- 1Australian Rivers Institute, Griffith University, Nathan, QLD, Australia
- 2Malacology, Department of Aquatic Zoology, Western Australian Museum, Welshpool, WA, Australia
- 3Centre for Ecosystem Science, School of Biological, Earth and Environmental Sciences, The University of New South Wales, Sydney, NSW, Australia
- 4Environmental Research Institute of the Supervising Scientist (ERISS), Department of Climate Change, Energy, the Environment and Water, Darwin, NT, Australia
- 5National Institute of Water and Atmospheric Research, Hamilton, New Zealand
- 6Arthur Rylah Institute for Environmental Research, Department of Energy, Environment, and Climate Action, Heidelberg, VIC, Australia
- 7Applied Chemistry and Environmental Science, RMIT University, Melbourne, VIC, Australia
Most freshwater mussels have larvae (glochidia in Unionidae, Margaritiferidae and Hyriidae) that are parasitic on fishes. This study describes and compares the diversity of glochidia among 17 species of Australasian Hyriidae. Here, scanning electron microscopy was used to illustrate shell morphology, while patterns of diversity in size, shape and morphological characteristics were analysed quantitatively and qualitatively with Principal Component, Linear Discriminant, and Multiple Correspondence Analyses to separate species with varying degrees of discrimination. Results showed shell lengths ranged from 50 to 390 μm. Shape varied, from sub-oval to sub-triangular, bilaterally symmetrical to scalene, and hook morphology varied from unicuspid, bicuspid, tricuspid or complex with varying length and structure. Unique observations of this study include the consistent variation in glochidial release mechanisms among Australian subfamilies and New Zealand genera. Hyridellini species and Echyridella aucklandica (Gray and Dieffenbach, 1843) are released either freely or as “mesoconglutinates” (presumed brood lures), whereas Velesunioninae and Echyridella menziesii (Gray and Dieffenbach, 1843) release glochidia in “amorphous mucous conglutinates”. Hyridellini predominantly occur within perennially flowing rivers of coastal south-eastern Australia with generally low turbidity, whereas the Velesunioninae occur more typically in slower flowing, intermittent waters, many prone to extended periods of high turbidity. Thus, where Hyridellini occur, mesoconglutinates as visual brood lures may be a more efficient mechanism for infesting host fishes than the passive infestation strategy typical of velesunionine species frequenting more turbid waters. Finally, this study presents systematic descriptions of glochidia and a provisional key for identification of Australasian hyriid glochidia, making an important contribution to the current understanding of taxonomy and life history traits, both critical for hyriid conservation.
1 Introduction
Extant freshwater mussels (Bivalvia) of the order Unionoida occupy waterbodies on every continent except Antarctica, with a global diversity approaching 1,000 species (Bogan and Roe, 2008; Graf and Cummings, 2021). During reproduction, males release sperm into the water column that are then taken in through the inhalant siphons of females; eggs are fertilised internally and brooded in specialised chambers of the ctenidia called marsupia. Widely accepted as a dispersal strategy, the larvae of almost all freshwater mussel species are parasitic on fishes and rarely, amphibians (Howard, 1915; Kat, 1984). Larvae occur in two distinct forms: lasidia in the Etheriidae, Iridinidae and Mycetopodidae and glochidia in the Unionidae, Margaritiferidae and Hyriidae (Bauer and Wächtler, 2001). This study focuses on the glochidia of Australasian Hyriidae.
The mechanism of glochidial release and host infestation strategy varies widely. Some species broadcast individual glochidia directly to the water column to infest host fishes specifically during spawning migrations (Araujo et al., 2000; Hastie and Young, 2003; Soler et al., 2018). Other species have evolved modifications of the simple glochidia broadcast release strategy, such as Unio crassus Nilsson, 1822, that moves into shallow water to spurt water streams while releasing glochidia, presumably to attract potential hosts (Vicentini, 2005). In the Lampsilini (Unionidae), strategies for attracting hosts include mimicry via the production of various types of conglutinates, mantle lures and host-trapping (Barnhart et al., 2008). Functional conglutinates are structures that contain glochidia that often resemble food or prey items that infest hosts with glochidia when ingested; these vary in form and complexity (Barnhart et al., 2008; Watters, 2008). In amorphous, mucous conglutinates (or “mucous strands” or “mucous strings”), glochidia are released in a loose mucous matrix that dissociates in water (Watters, 2008). This type of glochidial release mechanism usually facilitates passive infestation where hosts contact glochidia as they swim through suspended mucus and glochidia (e.g., Matteson, 1948; Barnhart et al., 2008). The snuffbox mussel, Epioblasma triquetra (Rafinesque, 1820), has taken this further to actively trap the host between its modified shell valves whilst releasing glochidia for infestation (Barnhart and Roston, 2005; Barnhart et al., 2008).
Among species, glochidia vary widely in size, shape, and morphological features. Shell sizes range in length from 47.5 µm in Margaritifera margaritifera (Linnaeus, 1758) (Soler et al., 2018) to 500 µm in Strophitus undulatus (Say, 1817) (Hoggarth, 1999), while in shape outline they can vary from subtriangular (equilateral, isosceles, or scalene), suborbicular, hatchet-shaped (Ortmann, 1921; Kat, 1984; Jones et al., 1986; Hoggarth, 1999; Bauer and Wächtler, 2001; Sayenko, 2006, 2016; Pimpão et al., 2012) to an amorphous shape and bilateral asymmetry of opposing valves (Pfeiffer and Graf, 2015 and references therein). The glochidial stage of development in freshwater mussels is monomyarian, possessing a single adductor muscle that redevelops into a dimyarian state during metamorphosis to the juvenile stage (Bauer and Wächtler, 2001). Apart from Lillie (1895), there have been few studies of early development in freshwater mussels, but specific morphological features have been investigated, including sensory hairs, the larval mantle, ciliary fields and the larval filament, the latter of which is either present or absent, depending on species (Bauer and Wächtler, 2001; Nivischenko et al., 2022). The ventral apex of the glochidial valves in many species are equipped with hooks (as defined by Carter et al., 2012) of varying forms, also referred to as ‘larval teeth’, that assist in attachment to their host (Bauer and Wächtler, 2001). Morphological and morphometrical studies of glochidia and marsupia, as well as the mechanism and timing of glochidial release, have proven valuable in systematics and taxonomy (Ortmann, 1921; Parodiz and Bonetto, 1963; Heard and Guckert, 1970; Pimpão et al., 2012; O’Brien et al., 2019; Chernyshev et al., 2020), mode of parasitism (Barnhart et al., 2008; Huber and Geist, 2019), identification of host fishes (Shephard et al., 2021), conservation (Zając and Zając, 2021; Aldridge et al., 2022) and captive breeding programs (Benedict and Geist, 2021).
Among the glochidia-bearing families of freshwater mussels, extant Hyriidae are restricted to the Southern Hemisphere in Australasia (including Australia, New Zealand, Papua New Guinea, Solomon Islands, and Indonesian West Papua) and South America (Graf and Cummings, 2007; Bogan, 2008; Miyahira et al., 2017). The Australasian Hyriidae are split between two subfamilies: the Velesunioninae (genera: Alathyria, Lortiella, Microdontia, Velesunio and Westralunio), exclusive to Australia and New Guinea, and the Hyriinae (genera: Cucumerunio, Hyridella and Virgus), but the taxonomic position of the New Zealand genus, Echyridella, remains uncertain as representatives of this genus have not been included in recent molecular phylogenetic studies of the Hyriidae (Graf et al., 2015; Santos-Neto et al., 2016; Miyahira et al., 2017). Consequently, Graf and Cummings (2021) have assigned Echyridella as incertae sedis within the Hyriinae. The Hyriinae are partitioned into three tribes from South America, Hyriini, Castaliini and Rhipidodontini, and one tribe from eastern Australia and Papua New Guinea, Hyridellini (genera: Cucumerunio, Hyridella and Virgus) (Walker et al., 2014; Graf and Cummings, 2021). Glochidial morphology and morphometry, and in some cases, release mechanisms and host fishes, have been documented for several species of Hyriidae from Australia (McMichael and Hiscock, 1958; Parodiz and Bonetto, 1963; Atkins, 1979; Walker, 1981; Humphrey and Simpson, 1985; Jones et al., 1986; Widarto, 1993; Jupiter and Byrne, 1997; Klunzinger et al., 2012, Klunzinger et al., 2013; Klunzinger, 2020; Klunzinger, 2023a), South America (Bonetto, 1951; Bonetto, 1959; Bonetto, 1961; Bonetto and Ezcurra, 1963; Parodiz and Bonetto, 1963; Alvarenga and Ricci, 1977; Bonetto et al., 1986;Mansur, 1999; Mansur and Campos-Velho, 1990; Ricci et al., 1990; Semenas et al., 1994; Mansur and Silva, 1999; Viozzi and Brugni, 2001; Beasley et al., 2005; Pimpão et al., 2012) and New Zealand (Percival, 1931; Clearwater et al., 2014; Brown et al., 2017; Hanrahan, 2019; Melchior et al., 2021a; Melchior et al., 2021b). However, glochidial morphometry and morphology and their mechanism of release, remain unknown in many Hyriidae. This study incorporates published information, together with new observations from recent acquisitions of glochidia, to describe and illustrate behavioural, morphological and morphometrical data for a total of 17 species of Australasian Hyriidae from 24 localities of Australia, New Zealand, and Papua New Guinea, supplemented with a provisional dichotomous key for their identification.
2 Materials and methods
2.1 Mussel collections and new observations
Details of collection localities and glochidial taxa included in this study are provided in Table 1. Distributions of focal taxa included in this study (based on Marshall et al., 2014; Walker et al., 2014; Klunzinger et al., 2022; Ponder et al., 2023) are provided in Supplementary Figure S1. Taxonomy follows Walker et al. (2014), Marshall et al. (2014), Klunzinger et al. (2022), Ponder et al. (2023) and Graf and Cummings (2023). Live samples of adult mussels of Cucumerunio novaehollandiae (Gray, 1843), Hyridella australis (Lamarck, 1819), Hyridella drapeta (Iredale, 1934), Hyridella glenelgensis (Dennant, 1898), Hyridella narracanensis (Cotton and Gabriel, 1932), and Velesunio angasi (Sowerby II, 1867) were hand-collected from multiple localities at varying times of the year; known times of brooding were gleaned from a combination of unpublished field observations and literature (Humphrey and Simpson, 1985; Jones et al., 1986; Playford and Walker, 2008; Jones, 2014; Treby, 2016). Atkins (1979) described the glochidia of H. drapeta from Diamond Creek, Victoria as having hooks, a larval filament and release mechanism resembling that of Velesunio ambiguus (Philippi, 1847) (as per Walker, 1981). Jones et al. (1986) found freshwater mussel specimens from the Macleay River, New South Wales, consistent with published and museum collections of H. drapeta, but the glochidia did not match the descriptions of Atkins (1979). Samples of glochidia from the species resembling H. drapeta were collected from adults in Diamond Creek (Table 1) to determine whether Atkins (1979) had potentially misidentified the species. Velesunio ambiguus was absent from the locality and subsequent investigations indicate that the species most recently collected has affinities with H. drapeta, but unpublished molecular sequence data indicate that it is an undescribed taxon, hereafter, Hyridella sp. ‘Diamond Creek’ (Klunzinger et al., unpublished data). Hand-collected mussels were retained in vessels containing water from each collection site, maintained at room temperature, and observed for glochidial release over several days, except for H. sp. ‘Diamond Creek’, where glochidia were collected directly from gravid females’ ctenidia and observed for maturity under a compound microscope in the field. Glochidia are deemed mature when they expand their valves to rupture vitelline membranes and then begin “winking”: rapidly opening and closing of the glochidial valves (cf. Jones et al., 1986; Kleinhenz et al., 2019). Glochidia of E. aucklandica and E. menziesii were collected and observed according to methods described by Melchior et al. (2021a). Glochidia of Alathyria profuga (Gould, 1851) were collected according to methods described by Jones et al. (1986). Samples of glochidia of Lortiella froggatti Iredale, 1934 were obtained, under license, from gravid females held in the malacology collection of the Australian Museum, Sydney (Museum Voucher No. C.414981).
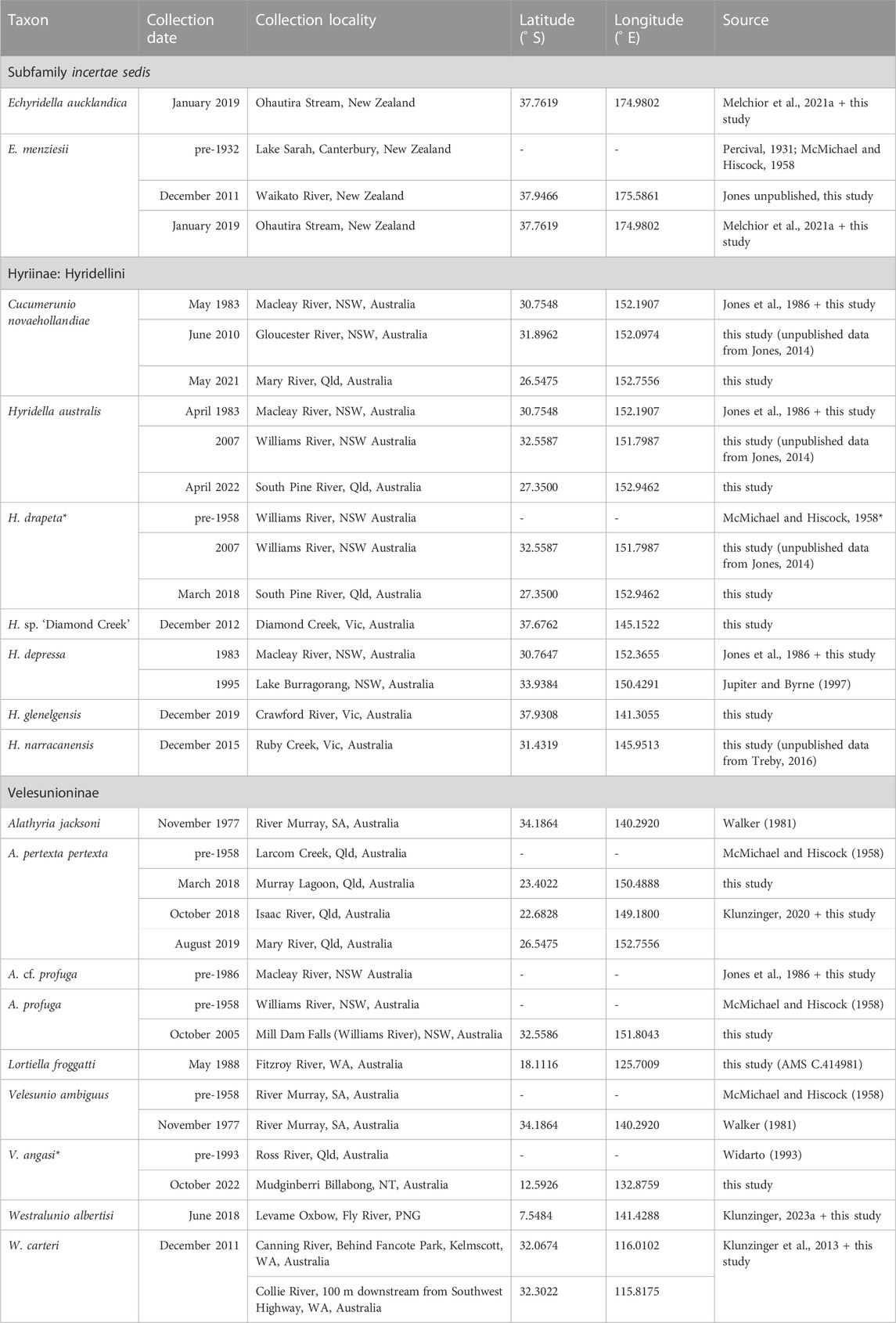
TABLE 1. Provenance details of glochidia from Australasian Hyriidae included in this study. NSW—New South Wales , NT—Northern Territory, PNG—Papua New Guinea, Qld—Queensland, SA—South Australia, Vic—Victoria, WA—Western Australia. *Note: McMichael and Hiscock (1958) incorrectly identified Hyridella drapeta as Hyridella australis in their glochidia figure and Widarto (1993) reported data for Velesunio ambiguus, but the species does not occur in the Ross River, Queensland and is more likely Velesunio angasi. Coordinate system: WGS 1984 EPSG: 4326.
Definitions of glochidial release mechanisms follow Watters (2008) and Haag (2012). Material released from exhalent siphons of live mussels was collected using a glass pipette, placed on a glass slide, and examined under light microscopy for the presence of glochidia with their behaviour observed. In C. novaehollandiae, H. australis and H. drapeta, brooding was confirmed by prying open the shell valves with reverse pliers or through dissection by inserting a scalpel between the two valves to cut adductor muscles to observe the inner pair of demibranchs for the presence of thickened, red-brown marsupia. Dissection was not performed in H. glenelgensis or H. narracanensis given these species have small sub-populations, restricted in geographic range, and listed as threatened (critically endangered or endangered) under the IUCN Red List, the Victorian Flora and Fauna Guarantee Act 1988, and the Commonwealth of Australia Environment Protection and Biodiversity Conservation Act 1999 (Klunzinger, 2023b). Live individuals of each of these two species were returned to the wild after glochidia were collected.
