and deer: Positive relationships among common forest understory stressors. Biol. Invasions 20 (5), 1337–1348. doi:10.1007/s10530-017-1630-y
Foomani, A., Gholizadeh, M., Harsij, M., and Salavatian, S. M. (2020). River health assessment using macroinvertebrates and water quality parameters: A case of the shanbeh-bazar river, anzali wetland, Iran. Iran. J. Fish. Sci. 19 (5), 2274–2292. doi:10.22092/ijfs.2020.122380
Fujiki, S., Aoyagi, R., Tanaka, A., Imai, N., Kusma, A. D., Kurniawan, Y., et al. (2016). Large-scale mapping of tree-community composition as a surrogate of forest degradation in Bornean tropical rain forests. Land 5 (4), 45. doi:10.3390/land5040045
Gnass Giese, E. E., Howe, R. W., Wolf, A. T., Miller, N. A., and Walton, N. G. (2015). Sensitivity of breeding birds to the “human footprint” in weWesternreat Lakes forest landscapes. Ecosphere 6 (6), art90. doi:10.1890/ES14-00414.1
Gómez, S., Gonda-King, L., Orians, C. M., Orwig, D. A., Panko, R., Radville, L., et al. (2015). Interactions between invasive herbivores and their long-term impact on New England hemlock forests. Biol. Invasions 17 (2), 661–673. doi:10.1007/s10530-014-0757-3
Gottesman, B. L., Sprague, J., Kushner, D. J., Bellisario, K., Savage, D., McKenna, M. F., et al. (2020). Soundscapes indicate kelp forest condition. Mar. Ecol. Prog. Ser. 654, 35–52. doi:10.3354/meps13512
Green, E. J., McRae, L., Freeman, R., Harfoot, M. B. J., Hill, S. L. L., Baldwin-Cantello, W., et al. (2020). Below the canopy: Global trends in forest vertebrate populations and their drivers. Proc. R. Soc. B Biol. Sci. 287, 20200533. doi:10.1098/rspb.2020.0533
Guyon, L. J., and Battaglia, L. L. (2018). Ecological characteristics of floodplain forest reference sites in the Upper Mississippi River System. For. Ecol. Manag. 427, 208–216. doi:10.1016/j.foreco.2018.06.007
Hermans, S. M., Buckley, H. L., Case, B. S., Curran-Cournane, F., Taylor, M., and Lear, G. (2020). Using soil bacterial communities to predict physico-chemical variables and soil quality. Microbiome 8 (1), 79. doi:10.1186/s40168-020-00858-1
Highland, S. A., Miller, J. C., and Jones, J. A. (2013). Determinants of moth diversity and community in a temperate mountain landscape: Vegetation, topography, and seasonality. Ecosphere 4 (10), art129. doi:10.1890/ES12-00384.1
Jongsma, G. F. M., Hedley, R. W., Durães, R., and Karubian, J. (2014). Amphibian diversity and species composition in relation to habitat type and alteration in the mache-chindul reserve, northwest Ecuador. Herpetologica 70 (1), 34–46. doi:10.1655/HERPETOLOGICA-D-12-00068
Kamoske, A. G., Dahlin, K. M., Stark, S. C., and Serbin, S. P. (2019). Leaf area density from airborne LiDAR: Comparing sensors and resolutions in a temperate broadleaf forest ecosystem. For. Ecol. Manag. 433, 364–375. doi:10.1016/j.foreco.2018.11.017
Kashian, D. M., Bauer, L. S., Spei, B. A., Duan, J. J., and Gould, J. R. (2018). Potential impacts of emerald ash borer biocontrol on ash health and recovery in southern Michigan. Forests 9 (6), 296. doi:10.3390/f9060296
Kasprzyk, A., Kilar, J., Chwil, S., and Rudaś, M. (2020). Content of selected macro-and microelements in the liver of free-living wild boars (Sus scrofa l.) from agricultural areas and health risks associated with consumption of liver. Animals 10 (9), 1–15. doi:10.3390/ani10091519
