- 1Centre of Marine Sciences (CCMAR), University of Algarve, Faro, Portugal
- 2GenoGla Diagnostics, Centre of Marine Sciences (CCMAR), University of Algarve, Faro, Portugal
- 3Centre for Marine and Environmental Research (CIMA), University of Algarve, Faro, Portugal
- 4Research Centre for Tourism, Sustainability and Wellbeing, CinTurs, University of Algarve, Faro, Portugal
Under certain environmental and oceanographic conditions, macroalgae can overgrow and accumulate in massive quantities on beaches, causing serious ecological and economic impacts. To address this problem, a citizen science monitoring platform was created to determine the spatial and temporal distribution of macroalgae accumulations along the beaches of Algarve in southern Portugal, with the aim to assess the extent of beach-cast events and their relationship with abiotic factors. A Redundancy Analysis (RDA) and a permutational analysis of variance (PERMANOVA) were carried out to explore the relationship between macroalgae accumulation level and the abiotic variables: sea surface temperature, wind speed, wind direction, currents, maximum sea level, significant wave height, salinity, nitrate, ammonium, phosphate, precipitation and radiation. The citizen science campaign showed great participation, resulting in 404 submissions between July 2021 and September 2023. The campaign revealed that three species of macroalgae accumulated on the beaches of Algarve, Ulva sp. (with the presence of Ectocarpales and Dyctiotales) along the sandy eastern coast, and the invasive species Asparagopsis armata and Rugulopteryx okamurae in the rocky central and western beaches, respectively. The accumulations of R. okamurae increased from 2021 to 2023, were registered throughout the year and were more abundant than those of Ulva sp. and A. armata, which were only observed in spring and summer. The highest levels of R. okamurae beach-cast depositions were related to strong wave conditions, and high sea surface temperature and salinity. The accumulation of Ulva sp. was related to high sea surface temperature and salinity whereas A. armata was also correlated with winds parallel to the shore (NW-W). PERMANOVA analysis revealed that sea surface temperature and wave conditions had a significant effect on the overall abundance of macroalgae beach-cast accumulations. Overall, our citizen science campaign effectively involved the public, leading to the collection of important data on monitoring macroalgae accumulations. Through these findings, we were able to pinpoint the environmental, atmospheric, and hydrodynamic factors that contribute to their development, movement, and buildup along the Algarve coastlines.
1 Introduction
Macroalgae are extremely beneficial since they provide important ecosystem services such as essential structural habitat and food for invertebrates and fish. However, under specific environmental and oceanographic conditions, macroalgae can grow excessively and accumulate on beaches (Lapointe et al., 2018). Harmful macroalgal blooms (HMBs) cause high environmental and economic impacts (Lapointe et al., 2018) such as habitat loss, disruption of biogeochemical cycles and food webs, alteration of grazing, and the disappearance of seagrasses and corals (United Nations Environment Program [UNEP], 2005). In shallow coastal ecosystems, HMBs can deplete dissolved oxygen in the water column through respiratory decomposition, leading to the death of numerous aquatic organisms (Van Tussenbroek et al., 2017). In fact, several studies (NRC, 2000; Burkholder and Glibert, 2013; Lyons et al., 2014) have shown that HMBs cause a decrease in biodiversity. In addition, some bloom-forming species are invasive, which may lead to alterations in ecosystem structure and functioning (Convention on Biological Diversity, 2020). Beyond the ecological consequences, HMBs can cause social and economic impacts, including inhibiting recreation and enjoyment of the coastal zone, interfering with tourism, preventing water usage (e.g., obstructing navigation, pipelines, and fouling fishing lines and nets) (Smetacek and Zingone, 2013; Casal-Porras et al., 2021), and emitting toxic substances that result from macroalgal decomposition threatening human health (Samuel, 2011; Olguin-Maciel et al., 2022).
Monitoring programs for HMBs and biological invasions are crucial to assist stakeholders in the development of management strategies. Monitoring programs have shown an increased occurrence of HMBs and this trend is expected to remain for the foreseeable future (Smetacek and Zingone, 2013; Xiao et al., 2021). HMBs occur throughout the world in both temperate and tropical areas, mostly near the developed coastlines of Europe, North America, and East Asia (Joniver et al., 2021). One of the most well-known bloom former taxon is Sargassum spp (Phaeophyceae). Since 2011, there has been a significant build-up of Sargassum spp. along the coasts of the Caribbean Sea, the northern coast of South America, across the Atlantic Ocean, and up to the west coast of Africa, resulting in what is known as the Great Atlantic Sargassum Belt (Oviatt et al., 2019; Wang et al., 2019). In southern Portugal, Stigter et al. (2013) showed the relevance of groundwater nutrient loads on the formation of green algae blooms. When these large quantities of green algae cover vast areas of the sea, they are called green tides which can deteriorate the structure and function of marine biomes (Fletcher, 1996). Red HMBs have also been increasing along developing coastlines for decades (Lapointe et al., 2018). The red macroalgae Asparagopsis armata (Rhodophyta) is native to Southern Australia and New Zealand (Horridge, 1951) and was introduced in Europe in the 1920s. This species is now widely distributed from the British Isles to Senegal, including the Azores, Canary and Madeira Islands (Cacabelos et al., 2020), where it is considered an invasive species. The invasive species Rugulopteryx. okamurae (Phaeophyceae), which originated in the northwest Pacific (Verlaque et al., 2009), was introduced in the Thau lagoon in the Mediterranean in 2002 and found in 2015 on the shores of the Strait of Gibraltar (South Spain), where it showed extremely invasive behavior with important ecological and economic negative impacts (Garcia-Gómez et al., 2021). The arrival of this species to southern Portugal and to the Azores Atlantic islands was reported by Liulea et al. (submitted) and Faria et al. (2021), respectively. Several studies have monitored the spread and impact of R. okamurae (Ocaña et al., 2016; Sempere-Valverde et al., 2020; Liulea et al., submitted), including citizen science monitoring (Garcia-Gómez et al., 2021).
The ability of algae to become successful bloom formers depends on their physiological response to the growth-limiting factors of the local environment (Lapointe et al., 2018). The overgrowth of macroalgae has been attributed mainly to high nutrient loads originating from water run-off from agricultural and urban areas (Lotze and Schramm, 2001; Teichberg et al., 2010). Despite the critical role of nutrient load in the occurrence of HMBs, other abiotic and biotic factors such as light, temperature, CO2, grazing, precipitation, propagule bank size, and local species pool contribute to bloom development (Bermejo et al., 2023). Local hydrodynamics, waves, wind action and spring tides (when there is the greatest difference between the high and low) are also relevant to the transport and accumulation of beach-cast biomass on the shore (Ochieng and Erftemeijer, 1999; Martins et al., 2001; Lapointe et al., 2018; Isachenko et al., 2023). Addressing the causes of HMBs in various coastal regions can assist in developing effective management and mitigation strategies for this growing environmental issue (Smetacek and Zingone, 2013). Traditional monitoring and mapping strategies, such as field survey and sampling programs, are time-consuming, expensive, and spatially and temporally constrained (Mannino et al., 2021), and therefore scientists must consider all available approaches, including citizen science, to improve the early detection of HMBs. Environmental authorities and scientists are working to develop effective citizen science monitoring strategies (Courchamp et al., 2017). Being defined as “any environmental and/or biological data collection and analysis, undertaken by members of the general public, as individuals or as organized groups of citizens, with the guidance and/or assistance of scientists towards solving environmental and/or community questions” (Encarnação et al., 2021), citizen science programs may be useful supplementary tools for monitoring the distribution of HMBs.
Although citizen science is an unconventional technique for data acquisition, it may also be one of the most efficient ways to monitor and gather data. The ability of citizen science to generate high-quality datasets has been shown in various studies (Kosmala et al., 2016; McKinley et al., 2017; Iporac et al., 2022). The aims of this research are: 1) to use citizen science to monitor the spatial and temporal distribution of macroalgal accumulations on the beaches of southern Portugal, and 2) to investigate the environmental and oceanographic factors (sea surface temperature (SST), salinity, nutrients, winds, currents, waves, tides, precipitation and radiation), that are related to those events.
2 Material and methods
2.1 Study area
The Algarve region (Figure 1) in southern Portugal has a Mediterranean climate with an annual rainfall of less than 400 mm (Santos et al., 2010). The average air temperature ranges from 11°C in January to 27°C in August (https://www.worldweatheronline.com/). The monthly average SST is between 15°C in January and February and above 20°C in the summer months (Baptista et al., 2017). The eastern coast of Algarve is constituted by sandy beaches and the Ria Formosa coastal lagoon, whilst the central and western coasts are characterized by limestone and sandstone rocky shores with pockets of sandy beaches, and cliffs towards the west coast (Moura et al., 2006). The Ria Formosa is influenced by semidiurnal tides with a mean tidal range of 2 m, ranging from 1.5 m to 3.5 m (Jacob and Cravo, 2019). The ocean circulation in the Algarve follows a distinct seasonal pattern (Relvas et al., 2007). In the winter, southerly winds dominate ocean circulation, with poleward surface circulation present along the west coast. In late spring and summer, the prevailing northerly winds cause upwelling conditions. This phase is distinguished by a colder southward flow along the western coast, while westerly winds push upwelled water eastward along the southern shore (Relvas and Barton, 2005). However, during periods of northerly wind relaxation, warm water counter-currents run alongshore from the Gulf of Cadiz (Relvas and Barton, 2005; Garel et al., 2016). Moreover, the strong easterly winds blowing from the Gibraltar Strait (commonly known as “Levante”), which are quite frequent during summer, generate waves from east-southeast spreading warm waters from the Gulf of Cadiz to the southern coast of Portugal (Costa et al., 2001).