2.2 Glochidial morphometry and morphology
Samples of live-released glochidia from H. sp. ‘Diamond Creek’ were collected by glass pipette, fixed in 2% glutaraldehyde following Jupiter and Byrne (1997) and rinsed and stored in Sorenson’s sodium phosphate buffer for later study. Samples of live-released V. angasi (Mudginberri Billabong, Magela Creek, Northern Territory, Australia, Table 1) and Westralunio carteri Iredale, 1934 (from Canning River, Western Australia, Table 1) were separately collected by glass pipette, placed on a glass slide, and digitally photographed under compound light microscopy to capture details of soft tissues. Samples of glochidia from L. froggatti and A. profuga were flushed from the ctenidia of ethanol-preserved specimens of gravid females using a glass pipette. Samples of live-released glochidia for other species were collected by glass pipette and held in a glass jar with tap water for several days or up to a week, after which time glochidia had died and soft tissues had autolyzed, confirmed by examining a small sample of glochidia on a glass slide under light microscopy. Glochidial shells were then transferred by glass pipette into 5–mL microcentrifuge tubes containing 95% ethanol for preservation. Preserved glochidia were then deposited onto specimen stubs affixed with double-sided carbon tape using a glass pipette and allowed to air dry. Samples of H. drapeta and H. sp. ‘Diamond Creek’ were photographed on a Hitachi TM 3030 Plus Scanning Electron Microscope (SEM) and associated software at the Western Australian Museum in May 2019. Other samples were sputter-coated with platinum using a Safematic CCU-010 Compact Coating Unit and photographed using a Hitachi TM4000 Plus SEM and associated digital software at the University of Queensland Centre for Microscopy and Microanalysis between April 2022 and August 2023. Images or illustrations of glochidia from other studies (Alathyria jacksoni Iredale, 1934 and V. ambiguus, as per Walker, 1981; Hyridella depressa (Lamarck, 1819), as per Jones et al., 1986; W. carteri, as per Klunzinger et al., 2013; H. narracanensis, as per Treby, 2016; Alathyria pertexta pertexta Iredale, 1934, as per Klunzinger, 2020; Westralunio albertisi Clench, 1957, as per Klunzinger, 2023a; H. sp. ‘Diamond Creek’, taken at the Australian Centre for Microscopy and Microanalysis, University of Sydney, using a Zeiss EVO SEM (Jones, unpublished)) were also included for additional details of hook morphology.
Glochidia were measured to the nearest 1 µm from scale-imprinted SEM images for length (L), height (H), hinge length (Hg) and larval hook length (LHL) and to the nearest 1 degree (°) for angle of obliquity (Á) (see Figure 1). The Á was measured on just one individual valve that appeared to be resting flat on the specimen stub for each individual glochidium. The LHL values for each individual glochidium were measured either as the mean of two larval hooks (in the case where two opposing larval hooks could be accurately measured from an individual glochidium) or as an individual LHL (in the case where only one larval hook could be measured on an individual glochidium). Size was determined as the average of L and H. Additional data on shell size measurements of glochidia of various Australasian hyriid taxa were included from published literature and unpublished data of the authors.
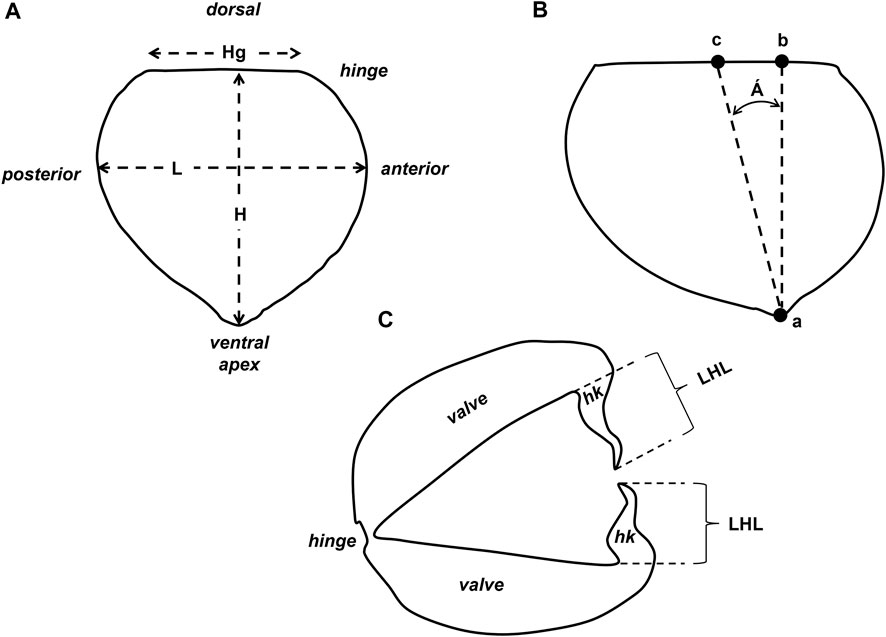
FIGURE 1. Morphometrical measurements of glochidial shells (right valve): (A) L, length, measured from anterior to posterior; Hg, hinge length; H, height, measured from dorsal hinge to ventral apex; (B) Á, angle of obliquity, measured in degrees (°), as the angle between the line that joins the ventral point (a) to the point perpendicular to the hinge (b) and the line that joins the ventral point (a) to the middle of the hinge line (c); (C) LHL, larval hook length, measured from the base of the junction with each valve to the terminal point of each larval hook (hk).
2.3 Statistical analyses
All statistical analyses were carried out using the R statistical package (R Core Team, 2022). To understand the similarities of complex glochidial shapes across species, metrics of glochidial dimensions and shape (H, L, Hg, H/L, Hg/L, LHL and Á) were normalised and Principal Component Analysis (PCA) used to reduce shape complexity into two or three explanatory dimensions. The closer two glochidial samples are in this reduced dimensional space, the more similar their overall shape. Samples within this space were then coded by their species affinity, highlighting species differentiation based on glochidial shape.
To further understand the specific morphometrical variables (i.e., discriminant functions) that best separated species, Linear Discriminant Analysis (LDA) was used (MASS package: Venables and Ripley, 2002); this analysis tests for group structure in multivariate data and identifies linear combinations of variables that best separate the input categories, in this case, the species.
Three measures of accuracy were then calculated to assess the classification of the glochidial samples into the species (as categories) based on morphological traits: 1) overall classification accuracy, 2) errors of omission (also known as producer’s accuracy) and 3) errors of commission (also called user’s accuracy) (Story and Congalton, 1986). An error of omission is the proportion of incorrectly classified glochidia for a known reference species, calculated as the number of correctly classified glochidia (on the major diagonal of the confusion matrix), divided by the number of samples of the reference species (i.e., the column total for the reference species). An error of commission is a measure of the reliability of the classification rule and addresses the question, “What percentage of glochidia classified as species i are actually species i?”. This is calculated as number of correctly classified glochidia divided by the row total for species i (i.e., the number of glochidia classified as species i). Error matrices compare the allocation of glochidia among species using discriminant functions with their known species identities.
In addition to quantitative analysis of glochidial shell metrics, multiple correspondence analysis (MCA) was used to group species according to qualitative measures of glochidial morphology and to overlay species associations with morphological characters (Table 2) using the functions in the FactoMineR package (Lê et al., 2008). Multiple correspondence analysis is essentially PCA of qualitative (i.e., categorical) variables instead of quantitative measurements (Abdi and Williams, 2012). Qualitative glochidial characters were scored for the glochidia of all species of Australasian freshwater mussels for which there were data (Table 1), and MCA was used to ordinate species in two dimensions.
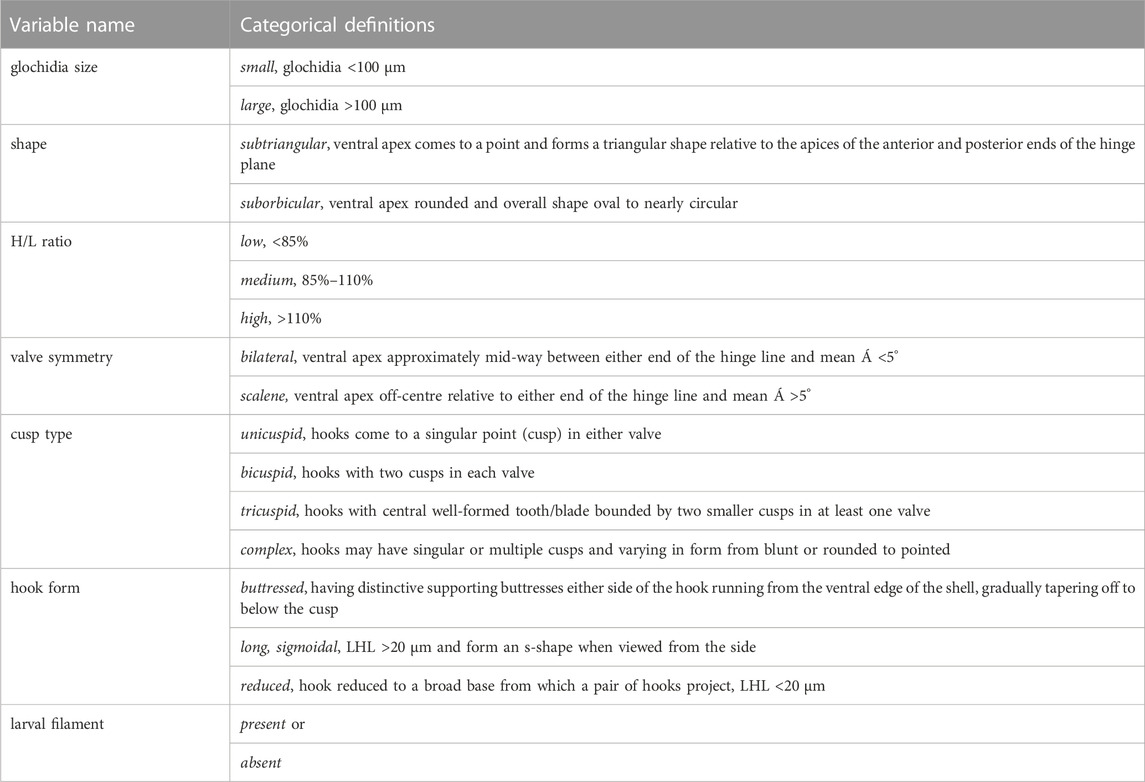
TABLE 2. Definitions of categorical variables used in multiple correspondence analysis (MCA) of glochidia of Australasian freshwater mussels (Unionoida: Hyriidae). Each defined category for a particular variable is provided in italic font.
3 Results
3.1 Marsupial occupancy and glochidial release
Marsupia occupied the majority of the inner demibranchs of ctenidia in C. novaehollandiae, the inner four-fifths in H. australis, and the inner two-thirds in H. drapeta and H. sp. ‘Diamond Creek’. Additionally, in H. australis, the dorsal one-third of the marsupia within the inner demibranchs were thickened and white in two dissected females. Marsupia occupied the upper four-fifths of the inner demibranchs of ctenidia in E. aucklandica (Melchior et al., 2021a) and the inner one third in E. menziesii (McMichael and Hiscock, 1958; Melchior et al., 2021a). The marsupia of members of the Velesunioninae generally occupy the inner two-thirds of the inner demibranchs (Humphrey and Simpson, 1985; Walker, 1981; Klunzinger et al., 2013; Klunzinger, 2020; 2023a; Jones, pers. obs.). Reproductive output for H. depressa is positively correlated with nutrient status of ambient waters (Byrne, 1998), while under eutrophic conditions, the marsupia may occupy the entire inner demibranchs in V. angasi (Humphrey and Simpson, 1985). As gravid adult females of H. glenelgensis and H. narracanensis were not dissected, the proportion of ctenidia occupied by marsupia was not determined.
Undissected H. australis released several white botuliform conglutinates, approximately 20–30 mm in length within 4 days post-collection. Glochidia released from H. glenelgensis and H. narracanensis released glochidia contained within sticky white to light yellow conglutinates, preceded by an enlargement of the exhalent siphon that turned from pale grey to dark grey or reddish brown and exhibited a “waving” motion prior to the release of conglutinates (Figure 2A–D, Figures 2G–L for H. glenelgensis and H. narracanensis, respectively); glochidia were only released after disturbance by squirting water in short bursts against the siphons of H. glenelgensis or tapping the shells of H. narracanensis with a blunt probe. Glochidia were released from C. novaehollandiae freely and were not contained in amorphous mucous conglutinates or any other form of conglutinate within 2 days post-capture (Figures 2E, F). Glochidia of H. drapeta were extruded from the exhalent siphons of brooding females as clear, amorphous, mucous conglutinates. Hyridella sp. ‘Diamond Creek’ are also thought to release glochidia as amorphous mucous conglutinates (Atkins, 1979, see discussion), although glochidial release of this taxon was not observed in the present study. Live samples of glochidia of H. sp. ‘Diamond Creek’ examined during the present study did not possess larval threads, unlike Atkins (1979) who described them as being present, presumably from the same taxon collected from the same stream. Westralunio carteri, A. pertexta pertexta and V. angasi release glochidia in amorphous mucous conglutinates (Humphrey and Simpson, 1985; Klunzinger et al., 2013; Klunzinger, 2020). Echyridella menziesii has been observed to release glochidia singularly and in amorphous mucous conglutinates (Melchior et al., 2021a). This form of glochidial release is illustrated in Figures 2M, N for E. menziesii and A. pertexta pertexta, respectively. Glochidia are released as functional conglutinates resembling vermiform macroinvertebrate prey items of fishes in E. aucklandica (Melchior et al., 2021a, Figures 2O–Q), similar in form to conglutinates released by those of H. australis (Jones et al., 1986; see also Section 4.1 below).
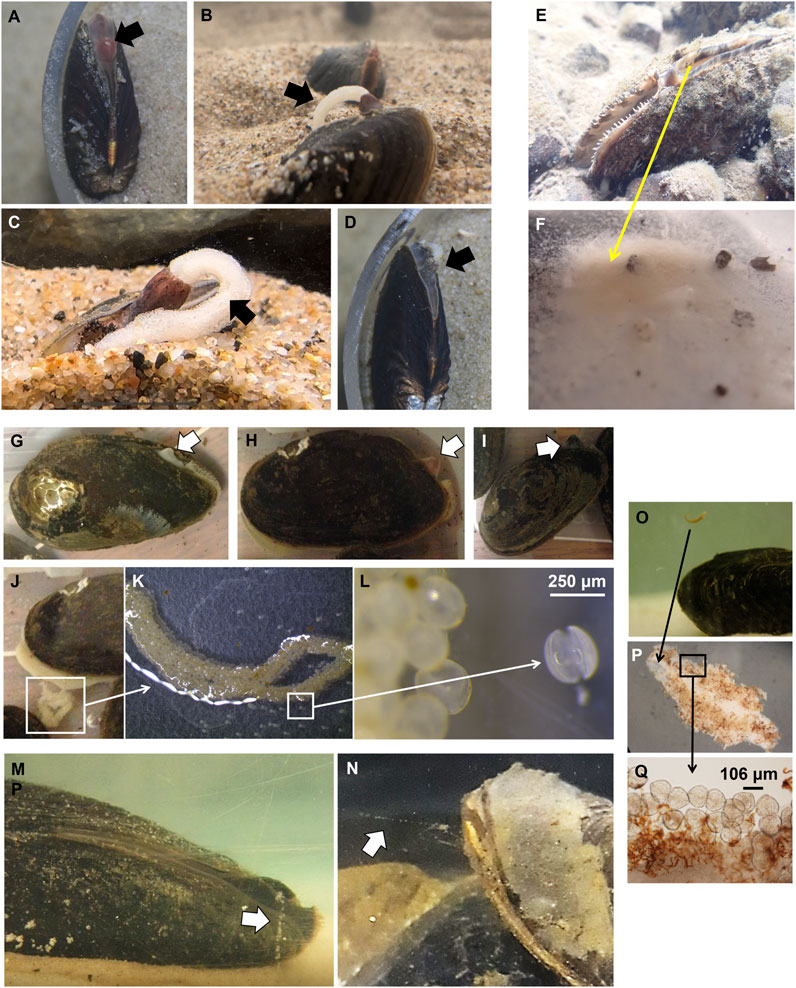
FIGURE 2. Glochidial release in Hyridella glenelgensis (Crawford River, Victoria, Australia): (A) exhalant siphon (block arrow) becomes enlarged and changes to bright pink in colour, followed by (B, C) release of thick white mesoconglutinates (block arrows), photos by Timothy Fernando, with permission, and (D) returning to normal size and mottled grey-brown (block arrow) post-release; Cucumerunio novaehollandiae (Mary River, Queensland, Australia): (E) Live gravid female in the wild in the burrowed position released, (F) individual mature, active glochidia as individuals, from the exhalant siphon (yellow arrow); Hyridella narracanensis (Ruby Creek, Victoria, Australia): siphon enlarges and changes colour from (G) white to (H) pink and (I) dark grey, followed by a waving motion and release of (J, K) conglutinates comprised of (L) individual glochidia held together by viscous adherent gel-like material; (M) Echyridella menziesii (Ohautira Stream, New Zealand; Melchior, 2021) and (N) Alathyria pertexta pertexta (Mary River, Queensland, Australia; Klunzinger, 2020), block arrows indicating amorphous mucous conglutinates; Echyridella aucklandica (Ohautira Stream, New Zealand; Melchior, 2021): (O, P) mesoconglutinates comprised of (Q) individual glochidia attached to conglutinate.