Kim, D. H., Chon, T. S., Kwak, G. S., Lee, S. B., and Park, Y. S. (2016). Effects of land use types on community structure patterns of benthic macroinvertebrates in streams of urban areas in the South of the Korea Peninsula. WaterSwitzerl. 8 (5), 187. doi:10.3390/w8050187
Kleinman, S. J., De Gomez, T. E., Snider, G. B., and Williams, K. E. (2012). Large-scale pinyon ips (<I>Ips confusus</I>) outbreak in southwestern United States tied with elevation and land cover. J. For. 110 (4), 194–200. doi:10.5849/jof.11-060
Kłos, A., Rajfur, M., Šrámek, I., and Wacławek, M. (2011). Use of lichen and moss in assessment of forest contamination with heavy metals in Praded and Glacensis Euroregions (Poland and Czech Republic). Water, Air, Soil Pollut. 222 (1–4), 367–376. doi:10.1007/s11270-011-0830-9
Krapfl, K. J., Holzmueller, E. J., and Jenkins, M. A. (2011). Early impacts of hemlock woolly adelgid in Tsuga canadensis forest communities of the southern Appalachian Mountains. J. Torrey Botanical Soc. 138 (1), 93–106. doi:10.3159/10-RA-031.1
Laiolo, P., Bañuelos, M. J., Blanco-Fontao, B., García, M., and Gutiérrez, G. (2011). Mechanisms underlying the bioindicator notion: Spatial association between individual sexual performance and community diversity. PLoS ONE 6 (7), e22724. doi:10.1371/journal.pone.0022724
Lausch, A., Borg, E., Bumberger, J., Dietrich, P., Heurich, M., Huth, A., et al. (2018). Understanding forest health with remote sensing, Part III: Requirements for a scalable multi-source forest health monitoring network based on data science approaches. Remote Sens. 10, 1120. doi:10.3390/rs10071120
Li, J., Dirzo, R., Wang, Y., Zeng, D., Liu, J., Ren, P., et al. (2021). Rapid morphological change in a small mammal species after habitat fragmentation over the past half-century. Divers. Distributions 27 (12), 2615–2628. doi:10.1111/ddi.13437
López-Baucells, A., Casanova, L., Puig-Montserrat, X., Espinal, A., Páramo, F., and Flaquer, C. (2017). Evaluating the use of Myotis daubentonii as an ecological indicator in Mediterranean riparian habitats. Ecol. Indic. 74, 19–27. doi:10.1016/j.ecolind.2016.11.012
Loppi, S., and Baragatti, E. (2011). Influence of the health status of pine trees on the diversity of epiphytic lichens. Nova Hedwig. 93 (1–2), 201–210. doi:10.1127/0029-5035/2011/0093-0201
Malek, J. A., Lim, S. B., and Yigitcanlar, T. (2021). Social inclusion indicators for building citizen-centric smart cities: A systematic literature review. Sustainability 13 (1), 376. doi:10.3390/su13010376
McMahon, S. M., Arellano, G., and Davies, S. J. (2019). The importance and challenges of detecting changes in forest mortality rates. Ecosphere 10 (2). doi:10.1002/ecs2.2615
Medhi, J., Dutta, J., and Chandra Kalita, M. (2020). Biomonitoring ecosystem: Modelling relationship with arthropods. Arthropods. doi:10.5772/intechopen.94313
Meehan, M. L., Song, Z., Lumley, L. M., Cobb, T. P., and Proctor, H. (2019). Soil mites as bioindicators of disturbance in the boreal forest in northern Alberta, Canada: Testing taxonomic sufficiency at multiple taxonomic levels. Ecol. Indic. 102, 349–365. doi:10.1016/j.ecolind.2019.02.043
Millennium Ecosystem Assessment (MEA) (2005). Ecosystems and human wellbeing: Synthesis report. Washington, DC: Island Press.
