- Instituto Politécnico Nacional, Escuela Nacional de Ciencias Biológicas, Laboratorio de Hidrobiología Experimental, Mexico City, Mexico
Cladocerans are frequently used as test organisms in aquatic toxicology studies. For practical reasons (bioethical and economic), efforts are currently made in search of alternative animal models and in defining short-lasting test methods that will allow reliable evaluation of the chronic effect of chemical contaminants. The use of small-sized invertebrates, like the Ceriodaphnia dubia cladoceran, represents a good option and has been included in diverse testing protocols; however, the use of reduced volumes and shortened exposure times, as well as higher temperatures to accelerate their development could influence the results and lead to imprecise conclusions. The present study aimed to evaluate the effects of different test volumes, temperature, and exposure time on the toxicity of hexavalent chrome in C. dubia. In acute and chronic assays, three test volumes (5, 15, and 25 mL) were used. Once the LC50 was determined, four sublethal concentrations (0.01, 0.02, 0.04, and 0.08 mg L−1) of Cr(VI) were applied daily to each of the three test volumes for 21 days, evaluating the progeny and survival of adults. The LC50 values did not differ at 20°C, but at 25°C they were significantly lower in 5 mL. The chronic toxic effects varied depending on the test volume and exposure time. Significant effects were observed on day 7 of exposure (corresponding to the third reproduction) in the accumulated progeny only in the volume of 5 mL. Based on the aforementioned, it is necessary to revise whether the short-term and small-scale methods are not underestimating the detection of chronic effects.
1 Introduction
Microcrustaceans of the Daphniidae family represent one of the most important zooplankton groups in freshwater ecosystems as they are one of the main primary consumers (filter-feeders), influencing the energy transfer of primary producers to higher trophic levels. In addition, they affect the population dynamics of bacteria, flagellated heterotrophic protozoa, Chlorophyceae microalgae, and even some rotifers (Arnold, 1971; Pace and Vaqué, 1994).
Some cladoceran species have been used as test organisms in aquatic toxicology and ecotoxicology studies because they present advantages like ease of handling and cultivation under laboratory conditions (Versteg et al., 1997). Noteworthy is also their short life cycle, which can fluctuate, depending on temperature, from between 13 and 15 days for Moina micrura (Murugan, 1975) to more than 60 days for Daphnia magna (Martínez-Jerónimo et al., 1994). These organisms reproduce asexually by parthenogenesis in favorable environmental conditions; the produced embryos undergo direct development (egg development occurs in the incubating chamber, without larval stages), giving rise to juveniles morphologically and genetically similar to the mother (Pennak, 1978). The species most used in aquatic toxicology due to its outstanding sensitivity to a wide range of toxic compounds is Daphnia magna, which has been used as a test organism since 1933 (Baudo, 1987).
However, because of the restricted geographical distribution of D. magna (Holarctic biogeographical region), other species of broader distribution have also been chosen, like Ceriodaphnia dubia, which is a small-sized species (adult reach approximately 1 mm length) globally distributed (Versteg et al., 1997; Ceresoli and Gagneten, 2003) that develops adequately both in lentic ecosystems and in shallow and temporal water bodies.
Both D. magna and C. dubia have been used for decades as test organisms to assess the toxic effects of chemical and effluent contaminants. A profuse statistical analysis has revealed similitudes in the sensitivity of both species in both acute and chronic exposures (Connors et al., 2022); therefore, according to these authors, the use of the 7-day abbreviated method for C. dubia is equally reliable and informative as the 21-day test for D. magna.
The first proposal to evaluate the chronic toxic effects in a short-lasting bioassay with Daphnia was made in the early 1970s. However, other options have been reviewed because the assay with D. magna requires 21 days, which is costly and time-consuming, and this cladoceran is a temperate northern hemisphere species (Versteeg et al., 1997). Afterward, Mount and Norberg (1984) suggested a method with Ceriodaphnia reticulata and C. dubia/affinis, applicable to poor volume samples (effluents or leachates) or when results in short times were required (v. gr. in expensive on-site effluent studies). According to the method, this evaluation is performed in 7 days (the time in which three reproductions are obtained) in 30-mL beakers, using 15 mL of test solution, feeding daily with baker’s yeast, and incubating at 25°C. With some modifications of the original method, like feeding with yeast-Cerophyl®-trout chow (YCT) and an algal suspension, USEPA included this test since its proposal and is currently among the short-term methods for the evaluation of effluents (Method 1002.0, US EPA, 2002), having determined that the intra- and inter-laboratory variability is similar to that of other biological tests (DeGraeve et al., 1992). In the face of the possibility of attaining information on chronic toxic effects in a relatively short time, but with organisms that would allow for a better understanding and interpretation of the possible effects on the aquatic biota, the same proposal of three reproductions has been applied to similar species, like Ceriodaphnia cornuta (Dehui, 1989).
In its origin, this was a proposal that presented advantages over the one suggested by the OECD for D. magna, which requires 21 days (Sims et al., 1993; OECD, 2012); therefore, it was widely accepted as a short-term method to determine the chronic toxic effects of effluents and chemical substances in general (Versteg et al., 1997).
Although Mount and Norberg (1984) suggest that the 7-day period can be extended if more broods are desired, the truth is that a large number of subsequent studies were performed taking 7 days and three reproductions (at 25°C), as attested in the vast specialized scientific literature. However, other studies have questioned both the variability of the method and the validity of the results obtained to infer chronic toxic effects (Serben, 2015).
The need to have information in even shorter times has led to investigating the possibility of reducing the exposure time using C. dubia, aiming at obtaining results in 4 days (Oris et al., 1991). Although in this proposal the time of follow-up is the one reduced and not the exposure time because it is until day three that organisms (pre-adults) are exposed to the material to be evaluated, making observations on fecundity and survival on the following 4 days, resulting in total the same period of 7 days.
The idea of attaining chronic exposures in less time has also been oriented to using test organisms with shorter life cycles, like rotifers (Snell and Moffat, 1992). Although this test would allow evaluation in 2 days, its inconvenience lies in the handling of organisms because, besides their small size, two consecutive generations can be mixed depending on the evaluator’s expertise.
The increase in incubation temperature, within the tolerance interval of the test organisms, is another way of accelerating their development and shortening the time between successive reproductions (Martínez-Jerónimo et al., 1999; Rodríguez-Estrada et al., 2003), allowing for a reduction in exposure times in chronic assays.
Reduction in the test volumes in acute toxicity evaluations has also been investigated to reduce the required volumes of samples, the generation of wastes, and the space and infrastructure for toxicity evaluations. The reduced availability of some solutions (Powell et al., 1996) or the product whose toxicity will be evaluated (Baumann et al., 2014) has also been determined. In this regard, Powell et al. (1996) used 48-well microtiter plates containing 1 mL of the test solution and compared it with the standard USEPA assay for the evaluation of acute toxicity and found a remarkable similarity in both tests, although they did not report the statistical comparison. In turn, Baumann et al. (2014) performed acute toxicity tests in 6- and 24-well microtiter plates and compared their results with the 202-OECD protocol for the determination of acute effects (OECD, 2012); they concluded that even the reduction to the smallest volume (one neonate in 2 mL) produced comparable results. In another study, Grintzalisa et al. (2017) evaluated the effect of reducing the volume and size of the test vessels using 6-, 12-, 24-, 48-, and 96-well microtiter plates, with densities of 0.1, 1, and 3.3 neonates mL−1; in all cases, they recorded similar mortality effects produced by CdCl. The previously described evidence demonstrates the feasibility of reducing the test volume in acute toxicity evaluations with a large cladoceran like D. magna and allows inferring that similar results could be obtained in smaller-sized cladocerans.
During the evaluation of the toxic response in bioassays, it is essential to know the effect exerted by both the testing volume and the exposure time and to determine whether an increase in temperature could modify the results in acute assays. The latter are fundamental methodological conditions in the detection and quantification of toxicant effects since, currently, there is a marked tendency to modify them to decrease costs and attain quantifiable information in less time. However, the consequences of modifying factors on the toxic response of organisms must be known for both acute and chronic exposures.
Therefore, this study aimed to establish the effect of temperature (20°C and 25°C) on the acute toxic response in three test volumes to determine whether the volume can affect the acute toxic response (LC50). We also evaluated how different combinations of test volumes and exposure times affect fecundity and survival of C. dubia, determining the best-suited values of both factors that will allow detecting adequately and reliably the effects of a toxic compound like hexavalent chrome, which is considered a reference toxicant (Dorn et al., 1987; USEPA, 2002).