3.2 Glochidial morphology
Of the species studied, glochidia of E. menziesii and all velesunionine taxa except A. profuga possess larval filaments (Table 3). None of the glochidia in the Hyridellini in this study possessed larval filaments. Glochidia varied in size and shape among species and to a lesser extent within species. A summary of traditional morphometrical measurements obtained by this study, in comparison with other species and populations of Australasian glochidia for which published information is available, is provided in Table 4. The raw data from which this summary was tabulated are provided in Supplementary Table S1. To illustrate the variation in size and shape of glochidia, SEM images of shell valves are provided in Figure 3. Hook morphology is illustrated in Figure 4 with more detailed views and additional images of glochidia provided in the Supplementary Material (Supplementary Figures S2-S18). Soft anatomy of glochidia was generally not available, owing to the methods of preservation in this study, but an example of soft anatomical features from a live V. angasi glochidium, including adductor musculature and sensory hairs, is provided in Figure 5A and an example of a larval filament from a live image of W. carteri is shown in Figure 5B. Two valve shapes were apparent in the glochidia from this study (Table 3 and Figure 3): suborbicular in C. novaehollandiae, H. glenelgensis and H. narracanensis and subtriangular for all other species in this study. The H/L ratio, a measure of “squatness”, varied from tall and narrow (H/L >100%) to short and broad (H/L <100%) with the two extremes in form represented by C. novaehollandiae and H. australis (H/L 110%–128%) compared with E. aucklandica, A. profuga and W. carteri (H/L 81%–85%). In terms of valve symmetry, most glochidia studied here are scalene, apart from A. jacksoni, C. novaehollandiae, E. aucklandica, H. australis, H. glenelgensis, H. narracanensis and one population of V. ambiguus that have ventral apices located approximately mid-way between either end of the hinge line (Á, 0–5°), imparting bilaterally symmetrical valves (Table 3). The most bilaterally asymmetrical species is W. carteri that has a much larger Á (19.8°) than other scalene species which ranged from about 8 to 10° in A. profuga, H. drapeta, A. pertexta pertexta and V. angasi, to about 12–13° in L. froggatti, W. albertisi and one population of V. ambiguus, with E. menziesii having the second largest Á at 15.2°.
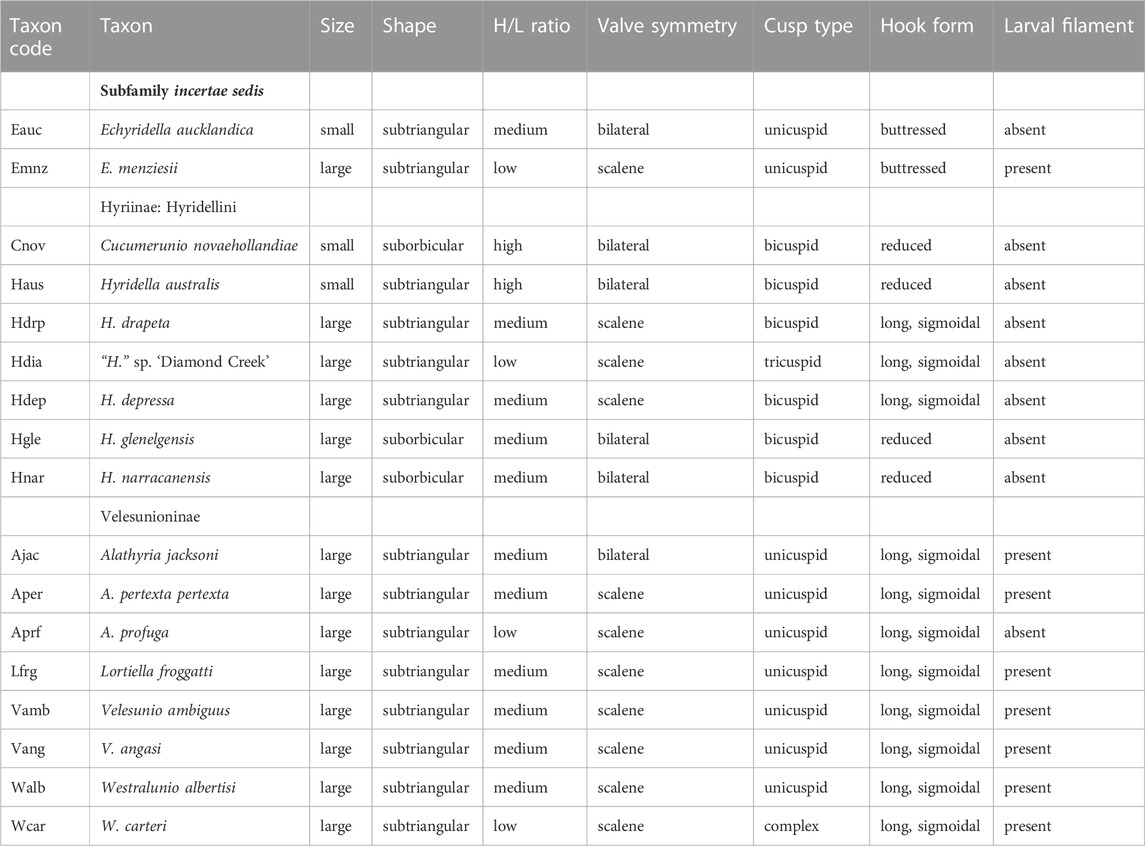
TABLE 3. Qualitative traits of Australasian glochidia used in multiple correspondence analysis (MCA). Definitions of each trait are provided in Table 2.
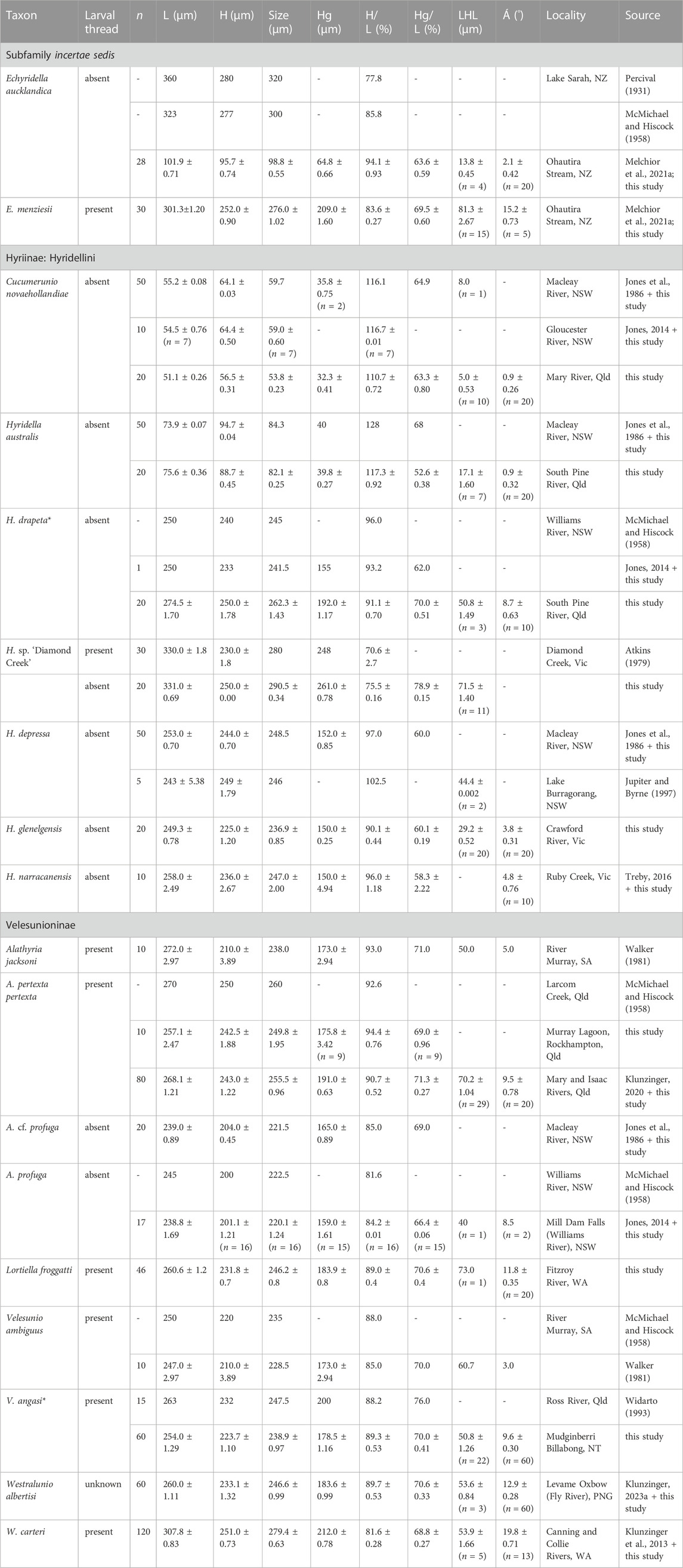
TABLE 4. Glochidial dimensions in Australasian freshwater mussels (Bivalvia: Hyriidae) included in this study. Values presented are means ± standard errors. Taxonomy follows Walker et al. (2014) and Marshall et al. (2014); note, unpublished preliminary analysis of genetic sequences suggest Hyridella sp. ‘Diamond Creek’ is an undescribed species. Abbreviations: H, height; L, length; Hg, hinge length; LHL, larval hook length; Á, angle of obliquity; Australia: NSW—New South Wales, NT—Northern Territory, Qld—Queensland, SA—South Australia, Vic—Victoria, WA—Western Australia; NZ—New Zealand; PNG—Papua New Guinea. Data of individually measured glochidia are provided in Supplementary Material. *Note: data reported for Hyridella drapeta was originally misidentified by McMichael and Hiscock 1958 as Hyridella australis and data reported for Velesunio angasi was originally misidentified as Velesunio ambiguus by Widarto (1993).
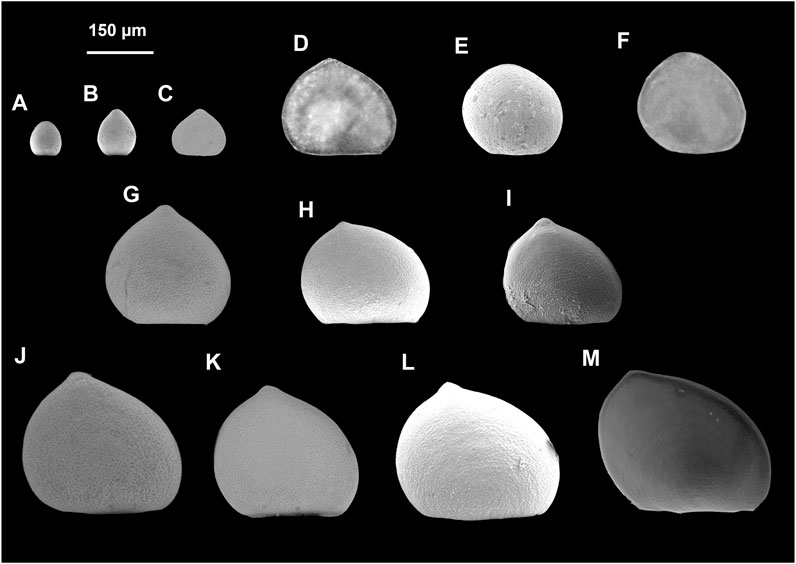
FIGURE 3. Size and shape variation in shell outlines of Australasian hyriid glochidia from this study: (A) Cucumerunio novaehollandiae, (B) Hyridella australis, (C) Echyridella aucklandica, (D) Alathyria profuga, (E) Hyridella glenelgensis, (F) Hyridella narracanensis, (G) Hyridella drapeta, (H) Echyridella menziesii, (I) Lortiella froggatti, (J) Alathyria pertexta pertexta (see also Klunzinger, 2020), (K) Velesunio angasi, (L) Westralunio albertisi (see also Klunzinger, 2023a), (M) Westralunio carteri (see also Klunzinger et al., 2013).
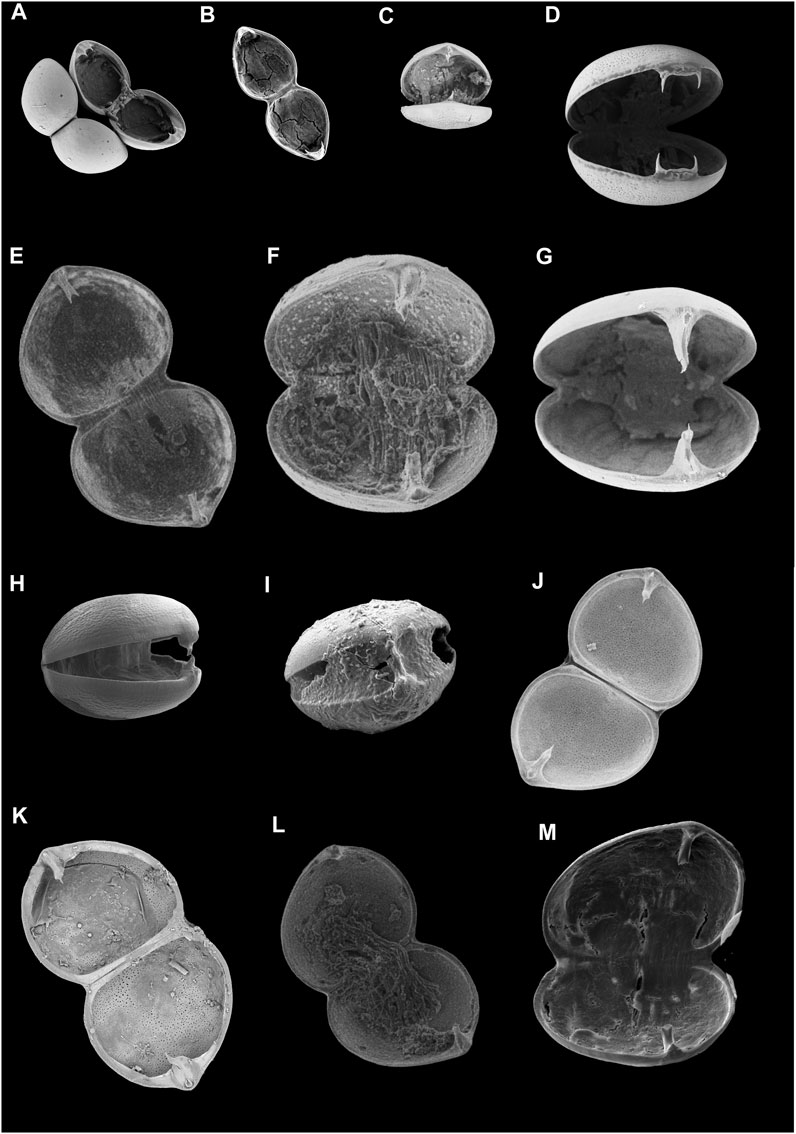
FIGURE 4. Open valves of glochidia showing hook morphology in species from this study. Hyrrinae: Hyridellini and Echyridella: (A) Cucumerunio novaehollandiae, (B) Hyridella australis, (C) Echyridella aucklandica, (D) Hyridella glenelgensis, (E) Hyridella drapeta, (F) Hyridella sp. ‘Diamond Creek’, (G) Echyridella menziesii; Velesunioninae: (H) Alathyria profuga, (I) Lortiella froggatti, (J) Alathyria pertexta pertexta, (K) Velesunio angasi, (L) Westralunio albertisi, (M) Westralunio carteri. Images not to scale. Additional images and species are provided in the Supplementary Material.
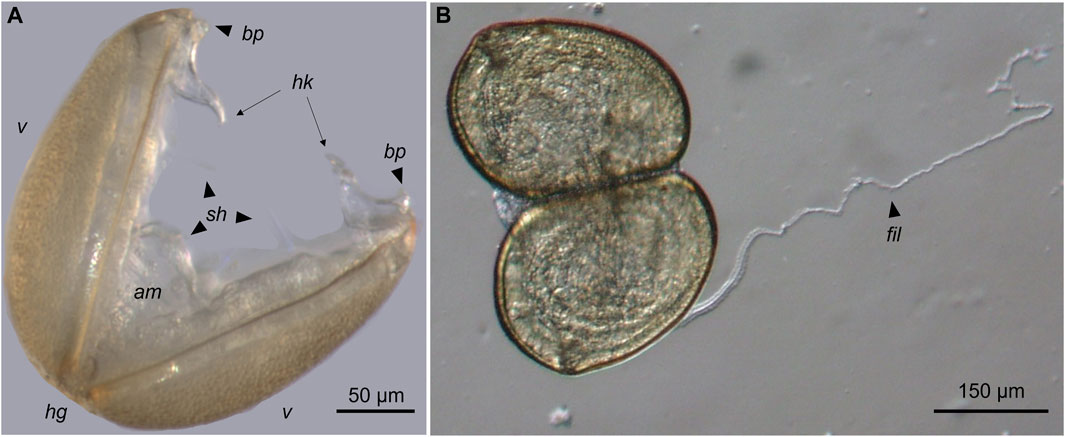
FIGURE 5. (A) Mature, live glochidium of Velesunio angasi (Mudginberri Billabong, Northern Territory, Australia) immediately post-release from a gravid female showing details of anatomical features: am–adductor muscle, bp–basal protuberance; hg–hinge; hk–hook, sh–sensory hairs, v–valves of shell. (B) Example of a larval filament (fil), this specimen of Westralunio carteri (Canning River, Western Australia).