Mohd-Taib, F. S., Mohd-Saleh, W., Asyikha, R., Mansor, M. S., Ahmad-Mustapha, M., Mustafa-Bakray, N. A., et al. (2020). Effects of anthropogenic disturbance on the species assemblages of birds in the back mangrove forests. Wetl. Ecol. Manag. 28 (3), 479–494. doi:10.1007/s11273-020-09726-z
Moher, D., Liberati, A., Tetzlaff, J., and Altman, D. G.The PRISMA Group (2009). Preferred reporting items for systematic reviews and meta-analyses: The PRISMA statement. PLoS Med. 6 (7), e1000097. doi:10.1371/journal.pmed1000097
Moradi, S., Sheykhi Ilanloo, S., Kafash, A., and Yousefi, M. (2019). Identifying high-priority conservation areas for avian biodiversity using species distribution modeling. Ecol. Indic. 97, 159–164. doi:10.1016/j.ecolind.2018.10.003
Morris, J. L., Cottrell, S., Fettig, C. J., DeRose, R. J., Mattor, K. M., Carter, V. A., et al. (2018). Bark beetles as agents of change in social–ecological systems. Front. Ecol. Environ. 16, S34–S43. doi:10.1002/fee.1754
Muhammad, N., Hashim, N. H., Khairuddin, N. A., Yusof, H., Jusoh, S., Abas, A., et al. (2018). Identification of most tolerant lichen species to vehicular traffic’s pollutants: A case study at batu pahat. J. Adv. Res. Fluid Mech. Therm. Sci. 42 (1), 57–64.
Mulvey, R. L., and Bisbing, S. M. (2016). Complex interactions among agents affect shore pine health in southeast Alaska. Northwest Sci. 90 (2), 176–194. doi:10.3955/046.090.0209
Myron, K. J., Clarkson, B. D., and Gemmill, C. E. C. (2021). Biological flora of New Zealand 16: Pittosporum kirkii Hook.f. ex kirk, kirk’s kōhūhū, thick-leaved kohukohu. N. Z. J. Bot. 59 (1), 112–136. doi:10.1080/0028825X.2020.1770303
Naderi, M., Farashi, A., and Markov, G. (2017). Exploring contents of lead and cadmium in tissues of fat dormouse Glis glis (Linnaeus, 1766) (Rodentia: Gliridae) for use in monitoring of environmental pollutants in the Southern Caspian Coast Forests, Iran. Acta Zool. Bulg. 69 (1), 61–64.
Newton, P., Kinzer, A. T., Miller, D. C., Oldekop, J. A., and Agrawal, A. (2020). The number and spatial distribution of forest-proximate people globally. One Earth 3 (3), 363–370. doi:10.1016/j.oneear.2020.08.016
Nisa, R. U., Tantray, A. Y., Kouser, N., Allie, K. A., Wani, S. M., Alamri, S. A., et al. (2021). Influence of ecological and edaphic factors on biodiversity of soil nematodes. Saudi J. Biol. Sci. 28 (5), 3049–3059. doi:10.1016/j.sjbs.2021.02.046
Nizam, M. S., Ismail, P., Latiff, A., Shamsudin, I., and Faridah-Hanum, I. (2009). Diversity of tree communities and its relationships with soil properties in a peat swamp forest in Pahang, Peninsular Malaysia. Ecol. Environ. Conservation 15 (2), 307–318.
Norhazrina, N., Vanderpoorten, A., Hedenäs, L., and Patiño, J. (2016). What are the evolutionary mechanisms explaining the similar species richness patterns in tropical mosses? Insights from the phylogeny of the pantropical genus pelekium. Mol. Phylogenetics Evol. 105, 139–145. doi:10.1016/j.ympev.2016.08.008
Okoli, C. (2015). A guide to conducting a standalone systematic literature review. Commun. Assoc. Inf. Syst. 37, 43. doi:10.17705/1cais.03743
Osman, M. F., Lee, S. Y., Sarbini, S. R., Faudzi, S. M. M., Khamis, S., Zainudin, B. H., et al. (2021). Metabolomics-driven discovery of an introduced species and two Malaysian Piper betle L. Variants. Plants 10 (11), 2510. doi:10.3390/plants10112510
Peterson, E., Hansen, E., and Hulbert, J. (2014). Source or sink? The role of soil and water borne inoculum in the dispersal of phytophthora ramorum in Oregon tanoak forests. For. Ecol. Manag. 322, 48–57. doi:10.1016/j.foreco.2014.02.031
Petticrew, M., and Roberts, H. (2006). Systematic reviews in the social sciences: A practical guide. Malden, MA: Blackwell Publishing Ltd.
Poorter, L., van der Sande, M. T., Thompson, J., Arets, E. J. M. M., Alarcón, A., Álvarez-Sánchez, J., et al. (2015). Diversity enhances carbon storage in tropical forests. Glob. Ecol. Biogeogr. 24 (11), 1314–1328. doi:10.1111/geb.12364
PRISMA (2021). PRISMA. [online] Prisma-statement.org. Available at: <http://www.prisma-statement.org/> (Accessed January 31, 2022).