2 Materials and methods
2.1 Test organisms
A clonal strain of Ceriodaphnia dubia obtained from the Cladoceran strain collection of the Experimental Hydrobiology Laboratory, National School of Biological Sciences, Instituto Politécnico Nacional (IPN), Mexico City, was used. The strain was isolated from different samplings performed in the Estado de México and has been kept under controlled culture conditions for more than 30 years.
C. dubia was fed the green microalga Pseudokirchneriella subcapitata, which was obtained from the microalgae collection of the Experimental Hydrobiology Laboratory, National School of Biological Sciences, IPN, Mexico City, and cultured in autoclave-sterilized Bold Basal medium, under constant light and continuous aeration. The algal biomass was separated from the culture medium and refrigerated for a maximum of 1 week. During this time, microalgae were supplied as feed to provide always fresh food.
The C. dubia strain was maintained in reconstituted hard water (RHW) (206 mg L−1 CaCl2⋅2H2O, 247 mg L−1 MgSO4⋅7H2O, 193 mg L−1 NaHCO3, and 8 mg L−1 KCl) (US EPA, 2002b). For the acute toxicity effect, the organisms were pre-conditioned at 20°C and 25°C for 30 days to avoid effects on the progeny due to temperature changes. After this period, a reproducer batch was established for each experimental condition, obtaining neonates (age <2 h) from this batch to perform the experiments.
2.2 Effect of temperature and test volume on the mean lethal concentration (LC50)
We determined the 48-h LC50 for the reference toxicant hexavalent chromium (Cr(VI)) (as K2Cr2O7, J. T. Baker, 99% purity), applying the test procedure established by US EPA (2002b), but handling test volumes of 5, 15, and 25 mL; assays were performed in borosilicate glass tubes (10 mL) or beakers (25 and 50 mL). As dilution water, RHW was used. Bioassays were incubated in an environmental chamber at 20°C and 25°C, with a 16:8 photoperiod. For each bioassay, six Cr(VI) concentrations with 30 neonates per concentration divided in three replicates were used; at least seven determinations of LC50 were performed for each volume and temperature.
2.3 Subchronic exposure to Cr(VI) in different test volumes at 25°C
Once the LC50 at 25°C had been determined, four sublethal concentrations were established to evaluate the Cr(VI) effects in subchronic exposures in the three test volumes (5, 15. and 25 mL). Following Mount and Norberg (1984), for these experiments, a total of 10 replicates were performed with neonates (age <2 h) that were individually and randomly distributed in the test vessels of each treatment. A negative control series was also established for each volume with the same number of replicates containing only the respective RHW volume. The microalga P. subcapitata was used as food at a concentration of 1 ×106 cells mL−1. The test solution and the food were renewed daily. The test vessels were monitored daily, and the produced progeny was separated, counted, and discarded at the start of reproduction and after that. Experiments lasted 21 days at 25°C, with a photoperiod of 16:8, in an environmental chamber.
The progeny was recorded daily and grouped per the number of clutch to analyze the chronic effects of Cr(VI) at successive times in each treatment until the end of the assay (day 21). Because age had also been recorded, the average age of the progenitors in each clutch could be established. The accumulated progeny in all treatments was also determined.
The actual Cr(VI) concentration in treatments was determined with the HACH 8023 (1,5-diphenylcarbohydrazide) method.
2.4 Statistical analysis
The LC50 values for acute and chronic effects were determined with the Probit method by applying the Risk Hazard Assessment Tools (RA), ver.1.0, academic software. LC50 results were compared with two-way ANOVA, taking temperature (20°C and 25°C) test volume (5, 15, and 25 mL) as factors; normality of data (Shapiro-Wilk test) and homoscedasticity (Bartlett’s Test for Homogeneity of Variances) were confirmed before performing the ANOVAs. The post hoc multiple comparisons were performed with the Tukey test, and the Dunnett test was used for comparisons with the control.
Survival curves were determined using the product limit (Kaplan-Meir) method and compared using the Log-rank (Mantel-Cox) test. When significant differences were determined (p = 0.05), pairwise comparisons with the control were computed through the Bonferroni corrected threshold; all these determinations were performed with the software Prism 6.0 for Windows.
A two-way ANOVA was applied to the recorded progeny in each clutch for the different Cr(VI) concentrations in the three test volumes. The results of the total accumulated progeny were analyzed with a simple variance analysis. As pairwise post hoc comparisons, the Tukey test was applied. These analyses were performed with the Statistica ver. 10 software.
3 Results
3.1 Effect of temperature and test volume on the mean lethal concentration (LC50)
Figure 1 shows the LC50 48-h determined at 20°C and 25°C in the three test volumes. The average LC50 (± standard error, SE) for Cr(VI) in 5 mL was 0.43 (±0.0122) and 0.19 (±0.0039) mg L−1; in 15 mL was 0.49 (±0.0144) and 0.25 (±0.0156) mg L−1; and in 25 mL the calculated values were 0.48 (±0.0094) and 0.24 (±0.0131) mg L−1, respectively at 20°C and 25°C. LC50 was lower at 25°, indicating that the Cr(VI) was less toxic at 20°C. The two-way ANOVA revealed highly significant effects of both temperatures (p = 1 × 10−5) and the test volume (p = 1.6 × 10−4), whereas the interaction time (T) × volume (V) was not significant (p = 0.95). With both temperatures, the lowest LC50 values were recorded with the 5 mL test volume (p < 0.01). The Tukey test also revealed that, at both 20°C and 25°C, no significant differences occurred in the LC50 values recorded with 15 and 25 mL. Results evidence that at 25°C (temperature suggested by the USEPA, 2002, for the evaluation of subchronic toxicity in 7-day tests, three reproductions), with the 5-mL test volume, the Cr(VI) toxicity increased significantly (p = 1 × 10−5).
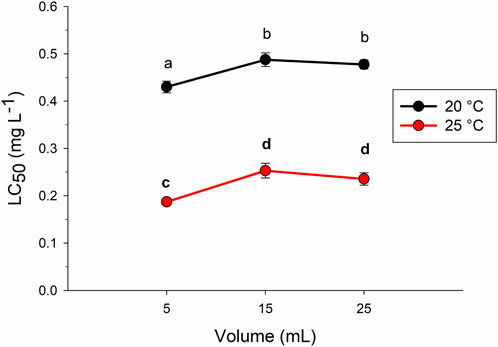
FIGURE 1. Average value and standard error bars of the mean lethal concentration (LC50-48 h) for Ceriodaphnia dubia exposed to Cr(VI) in different test volumes. Different letters indicate significant differences (Tukey, p < 0.05).
3.2 Survival of C. dubia under chronic exposure to Cr(VI) at 25°C in different test volumes
Based on the average LC50 values determined in 15 mL (0.253 ± 0.030 mg L−1, p = 0.05) and 25 mL (0.236 ± 0.026 mg L−1, p = 0.05), the following Cr(VI) concentrations were used for the chronic exposures: 0.01, 0.02, 0.04, and 0.08 mg L−1, these values correspond approximately to the 1/3, 1/6, 1/12, and 1/24 fractions of the LC50 determined at 25°C.
Survival of organisms in the chronic exposure to sublethal Cr(VI) concentrations is depicted in Figure 2. With the 5- and 25-mL volume, a 100% survival was recorded in the controls at the end of the assay (21 days), whereas one organism of the control died on day 18 with the 15 mL volume; survival is within the limits established in the acceptance criteria of the test (mortality in the negative control ≤20% for 7-day assays). Variable mortality was recorded in some treatments starting on day 7; at the end of the 21 days, a general direct positive relationship was observed between the Cr(VI) concentration and mortality, but survival was only significantly lower compared to the control at the concentrations of 0.04 and 0.08 mg L−1, in the volume of 5 and 15 mL, and at the three highest Cr(VI) concentrations in the 25-mL volume (Figure 2). According to this result, mortality, as an effect related to the chronic Cr(VI) exposure, was best observed in the highest test volume.