The glochidia of Velesunioninae generally have unicuspid sigmoidal hooks, apart from W. carteri that has complex hooks which are variably uni-, bi-, or tricuspid (Table 3; Figures 4H–M). The hooks of E. aucklandica are distinctly unicuspid. In E. menziesii they are also unicuspid although the cusps of the opposing hooks in E. menziesii are not identical (Table 3; Figure 4C). In E. menziesii one hook terminates as a spatulate knob with a spinose tooth projecting from the end; the opposing hook is more claw-like but with two notches on each side of the hook (Figure 4G) that may allow the hook to snap off when the valves close.
Glochidial hook morphology in the Hyridellini is much more morphologically diverse than in the Velesunioninae (Table 3; Figures 4A, B, D, E, F). The Hyridellini generally have bicuspid hooks, apart from H. sp. ‘Diamond Creek’ that is tricuspid (Figure 4F). Hooks in C. novaehollandiae, E. aucklandica, H. australis, H. glenelgensis, and H. narracanensis are greatly reduced in length compared to other species studied (Tables 3, 4; Figures 4A, B, D, E; Supplementary Material), having a common base with two sharp cusps on either valve, but the length of teeth and degree of separation between cusps varies among taxa. In C. novaehollandiae, hooks are reduced to a pair of sharp spines just below the apex of each valve (Figure 4A) but in H. glenelgensis and H. narracanensis, the paired spines are longer, separated by a blade-like cutting edge (Figure 4D; Supplementary Material). The modified hooks of H. australis differ again and consist of a pair of curved, conjoined spines united on a common, slightly-raised base, that curve in the latero-dorsal plane (Figure 4B).
3.3 Comparative analyses
The five metrics of glochidial valve dimensions (L, H, Hg, H/L and Hg/L) were highly correlated, especially L, H, and Hg (Table 5). PCA separated the glochidial species into two distinct groups along the first principal component (Figures 6A, B), based mainly on size and H/L ratio along PC1, which explained 81% of the total variation (Table 6). Glochidia were further separated along the second and third principal component axes (explaining an additional 15% of variation) according to shape (Á and H/L ratio) and LHL (Table 6). Principal Component 2 can be interpreted as contrasting scalene glochidia with those that have high H/L ratios and possess long sigmoidal hooks. Principal Component 3 contrasts glochidia that are tall (high H/L ratio) and bilaterally symmetrical with scalene glochidia that have short, wide valves. Many of the medium-sized and large glochidia could not be separated using these morphometrical criteria.
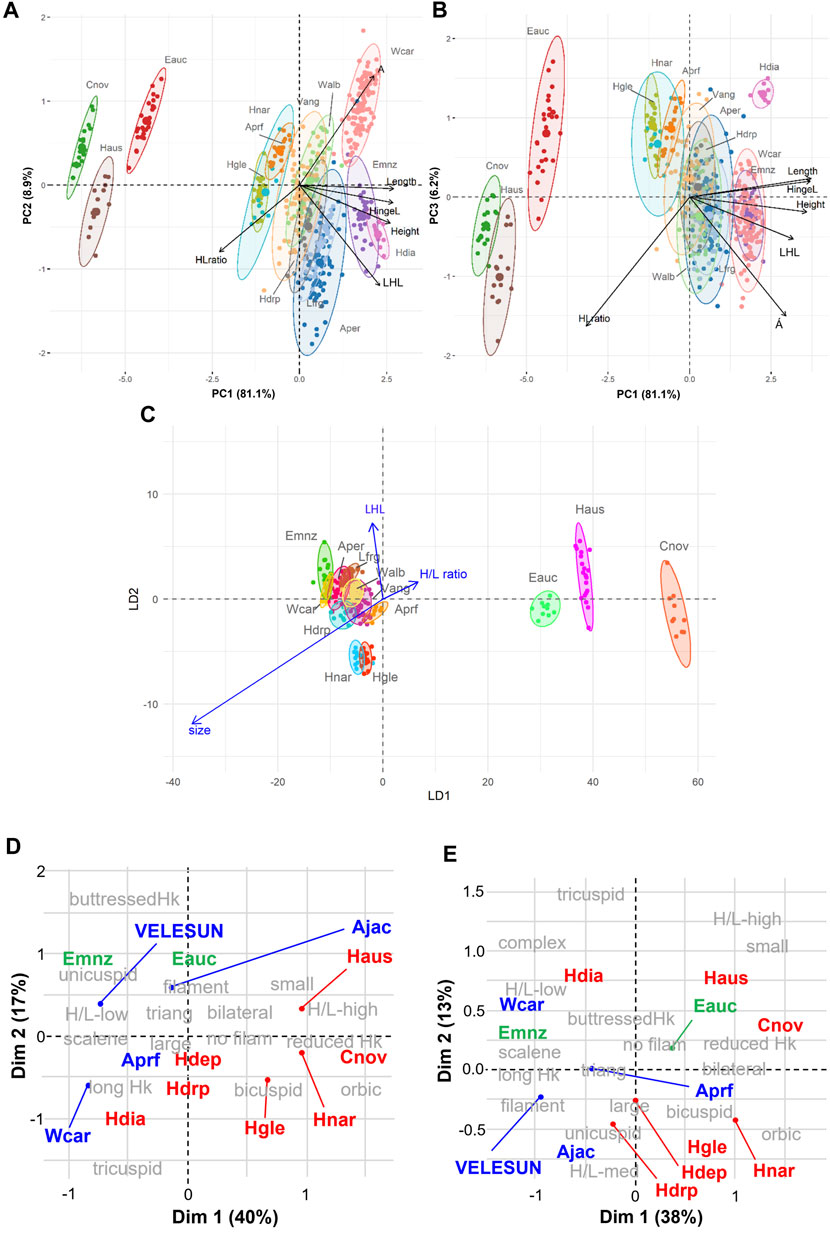
FIGURE 6. Principal component biplots for six morphometrical metrics of glochidial size and shape for (A) first and second principal components (PC1 and PC2, respectively), and (B) first and third principal components (PC1 and PC3, respectively). (C) Linear discriminant biplot for three morphometrics of glochidial size and shape for first and second linear discriminant functions (LD1 and LD2). Different colours in A-C represent different species. Multiple correspondence biplots for (D) Dimensions 1 and 2 (Dim 1 and Dim 2, respectively), and (E) Dimensions 1 and 3 (Dim 1 and Dim 3, respectively); subfamilies are colour-coded with Velesunioninae in blue text, Hyriinae in red text and the two Echyridella species in green; categorical data have grey text; ‘VELESUN’ includes five species of Velesunioninae (Vamb, Vang, Lfrg, Aper and Walb). See Table 3 for species abbreviations. Glochidial morphomertics: Á—angle of obliquity, H—height, L—length, HL ratio = H/L, LHL—larval hook length, Size = average of H and L. Abbreviations for categorical morphological traits (defined in Table 2): Hk—hook, med—medium, nofilam—larval filament absent, orbic—suborbicular, triang—subtriangular.
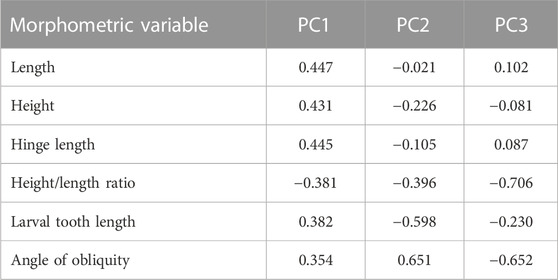
TABLE 6. Loadings on morphological variables for the first three principal components. The first three principal components accounted for 96% of the variation in glochidial morphometry (81.1%, 8.9% and 6.2% for PC1, PC2 and PC3, respectively).
LDA showed that 97.5% of the between-group variance was accounted for by the first discriminant function (LD1), which reflected glochidial size and H/L ratio (Table 7). Only 1.8% of the between-group variance was accounted for by the second discriminant function (LD2), which further emphasized size but also separated species on hook length. The first two discriminant functions (LD1 and LD2) had an overall classification accuracy of 82.7%, a substantial improvement on the first discriminant function alone (57.5%). The three species with small glochidia, C. novaehollandiae, H. australis and E. aucklandica, were readily distinguished from all other species (Figure 6C) with no errors of omission or commission (Tables 8, 9). While there was considerable overlap in glochidial morphometrics of the remaining ten species, the first two discriminant functions classified most species with a high level of accuracy (Table 8). Linear Discriminant Analysis misclassified 27.5% of A. pertexta pertexta and 17% of L. froggatti glochidia. The largest errors of omission and commission were between V. angasi and W. albertisi, which show considerable overlap in size and shape metrics (Supplementary Table S2); 28% of V. angasi glochidia were misclassified as W. albertisi while 16.6% of W. albertisi were misclassified as V. angasi (Table 9).
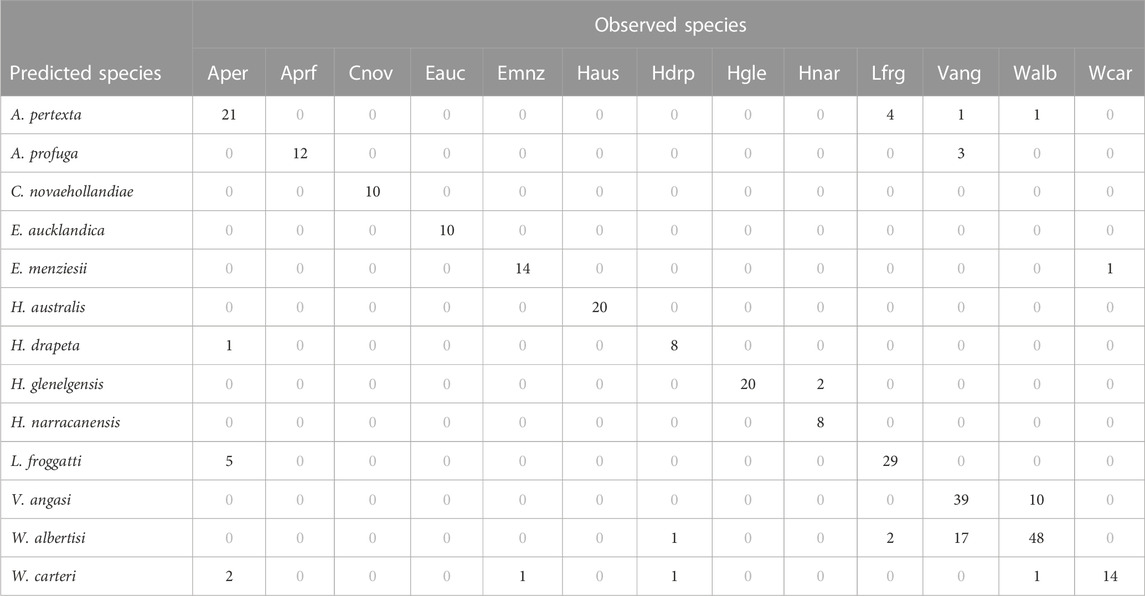
TABLE 8. Confusion matrix summarising the classification accuracy of the first two linear discriminant functions in assigning glochidia to the correct species class. See Table 3 for species abbreviations.

TABLE 9. Accuracy measures (%) for each species from an LDA classification based on two discriminant functions. See Table 3 for species abbreviations. Overall accuracy is 82.7%.
Differences among species were also assessed using qualitative morphological traits by MCA (Figures 6D, E). Dimension 1 explained 40% of the variance in glochidial morphology; Dimension 2 explained 17%; and Dimension 3 explained 13% of the variance. Glochidia were partitioned into two broad morphological groups in the MCA biplots: i) large, subtriangular, scalene shells with a low to medium H/L ratio, and ii) small, suborbicular, bilaterally symmetrical shells with a high H/L ratio. The latter group also lacked a larval filament, and in the Australian Hyridellini, the hooks were bicuspid with a pair of short teeth projecting from a reduced base. Five of the velesunionine species shared the same morphological characters (Table 3) and were grouped together as “VELESUN” in the MCA biplots. The glochidia of Velesunioninae were typically large, subtriangular, and scalene with a sigmoidal hook terminating in a fluted, single pointed cusp (Figures 6D, E) except for W. carteri that had a complex cusp. The glochidia of all known members of the Velesunioninae possessed a larval filament except for A. profuga. The glochidia of several members of the Hyriinae, including E. menziesii from New Zealand, were similar in morphology to those of the Velesunioninae. Glochidia of the Hyridellini differed from those of the Velesunioninae in having bicuspid larval hooks or, in the case of H. sp. ‘Diamond Creek’, tricuspid. The hooks of E. menziesii were unicuspid, like those of the glochidia of the Velesunioninae but were also spinose and had more claw-like cusps, compared with the Velesunioninae that had hooks that were more fluted and had more blade-like cusps. In addition, the sigmoidal hooks of Echyridella spp. were buttressed and reinforced along their length.
4 Discussion
While adult freshwater mussels are moderately sedentary, the potential widespread dispersal is facilitated during the (usually) parasitic larval phase, a comparatively rapid phase in their otherwise relatively long lifespan. The ecology of glochidial release from adult mussels and the morphology of the glochidia themselves are therefore integral components of freshwater mussel survival. Freshwater mussels globally are threatened and while the focus has often been on the adult life stage, an understanding of glochidial form, function and environmental requirements is also important for their conservation (Ferreira-Rodríguez et al., 2019; Aldridge et al., 2022; Geist et al., 2023). This study is the first to statistically compare the morphology and release mechanisms of glochidia from a large suite of species belonging to the Australasian Hyriidae. The addition of new data in the present study significantly expands the current knowledge of glochidial release mechanisms and glochidial morphological diversity among Australasian Hyriidae which are inherently important in taxonomy and conservation.
4.1 Glochidial release mechanism
The first description of glochidial release in functional conglutinates in Australasian Hyriidae (H. australis) was made by Jones et al. (1986); the present study now provides illustrations and descriptions of similar release mechanisms in a further two Australian hyriids (H. glenelgensis and H. narracanensis). Laboratory studies of the hyriid E. aucklandica provided the first photographic evidence of mesoconglutinate release (Melchior et al., 2021a) with further studies demonstrating that fishes are attracted to the mesoconglutinate as a food item that resembles prey (Melchior et al., 2021b); this supported the conjecture of Jones et al. (1986) that the conglutinate is a form of lure (i.e., functional conglutinate). Preliminary evidence indicates that glochidia of H. glenelgensis attach to the gills of Galaxias maculatus (Jenyns, 1842) (= Mesites attenuatus and M. maculatus) suggesting the mesoconglutinates may be ingested by host fish and thus, may indeed be a lure (Raadik et al., unpublished data). This phenomenon is not dissimilar to some species of North American Lampsilini (Unionidae) that release mesoconglutinates in a similar way (Barnhart et al., 2008). However, by comparison, the conglutinates of the hyriids are not as elaborate and sophisticated as seen in some Unionidae, such as the blackfly pupae mimics of Ptychobranchus subtentum (Say, 1829) (see Barnhart et al., 2008), complex baited brood lures of Cyprogenia stegaria (Rafinesque, 1820) or complex tethered pisciform brood lures of Hamiota perovalis (Conrad, 1834) (Hewitt et al., 2021).
The release of mesoconglutinates from H. glenelgensis and H. narracanensis was consistent with the observations of Jones et al. (1986) for H. australis, as well as H. australis from South Pine River, Queensland, observed in this study. Laboratory observations confirm that the glochidia of H. glenelgensis attach to the gills of fish but may not be retained on the fins (Fernando and Raadik, unpublished data). Although glochidial infestation on wild-caught fishes or from laboratory exposure studies have not been reported for these Hyridella species, the release of mesoconglutinates would suggest that glochidia of these species preferentially attach to gills in a manner like the glochidia of E. aucklandica (Melchior et al., 2021b). The colour change of the exhalant siphons in H. glenelgensis and H. narracanensis and pulsating or “waving” behaviour exhibited by H. glenelgensis and H. narracanensis (and by H. australis from Jones et al., 1986) may also provide additional stimulus to attract host fishes. Similarly, distinct morphological changes in siphon colour, size and shape prior to glochidial release have also been observed for the South American hyriid, Castalia ambigua Lamarck, 1819, that the authors suggested were host attraction mechanisms, although this species does not release conglutinates (Santos et al., 2021).