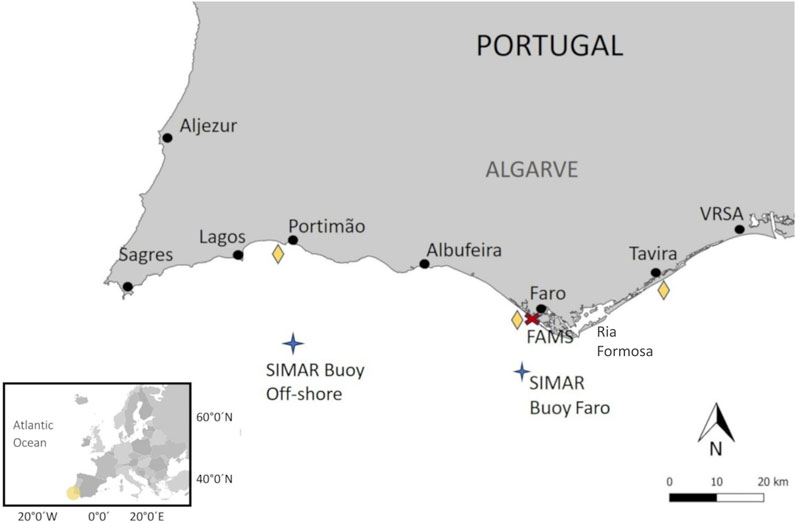
FIGURE 1. Location of the Algarve region (southern Portugal; southwestern Europe). The yellow diamonds represent the three stations used to obtain the environmental variables of the Copernicus Marine Service of the E.U. The location of the SIMAR Buoy, used to obtain the oceanographic variables from Puertos del Estado, is represented by a blue star. VRSA: Vila Real de Santo António. FAMS (red cross): Faro Airport Meteorological Station.
2.2 “Algas na praia” citizen science campaign
The “Algas na praia” (seaweed on the beach) web platform (https://www.ualg.pt/algas-na-praia) is a citizen science initiative to monitor the spatial and temporal accumulations of large amounts of macroalgae along the Portuguese beaches. The program, which started in July 2021 and is currently ongoing, seeks to engage beach users, beach life guards and professionals from touristic facilities, to provide data on macroalgae accumulations. An online form is available on the website in which citizens can register the occurrence of algae accumulations by submitting photos of handheld algae to allow their identification (Figure 2A) together with general view photographs of the accumulated macroalgae (Figure 2B), as well as the date and location of the macroalgae accumulation. Adhering to the ESCA 10 principles of citizen science (European Citizen Science Association, 2015), the project’s website outlines its scientific objectives and how citizen-submitted information will be utilized. Additionally, citizens can contact the research team directly at algasnapraia@ualg.pt for more information and updates. The results of the citizen science campaign are being made public on the website, where citizens can view the project’s development and the preliminary scientific findings.
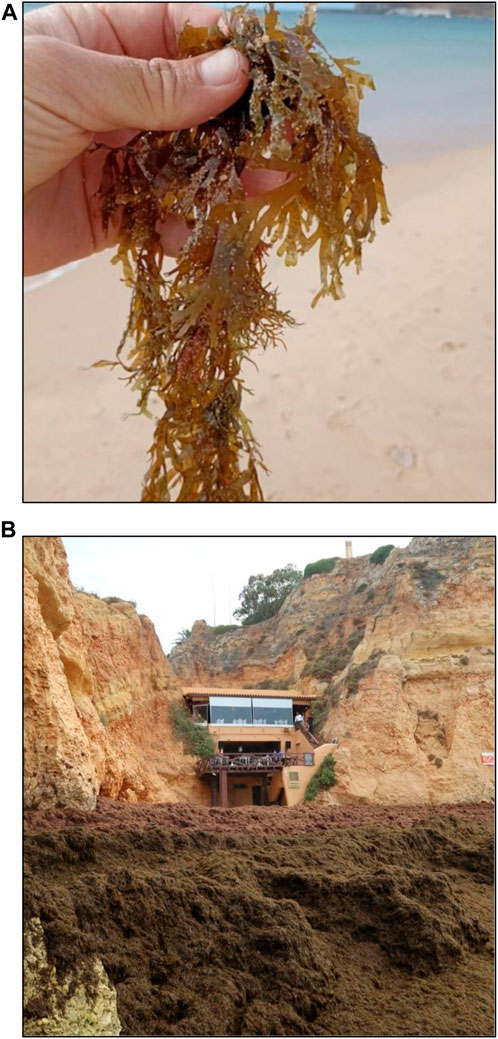
FIGURE 2. Photographs provided by anonymous citizens through “Algas na praia” platform. (A) Photograph of handheld Rugulopteryx okamurae and (B) overview of the species accumulation at Prainha beach, Alvor (Portimão) on 17 September 2022.
In order to enhance the program’s visibility and audience reach, a dissemination campaign was established in collaboration with the National Maritime Authority and the Portuguese Environment Agency. As part of this campaign, informative posters were prominently displayed in bathing areas to raise awareness of “Algas na Praia.” The University of Algarve (UAlg) also lent its support to the citizen science initiative, issuing press releases to promote the program. Additionally, the Centre of Marine Sciences (CCMAR) maintained consistent communication through its social media channels, including Facebook, Instagram, and LinkedIn, to further promote the program’s objectives. The program’s achievements were communicated through press releases, social media posts, and periodic website updates following each year’s data analysis. These efforts collectively contributed to a strong media presence and a significant audience reach across multiple social media platforms.
2.3 Environmental, oceanographic and atmospheric variables
The environmental and oceanographic variables considered to analyze their relationships with the presence of macroalgae accumulations on the beaches, were: 1) SST, 2) nitrate (NO3−), 3) ammonium (NH4+), 4) phosphate (PO4 3−), 5) salinity, 6) pH, 7) dissolved oxygen, and 8) currents. For currents, only the E-W component was included as it is the main surface current pattern in the study area (de Oliveira Júnior et al., 2022). These variables were gathered from the Copernicus Marine Service of the European Union (https://resources.marine.copernicus.eu) and averaged weekly. Outputs of the Atlantic-Iberian Biscay Irish- Ocean Biogeochemical Analysis and Forecast were downloaded. (IBI_ANALYSISFORECAST_PHY_005_001; processing level: L4). IBI-MFC product is based on an application of the biogeochemical model PISCES running simultaneously with the ocean physical IBI reanalysis, at a horizontal resolution of 1/12°. The retrieved modelled variables at 8-day temporal resolution included salinity, nitrate, phosphate, ammonium, pH, and dissolved oxygen. SST and currents (eastward seawater velocities, negative values indicate westward) were obtained from Iberian Biscay Irish- Ocean Physics Analysis and Forecast (IBI_ANALYSISFORECAST_BGC_005_004; processing level: L4), which is based on an eddy-resolving NEMO model application at 1/36° horizontal resolution. Three points along the Algarve coast and 2 km offshore were used as a proxy of the environmental conditions at the areas where macroalgae accumulate, from east to west: Tavira (37.08N, 7.65W), Faro (36.99N, 8.01W), and Portimão (37.1N, 8.6W).
Further oceanographic and atmospheric variables were included in the analysis: 1) wind direction (Wd), 2) wind speed (Ws), 3) significant wave height (SWH), 4) maximum sea level (MSL), 5) precipitation, and 6) radiation. Hourly wind speed (m/s) and wind direction (o) reported by oceanographic buoy 5018019 - SIMAR (36.83N, 8.0W) Faro, as well as significant wave height recorded by buoy 1046048–SIMAR (37.0N, 8.5W) Off-shore, were obtained from the Spanish public organization Puertos del Estado (https://www.puertos.es/es-es). Hourly wind speed and direction were weekly averaged following Grange, (2014) methodology and using the function timeaverage in the Openair package (v2.8-1; Carslaw and Ropkins, 2012) in the R software. Tidal data (MSL) was obtained from the Portuguese National Hydrographic Institute (https://www.hidrografico.pt/) for Faro harbour, and weekly maximum tidal level was calculated. Radiation was obtained for Faro station (36.99N, 8.01W) from Copernicus Atmosphere Data Store (https://ads.atmosphere.copernicus.eu/), based on observations from the Sea and Land Surface Temperature Radiometer (SLSTR), on board the ESA Sentinel 3A and 3B satellite. Daily cumulative precipitation was obtained from the data provided by IPMA (https://www.ipma.pt/pt/oclima/monitoriza.dia/) for the Faro Airport meteorological station.
2.4 Data analysis
The information provided by citizens in the online form of the “Algas na Praia” web platform included: 1) date, 2) location (including geolocation), 3) presence of the macroalgae in the water and/or sand, 4) macroalgae color, and 5) photographs of the specimen(s). The present study considers data on macroalgae accumulations submitted between July 2021 and September 2023. Only submissions that included the date of observation, a detailed location and photographs that allowed the identification of the species and its general abundance, were considered valid. Submissions for the same day and location were removed to avoid recurrence. Based on the submitted images of beaches and on the public available webcam images of some of the monitored beaches (https://beachcam.meo.pt/livecams/), a database of weekly abundances of accumulations between March and September 2023, was created. Five different levels of abundance (1-5) were considered (adapted from Iporac et al., 2022), following the description and image examples presented in Table 1. To assess the impact of the citizen science communication campaign, we tested the relationship between the number of press articles and the number of submissions on the platform using the Pearson’s correlation coefficient (r).