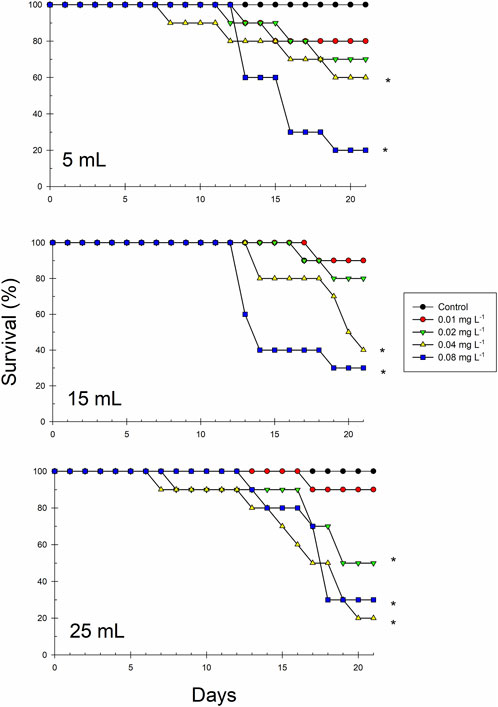
FIGURE 2. Survival curves of Ceriodaphnia dubia exposed to four sublethal Cr(VI) concentrations (0.01, 0.02, 0.04, and 0.08 mg L−1) in three different test volumes (5, 15, and 25 mL) during 21 days. Asterisks indicate significant differences with respect to controls (p = 0.05) (n = 10 replicates).
3.3 Accumulated and per brood progeny of C. dubia chronically exposed to Cr(VI) at 25°C in different test volumes
The average progeny values per clutch in the different volumes and Cr(VI) concentrations are shown in Table 1. When ANOVA-I applied to each clutch, and each test volume was significant, the No Observed Effect Concentrations (NOEC) and Lowest Observed Effect Concentrations (LOEC) shown in Table 1 were established using the Dunnett test, comparing with the control. In the 5-mL volume, significant differences were recorded starting with brood 2, although not consistent with the Cr(VI) concentration. Starting with brood 3, the differences are highly significant and allow identifying the LOEC value of 0.01 mg L−1 for all broods, except for brood 11 and for the accumulated progeny on day 21, which had values of 0.02 mg L−1.
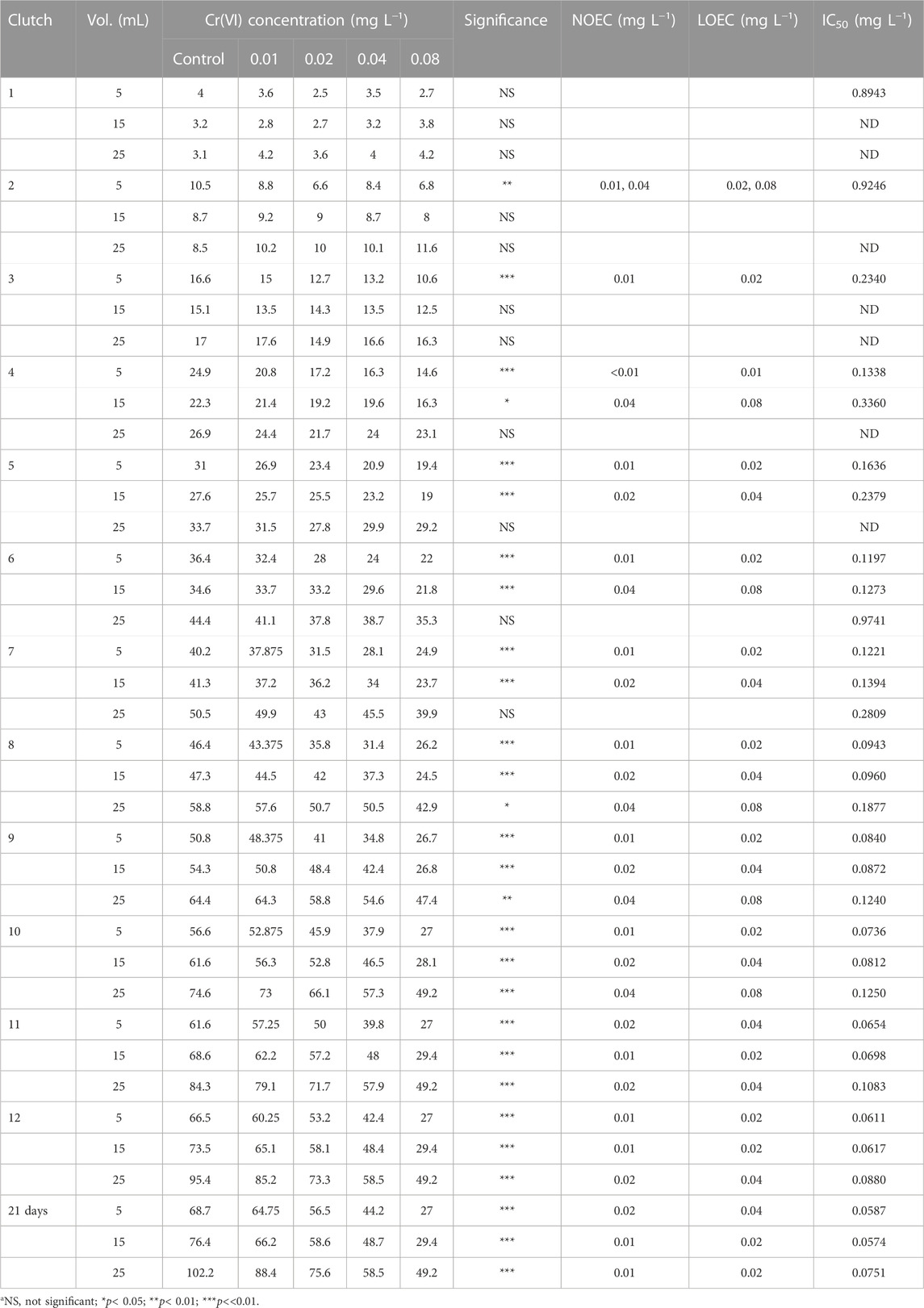
TABLE 1. Average values for the accumulated progeny (n = 10 replicates) recorded in the first 12 broods and at 21 days for Ceriodaphnia dubia exposed to Cr(VI) in different test volumes. Significance and ANOVA-I results are shown, along with the NOEC and LOEC values after Dunnett’s test.
For the 15-mL volume, significant differences were recorded starting with brood 4, with variable LOEC values that included the 0.04 and, predominantly, the 0.02 concentrations, with 0.01 mg L−1 recordings starting with brood 11.
For the 25-mL volume, the significant differences among the Cr(VI) concentrations started to appear with brood 8, with LOEC values of 0.04 mg L−1 for the progenies recorded in broods 8 to 10, with 0.02 mg L−1 for clutches 11 and 12, and for the accumulated on day 21, the LOEC was 0.01 mg L−1 (Table 1).
Figure 3 depicts the progeny accumulated per brood with successive reproductions. As a general tendency, fecundity was reduced in direct relationship to the Cr(VI) concentration, but this is only observed clearly starting with the third brood in the 5- and 15-mL volumes, whereas in the 25-mL volume, this is only observed starting with brood 6. Reproduction tended to be affected proportionally to the Cr(VI) concentration as time elapsed, and effects were clearly marked at the end of exposure (21 days). The highest similarity in accumulated progeny results was observed between the 15-mL and the 5-mL test volumes, whereas the values of accumulated progeny were significantly higher in the 25-mL volume. Two-way ANOVA applied to each brood revealed that, in the first and second reproductions, significant differences occurred due to the test volume, but a consistent concentration-response pattern was not recorded. From the third brood until day 21, two-way ANOVA indicated significant effects in the accumulated progeny due to the Cr(VI) concentration and the test volume. In the third brood in the 25-mL volume, no significant differences were observed between the control and the Cr(VI) concentrations (p > 0.05). In contrast, in the 5- and 15 mL volumes, a smaller accumulated progeny was beginning to be observed at the higher Cr(VI) concentrations.