Rahman, A., Talukdar, N. R., and Choudhury, P. (2020). Assessing some essential trace elements concentration in micro chiropteran bat (Megaderma lyra): A study in barak valley of Assam, India. Environ. Chem. Ecotoxicol. 2, 56–63. doi:10.1016/j.enceco.2020.02.002
Rassati, D., Faccoli, M., Battisti, A., and Marini, L. (2016). Habitat and climatic preferences drive invasions of non-native ambrosia beetles in deciduous temperate forests. Biol. Invasions 18 (10), 2809–2821. doi:10.1007/s10530-016-1172-8
Rogers, P. (2002). Using Forest Health Monitoring to assess aspen forest cover change in the southern Rockies ecoregion. For. Ecol. Manag. 155 (1–3), 223–236. doi:10.1016/S0378-1127(01)00560-6
Roulier, M., Bueno, M., Thiry, Y., Coppin, F., Redon, P. O., Le Hécho, I., et al. (2018). Iodine distribution and cycling in a beech (Fagus sylvatica) temperate forest. Sci. Total Environ. 645, 431–440. doi:10.1016/j.scitotenv.2018.07.039
Sánchez-Salguero, R., Camarero, J. J., Grau, J. M., de la Cruz, A. C., Gil, P. M., Minaya, M., et al. (2017). Analysing atmospheric processes and climatic drivers of tree defoliation to determine forest vulnerability to climate warming. Forests 8 (1), 13. doi:10.3390/f8010013
Shearman, T. M., Wang, G. G., and Bridges, W. C. (2015). Population dynamics of redbay (persea borbonia) after laurel wilt disease: An assessment based on forest inventory and analysis data. Biol. Invasions 17 (5), 1371–1382. doi:10.1007/s10530-014-0799-6
Shi, L., Dossa, G. G. O., Paudel, E., Zang, H., Xu, J., and Harrison, R. D. (2019). Changes in fungal communities across a forest disturbance gradient. Appl. Environ. Microbiol. 85 (12), 1–11. doi:10.1128/AEM.00080-19
Skaldina, O., and Sorvari, J. (2017). Wood ant colouration as an ecological indicator for the level of disturbance in managed coniferous forests. Ecol. Indic. 72, 444–451. doi:10.1016/j.ecolind.2016.08.039
Smith, G. (2012). Ambient ozone injury to forest plants in northeast and North central USA: 16 years of biomonitoring. Environ. Monit. Assess. 184 (7), 4049–4065. doi:10.1007/s10661-011-2243-z
Somay, L., Szigeti, V., Boros, G., Ádám, R., and Báldi, A. (2021). Wood pastures: A transitional habitat between forests and pastures for dung beetle assemblages. Forests 12 (1), 25–16. doi:10.3390/f12010025
Song, L., Liu, W. Y., and Nadkarni, N. M. (2012). Response of non-vascular epiphytes to simulated climate change in a montane moist evergreen broad-leaved forest in southwest China. Biol. Conserv. 152, 127–135. doi:10.1016/j.biocon.2012.04.002
Sullivan, C. D., Slade, E. M., Bai, M., Shi, K., and Riordan, P. (2018). Evidence of forest restoration success and the conservation value of community-owned forests in Southwest China using dung beetles as indicators. PLoS ONE 13 (11), e0204764. doi:10.1371/journal.pone.0204764
Suni, S. S. (2017). Dispersal of the orchid bee Euglossa imperialis over degraded habitat and intact forest. Conserv. Genet. 18 (3), 621–630. doi:10.1007/s10592-016-0902-x
Swislowski, P., Kríž, J., and Rajfur, M. (2020). The use of bark in biomonitoring heavy metal pollution of forest areas on the example of selected areas in Poland. Ecol. Chem. Eng. S 27, 195–210. doi:10.2478/eces-2020-0013
Tallis, H. M., Hawthorne, P. L., Polasky, S., Reid, J., Beck, M. W., Brauman, K., et al. (2018). An attainable global vision for conservation and human well-being. Front. Ecol. Environ. 16 (10), 563–570. doi:10.1002/fee.1965
Tebbett, S. B., Morais, R. A., Goatley, C. H., and Bellwood, D. R. (2021). Collapsing ecosystem functions on an inshore coral reef. J. Environ. Manag. 289, 112471. doi:10.1016/j.jenvman.2021.112471