To assess the variability of macroalgal biomass over time and between species an ANOVA Type II (unbalanced data) was carried out. A posteriori pairwise comparison t-test was used when significant differences were found. All data were evaluated for normality and homogeneity of variance prior to statistical analysis. All differences were considered significant at p-value <0.05. To investigate the association between environmental-oceanographic variables and macroalgal accumulations on beaches, a Redundancy Analysis (RDA) was carried out (Legendre and Legendre, 1998). The variables were scaled, and multicollinearity was analyzed using the variance inflation factor (VIF). Since dissolved oxygen, pH, and radiation showed high collinearity (VIF >10) between them, only radiation (which explained more variability) was used in the analysis. Using the adonis2 function, a hierarchical PERMANOVA based on Bray-Curtis dissimilarities, with 999 permutations, was performed to analyze the partitioning of the distance matrix across sources of variations. The Vegan package was used to conduct all analyzes in R program (Oksanen, 2017).
3 Results
3.1 Citizen science contribution
The public engagement activities and scientific findings of “Algas na Praia” have garnered substantial media attention, including two interviews on national television, one interview on a radio channel, six radio appearances, one podcast interview, 18 printed newspaper articles, and 199 articles in various online newspapers (a few examples of which are shown in Figure 3). Furthermore, through social media, there have been 14 Facebook posts, two Instagram posts, and three LinkedIn posts, reaching an average of 1,600, 525, and 120 people per post, respectively.
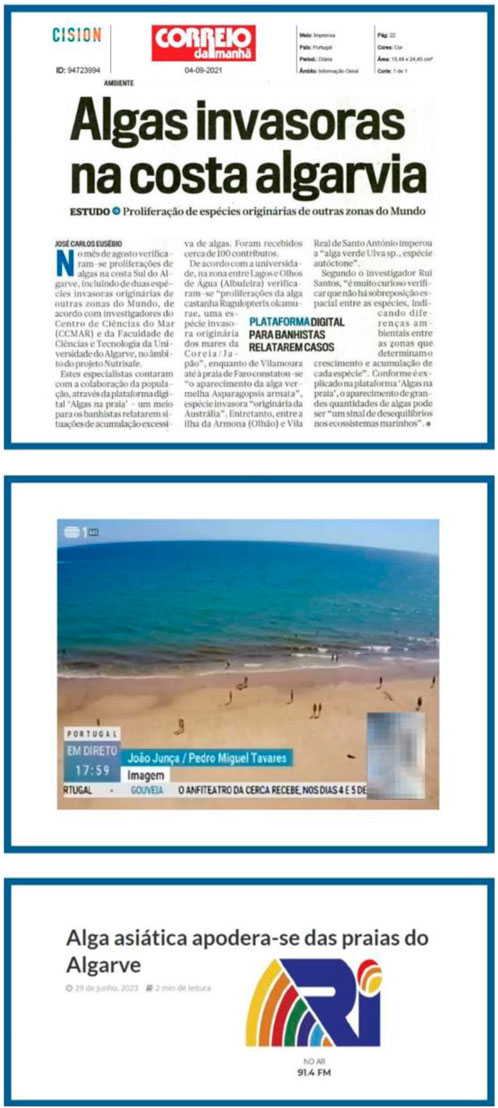
FIGURE 3. Some examples of media coverage including interviews on national television and radio as well as printed newspaper articles.
UAlg issued press releases to coincide with the launch of the “Algas na Praia” website in July 2021, and the summer season in June 2022. Additional press releases in August 2021 and April 2023 shared the results obtained from citizen participation. These press releases naturally led to a surge in media coverage and submissions to the “Algas na Praia” web platform (Figure 4), except in 2023 when the peak of submissions did not follow the peak of media articles. The number of submissions varied throughout the summer and fall of 2022 and 2023, with a total of 167 and 161 submissions in 2021 and 2022, respectively, and 76 submissions between January and September 2023. The highest number of weekly submissions was in August 2021, with 30 submissions received, likely due to the media’s high interest at the “Algas na Praia” campaign’s outset, coinciding with the peak of the bathing season. There was found to be a moderate positive correlation (r = 0.45, p-value <0.05) between the number of press articles and the number of submissions received on the platform.
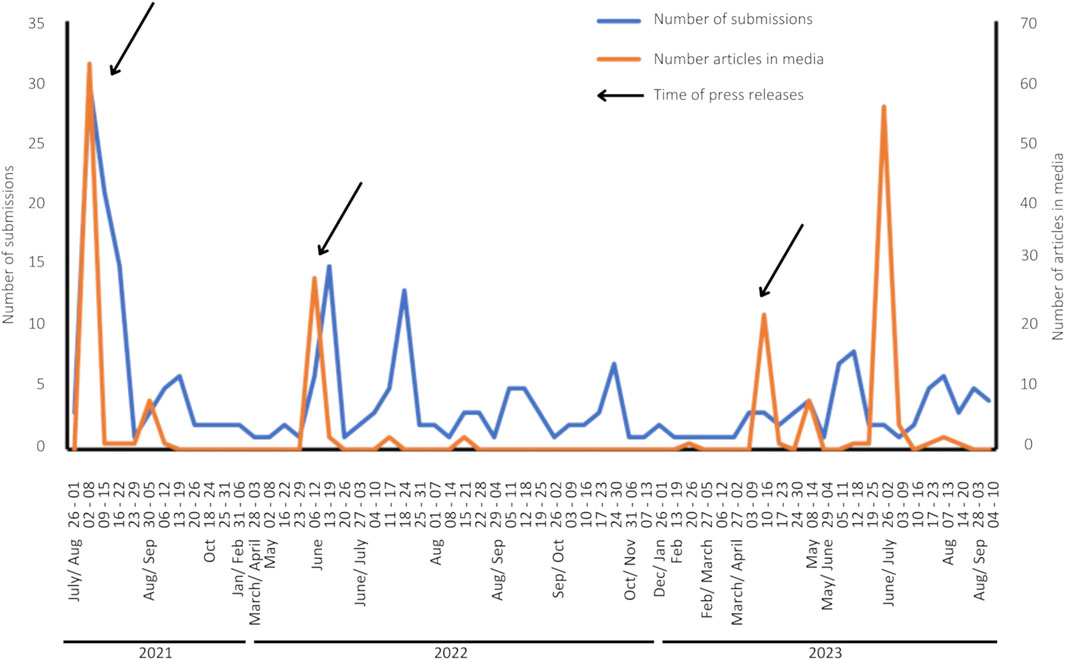
FIGURE 4. Timeline of the number of publications in media (orange) and number of weekly submissions in the “Algas na praia” platform (blue), arrows indicate the timing of press releases.
3.2 Spatio-temporal distribution of HMBs
Since launching the citizen science campaign in July 2021, 249 valid observations of macroalgae accumulations on Algarve beaches were reported, from which abundance levels were analyzed. No correlation was found (r = 0.08; p-value <0.05) between the weekly total number of submissions to the platform and the abundance level of macroalgae accumulation. HMBs of three main species were observed, the green algae Ulva sp., which bloomed mostly in the summer of 2021 (Figure 5) with the presence of additional species of brown macroalgae (Ectocarpales and Dyctiotales) at lower abundance levels during the summers of 2021 and 2023. The invasive red algae A. armata (tetrasporophyte stage), whose blooms were observed in the central zone during the summers of 2021 and of 2022, and the invasive brown R. okamurae that bloomed along the western coast throughout the years from 2021 to 2023, with high accumulations occurring all seasons except winter (only the week 26 December 2022 - 1 January 2023 concentrated high accumulations) (Figure 5).
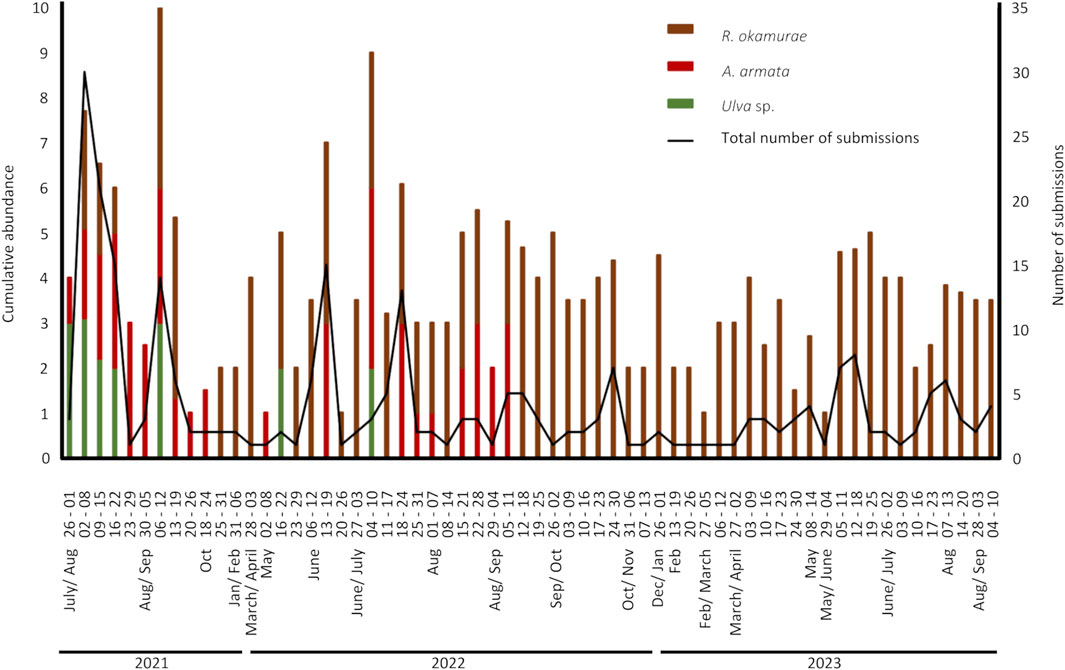
FIGURE 5. Cumulative abundance of macroalgae beach-casts per week (only weeks with submissions are shown) and total number of submissions (black line) in the “Algas na praia” platform between July 2021 and September 2023.