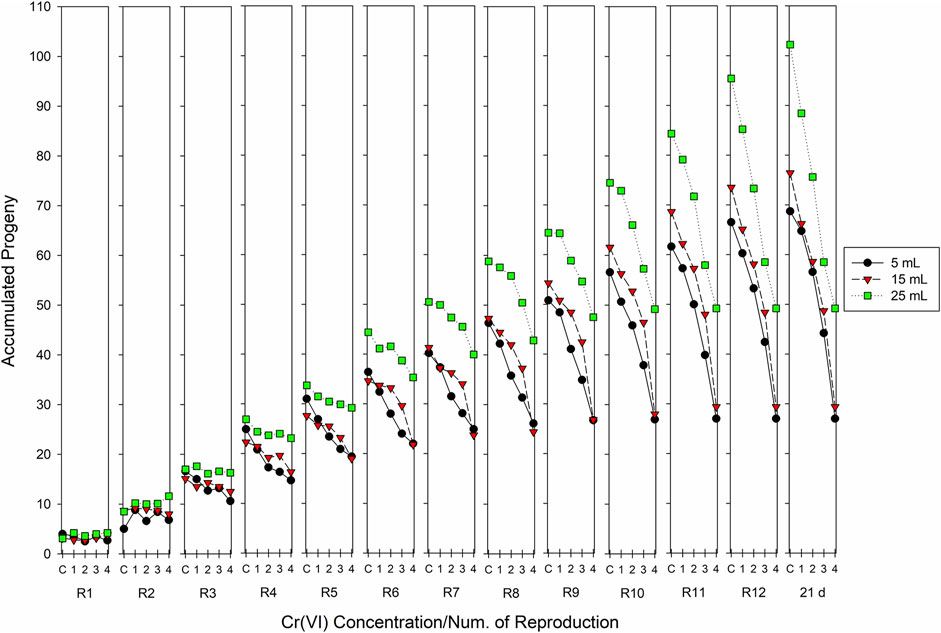
FIGURE 3. Accumulated progeny of Ceriodaphnia dubia recorded in successive broods from 1 to 12 and at the end of exposure (21 days), in organisms exposed chronically to different concentrations of hexavalent chromium. C=Control, 1 = 0.01, 2 = 0.02, 3 = 0.04, and 4 = 0.08 mg Cr(VI) L−1 (n = 10 replicates).
As observed in Figure 3, the accumulated progeny until brood three does not allow for the detection of the proportional adverse effects of Cr(VI) on C. dubia reproduction, particularly in the higher test volume, despite recording significant effects (p < 0.01) for both the test volume and the Cr(VI) concentration. The significant effects produced by the different Cr(VI) concentrations are best seen with increasing exposure times; at shorter times, perception of effects was clear only at the lower test volumes (5- and 15− mL).
At 21 days of exposure, the results of total progeny recorded in the controls with the 5- and 15-mL volumes were not statistically different (p < 0.05); the same was observed for each Cr(VI) concentration in both volumes (p < 0.05). All the fecundity values observed in the control and at each Cr(VI) concentration in the 25-mL volume were significantly higher than those recorded with 5 and 15 mL; they also showed a clear response-concentration tendency starting with brood 6, with less values of accumulated progeny as the toxicant’s concentration increases.
The average number of neonates in each reproduction and in all treatments is depicted in Table 2. In the first two broods, the highest average progeny was recorded in the 5-mL control volume, but starting with the third reproduction, almost in all cases, the highest progeny was registered in the 25-mL controls. The brood size was generally diminished with the Cr(VI) concentrations in all tested volumes. The smallest numbers of neonates in controls were 3.8, 3.2, and 3.1, whereas the maximal were 8.3, 7.8, and 13.9, respectively, for 5, 15, and 25 mL. At the 0.01 mg L−1 Cr(VI) concentration, the minimal values were 3.4, 2.8, and 4.2, and the maximal were 6.8, 8.0, and 10.9, respectively, for 5, 15, and 25 mL. At the maximal Cr(VI) concentration (0.8 mg L−1), the minimal average progeny values were 2.5, 2, and 4.2 and the maximal were 5.8, 5.8, and 9, respectively for 5, 15, and 25 mL (Table 2).
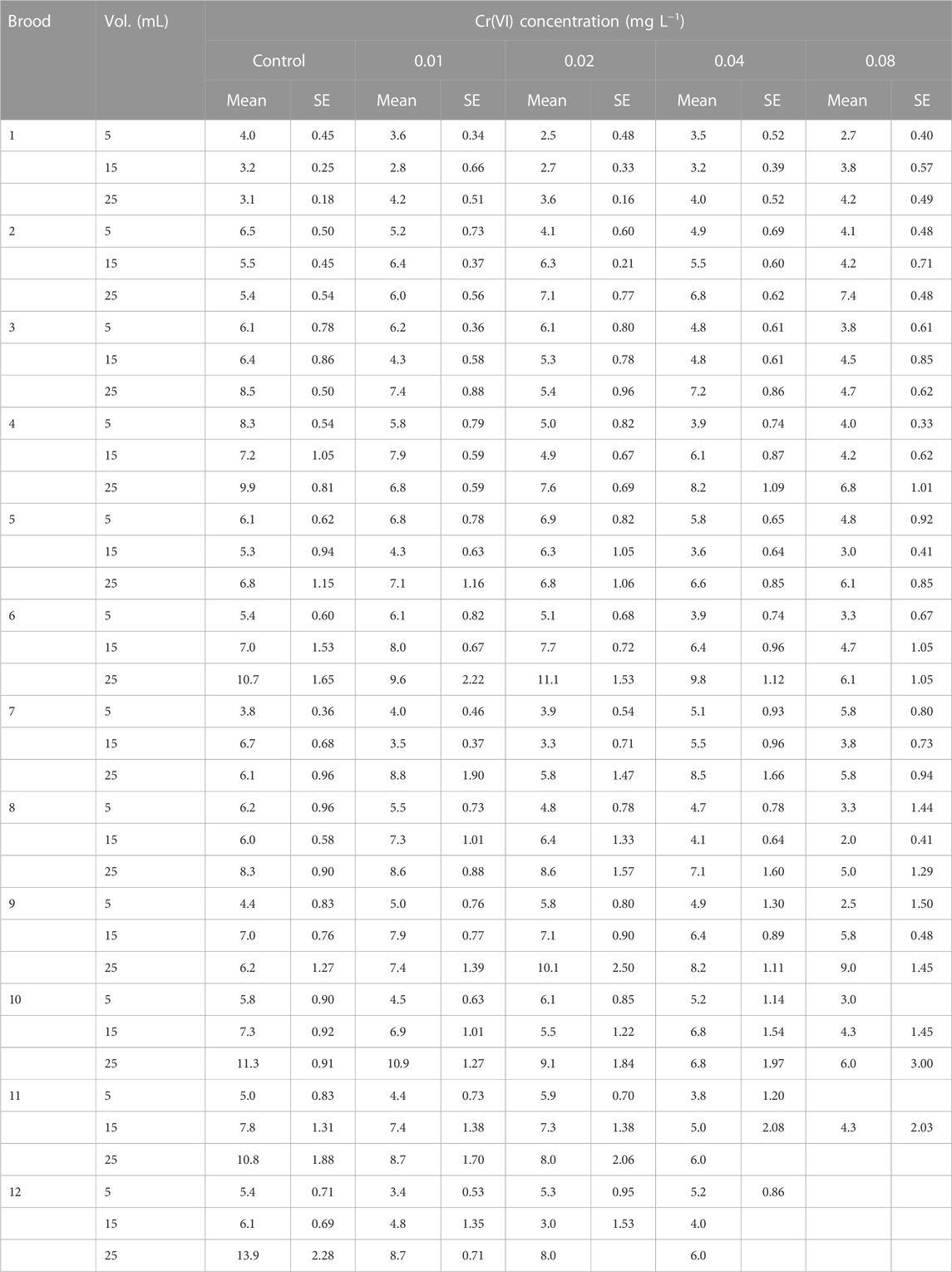
TABLE 2. Average progeny (number of neonates) and standard error values (n = 10 replicates) for consecutive broods of Ceriodaphnia dubia exposed to different Cr(VI) concentrations in three test volumes.
3.4 Median inhibitory concentration (IC50) of Cr(VI) in the fecundity of C. dubia chronically exposed at 25°C in different test volumes
Considering the reduction in the accumulated progeny in consecutive broods, we determined the inhibition in fecundity produced in C. dubia by exposure to different Cr(VI) concentrations. To assess the inhibition of reproduction, the fecundity values recorded in each control brood and test volume were taken as basis. The IC50 values shown in Table 1 were obtained using these values and the Probit method. Due to the inhibition tendencies related to the Cr(VI) concentrations and because inhibition values higher than 50% were not recorded in some treatments, the determined IC50 values were higher than the maximal assayed Cr(VI) value in many cases.