The present study also offers some contradictions on larval release to those reported in the literature; the glochidia from the Diamond Creek population of H. sp. ‘Diamond Creek’ did not possess a larval filament despite Atkins (1979) previously reporting its presence, and the glochidia of E. menziesii examined in this study does possess a larval filament (H.A. Jones, pers. obs.), although it was not reported by Melchior et al. (2021a). Larval filaments have also been reported in the velesunionine glochidia of A. jacksoni (Walker, 1981), A. pertexta pertexta (Klunzinger, 2020), V. ambiguus (Parodiz and Bonetto, 1963; Walker, 1981), V. angasi (Humphrey and Simpson, 1985), W. carteri (Klunzinger et al., 2013), and L. froggatti (this study). Amorphous mucous conglutinates are typically a passive entanglement mechanism and the larval filament may assist glochidia to remain in suspension over habitat substrates such as aquatic macrophytes, silty sediment, and woody debris, where potential host fishes are likely to frequent and subsequently contact glochidia from mucous webs (Matteson, 1948; Haag and Warren, 2003; Watters, 2008; Haag, 2012; Klunzinger et al., 2012; Klunzinger et al., 2013; Melchior et al., 2021a; Melchior et al., 2021b).
Glochidia arising from amorphous mucous conglutinates typically attach to the fins of host fishes, but occasionally also to gills and other body surfaces (Humphrey and Simpson, 1985; Barnhart et al., 2008; Klunzinger et al., 2012; Haag, 2012), whereas species that release glochidia via mantle lures, mesoconglutinates or freely into the water column, preferentially parasitise gills as a consequence of active host predation (Bauer and Wächtler, 2001; Strayer, 2008; Haag, 2012). While it was beyond the scope of this paper to explore host-fish relationships and deeper discussions about host fish use, the literature indicates that Australasian hyriid glochidia are host fish generalists, utilising a broad suite of both native and alien fishes that support metamorphosis to the juvenile stage, although not in equal proportions. The exception to this, however, is the glochidia of E. aucklandica that have only been found on the gills of New Zealand smelt, Retropinna retropinna (Richardson, 1848) and no other fishes, and indeed this has been the only host fish species to date shown capable of supporting metamorphosis of glochidia to the juvenile stage under laboratory conditions (Melchior et al., 2021b).
The glochidia of a larger group of hyriids, including C. novaehollandiae, H. australis, H. depressa, H. drapeta, H. glenelgensis, H. narracanensis, A. profuga and E. aucklandica, lack larval filaments. Cucumerunio novaehollandiae produces many more glochidia than other Australasian hyriids, owing to the small size of the glochidia and the large marsupia of adult female mussels (Jones et al., 1986; Jones, 2014). Jones (2014) found that some C. novaehollandiae produce broods of up to 4 million glochidia, whereas H. depressa has glochidial counts of less than 120,000 (Byrne, 1998). However, C. novaehollandiae reproduces once per year whereas other hyriids can produce multiple broods throughout the warmer months of the year (e.g., H. depressa from Jones et al., 1986; Byrne, 1998). Cucumerunio novaehollandiae is also a known gill parasite (Jones, 2014) and release of free glochidia into the water column and surrounding habitat (that tends to be free from fine sediments that could otherwise smother glochidia) as fish pass by would mean that their mode of release is passive. A strategy of release of mesoconglutinates for this species may be negated by scour and potential dislodgement from the stronger currents in which this species inhabits; thus, releases of free glochidia but in very large numbers may nullify losses in faster stream currents. On the other hand, C. novaehollandiae occupies microhabitats that are between or in the lee of large boulders, so the glochidia may accumulate within the protection of the separation zones and within the boundary layer of the stream where fishes may seek shelter from water currents, resulting in close contact with the large numbers of glochidia and infestation of the gills. At least some margaritiferids release their tiny glochidia in a similar way and occupy similar habitats elsewhere (Araujo et al., 2000; Hastie et al., 2000). Melchior et al. (2021a) reported a mean fecundity in E. aucklandica of 17,840 glochidia (range 1,737 to 34,570) compared to nearly double that exhibited by coexisting E. menziesii (mean 44,016, range 28,840 to 72,000). Here, through its specialised “brood luring” mesoconglutinates, E. aucklandica may face a trade-off in increased energy costs for reduced fecundity. Conversely, E. menziesii, as a host generalist, uses a more energy-efficient reproductive strategy, which may allow for greater reproductive output.
The Australian Hyriinae are almost exclusively associated with lowland sections of coastal rivers of Victoria, New South Wales, and southern Queensland (Walker et al., 2014) that arise steeply in the escarpment of the Great Dividing Range (Rustomji et al., 2009). By Australian standards, this region is relatively high in species diversity with as many as six species of freshwater mussel occurring within a river system. The rivers of southeastern, coastal Australia are perennial, with good water quality, relatively high in dissolved oxygen and water clarity (Jones and Byrne, 2014; Walker et al., 2014). Echyridella aucklandica, which produces mesoconglutinates, is almost exclusively a lotic habitat species, occurring primarily in relatively clear northern New Zealand coastal streams (Marshall et al., 2014; Melchior et al., 2021a). In contrast, velesunionine species of Australia and E. menziesii of New Zealand are widely distributed in lakes and rivers throughout much of each country and are typically resident in more languid and (periodically at least) turbid waters, many of which are characteristic of temporary and/or lentic waterbodies (Marshall et al., 2014; Walker et al., 2014). Studies on patterns of distribution and abundance of Australasian freshwater mussels are limited, but generally, predation pressures and food limitation are thought not to limit freshwater mussel distribution (Haag and Warren, 1998; Raikow and Hamilton, 2001; Hewitt et al., 2021). The available, albeit limited, data show Australian freshwater mussels to be host generalists, coinciding with studies pertaining to species that release glochidia freely or contained in amorphous mucous conglutinates; there is little evidence amongst these species that the distribution patterns of freshwater mussels, within drainages, are limited by host fish behaviour and movements (Walker, 1981; Humphrey and Simpson, 1985; Klunzinger et al., 2012; Jones, 2014). Rather, spatial patterns within rivers appear to be controlled by abiotic factors (Humphrey and Simpson, 1985; Brainwood et al., 2006, Brainwood et al., 2008a, Brainwood et al., 2008b; Jones, 2006, Jones, 2014; Jones and Byrne, 2010, Jones and Byrne, 2014; Klunzinger et al., 2015), but contemporary distributions have been fragmented by habitat loss (Jones and Byrne, 2010, Jones and Byrne, 2014; Klunzinger et al., 2015; Sheldon et al., 2020) and declines in fish populations in many waterbodies may be inhibiting recruitment of young freshwater mussels (Byrne, 1998; Walker, 2017). Haag and Warren (1998), however, found no evidence of partitioning amongst unionids in North American streams because of limited space, but highlighted the importance of the host-fish and glochidial release relationship, noting host-specificity for species that utilise lure mechanisms to attract host species. It is worth noting that the concept of host-specificity amongst unionid mussels using such glochidial prey-mimetic lures rarely applies to a single fish species, but rather to a very limited number of fish species (e.g., Hewitt et al., 2021). Hewitt et al. (2019) noted as well that many glochidial–host interactions still remain to be identified, making it difficult in some cases to assign particular freshwater mussel species as either host specialists or host generalists. In locations where Australian freshwater mussel diversity is relatively high, whether seasonality in glochidial release and host specificity may have evolved to partition host fish resources is debatable, particularly given the currently limited published data available to pursue this hypothesis. Nevertheless, in high clarity waters where most Australasian Hyriinae occur, mesoconglutinates may be a more efficient mechanism for infesting host fishes than the passive infestation strategy typical of velesunionine species that tend to occur in more turbid habitats (for which visual attractants would serve limited purpose). Only more complete data on field-infested fishes to identify hosts for species that release glochidia in mesoconglutinates may elucidate the underlying evolutionary advantage of this strategy amongst the Hyriinae.
4.2 Glochidial shell morphology
In agreement with Jones et al. (1986), glochidia from Queensland populations of C. novaehollandiae, H. australis and H. drapeta have bifurcated hooks, and similar shell size and shape. Hyridella glenelgensis and H. narracanensis also have hook bifurcation, although the separation between cusps is set wider apart than other species studied to date, apart from H. australis. Hooks are, however, much straighter, and perpendicular to opposing valves than H. australis that are more laterally splayed. Bifurcated hooks in glochidia of H. depressa were illustrated in each of Jones et al. (1986) and Jupiter and Byrne (1997) (see also Supplementary Figure S4).
Atkins (1979) illustrated H. sp. ‘Diamond Creek’ glochidia as having hooks similar in form to several members of the Velesunioninae, including A. jacksoni and V. ambiguus (Walker, 1981; Klunzinger, 2020), L. froggatti and A. profuga (this study), V. angasi (Humphrey and Simpson, 1985 and this study), and A. pertexta pertexta (Klunzinger, 2020), which typically have s-shaped hooks with singular pointed cusps on opposing valves. We questioned whether the species Atkins (1979) illustrated may have been V. ambiguus given this similarity and because research by Jones et al. (1986) revealed hooks that are bifurcated in Hyridella species, very different in form to velesunionine glochidia. Furthermore, the dimensions of this species’ glochidia are distinct from northern populations of H. drapeta. To investigate, specimens of gravid adults were collected from the same location as in Atkins (1979); however, whilst the adults were similar in form to other H. drapeta, unpublished genetic evidence has indicated that the taxon is not H. drapeta and is an undescribed species. The present study confirmed that this taxon does indeed have s-shaped hooks, in support of Atkins (1979); however, upon closer examination of hooks from SEM photography, the cusps were revealed to be tripartite rather than singular as illustrated by Atkins (1979), and thus different to the velesunionine glochidia, thereby supporting their position in the Hyridellini as opposed to Velesunioninae as originally implicated by the illustrations of Atkins (1979). Similarly, hooks of E. menziesii superficially resemble those of Velesunioninae, but the hooks are distinctively spinose with wide supporting buttresses and may also be mildly tripartite. Westralunio carteri has atypical and complex hooks in comparison to other Velesunioninae (Klunzinger et al., 2013 and this study). Westralunio albertisi, however, has glochidia that are morphologically similar to other Velesunioninae and although Klunzinger (2023a) described them as being divergent, there is the possibility that the specimens examined may have not been fully mature and hooks had not yet fully developed.
Glochidia from C. novaehollandiae, H. australis and E. aucklandica were exceptional in their smaller size compared to those of the other species included in this study. Cucumerunio novaehollandae has perhaps one of the smallest recorded glochidia globally, rivalled only by a few margaritiferid species, Margaritifera dahurica (Middendorff, 1850), Margaritifera falcata (Gould, 1851), M. margaritifera and Cumberlandia monodonta (Say, 1829) (see Harms, 1909; Howard, 1915; Murphy, 1942; Bauer, 1994; Nezlin et al., 1994; Pekkarinen and Valovirta, 1996; Baird, 2000; Ieshko et al., 2014; Vikhrev et al., 2019), all of which are similarly miniscule in shell length (<60 μm). It has been proposed that such small glochidia (<65 μm in size) may be an adaptation to enhance suspension in the water column (Barnhart et al., 2008). At the other extreme, glochidia of E. menziesii, W. carteri and H. sp. ‘Diamond Creek’ are the largest of the Australasian hyriids measured to date with mean shell lengths between 300 and 330 μm. While they are certainly not the largest in the world, they are comparable in size to several unionids including Prolasmidonta heterodon (Lea, 1859) (= Alasmidonta heterdon), Pressodonta viridis (Rafinesque, 1820) (= Alasmidonta viridis), Utterbackiana suborbiculata (Say, 1831) (= Anodonta suborbiculata), Anodonta cygnea (Linnaeus, 1758) and Utterbackia imbecillis (Say, 1829) (see Surber, 1912; Claes, 1987; Niemeyer, 1992 in Wächtler et al., 2001; Hoggarth, 1999; Kennedy and Haag, 2005). Among Hyriidae, few glochidia exceed shell lengths of more than 300 μm, although the South American hyriids Diplodon charruanus (d’Orbigny, 1835) (= Diplodon trivialis), Diplodon martensi (Ihering, 1893) (= Diplodon decipiens + Diplodon suppositus), Diplodon delodontus (Lamarck, 1819), Diplodon multistriatus (Lea, 1831) (= Diplodon expansus), Diplodon garbei (Ihering, 1910), Diplodon uruguayensis (Lea, 1859) (= Diplodon hasemani), Diplodon iheringi (Simpson, 1914), Diplodon obsolescens (Baker, 1913), Diplodon paulistus (Ihering, 1893) (= D. paulista), Diplodon suavidicus (Lea, 1859), Diplodon rhuacoicus (d’Orbigny, 1835) (= Diplodon trivialis + Diplodon yaguaronis) and Triplodon corrugatus (Lamarck, 1819) reach lengths of 300–320 μm (Bonetto, 1951; Bonetto, 1959; Bonetto, 1961; Bonetto and Ezcurra, 1963; Parodiz and Bonetto, 1963; Alvarenga and Ricci, 1977; Bonetto et al., 1986; Mansur, 1999; Mansur and Campos-Velho, 1990; Ricci et al., 1990; Semenas et al., 1994; Mansur and Silva, 1999; Viozzi and Brugni, 2001; Beasley et al., 2005; Pimpão et al., 2012), making the glochidia of H. sp. ‘Diamond Creek’ perhaps the largest among the Hyriidae.
Small glochidia that either lack hooks or have short hooks are typically gill parasites (Hoggarth and Gaunt, 1988; Araujo and Ramos, 1998; Bauer, 2001; Barnhart et al., 2008; Haag, 2012). The position of the adductor muscle in such glochidia results in long resistance arms, adapted for a large area of sweep that aids in attachment to host gill tissue (Hoggarth and Gaunt, 1988). In comparison, larger glochidia with longer hooks tend to parasitize the fins or epidermis of their hosts and are adapted for stronger gripping strength with a hook (or “larval tooth”), a large adductor muscle and depressed shell (Hoggarth and Gaunt, 1988; Bauer, 2001; Barnhart et al., 2008; Haag, 2012). Small glochidia that parasitise gills of their host fishes have also been shown to metamorphose to their juvenile form and grow considerably in size while still on the host (Araujo and Ramos, 1998; Bauer, 2001), whereas larger, hooked glochidia that typically parasitise fins and epithelia tend not to grow while on their host (Bauer, 2001). This is corroborated in Australasian studies of glochidial parasitism. The small, short-hooked glochidia of C. novaehollandiae and E. aucklandica parasitise gills of their hosts, grow while on the fish and are attached to their host for a considerable period (Jones, 2014; Melchior et al., 2021b). Indeed, Melchior et al. (2021b) showed that E. aucklandica larvae increased in size from 99.5 ± 4.7 µm SD to 449.2 ± 28.2 µm SD during metamorphosis while encysted on New Zealand Common Smelt R. retropinna, a quadrupling in size during the parasitic mussel stage. Similarly, C. novaehollandiae undergoes a considerable increase in size while attached to host fishes (Jones, 2014). In contrast, glochidia with larger shells that are equipped with larger hooks, e.g., A. profuga, A. jacksoni, E. menziesii, H. drapeta, V. angasi, V. ambiguus and W. carteri, do not appear to grow much on the host, if at all, and may be largely phoretic (Walker, 1981; Humphrey and Simpson, 1985; Widarto, 1993; Klunzinger et al., 2012; Jones, 2014; Melchior et al., 2021b; Melchior, 2021).
Most of the glochidia from species in this study are typical of those from other Hyriidae (i.e., South American Hyrrinae), in having shells with a subtriangular outline. Hyridella glenelgensis, H. narracanensis and to a lesser extent, C. novaehollandiae, are atypically round and more ‘horseshoe’ or nearly D-shaped. Cucumerunio novaehollandiae bears some resemblance in outline to South American glochidia of C. ambigua (Pimpão et al., 2012), albeit with much smaller glochidial shells. Although glochidia of C. novaehollandiae appear more rounded than most other hyriid glochidia, with a non-protruding ventral apex, their shells still come to a narrower point along the ventral edge than the wider hinge line, unlike D-shaped or horseshoe shaped glochidia such as North American Lampsilis or Quadrula species (Surber, 1912), for example, that have a much broader ventral edge, wider than their hinge line, giving a characteristic D-shape or horseshoe shape.
The soft anatomy of Australasian hyriid glochidia has not been examined in detail, but Jupiter and Byrne (1997), Percival (1931), and Parodiz and Bonetto (1963) have hinted at anatomical variation among species. The preservation techniques used in this study focused on glochidial shell examination for detailed SEM photography of shell structures. However, some preserved specimens show sensory hair arrangements and other soft anatomy in H. sp. ‘Diamond Creek’ and H. australis (see Supplementary Material), while a live image of V. angasi collected at the time of glochidial release shows sensory hair arrangements like those illustrated by Parodiz and Bonetto (1963). It would appear from the few images or illustrations available that V. ambiguus and V. angasi have sensory hairs located toward the dorsal portion of the shell, more basally to the adductor muscle, whereas H. australis and H. depressa have series of sensory hairs in several locations from just behind the larval hooks as well as medially and basally to the adductor muscle. The arrangement of sensory hairs may be a function of different attachment mechanism in gill versus fin parasitism (Hoggarth and Gaunt, 1988; Nivischenko et al., 2022).