The accumulation levels of R. okamurae increased annually, reaching higher levels in both 2022 and 2023 (Figure 6). Average accumulations of R. okamurae were significantly higher than those of Ulva sp. and A. armata (p-value <0.05), which did not vary significantly between 2021 and 2022, and were not present in 2023 (Figure 6).
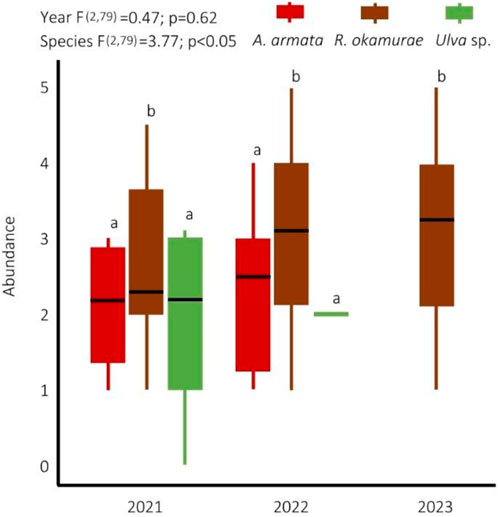
FIGURE 6. Yearly average abundance of macroalgae species from July 2021 to September 2023; Different letters above boxes represent significant differences between groups (two-way ANOVA followed by post hoc t-test; p-value <0.05).
The spatial distribution of the observations submitted by citizens revealed three differentiated zones: 1) western Algarve (between 40.91N, 8.91W and 37.09N, 8.18W), with accumulations of R. okamurae, which reached north Algarve (Vale da Telha, Aljezur) only in 2023, 2) central Algarve (between 37.07N, 8.11W and 37.03N, 8.04W) with a predominance of A. armata (tetrasporophyte stage) and eastern Algarve (between 37.05N, 7.74W and 37.17N, 7.40W) with accumulations of Ulva sp. and brown algae, from the Ectocarpales and Dictyotales order, in the eastern zone (Figure 7).
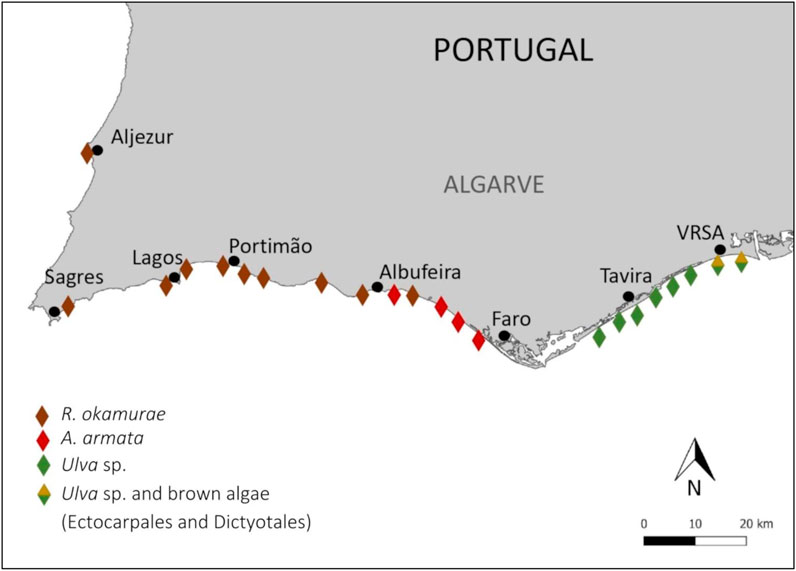
FIGURE 7. Spatial distribution of macroalgae accumulations on the Algarve coast. Diamonds represent the locations where the accumulations were observed. Colors show the different species of macroalgae. VRSA: Vila Real de Santo António.
3.3 Relationships with abiotic variables
The first axis of variation of RDA, which explained 18% of the total variance, represents mostly the variation of SWH (0.66) (Figure 8; Table 2). The second axis, which explained 9% of the total variance, represents mostly the variation of Salinity (Sal) and SST that were positively correlated with each other (−0.62 and −0.52, respectively). The third axis explained only 1% of the total variance and was positively correlated with SST (0.55). The abiotic variables represented by the constrained inertia significantly explained 28% of the total correlation.
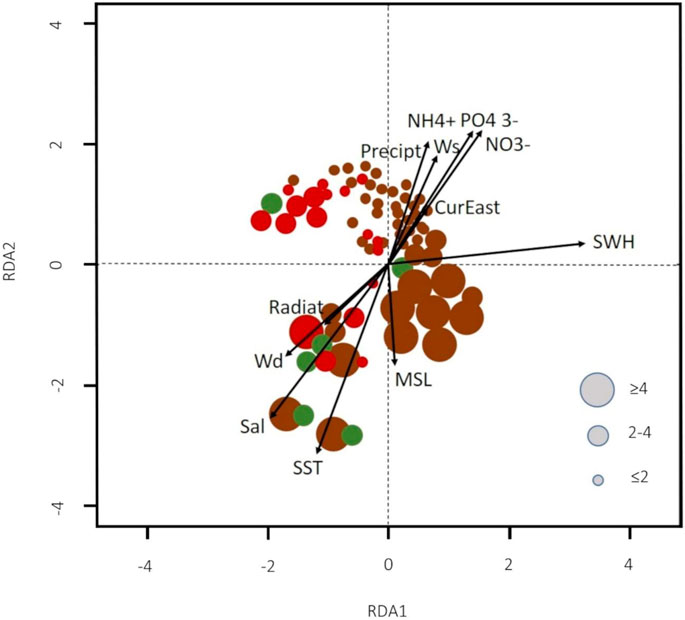
FIGURE 8. Redundancy analysis (RDA) showing the relationship between the abundance of weekly accumulations of macroalgae and environmental variables. The circles represent dates in weeks, the size shows the abundance levels and the colors represent the species of macroalgae (brown for Rugulopteryx okamurae, green for Ulva sp., and red for Asparagopsis armata). Positive values on currents data indicate eastward. Positive wind direction represents N-NW wind.
The relationships among environmental variables and the weekly accumulations of the three species on the beaches presented in Figure 8, reveal that the highest accumulations of R. okamurae are related to the combined effects of high SWH, SST and Sal, whereas the highest accumulations of A. armata are also related to low SWH and Wd (NW-W winds). The accumulations of Ulva sp. are more related to high Sal and SST. High nutrient concentration was not related to high accumulations of macroalgae on the beaches of Algarve.
The results of the PERMANOVA (Table 3A) indicated that the factors that had a significant influence on the overall abundance of the accumulations for the three species considered (Ulva sp., A. armata and R. okamurae) were SST and SWH (p-value <0.05), and Precipitation (p-value = 0.06). PERMANOVAs that were performed individually for each species, showed that SST was the only significant driver of R. okamurae accumulations (Table 3B) whereas SWH and Precipitation were significant for A. armata (Table 3C). PERMANOVA was not performed on Ulva sp. due to the limited sample size (n = 8).