For the inhibition values at 5 mL, in the first three reproductions, the determined values were very high; hence, estimation of the IC50 values is considered acceptable only from the fourth reproduction onward. From clutch 7 to 12, the IC50 diminished slowly, and the lowest value was recorded for the accumulated progeny on day 21 (0.05871 mg L−1 Cr(VI)).
The IC50 for the 15-mL volume could not be determined in the first three reproductions. Starting with the fourth brood, in general, the IC50 diminished from a maximal value of 0.3360 to 0.0617 mg L−1 in clutch 12, although the smallest value was recorded for the accumulated progeny on day 21 (0.0574 mg L−1).
In the 25-mL volume, IC50 values could not be recorded for broods 1 to 5, but a very high value (0.9741 mg L−1) was recorded in brood 6. From brood 7 (0.2809 mg L−1) on, the IC50 value diminished until 0.0880 mg L−1 in brood 12; the lowest value was determined in the accumulated progeny on day 21 (0.0751 mg L−1).
In general, in broods 6 to 12 and the progeny accumulated on day 21, the IC50 values were similar in the 15− and 25-mL volumes, although consistently lower those determined in the 15-mL volume, and the latter was similar to those calculated for the 5 mL, as shown in Table 1.
3.5 Age of first reproduction in each C. dubia brood chronically exposed to Cr(VI) at 25°C in different test volumes
The volume and Cr(VI) exposure also affected the age in each brood. Figure 4 depicts graphically the average age recorded in C. dubia at each reproduction. The age of first reproduction was affected by the test volume (p = 0.0003) but not by the Cr(VI) concentration; the females in the 5-mL volume produced their first brood at a lower age (5.7 days in the control), whereas in the volume of 25 mL age of first reproduction was 6 days. The average age of the first two broods did not show a clear tendency related to the Cr(VI) concentration, but starting with the third brood, as Cr(VI) concentration increased, the age of the mothers was delayed in each clutch, although this was not consistent in some broods. The two-way ANOVA revealed that, in all broods, the volume significantly affected the age of the females in each brood, except in the 10th. The Cr(VI) concentration also significantly affected the age of reproducers in each brood except in broods 1, 5, and 6. Results obtained in broods 11 and 13 could not be analyzed with the two-way ANOVA because the mortality recorded in some treatments produced incomplete cells for this type of analysis. Table 3 shows the average age and the standard error values for each clutch in the different test volumes and all Cr(VI) concentrations.
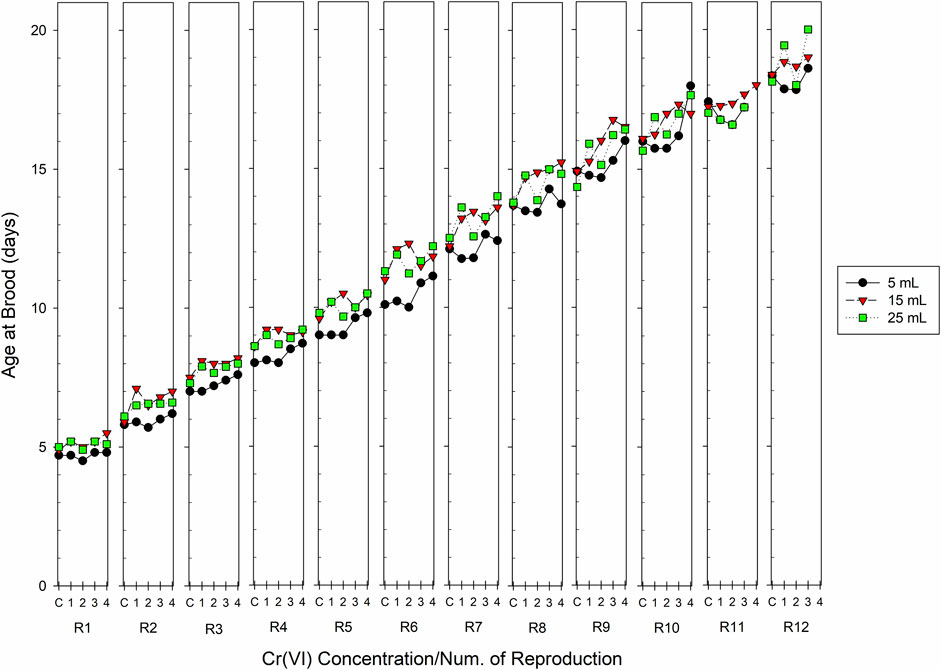
FIGURE 4. Age at the brood of Ceriodaphnia dubia recorded in successive clutches, from 1 to 12, and at the end of exposure time (21 days), in organisms exposed chronically to different Cr(VI) concentrations in different test volumes. C=Control, 1 = 0.01, 2 = 0.02, 3 = 0.04, and 4 = 0.08 mg Cr(VI) L−1 (n = 10 replicates).
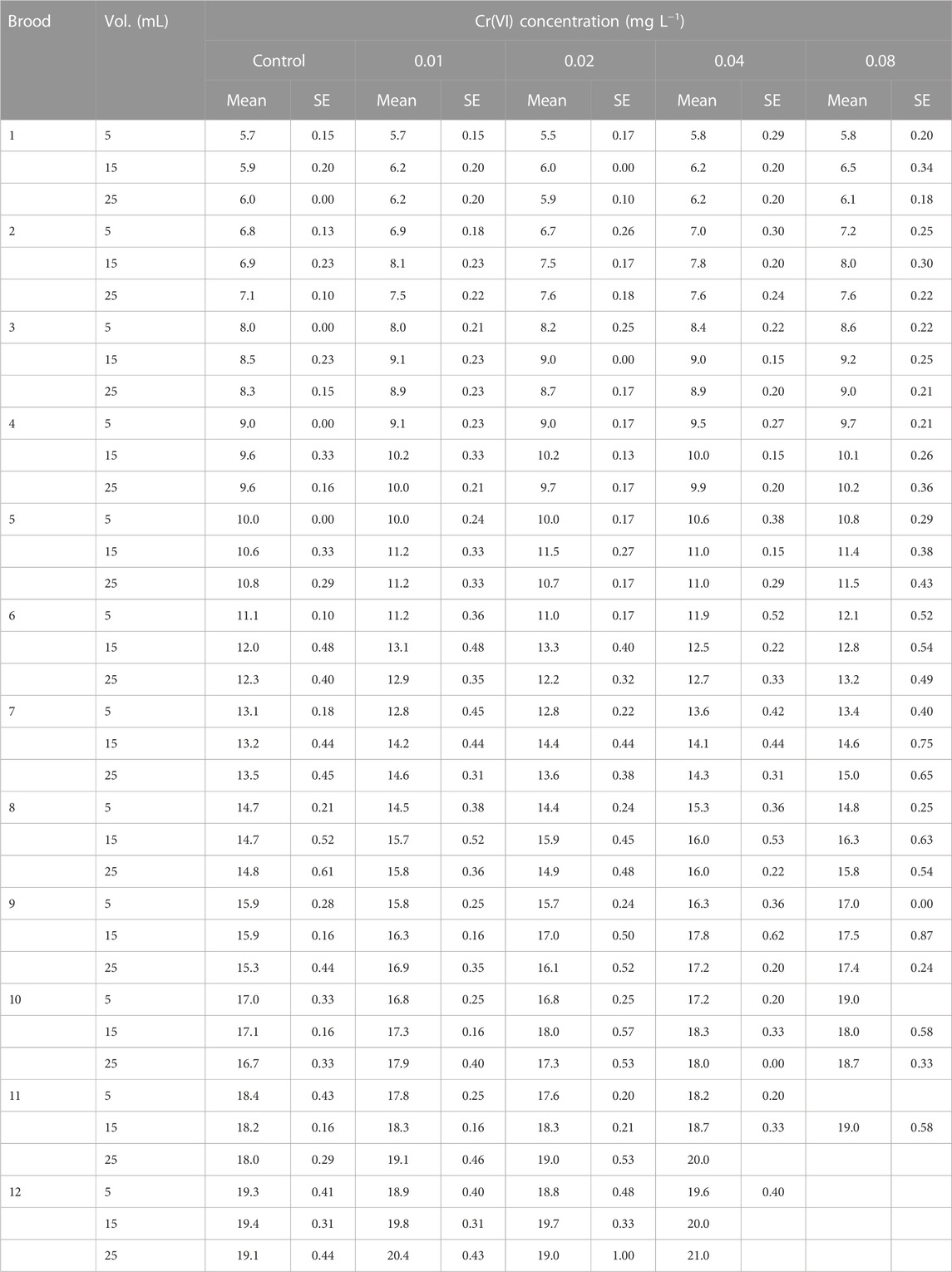
TABLE 3. Average age (days) and standard error values (n = 10 replicates) for consecutive broods of Ceriodaphnia dubia exposed to different Cr(VI) concentrations in three test volumes.