4.3 Values and limitations of glochidial diversity in phylogenetic relationships
Although glochidial morphology and metrics of shell characters have some value as a tool to help identify some taxa (e.g., Kennedy and Haag, 2005; Pimpão et al., 2012), and indeed have been used for taxonomic re-arrangements in some instances (e.g., Pimpão et al., 2012; Pfeiffer and Graf, 2015; Cruz and Quesada, 2017; Lopes-Lima et al., 2018; Miyahira et al., 2019; Chernyshev et al., 2020), they are not always sufficiently unique within species or among taxonomic groups, as this study has demonstrated. Yet, this study also showed that for most taxa examined, certain characteristics were common at the subfamily and tribal level. For instance, larval hook bifurcation is a trait that appears to be restricted exclusively to the Hyridellini (C. novaehollandiae, H. australis, H. depressa, H. drapeta, H. glenelgensis and H. narracanensis). This is not yet fully resolved, however, given that glochidia of Hyridella guppyi (Smith, 1885), Hyridella misoolensis (Schepman, 1897) and Virgus beccarianus (Tapparone Canefri, 1883) have not yet been examined. Additionally, if H. sp. ‘Diamond Creek’ proves to be a member of the Hyridellini, it would be the only exception to this characteristic given its very different hook to other Hyridellini.
Glochidia of the vast majority Velesunioninae (A. jacksoni, A. pertexta pertexta, L. froggatti, V. ambiguus, V. angasi, W. albertisi and W. carteri) have a larval filament and subtriangular scalene shells of a relatively similar size, although A. profuga is the exception in being smaller and lacking a larval filament. In terms of hook morphology, most velesunionines have long, sigmoidal singularly pointed, fluted hooks whereas W. carteri has a complex hook structure. The glochidia of W. carteri are also much more scalene in shape and larger than the other glochidia within the subfamily. Again, however, glochidial characteristics are not yet fully resolved for the subfamily given that glochidia of additional taxa within the Velesunioninae remain to be examined (see note in provisional glochidia key below).
As for the New Zealand Echyridella, glochidia of the two species included in this study exhibit wide differences in several characters including size, shape, hook length, presence or absence of shell sculpturing, and the presence or absence of a larval filament. Both, however, have spinose hooks with wide buttressed supports. The glochidia of the third New Zealand hyriid, Echyridella onekaka Fenwick and Marshall, 2006, remains unknown. In a practical sense, identifying glochidia on host fishes could be problematic for L. froggatti and Velesunio species, A. jacksoni and V. ambiguus, and A. pertexta and V. ambiguus groupings, that may be sympatric in distribution and may produce glochidia around the same time of year (Walker, 1981; Humphrey and Simpson, 1985; Jones et al., 1986; Klunzinger, 2020). However, where LDA failed to accurately predict some species on glochidial shell metrics, in most cases this would be a moot point. For instance, where W. carteri was mistaken for E. menziesii or L. froggatti for A. profuga and vice versa, this would be irrelevant given these species are found in widely separate geographical ranges. Where uncertainty is an issue, genetic barcoding of glochidia on host fishes has proven useful for resolving such uncertainty (Kneeland and Rhymer, 2007; Boyer et al., 2011; Zieritz et al., 2012, 2018). Thus, using glochidial characteristics in species identification must be treated with caution.
5 Conclusion
(1) Of the 29 extant species of Australasian Hyriidae, glochidia are now described from 17 species (58.6%), five of which (17.2%) are described in this study for the first time.
(2) In combination with other published studies covered in the discussion, this study has added to the diversity of glochidia and mechanisms of release among Australasian freshwater mussels and this will be beneficial in helping to understand host fish use, dispersal mechanisms and designing captive breeding technologies in future work.
(3) Through statistical comparative analyses, this study highlights the utility of glochidial morphological diversity as an important aspect of systematics that, when coupled with genetic analyses, is likely to aid in modernizing the taxonomy of the Australasian Hyriidae. However, as statistical analyses showed in this study, reliance on glochidial characters alone for freshwater mussel systematics is not recommended, even though it revealed similarities and differences among and between taxonomic groups. Examining yet unknown Australasian hyriid glochidia and integrating glochidial characters with adult and juvenile morphology and molecular phylogenetic techniques will be a logical next step for modernizing the taxonomy of the Australasian Hyriidae and indeed for the Unionoida as a whole.
(4) Different glochidial release mechanisms were found between the Hyridellini and Velesunioninae. While this distinction appears to be related to different riverine typologies, the evolutionary significance remains to be fully explored among and between taxa.
Provisional key to the glochidia of Australasian Hyriidae
1. Glochidia small, length <100 μm; larval thread absent—2
- Glochidia large, length >100 μm, larval thread absent—4
2(1). Glochidial height/length ratio >105%; hooks unicuspid
…………………………………. .Echyridella aucklandica.
- Glochidial height/length ratio <105%; hooks bicuspid—3
3(2). Glochidial shape suborbicular; hooks reduced to a pair of spinose cusps set on a broad base (larval hook length <10 µm) ……………………………. .Cucumerunio novaehollandiae
- Glochidial shape subtriangular; hooks conjoined and arching (larval hook length >10 µm) ………………Hyridella australis
4(1). Glochidial shape suborbicular, larval hook reduced, length <20 μm; larval thread absent—5
- Glochidial shape subtriangular; larval hook length long, >20 μm; larval thread present or absent—6
5(4). Glochidial length <253 μm, height <230 µm and size <240 µm
…………………………………. ..Hyridella narracanensis
- Glochidial length >253 μm, height >230 µm and size >240 µm
……………………………………. ..Hyridella glenelgensis
6(4). Larval hook widely buttressed; larval thread present ………………………………………. ..Echyridella menziesii
- Hook not widely buttressed; larval thread present or absent—7
7(6). Glochidial length <300 μm; larval thread present—8
- Glochidial length <300 μm; larval thread present or absent—9
8(7). Hinge length >220 μm; larval hook length >60 µm and cusps tripartite Hyridella sp. ‘Diamond Creek’
- Hinge length <220 μm; larval hook length <60 µm …………………………………………. Westralunio carteri
9(7). Larval hook bicuspid; larval thread absent—10
- Larval hook unicuspid; larval thread present or absent—11
10(9). Larval hook length >58 µm …………Hyridella drapeta
- Larval hook length <58 µm …………………Hyridella depressa
11(9). Glochidial height/length ratio <86%; larval thread present or absent—12
- Glochidial height/length ratio >86%; larval thread present—13
12(11). Larval hook length >50 μm; larval thread present …………………………………………Velesunio ambiguus
- Larval hook length <50 μm; Larval thread absent ……………………………………………. Alathyria profuga
13(11). Larval hook length >60 µm—14
- Larval hook length <60 µm—16
14(13). Glochidial height <235 µm ………. .. Lortiella froggatti
- Glochidial height >235 µm—15
15(14). Larval hook length <60 µm ………Alathyria jacksoni
- Larval hook length >60 µm …………………………………. .. Alathyria pertexta pertexta
16(13). Larval hook length <52 μm; mean Á <15° …………………………………………….Velesunio angasi
- Larval hook length >52 μm; mean Á >15° …………………………………………. Westralunio albertisi
Note: the following taxa of Australasian Hyriidae are excluded from this identification guide because no information on their glochidia is currently available:
Hyriinae: Hyridellini
Hyridella misoolensis (Schepman, 1897).
Hyridella guppyi guppyi (Smith, 1885).
Hyridella guppyi aipiana McMichael, 1956.
Hyridella sp. Jones et al., unpublished data (see Jones et al., 1986).
Hyridella sp. ‘Southwest Victoria’ Raadik et al., unpublished data
Velesunioninae
Velesunio wilsonii (Lea, 1859).
Velesunio sentaniensis (Haas, 1924).
Velesunio sp. B (see Baker et al., 2004).
Velesunio sp. D (see Baker et al., 2004).
Velesunio sp. ‘Magela Creek’ (see Kleinhenz et al., 2019).
Microdontia anodontaeformis Tapparone Canefri, 1883.
Alathyria pertexta wardi (Iredale, 1943a).
Alathyria pertexta magnifica McMichael and Hiscock, 1958.
Alathyria condola Iredale, 1943b.
Westralunio flyensis (Tapparone Canefri, 1883).
Westralunio inbisi inbisi Klunzinger, Whisson, Zieritz, Benson, Stewart & Kirkendale, 2022.
Westralunio inbisi meridiemus Klunzinger, Whisson, Zieritz, Benson, Stewart & Kirkendale, 2022.
Lortiella rugata (Sowerby, 1868).
Lortiella opertanea Ponder and Bayer, 2004.
Subfamily incertae sedis
Echyridella onekaka Fenwick and Marhsall, 2006.
Data availability statement
The original contributions presented in the study are included in the article/Supplementary Material, further inquiries can be directed to the corresponding author.
Ethics statement
The manuscript presents research on animals that do not require ethical approval for their study.
Author contributions
MK: Conceptualization, Data curation, Formal Analysis, Investigation, Methodology, Project administration, Resources, Software, Supervision, Validation, Visualization, Writing–original draft, Writing–review and editing. HJ: Conceptualization, Data curation, Formal Analysis, Investigation, Methodology, Software, Visualization, Writing–original draft, Writing–review and editing, Validation. CH: Conceptualization, Data curation, Investigation, Methodology, Resources, Validation, Visualization, Writing–review and editing. MM: Conceptualization, Data curation, Investigation, Methodology, Resources, Validation, Visualization, Writing–review and editing. TR: Conceptualization, Data curation, Investigation, Methodology, Resources, Validation, Visualization, Writing–review and editing. ST: Investigation, Visualization, Writing–review and editing. LC: Investigation, Resources, Writing–review and editing, Visualization. FS: Conceptualization, Funding acquisition, Investigation, Methodology, Project administration, Resources, Supervision, Validation, Writing–review and editing.
Funding
The author(s) declare financial support was received for the research, authorship, and/or publication of this article. This research was funded by Griffith University.
Acknowledgments
This study was presented at the joint meeting of the Australian Freshwater Sciences Society, the New Zealand Freshwater Sciences Society, and the Society for Freshwater Science held in Brisbane, Queensland, Australia 3–7 June 2023 (Klunzinger et al., 2023). The authors thank the reviewers and the editor whose comments and suggestions greatly improved the manuscript. We thank Erica Lovas, Candice Goodwin, Nicole Scheiber, Jennifer Brown and Ron Rasch of the University of Queensland Centre for Microscopy and Microanalysis and Lisa Kirkendale, Corey Whisson and Janelle Ritchie of the Western Australian Museum for technical support in the use and access to scanning electron microscope laboratories. We thank Timothy Fernando from Arthur Rylah Institute for Environmental Research, Department of Energy, Environment, and Climate Action (Heidelberg, Victoria) for generously providing images of recently released mesoconglutinates of Hyridella glenelgensis during a captive rearing trial in September 2023.
Conflict of interest
This study was conducted in a manner free from any activity that could be construed as a conflict of interest.
The remaining authors declare that the research was conducted in the absence of any commercial or financial relationships that could be construed as a potential conflict of interest.
The author(s) declared that they were an editorial board member of Frontiers, at the time of submission. This had no impact on the peer review process and the final decision.
Publisher’s note
All claims expressed in this article are solely those of the authors and do not necessarily represent those of their affiliated organizations, or those of the publisher, the editors and the reviewers. Any product that may be evaluated in this article, or claim that may be made by its manufacturer, is not guaranteed or endorsed by the publisher.
Supplementary material
The Supplementary Material for this article can be found online at: https://www.frontiersin.org/articles/10.3389/fenvs.2023.1305077/full#supplementary-material
References
Abdi, H., and Williams, L. J. (2012). “Correspondence analysis,” in Encyclopedia of research design. Editor N. J. Salkind (Thousand Oaks, California: SAGE Publications, Inc.), 37.
Aldridge, D. C., Ollard, I. S., Bespalaya, Y. V., Bolotov, I. N., Douda, K., Geist, J., et al. (2022). Freshwater mussel conservation: a global horizon scan of emerging threats and opportunities. Glob. Change Biol. 29, 575–589. doi:10.1111/gcb.16510
Alvarenga, L. C. F., and Ricci, C. (1977). Contribuição ao conhecimento dos gloquídios do gênero Diplodon Spix, 1827: D. besckeanus (Dunker, 1849) (Bivalvia: Unionoidea: Hyriidae). An. do V. Encontro de Malacol. Bras., 33–38.
Araujo, R., Bragado, D., and Ramos, M. A. (2000). Occurrence of glochidia of the endangered Margaritifera auricularia (Spengler, 1793) and other mussel species (Bivalvia: Unionoida) in drift and on fishes in an ancient channel of the Ebro River, Spain. Arch. Hydrobiol. 148, 147–160. doi:10.1127/archiv-hydrobiol/148/2000/147
Araujo, R., and Ramos, M. A. (1998). Description of the glochidium of Margaritifera auricularia (spengler 1793) (Bivalvia, Unionoidea). Philos. Trans. Roy. Soc. Lon. B 353, 1553–1559. doi:10.1098/rstb.1998.0309
Atkins, L. (1979). Observations on the glochidial stage of the freshwater mussel Hyridella (Hyridella) drapeta (Iredale) (Mollusca: pelecypoda). Aust. J. Mar. Freshw. Res. 30, 411–416. doi:10.1071/MF9790411
Baird, M. (2000). Life history and population structure of the spectaclecase mussel, Cumberlandia monodonta (Bivalvia, Margaritiferidae). Springfield (MO): Missouri State University. [Master’s thesis].
Baker, F. (1913). The land and fresh-water mollusks of the Stanford Expedition to Brazil. P. Acad. Nat. Sci. Phila. 65, 618–672.
Baker, A. M., Sheldon, F., Somerville, J., Walker, K. F., and Hughes, J. M. (2004). Mitochondrial DNA phylogenetic structuring suggests similarity between two morphologically plastic genera of Australian freshwater mussels (Unionoida: Hyriidae). Mol. Phylogenet. Evol. 32, 902–912. doi:10.1016/j.ympev.2004.02.017
Barnhart, C., and Roston, B. (2005). “Host infection strategy of the snuffbox mussel, Epioblasma triquetra,” in Fourth biennial symposium, Freshwater Mollusk Conservation Society, Conference Proceedings, St. Paul, Minnesota, November 2005, 43.
Barnhart, M. C., Haag, W. R., and Roston, W. N. (2008). Adaptations to host infection and larval parasitism in Unionoida. J. N. Am. Benthol. Soc. 27, 370–394. doi:10.1899/07-093.1
Bauer, G. (1994). The adaptive value of offspring size among freshwater mussels (Bivalvia: Unionoidea). J. Anim. Ecol. 63, 933–944. doi:10.2307/5270
Bauer, G. (2001). “Life-history variation on different taxonomic levels of Naiads,” in Ecology and evolution of the freshwater mussels Unionoida. Editors G. Bauer, and K. Wächtler (Berlin: Springer), 83–84.
Beasley, C. R., Miranda, L. Q., Alves, S. T. M., Melo, A. G., Souza, J. O., and Tagliaro, C. H. (2005). Brood size and larval length of paxyodon syrmatophorus (Bivalvia, Hyriidae) from the tocantins river, Brazil. Amazoniana 18, 173–184.
Benedict, A., and Geist, J. (2021). Effects of water temperature on glochidium viability of Unio crassus and Sinanodonta woodiana: implications for conservation, management and captive breeding. J. Mollus. Stud. 87, eyab011. doi:10.1093/mollus/eyab011
Bogan, A. E. (2008). Global diversity of freshwater mussels (Mollusca, Bivalvia) in freshwater. Hydrobiologia 595, 139–147. doi:10.1007/s10750-007-9011-7
Bogan, A. E., and Roe, K. J. (2008). Freshwater bivalve (Unioniformes) diversity, systematics, and evolution: status and future directions. J. N. Am. Benthol. Soc. 27, 349–369. doi:10.1899/07-069.1
Bonetto, A. A. (1951). Acerca de las formas larvales de Mutelidae Ortmann. Jornadas Icticas 1 (1). Dir. Gral. Inv. Fom. Agr. Gan. Santa Fe, Argentina.
Bonetto, A. A. (1959). “Contribución al conocimiento de las glochidias del género Diplodon y su aplicación a los estudios sistemáticos,” in 1er. Congreso sudamericano de Zoología. Tomo II (invertebrados) (La Plata, Argentina, 43–59.
Bonetto, A. A. (1961). Investigaciones acerca de las formas larvales en el genero “Diplodon” y su aplicacion a los studios sistematicos. Santa Fé: Direccion General de Recursos Naturales – Publicación Técnica.
Bonetto, A. A., and Ezcurra, I. D. (1963). Notas Malacológicas. I. (1) El Desarrollo del glochidium de Diplodon delodontus delodontus (Lam.); (2) El glochidium de Prisodon (triplodon) corrugatus Lam.; (3) El lasidium de Monocondylea paraguayana d’Orb. Physis 24, 17–22. X.
Bonetto, A. A., Tassara, M. P., and Rumi, A. (1986). Australis N. Subgen. De Diplodon Spix (Bivalvia, Unionacea) y posibles relaciones con Hyriidae Australianos. Bol. Soc. Biol. Concepción, Chile 57, 55–61.