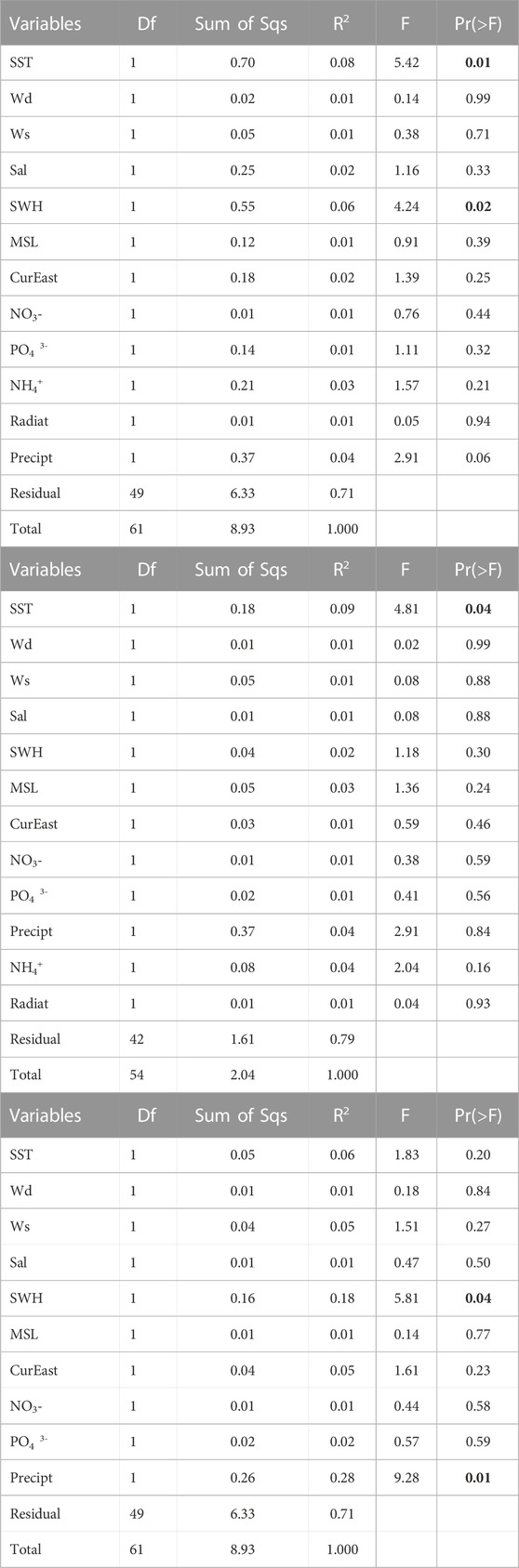
TABLE 3. Results of PERMANOVA based on Bray-Curtis dissimilarities for (A) the three species (Rugulopteryx okamurae, Asparagopsis armata and Ulva sp.), (B) Rugulopteryx okamurae and (C) Asparagopsis armata. In bold are significant effects (p-value <0.05).
4 Discussion
The “Algas na Praia” project has achieved tremendous success in acquiring long-term data on macroalgae accumulation levels along the coastline, using community-contributed data. The project has not only helped raise awareness and educate community members but also revealed some critical insights. The study found that HMB’s in southern Portugal are caused by three distinct species, each with a unique distribution pattern. The alien species R. okamurae and A. armata are found along western and central beaches, respectively, and the autochthonous species Ulva sp. is found along eastern beaches. The citizen science data also helped to investigate the relationship between beach-cast abundance levels and environmental factors that influence the development, transport, and accumulation of these species on the beaches.
Citizen science monitoring programs are particularly helpful in the monitoring of extensive areas at a low cost. Citizen science has already been implemented in the Algarve to monitor and detect invasive species such as the Atlantic blue crab (Encarnação, J. et al., 2021). García-Gómez et al. (2021) used photographs, provided by recreational divers, to monitor the effects of R. okamurae in the Strait of Gibraltar. Iporac et al. (2022) used community monitoring to investigate the spatiotemporal distribution of Sargassum spp. in the Caribbean, collecting photographs from volunteers using an online application. The “Algas na Praia” project, represents for the first time the use of citizen science data, without the recruitment of volunteers, to show spatiotemporal trends in macroalgae accumulations of different species and their relationship with various abiotic variables that may contribute to their development and transport. The dissemination campaign that resulted in extensive media coverage, and its positive correlation with the number of submissions to the platform shows the success of engagement efforts. However, since the number of observations was related to the number of media articles it was not possible to use those data as a proxy for the level of accumulations. Consequently, the abundance levels of macroalgal accumulations were inferred from the photographs submitted and the visualization of beach cams. Improvements in data collection are needed, such as complementing the citizen science program with in situ monitoring in several locations along the coast.
Interestingly, the spatial distribution of the three species that cause massive beach-cast depositions in southern Portugal are spatially separated. R. okamurae accumulations develop along the western shores of Algarve, whereas A. armata and Ulva sp. develop along central and eastern Algarve. Here, two other species of brown algae of the orders Ectocarpales and Dictyotales were also observed with lower abundance levels in the summer of 2021 and 2023 when there were no reports of Ulva sp. The spatial distribution of these three algae species along southern Portugal may be explained by substrate preference or limitation. While the western and central south coast of Algarve is characterized by rocky shores with pockets of sandy beaches and cliffs, the eastern zone is mostly sandy (Moura et al., 2006), including the Ria Formosa Barrier Island System with its coastal lagoon. The Ria Formosa lagoon encompasses a large region of intertidal mudflats where Ulva genus is predominant (Aníbal et al., 2007). The hydrodynamic patterns of the lagoon at Ria Formosa are primarily influenced by tides and wind (Mudge et al., 2008; Jacob and Cravo, 2019). The Ulva sp. blooms may be originated in the Ria Formosa lagoon system and due to tidal currents and certain wind conditions be transported to the beaches beyond the lagoon system, where they accumulate. In western Portugal, A. armata gametophyte is found from the intertidal to shallow subtidal areas, on rock or epiphytic, forming dense vegetation belts on exposed coasts (Silva et al., 2021). The beach-casts of the filamentous tetrasporophyte of this species along the sandy shores of central Algarve is strange, its origin may be the macro gametophytes that may develop on the rocky reef system that develops parallel to the shore at depths higher than 10 m (Leitão et al., 2007). On the other hand, R. okamurae shows a preference for illuminated rocky bottoms, with higher cover at depths between 0 and 20 m (García-Gómez et al., 2020; García-Gómez et al., 2021). In the Alboran Sea, Estévez et al. (2022) found a high capacity of R. okamurae to colonize different types of substrates such as rocky bottoms, multiple coralligenous organisms, artificial substrates and as epiphytes of the coral Dendrophyllia ramea.
The geographical expansion of R. okamurae along the western shore of Portugal, towards north was reported at the citizen platform in 2023. The rapid geographic spread of this species and the high capacity to colonized new areas have been described (García-Gómez et al., 2020; García-Gómez et al., 2021; Ruitton et al., 2021; Estévez et al., 2022). In addition, distribution models for R. okamurae (Ministerio de Transición Ecológica, 2022) showed the areas with favorable environmental conditions for the expansion of this species, including its occupation of the Mediterranean and North African coasts and almost the entire Iberian Peninsula coastline, reaching the Bay of Biscay.
Data from the citizen science platform revealed different temporal patterns for the three species of macroalgae. Information on Ulva sp. and A. armata, accumulations were only received during spring and summer 2021 and 2022 whereas R. okamurae accumulations were reported every season, with lower levels in winter. A similar seasonal pattern for Ulva blooms was found elsewhere. Bermejo et al. (2019) reported in two Irish estuaries Ulva blooms starting in April and ending in late November. Wang et al. (2010), Wang et al. (2012) and Liu et al. (2009) have also found this seasonal pattern for Ulva sp, with bloom formation from May to July and bloom decrease from late July to September. As well, the seasonal trend of biomass peaking during spring and starting to decrease in the summer was also found for A. armata in western Portugal (Silva et al., 2021). García-Gómez et al. (2020) and Ruitton et al. (2021) have also reported that R. okamurae is present throughout the year with higher abundance in summer in southern Spain and France, respectively. R. okamurae beach-cast abundance increased since 2021, the year of its introduction in southern Portugal, reaching a maximum in 2023.
The redundancy analysis of the relationships among data provided by the “Algas na Praia” platform and environmental and oceanographic variables revealed that the beach-cast depositions of R. okamurae are related to strong wave conditions, and high sea surface temperature and salinity. The accumulation of Ulva sp. is related to high sea surface temperature and salinity whereas A. armata was also correlated with winds parallel to the shore (NW-W). High nutrient concentrations were not related to high accumulations of macroalgae on the beaches of Algarve. However, it is important to note that this data was sourced from ocean biogeochemical/physical models, and the locations utilized as a proxy for environmental variables were offshore. Monitoring near-shore nutrient concentrations before and during the beach-casts events is needed to properly assess the effects of nutrients as diffuse loads of nutrients from agricultural or urban effluents may be an important trigger of macroalgae blooms. Nutrient availability is considered one of the main factors controlling the proliferation of HMBs (Lapointe et al., 2018). Despite the abiotic factors explaining only 28% of the total RDA correlation, the PERMANOVA analysis revealed that sea surface temperature and wave conditions had a significant effect on the overall abundance of macroalgae beach-cast accumulations.
The occurrence of large quantities of beach-cast biomass is conditioned by hydrodynamic forces and oceanographic factors that allow their detachment, transport and accumulation (e.g., Martins et al., 2001). In the Baltic Sea, Isachenko et al. (2023) described the importance of large wave heights in the periods (3 days) before beach-cast appearance and they suggested that a fully developed wave field with high wave heights was essential for the appearance of beach-cast biomass. In Kenya, increased amounts of beach-cast biomass have also been correlated to spring tides in combination with high wave energy and wind speed (Ochieng and Erftemeijer, 1999). In the contrary, Rutten et al. (2021) showed that arrivals of pelagic Sargassum spp. were associated with relatively low-energetic wave and wind conditions, and onshore directed waves and winds while high wave and wind activity would inundate the landed sargasso and flush it back into the water column. The combination of strong wave forces and spring tides results in high exposure to sea action, which may be responsible for the detachment of large quantities of R. okamurae, while the relaxation of wave energy accumulates them on the beach. The tetrasporophyte stage of A. armata occurs naturally as a small “pompon” of filaments that floats freely, and therefore without the need for detachment to be transported to the beaches. In fact, accumulations of this species are significantly correlated with lower SWH and NW-W winds (parallel to the southern Algarve coast) (Figure 8; Table 3C). The wind, transverse to the coast (NW-W) at the areas where A.armata accumulates, may transport the algae to the shore whereas the low wave energy allows their accumulation on the beach. The frequency and intensity of macroalgal deposition may also be influenced by small-scale coastal factors that occur daily and locally, which should be considered in future research.