4 Discussion
The acute toxic response of C. dubia was affected by temperature, with a lower LC50 at 25°C. The toxic effect of Cr(VI) increased in the lowest test volume (5 mL), but no differences were observed in the LC50 value between 15− and 25 mL at both temperatures. It has been documented that a higher temperature modifies the physiology of some ectothermic organisms and increases the sensitivity to the toxic effect of metals (Sokolova and Lanning, 2008); notwithstanding, 25°C cannot be considered a temperature outside the tolerance limit of C. dubia, as it is suggested for the determination of subchronic effects in several short-lasting standardized testing methods (USEPA, 2002), which take advantage of the fact that a higher temperature accelerates sexual maturation and the reproductive process, allowing to shorten the life cycle and having three reproductions in 7 days, as established in the protocols of abbreviated exposure with this cladoceran. Hence, the LC50 values obtained herein confirm the higher sensitivity at 25°C and evidence that the toxic response increases comparatively at the lower test volume at both temperatures. This finding implies that in toxicity reports, it will be necessary to indicate the volumes and temperature at which the tests were performed to establish comparisons. This value can change significantly due to both factors, even in the same strain of the test organism.
Regarding the toxicity of Cr(VI) in C. dubia, Baral et al. (2006) reported a value of LC50-48 h = 145 μg L−1 (135–154 μg L−1), determined in a 20-mL test volume; this value is lower than the one recorded herein of 190 and 250 μg L−1 at 25°C in the 5- and 25- mL volumes, respectively. In turn, Nieto (2014) determined an LC50-48 h of 230 μg L−1 (180–280 μg L−1), which is similar to the one recorded in the 25-mL volume in this work. However, Mount and Norber (1984) reported relatively low values, with an LC50-48 h of 45 μg L−1, and Hickey (1989) determined an LC50-24 h of 53 μg L−1 (39–79 μg L−1), which are lower values than those reported herein with all three volumes tested, although the latter was recorded at 24 h of exposure. It can be considered that the differences among the published values can be due to multiple factors, including the assay method comprising differences in type, amount, and origin of the supplied diet. It could even be attributed to the strain of cladoceran used. Another factor could be the expertise and ability of the operators, as well as the reproducers’ age, condition, and sensitivity (Serben, 2015). Differences in sensitivity were observed since the origins of the proposal of the abbreviated method with C. dubia by USEPA in 1985 in inter-calibration exercises, attributing the primary variability sources to the food, the culture water, and the exposure of organisms (DeGraeve and Cooney, 1987).
In D. magna, the LC50 determined at 48 h was 130 μg L−1 with Na2Cr2O7•2H2O (Okamoto et al., 2015). For Cr(VI) with D. magna, the LC50-48 h determined at 20°C was 0.2076 ± 0.0164 mg L−1 and 0.1544 ± 0.0175 mg L−1 at 25°C (Martínez-Jerónimo et al., 2006), although higher values have been reported, like those by Reyes and Díaz (1999) (0.35 mg L−1 at 20°C). These reports confirm a higher sensitivity to this metal at a higher exposure temperature with values similar to those obtained herein with C. dubia.
In the tropical species Ceriodaphnia cornuta, the LC50 reported by Pérez-Legaspi et al. (2017) for Cr(VI) was 1.16 mg L−1, which indicates a lesser sensitivity to this metal than that of C. dubia, contrary to what these authors suggest that C. cornuta is a more sensitive cladoceran. In other tropical cladocerans, like Pseudosida ramosa, the LC50 was 29 µg L−1 (20–60 µg L−1), indicating a higher sensitivity.
According to our results, it is possible to determine acute toxicity using lesser test volumes than those suggested currently, both at 20 and 25°C, and results indicate even a higher sensitivity in the 5-mL volume; these determinations are comparable to those reported for C. dubia in other studies. It is fundamental to establish with greater precision the test protocols aimed at reducing as much as possible the effect that could be exerted on the acute toxic response by the operational factors and the handling and feeding of the reproducers. We consider that the procedures suggested herein could contribute to improve these guidelines, as we observed that the critical aspect of feeding the progenitors can be solved satisfactorily by using exclusively a diet of cultured microalgae, without resorting to complements that contribute to loss of control, like the use of Cerophyl®, food for trout, and bakery`s yeast.
Evaluation of the chronic toxicity of Cr(VI) revealed affectations in the reproduction and life cycle of C. dubia by the test volume and the exposure time. The NOEC and LOEC are the results of the Dunnett test in cases of significant ANOVAs in determining the Cr(VI) effect on the reproductive response. These concentrations are part of the traditional information handling in this type of toxicity assay. Notwithstanding, the NOEC and LOEC values could not be determined for all test volumes or the evaluated broods. Only with the lowest volume (5 mL) could NOEC and LOEC values be identified in all broods and, in most of them, the NOEC was 0.01, and LOEC was 0.02 mg L−1, in general, being the lowest values recorded for the three test volumes (Table 1).
The NOEC values for all broods and all volumes fluctuated in most cases from 0.01 to 0.02 and LOEC from 0.02 to 0.04 mg L−1. According to the European Chemical Agency (2023), the NOEC for effects of Cr(VI) on the reproduction in C. dubia is 0.0047 mg L−1, which is lower than the value reported herein, although it is not indicated in which conditions it was determined. On the other hand, Gutiérrez et al. (2012) reported, that for the escape behavior in C. dubia, NOEC and LOEC for Cr(VI) of <7.5 and 7.5 mg L−1, respectively, both values are higher than those reported herein for effects on reproduction; the difference could be explained as a function of the evaluated endpoint in each case. The evaluation of the reproductive effects is possibly a more sensitive indicator than the escape behavior of this cladoceran. According to Maycock et al. (2007), a NOEC value of 0.0047 mg L−1 for the reproductive response of C. dubia allows establishing a Predicted No-Effect Concentration (PNEC) for Cr(VI) of a tenth fraction of this value (0.47 μg L−1), that it is a value that could also be inferred from the results here presented.
It is worth mentioning that a greater consistency was obtained in the concentration-response relationship at the lowest assessed test volume, even since the first broods. In contrast, with 25 mL, only until the last reproductions could a proportional concentration-response relationship be identified to determine the IC50. Based on this result, the conditions prevailing in 25 mL, related to a larger space and food availability, could mask the toxicant’s effect, although this does not necessarily indicate that a reduced volume contributes to a greater stress that would have made the toxic effect more detectable, fostered mainly by lesser food availability. The latter could be discarded because the number of supplied microalgae (1 × 106 cells mL−1) was above the food requirement of C. dubia, and the possibility of its significant reduction is also discarded due to the daily renovation of the food and the culture medium. On the contrary, a larger volume with a higher absolute number of microalgae could affect the availability of the toxicant due to bioremoval and retention in the cell wall of microalgae, as reported by Daneshvar et al. (2019). It has also been documented that the conditions for Cr(VI) removal by microalgae are special (Han et al., 2007); hence, it is an effect that would have to be demonstrated experimentally and would suggest that it is possible to reduce the test volume to the minimum assayed herein and that increasing the assay time one or two additional days to those indicated by USEPA (2002) could help to attain more certainty in the toxicological evaluation.
Regarding the chronic effects of Cr(VI), the IC50 determined by Okamoto et al. (2020) for C. dubia is 75 μg L−1, which is similar to that specified in our study in some test volumes and some broods (Table 1). In turn, Baral et al. (2006) determined an IC50 of 37 μg L−1 after 7 days of exposure. Regalado et al. (2014) evaluated the effect of Cr(VI) in C. dubia in 30-mL volumes for 15 days, feeding Chlorella vulgaris, although they do not report IC50 values. They argued significant effects on fecundity by the exposure to 25 μg L−1 of Cr(VI); however, their results show great variability and a very low fecundity (average of 1.5 neonates female−1 at 15 days). It is difficult to compare the results obtained in our study because much of the available information on the chronic effects of C. dubia is based on the abbreviated method of 7 days, with test volumes of 15 mL. Notwithstanding, the obtained information allows determining that there are effects on the reproductive response related with both the test volume and the exposure time.