Boyer, S. L., Howe, A. A., Juergens, N. W., and Hove, M. C. (2011). A DNA-barcoding approach to identifying juvenile freshwater mussels (Bivalvia:Unionidae) recovered from naturally infested fishes. J. N. Am. Benthol. Soc. 30, 182–194. doi:10.1899/10-004.1
Brainwood, M., Burgin, S., and Byrne, M. (2006). Is the decline of freshwater mussel populations in a regulated coastal river in south-eastern Australia linked with human modification of habitat? Aquat. Cons. 16, 501–516. doi:10.1002/aqc.758
Brainwood, M., Burgin, S., and Byrne, M. (2008a). The impact of small and large impoundments on freshwater mussel distribution in the Hawkesbury–Nepean River, southeastern Australia. River Res. Appl. 24, 1325–1342. doi:10.1002/rra.1087
Brainwood, M., Burgin, S., and Byrne, M. (2008b). The role of geomorphology in substratum patch selection by freshwater mussels in the Hawkesbury–Nepean River (New South Wales) Australia. Aquat. Cons. 18, 1285–1301. doi:10.1002/aqc.949
Brown, R. L., Clearwater, S. J., Thompson, K. J., Martin, M. L., and Jellyman, P. G. (2017). “Comparison of host fish suitability for larvae (glochidia) of the native freshwater mussel, Echyridella menziesii,” in Poster presentation to the Integrating Multiple Aquatic Values, 5th Biennial Symposium of the International Society for River Science, Hamilton, New Zealand, 19–24 November 2017.
Byrne, M. (1998). Reproduction of river and lake populations of Hyridella depressa (Unionacea: Hyriidae) in New South Wales: implications for their conservation. Hydrobiologia 389, 29–43. doi:10.1023/a:1003528431775
G. Bauer, and K. Wächtler (Editors) (2001). Ecology and evolution of the freshwater mussels Unionoida (Berlin: Springer).
Carter, J. G., Harries, P. J., Malchus, N., Sartori, A. F., Anderson, L. C., Bieler, R., et al. (2012). Part N, revised, volume 1, chapter 31: illustrated glossary of the Bivalvia. Treatise Online 48, 1–209.
Chernyshev, A. V., Sayenko, E. M., and Bogatov, V. V. (2020). Superspecific taxonomy of the far eastern unionids (Bivalvia, Unionidae): review and analysis. Biol. Bull. 47, 267–275. doi:10.1134/S1062359020010045
Clearwater, S. J., Thompson, K. J., and Hickey, C. W. (2014). Acute toxicity of copper, zinc, and ammonia to larvae (Glochidia) of a native freshwater mussel Echyridella menziesii in New Zealand. Arch. Environ. Con. Tox. 66, 213–226. doi:10.1007/s00244-013-9972-7
Conrad, T. A. (1834). New fresh water Shells of the united States with coloured Illustrations and a Monograph of the genus anculotus of say; also A Synopsis of the American naiades. Philadelphia: E.G. Dorsey.
Cotton, B. C., and Gabriel, C. J. (1932). Australian Unionidae. Proc. R. Soc. Vict. New. ser.) 44, 155–161.
Cruz, R. A., and Quesada, R. (2017). Taxonomic position and glochidia from the freshwater mussel, Anodonta luteola (Unionoida: Unionidae), in Costa Rica. Cuad. Invest. UNED 9, 39–43. doi:10.22458/urj.v9i1.1676
Dennant, J. (1898). Description of a new species of Unio from the river glenelg. P. Roy. Soc. Vict. 10, 112–113.
d’Orbigny, A. (1835). Synopsis terrestrium et fluviatilium molluscorum, in suo per Americam meridionalem itinere. Mag. Zool. 5, 1–44.
Fenwick, M. C., and Marshall, B. A. (2006). A new species of Echyridella from New Zealand, and recognition of Echyridella lucasi (Suter, 1905) (Mollusca: Bivalvia: Hyriidae). Molluscan Res. 26, 69–76.
Ferreira-Rodríguez, N., Akiyama, Y. B., Aksenova, O. V., Araujo, R., Barnhart, M. C., Bespalaya, Y. V., et al. (2019). Research priorities for freshwater mussel conservation assessment. Biol. Cons. 231, 77–87. doi:10.1016/j.biocon.2019.01.002
Geist, J., Thielen, F., Lavictoire, L., Hoess, R., Altmueller, R., Baudrimont, M., et al. (2023). Captive breeding of European freshwater mussels as aconservation tool: a review. Aquat. Conserv. 33, 1321–1359. doi:10.1002/aqc.4018
Gould, A. A. (1851). Shells from the United States exploring expedition. Proc. Boston Soc. Nat. Hist. 3, 292–296.
Graf, D. L., and Cummings, K. S. (2007). Review of the systematics and global diversity of freshwater mussel species (Bivalvia: Unionoida). J. Molus. Stud. 73, 291–314. doi:10.1093/mollus/eym029
Graf, D. L., and Cummings, K. S. (2021). A ‘big data’ approach to global freshwater mussel diversity (Bivalvia: Unionoida), with an updated checklist of genera and species. J. Mollus. Stud. 87, 1–36. eyaa034. doi:10.1093/mollus/eyaa034
Graf, D. L., and Cummings, K. S. (2023). The Freshwater Mussels (Unionoida) of the World (and other less consequential bivalves). MUSSEL Project Web Site Availabet at: http://www.mussel-project.net/ (Accessed November 11, 2023).
Graf, D. L., Jones, H. A., Geneva, A. J., Pfeiffer, J. M., and Klunzinger, M. W. (2015). Molecular phylogenetic analysis supports a Gondwanan origin of the Hyriidae (Mollusca: Bivalvia: Unionida) and the paraphyly of Australasian taxa. Mol. Phylogenet. Evol. 85, 1–9. doi:10.1016/j.ympev.2015.01.012
Gray, J. E. (1843). “Catalogue of the species of Mollusca and their shells, which have hitherto been recorded as found at New Zealand, with the description of some lately discovered species. Appendix 4,” in Travels in New Zealand; with contributions to the geography, geology, botany, and natural history of that country 2. Editor E. Dieffenbach (London: Murray), 228–265.
Haag, W. R., and Warren, M. L. (1998). Role of ecological factors and reproductive strategies in structuring freshwater mussel communities. Can. J. Fish. Aquat. Sci. 55, 297–306. doi:10.1139/f97-210
Haag, W. R., and Warren, M. L. (2003). Host fishes and infection strategies of freshwater mussels in large Mobile Basin streams, USA. J. N. Am. Benthol. Soc. 22, 78–91. doi:10.2307/1467979
Haas, F. (1924). Unsere bisherigen kenntnisse der Najadenfauna neu-guineas. Nova Guin. 15 (Zool), 65–76.
Hanrahan, N. J. (2019). Field and laboratory investigations of Echyridella menziesii (Unionida: Hyriidae) interactions with host fishes. The University of Waikato. [MSc(Research) thesis] [Hamilton (New Zealand)] https://hdl.handle.net/10289/12970.
Harms, W. (1909). Postembryonale entwicklungsgeschichte der Unioniden. Zool. Jahrb. Abt. Anat. Ont. Tiere 28, 325–386.
Hastie, L. C., and Young, M. R. (2003). Timing of spawning and glochidia release in Scottish freshwater pearl mussel (Margaritifera margaritifera) populations. Freshw. Biol. 48, 2107–2117. doi:10.1046/j.1365-2427.2003.01153.x
Hastie, L. C., Young, M. R., and Boon, P. J. (2000). Growth characteristics of freshwater pearl mussels, Margaritifera margaritifera (L.). Freshw. Biol. 43, 243–256. doi:10.1046/j.1365-2427.2000.00544.x
Heard, W. H., and Guckert, R. H. (1970). A re-evaluation of the recent unionacea (pelecypoda) of North America. Malacologia 10, 333–335.
Hewitt, T. L., Wood, C. L., and Ó Foighil, D. (2019). Ecological correlates and phylogenetic signal of host use in North American unionid mussels. Int. J. Parasitol. 29, 71–81. doi:10.1016/j.ijpara.2018.09.006
Hewitt, T. L., Haponski, A. E., and Ó Foighil, D. (2021). Evolution of diverse host infection mechanisms delineates an adaptive radiation of lampsiline freshwater mussels centered on their larval ecology. PeerJ 9, 28, e12287. doi:10.7717/peerj.12287
Hoggarth, M. A. (1999). Descriptions of some glochidia of the Unionidae (Mollusca: Bivalvia). Malacologia 41, 1–118.
Hoggarth, M. A., and Gaunt, A. S. (1988). Mechanics of glochidial attachment (Mollusca: Bivalvia: Unionidae). J. Morphol. 198, 71–81. doi:10.1002/jmor.1051980108
Huber, V., and Geist, J. (2019). Reproduction success of the invasive Sinanodonta woodiana (Lea, 1834) in relation to native mussel species. Biol. Invasions 21, 3451–3465. doi:10.1007/s10530-019-02060-3
Humphrey, C. L., and Simpson, R. D. (1985). Biology and ecology of the freshwater mussel Velesunio angasi (Bivalvia: Hyriidae) in the Magela Creek, northern territory. Open file record 38. Canberra: Supervising Scientist Branch. Available at: https://www.environment.gov.au/science/supervising-scientist/publications/open-file-record/biology-and-ecology-velesunio-angasi.
W. R. Haag (Editor) (2012). North American freshwater mussels: natural history, ecology, and conservation. (New York: Cambridge University Press).
Ieshko, E. P., Veselob, A., Murzina, S., Da, E., Ma, R., and Zotin, A. A. (2014). Freshwater pearl mussel Margaritifera margaritifera L. in Syuskyuyan Loki (Ladoga’s lake basin). P. Kar. Sci. Cent. Russ. Acad. Sci. 6, 122–130.
Ihering, H. V. (1893). Najaden von S. Paulo und die geographische Verbreitung der Süsswasser-Faunen von Südamerika. Arch. Naturg. 59, 45–140.
Iredale, T. (1943a). A basic list of the fresh-water Mollusca of Australia. Aust. Zool. 10, 188–230.
Iredale, T. (1943b). Guide to the freshwater shells of New South Wales. Part I. Aust. Nat. 11, 85–95.
Jenyns, L. (1842). “Part 4 fishes,” in The zoology of the voyage of H.M.S. Beagle under the command of captain Fitzroy, R.N. During the years 1832 to 1836. Editor C. Darwin (London: Smith, Elder & Co.). 97-172, pls 21-29.
Jones, H. A. (2006). “The influence of hydrology on freshwater mussel (Bivalvia: Hyriidae) distributions in a semi-arid river system, the Barwon-Darling River and intersecting streams,” in Animals of arid Australia: out on their own? Editors C. Dickman, D. Lunney, and S. Burgin (Mossman, NSW, Australia: Royal Zoological Society of New South Wales), 132–142.
Jones, H. A. (2014). Landscape scale impacts on freshwater mussel (Unionoida: Hyriidae) distribution and status in southeastern Australia. Sydney (New South Wales): University of Sydney. [PhD thesis] Available from: http://hdl.handle.net/2123/10110.
Jones, H. A., and Byrne, M. (2010). The impact of catastrophic channel change on freshwater mussels in the Hunter River system, Australia: a conservation assessment. Aquat. Conserv. 20, 18–30. doi:10.1002/aqc.1080
Jones, H. A., and Byrne, M. (2014). Changes in the distributions of freshwater mussels (Unionoida: Hyriidae) in coastal south-eastern Australia and implications for their conservation status. Aquat. Cons. 24, 203–217. doi:10.1002/aqc.2402
Jones, H. A., Simpson, R. D., and Humphrey, C. L. (1986). The reproductive cycles and glochidia of freshwater mussels (Bivalvia: Hyriidae) of the Macleay River, northern New South Wales, Australia. Malacologia 27, 185–202.
Jupiter, S. D., and Byrne, M. (1997). Light and scanning electron microscopy of the embryos and glochidia larvae of the Australian freshwater bivalve Hyridella depressa (Hyriidae). Invertebr. Reprod. Dev. 32, 177–186. doi:10.1080/07924259.1997.9672620
Kat, P. W. (1984). Parasitism and the unionacea (Bivalvia). Biol. Rev. Camb. Philos. 59, 189–207. doi:10.1111/j.1469-185x.1984.tb00407.x
Kennedy, T. B., and Haag, W. R. (2005). Using morphometrics to identify glochidia from a diverse freshwater mussel community. J. N. Am. Benthol. Soc. 24, 880–889. doi:10.1899/05-001.1
Kleinhenz, L. S., Humphrey, C. L., Mooney, T. M., Trenfield, M. A., van Dam, R. A., Nugegoda, D., et al. (2019). Chronic ammonia toxicity to juveniles of two tropical Australian freshwater mussels (Velesunio spp.): toxicity test optimization and implications for water quality guideline values. Environ. Toxicol. Chem. 38, 841–851. doi:10.1002/etc.4370
Klunzinger, M. W. (2020). Description of the glochidia of Alathyria pertexta pertexta Iredale, 1934 (Bivalvia: Hyriidae) from Southsouth-eastern Queensland. Aust. J. Zool. 67, 1–8. doi:10.1071/ZO19056
Klunzinger, M. W. (2023a). Description of the glochidia of Westralunio albertisi Clench, 1957 (Bivalvia: Unionida: Hyriidae) from the fly River system, Papua New Guinea. Molluscan Res. 43, 96–100. doi:10.1080/13235818.2023.2203075
Klunzinger, M. W. (2023b). Conservation status of freshwater mussels in Australia – new species, new listings, and work to come. Tentacle 31, 3–5.
Klunzinger, M. W., Beatty, S. J., Morgan, D. L., Lymbery, A. J., and Thomson, G. J. (2012). Glochidia ecology in wild fish populations and laboratory determination of competent host fishes for an endemic freshwater mussel of south-western Australia. Aust. J. Zool. 60, 26–36. doi:10.1071/ZO12022
Klunzinger, M. W., Beatty, S. J., Morgan, D. L., Pinder, A. M., and Lymbery, A. J. (2015). Range decline and conservation status of Westralunio carteri Iredale, 1934 (Bivalvia: Hyriidae) from south-western Australia. Aust. J. Zool. 63, 127–135. doi:10.1071/ZO15002
Klunzinger, M. W., Beatty, S. J., Morgan, D. L., Thomson, G. J., and Lymbery, A. J. (2013). Morphological and morphometrical description of the glochidia of Westralunio carteri Iredale, 1934 (Bivalvia: Unionoida: Hyriidae). Molluscan Res. 33, 104–109. doi:10.1080/13235818.2013.782791
Klunzinger, M. W., Whisson, C., Zieritz, A., Benson, J. A., Stewart, B. A., and Kirkendale, L. (2022). Integrated taxonomy reveals new threatened freshwater mussels (Bivalvia: Hyriidae: Westralunio) from southwestern Australia. Sci. Rep. 12, 20, 20385. doi:10.1038/s41598-022-24767-5
Klunzinger, M. W., Jones, H. A., Melchior, M., Raadik, T., Treby, S., and Sheldon, F. (2023). “Comparative diversity in glochidia of Australasian freshwater mussels and its value for taxonomy and conservation,” in Freshwater Sciences 2023, Joint meeting of the Australian Freshwater Sciences Society, New Zealand Freshwater Sciences Society and Society for Freshwater Science, Brisbane, Queensland, Australia, 3-7 June, 2023. Abstract # 463.
Kneeland, S. C., and Rhymer, J. M. (2007). A molecular identification key for freshwater mussel glochidia encysted on naturally parasitized fish hosts in Maine, USA. J. Mollus. Stud. 73, 279–282. doi:10.1093/mollus/eym017
Lamarck, J. B. P. (1819). Histoire naturelle des animaux sans vertèbres. Paris: Tome 6, lère partie.
Lê, S., Josse, J., and Husson, F. (2008). FactoMineR: an R package for multivariate analysis. J. Stat. Softw. 25, 1–18. doi:10.18637/jss.v025.i01
Lea, I. (1831). Observations on the Naïades, and descriptions of new species of that and other families. Trans. Am. Philos. Soc. 4, 63–121. doi:10.2307/1004831
Lea, I. (1859). Descriptions of twenty-one new species of exotic Unionidae. Proc. Acad. Nat. Sci. Philad. 11, 151–154.
Lillie, F. R. (1895). The embryology of the Unionidae. A study in cell-lineage. J. Morphol. 10, 1–100. doi:10.1002/jmor.1050100102
Linnaeus, C. (1758). Systema Naturae per regna tria naturae, secundum classes, ordines, genera, species, cum characteribus, (differentiis, synonymis, locis 1: 823, emendanda).