An important factor for the development of enormous quantities of macroalgae biomass may be the possibility of macroalgae to grow free-floating during the transport and accumulation at the shores. This was observed for Ulva sp. (Yoshida et al., 2015), which allows the increase of its biomass during transportation. Mateo-Ramírez et al. (2023) and Figueroa et al. (2020) showed that floating R. okamurae also maintained a relatively high photosynthetic capacity, even after long dark periods.
Temperature and light have been suggested to be environmental variables that influence the proliferation of HMBs in addition to nutrient concentration (Lotze and Schramm, 2001). Mantri et al. (2011) showed that enhanced metabolism caused macroalgae growth to accelerate rapidly when temperatures were elevated. Increased temperature is also one of the environmental conditions that can enhance the growth and competitive output of macroalgal invaders (Cebrián and Ballesteros, 2010). Summers in the Algarve when higher macroalgae abundance levels were recorded, are characterized by increased light intensity, daylight duration, and temperature, which has been correlated to Asparagopsis sp. growth (Mata et al., 2017). Mihaila et al. (2023) studied the effect of temperature and light in the tetrasporangial production for A. armata, showing that increased temperature resulted in a mass increase in tetraspore release. According to Guiry and Dawes (1992), the greatest levels of tetrasporangial production for A. armata were found at 20°C, which matches the average summer temperatures in the Algarve (20.35 C° between 2016 and 2022; SIMAR Faro buoy). Warmer summer waters may increase A.armata tetrasporangial production, resulting in greater abundance of the accumulations found during this time. High level of A. armata abundance (>4) was shown to be significantly affected and negatively correlated with precipitation (Table 3C). While precipitation has been shown to impact HMBs proliferation through nutrient runoff, it is worth noting that the summers in Algarve, where A. armata is most abundant, are frequently dry. This observation suggests that the apparent correlation between the species’ accumulation levels and precipitation may be misleading.
García-Gómez et al. (2020) and Ruitton et al. (2021) showed that R.okamurae persists throughout the winter (less coverage), and spreads rapidly when conditions are favorable, which could explain the different peaks across the year. In its native habitat, R. okamurae is present around the year and its maximal growth rate is reached in spring and summer when the highest temperature is reached (22.4°C). Mercado et al. (2022) compared the minimum SST for the Strait of Gibraltar with the native habitats of R. okamurae, to conclude that higher winter temperatures could explain the invader success of this species. Lee and Kang (2020) tested the effect of increasing temperature on the growth of Ulva linza, showing a positive effect of increased temperature when in combination with high nutrient concentration.
The development and beach-cast deposition of macroalgae is the result of a complex interaction of various physical and environmental factors. This is the first study that uses citizen science data to show spatiotemporal trends in macroalgae accumulations and their relationship with abiotic and oceanographic variables. Further research must consider the ecophysiological responses of bloom-forming macroalgae to near shore, site-specific environmental conditions. This will enable the integration of production models with hydrodynamic transport models, thereby enabling the prediction and early detection of beach-cast depositions in southern Portugal. Such approach will allow the implementation of effective management strategies.
Data availability statement
The original contributions presented in the study are included in the article/Supplementary Material, further inquiries can be directed to the corresponding author.
Author contributions
JH: Data curation, Formal Analysis, Writing–original draft, Investigation. DS: Conceptualization, Funding acquisition, Project administration, Writing–review and editing. RA: Writing–review and editing. PR: Writing–review and editing. EG: Writing–review and editing. PV: Writing–review and editing. RS: Conceptualization, Funding acquisition, Project administration, Supervision, Writing–review and editing, Methodology.
Funding
The author(s) declare financial support was received for the research, authorship, and/or publication of this article. This study received funding from the NUTRISAFE project (AAC No. 41/ALG/2020—Project No. 072583—NUTRISAFE), and from FCT—Foundation for Science and Technology, through projects UIDB/04326/2020, UIDP/04326/2020 and LA/P/0101/2020.
Acknowledgments
The authors greatly appreciate all participants and citizens for their contribution to data collection on the “Algas na praia” platform. EG acknowledge the support of the Portuguese Foundation for Science and Technology (FCT) through the grant UID/MAR/00350/2020 attributed to CIMA, University of Algarve.
Conflict of interest
The authors declare that the research was conducted in the absence of any commercial or financial relationships that could be construed as a potential conflict of interest.
Publisher’s note
All claims expressed in this article are solely those of the authors and do not necessarily represent those of their affiliated organizations, or those of the publisher, the editors and the reviewers. Any product that may be evaluated in this article, or claim that may be made by its manufacturer, is not guaranteed or endorsed by the publisher.
Supplementary material
The Supplementary Material for this article can be found online at: https://www.frontiersin.org/articles/10.3389/fenvs.2023.1324600/full#supplementary-material
References
Aníbal, J., Rocha, C., and Sprung, M. (2007). Mudflat surface morphology as a structuring agent of algae and associated macroepifauna communities: a case study in the Ria Formosa. J. Sea Res. 57 (1), 36–46. doi:10.1016/j.seares.2006.07.002
Baptista, V., Silva, P. L., Relvas, P., Teodósio, M. A., and Leitão, F. (2017). Sea surface temperature variability along the Portuguese coast since 1950. Int. J. Climatol. 38 (3), 1145–1160. doi:10.1002/joc.5231
Bermejo, R., Green-Gavrielidis, L., and Gao, G. (2023). Editorial: macroalgal blooms in a global change context. Front. Mar. Sci. 10, 1204117. doi:10.3389/fmars.2023.1204117
Bermejo, R., Heesch, S., Mac Monagail, M., O’Donnell, M., Daly, E., Wilkes, R. J., et al. (2019). Spatial and temporal variability of biomass and composition of green tides in Ireland. Harmful Algae 81, 94–105. doi:10.1016/j.hal.2018.11.015
Burkholder, J. M., and Glibert, P. M. (2013). Eutrophication and oligotrophication. Encycl. Biodivers., 347–371. doi:10.1016/b978-0-12-384719-5.00047-2
Cacabelos, , Martins, G. M., Faria, J., Prestes, A. C., Costa, T., Moreu, I., et al. (2020). Limited effects of marine protected areas on the distribution of invasive species, despite positive effects on diversity in shallow-water marine communities. Biol. Invasions 22 (3), 1169–1179. doi:10.1007/s10530-019-02171-x
Carslaw, D. C., and Ropkins, K. (2012). Openair — an R package for air quality data analysis. Environ. Model. Softw. Environ. Data News 27–28, 52–61. doi:10.1016/j.envsoft.2011.09.008
Casal-Porras, I., Zubía, E., and Brun, F. G. (2021). Dilkamural: a novel chemical weapon involved in the invasive capacity of the alga Rugulopteryx okamurae in the Strait of Gibraltar. Estuar. Coast. Shelf Sci. 257, 107398. doi:10.1016/j.ecss.2021.107398
CBD Convention on Biological Diversity (2023). Invasive alien species. https://www.cbd.int/invasive.2020.CBD/WG2020/4/INF/2/Rev.2.
Cebrián, E., and Ballesteros, E. (2010). Invasion of Mediterranean benthic assemblages by red alga Lophocladia lallemandii (Montagne) F. Schmitz: depth-related temporal variability in biomass and phenology. Aquat. Bot. 92, 81–85. doi:10.1016/j.aquabot.2009.10.007
Copernicus Climate Change Service, (2021). Earth's radiation budget from 1979 to present derived from satellite observations. Copernic. Clim. Change Serv. (C3S) Clim. Data Store (CDS). doi:10.24381/cds.85a8f66e
Costa, M., Silva, R., and Vitorino, J. (2001). “Contribuição para o estudo do clima de agitação marítima na costa portuguesa,” in Proceedings of 2as Jornadas Portuguesas de Engenharia Costeira e Portuária (Sines: International Navigation Association PIANC), 20.