Thakur, T. K., Swamy, S. L., Bijalwan, A., and Dobriyal, M. J. (2019). Assessment of biomass and net primary productivity of a dry tropical forest using geospatial technology. J. For. Res. 30 (1), 157–170. doi:10.1007/s11676-018-0607-8
Wade, A. S. I., Barov, B., Burfield, I. J., Gregory, R. D., Norris, K., and Butler, S. J. (2013). Quantifying the detrimental impacts of land-use and management change on European forest bird populations. PLoS ONE 8 (5), e64552. doi:10.1371/journal.pone.0064552
Warwell, M. V., McDonald, G. I., Hanna, J. W., Kim, M. S., Lalande, B. M., Stewart, J. E., et al. (2019). Armillaria altimontana Is associated with healthy Western white pine (Pinus monticola): Potential in situ biological control of the Armillaria root disease pathogen, A. solidipes. Forests 10 (4), 294. doi:10.3390/f10040294
Will-Wolf, S., Jovan, S., Nelsen, M. P., Trest, M. T., Rolih, K. M., and Reis, A. H. (2018). Lichen indices assess local climate and air quality status in the Mid-Atlantic Region, USA. Bryologist 121 (4), 461–479. doi:10.1639/0007-2745-121.4.461
Williams, D. R., Clark, M., Buchanan, G. M., Ficetola, G. F., Rondinini, C., and Tilman, D. (2021). Proactive conservation to prevent habitat losses to agricultural expansion. Nat. Sustain. 4 (4), 314–322. doi:10.1038/s41893-020-00656-5
Woodward, B. D., Evangelista, P. H., and Vorster, A. G. (2018). Mapping progression and severity of a Southern Colorado spruce beetle outbreak using calibrated image composites. Forests 9 (6), 336. doi:10.3390/f9060336
Wulffraat, S., and Morrison, J. (2013). Measuring biological indicators for status assessment of the heart of Borneo. Environ. Conserv. 40 (3), 277–286. doi:10.1017/S0376892913000064
Xu, A., Liu, C., Wan, Y., Bai, Y., and Li, Z. (2021). Monkeys fight more in polluted air. Sci. Rep. 11 (1), 654. doi:10.1038/s41598-020-80002-z
Xu, L., Ravnskov, S., Larsen, J., and Nicolaisen, M. (2012). Linking fungal communities in roots, rhizosphere, and soil to the health status of Pisum sativum. FEMS Microbiol. Ecol. 82 (3), 736–745. doi:10.1111/j.1574-6941.2012.01445.x
Zgrablić, Ž., Marjanović, H., and Diminić, D. (2016). Can we predict Sphaeropsis sapinea outbreak by monitoring fungal diversity in Austrian pine plantations? Sumar. List. 140 (1–2), 7–15. doi:10.31298/sl.140.1-2.1
Zúbrik, M., Galko, J., Gubka, A., Rell, S., Kunca, A., Nikolov, C., et al. (2017). Dispersal and larval hosts of the zigzag sawfly Aproceros leucopoda (Hymenoptera) in Slovakia, central Europe. Period. Biol. 119 (1), 55–62. doi:10.18054/pb.v119i1.4204
Keywords: forest ecosystem, ecological indicator, environmental monitoring, forest health, biomonitoring
Citation: Abas A (2023) A systematic literature review on the forest health biomonitoring technique: A decade of practice, progress, and challenge. Front. Environ. Sci. 11:970730. doi: 10.3389/fenvs.2023.970730
Received: 16 June 2022; Accepted: 12 January 2023;
Published: 20 January 2023.
Edited by:
Sharif A. Mukul, University of the Sunshine Coast, AustraliaReviewed by:
Md. Habibur Rahman, Kyoto University, JapanMarina Frontasyeva, Joint Institute for Nuclear Research (JINR), Russia
Copyright © 2023 Abas. This is an open-access article distributed under the terms of the Creative Commons Attribution License (CC BY). The use, distribution or reproduction in other forums is permitted, provided the original author(s) and the copyright owner(s) are credited and that the original publication in this journal is cited, in accordance with accepted academic practice. No use, distribution or reproduction is permitted which does not comply with these terms.
*Correspondence: Azlan Abas, YXpsYW5hYmFzQHVrbS5lZHUubXk=