Lopes-Lima, M., Bolotov, I. N. D., Aldridge, V. T., Fonseca, D. C., Gan, M. M., Gan, H. M., et al. (2018). Expansion and systematics redefinition of the most threatened freshwater mussel family, the Margaritiferidae. Mol. Phylogenet. Evol. 127, 98–118. doi:10.1016/j.ympev.2018.04.041
Mansur, M. C. D. (1999). Gloquídio de Diplodon martensi (Ihering) (Mollusca, Bivalvia, Hyriidae) e seu ciclo parasitário. Rev. Bras. Zool. 16, 185–194. Supl. 2. doi:10.1590/s0101-81751999000600019
Mansur, M. C. D., and Campos-Velho, N. M. R. (1990). Técnicas para o estudo dos gloquídios de Hyriidae (Mollusca, Bivalvia, Unionoida). Acta Biol. leop. 12, 5–18.
Mansur, M. C. D., and Silva, M. G. O. (1999). Description of glochidia of five species of freshwater mussels (Hyriidae: Unionoidea) from South America. Malacologia 41, 475–483.
Marshall, B. A., Fenwick, M. C., and Ritchie, P. A. (2014). New Zealand recent Hyriidae (Mollusca: Bivalvia: Unionida). Molluscan Res. 34, 181–200. doi:10.1080/13235818.2014.889591
Matteson, M. R. (1948). Life history of Elliptio companatus (dillwyn, 1817). Am. Midl. Nat. 40, 690–723. doi:10.2307/2421491
McMichael, D. F., and Hiscock, I. D. (1958). A monograph of the freshwater mussels (Mollusca: pelecypoda) of the Australian Region. Aust. J. Mar. Fresh. Res. 9, 372–507. doi:10.1071/MF9580372
Melchior, M. (2021). Partitioning along reproductive niche dimensions in sympatric New Zealand freshwater bivalve species. Hamilton, (New Zealand): The University of Waikato. [PhD thesis].
Melchior, M., Collier, K. J., and Clearwater, S. J. (2021a). First record of complex release strategies and morphometry of glochidia in sympatric Echyridella species (Bivalvia: Unionida: Hyriidae). Hydrobiologia 848, 3115–3126. doi:10.1007/s10750-019-03995-3
Melchior, M., Squires, N. J., Clearwater, S. J., and Collier, K. J. (2021b). Discovery of a host fish species for the threatened New Zealand freshwater mussel Echyridella aucklandica (Bivalvia: Unionida: Hyriidae). New zeal. J. Mar. fresh. 57, 152–159. doi:10.1080/00288330.2021.1963290
Middendorff, A. T. V. (1850). Beschreibung einiger neuer Mollusken-Arten, nebst einem Blicke auf den geographischen Charakter der Land-und Süsswasser-Mollusken Nord-Asiens. B. Cl. Phys. Ac. Imp. Sci. Pétersbg. 9, 108–112.
Miyahira, I. C., Barbosa dos Santos, S., and Mansur, M. C. D. (2017). Freshwater mussels from South America: state of the art of Unionida, specially Rhipidodontini. Biota Neotrop 17, 13, e20170341. doi:10.1590/1676-0611-BN-2017-0341
Miyahira, I. C., Mansur, M. C. D., and dos Santos, S. B. (2019). Redescription of Diplodon ellipticus spix in wagner, 1827, Diplodon multistriatus (lea, 1831), and Rhipidodonta garbei (ihering, 1910) (Bivalvia: Hyriidae) from coastal rivers of eastern and northeastern Brazil. Arch. Molluskenkd. 148, 9–34. doi:10.1127/arch.moll/148/009-034
Murphy, G. (1942). Relationship of the fresh-water mussel to trout in the Truckee River. Calif. Fish. Game 28, 89–102.
Nezlin, L. P., Cunjak, R. A., Zotin, A. A., and Ziuganov, V. V. (1994). Glochidium morphology of the freshwater pearl mussel (Margaritifera margaritifera) and glochidiosis of Atlantic salmon (Salmo salar): a study by scanning electron microscopy. Can. J. Zool. 72, 15–21. doi:10.1139/z94-003
Nilsson, S. (1822). Historia Molluscorum Sveciae Terrestrium et Fluviatilium Breviter Delineata. Lundae, Sumtibus J.H. Schubothii.
Nivischenko, V. E., Sayenko, E. M., and Dyachuk, V. A. (2022). First immunodetection of sensory and nervous systems of parasitic larvae (glochidia) of freshwater bivalve Nodularia douglasiae. Front. Physiol. 13, 17, 879540. doi:10.3389/fphys.2022.879540
O’Brien, C., Maine, A., Nez, D., and Box, J. B. (2019). A comparison of glochidial shells of the freshwater mussels Anodonta califoriniensis, Anodonta kennerlyi, Anodonta nuttalliana, and Anodonta oregonensis. Fresh. Moll. Biol. Cons. 22, 20–24. doi:10.31931/fmbc.v22i1.2019.20-24
Ortmann, A. E. (1921). South American naiades: a contribution to the knowledge of the freshwater mussels of South America. Mem. Carn. Mus. 8, 451–670/. doi:10.5962/p.234837
Parodiz, J. J., and Bonetto, A. A. (1963). Taxonomy and zoogeographic relationships of the South American naiades. Int. J. Malacol. 1, 179–213.
Pekkarinen, M., and Valovirta, I. (1996). Anatomy of the glochidia of the freshwater pearl mussel, Margaritifera margaritifera (L.). Arch. Hydrobiol. 137, 411–423. doi:10.1127/archiv-hydrobiol/137/1996/411
Percival, E. (1931). A note on the life history of Diplodon lutulentus Gould. Trans. P. R. Soc. New zeal. 62, 86–91.
Pfeiffer, J. M., and Graf, D. L. (2015). Evolution of bilaterally asymmetrical larvae in freshwater mussels (Bivalvia: Unionoida: Unionidae). Zool. J. Linn. Soc. 175, 307–318. doi:10.1111/zoj.12282
Philippi, R. A. (1847). Abblildungen und Beschreibungen neuer oder wenig gekannter Conchylien 3, 1–50.
Pimpão, D. M., Mansur, M. C. D., Bergonci, P. E. A., and Beasley, C. R. (2012). Comparative morphometry and morphology of glochidial shells of amazonian Hyriidae (Mollusca: Bivalvia: Unionida). Am. Malacol. Bull. 30, 73–84. doi:10.4003/006.030.0106
Playford, T. J., and Walker, K. F. (2008). Status of the endangered glenelg river mussel Hyridella glenelgensis (Unionoida: Hyriidae) in Australia. Aquat. Conserv. 18, 679–691. doi:10.1002/aqc.877
Ponder, W. F., and Bayer, M. (2004). A new species of Lortiella (Mollusca: Bivalvia: Unionoidea: Hyriidae) from northern Australia. Molluscan Res. 24, 89–102. doi:10.1071/mr04007
Ponder, W. F., Hallan, A., Shea, M. E., Clark, S. A., Richards, K., Klunzinger, M. W., et al. (2023). Australian freshwater molluscs. Version 3. Available at: https://keys.lucidcentral.org/keys/v3/freshwater_molluscs/ (Accessed August 15, 2023).
Rafinesque, C. S. (1820). Monographie des coquilles bivalves fluviatiles de la Riviere Ohio, contenant douze genres et soixante-huit especes. Ann. G. Sci. Phys. 5, 287–322. + plates 80-82.
Raikow, D. F., and Hamilton, S. K. (2001). Bivalve diets in a midwestern U.S. stream: a stable isotope enrichment study. Limnol. Oceanogr. 46, 514–522. doi:10.4319/lo.2001.46.3.0514
R Core Team (2022). R: a language and environment for statistical computing. Vienna, Austria: R Foundation for Statistical Computing. URL Available at: https://www.R-project.org/.
Ricci, C. N., Alvarenga, L. C. F., and Coelho, A. C. S. (1990). Gloquídios de Diplodon spix, 1827: D. (D.) multistriatus (lea, 1831) (Mollusca, Bivalvia, Hyriidae). B. Mus. N. Nova Ser. Zool. 344, 1–10.
Richardson, J. (1848). “Fishes,” in The zoology of the voyage of H. M. S. Samarang; under the command of captain sir edward belcher, during the years 1843-1846. Editor A. Adams (London: Reeve & Benham). 1-28, Pls. 1-10.
Rustomji, P., Bennett, N., and Chiew, F. (2009). Flood variability east of Australia’s Great dividing range. J. Hydrol. 374, 196–208. doi:10.1016/j.jhydrol.2009.06.017
Santos, R. C. L., Wakinaguni Michiura, A. W., and Callil, C. T. (2021). Host attraction behaviour: the red exhalent aperture extension of the Neotropical freshwater mussel Castalai ambigua. J. Mollus. Stud. 87 (4), eyab025. doi:10.1093/mollus/eyab025
Santos-Neto, G. C., Beasley, C. R., Schneider, H., Pimpão, D. M., Hoeh, W. R., Simone, L. R. L., et al. (2016). Genetic relationships among freshwater mussel species from fifteen Amazonian rivers and inferences on the evolution of the Hyriidae (Mollusca: Bivalvia: Unionida). Mol. Phylogenet. Evol. 100, 148–159. doi:10.1016/j.ympev.2016.04.013
Say, T. (1817). “Conchology,” in American edition of the British encyclopedia or dictionary of arts and sciences, comprising an accurate and popular view of the present improved state of human knowledge vol. II. Editor W. Nicholson (Philadelphia: Samuel A. Mitchell and Horace Ames W. Brown).
Say, T. (1829). Descriptions of new terrestrial and fluviatile shells of North America. New harm. Dissem. Useful Knowl. 2, 291–293.
Say, T. (1831). Descriptions of some new terrestrial and fluviatile shells of North America. New Harmony, Indiana.
Sayenko, E. M. (2006). Morphology of glochidia (Bivalvia: Unionidae: anodontinae, pseudanodontinae) of Russia. Vladivostok, Primorye Territory, Russia: Dalnauka.
Sayenko, E. M. (2016). The microsculpture of glochidia of some anodontine bivalves (Unionidae). Biol. Bull. 43, 127–135. doi:10.1134/S1062359016020072
Semenas, L., Ortuba, S., and Ubeda, C. (1994). Presencia de gloquidios de Diplodon chilensis Haas, 1931 (Mollusca, Pelecypoda) en peces dluceacuícolas patagónicos. Bol. Chil. Parasitol. 49, 85–86.
Sheldon, F., McCasker, N., Hobbs, M., Humphries, P., Jones, H., Klunzinger, M., et al. (2020). Habitat and flow requirements of freshwater mussels in the northern Murray-Darling Basin. Nathan, QLD, Australia: Australian Rivers Institute, Griffith University and Institute of Land, Water and Society, Charles Sturt University Report to the Commonwealth Environmental Water Holder. Available at: https://www.dcceew.gov.au/water/cewo/publications/habitat-flow-requirements-freshwater-mussels-northern-murray-darling-basin.
Shephard, A., McGregor, M. A., and Haag, W. R. (2021). Host fishes and life history of the Round Hickorynut (Oboivaria subrotunda). Fresh. Moll. Biol. Cons. 24, 18–25.
Simpson, C. T. (1914). A descriptive catalogue of the naiades, or pearly freshwater mussels parts I-III. Ann Arbor: The Ann Arbor Press.
Smith, E. A. (1885). On a collection of shells (chiefly land and fresh-water) from the Solomon Islands. Proc. Zool. Soc. Lond. 1885, 588–609. doi:10.1111/j.1469-7998.1885.tb07865.x
Soler, J., Wantzen, K. M., Jugé, P., and Araujo, R. (2018). Brooding and glochidia release in Margaritifera auricularia (spengler, 1793) (Unionoida: Margaritiferidae). J. Mollus. Stud. 84, 182–189. doi:10.1093/mollus/eyy008
Sowerby, G. B. (1867). “Monograph of the genus Unio,” in Conchologica iconica, vol. 16. Editors L. Reeve, and G. B. Sowerby (London: L. Reeve & Co.), 55–60. pls.
Sowerby, G. B. (1868). “Genus mycetopus,” in Conchologica iconica, vol. 16. Editors L. Reeve., and G. B. Sowerby (London: L. Reeve & Co.), 1–4. pls.
Story, M., and Congalton, R. G. (1986). Accuracy assessment: a user’s perspective. Photogramm. Eng. Rem. S. 52, 397–399.
Strayer, D. L. (2008). Freshwater mussel ecology: a mutlifactor approach to distribution and abundance. Berkley and Los Angeles: University of California Press.
Surber, T. (1912). Identification of the glochidia of freshwater mussels. Report and Special Papers of the U.S. Bureau of Fisheries. [Issued separately as U.S. Bureau of Fisheries Document 771] 1912 1–10+3.
Tapparone Canefri, C. (1883). Fauna malaoologica della Nuova Guinea e delle isole adiacenti. Ann. Mus. Stor. Nat. Genova 19, 6–313.
Treby, S. (2016). Building mussels: determining causes of relative abundance in two sympatric freshwater mussels (Bivalvia, Huriidae, Hyridella) in southeastern Australia. Melbourne (Vic): LaTrobe University. [BSc (Hons) thesis].
Vicentini, H. (2005). Unusual spurting behavior of the freshwater mussel Unio crassus. J. Mollus. Stud. 71, 409–410. doi:10.1093/mollus/eyi045
Vikhrev, I. V., Makhrov, A. A., Aramonova, V. S., Ermolenko, A. V., Gofarov, M. Y., Kabakov, M. B., et al. (2019). Fish hosts, glochidia features and life cycle of the endemic freshwater pearl mussel Margaritifera dahurica from the Amur Basin. Sci. Rep. 9 (13), 8300. doi:10.1038/s41598-019-44752-9
Viozzi, G. P., and Brugni, N. L. (2001). Relación parasitaria y nuevos registros de gloquidios de Diplodon chilensis (Unionacea: Hyriidae) en peces de la Patagonia Argentina. Neotropica 47, 3–12.
Wächtler, K., Dreher-Mansur, M. C., and Richtler, T. (2001). “Larval types and early postlarval biology in naiads (Unionoida),” in Ecology and evolution of the freshwater mussels Unionoida. Editors G. Bauer, and K. Wächtler (Berlin: Springer), 96.
Walker, K. F. (1981). Ecology of freshwater mussels in the River Murray. Canberra: Australian Water Resources Council. Australian Water Resources Council Technical Paper No. 63.
Walker, K. F. (2017). Reproductive phenology of river and lake populations of freshwater mussels (Unionida: Hyriidae) in the River Murray. Molluscan Res. 37, 31–44. doi:10.1080/13235818.2016.1206166
Walker, K. F., Jones, H. A., and Klunzinger, M. W. (2014). Bivalves in a bottleneck: taxonomy, phylogeography and conservation of freshwater mussels (Bivalvia: Unionoida) in Australasia. Hydrobiologia 735, 61–79. doi:10.1007/s10750-013-1522-9
Watters, G. T. (2008). The morphology of conglutinates and conglutinate like structures in North American freshwater mussels: a scanning electron microscopy survey. Novapex 9, 1–20.
Widarto, T. H. (1993). Aspects of the biology of Velesunio ambiguus philippi from a tropical freshwater environment, Ross River, townsville, Australia. Townsville, Qld: James Cook University of North Queensland. [M.Sc. thesis].
Zając, K., and Zając, T. A. (2021). Seasonal patterns in the developmental rate of glochidia in the endangered thick-shelled river mussel, Unio crassus Philipsson, 1788. Hydrobiologia 848, 3077–3091. doi:10.1007/s10750-020-04240-y
Zieritz, A., Gum, B., Kuehn, R., and Geist, J. (2012). Identifying freshwater mussels (Unionoida) and parasitic glochidia larvae from host fish gills: a molecular key to the North and Central European species. Ecol. Evol. 2, 740–750. doi:10.1002/ece3.220
Zieritz, A., Yasaeng, P., Razak, N. F. A., Hongtrakul, V., Kovitvadhi, U., and Kanchanaketu, T. (2018). Development and evaluation of hotshot protocols for cost- and time-effective extraction of PCR-ready DNA from single freshwater mussel larvae (Bivalvia: Unionida). J. Mollus. Stud. 84, 198–201. doi:10.1093/mollus/eyy011
Keywords: Unionoida, Hyriidae, conglutinate, glochidial release, reproduction, New Zealand, Australia, bivalve larvae
Citation: Klunzinger MW, Jones HA, Humphrey CL, Melchior M, Raadik TA, Treby S, Chandler L and Sheldon F (2023) Comparative diversity in glochidia of Australasian freshwater mussels. Front. Environ. Sci. 11:1305077. doi: 10.3389/fenvs.2023.1305077
Received: 30 September 2023; Accepted: 17 November 2023;
Published: 21 December 2023.
Edited by:
Benjamin Cook, FRC Environmental, AustraliaReviewed by:
Arthur Bogan, North Carolina Museum of Natural Sciences, United StatesIan Duggan, University of Waikato, New Zealand
Copyright © 2023 Klunzinger, Jones, Humphrey, Melchior, Raadik, Treby, Chandler and Sheldon. This is an open-access article distributed under the terms of the Creative Commons Attribution License (CC BY). The use, distribution or reproduction in other forums is permitted, provided the original author(s) and the copyright owner(s) are credited and that the original publication in this journal is cited, in accordance with accepted academic practice. No use, distribution or reproduction is permitted which does not comply with these terms.
*Correspondence: Michael W. Klunzinger, bS5rbHVuemluZ2VyQGdtYWlsLmNvbQ==