Courchamp, F., Fournier, A., Bellard, C., Bertelsmeier, C., Bonnaud, E., Jeschke, J. M., et al. (2017). Invasion biology: specific problems and possible solutions. Trends Ecol. Evol. 32 (1), 13–22. doi:10.1016/j.tree.2016.11.001
de Oliveira Júnior, L., Relvas, P., and Garel, E. (2022). Kinematics of surface currents at the northern margin of the Gulf of Cádiz. Ocean Sci. 18 (4), 1183–1202. doi:10.5194/os-18-1183-2022
Encarnação, J., Baptista, V., Teodósio, M. A., and Morais, P. (2021). Low-cost citizen science effectively monitors the rapid expansion of a marine invasive species. Front. Environ. Sci. 9. doi:10.3389/fenvs.2021.752705
Estévez, R. M., Palacios, M., Cervera, J. L., and González-Duarte, M. M. (2022). Expansion of the invasive alga Rugulopteryx okamurae (Dictyotaceae, Ochrophyta) in the Mediterranean Sea: first evidence as epiphyte of the cold-water coral Dendrophyllia ramea (Cnidaria: scleractinia). BioInvasions Rec. 11 (4), 925–936. doi:10.3391/bir.2022.11.4.11
European Citizen Science Association, (2015). Ten principles of citizen science. Berlin, Germany: European Citizen Science Association. doi:10.17605/OSF.IO/XPR2N
Faria, J., Prestes, A. C., Moreu, I., Cacabelos, E., and Martins, G. M. (2022). Dramatic changes in the structure of shallow-water marine benthic communities following the invasion by Rugulopteryx Okamurae (Dictyotales, Ochrophyta) in Azores (Ne Atlantic). Mar. Pollut. Bull. 175, 113358. doi:10.1016/j.marpolbul.2022.113358
Faria, J., Prestes, A. C., Moreu, I., Martins, G. M., Neto, A. I., and Cacabelos, E. (2021). Arrival and proliferation of the invasive seaweed Rugulopteryx okamurae in NE Atlantic islands. Bot. Mar. 65, 45–50. doi:10.1515/bot-2021-0060
Figueroa, F. L., Vega, J., Gómez-Valderrama, M., Korbee, N., Mercado-Carmona, J. M., Bañares, E., et al. (2020). Invasión de la especie exótica Rugulopteryx okamurae en Andalucía: estudios preliminares de la actividad fotosintética. Algas 56, 35–46.
Fletcher, R. (1996). “The occurrence of “green tides”—a review,” in Marine benthic vegetation: recent changes and the effects of eutrophication. Editors W. Schramm, and P. H. Nienhuis (Berlin, Germany: Springer), 7–43.
García-Gómez, J. C., Florido, M., Olaya-Ponzone, L., Sempere-Valverde, J., and Megina, C. (2021). The invasive macroalga Rugulopteryx okamurae: substrata plasticity and spatial colonization pressure on resident macroalgae. Front. Ecol. Evol. 9. doi:10.3389/fevo.2021.631754
García-Gómez, J. C., Sempere-Valverde, , González, R. A., Martínez-Chacón, M., Olaya-Ponzone, L., Sánchez-Moyano, E., et al. (2020). From exotic to invasive in record time: the extreme impact of Rugulopteryx okamurae (Dictyotales, Ochrophyta) in the Strait of Gibraltar. Sci. Total Environ. 704, 135408. Available at:. doi:10.1016/j.scitotenv.2019.135408
Garel, E., Laiz, I., Drago, T., and Relvas, P. (2016). Characterisation of coastal counter-currents on the inner shelf of the Gulf of Cadiz. J. Mar. Syst. 155, 19–34. doi:10.1016/j.jmarsys.2015.11.001
Greening, A. J., Sherwood, E. T., Pribble, R., and Johansson, J. O. R. (2014). Ecosystem responses to long-term nutrient management in an urban estuary: tampa Bay, Florida, USA. Coast. Shelf Sci. 151, A1–A16. doi:10.1016/j.ecss.2014.10.003
Guiry, M. D., and Dawes, C. J. (1992). Daylength, temperature and nutrient control of tetrasporogenesis in Asparagopsis armata (Rhodophyta). J. Exp. Mar. Biol. Ecol. 158, 197–217. doi:10.1016/0022-0981(92)90227-2
Horridge, (1951). Occurrence of Asparagopsis armata harv. Scilly Isles Nat. 167 (4253), 732–733. doi:10.1038/167732c0
Iporac, L. A. R., Hatt, D. C., Bally, N. K., Castro, A., Cardet, E., Mesidor, R., et al. (2022). Community-based monitoring reveals spatiotemporal variation of sargasso inundation levels and morphotype dominance across the Caribbean and South Florida. Aquat. Bot. 182, 103546. doi:10.1016/j.aquabot.2022.103546
Isachenko, I., Esiukova, E., and Chubarenko, I. (2023). Beach-cast appearance on the tide-less sea shore: parameters of favoring surface waves. Estuar. Coast. Shelf Sci. 281, 108219. doi:10.1016/j.ecss.2023.108219
Jacob, J., and Cravo, A. (2019). Recent evolution of the tidal prisms at the inlets of the western sector of the Ria Formosa, south coast of Portugal. Regional Stud. Mar. Sci. 31, 100767. doi:10.1016/j.rsma.2019.100767
Joniver, C. F., Photiades, A., Moore, P. J., Winters, A. L., Woolmer, A., and Adams, J. M. (2021). The global problem of nuisance macroalgal blooms and pathways to its use in the circular economy. Algal Res. 58, 102407. doi:10.1016/j.algal.2021.102407
Kosmala, M., Wiggins, A., Alexandra, S., and Simmons, B. (2016). Assessing data quality in citizen science. Inf. Syst. Quantitative Analysis Fac. 14, 551–560. doi:10.1002/fee.1436
Lapointe, B., Burkholder, J., and Van Alstyne, K. (2018). Harmful macroalgal blooms in a changing world: causes, impacts, and management. Harmful Algal Blooms, 515–560. doi:10.1002/9781118994672.ch15
Lee, J. E., and Kang, J. W. (2020). The interactive effects of elevated temperature and nutrient concentrations on the physiological responses of Ulva linza Linnaeus (Ulvales, Chlorophyta). J. Appl. Phycol. 32, 2459–2467. doi:10.1007/s10811-019-02031-0
Leitão, F., Santos, M., and Monteiro, C. C. (2007). Contribution of artificial reefs to the diet of the white sea bream (Diplodus sargus). ICES J. Mar. Sci. 64 (3), 473–478. doi:10.1093/icesjms/fsm027
Liu, D. Y., Keesing, J. K., Xing, Q. G., and Shi, P. (2009). World’s largest macroalgal bloom caused by expansion of seaweed aquaculture in China. Mar. Pollut. Bull. 58, 888–895. doi:10.1016/j.marpolbul.2009.01.013
Lotze, H., and Schramm, W. (2001). Ecophysiological traits explain species dominance patterns in macroalgal blooms. J. Phycol. 36 (2), 287–295. doi:10.1046/j.1529-8817.2000.99109.x
Lyons, D. A., Arvanitidis, C., Blight, A. J., Chatzinikolaou, E., Guy-Haim, T., Kotta, J., et al. (2014). Macroalgal blooms alter community structure and primary productivity in marine ecosystems. Glob. change Biol. 20 (9), 2712–2724. doi:10.1111/gcb.12644
Mannino, A. M., Borfecchia, F., and Micheli, C. (2021). Tracking marine alien macroalgae in the Mediterranean Sea: the contribution of citizen science and Remote Sensing. J. Mar. Sci. Eng. 9 (3), 288. doi:10.3390/jmse9030288
Mantri, V. A., Singh, R. P., Bijo, A. J., Kumari, P., Reddy, C. R. K., and Jha, B. (2011). Differential response of varying salinity and temperature on zoospore induction, regeneration and daily growth rate in Ulva fasciata (Chlorophyta, Ulvales). J. Appl. Phycol. 23, 243–250. doi:10.1007/s10811-010-9544-4
Martins, G. M., Cacabelos, E., Faria, J., Álvaro, N., Prestes, A. C. L., and Neto, A. I. (2019). Patterns of distribution of the invasive alga Asparagopsis armata Harvey: a multiscaled approach. Aquat. Invasions 14 (4), 582–593. doi:10.3391/ai.2019.14.4.02
Martins, I., Pardal, M. A., Lillebø, A. I., Flindt, M. R., and Marques, J. C. (2001). Hydrodynamics as a major factor controlling the occurrence of green macroalgal blooms in a eutrophic estuary: a case study on the influence of precipitation and river management. Estuar. Coast. Shelf Sci. 52, 165–177. doi:10.1006/ecss.2000.0708
Mata, L., Lawton, R. J., Magnusson, M., Andreakis, N., de Nys, R., and Paul, N. A. (2017). Within-species and temperature-related variation in the growth and natural products of the red alga Asparagopsis taxiformis. J. Appl. Phycol. 29 (3), 1437–1447. doi:10.1007/s10811-016-1017-y
Mateo-Ramírez, Á., Iñiguez, C., Fernández-Salas, L. M., Sánchez-Leal, R. F., Farias, C., Bellanco, M. J., et al. (2023). Healthy thalli of the invasive seaweed Rugulopteryx okamurae (Phaeophyceae) being massively dragged into deep-sea bottoms by the Mediterranean Outflow Water. Phycologia 62 (2), 99–108. doi:10.1080/00318884.2023.2177057
McKinley, D. C., Miller-Rushing, A. J., Ballard, H. L., Bonney, R., Brown, H., Cook-Patton, S. C., et al. (2017). Citizen science can improve conservation science, natural resource management, and environmental protection. Biol. Conserv. 208, 15–28. doi:10.1016/j.biocon.2016.05.015
Mercado, J., Gómez-Jakobsen, F., Korbee, A. A., Bonomi-Baruf, J. i., Muñoz, M., Muñoz, M., et al. (2022). Analyzing environmental factors that favor the growth of the invasive brown macroalga Rugulopteryx okamurae (Ochrophyta): the probable role of the nutrient excess. Mar. Pollut. Bull. 174, 113315. doi:10.1016/j.marpolbul.2021.113315
Mihaila, A. A., Lawton, R. J., Glasson, C. R., and Magnusson, M. (2023). Early hatchery protocols for tetrasporogenesis of the antimethanogenic seaweed Asparagopsis armata. J. Appl. Phycol. 35, 2323–2335. doi:10.1007/s10811-023-03029-5
Ministerio para la Transición Ecológica (2020). Estrategia de control del alga Rugulopteryx okamurae en España. https://www.miteco.gob.es/content/dam/miteco/es/biodiversidad/publicaciones/estrategia_rokamurae_cs_28072022_tcm30-543560.pdf.