It must also be emphasized that a relevant cause for the variability in results among different studies is the operational aspects, mainly the diet provided to the progenitors and the test organisms during the chronic or subchonic toxicity assessments. In this sense, Cerda and Olive (1993) demonstrated that the diet provided in the 7-day test with C. dubia influences the toxicity results, determining that the organisms fed Selenastrum capricornutum (Pseudokirchneriella subcapitata) showed more sensitivity to copper than those fed other microalgae, like Chlamydomonas, or with a mixture of yeast + Cerophyl®+trout food (YCT), or YCT plus Selenastrum. Although Cerda and Olive (1993) conclude that the higher sensitivity was due to a nutritional deficiency in the diet, it could also be interpreted that a more natural diet will better evaluate the effects of chemical stressors.
In turn, Nieto (2014) determined as accumulated progeny, until clutch 12, an amount of 45 and 41 neonates from mothers fed S. capricornutum and YCT, respectively, whereas in progenitors fed S. capricornutum + YCT, the amount was 134 neonates, all in a 15-mL volume. In our case, the average progeny recorded in brood 12 was 66.5, 73.5, and 95.4 neonates in the 5-, 15-, and 25-mL volumes, respectively, in females fed exclusively P. subcapitata; although these values are lower than those recorded by Nieto (2014) with a mixed diet, we consider that providing only microalgae allows for a better control of the reproducers’ diet.
It should be noted that the test method mostly used currently to evaluate the chronic effects of toxicants with C. dubia is performed in 7 days. The acceptance criterion of the test requires a higher than 80% survival of control organisms and that at least 60% of the surviving control females release at least three broods, with 15 neonates in the average of accumulated progeny (US EPA, 2002). Based on our results and other studies, mortality could be avoided in controls (or be reduced to 10% as maximal) and could allow revising whether it is possible to obtain confident information on the chronic response in three clutches.
The information obtained in this study allows counting upon a procedure to be used to evaluate the chronic effects of toxic pollutants, with the possibility of different test volume options and execution times. Besides, our study contributes to a better knowledge of this species’s biology under experimental conditions. C. dubia modified its reproductive responses to the Cr(VI) exposure influenced by factors like the test volume and the exposure time. According to the obtained results, it is necessary to re-assess the proposal of both short-duration methods and the suggestion of using smaller test volumes to be able to discard possible omissions or incorrect evaluations of the toxic effects of chemical stressors.
Data availability statement
The raw data supporting the conclusion of this article will be made available by the authors, without undue reservation.
Ethics statement
The manuscript presents research on animals that do not require ethical approval for their study.
Author contributions
FM-J: Conceptualization, Data curation, Formal Analysis, Funding acquisition, Investigation, Methodology, Resources, Writing–original draft, Writing–review and editing. LM-J: Data curation, Investigation, Methodology, Writing–original draft.
Funding
The author(s) declare that no financial support was received for the research, authorship, and/or publication of this article.
Acknowledgments
Authors acknowledge the support for this study to the Instituto Politécnico Nacional (IPN). Also, thanks to COFAA-IPN and EDI-IPN for the fellowships granted to FM-J.
Conflict of interest
The authors declare that the research was conducted in the absence of any commercial or financial relationships that could be construed as a potential conflict of interest.
The author(s) declared that they were an editorial board member of Frontiers, at the time of submission. This had no impact on the peer review process and the final decision.
Publisher’s note
All claims expressed in this article are solely those of the authors and do not necessarily represent those of their affiliated organizations, or those of the publisher, the editors and the reviewers. Any product that may be evaluated in this article, or claim that may be made by its manufacturer, is not guaranteed or endorsed by the publisher.
References
Arnold, E. D. (1971). Ingestion, assimilation, survival, and reproduction by Daphnia pulex fed with seven species of blue-green algae. Limnol. Oceanogr. 16, 906–920. doi:10.4319/lo.1971.16.6.0906
Baral, A., Engelken, R., Stephens, W., Farris, J., and Hannigan, R. (2006). Evaluation of aquatic toxicities of chromium and chromium-containing effluents in reference to chromium electroplating industries. Arch. Environ. Contam. Toxicol. 50, 496–502. doi:10.1007/s00244-005-0068-x
Baudo, R. (1987). “Ecotoxicological testing with Daphnia,” in Daphnia. Memorie dell’Istituto italiano di Idrobiologia. Editors R. H. Peters, and R. de Bernardi, 45, 461–482.
Baumann, J., Sakka, Y., Bertrand, C., Köser, J., and Filser, J. (2014). Adaptation of the Daphnia sp. acute toxicity test: miniaturization and prolongation for the testing of nanomaterials. Environ. Sci. Pollut. R. 21, 2201–2213. doi:10.1007/s11356-013-2094-y
Cerda, H., and Olive, J. (1993). Effects of diet on seven-day Ceriodaphnia dubia toxicity tests. Ohio J. Sci. 93 (3), 44–47.
Ceresoli, N., and Gagneten, A. M. (2003). Efectos del efluente de curtiembre sobre Ceriodaphnia dubia (Crustacea, Cladocera) en condiciones experimentales. Interciencia 28 (8), 469–475.
Connors, K. A., Brill, J. L., Norberg-King, T., Barron, M. G., Carr, G., and Belanger, S. E. (2022). Daphnia magna and Ceriodaphnia dubia have similar sensitivity in standard acute and chronic toxicity tests. Environ. Toxicol. Chem. 41 (1), 134–147. doi:10.1002/etc.5249
Daneshvar, E., Zarrinmehr, M. J., Kousha, M., Hashtjin, A. M., Saratale, G. D., Maiti, A., et al. (2019). Hexavalent chromium removal from water by microalgal-based materials: adsorption, desorption and recovery studies. Bioresour. Technol. 293, 122064. doi:10.1016/j.biortech.2019.122064
Degraeve, G. M., and Cooney, J. D. (1987). Ceriodaphnia: an update on effluent toxicity testing and research needs. Environ. Toxicol. Chem. 6, 331–333. doi:10.1897/1552-8618(1987)6[331:cauoet]2.0.co;2
Degraeve, G. M., Cooney, J. D., Marsh, B. H., Pollock, T. L., and Reichenba, N. G. (1992). Variability in the performance of the 7-d Ceriodaphnia dubia survival and reproduction test. An intra- and interlaboratory study. Environ. Toxicol. Chem. 11, 851–866. doi:10.1897/1552-8618(1992)11[851:vitpot]2.0.co;2
Dehui, Z. (1989). Growth, reproduction and population growth of Ceriodaphnia cornuta Sars and comparison of 7-day fecundity with Ceriodaphnia dubia Richard. Chin. J. Oceanol. Limnol. 7, 105–111. doi:10.1007/bf02842746
Dorn, P. B., Rodgers, J. H., Jop, K. M., Raia, J. C., and Dickson, K. L. (1987). Hexavalent chromium as a reference toxicant in effluent toxicity tests. Environ. Toxicol. Chem. 66, 435–444. doi:10.1002/etc.5620060604
European Chemical Agency (Echa), (2023). Chromium Trioxide. Registered dossier. EC number: 215-607-8. CAS number: 1333-82-0. https://echa.europa.eu/registration-dossier/-/registered-dossier/15927/6/2/1#.
Freitas, E. C., and Rocha, O. (2011). Acute and chronic toxicity of chromium and cadmium to the tropical cladoceran Pseudosida ramosa and the implications for ecotoxicological studies. Environ. Toxicol. 29, 176–186. doi:10.1002/tox.20784
Grintzalisa, K., Daia, W., Panagiotidisa, K., Belavgenia, A., and Vianta, M. (2017). Miniaturising acute toxicity and feeding rate measurements in Daphnia magna. Ecotoxicol. Environ. Saf. 139, 352–357. doi:10.1016/j.ecoenv.2017.02.002
Gutierrez, M. F., Paggi, J. C., and Gagneten, A. M. (2012). Microcrustaceans escape behavior as an early bioindicator of copper, chromium and endosulfan toxicity. Ecotoxicology 21, 428–438. doi:10.1007/s10646-011-0803-1
Han, X., Wong, Y. S., Wong, M. H., and Tam, N. F. Y. (2007). Biosorption and bioreduction of Cr(VI) by a microalgal isolate, Chlorella miniata. J. Hazard. Mater. 146, 65–72. doi:10.1016/j.jhazmat.2006.11.053
Hickey, C. W. (1989). Sensitivity of four New Zealand cladoceran species and Daphnia magna to aquatic toxicants, New Zealand. J. Mar. Fresh. Res. 23, 131–137. doi:10.1080/00288330.1989.9516348
ISO (2008). Water Quality – determination of chronic toxicity to Ceriodaphnia dubia. https://www.iso.org/standard/39673.html.
Martínez-Jerónimo, F., Espinosa-Chávez, F., and Villaseñor-Córdova, R. (2000). Effect of culture volume and adult density on the neonate production of Daphnia magna, as test organisms for aquatic toxicity tests. Environ. Toxicol. 15 (3), 155–159. doi:10.1002/1522-7278(2000)15:3<155::aid-tox1>3.0.co;2-x
Martínez-Jerónimo, F., Martínez-Jerónimo, L., and Espinosa-Chávez, F. (2006). Effect of culture conditions and mother’s age on the sensitivity of Daphnia magna Straus 1820 (Cladocera) neonates to hexavalent chromium. Ecotoxicology 15, 259–266. doi:10.1007/s10646-006-0057-5
Martínez-Jerónimo, F., Villaseñor, R., Ríos, G., and Espinosa, F. (1994). Effect of food type and concentration on the survival, longevity, and reproduction of Daphnia magna. Hydrobiologia 287, 207–214. doi:10.1007/bf00010735
Maycock, D., Sorokin, N., Atkinson, C., Rule, K., and Crane, M. (2007). Proposed EQS for water framework directive annex VIII substances: chromium(VI) and chromium(III). https://assets.publishing.service.gov.uk/media/5a7c31b6e5274a1f5cc766a5/scho0407blvq-e-e.pdf.
Mount, D. I., and Norberg, T. J. (1984). A seven-day life-cycle cladoceran toxicity test. Environ. Toxicol. Chem. 3, 425–434. doi:10.1002/etc.5620030307
Murugan, N. (1975). Egg production, development and growth in Moina micrura kurz (1874) (Cladocera: moinidae). Freshw. Biol. 5, 245–250. doi:10.1111/j.1365-2427.1975.tb00137.x
Nieto, D. L. (2014). Revisión de los efectos subletales que causa el cromo hexavalente, sobre una especie indicadora de contaminación acuática, Ceriodaphnia dubia, M. Sc. Thesis. Colombia: Universidad Militar Nueva Granada. Retrieved from: http://hdl.handle.net/10654/10953.
OECD (2012). OECD Guideline for testing of chemicals. Daphnia magna reproduction test. Guideline 211. Paris: OECD Publishing.
Okamoto, A., Masunaga, S., and Tatarazako, N. (2015). Acute toxicity of 50 metals to Daphnia magna. J. Appl. Toxicol. 35, 824–830. doi:10.1002/jat.3078
Okamoto, A., Masunaga, S., and Tatarazako, N. (2021). Chronic toxicity of 50 metals to Ceriodaphnia dubia. J. Appl. Toxicol. 41, 375–386. doi:10.1002/jat.4049
Oris, J. T., Winner, R. W., and Moore, M. V. (1991). A four-day survival and reproduction toxicity test for Ceriodaphnia dubia. Environ. Toxicol. Chem. 10, 217–224. doi:10.1897/1552-8618(1991)10[217:afsart]2.0.co;2
Pace, L. M., and Vaqué, D. (1994). The importance in determining mortality rates of protozoans and rotifers in lakes. Limnol. Oceanogr. 35, 985–996. doi:10.4319/lo.1994.39.5.0985
Pennak, R. W. (1978). Freshwater invertebrates of the United States. Hoboken, NJ, USA: Wiley-Interscience Publication.
Pérez-Legaspi, I. A., Garatachia-Vargas, M., García-Villar, A. M., and Rubio-Franchini, I. (2017). Evaluación de la sensibilidad del cladócero tropical Ceriodaphnia cornuta a metales pesados. Rev. Int. Contam. Ambie. 33 (1), 49–56. doi:10.20937/RICA.2017.33.01.04
Powell, R. L., Moser, E. M., Kimerle, R. A., Mckenzie, D. E., and Mckee, M. (1996). Use of a miniaturized test system for determining acute toxicity of toxicity identification evaluation fractions. Ecotoxiol. Environ. Safe. 35, 1–6. doi:10.1006/eesa.1996.0075
Regalado, L., Reno, U., Gervasio, S., Troinani, H., and Gagneten, A. M. (2014). Effect of metals on Daphnia magna and cladocerans representatives of the Argentinean fluvial littoral. J. Environ. Biol. 35, 689–697.
Reyes, C. B., and Díaz, C. B. (1999). Efectos del cromo hexavalente y un carbamato sobre dos especies de cladóceros. Acta Biológica Colomb. 4 (1), 21–34.
Rodríguez-Estrada, J., Villaseñor-Córdova, R., and Martínez-Jerónimo, F. (2003). Efecto de la temperatura y tipo de alimento en el cultivo de Moina micrura (Kurz, 1874) (Anomopoda: moinidae) en condiciones de laboratorio. Hidrobiologica 13, 239–245.
Serben, K. (2015). Ceriodaphnia dubia chronic toxicity test variability and possibility of false positives. Learned Discourses: timely Scientific Opinions. Integr. Environ. Assess. Manag. 11 (4), 724–725. doi:10.1002/ieam.1685
Sims, I. R., Watson, S., and Holmes, D. (1993). Toward a standard Daphnia juvenile production test. Environ. Toxicol. Chem. 12, 2053–2058. doi:10.1897/1552-8618(1993)12[2053:tasdjp]2.0.co;2
Snell, T. W., and Moffat, B. D. (1992). A 2-d life cycle test with the rotifer Brachionus calyciflorus. Environ. Toxicol. Chem. 11, 1249–1257. doi:10.1897/1552-8618(1992)11[1249:adlctw]2.0.co;2
Sokolova, I. M., and Lannig, G. (2008). Interactive effects of metal pollution and temperature on metabolism in aquatic ectotherms: implications of global climate change. Clim. Res. 37, 181–201. doi:10.3354/cr00764
U. S. Environmental Protection Agency (2002b). Methods for measuring the acute toxicity of effluents and receiving waters to freshwater and marine organisms. Washington, D.C., United States: United States Environmental Protection Agency.
US EPA (2002). Short-term methods for estimating the chronic toxicity of effluents and receiving waters to freshwater organisms. Washington, D.C., United States: United States Environmental Protection Agency.
Keywords: Cr(VI), cladocera, Daphnia, chronic toxicity, aquatic toxicology, aquatic toxicity guidelines
Citation: Martínez-Jerónimo F and Martínez-Jerónimo L (2023) Do short-term, reduced-volume methods accurately reflect chronic toxic effects in the cladoceran Ceriodaphnia dubia? A study with the reference toxicant hexavalent chromium. Front. Environ. Sci. 11:1321257. doi: 10.3389/fenvs.2023.1321257
Received: 13 October 2023; Accepted: 04 December 2023;
Published: 14 December 2023.
Edited by:
Mallavarapu Megharaj, The University of Newcastle, AustraliaReviewed by:
Sara Long, RMIT University, AustraliaEdison Barbieri, Agência de Agronegócio e Tecnologia de São Paulo (APTA), Brazil
Copyright © 2023 Martínez-Jerónimo and Martínez-Jerónimo. This is an open-access article distributed under the terms of the Creative Commons Attribution License (CC BY). The use, distribution or reproduction in other forums is permitted, provided the original author(s) and the copyright owner(s) are credited and that the original publication in this journal is cited, in accordance with accepted academic practice. No use, distribution or reproduction is permitted which does not comply with these terms.
*Correspondence: Fernando Martínez-Jerónimo, Zmplcm9uaUBpcG4ubXg=, ZmVybWFyamVyQG91dGxvb2suY29t