Moura, L. A., Veiga-Pires, C., Boski, T., and Tigano, E. (2006). Morphological features and processes in the central Algarve rocky coast (South Portugal). Geomorphology 81, 345–360. doi:10.1016/j.geomorph.2006.04.014
Mudge, S. M., Icely, J. D., and Newton, A. (2008). Residence times in a hypersaline lagoon: using salinity as a tracer. Estuar. Coast. Shelf Sci. 77 (2), 278–284. doi:10.1016/j.ecss.2007.09.032
Nrc National Research Council, (2000). Clean coastal waters: understanding and reducing the effects of nutrient pollution. Washington, DC, USA: National Academy Press.
Ocaña, O., Alfonso-Carrillo, J. M., and Ballesteros, E. (2016). Massive proliferation of a dictyotalean species (Phacophyccae, Ochriohyta) througn the strait of Gibraltar. Rev. Acad. Canar. Ciencias 28, 165–169.
Ochieng, C. A., and Erftemeijer, P. L. (1999). Accumulation of seagrass beach-cast along the Kenyan coast: a quantitative assessment. Aquat. Bot. 65 (1-4), 221–238. doi:10.1016/s0304-3770(99)00042-x
Oksanen, J. (2017). Vegan: an introduction to ordination. Accessed https://cran.r-project.org/package=vegan.
Olguin-Maciel, E., Leal-Bautista, R. M., Alzate-Gaviria, L., Domínguez-Maldonado, J., and Tapia-Tussell, R. (2022). Environmental impact of Sargassum spp. landings: an evaluation of leachate released from natural decomposition at Mexican Caribbean coast. Environ. Sci. Pollut. Res. 29 (60), 91071–91080. doi:10.1007/s11356-022-22123-8
Oviatt, C. A., Huizenga, K., Rogers, C. S., and Miller, W. J. (2019). What nutrient sources support anomalous growth and the recent Sargassum mass stranding on Caribbean beaches? A review. Mar. Pollut. Bull. 145, 517–525. doi:10.1016/j.marpolbul.2019.06.049
Relvas, P., and Barton, E. D. (2005). A separated jet and coastal counterflow during upwelling relaxation off Cape São Vicente (Iberian Peninsula). Cont. Shelf Res. 25, 29–49. doi:10.1016/j.csr.2004.09.006
Relvas, P., Barton, E. D., Dubert, J., Oliveira, P. B., Peliz, A., Da Silva, J. C. B., et al. (2007). Physical oceanography of the western Iberia ecosystem: latest views and challenges. Prog. Oceanogr. 74 (2-3), 149–173. doi:10.1016/j.pocean.2007.04.021
Ruitton, S., Blanfuné, A., Boudouresque, C.-F., Guillemain, D., Michotey, V., Roblet, S., et al. (2021). Rapid spread of the invasive Brown alga Rugulopteryx okamurae in a national park in provence (France, mediterranean sea). Water 13, 2306. doi:10.3390/w13162306
Rutten, J., Arriaga, J., Montoya, L. D., Mariño-Tapia, I. J., Escalante-Mancera, E., Mendoza, E. T., et al. (2021). Beaching and natural removal dynamics of pelagic sargassum in a fringing-reef lagoon. J. Geophys. Res. Oceans 126 (11), e2021JC017636. doi:10.1029/2021jc017636
Santos, J. F., Pulido-Calvo, I., and Portela, M. M. (2010). Spatial and temporal variability of droughts in Portugal. Water Resour. Res. 46 (3). doi:10.1029/2009wr008071
Sempere-Valverde, J., Ostal, E., Maestre, M., González, R., Bazairi, H., and Espinosa, F. (2020). Impacts of the non-indigenous macroalgae Rugulopteryx okamurae on a Mediterranean coralligenous community (Strait of Gibraltar): the role of long-term monitoring. Ecol. Indic. 121, 107135. doi:10.1016/j.ecolind.2020.107135
Silva, C. O., Lemos, M., Gaspar, R., Gonçalves, C., and Neto, J. M. (2021). The effects of the invasive macroalgae Asparagopsis armata on native rock pool communities: evidences from experimental exclusion. Ecol. Indic. 125, 107463. doi:10.1016/j.ecolind.2021.107463
Simpson, A., Jarnevich, C., Madsen, J., Westbrooks, R., Fournier, C., Mehrhoff, L., et al. (2009). Invasive Species Information Networks: collaboration at multiple scales for prevention, early detection, and rapid response to invasive alien species. Biodiversity 10 (2-3), 5–13. doi:10.1080/14888386.2009.9712839
Smetacek, V., and Zingone, A. (2013). Green and golden seaweed tides on the rise. Nature 504, 84–88. doi:10.1038/nature12860
Stigter, T. Y., Carvalho Dill, A., Malta, E.-j., and Santos, R. (2013). “Nutrient sources for green macroalgae in the Ria Formosa lagoon – assessing the role of groundwater,” in Selected papers on hydrogeology - groundwater and ecosystems. Editors L. Ribeiro, T. Y. Stigter, A. Chambel, M. T. Condesso de Melo, J. P. Monteiro, and A. Medeiros (Boca Raton, Florida, United States: Taylor and Francis), 153–167.
Teichberg, M., Fox, S., Olsen, Y., Valiela, I., Martinetto, P., Iribarne, O., et al. (2010). Eutrophication and macroalgal blooms in temperate and tropical coastal waters: nutrient enrichment experiments with Ulva spp. Glob. Change Biol. 16 (9), 2624–2637. doi:10.1111/j.1365-2486.2009.02108.x
United Nations Environment Program (UNEP) (2005). Marine and coastal ecosystems and human wellbeing: a synthesis report based on the findings of the millennium ecosystem assessment. Nairobi: UNEP, 76.
Van Tussenbroek, B. I., Arana, H. A. H., Rodríguez-Martínez, R. E., Espinoza-Avalos, J., Canizales-Flores, H. M., González-Godoy, C. E., et al. (2017). Severe impacts of brown tides caused by Sargassum spp. on near-shore Caribbean seagrass communities. Mar. Pollut. Bull. 122 (1-2), 272–281. doi:10.1016/j.marpolbul.2017.06.057
Verlaque, M., Steen, F., and De Clerck, O. (2009). Rugulopteryx (Dictyotales, phaeophyceae), a genus recently introduced to the mediterranean. Phycologia 48 (6), 536–542. doi:10.2216/08-103.1
Wang, C., Yu, R.-C., and Zhou, M.-J. (2012). Effects of the decomposing green macroalga Ulva (Enteromorpha) prolifera on the growth of four red-tide species. Harmful Algae 16, 12–19. doi:10.1016/j.hal.2011.12.007
Wang, J., Jiang, P., Cui, Y., Li, N., Wang, M., Lin, H., et al. (2010). Molecular analysis of green-tide-forming macroalgae in the Yellow Sea. Aquat. Bot. 93 (1), 25–31. doi:10.1016/j.aquabot.2010.03.001
Wang, M., Hu, C., Barnes, B. B., Mitchum, G., Lapointe, B., and Montoya, J. P. (2019). The great Atlantic sargassum belt. Science 365 (6448), 83–87. doi:10.1126/science.aaw7912
Xiao, J., Wang, Z., Liu, D., Fu, M., Yuan, C., and Yan, T. (2021). Harmful macroalgal blooms (HMBs) in China's coastal water: green and golden tides. Harmful Algae 107, 102061. doi:10.1016/j.hal.2021.102061
Xiao, J., Xiaohong, Z., Chunlei, G., Meijie, J., Ruixiang, L., Zongling, W., et al. (2016). Effect of temperature, salinity and irradiance on growth and photosynthesis of ulva prolifera. Acta Oceanol. Sin. 35, 114–121. doi:10.1007/s13131-016-0891-0
Keywords: beach-cast, citizen science, environmental factors, invasive macroalgae, monitoring, coast of Portugal
Citation: Herrero JJ, Simes DC, Abecasis R, Relvas P, Garel E, Ventura Martins P and Santos R (2023) Monitoring invasive macroalgae in southern Portugal: drivers and citizen science contribution. Front. Environ. Sci. 11:1324600. doi: 10.3389/fenvs.2023.1324600
Received: 19 October 2023; Accepted: 13 November 2023;
Published: 27 November 2023.
Edited by:
Ahmet Erkan Kideys, Middle East Technical University, TürkiyeReviewed by:
Antonella Petrocelli, National Research Council (CNR), ItalyLeonel Pereira, University of Coimbra, Portugal
Copyright © 2023 Herrero, Simes, Abecasis, Relvas, Garel, Ventura Martins and Santos. This is an open-access article distributed under the terms of the Creative Commons Attribution License (CC BY). The use, distribution or reproduction in other forums is permitted, provided the original author(s) and the copyright owner(s) are credited and that the original publication in this journal is cited, in accordance with accepted academic practice. No use, distribution or reproduction is permitted which does not comply with these terms.
*Correspondence: Javier Jiménez Herrero, jjherrero@ualg.pt